- 1Beijing Key Laboratory of Biodiversity and Organic Farming, College of Resources and Environmental Sciences, China Agricultural University, Beijing, China
- 2School of Ecology and Environment, Inner Mongolia University, Hohhot, China
- 3College of Plant Protection, China Agricultural University, Beijing, China
Bees provide key pollination services for a wide range of crops. Accumulating evidence shows the effect of semi-natural habitats at the landscape level and local management practices on bee diversity in fields. However, most of the evidence is derived from studies in North America and Europe. Whether this paradigm is applicable in China, which is characterized by smallholder-dominated agricultural landscapes, has rarely been studied. In this study, we aimed to investigate how bee diversity affected apple production, and how landscape and local variables affected bee diversity and species composition on the Northern China Plain. The results showed that bees significantly increased apple fruit set compared to bagged controls. Wild bee diversity was positively related to apple seed numbers. Higher seed numbers reduced the proportion of deformed apples and thus increased fruit quality. Wild bee abundance was positively correlated with flowering ground cover, and both the abundance and species richness of wild bees were positively affected by the percentage of semi-natural habitats. We conclude that apple quality can benefit from ecological intensification comprising the augmentation of wild bees by semi-natural habitats and flowering ground cover. Future pollination management should therefore reduce the intensification level of management at both the local and landscape scales.
Introduction
Pollinators provide essential pollination services to 75% of major global crops (Klein et al., 2007) and 87.5% of wild plants (Ollerton et al., 2011). Pollinators not only increase crop yield (Hoehn et al., 2008; Garibaldi et al., 2011; Woodcock et al., 2019) but also improve food quality and nutrient content (Eilers et al., 2011; Chaplin-Kramer et al., 2014; Smith et al., 2015). Among pollinators, bees (Hymenoptera: Apoidea) are important pollen vectors of flowers due to their universality, species diversity, and specific pollen-carrying body structures (Michener, 2007).
Unfortunately, dramatic declines are reported in both honeybee colonies and wild bee diversity, which seriously threaten the provision of pollination services (Biesmeijer et al., 2006; Potts et al., 2010). The reasons for the decline in wild bees are mainly attributed to local agricultural intensification and landscape simplification (Potts et al., 2010; Tscharntke et al., 2012). Wild bees need continuous floral and nest resources during their life history to grow and reproduce, but agricultural habitats cannot meet their full resource demands due to the short crop flowering period and frequent human disturbance (Mandelik et al., 2012). Wild bees have to forage for resources in other surrounding habitats. As a result, the composition and configuration of different habitat types may drive the dispersal and distribution of bees in the whole landscape, which further affects wild bee diversity and associated pollination services to crops (Williams and Kremen, 2007; Tscharntke et al., 2012).
Semi-natural habitats are often the population source for wild bees to disperse into surrounding fields due to the diverse available resources, and it is therefore encouraged that such habitats should be protected to improve bee diversity and associated crop pollination services (Öckinger and Smith, 2007; Klein et al., 2012). In contrast, the contribution of agricultural habitats to bee resources is uncertain. Previous studies have shown that a large percentage of agricultural habitats in landscapes may decrease pollinator resources and associated pollination services due to pollinator dilution (Holzschuh et al., 2016; Proesmans et al., 2019). However, other studies suggested that flowering crops may provide important floral resources for wild bees, and mass flowering crops therefore enhance wild bee densities at the landscape scale (Westphal et al., 2003; Holzschuh et al., 2012). Furthermore, the evidence for landscape structure affecting wild bee diversity is mainly derived from Europe and North America, which are characterized by large field sizes (Steward et al., 2014). Our understanding of the relationship between landscape variables and bee diversity in smallholder agricultural landscapes has remained very limited (Zou et al., 2017; Wu et al., 2021), but it is urgent to consider this scenario because approximately 2.5 billion people depend on smallholder agriculture for survival worldwide (IFAD and UNEP, 2013).
In addition to landscape structure, local management practices may also affect bee diversity, such as pesticide application (Park et al., 2015; Sgolastra et al., 2020) and flowering ground cover (Campbell et al., 2017; Wu et al., 2019). A growing number of studies have shown that local management practices can interact with landscape variables, and they may counteract the negative effects of each other (Rundlöf et al., 2008; Park et al., 2015; Wu et al., 2019). Landscape complexity can also constrain the effectiveness of local management practices (Batary et al., 2011; Concepcion et al., 2012). However, the consistency of local and landscape scales and their interactions across global agricultural landscapes still need further verification due to the limited number of studies (Kennedy et al., 2013). In particular, evidence from Asian smallholder farming landscapes is needed (Steward et al., 2014), as most results are drawn from studies in Europe and North America (Archer et al., 2014).
China is the most important producer of crops profiting from pollination, accounting for 30–50% of global pollination benefits (Lautenbach et al., 2012), and is generally characterized by small field sizes (Cui et al., 2018). China has also faced agricultural intensification and landscape simplification in recent decades, inducing the loss of wild bee diversity and pollination services (Meng et al., 2012). Few studies have been conducted to investigate pollinator diversity and pollination services in China and how local and landscape variables affect the diversity and species composition of pollinators (Garibaldi et al., 2013; Zou et al., 2017; Wu et al., 2019).
To narrow the knowledge gap, we focus on the pollination services of apple (Malus domestica), a self-incompatible fruit crop. Apple is cultivated worldwide, half of which is produced in China (United States Department of Agriculture [USDA], 2019). However, it has been reported that apple is experiencing global pollination deficits due to insufficient pollinators (Garratt et al., 2014; Pardo and Borges, 2020; Wu et al., 2021). Previous research (Pardo and Borges, 2020) and our preliminary investigations have shown that bees are the main pollinators of apple. In this study, we attempted to answer the following questions: (1) What is the dependence of Fuji apple production on pollination services provided by bees? (2) How does bee diversity affect apple quality and quantity in natural pollination? (3) How do wild bee diversity and species composition respond to local and landscape variables? We hypothesized that (1) bees provide important pollination services for apples, (2) apple quality and quantity are positively affected by bee diversity, and (3) improving habitat quality at local and landscape scales increases wild bee assemblages and associated pollination services in apple orchards.
Materials and Methods
Study Sites
The study was conducted in Changping District (40°2′–40°23′ N, 115°50′–116°29′ E), one of the major apple-producing areas in China, in the northwest suburbs of Beijing (Figure 1). The altitude ranges from 30 to 1,400 m. The local climate is continental, with an average annual temperature of ∼12°C and an average annual rainfall of ∼550 mm. The mountainous area dominated by shrublands and orchards accounts for 59% of the total area of the district. The plain area, lying southeast of the district, is dominated by orchards, urban areas, and woodlands.
“Fuji” is the main cultivated apple variety in this study area. Twelve apple “Fuji” orchards were selected, surrounded by different landscape contexts that varied with structural complexity within a 1-km radius. The orchards were at least 2 km apart from each other to reduce possible autocorrelation between landscape structure and bee samples.
Pollination Treatments
In each orchard, three rows of apple trees were selected, with intervals of at least one row and at least 5 m away from the edge. Within each row, five trees with intervals of one tree were selected. On each tree, two branches and, on each branch, two inflorescences were randomly chosen to count the number of flower buds before bloom. One inflorescence on each branch was covered with nylon mesh (1 mm width) bags as a pollinator exclusion treatment, and the other inflorescence was freely exposed to pollinators as a natural pollination treatment. In total, 30 inflorescences were covered, and the other 30 inflorescences were uncovered at each site. After the flowers faded, bags were removed, and all inflorescences were marked with colored tabs for later harvest.
