- 1Centre for Ecology, Evolution and Environmental Changes (cE3c), Faculdade de Ciências, Universidade de Lisboa, Lisbon, Portugal
- 2Departamento de Biologia Vegetal e Departamento de Geologia, Faculdade de Ciências, Universidade de Lisboa, Lisbon, Portugal
Reviewing the ecological studies on the endangered endemic Plantago almogravensis Franco, an Al-hyperaccumulator plant, and combining these with morphological, physiological, biochemical, and molecular data, significant knowledge on the limiting factors that cause its narrow geographical distribution and rarity status is achieved, which can contribute to suited conservation guidelines. Emphasis was given on (i) the major factors limiting P. almogravensis’ ecological niche (biotic and abiotic); (ii) phases of the life cycle and population dynamics; and (iii) and the phylogenetically close taxa (Plantago subulata aggregate) in order to fill the knowledge gaps in the uniqueness of P. almogravensis ecology, its phylogeny, and conservation status. The identification of relevant ecological data and using plant functional (morphological and physiological) traits, as well as genetic attributes, substantiate into a powerful tool to guide protection and conservation measures, usable toward this and other endangered hyperaccumulator plant species. Knowledge of the limitations of this strongly narrowly distributed plant allows for better design of conservation measures and to guide value and investment strategies in order to secure the species’ current area (habitat conservation and reclamation), direct the expansion of the existing population (assisting in populational densification and colonization), and/or grant ex situ conservation (genetic resources conservation).
Introduction
Plantago almogravensis Franco (Plantaginaceae) is an extremely rare Portuguese endemic plant species only found naturally in a 3 ha area, in the southwest coast, scattered in several small subpopulations (generally ≤ 25 individuals) (Serrano, 2015). The plant is a dwarf woody hemicryptophyte with leaf rosettes forming a cushion less than 15 cm wide and 7 cm high that inhabits an unusual metalliferous environment (Serrano et al., 2015), in which it has the ability to thrive while hyperaccumulating Al (Branquinho et al., 2007; Serrano et al., 2011, 2017).
The genus Plantago L. is considered to be monophyletic, supported by morphological, embryological, and chemical data (Rahn, 1996) as well as molecular phylogenetic studies (Rønsted et al., 2002; Hoggard et al., 2003; Albach et al., 2005). According to these authors, the genus is divided into four or five subgenera including Coronopus (Lam. & DC.) Rahn, represented by two sections, one being sect. Maritima H. Dietr., mostly composed of Mediterranean taxa whose nomenclature has been of difficult resolution and agreement among taxonomists because of variations in morphology due to ecological conditions, as the frequent revisions indicate, e.g., in Flora Europaea (Moore et al., 1976), Flora de Portugal (Franco, 1984), in Flora Iberica (Pedrol, 2009), and taxonomy notes on the Plantago subulata group by Hassemer et al. (2017), Hassemer (2018), Iamonico et al. (2017), or Höpke et al. (2019). For Portugal (continental), those floras described two to seven taxa belonging to two groups: P. subulata group (Plantago algarbiensis Samp., P. almogravensis Franco, Plantago holosteum Forssk., P. subulata L., Plantago radicata Hoffmans & Link., and Plantago penyalarensis Pau.) and Plantago maritima group [Plantago alpina L., P. maritima subsp. maritima L., and P. maritima subsp. serpentina (All.) Arcang.]. In this article, we follow Franco’s (1984) nomenclature, for P. almogravensis, as there are still doubts about its classification at the species or infraspecific level in relation to P. algarbiensis (Pedrol, 2009; Carapeto et al., 2020).
These two taxa are present in the Mediterranean biogeographical region and occupy the southwest coast of the Iberian Peninsula. Populations related to P. algarbiensis and P. almogravensis sensu Franco (1984) are allopatric (>75 km), the former occupying a different inland habitat and under more divergent Mediterranean climate (Serrano et al., 2017). Both are coastal species (<30 km from the sea), especially P. almogravensis, which is strictly coastal and subject to maritime moist winds and sea mists (Serrano, 2015). They occupy dominantly sandy podzolic soils, with ferruginous hardpans often forming outcrops (Branquinho et al., 2007), but with different elemental composition (Serrano, 2015). Both taxa were considered highly endangered endemics at risk for conservation and given especial attention in the Portuguese legislation related to the Habitats Directive (RCM115-A/2008, 2008) due to their limited distribution (one or two known populations in 2000) and low number of individuals. Information about the conservation status of P. almogravensis and P. algarbiensis (Critically Endangered and Endangered, respectively) was accepted by the IUCN Red List (Bilz, 2011a,b); recently, the Portuguese Red List of Vascular Plants (Carapeto et al., 2020) updated the classification of both to Endangered using the IUCN criteria. This “improvement” was due to the creation of two populations (ex situ introductions) up to 15 km south (Pinto et al., 2013); thus, it was the increase in the Extent of Occurrence (IUCN criteria) that reduced the perceived risk of extinction. Despite being new populations, these have less than 10 individuals, while in the natural extant population they are estimated between 3,000 and 4,000 (<10,000), although declining (Pinto et al., 2013; Serrano, 2015; EEA/MS, 2019).
Rarity in this genus is common at a continental scale, most particularly on peripheral distribution areas such as oceanic islands and climatic transitional limits/boundaries (e.g., Dunbar-Co et al., 2009; Hassemer and Rønsted, 2016; Hassemer et al., 2018; Hassemer, 2019). Considering that the reunion of P. almogravensis and P. algarbiensis (after Pedrol, 2009) might have repercussions regarding the level of protection given to both taxa (being allopatric by more than 75 km, the Extent of Occurrence will increase, thus diminishing the perceived extinction risk), the addition of other diagnostic criteria regarding their population ecology and molecular phylogeny, besides revisiting the morphological comparisons already published, would give a stronger support to the conservation of both these taxa and of P. almogravensis in particular. From being described as an abundant plant in the beginning of the 20th century, in the southwest coast (Plantago acanthophylla Dsne.; Sampaio, 1909) through the 1970s and 1990s, when its populations were rediscovered, until nowadays with very limited numbers observed, many populations were lost, inclusive in the last 20 years, presumedly extinct (Franco, 1984; Pinto et al., 2013; Serrano, 2015). Land use change (intensification of agriculture) and even tourism have increased the pressure over P. almogravensis habitat, and life history characteristics related to dispersal capacity, ability to persist in unfavorable conditions, as well as population dynamics have narrowed their distribution area (Bilz, 2011b; Serrano, 2015; EEA/MS, 2019; Carapeto et al., 2020). It is still controversial to address conservation and management measures to rare/endangered species, leading to debates on how to set priorities and searching for new approaches and tools for diagnosis and decision making, particularly when dealing with narrowly distributed species with distinctive physiological adaptation strategies for survival. Often, the possible ecological functions that these rare species provide to their ecosystems are forgotten, and these would also be vulnerable to extinction (Lyons et al., 2005; Mouillot et al., 2013). Understanding the ecological factors driving species’ rarity is a step toward understanding the best means for maintaining biodiversity while contributing to its conservation. The factors commonly associated with rarity (Stebbins, 1980; Kruckeberg and Rabinowitz, 1985; Fiedler, 1986; Purvis et al., 2000; Klironomos, 2002; Ferreira and Boldrini, 2011; Gaston, 2011) include: (i) environmental change (e.g., habitat degradation, land use alteration, and climate change); (ii) negative biotic interactions (e.g., competition with invasive species, herbivory, and pathogenicity) or lack of positive symbiotic associations (e.g., nursing species, mycorrhizae, and pollinators); (iii) reproductive factors (e.g., infertility, imbalanced sex ratios, and lack of pollinators or seed dispersal agents); (iv) populational factors (e.g., sympatry and hybridization, fragmentation, local stochastic extinction events, and slow growth); (v) low genetic variability (e.g., due to endogamy, mutations, or genetic drift); and (vi) evolutionary age and history (e.g., new species still have not expanded and old species may have suffered biotype depletion being reduced to relict populations).
Thus, in this review, we will summarize the ecological limitations (related to the environment and to other organisms), population dynamics, and the morphological and molecular idiosyncrasies that are possibly related to its rare status and that make this taxon unique. These features of P. almogravensis should be used for tailored conservation measures, as the ones suggested, for this highly endangered species.
Plantago almogravensis and Its Niche
Higher abundances of P. almogravensis plants are found in stable vegetation gaps, devoid of the typical native shrub vegetation (Serrano et al., 2015). Soil analysis confirmed that the gaps were not random or occasional: these long-lasting gaps correspond to geochemical islands with a particular geological origin and composition, namely, rich in Al and Fe and poor in calcium (Ca) and magnesium (Mg) (Branquinho et al., 2007; Serrano et al., 2011, 2015). In its extant population, P. almogravensis seems to be an ecologically obligate metallophyte (Serrano et al., 2015), although physiologically facultative, which uses the hyperaccumulation strategy to cope with the high Al availability and toxicity (Serrano et al., 2011). According to the inclusive niche theory (Colwell and Fuentes, 1975; McGill, 2012), the trade-off of having a wider tolerance to a stressful environment was to lose competitive ability toward the other vegetation. Despite the higher abundance (ecological performance) in the geochemical islands that provide refuge from stronger competitors, in those areas, the plants are not at their best physiological performance, as indicated by the higher ∂13C values, higher leaf sclerophylly, smaller leaves, and more branching (Serrano et al., 2015). Thus, the realized niche of P. almogravensis is limited by both abiotic and biotic factors, having a smaller width than it would have if competitors were not present (Figure 1). Competition with shrub species (native and invasive) for areas with optimal conditions limits the expansion of the populations outside the geochemical islands. Inside these geochemical islands, there are mainly chemo-edaphic-climatic (abiotic) restrictions (Figure 1; Serrano et al., 2015) and biotic interactions with microorganisms (see Biotic Limitations), limiting its performance.