Bee Sampling
Apple is self-incompatible, and previous research and our preliminary field investigation have shown that bees are its main pollinator (Pardo and Borges, 2020). Pollinating bees were sampled continuously during the whole apple flowering period using pan traps (April1 4–28, 2015). At each site, within each selected row, three colored pan traps (blue, yellow, and white following Westphal et al., 2008, with 21 cm diameter and 10 cm depth) were placed at a height of 1.5 m above the ground and 10 m apart from one another. Pan traps were filled with approximately 300 mL of detergent water solution (two drops of detergent per 5 L of water) and then emptied and refilled every 2 days. All bee specimens were collected and preserved in 75% alcohol and then pinned for subsequent identification to the species level. Bee data from nine traps per orchard were pooled for the entire sampling period, and bee abundance refers to total bee individuals collected by pan traps per orchard.
Local Management Survey
To protect bees, no pesticides or fungicides were applied by farmers during the apple flowering period, and flowering ground cover was the main local factor affecting bee diversity within orchards. We placed five 1 m × 1 m quadrats at the four corners and the center of the bee sampling area during the apple bloom. Species richness and coverage of ground flowering plants in five quadrats were recorded and averaged to achieve mean values at each site.
Landscape Parameters
The land cover types in selected landscapes were classified into shrubland, woodland, wasteland, orchard, urban area, water area, and other. Shrublands are mainly distributed in mountains and are characterized by large areas of natural shrub species, such as Vitex negundo var. cannabifolia and Cotinus coggygria. The main habitat types in the plain area are woodland, orchards, wasteland, and urban areas. The dominant tree species of woodland are Pinus tabuliformis, Ginkgo biloba, Populus × tomentosa, Platycladus orientalis, and Robinia pseudoacacia. Wasteland was defined as areas where plants naturally generated with limited human disturbances. We defined the combination of shrubland, woodland, and wasteland as a semi-natural habitat. Land use maps within a radius of 1 km surrounding the study sites were digitized according to the extensive field inspections (resolution of 2 m) based on Online Bing Maps of the landscapes.1 We chose this spatial scale because the foraging distance of most wild bees was less than 1 km (Gathmann and Tscharntke, 2002; Zurbuchen et al., 2010). The landscape metrics and area percent of each habitat within a 1-km radius of the study sites were calculated at the class level using FRAGSTATS 4.2 (McGarigal et al., 2002). The area percentage of the semi-natural habitats was further calculated by summing the area percentage of the corresponding habitats.
Pollination Services
Fruit set, seed number, and deformation were measured to reflect the degree of pollination services. Framers usually thin the fruit clusters if there are multiple apples on one inflorescence. One week after flowers faded and before hand thinning in early May, we recorded the number of set apples on the marked inflorescences, although we were missing the data in two orchards where hand thinning was conducted by farmers before our visit. Approximately 6 1/2 months after the flowers faded, apples were ripe. In the middle of October, we picked all ripe apples from the marked inflorescences prior to commercial harvest and then labeled them by the treatment, tree, row, and orchard. Because of the low self-fertilized fruit set, only nine apples grew ripe on the pollinator-excluded inflorescences. The seed number of each fruit was counted. Therefore, we have fruit set data from 10 orchards and other apple quality parameters from 12 orchards. The deformation of each apple was determined visually by the same investigator. After placing each apple horizontally, if the shape of the apple was severely skewed to one side, it was deformed; if the apple shape was normal, it was not deformed.
Statistical Analysis
First, to investigate the effects of pollination treatment on fruit set, the generalized linear mixed effects model (glmer, in lme4 package, Bates et al., 2015) was computed with binomial error distribution. The fixed effect was pollination treatment, and the random effect was inflorescences nested within trees within rows within orchards.
Second, we analyzed the effects of bee diversity on pollination service parameters in the open pollination treatment at the site level. Fruit set, seed number, and deformation were added into the full linear models as the response variables, respectively. Honeybee abundance and one of wild bee variables [wild bee abundance, wild bee species richness, or wild bee diversity (Shannon–Weaver index, the diversity function, R package “vegan,” Oksanen et al., 2019)] were added as explanatory variables (Table 1), considering the significant positive relationship between abundance and species richness of wild bees (Pearson’s r = 0.90, p < 0.001). The correlation among bee variables was tested according to correlation test (the cor.test function, R package “stats,” R Core Team, 2018).
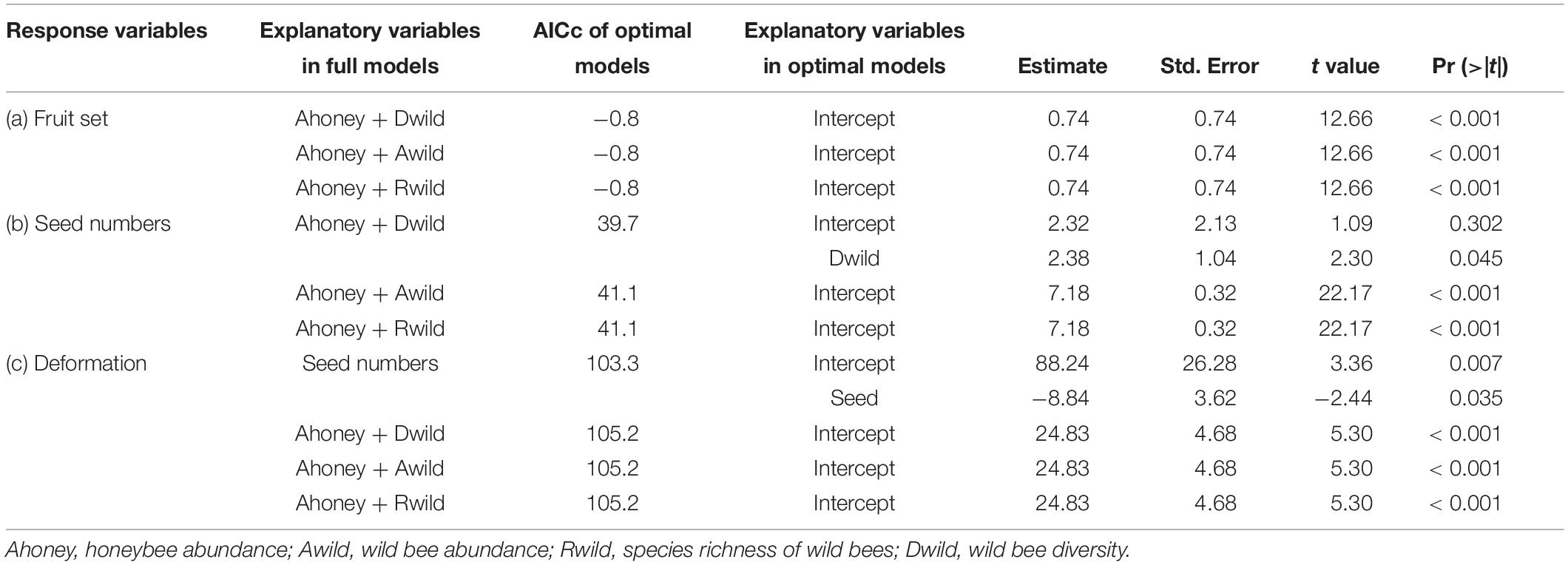
Table 1. Results from linear models (lm) indicating the effects of bees on apple fruit set (a), seed numbers (b), and deformation (c), and the effect of seed numbers on deformation (c).
Third, we used generalized linear models to assess the effects of the local management practice and landscape composition parameters on bees. In the case of overdispersion, generalized linear models with a negative binomial distribution (glm.nb, R package “MASS,” Ripley et al., 2018) were used. Flowering ground cover, percent of semi-natural habitats, percent of orchards, and the two-way interaction between the local management practice and landscape composition variables were included in full models as explanatory variables, and honeybee abundance as well as abundance, species richness, and diversity of wild bees were included as the response variables, respectively.
In the second and the third analyses, we used the dredge function in MuMIn package (Barton, 2018) to select the best-fitting models for each response variable based on AICc (Akaike’s information criterion). If ΔAICc < 2, the model containing more explanatory variables was chosen, and we used the rsq function (R package “rsq,” Zhang, 2020) to calculate adjusted R2 for generalized linear models. Spatial autocorrelation of the final model residuals was tested for each response variable using the “ncf” package (Bjornstad, 2019), and no significant spatial autocorrelation was detected in any case. The models were further validated based on visual inspection of the plotted residuals versus the predicted values (Zuur et al., 2009). All of the above analyses were performed using R (version 3.5.1, R Core Team, 2018).
Finally, redundancy analyses (RDA) was conducted to assess the effects of local and landscape variables on the species composition of wild bees in Canoco 5 (Šmilauer and Lepš, 2014). The percentage of semi-natural habitats and flowering ground cover were the environmental variables. Prior to analyses, species data were Hellinger-transformed to allow the use of the ordination method, due to the presence of many zero values (Legendre and Gallagher, 2001).
Results
Bee Diversity and Composition
In total, 1,616 bee individuals were collected. Wild bees accounted for 53.1% (858) of all individuals and represented 43 species (Supplementary Table A1). The most abundant wild bee species were Andrena minutula and Andrena hebes, accounting for 17.8 and 6.2% of the total bees, respectively. In addition, 758 honeybees (Apis mellifera) were collected.
Relationships Between Bee Diversity and Pollination Services
There were 3,082 flowers and 216 ripe apples measured, and only nine ripe apples were collected in the pollinator-excluded control. The fruit set in the open pollination treatment was significantly greater than that in the bagged control (z = 9.017, p < 0.0001, Figure 2). The fruit set in the open pollination treatment was not related to honeybee abundance or abundance, species richness, or diversity of wild bees (Table 1). The number of seeds in naturally pollinated apples increased with wild bee diversity (t = 2.30, R2 = 0.28, p = 0.045, Figure 3A) and was negatively correlated with the apple deformation (t = −2.44, R2 = 0.31, p = 0.035, Figure 3B).
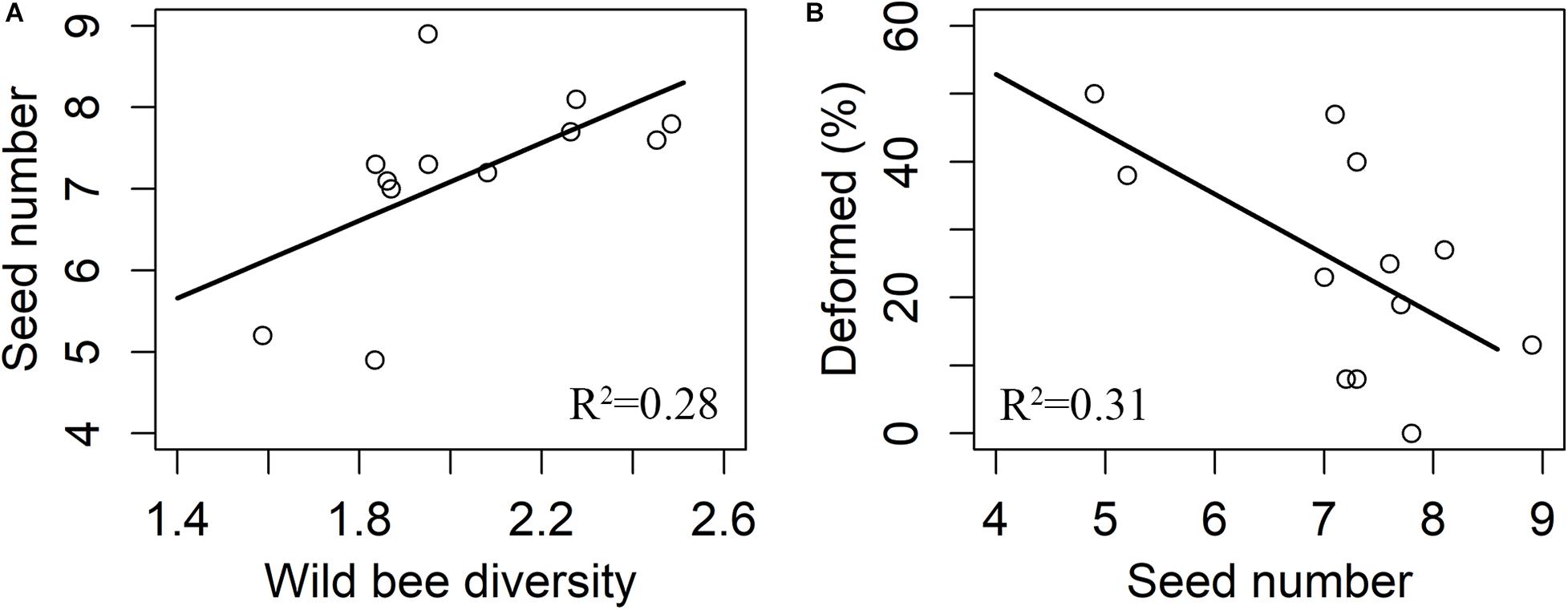
Figure 3. Responses of seed number to bees (A) and the effect of seed number on deformation (B). R2 means adjusted R-squared of the final model.
Effects of Local Management and Landscape Composition on Bees
Wild bee abundance increased with flowering ground cover within orchards (z = 2.63, R2 = 0.35, p = 0.009, Figure 4A). Both the abundance (z = 3.22, R2 = 0.35, p = 0.001, Figure 4B) and species richness (z = 2.23, R2 = 0.33, p = 0.026, Figure 4C) of wild bees were positively correlated with the percentage of semi-natural habitats. Wild bee diversity did not significantly respond to local or landscape predictors (Table 2).
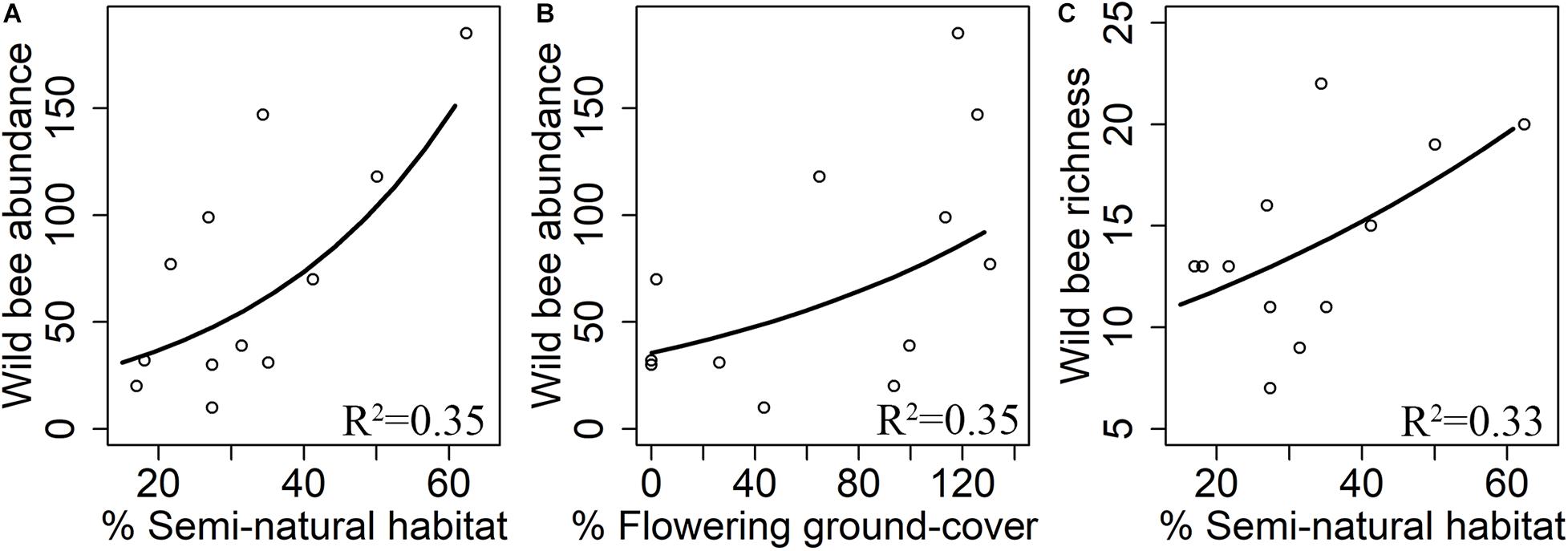
Figure 4. Effects of flowering ground cover and landscape composition on abundance (A,B) and species richness (C) of wild bees. R2 means adjusted R-squared of the final model.