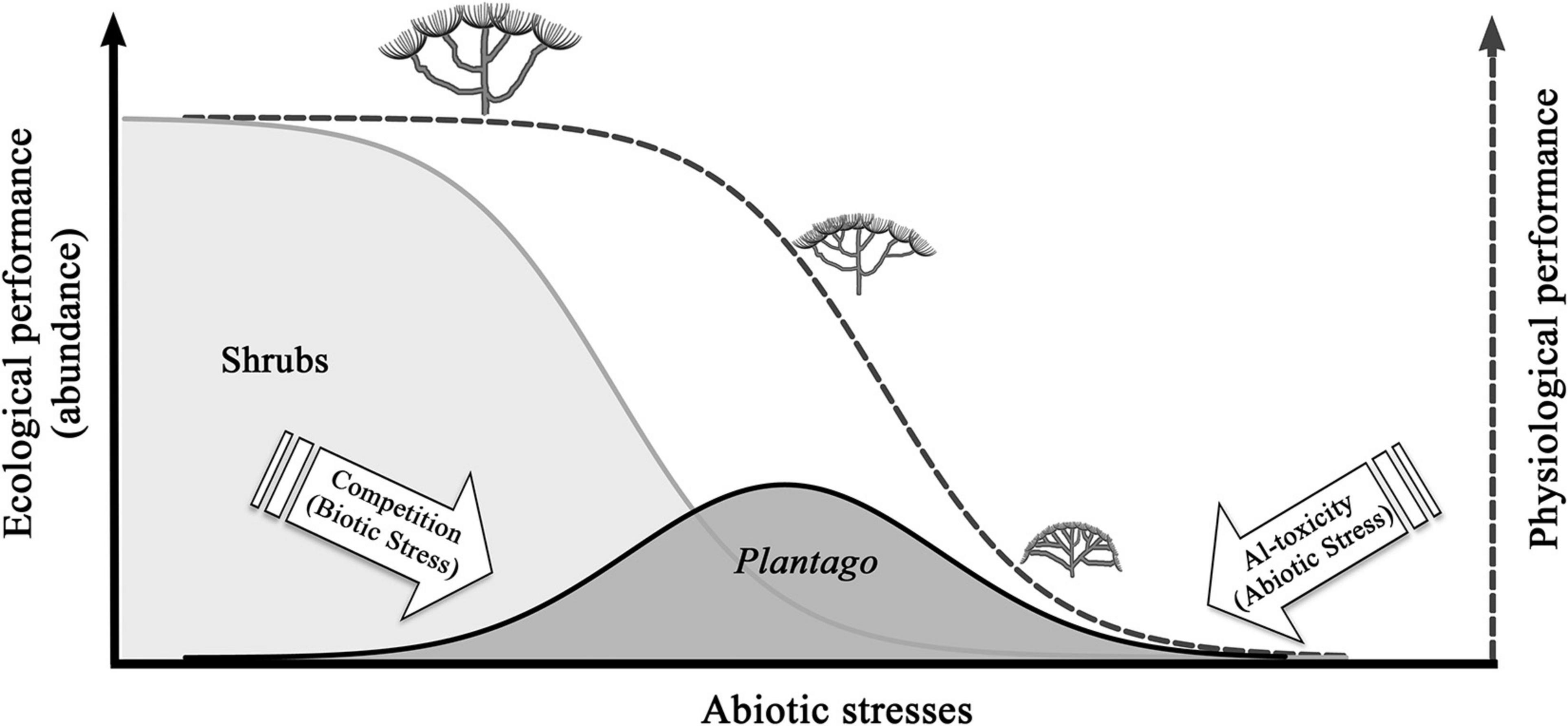
Figure 1. Hypothetical realized niche for Plantago almogravensis (Plantago). Filled line: ecological performance of P. almogravensis and of the shrub community along the abiotic stress gradient; dashed line: physiological performance of P. almogravensis, in the absence of competition, along the abiotic stress gradient. The plant schemes represent P. almogravensis morphotypes, from good fitness (left) to increased ramification and nanism in areas of more abiotic stress (right). Adapted from Serrano (2015), p. 152.
Abiotic Limitations
The Geochemical Island
Plantago almogravensis has its current realized niche in a podzol soil matrix, in geochemical islands (gaps) that are eroded areas where the ferruginous hardpan (B-horizon) is exposed at the soil surface. Those areas are enriched in Al and Fe, thus considered metalliferous areas (Branquinho et al., 2007; Serrano et al., 2011, 2015). The plant realized niche is limited by factors common in metalliferous areas: chemical (high bioavailability of metals, namely Al), nutritional (low content of macronutrients), hydric (thin soils that do not retain much water and wind exposure, facilitating drought), thermic (high soil metal content absorbs heat), and photochemical (excessive light input in areas scarce in vegetation, where the sunlight reflects off the ground) (Serrano et al., 2015). The combination of these factors is responsible for the lower physiological performance (closure of stomata and photosynthetic limitation) and growth adaptations (increased ramification with formation of the cushion-like habit, nanism, shortening of the leaves and increased sclerophylly) observed in the gaps (Figure 1; Serrano et al., 2015).
One of the most significant limiting factors identified was soil Al toxicity (ratio of Al to Ca or to macronutrients). The presence of plants in the gaps is generally negatively affected by Al toxicity, but P. almogravensis seems the most tolerant plant within the community; conversely, the various shrubs present were more sensitive. Thus, the geochemical island prevents shrub colonization (see shrub abundance in Figure 1) and became a refuge for P. almogravensis (Serrano et al., 2015) from those stronger competitors.
Nevertheless, some gap areas are not only too stressful for P. almogravensis survival but are also not suited for seed anchorage and plant establishment (e.g., hard soil or rock, lack of fissures and holes, slope and slippery soil surface, easily detachable soil particles, and runoff), thus limiting the colonization of the entire gap area (Serrano et al., 2015). Also, these open areas devoid of high vegetation are often used as pathways for animals (e.g., cows) and human tourists doing nature walks, both trampling the more exposed P. almogravensis individuals. Hence, the geochemical island niche acts as a refuge and, simultaneously, as a cause for aggravating the rarity status of P. almogravensis. Further isolation of the populations could have been driven by agricultural and forestry practices, still ongoing, destroying nearby suitable habitats, promoting fragmentation, and altering the native ecosystem (Pinto-Cruz, 2010). The range of abiotic soil conditions suitable for rare species is often narrower than that of common species (Kleijn et al., 2008); thus, the conservation of those conditions, and its gradient within ecosystems, is particularly important for preserving rare species (Wamelink et al., 2014).
The natural population is located within a Natural Park (PNSACV—Parque Natural do Sudoeste Alentejano e Costa Vicentina; see protected area details in the Sectorial Plan for Natura 2000 Network, Portugal, RCM115-A/2008, 2008) whose status ought to prevent further destruction of the extant population habitat. The gradual diminishing of both human and financial resources allocated to the PNSACV has led, however, to disregarding surveillance and adequate conservation measurements. Furthermore, the protection area is small, lacking a buffer zone to prevent indirect environmental changes, and the practical management actions do not favor the natural expansion of the population (see final recommendations).
Hyperaccumulation Strategy
Plantago almogravensis is an Al-hyperaccumulator plant (Branquinho et al., 2007; Serrano et al., 2011). The soil Al gradient corresponded to a saturation curve of Al accumulation in the plant, whose asymptote represents the physiological limit for the amount of metal accumulated by the species (approx. 3 mg Al g–1; Figure 2; Serrano et al., 2011). As that limit is reached, the plants’ physiological performance would decline since more resources are diverted to cope with the metal (Serrano et al., 2015). The ecological study of P. almogravensis suggests that leaf (and branch) turnover, together with branch growth, would help in diluting Al toxicity, as new leaves could replace those saturated by Al (Serrano et al., 2015). In other species, higher leaf and root turnovers have been observed as attenuation strategies when saturation of Al has been reached (Jansen et al., 2002; Brunner and Sperisen, 2013). For P. almogravensis, a decline in physiological performance was detected in the highest levels of soil Al toxicity (Serrano et al., 2015). In extreme cases of soil toxicity, the plants can no longer tolerate the metal and fade. In the case of natural populations, this is observed by the rarefication and absence of colonization (Figure 1). In the laboratory, higher plant and rosette mortality was observed after forced exposure to high Al concentrations (Serrano, 2015).