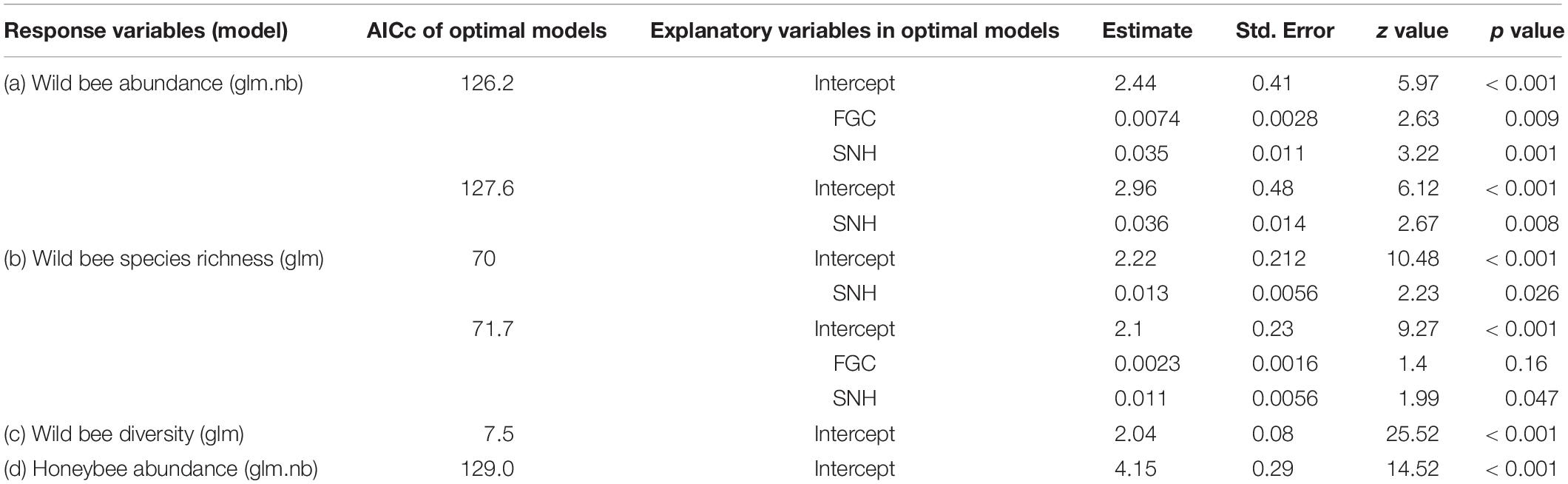
Table 2. Results from general linear models (or those with a negative binomial distribution) indicating the effects of landscape variables, flowering ground cover, and their interaction on the abundance (a), species richness (b), and diversity (c) of wild bees, as well as honeybee abundance (d). FGC = flowering ground cover, SNH = percentage of seminatural habitats.
The RDA results showed that the percentage of semi-natural habitats explained the greatest percentage of all variance (Explains = 25%, pseudo-F = 3.3, p = 0.004), and significantly affected wild bee species composition. The effect of flowering ground cover was marginal and explained 11.9% of the variation (pseudo-F = 1.7, p = 0.076). Most species were positively affected by the percentage of semi-natural habitats, except for Andrena solidago (AndSol) and Lasioglossum sp2 (LasSp2). The effect of flowering ground cover on Lasioglossum vulsum (LasVul) was negative, but it was positive on other wild bee species (Figure 5).
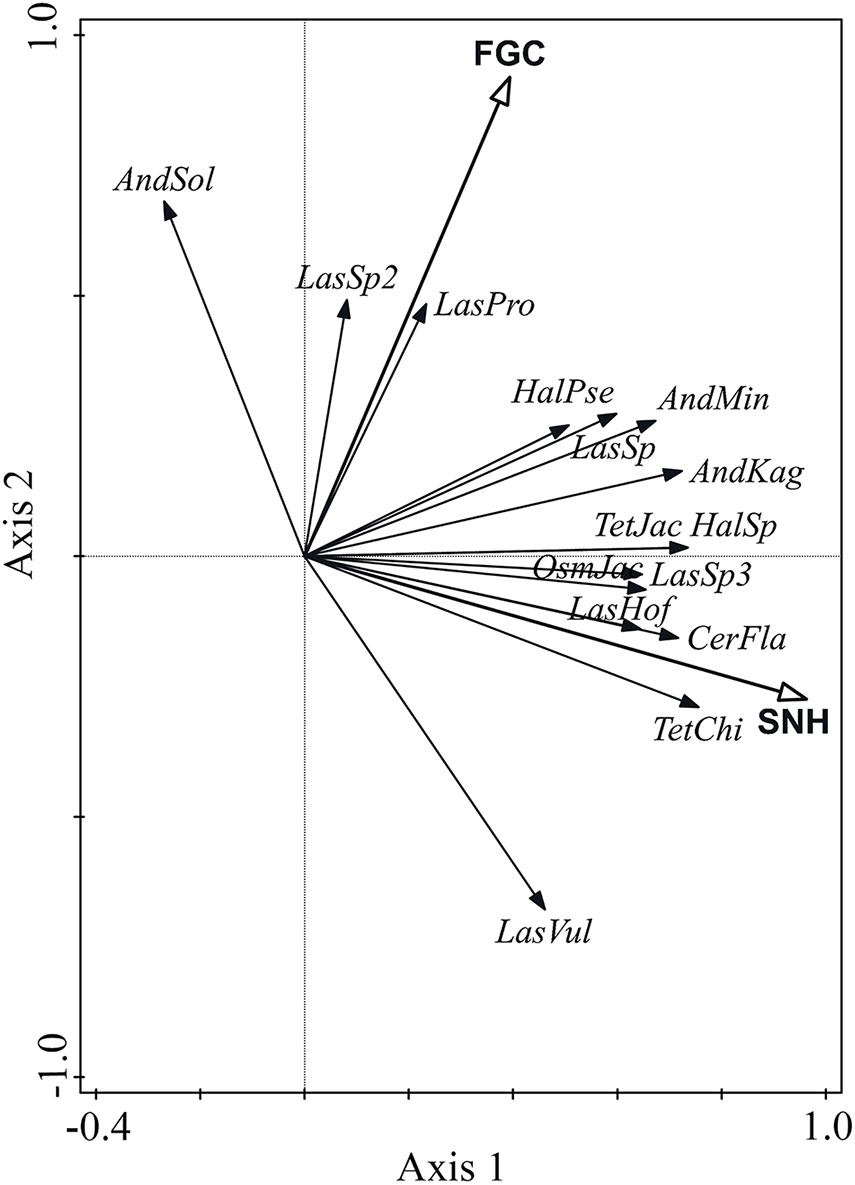
Figure 5. RDA biplot showing the effects of flowering ground cover (FGC) and percent of semi-natural habitats (SNH) on wild bee species composition. The first 15 species with the highest fit in the ordination plot are displayed. AndSol = Andrena solidago, LasSp2 = Lasioglossum sp2, LasPro = Lasioglossum proximatum, HalPse = Halictus pseudovestitus, AndMin = Andrena minutula, LasSp1 = Lasioglossum sp1, AndKag = Andrena kaguya, TetJac = Tetralonia jacoti, HalSp = Halictus sp, OsmJac = Osmia jacoti, LasSp3 = Lasioglossum sp3, LasHof = Lasioglossum hoffmanni, CerFla = Ceratina flavipes, TetChi = Tetralonia chinensis, LasVul = Lasioglossum vulsum.