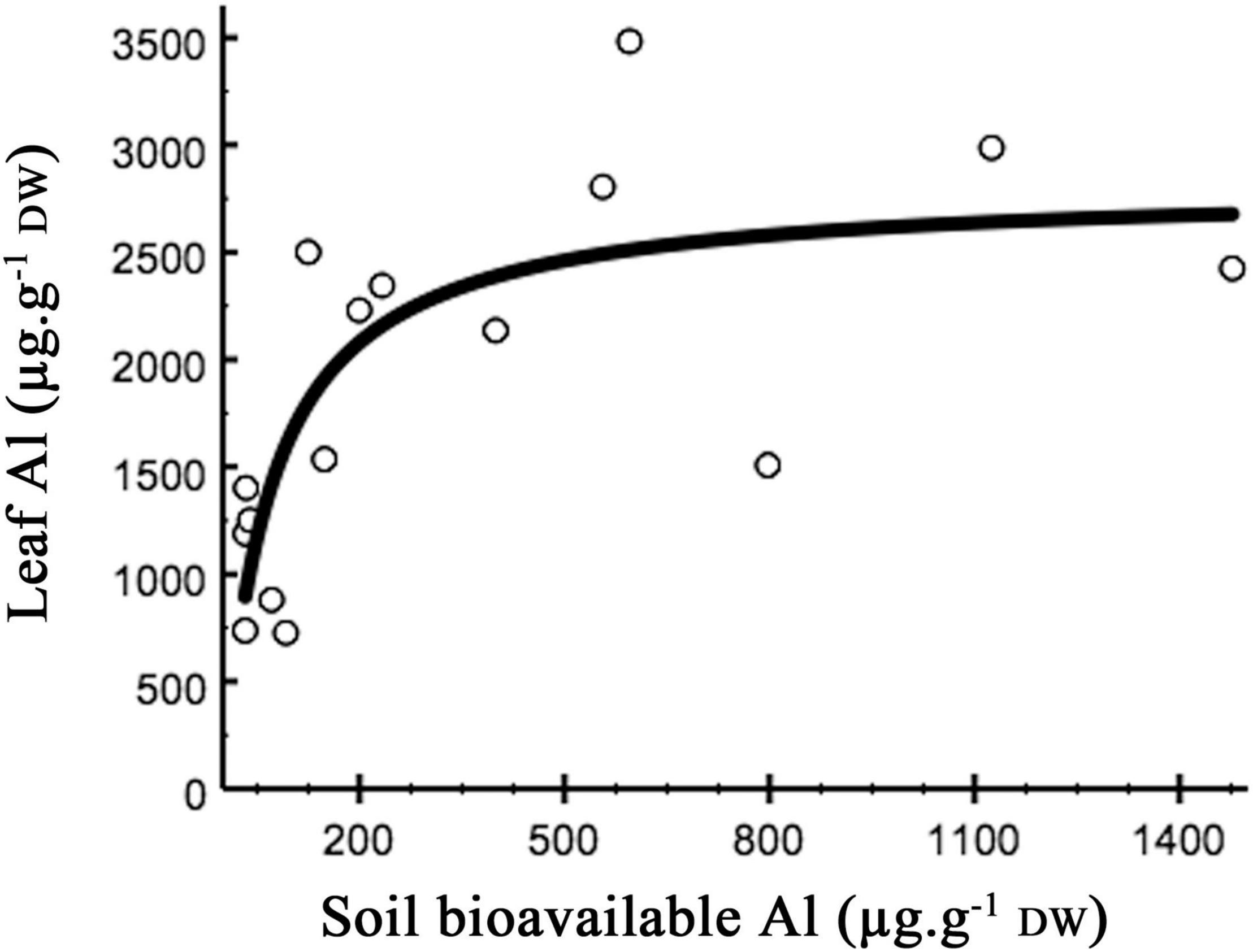
Figure 2. Accumulation of Al in Plantago almogravensis leaves related to soil-bioavailable Al. Fitted model: y = (2,804 × x)/(69.7 + x); R2 = 0.576 (observed vs. fitted values). Data from Serrano et al. (2011); adapted from Serrano (2015), p. 153.
The ability to maintain a stable population (with survival and the recruitment of new individuals) in otherwise toxic environments is characteristic of tolerant populations (Baker et al., 2010). Thus, P. almogravensis is able to develop two levels of tolerance: to soil Al toxicity (presence of populations in toxic environments) and to internal physiological toxicity (the hyperaccumulation trait). The Al-hyperaccumulation trait has been observed in various species of Plantago and also high- to hyperaccumulation of Fe and other metals (Serrano, 2015; Serrano et al., 2017). Considering (i) the wide proportion of metal accumulators within the Plantago phylogeny, (ii) the variety of metals accumulated, and (iii) the facultative nature of Plantago hyperaccumulation, dependent on soil availability, it seems likely that the accumulation traits could have been inherited from a common ancestor (phylogenetic origin) and would rest upon general stress tolerances (Antonovics et al., 1971; Baker and Proctor, 1990; Meharg, 1994, 2003; Boyd and Martens, 1998). The species of the Mediterranean subgenus Coronopus are common in Fe-based environments (e.g., pyrites, rubifacted red soils, Fe ores, exposed indurated cemented podzol B-horizon, and serpentine soils), where extreme drought and temperature stresses coexist with metal stress. The ability of Plantago to occupy these spatial niches rich in metals, while accumulating those metals, seems to have been taken to an extreme in P. almogravensis. Ultimately, the trade-off between toxic metal resistance and tolerance to competition (Serrano et al., 2015), favorable to the first, could have limited the expansion of the population and progressively reduced its distribution width, contributing to increase its rarity status.
Both Portugal and Spain are rich in metalliferous areas and old mines (e.g., along the Iberian Pyrite Belt). Eventually, the species could find in those areas an alternative suitable habitat after aided introductions of plants (ex situ conservation), which could help prevent a catastrophic extinction affecting the natural population of P. almogravensis.
Biotic Limitations
Competition With Shrubs
Thriving in the geochemical islands, P. almogravensis increased ramification with the formation of the cushion-like habit, shortened the leaves (nanism) and increased sclerophylly, while dealing with high Al toxicity levels (Serrano et al., 2015). All these processes have a cost, probably reflected in the loss of competitive ability toward shrubs (Figure 1).
Plantago almogravensis is able to survive with low nutrient resources, such as those found in the gap areas (Serrano et al., 2015). Microorganisms are known for having an important role in taking up nutrients (namely, phosphorus, manganese, or zinc) while modifying the uptake of toxic elements by plants (van der Heijden et al., 2008). The wide range of leaf ∂15N observed (from −3.17 to 1.43‰) suggests that microorganisms may be involved in the process of N acquisition facilitation for these plants and, thus, are involved in the plants’ ecological performance and survival. The microbial community’s abundance and diversity are reduced in gap areas, and the proportion between microbial functional groups is altered, in and out, in the gap areas (Serrano, 2015). In fact, one particular functional group of microorganisms (adapted to use organic acids) was found positively related to the distribution of P. almogravensis plants. This result was compatible with descriptions of organic acid exudation by P. almogravensis in response to AlCl3 (Martins et al., 2013a), which suggests that the same type of exudates might be produced under field conditions (although not yet checked). In fact, organic acid exudates, as well as the activity of soil microorganisms, have been suggested as explanations for the decrease observed in the exterior root Al saturation (5%) when compared to Al saturation in soil (51%) or inside the root (37%) (Serrano et al., 2011). Competition for water, namely, at germination and establishment, could also be important for this species. Germination occurs in the rainy winter season when water is available very near the surface in the gaps, but less accessible at lower depths in the shrubland (Serrano, 2015). Competition for light was apparently not important at the germination stage (Serrano, 2015). However, light does seem to have an important role afterward in the establishment of P. almogravensis, as it does for other Plantago species (Blom, 1992; Rahn, 1996). In the shaded shrubland, the absence of juveniles near the sporadic P. almogravensis adult plants further reinforces this idea. Therefore, competition for nutrients, water, and light seems to limit the expansion of the realized niche of P. almogravensis toward the wider fundamental niche of the species (Serrano et al., 2015).
Competition With Invasive Species
A major threat for the P. almogravensis population was the arrival of invasive species to the area (e.g., Acacia spp. and Carpobrotus edulis). Although the geochemical islands provided a refuge from competition with the native shrub species, they may not be enough to detain the colonization by invasive exotic species. In stands cleared of Acacia sp. (Figure 3), individuals of P. almogravensis had the opportunity to colonize without competition (Serrano, 2015). This shows the ability of these plants to increase abundance in areas cleared of competitors, although not capable of expansion to more distant areas. A similar colonization behavior was observed for populations of P. algarbiensis in the roadsides of Huelva region. There, the vegetation is methodically cut every year, for wildfire prevention, and P. algarbiensis is able to flourish in the cleared marginal strips along the road (Serrano, 2015). Similar examples are known for serpentine-endemic species capable of occupying areas cleared of competitors, but not to thrive in their presence (Anacker et al., 2011). Although it is very difficult to clear an area of invasive species, if that is not done methodically (Figure 3), it represents a major threat for P. almogravensis survival, increasing the chances of its prompt disappearance.
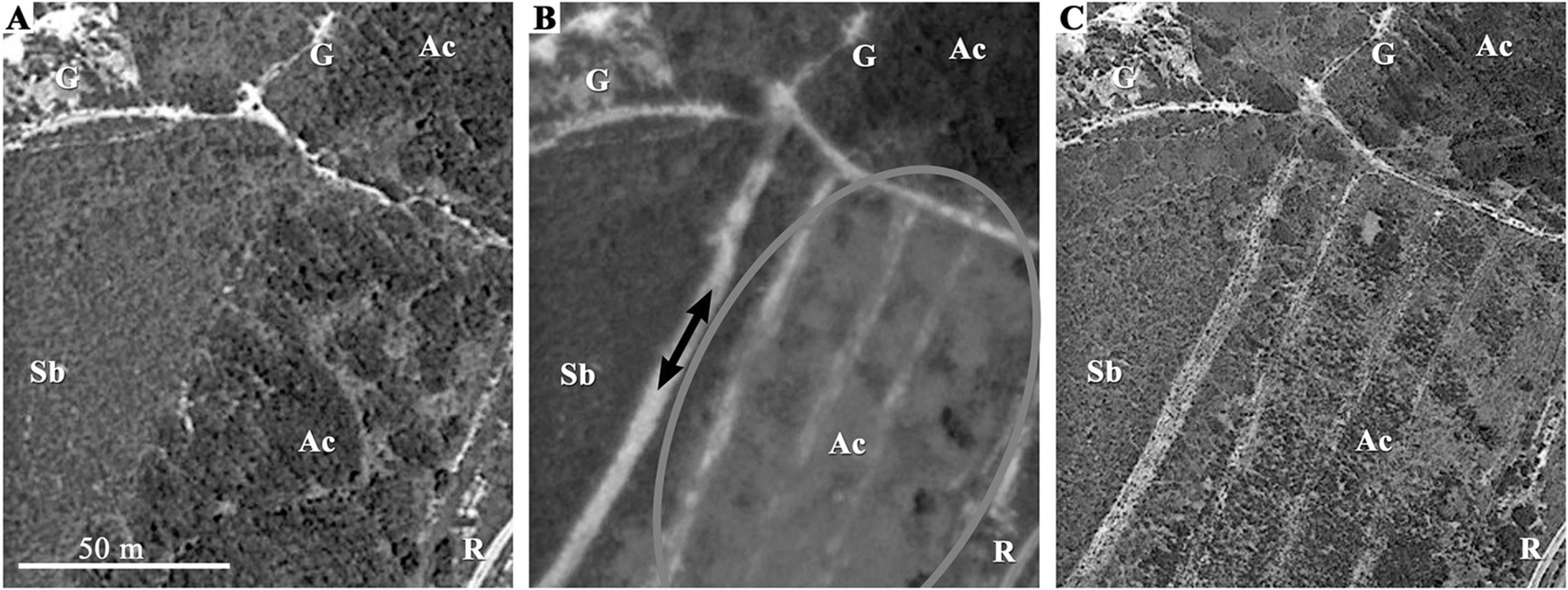
Figure 3. Aerial view of the habitat invaded by Acacia sp. with the chronological sequence of its partial removal. (A) May 2010: the invasive species (Ac) has occupied a large (>50 m) ribbon next to a dirt road (R) parallel to the coast. The native shrubland (Sb) as well as large gaps (G), where Plantago almogravensis ought to be found, are visible on the coast side (left). (B) April 2011: an area of approx. 50 m × 100 m of the Acacia sp. stand was cleared (oval) and P. almogravensis was later observed flowering on the cleared area (black arrows). (C) May 2013: the cleared area is still visible, but either Acacia sp. or other plants, rather than the native shrubland, are developing. Images:© DigitalGlobe/Google Earth 2015. Figure adapted from Serrano (2015), p. 155.
Limitations in the Life Cycle
Plantago almogravensis plants are long-lived (for decades), producing flowers (spikes) from May to June, and the seeds mature in July and can germinate from the autumn to the winter season. July to September represents a season of dormancy, where most leaves dry, to resprout after the autumn rain and grow until the beginning of the summer.
Considering seed production, significantly 86% of all potential seeds per flower spike (two per flower) were considered non-viable or absent, suggesting that pollination, or seed development, is impaired in the P. almogravensis population (Serrano, 2015). This may have not only environmental causes but also genetic causes, such as an inbreeding depression caused by the small population size (Ouborg et al., 2006). A low viable seed number was also recorded in the wider P. algarbiensis population (Serrano, 2015), suggesting the importance of environmental rather than only genetic causes. In fact, inter-simple sequence repeat (ISSR) and random amplified polymorphic DNA (RAPD) markers detected 61% of polymorphism in P. almogravensis (one population) and 68–74% in P. algarbiensis (three populations) and gene diversity coefficients (Nei’s h) of 0.1965 and 0.205–0.231, respectively, with the authors concluding that genetic diversity was not as low as expected for rare species (Ferreira et al., 2013; Coelho et al., 2017). For comparison, Plantago major L., a wide distribution range species, in a study using 17 populations, scored between 0.150 and 0.262 for gene diversity (Keivani et al., 2020).
The majority of the P. almogravensis individuals located in gaps have a cushion habit, with short inflorescences, often in small numbers (Figure 1; Serrano, 2015; Serrano et al., 2015). Lower peduncles and fewer flowers per spike reduce the conditions favorable for effective anemophilous pollination, limiting reproduction (Okubo and Levin, 1989; Martin et al., 2009). Outside the gaps, where the plants are under lower environmental stress, the dense sclerophyllous shrubland may act as a physical barrier to a broader dispersion of pollen, contributing to the low number of seeds produced. To overcome this limitation, assisted pollination could be a viable option. Seed dispersion to a suitable germination ground is very important for germination success. The same dispersion principles apply to pollen as to seed dispersal (Okubo and Levin, 1989): physical barriers and shorter seed-bearing structures maintain the seeds to a close distance from their origin, as long as water, wind, or animals do not disperse it further away. Thus, the larger size of the neighboring shrubs might limit seed dispersion. In the open gaps, the higher abundances and densities of P. almogravensis were associated with water runoff areas or gap margins (Serrano et al., 2015), suggesting that wind or rainwater lamina have a major role in dispersion by accumulating the seeds in small sediment places and marginal areas (Baskin and Baskin, 2014). Apparently, seed dispersion is limited within a short distance; hence, the first step of plant life, seed recruitment, is very limited due to environmental and physical barriers, affecting both seed dispersion and development.
In stressful situations, instead of investing in energy-expensive sexual structures, P. almogravensis has the ability to invest in vegetative reproduction (Serrano, 2015). This limits the genetic pool variation, and it is a rather slow method to expand the population. Energy diverted to resprouting is also a known cause for smaller seed production (Zeppel et al., 2014).
Germination, per se, does not seem a critical step for P. almogravensis reproductive success as the viable seeds can germinate over 60% in a wide range of test conditions (Figure 4), but in the field, that number was estimated to a much lower 24% (Serrano, 2015) due to environmental constraints. Germination was not impaired by Al, although it may have a delaying effect on its onset (Serrano, 2015). Calcium was also suggested as a possible delaying factor (Serrano, 2015) due to its water retention properties, mimicking flooded conditions to which the seed can react by delaying germination (as seen in other species with similar mucilaginous seed coating) (Zaady et al., 1997; Baskin and Baskin, 2014). A conservation program for P. almogravensis should consider these findings when preparing assisted germinations so that near-natural germinations could easily be favored to higher success (provided that relevant demographic sources are used).
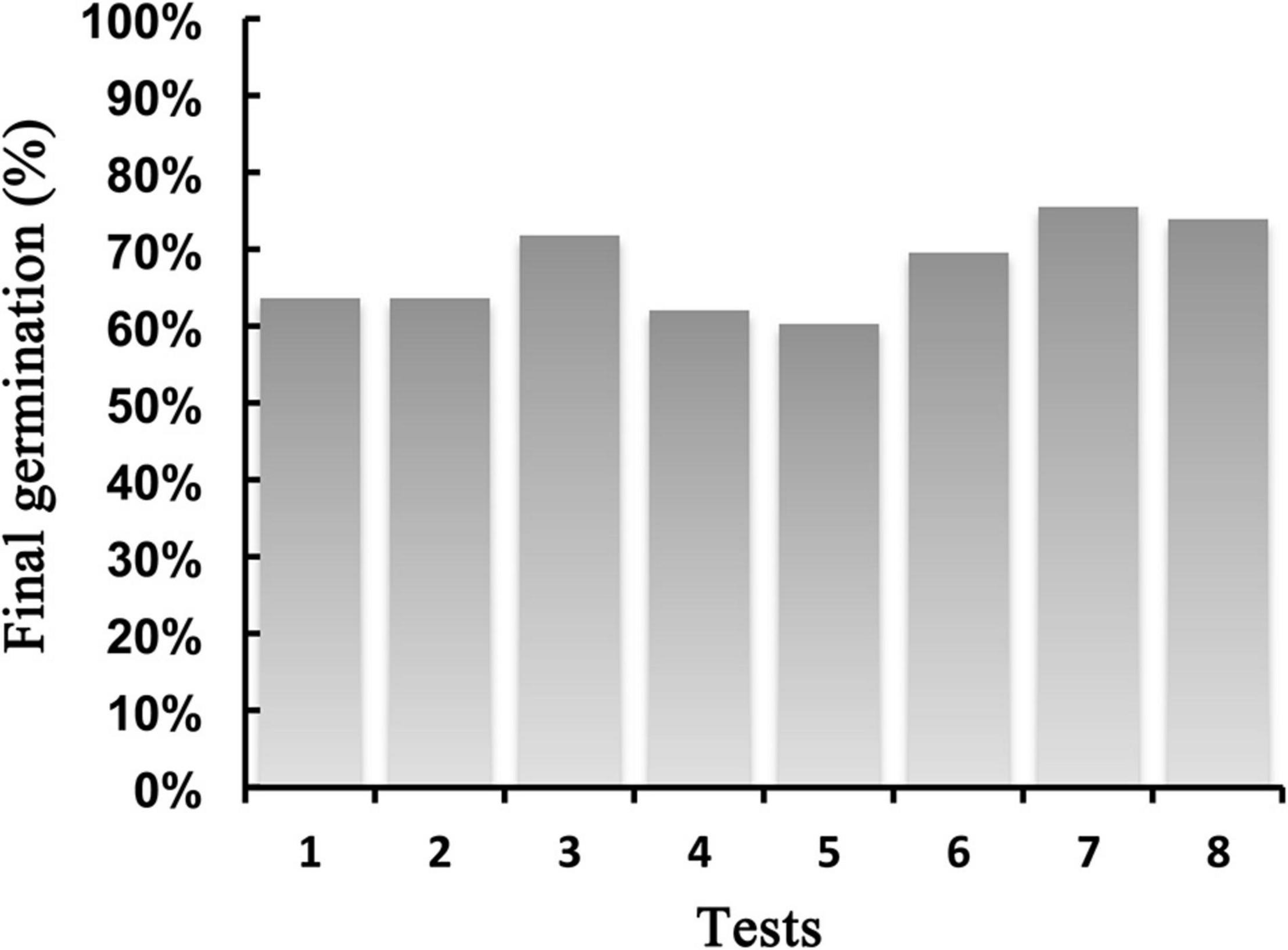
Figure 4. Best germination percentages achieved in different germination tests (substrate/luminosity/environment/other treatments). (1) Sterile soil, light, and room temperature. (2) Natural soil, light, and room temperature. (3) Cotton fibers, with soil suspension, light, room temperature, and seeds bleached. (4) Cotton fibers, in water; light; room temperature; and seeds bleached. (5) Agar, darkness, and controlled light and temperature. (6) Agar, light, and controlled light and temperature. (7) Agar, light, controlled light and temperature, and Al. (8) Agar, light, controlled light and temperature, and Al + Ca. Data from Serrano, 2015, Chapter 7.