Discussion
In this study, we found that bees significantly improved apple fruit set and provided critical pollination services for apple production. Seed number increased with wild bee diversity. A higher seed number reduced the proportion of deformed apples and thus increased fruit quality. Flowering ground cover positively affected wild bee abundance. Both the abundance and species richness of wild bees benefited from semi-natural habitats. Implementing measures of ecological intensification at both local and landscape scales therefore improves wild bee diversity and associated pollination services in Chinese agricultural landscapes.
Apple is a self-incompatible fruit crop and thus needs pollinators to transport pollen among different varieties (Ramírez and Davenport, 2013; Pardo and Borges, 2020). Our results showed that pollinators significantly increased apple fruit set compared with the exclusion control. Previous studies have shown that honeybees and wild bees are the most important pollinator groups of apples (Pardo and Borges, 2020). Our results suggested that wild bee diversity significantly increased apple seed numbers, which further decreased the proportion of deformed apples, but without a relationship between honeybees and apple pollination predictors. Similar to the findings in American and Hungarian apple orchards (Mallinger and Gratton, 2015; Földesi et al., 2016), apple pollination was more strongly related to wild bee diversity than honeybees. Because of their greater complementarity with ecological traits and resource use (Hoehn et al., 2008; Fruend et al., 2013), wild bee assemblages could promote the spatial and temporal stability of pollination services (Garibaldi et al., 2011) and buffer environmental disturbances (Williams et al., 2010) as well as climate change (Bartomeus et al., 2013). In contrast, honeybees sometimes rob nectar from an apple flower without pollinating it (Delaplane and Mayer, 2000) and may be low-efficacy pollinators for apples (Ramírez and Davenport, 2013). However, in intensive agricultural landscapes, the number of honeybees may far exceed that of wild bees due to the lack of semi-natural habitats (Rollin et al., 2013). In this case, farmers have to rely on honeybees, and honeybees may provide important pollination services for crops (Breeze et al., 2011), although their pollination efficiency may be low (Garibaldi et al., 2013; Pérez-Méndez et al., 2020), and their population is at risk of decline (Biesmeijer et al., 2006; Potts et al., 2010). Therefore, wild bees may provide a more reliable contribution to pollination services in changing environments than honeybees, which cannot be replaced but only supplemented by honeybees (Garibaldi et al., 2013).
However, there was no significant relationship between bees and fruit set, possibly due to the limitations of pan trap sampling. Although pan traps are effective, make it easier to sample several sites simultaneously, and lack collector bias (Westphal et al., 2008), they represent a passive sampling method, are not restricted to apple flower visitors, and have difficulty catching certain taxa (e.g., large bumblebees) (Popic et al., 2013). On the other hand, instead of wild bee species richness or diversity index, functional diversity of pollinators (Gagic et al., 2015) or trait matching of pollinators and crops (Garibaldi et al., 2015) might provide better prediction of the relationship between pollinators and pollination services; these approaches require more efforts and biological knowledge about the functional traits of all species, which are currently unavailable in our research region.
Wild bees benefited from flowering ground cover in our study, possibly due to additional pollen and nectar provided by the floral vegetation for wild bees during apple blooming. The apple flowering period is short and pulsed, but the demand of wild bees for foraging resources is long term to meet their needs for growth and reproduction. Similar positive effects of flowering ground cover on wild bees were also detected in apple orchards in United Kingdom (Campbell et al., 2017), China (Wu et al., 2019), and United States (Kammerer et al., 2016), and other fruit crops [such as mango in South Africa (Carvalheiro et al., 2012), sweet cherry in Germany (Holzschuh et al., 2012), and blueberry in the United States (Blaauw and Isaacs, 2014)], using sown flower strips or wild flowering vegetation within orchards. However, ground cover often promotes the abundance of wild bees, not species richness or diversity (Campbell et al., 2017; Wu et al., 2019). In orchards, flowering ground cover diversity is relatively low due to frequent disturbance and is even limited by agricultural practices, such as plowing, mowing, and application of pesticides and herbicides, resulting in an average of only 3.1 species per site in our study. Low diversity of floral vegetation may decrease their attraction to diverse wild bees, which may result in wild bee diversity or species richness not responding to flowering ground cover. Therefore, keeping or growing diverse flowering plant species within orchards may be more conducive to attracting richer wild bee species into orchards to provide pollination services for crops (Burkle et al., 2020).
Furthermore, the available floral resources provided by flowering ground cover may buffer the effect of the percentage of orchards on bees, resulting in no significant relationship between the percentage of orchards and bees in this study. Our results showed that semi-natural habitats promoted both the abundance and species richness of wild bees, and they therefore may contribute more to protecting wild bee resources than flowering ground cover in orchards. Different plant species possess various flowering phenologies, and rich flowering plant resources in semi-natural habitats, which not only meet the needs of wild bees for different nutrition statuses but also increase the possibility of acquiring resources during different periods of their life history (Ogilvie and Forrest, 2017). Moreover, the low level of human disturbance provides high-quality nesting sites for wild bees. Therefore, the restoration of diverse and high-quality semi-natural habitats is vital to improve wild bee diversity and associated pollination services in agricultural landscapes (Öckinger and Smith, 2007; Klein et al., 2012).
Previous studies have shown that local management may interact with landscape variables (Kennedy et al., 2013). For example, natural habitat modulated the effect of pesticide use in American apple orchards (Park et al., 2015), and flowering ground cover buffered the negative effects of plantation forests with intensive management and distance from natural shrubland in Chinese apple orchards (Wu et al., 2019). In our study, we did not find an effect of interactions between local and landscape scales on bees, but contrasting responses of different wild bee species to these environmental variables were detected based on the RDA analysis. For example, A. solidago benefited from flowering ground cover and negatively responded to the percentage of semi-natural habitats. Contrary to A. solidago, L. vulsum showed opposite responses to these two spatial scale factors. Bee species responded differently to local and landscape variables, possibly due to their specific resource requirements (Aguirre-Gutiérrez et al., 2016), but available resources were relatively scarce at the local and landscape scales in intensive agricultural landscapes. For example, although the average flowering ground cover is approximately 68%, the species richness of flowering vegetation is only 3.1 in our study. Low richness of flowering ground cover may increase the possibility that some wild bees dislike this pollen and nectar due to their unique requirements for foraging resources (Potts et al., 2003; Cusser and Goodell, 2013). Furthermore, various resource requirements among bee species result in local and landscape factors that may change bee functional diversity by mediating their species composition, such as foraging behavior, dietary specialization, and pollination efficiency, and further affect associated pollination services (Tscharntke et al., 2008; Forrest et al., 2015; Martins et al., 2015). We should therefore restore various high-quality semi-natural habitats at the landscape scale and improve the flowering vegetation diversity within orchards, to maximize the benefit to pollinators with diverse functional traits, other beneficial insects, and associated multifunctional ecosystem services (Tscharntke et al., 2008; Westphal et al., 2015; Albrecht et al., 2020).