The main problem envisaged is to correctly preserve the seeds to maintain their viability before seeding as it is lost rapidly (less than 5 years) when in non-controlled laboratory conditions (Serrano, 2015). Those observations favor the suggestion that the soil seed bank has no special role in the population expansion, yet its value must not be neglected for population endurance, particularly considering the dangers of depletion from overcollecting seeds. Some seeds have been stored for conservation collections in a seed bank (A.L. Belo Correia—BG.MNHN.UL, Lisbon), collected in 2011 and 2014 from 100–1,000 plants, but at present, no viability tests have been acknowledged. Seeds of P. algarbiensis have also been listed by the A.L. Belo Correia Seed Bank: eight accessions, from two populations in Portugal (2006, 2008, 2009, 2011, and 2014) and one in Spain (2009). These can also be found at the Banco de Germoplasma Vegetal Andaluz (BGVA) in Córdoba.
A successful colonization implies the seedlings’ pre-adaptation to edaphic stresses (O’Dell and Rajakaruna, 2011), and the seedlings of P. almogravensis seemed very resilient to high Al contents (Serrano, 2015). Yet, only approx. 3% of the gap’s total area is colonized by P. almogravensis; the remaining 97% is probably either too toxic (Al), has other environmental stresses (Serrano et al., 2015), or has physical barriers to establishment (e.g., slope, rock, and hard crust) (Zaady et al., 1997; Baskin and Baskin, 2014). Difficulties in establishing into the substrate, due to Al-induced reduction in root growth as well as water (dehydration), temperature and light (insolation), and nutrient limitations, may explain the failure to colonize large areas of the gap rich in Al. The role of Ca in alleviating Al toxicity seems important, but needs further study: seedlings grown in low-Al and high-Ca media (more alike the shrubland conditions) showed lower survival rates (Serrano et al., 2011; Serrano, 2015).
Seedling establishment is a highly risky phase that is dependent on environmental conditions, particularly water availability (Serrano, 2015), with approx. 79% mortality in field conditions after 1 year (38% surviving juveniles × 56% surviving young adults). Many plants, mostly the juveniles, do not survive the summer. Analysis of the 13C isotopes (Serrano et al., 2015) indicated that gap plants experience severe water stress in the summer. This would affect the survival of both adults and juveniles, especially if their root system is underdeveloped. The first year is the most critical as the juveniles have an undeveloped root system and no reserves to spare.
On occasions, older plants were observed not being able to resprout after a year of exceptional flower production (Serrano, 2015), which might indicate that their carbon and nutrient reserves were allocated to sexual reproduction, not supporting regrowth after the summer drought (Zeppel et al., 2014), and the plants died.
Overall, recruits and adults of P. almogravensis seem to be mostly limited by competition, Al toxicity (causing water and nutrient deficiencies), and drought (leading to unrecoverable necrosis). Recruitment is, therefore, a critical stage for P. almogravensis, so it is proposed that assisted transplants in conservation programs should be delayed until the plantlets have gained enough biomass to withstand those stresses, that is to say, about 1 year.
The P. almogravensis realized niche presents many explanations for the rarity of this species. The geochemical islands are scarce and isolated, representing obstacles for dispersion, thus lowering the chances of genetic exchanges that would strengthen the population. The edapho-climatic stressful nature of the niche (geochemical, plus longer direct sunlight periods, stronger wind, and sea spray exposure) could have led to nanism (an environmentally driven phenotype), with impacts on reproduction (e.g., less flowers, lower diffusion of pollen, smaller seeds, and lower distance of seed dispersion) and on general fitness. Dispersion to suitable areas, where recruits can establish and adults thrive, seems to be a strong limiting factor for population growth. To overcome this step, assisted germinations followed by transplants (outplanting) to suitable areas seem promising (Pinto et al., 2013; Serrano et al., 2014). Assisted germinations using native soil, in controlled environments, have provided a better survival rate (over 90%) for the seedlings (Pinto et al., 2013; Serrano, 2015), followed by successful adult plant transplantations to the field, which thus proved to be a method to consider in expanding conservation programs (Pinto et al., 2013). When using seeds or especially if using cloned plants, care should be taken to account for the genetic variability of the introductions as it may further reduce the natural population genetic pool.
Phylogeny
Generally, when discussing conservation measures, everyone agrees on the importance of preserving all biodiversity components, including evolutionary information. Furthermore, genetic diversity and evolutionary age are pointed to as factors of rarity and related to endangered species (Stebbins, 1980; Kruckeberg and Rabinowitz, 1985; Fiedler, 1986; Purvis et al., 2000; Klironomos, 2002; Ferreira and Boldrini, 2011; Gaston, 2011). When populations are lost, inevitably, evolutionary information is also lost, particularly if those populations have adapted to distinct ecological niches irrespective of being considered as species, subspecies, or ecotypes/varieties. Thus, infraspecific ranks are also recognized in IUCN conservation assessments, considering their importance for biodiversity.
Phylogenetic Diversity and Taxonomy
To be of value, taxa need to clearly demonstrate phylogenetic distinctness. Because this is neither an easy nor an objective task, several criteria/disciplines may provide important factors for decision.
The Flora Iberica (Pedrol, 2009) has driven the international adoption (e.g., Catalog of Life, Hassler, 2020) of the nomenclature that considers P. algarbiensis and P. almogravensis as synonyms (= P. algarbiensis). This view supports their conclusions on limited herbarium material (e.g., mature pyxidia and seeds were not observed for P. algarbiensis; in Pedrol, 2009), constraining the results regarding morphological ranges or trait taxonomic value and indicating a need of further studies, as acknowledged by the author. Franco (1984) considered as significant the differences between the two taxa in extent of ramification, size or shape of the leaves and reproductive structures, and color after drying, causing him to rank them both at species level. Most Portuguese botanists/taxonomists (e.g., Flora-On, 2014; Carapeto et al., 2020) as well as the Portuguese Governmental Institute for Nature Conservation and Forests (ICNF) use this classification, as well as those to whom they report [e.g., European Nature Information System (EUNIS) and International Union for Conservation of Nature (IUCN)] (Bilz, 2011a,b).
Data from plants in natural populations, pot grown, or from micropropagation (Martins et al., 2012; Serrano, 2015) have shown significant differences between the two taxa regarding ecology, phenology, and morphology (number of rosettes and branching; leaf size and plasticity; peduncle and inflorescence size, number of flowers, and compaction; bracts length; number of viable seeds per flower; and seed weight, among others). At the biochemical level, differences were significant for traits like tolerance to Al and its accumulation (Martins et al., 2013a; Serrano, 2015; Serrano et al., 2017), organic acid secretion (Martins et al., 2013a), chlorophyll, proline, carbohydrate, peroxide, catalase and superoxide dismutase contents (Martins et al., 2013b,c), lipid peroxidation, antioxidant activity, and protein content (Gonçalves et al., 2015, 2017). These differences could also be considered when deciding on the degree of separation between the two taxa.