Conclusion
Our findings demonstrate that bees provide important pollination services for apples in Chinese smallholder farming agricultural landscapes. Wild bees improve apple quality by increasing seed number. Wild bees could benefit from flowering vegetation resources in orchards and the surrounding complex landscapes. Future pollination management should therefore avoid intensification management at both the local and landscape scales and implement ecological intensification measures to improve wild pollinator diversity and maximize pollination services in agricultural landscapes.
Data Availability Statement
The raw data supporting the conclusions of this article will be made available by the authors, without undue reservation.
Author Contributions
YL acquired the funding and conceived the experiment. PD and PW collected the data. HX and PD identified the bees. XL and PD identified plants. PW and PD analyzed data and wrote the original draft of the manuscript under the advice of YL, MW, SF, and AO. All authors reviewed and revised the manuscript.
Funding
This study was supported by the National Key R&D Program of China (No. 2018YFC0507204), the National Natural Science Foundation of China (No. 41871186), the Natural Science Foundation of Beijing Municipality (No. 5162017), and the Chinese Universities Scientific Fund (No. 2019TC131).
Conflict of Interest
The authors declare that the research was conducted in the absence of any commercial or financial relationships that could be construed as a potential conflict of interest.
Acknowledgments
We are grateful to the farmers for allowing access to their apple orchards and supporting our experiment.
Supplementary Material
The Supplementary Material for this article can be found online at: https://www.frontiersin.org/articles/10.3389/fevo.2021.621469/full#supplementary-material
Footnotes
References
Aguirre-Gutiérrez, J., Kisslingc, W. D., Carvalheiro, L. G., WallisDeVries, M. F., Franzén, M., and Biesmeijer, J. C. (2016). Functional traits help .to explain half-century long shifts in pollinator distributions. Sci. Rep. 6:24451. doi: 10.1038/srep24451
Albrecht, M., Kleijn, D., Williams, N. M., Tschumi, M., Blaauw, B. R., Bommarco, R., et al. (2020). The effectiveness of flower strips and hedgerows on pest control, pollination services and crop yield: a quantitative synthesis. Ecol. Lett. 23, 1488–1498. doi: 10.1111/ele.13576
Archer, C. R., Pirk, C., Carvalheiro, L. G., and Nicolson, S. W. (2014). Economic and ecological implications of geographic bias in pollinator ecology in the light of pollinator declines. Oikos 123, 401–407. doi: 10.1111/j.1600-0706.2013.00949.x
Bartomeus, I., Park, M. G., Gibbs, J., Danforth, B. N., Lakso, A. N., and Winfree, R. (2013). Biodiversity ensures plant-pollinator phenological synchrony against climate change. Ecol. Lett. 16, 1331–1338. doi: 10.1111/ele.12170
Batary, P., Andras, B., Kleijn, D., and Tscharntke, T. (2011). Landscape-moderated biodiversity effects of agri-environmental management: a meta-analysis. Proc. R. Soc. B Biol. Sci. 278, 1894–1902. doi: 10.1098/rspb.2010.1923
Bates, D., Mächler, M., Bolker, B., and Walker, S. (2015). Fitting linear mixed-effects models using lme4. J. Stat. Softw. 67, 1–48. doi: 10.18637/jss.v067.i01
Biesmeijer, J. C., Roberts, S. P. M., Reemer, M., Ohlemueller, R., Edwards, M., Peeters, T., et al. (2006). Parallel declines in pollinators and insect-pollinated plants in Britain and the Netherlands. Science 313, 351–354. doi: 10.1126/science.1127863
Blaauw, B. R., and Isaacs, R. (2014). Flower plantings increase wild bee abundance and the pollination services provided to a pollination-dependent crop. J. Appl. Ecol. 51, 890–898. doi: 10.1111/1365-2664.12257
Breeze, T. D., Bailey, A. P., Balcombe, K. G., and Potts, S. G. (2011). Pollination services in the UK: how important are honeybees? Agric. Ecosyst. Environ. 142, 137–143. doi: 10.1016/j.agee.2011.03.020
Burkle, L. A., Delphia, C. M., and O’Neill, K. M. (2020). Redundancy in wildflower strip species helps support spatiotemporal variation in wild bee communities on diversified farms. Basic Appl. Ecol. 44, 1–13. doi: 10.1016/j.baae.2020.02.005
Campbell, A. J., Wilby, A., Sutton, P., and Wäckers, F. L. (2017). Do sown flower strips boost wild pollinator abundance and pollination services in a spring-flowering crop? a case study from UK cider apple orchards. Agric. Ecosyst. Environ. 239, 20–29. doi: 10.1016/j.agee.2017.01.005
Carvalheiro, L. G., Seymour, C. L., Nicolson, S. W., and Veldtman, R. (2012). Creating patches of native flowers facilitates crop pollination in large agricultural fields: mango as a case study. J. Appl. Ecol. 49, 1373–1383. doi: 10.1111/j.1365-2664.2012.02217.x
Chaplin-Kramer, R., Dombeck, E., Gerber, J., Knuth, K. A., Mueller, N. D., Mueller, M., et al. (2014). Global malnutrition overlaps with pollinator-dependent micronutrient production. Proc. R. Soc. B Biol. Sci. 281:1799. doi: 10.1098/rspb.2014.1799
Concepcion, E. D., Diaz, M., Kleijn, D., Baldi, A., Batary, P., Clough, Y., et al. (2012). Interactive effects of landscape context constrain the effectiveness of local agri-environmental management. J. Appl. Ecol. 49, 695–705. doi: 10.1111/j.1365-2664.2012.02131.x
Cui, Z., Zhang, H., Chen, X., Zhang, C., Ma, W., Huang, C., et al. (2018). Pursuing sustainable productivity with millions of smallholder farmers. Nature 555, 363–366. doi: 10.1038/nature25785
Cusser, S., and Goodell, K. (2013). Diversity and distribution of floral resources influence the restoration of plant–pollinator networks on a reclaimed strip mine. Restor. Ecol. 21, 713–721. doi: 10.1111/rec.12003
Delaplane, K. S., and Mayer, D. F. (2000). Crop Pollination by Bees. New York, NY: CABI Publishing. doi: 10.1051/apido/2009027
Eilers, E. J., Kremen, C., Greenleaf, S. S., Garber, A. K., and Klein, A. M. (2011). Contribution of pollinator-mediated crops to nutrients in the human food supply. PLoS One 6:e21363. doi: 10.1371/journal.pone.0021363
Földesi, R., Kovács-Hostyánszki, A., Körösi, Á, Somay, L., Elek, Z., Markó, V., et al. (2016). Relationships between wild bees, hoverflies and pollination success in apple orchards with different landscape contexts. Agric. For. Entomol. 18, 68–75. doi: 10.1111/afe.12135
Forrest, J. R., Thorp, R. W., Kremen, C., and Williams, N. M. (2015). Contrasting patterns in species and functional-trait diversity of bees in an agricultural landscape. J. Appl. Ecol. 52, 706–715. doi: 10.1111/1365-2664.12433
Fruend, J., Dormann, C. F., Holzschuh, A., and Tscharntke, T. (2013). Bee diversity effects on pollination depend on functional complementarity and niche shifts. Ecology 94, 2042–2054. doi: 10.1890/12-1620.1
Gagic, V., Bartomeus, I., Jonsson, T., Taylor, A., Winqvist, C., Fischer, C., et al. (2015). Functional identity and diversity of animals predict ecosystem functioning better than species-based indices. Proc. R. Soc. B Biol. Sci. 282:20142620. doi: 10.1098/rspb.2014.2620
Garibaldi, L. A., Bartomeus, I., Bommarco, R., Klein, A. M., Cunningham, S. A., Aizen, M. A., et al. (2015). Trait matching of flower visitors and crops predicts fruit set better than trait diversity. J. Appl. Ecol. 52, 1436–1444. doi: 10.1111/1365-2664.12530
Garibaldi, L. A., Steffan-Dewenter, I., Kremen, C., Morales, J. M., Bommarco, R., Cunningham, S. A., et al. (2011). Stability of pollination services decreases with isolation from natural areas despite honey bee visits. Ecol. Lett. 14, 1062–1072. doi: 10.1111/j.1461-0248.2011.01669.x
Garibaldi, L. A., Steffan-Dewenter, I., Winfree, R., Aizen, M. A., Bommarco, R., Cunningham, S. A., et al. (2013). Wild pollinators enhance fruit set of crops regardless of honey bee abundance. Science 339, 1608–1611. doi: 10.1126/science.1230200
Garratt, M. P. D., Breeze, T. D., Jenner, N., Polce, C., Biesmeijer, J. C., and Potts, S. G. (2014). Avoiding a bad apple: insect pollination enhances fruit quality and economic value. Agric. Ecosyst. Environ. 184, 34–40. doi: 10.1016/j.agee.2013.10.032
Gathmann, A., and Tscharntke, T. (2002). Foraging ranges of solitary bees. J. Anim. Ecol. 71, 757–764. doi: 10.1046/j.1365-2656.2002.00641.x
Hoehn, P., Tscharntke, T., Tylianakis, J. M., and Steffan-Dewenter, I. (2008). Functional group diversity of bee pollinators increases crop yield. Proc. R. Soc. B Biol. Sci. 275, 2283–2291. doi: 10.1098/rspb.2008.0405
Holzschuh, A., Dainese, M., González-Varo, J. P., Mudri-Stojnić, S., Riedinger, V., Rundlöf, M., et al. (2016). Mass-flowering crops dilute pollinator abundance in agricultural landscapes across Europe. Ecol. Lett. 19, 1228–1236. doi: 10.1111/ele.12657
Holzschuh, A., Dudenhöffer, J. H., and Tscharntke, T. (2012). Landscapes with wild bee habitats enhance pollination, fruit set and yield of sweet cherry. Biol. Conserv. 153, 101–107. doi: 10.1016/j.biocon.2012.04.032
IFAD and UNEP (2013). Smallholders, Food Security, and the Environment. Rome: International Fund For Agricultural Development.
Kammerer, M. A., Biddinger, D. J., Joshi, N. K., Rajotte, E. G., and Mortensen, D. A. (2016). Modeling local spatial patterns of wild bee diversity in Pennsylvania apple orchards. Landsc. Ecol. 31, 2459–2469. doi: 10.1007/s10980-016-0416-4
Kennedy, C. M., Lonsdorf, E., Neel, M. C., Williams, N. M., Ricketts, T. H., Winfree, R., et al. (2013). A global quantitative synthesis of local and landscape effects on wild bee pollinators in agroecosystems. Ecol. Lett. 16, 584–599. doi: 10.1111/ele.12082
Klein, A. M., Brittain, C., Hendrix, S. D., Thorp, R., Williams, N., and Kremen, C. (2012). Wild pollination services to California almond rely on semi-natural habitat. J. Appl. Ecol. 49, 723–732. doi: 10.1111/j.1365-2664.2012.02144.x
Klein, A. M., Vaissiere, B. E., Cane, J. H., Steffan-Dewenter, I., Cunningham, S. A., Kremen, C., et al. (2007). Importance of pollinators in changing landscapes for world crops. Proc. R. Soc. B Biol. Sci. 274, 303–313. doi: 10.1098/rspb.2006.3721
Lautenbach, S., Seppelt, R., Liebscher, J., and Dormann, C. F. (2012). Spatial and temporal trends of global pollination benefit. PLoS One 7:e35954. doi: 10.1371/journal.pone.0035954
Legendre, P., and Gallagher, E. (2001). Ecologically meaningful transformations for ordination of species data. Oecologia 129, 271–280. doi: 10.1007/s004420100716
Mallinger, R. E., and Gratton, C. (2015). Species richness of wild bees, but not the use of managed honeybees, increases fruit set of a pollinator-dependent crop. J. Appl. Ecol. 52, 323–330. doi: 10.1111/1365-2664.12377
Mandelik, Y., Winfree, R., Neeson, T., and Kremen, C. (2012). Complementary habitat use by wild bees in agro-natural landscapes. Ecol. Appl. 22, 1535–1546. doi: 10.1890/11-1299.1
Martins, K. T., Gonzalez, A., and Lechowicz, M. J. (2015). Pollination services are mediated by bee functional diversity and landscape context. Agric. Ecosyst. Environ. 200, 12–20. doi: 10.1016/j.agee.2014.10.018
McGarigal, K., Cushman, S. A., Neel, M. C., and Ene, E. (2002). FRAGSTATS v3: Spatial Pattern Analysis Program for Categorical Maps. Computer software program produced by the authors at the University of Massachusetts, Amherst.
Meng, L., Martin, K., Liu, J., Burger, F., and Chen, J. (2012). Contrasting responses of hoverflies and wild bees to habitat structure and land use change in a tropical landscape (southern Yunnan. SW China). Insect Sci. 19, 666–676. doi: 10.1111/j.1744-7917.2011.01481.x
Michener, C. D. (2007). The Bees of the World, 2nd Edn. Baltimore: The Johns Hopkins University Press.
Öckinger, E., and Smith, H. G. (2007). Semi-natural grasslands as population sources for pollinating insects in agricultural landscapes. J. Appl. Ecol. 44, 50–59. doi: 10.1111/j.1365-2664.2006.01250.x
Ogilvie, J. E., and Forrest, J. R. (2017). Interactions between bee foraging and floral resource phenology shape bee populations and communities. Curr. Opin. Insect Sci. 21, 75–82. doi: 10.1016/j.cois.2017.05.015
Oksanen, J., Blanchet, F. G., Kindt, R., Legendre, P., Minchin, P. R., O’hara, R. B., et al. (2019). Package ‘Vegan’. Community Ecology Package, Version 2.5-6.
Ollerton, J., Winfree, R., and Tarrant, S. (2011). How many flowering plants are pollinated by animals? Oikos 120, 321–326.
Pardo, A., and Borges, P. A. (2020). Worldwide importance of insect pollination in apple orchards: a review. Agric. Ecosyst. Environ. 293:106839. doi: 10.1016/j.agee.2020.106839
Park, M. G., Blitzer, E. J., Gibbs, J., Losey, J. E., and Danforth, B. N. (2015). Negative effects of pesticides on wild bee communities can be buffered by landscape context. Proc. R. Soc. B Biol. Sci. 282:20150299. doi: 10.1098/rspb.2015.0299
Pérez-Méndez, N., Andersson, G. K., Requier, F., Hipólito, J., Aizen, M. A., Morales, C. L., et al. (2020). The economic cost of losing native pollinator species for orchard production. J. Appl. Ecol. 57, 599–608. doi: 10.1111/1365-2664.13561
Popic, T. J., Davila, Y. C., and Wardle, G. M. (2013). Evaluation of common methods for sampling invertebrate pollinator assemblages: net sampling out-perform pan traps. PLoS One 8:e66665. doi: 10.1371/journal.pone.0066665
Potts, S. G., Biesmeijer, J. C., Kremen, C., Neumann, P., Schweiger, O., and Kunin, W. E. (2010). Global pollinator declines: trends, impacts and drivers. Trends Ecol. Evol. 25, 345–353. doi: 10.1016/j.tree.2010.01.007
Potts, S. G., Vulliamy, B., Dafni, A., Ne’eman, G., and Willmer, P. (2003). Linking bees and flowers: how do floral communities structure pollinator communities? Ecology 84, 2628–2642. doi: 10.1890/02-0136
Proesmans, W., Smagghe, G., Meeus, I., Bonte, D., and Verheyen, K. (2019). The effect of mass-flowering orchards and semi-natural habitat on bumblebee colony performance. Landsc. Ecol. 34, 1033–1044. doi: 10.1007/s10980-019-00836-5
R Core Team (2018). R: A Language and Environment for Statistical Computing. Vienna: R Foundation for Statistical Computing.