The evaluation of Plantago molecular phylogeny integrated P. almogravensis and P. algarbiensis within the P. subulata aggregate, of sect. Maritima, in subgen. Coronopus (Serrano et al., 2017). This classification is in agreement with previous phylogenies derived from morphological and chemical characteristics (Franco, 1984; Rahn, 1996; Marhold, 2011). More recently, Höpke et al. (2019) did a phylogenetic study more dedicated to the Coronopus section using molecular (ITS and trnL-F) and morphometric analysis that did not include these two taxa; nonetheless, they concluded that further investigations are needed, confirming the difficulties in studying this group. The only studies using these two taxa known at the moment are the ones from Serrano et al. (2017), after Serrano (2015), using the two combined genomic regions, ITS and trnF-L (Figure 5; Serrano, 2015), that positioned P. algarbiensis (including data from Portuguese and Spanish populations) and P. holosteum (sensu Pedrol, 2009, sampled > 450 km apart from the other species) in the same (74% bootstrap) monophyletic branch as P. almogravensis, paraphyletic with P. subulata (GenBank accessions AY101878 and AY101933, unknown origin; Rønsted et al., 2002), forming the P. subulata aggregate (80% bootstrap; Serrano et al., 2017) (GenBank accessions: P. almogravensis, KJ579144 and KJ579149; P. algarbiensis, KJ579143 and KJ579148; and P. holosteum, KJ579142 and KJ579147). Ferreira et al. (2013), using ISSR and RAPD markers for P. almogravensis and P. algarbiensis, obtained a low level of genetic differentiation (Gst = 0.1873) between them, but cluster analysis and principal component analysis (PCA) based on genetic similarity revealed two clearly separate clusters, corresponding to the plants isolated from each species. Further detailed studies on these sect. Maritima populations/taxa are essential to fully understand the phylogeny.
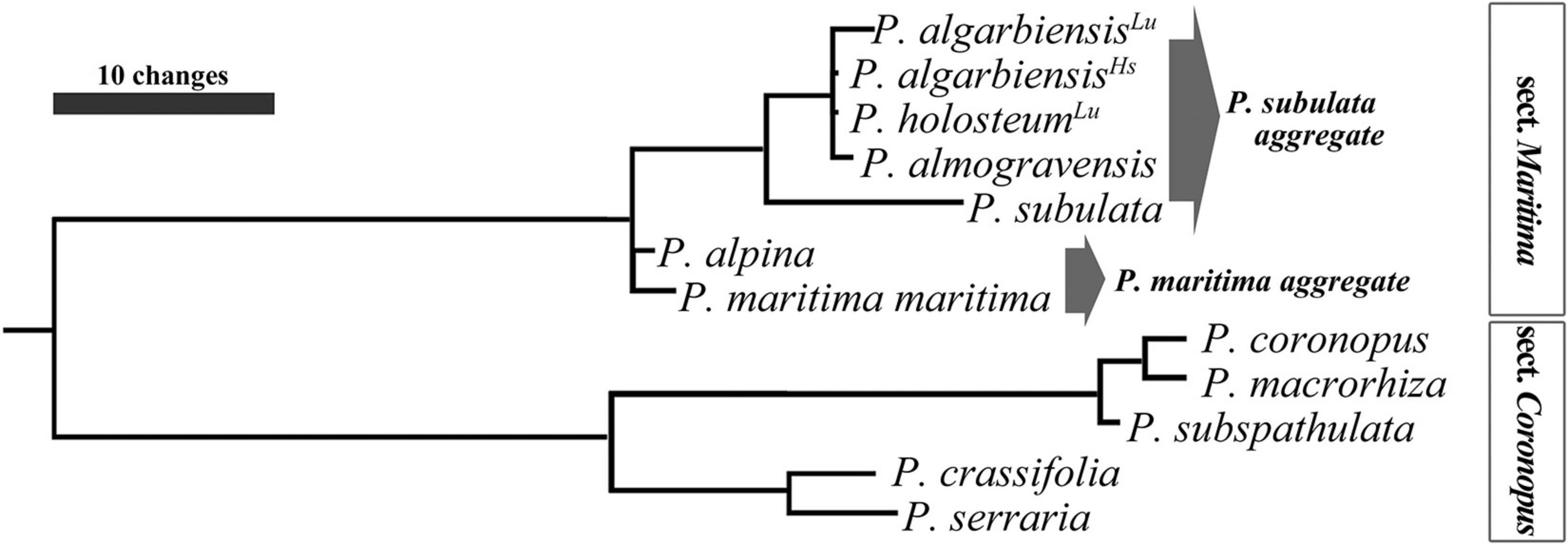
Figure 5. Phylogram of Plantago subgen. Coronopus. Detail of the ITS + trnL-F strict consensus tree (Serrano, 2015). P. algarbiensisLu: Portuguese population; P. algarbiensisHs: Spanish population; P. holosteumLu: syn. P. radicata, Portuguese populations. The sequences of the sister species P. almogravensis, P. algarbiensis (Lu & Hs), and P. holosteum analyzed share from 99.56% to 99.81% identity. Their 1,864-bp aligned matrix differs in eight base positions, six uncertain due to unresolved ambiguities and three with different bases or gaps. In comparison, P. subulata shares from 98.45% to 98.51% identity with those paraphyletic taxa in the same aggregate. Figure from Serrano (2015), p. 160.
For conservation purposes, genetic diversity is as important to be addressed for rare species with narrowed areas of distribution or isolated populations of infraspecific taxa (Campetella et al., 2020). However, the observed differences in rarity within this Plantago group might appear to be more dependent on local trait adaptation rather than on phylogenetic relations. According to Hermoso et al. (2019), halting loss of biodiversity in the EU requires moving beyond species lists, or even other taxonomic doubts. Priority for conservation must be given to threatened and highly endangered species. Thus, protection of rare narrowly distributed species/populations will automatically ensure the conservation of distinct species.
Putative Species Origin
Often, a rare endemic species is considered paleo- or neoendemic regarding their evolutionary age and history (Kruckeberg, 1954; Stebbins, 1980; Kay et al., 2011; Anacker, 2014). Paleoendemics are populations derived from former widespread species after biotype depletion, occupying now a small part of their former range. Neoendemics (also known as insular endemics) are younger lineages, originated after adaptive evolution. There are interesting options to explore, considering the “age” of the P. almogravensis extant population.
Arguments favoring the neoendemic hypothesis can be: (i) woodiness: secondary woodiness has been described in other endemic Plantago spp. associated with neoendemics arising from habitat shifts (Carlquist and Cole, 1974; Rønsted et al., 2002; Dulin, 2008; Dunbar-Co et al., 2008;, after Carlquist, 1970); (ii) isolation: a progressive isolation, together with particular climate and soils of the region, namely, metalliferous ones, would be strong drivers to ecotypic differentiation, followed eventually by speciation (O’Dell and Rajakaruna, 2011). Isolation and geochemical pressures have differentiated other taxa as short as in 150 years (Macnair, 1989 and thereafter).
Favoring the paleoendemic hypothesis: (i) the fact that P. almogravensis has weak competitive ability and may have been pushed to the geochemical islands. This suggests that the species could have lost adaptive ability to new competitors and environmental changes. (ii) If P. almogravensis woodiness is found to be an ancestral character (Mabberley, 1974), its extant population could then represent the biotypes that persisted from a formerly widely distributed species, and even the other sister species could have evolved from it (Figure 6). This would have similarities to some Macaronesian taxa derived from continental ancestors extinguished in Europe following the Pleistocene glaciation or in Africa due to desertification (Dulin and Kirchoff, 2010).
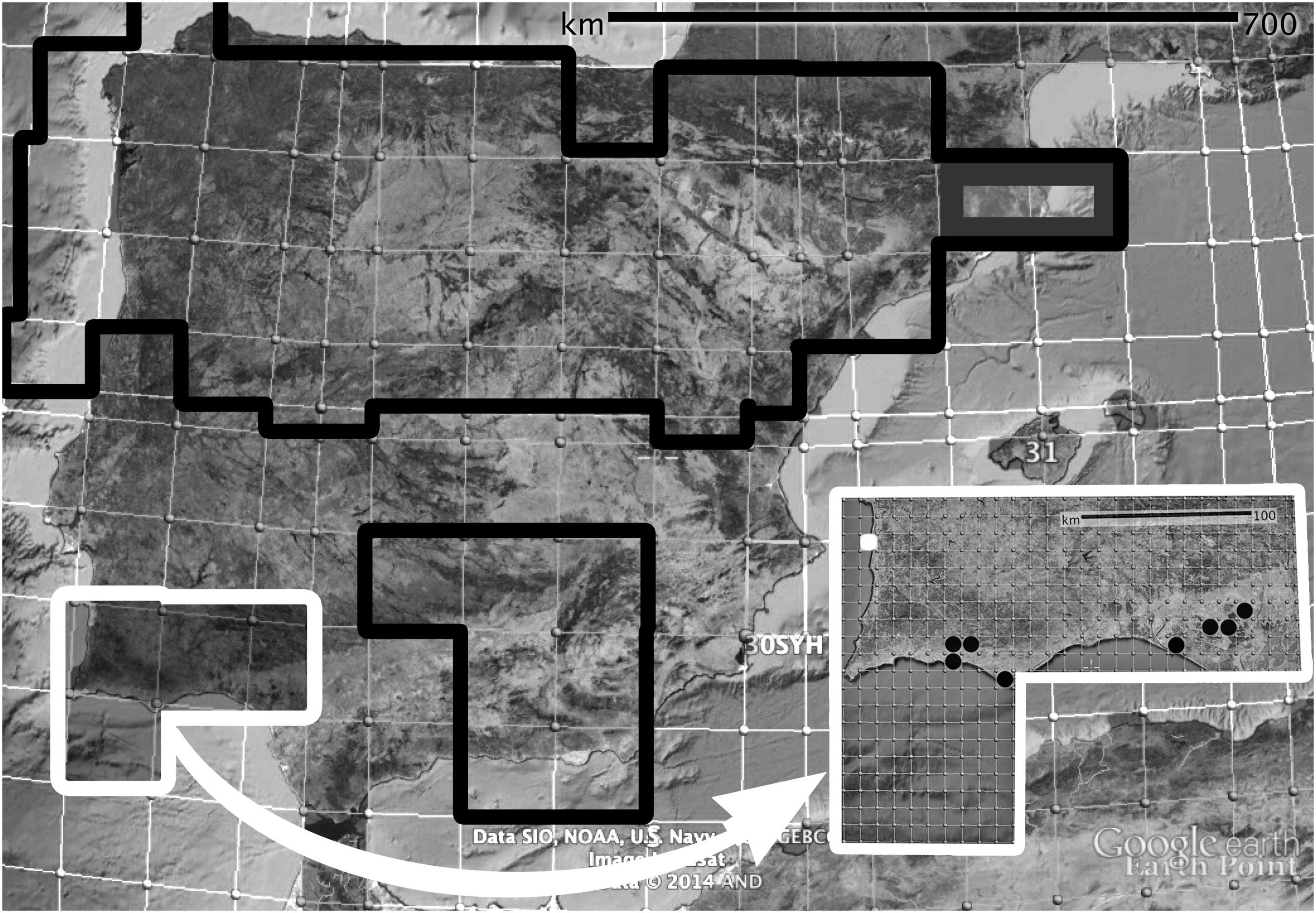
Figure 6. Relative locations of four Plantago species (P. subulata aggregate) in the Iberian Peninsula. MGRS grid (100 km): P. almogravensis and P. algarbiensis (white), P. holosteum (black), and P. subulata (gray). Detail (10 km grid): P. almogravensis (white dot) and P. algarbiensis (black dots). Images:© Google Earth 2015; gridlines:© www.earthpoint.us. Adapted from Serrano (2015), p. 161.