Ramírez, F., and Davenport, T. L. (2013). Apple pollination: a review. Sci. Hortic. 162, 188–203. doi: 10.1016/j.scienta.2013.08.007
Ripley, B., Venables, B., Bates, D. M., Hornik, K., Gebhardt, A., Firth, D., et al. (2018). Mass: Support Functions and Datasets for Venables and Ripley’s MASS. R Package Version 7.3-50.
Rollin, O., Bretagnolle, V., Decourtye, A., Aptel, J., Michel, N., Vaissière, B. E., et al. (2013). Differences of floral resource use between honey bees and wild bees in an intensive farming system. Agric. Ecosyst. Environ. 179, 78–86. doi: 10.1016/j.agee.2013.07.007
Rundlöf, M., Nilsson, H., and Smith, H. G. (2008). Interacting effects of farming practice and landscape context on bumblebees. Biol. Conserv. 141, 417–426. doi: 10.1016/j.biocon.2007.10.011
Sgolastra, F., Medrzycki, P., Bortolotti, L., Maini, S., Porrini, C., Simon-Delso, N., et al. (2020). Bees and pesticide regulation: lessons from the neonicotinoid experience. Biol. Conserv. 241:108356. doi: 10.1016/j.biocon.2019.108356
Šmilauer, P., and Lepš, J. (2014). Multivariate Analysis of Ecological Data using CANOCO 5. Cambridge: Cambridge university press.
Smith, M. R., Singh, G. M., Arian, D. M., and Myers, S. S. (2015). Effects of decreases of animal pollinators on human nutrition and global health: a modelling analysis. Lancet 386, 1964–1972. doi: 10.1016/S0140-6736(15)61085-6
Steward, P. R., Shackelford, G., Carvalheiro, L. G., Benton, T. G., Garibaldi, L. A., and Sait, S. M. (2014). Pollination and biological control research: are we neglecting two billion smallholders. Agric. Food Secur. 3, 1–13. doi: 10.1186/2048-7010-3-5
Tscharntke, T., Sekercioglu, C. H., Dietsch, T. V., Sodhi, N. S., Hoehn, P., and Tylianakis, J. M. (2008). Landscape constraints on functional diversity of birds and insects in tropical agroecosystems. Ecology 89, 944–951. doi: 10.1890/07-0455.1
Tscharntke, T., Tylianaskis, J. M., Rand, T. A., Didham, R. K., Fahrig, L., Batáry, P., et al. (2012). Landscape moderation of biodiversity patterns and processes–eight hypotheses. Biol. Rev. 87, 661–685. doi: 10.1111/j.1469-185X.2011.00216.x
United States Department of Agriculture [USDA] (2019). Fresh Apples, Graps, and Pears: World Markets and Trade. Washington, DC: United States Department of Agriculture.
Westphal, C., Bommarco, R., Carre, G., Lamborn, E., Morison, N., Petanidou, T., et al. (2008). Measuring bee diversity in different European habitats and biogeographical regions. Ecol. Monogr. 78, 653–671. doi: 10.1890/07-1292.1
Westphal, C., Steffan-Dewenter, I., and Tscharntke, T. (2003). Mass flowering crops enhance pollinator densities at a landscape scale. Ecol. Lett. 6, 961–965. doi: 10.1046/j.1461-0248.2003.00523.x
Westphal, C., Vidal, S., Horgan, F. G., Gurr, G. M., Escalada, M., Van Chien, H., et al. (2015). Promoting multiple ecosystem services with flower strips and participatory approaches in rice production landscapes. Basic Appl. Ecol. 16, 681–689. doi: 10.1016/j.baae.2015.10.004
Williams, N. M., Crone, E. E., Roulston, T. H., Minckley, R. L., Packer, L., and Potts, S. G. (2010). Ecological and life-history traits predict bee species responses to environmental disturbances. Biol. Conserv. 143, 2280–2291. doi: 10.1016/j.biocon.2010.03.024
Williams, N. M., and Kremen, C. (2007). Resource distributions among habitats determine solitary bee offspring production in a mosaic landscape. Ecol. Appl. 17, 910–921. doi: 10.1890/06-0269
Woodcock, B. A., Garratt, M. P. D., Powney, G. D., Shaw, R. F., Osborne, J. L., Soroka, J., et al. (2019). Meta-analysis reveals that pollinator functional diversity and abundance enhance crop pollination and yield. Nat. Commun. 10:1481. doi: 10.1038/s41467-019-09393-6
Wu, P. L., Axmacher, J. C., Li, X. D., Song, X., Yu, Z. R., Xu, H. L., et al. (2019). Contrasting effects of natural shrubland and plantation forests on bee assemblages at neighboring apple orchards in Beijing. China. Biol. Conserv. 237, 456–462. doi: 10.1016/j.biocon.2019.07.029
Wu, P. L., Tscharntke, T., Westphal, C., Wang, M. N., Olhnuud, A., Xu, H. L., et al. (2021). Bee abundance and soil nitrogen availability interactively modulate apple quality and quantity in intensive agricultural landscapes of China. Agric. Ecosyst. Environ. 305:107168.
Zou, Y., Bianchi, F. J. J. A., Jauker, F., Xiao, H. J., Chen, J. H., Cresswell, J., et al. (2017). Landscape effects on pollinator communities and pollination services in small-holder agroecosystems. Agric. Ecosyst. Environ. 246, 109–116. doi: 10.1016/j.agee.2017.05.035
Zurbuchen, A., Landert, L., Klaiber, J., Mueller, A., Hein, S., and Dorn, S. (2010). Maximum foraging ranges in solitary bees: only few individuals have the capability to cover long foraging distances. Biol. Conserv. 143, 669–676. doi: 10.1016/j.biocon.2009.12.003
Keywords: deformation, flowering ground cover, landscape composition, pollinators, seed number
Citation: Wu P, Dai P, Wang M, Feng S, Olhnuud A, Xu H, Li X and Liu Y (2021) Improving Habitat Quality at the Local and Landscape Scales Increases Wild Bee Assemblages and Associated Pollination Services in Apple Orchards in China. Front. Ecol. Evol. 9:621469. doi: 10.3389/fevo.2021.621469
Received: 26 October 2020; Accepted: 27 January 2021;
Published: 08 March 2021.
Edited by:
Yi Zou, Xi’an Jiaotong-Liverpool University, ChinaReviewed by:
Tuanjit Sritongchuay, Chinese Academy of Sciences, ChinaShudong Luo, Chinese Academy of Agricultural Sciences, China
Copyright © 2021 Wu, Dai, Wang, Feng, Olhnuud, Xu, Li and Liu. This is an open-access article distributed under the terms of the Creative Commons Attribution License (CC BY). The use, distribution or reproduction in other forums is permitted, provided the original author(s) and the copyright owner(s) are credited and that the original publication in this journal is cited, in accordance with accepted academic practice. No use, distribution or reproduction is permitted which does not comply with these terms.
*Correspondence: Yunhui Liu, bGl1eWhAY2F1LmVkdS5jbg==