From these two hypotheses, apparently, this rare Plantago species is more like the island endemics that have recently evolved through environmental adaptation (adapted to geochemical islands in this case).
Conservation Remarks
This review on the ecological niche of the rare endemic P. almogravensis has shown that a combination of several environmental factors, more than genetic reasons, led to the current endangered status of these populations (Carapeto et al., 2020). Altogether, biotic and abiotic stresses limit the ecological performance and expansion of the current population that cannot expand on its own, needing scientifically guided assistance; abiotic stress mainly impacts on the physiological traits, while competition with shrub species limits mostly the dispersion of P. almogravensis populations to nearby soils with better growth conditions. This leads to a particular case of rarity and endangered status similar to what is found in many islands of endemic or site-specific species. Here, we suggest putative conservation measures that can be applied to these rare and endangered Plantago species as the basis for other similarly rare narrowly distributed species, including other metalliferous-tolerant ones.
Conservation efforts should be primarily directed to continue the assisted management and monitoring, for this species in particular, on the natural park (PNSACV) southwest coast, beyond, of course, maintaining or improving the ecological conditions of the current population. This would imply not only the application of the original sectorial plan for the area but also an improved plan that considers expansion and buffer areas, as well as human resources to effectively manage the area. Therefore, the first conservation measure would be to preserve the extant population through maintaining the ecosystem balance to which the species is adapted (habitat conservation). Disturbances in the water availability and nutrient input (e.g., agriculture) or excessive trampling (e.g., human activities) may affect the ecological niche boundaries and the survival of the adult reproducing plants. The presence of invasive species with undetermined responses to Al toxicity may compromise the refuge of the plants in the gaps that were safe from natural shrubland colonization; thus, their timely management is imperative (habitat reclamation).
To foster the survival chances of the P. almogravensis population, further efforts should be considered too, namely: assisting in population densification and colonization by (i) improving the links between subpopulations. To increase pollination and reduce genetic isolation, the creation of corridors, clear of competing vegetation, uniting subpopulations that now are somewhat isolated is here suggested. This can be done by, for example, promoting the clearing of senescent shrubs, leaving enough open space for the growth of P. almogravensis individuals that can live and reproduce for many (10–20) years while still in protected microclimatic conditions. Connecting subpopulations would diminish the need for assisted dispersion or pollination, while it would potentiate genetic diversity (crossed pollination); it would reinforce P. almogravensis density and improve population fitness (Smith et al., 2014). As Colwell and Fuentes (1975) stated: “Broadening of the realized niche of a population with increased density,… can alter the trophic structure or spatial pattern of the communities, with secondary effects on the niches of other species.” Thus, increasing artificially the proportion of the rare species in the community might be an important step into further ecosystem changes that favor its natural recovery. Newly founded populations resulting from assisted translocation programs also fit this purpose since a putative set of traits might arise from the different interactions with the new colonizing environments. (ii) Identifying new suitable habitats: sustainable conservation efforts should identify other suitable areas, including those that are metalliferous, where P. almogravensis could establish and found new populations. A location that balances (less) competition and (lower) environmental stress load would improve the overall physiological performance of the population, including reproductive and survival fitness. Also, the existence of new populations represents a smaller risk of stochastic extinction. Thus, further translocation experiments should be recommended, seeking to uncover new favorable locations where the realized niche should not be so severe, allowing for demographic recovery and better coping with global warming and climate change. (iii) Assisted reproduction: To populate new sites and densify the existing population, previous experiments have confirmed that it is more successful to transplant young P. almogravensis adults to similar edaphic areas than to do direct seeding due to its lower seedling survival rates (Pinto et al., 2013; Serrano et al., 2014). Following successful recruitment, adults are expected to be more resilient to summer drought (and are occasionally capable of vegetative reproduction) after transplant to the final locations. Germination and survival tests recommend the use of native soil inoculum, in assisted germinations and transplants, as it is a source of beneficial symbiotic microorganisms. Finally, considering genetic resource conservation, the (iv) improvement of ex situ conservation collections. To guarantee that the genetic heritage is not lost for future generations in case of local extinction, but also to decrease the chances of that event happening by providing a source of genetic diversity, the conservation collections should be reinforced. Although there are a few seeds stored in seed banks, this Plantago has not been a priority in their lists. Living collections can also be an option, although there is the risk of loss of distinctive local adaptations over the generations, unless the edaphic and climatic characteristics are somehow preserved.
Any conservation measure, or project, should take into consideration ecological and taxonomic studies by incorporating knowledge on species evolutionary uniqueness and plant physiological performance.
Author Contributions
HS wrote the manuscript draft. All authors contributed to the interpretation of data and manuscript substantial revisions.
Conflict of Interest
The authors declare that the research was conducted in the absence of any commercial or financial relationships that could be construed as a potential conflict of interest.
Funding
This work and writing were funded by national funds through FCT—Fundação para a Ciência e Tecnologia, in the frame of the project UIDB/00329/2020 (cE3c).
Acknowledgments
We would like to thank the field work done during several years of monitoring, namely, by MP, and the authors’ combined efforts that resulted in a Ph.D. thesis by HS (http://hdl.handle.net/10451/22721), with the support of the Jardim Botânico do Museu Nacional de História Natural e da Ciência, da Universidade de Lisboa, and ICNF—Instituto da Conservação da Natureza e das Florestas, and funding by FCT—Fundação para a Ciência e Tecnologia. We would also like to thank the constructive comments made by the reviewers and the opportunity to improve the original manuscript.
References
Albach, D. C., Meudt, H. M., and Oxelman, B. (2005). Piecing together the “new” Plantaginaceae. Am. J. Bot. 92, 297–315. doi: 10.3732/ajb.92.2.297
Anacker, B. L. (2014). The nature of serpentine endemism. Am. J. Bot. 101, 219–224. doi: 10.3732/ajb.1300349
Anacker, B. L., Whittall, J. B., Goldberg, E. E., and Harrison, S. P. (2011). Origins and consequences of serpentine endemism in the California flora. Evolution 65, 365–376. doi: 10.1111/j.1558-5646.2010.01114.x
Antonovics, J., Bradshaw, A. D., Turner, R. G., and Cragg, J. B. (1971). “Heavy metal tolerance in plants,” in Advances in Ecological Research, ed. J. B. Cragg (New York, NY: Academic Press).
Baker, A. J. M., Ernst, W. H. O., van der Ent, A., Malaisse, F., and Ginocchio, R. (2010). “Metallophytes: the unique biological resource, its ecology and conservational status in Europe, central Africa and Latin America,” in Ecology of Industrial Pollution, eds L. C. Batty and K. B. Hallberg (Cambridge: Cambridge University Press).
Baker, A. J. M., and Proctor, J. (1990). The influence of cadmium, copper, lead, and zinc on the distribution and evolution of metallophytes in the British-Isles. Plant Syst. Evol. 173, 91–108. doi: 10.1007/bf00937765
Baskin, C. C., and Baskin, J. M. (2014). Seeds: Ecology, Biogeography, and Evolution of Dormancy and Germination. Amsterdam: Elsevier Science.
Bilz, M. (2011a). Plantago algarbiensis. The IUCN Red List of Threatened Species 2011: e.T161827A5500870. Available online at: https://dx.doi.org/10.2305/IUCN.UK.2011-1.RLTS.T161827A5500870.en (accessed April 1, 2021).
Bilz, M. (2011b). Plantago almogravensis. The IUCN Red List of Threatened Species 2011: e.T162360A5579429. Available online at: https://dx.doi.org/10.2305/IUCN.UK.2011-1.RLTS.T162360A5579429.en (accessed April 1, 2021).
Blom, C. W. P. M. (1992). “Germination and establishment,” in Plantago: A Multidisciplinary Study, eds P. J. C. Kuiper and M. Bos (Berlin: Springer-Verlag).
Boyd, R. S., and Martens, S. N. (1998). The significance of metal hyperaccumulation for biotic interactions. Chemoecology 8, 1–7. doi: 10.1007/s000490050002
Branquinho, C., Serrano, H. C., Pinto, M. J., and Martins-Loução, M. A. (2007). Revisiting the plant hyperaccumulation criteria to rare plants and earth abundant elements. Environ. Pollut. 146, 437–443. doi: 10.1016/j.envpol.2006.06.034
Brunner, I., and Sperisen, C. (2013). Aluminum exclusion and aluminum tolerance in woody plants. Front. Plant Sci. 4:172. doi: 10.3389/fpls.2013.00172
Campetella, G., Chelli, S., Simonetti, E., Damiani, C., Bartha, S., Wellstein, C., et al. (2020). Plant functional traits are correlated with species persistence in the herb layer of old-growth beech forests. Sci. Rep. 10:19253. doi: 10.1038/s41598-020-76289-7
A. Carapeto, A. Francisco, P. Pereira, and M (eds.) Porto (2020). Lista Vermelha da Flora Vascular de Portugal Continental. Sociedade Portuguesa de Botânica, Associação Portuguesa de Ciência da Vegetação – PHYTOS e Instituto da Conservação da Natureza e das Florestas (coord.). Coleção <<Botânica em Português>>, Vol. 7. Lisboa: Imprensa Nacional, pp. 374. ISBN 978 -972-27-2876 – 8. Available online at: https://listavermelha-flora.pt/wp-content/uploads/2020/10/Lista_Vermelha_Flora_Vascular_Portugal_Continental_2020_versao_digital.pdf (accessed April 1, 2021).
Carlquist, S. J. (1970). Wood anatomy of insular species of Plantago and problem of raylessness. Bull. Torrey Bot. Club 97, 353–361. doi: 10.2307/2483855
Coelho, N., Martín, C., González-Benito, M. E., and Romano, A. (2017). Estimation of genetic diversity in seedlings of Plantago algarbiensis, an endangered species endemic to the south of Portugal in risk of global extinction. Braz. J. Bot. 40, 257–264. doi: 10.1007/s40415-016-0340-5
Colwell, R. K., and Fuentes, E. R. (1975). Experimental studies of niche. Annu. Rev. Ecol. Syst. 6, 281–310. doi: 10.1146/annurev.es.06.110175.001433
Dulin, M. W. (2008). An Investigation of Paedomorphic Secondary Xylem and Secondary Woodiness in Xanthorhiza Simplicissima, Coreopsis Gigantea, and Mahonia Bealei. Greensboro, NC: The University of North Carolina at Greensboro College of Arts & Sciences.
Dulin, M. W., and Kirchoff, B. K. (2010). Paedomorphosis, secondary woodiness, and insular woodiness in plants. Bot. Rev. 76, 405–490. doi: 10.1007/s12229-010-9057-5
Dunbar-Co, S., Wieczorek, A. M., and Morden, C. W. (2008). Molecular phylogeny and adaptive radiation of the endemic Hawaiian Plantago species (Plantaginaceae). American Journal of Botany 95, 1177–1188. doi: 10.3732/ajb.0800132
Dunbar-Co, S., Sporck, M. J., and Sack, L. (2009). Leaf trait diversification and design in seven rare taxa of the Hawaiian Plantago radiation. Int. J. Plant Sci. 170, 61–75. doi: 10.1086/593111
EEA/MS (2019). Article 17 web tool on biogeographical assessments of conservation status of species and habitats under Article 17 of the Habitats Directive. 2013-2017 reporting – Biogeographical assessments at Member State level – Species Assessments. European Environmental Agency, European Topic Centre on Biological Diversity. Available online at: https://nature-art17.eionet.europa.eu/article17/species/report/ (accessed April 1, 2021).
Ferreira, P. M., and Boldrini, I. I. (2011). Potential reflection of distinct ecological units in plant endemism categories. Conserv. Biol. 25, 672–679. doi: 10.1111/j.1523-1739.2011.01675.x
Ferreira, V., Matos, M., Correia, S., Martins, N., Gonçalves, S., Romano, A., et al. (2013). Genetic diversity of two endemic and endangered Plantago species. Biochem. Syst. Ecol. 51, 37–44. doi: 10.1016/j.bse.2013.08.003
Fiedler, P. L. (1986). Concepts of rarity in vascular plant-species, with special reference to the genus Calochortus Pursh (Liliaceae). Taxon 35, 502–518. doi: 10.2307/1221904
Flora-On: Flora de Portugal Interactiva (2014). Sociedade Portuguesa de Botânica. Available online at: www.flora-on.pt (accessed on April 1, 2021).
Gonçalves, S., Grevenstuk, T., Martins, N., and Romano, A. (2015). Antioxidant activity and verbascoside content in extracts from two uninvestigated endemic Plantago spp. Indust. Crops Products 65, 198–202. doi: 10.1016/j.indcrop.2014.12.011
Gonçalves, S., Martins, N., and Romano, A. (2017). Physiological traits and oxidative stress markers during acclimatization of micropropagated plants from two endangered Plantago species: P. algarbiensis Samp. and P. almogravensis Franco. In Vitro Cell. Dev. Biol. Plant 53, 249–255. doi: 10.1007/s11627-017-9812-y
Hassemer, G. (2018). Advances to the taxonomic knowledge of Plantago subulata (Plantago sect. Maritima, Plantaginaceae). Turkish J. Bot. 42, 653–661. doi: 10.3906/bot-1801-51
Hassemer, G. (2019). Novelties and notes on Plantago sect. Virginica (Plantaginaceae), including the description of a new species and a revised identification key. Webbia 74, 29–41. doi: 10.1080/00837792.2019.1574114
Hassemer, G., Meudt, H. M., and Rønsted, N. (2017). Nomenclatural and taxonomic notes on Mediterranean narrow-leaved plantains (Plantago section Maritima, Plantaginaceae). Webbia 72, 197–205. doi: 10.1080/00837792.2017.1349066
Hassemer, G., and Rønsted, N. (2016). Yet another new species from one of the best-studied neotropical areas: Plantago humboldtiana (Plantaginaceae), an extremely narrow endemic new species from a waterfall in southern Brazil. PeerJ 4:e2050. doi: 10.7717/peerj.2050
Hassemer, G., Shipunov, A. B., Rønsted, N., and Meudt, H. M. (2018). Taxonomic and geographic novelties in the genus Plantago (Plantaginaceae) in Chile, including the description of a new species. Phytotaxa 340, 137–156. doi: 10.11646/phytotaxa.340.2.3
Hassler, M. (2020). “World plants: synonymic checklists of the vascular plants of the world (version sep 2020),” in Species 2000 & ITIS Catalogue of Life, 2020-12-01. (Digital Resource at www.Catalogueoflife.Org), eds Y. Roskov, G. Ower, T. Orrell, D. Nicolson, N. Bailly, P. M. Kirk, et al. (Leiden: Species 2000).
Hermoso, V., Moran-Ordonez, A., Canessa, S., and Brotons, L. (2019). Realising the potential of Natura 2000 to achieve EU conservation goals as 2020 approaches. Sci. Rep. 9:16087. doi: 10.1038/s41598-019-52625-4
Hoggard, R. K., Kores, P. J., Molvray, M., Hoggard, G. D., and Broughton, D. A. (2003). Molecular systematics and biogeography of the amphibious genus Littorella (Plantaginaceae). Am. J. Bot. 90, 429–435. doi: 10.3732/ajb.90.3.429
Höpke, J., Mucina, L., and Albach, D. C. (2019). Phylogenetic and morphometric analysis of Plantago section Coronopus (Plantaginaceae). Taxon 68, 315–339. doi: 10.1002/tax.12035
Iamonico, D., Hassemer, G., Rønsted, N., and di Pietro, R. (2017). The intricate nomenclatural questions around Plantago holosteum (Plantaginaceae). Phytotaxa 306, 75–84. doi: 10.11646/phytotaxa.306.1.6
Jansen, S., Watanabe, T., and Smets, E. (2002). Aluminium accumulation in leaves of 127 species in Melastomataceae, with comments on the order Myrtales. Ann. Bot. 90, 53–64. doi: 10.1093/aob/mcf142
Kay, K. M., Ward, K. L., Watt, L. R., and Schemske, D. W. (2011). “Plant speciation,” in Serpentine: The Evolution and Ecology of a Model System, eds S. P. Harrison and N. Rajakaruna (Berkeley, CA: University of California Press).
Keivani, M., Mehregan, I., and Albach, D. (2020). Genetic diversity and population structure of Plantago major (Plantaginaceae) in Iran. Iran. J. Bot. 26, 111–124. doi: 10.22092/ijb.2020.343166.1287
Kleijn, D., Bekker, R. M., Bobbink, R., De Graaf, M. C. C., and Roelofs, J. G. M. (2008). In search for key biogeochemical factors affecting plant species persistence in heathland and acidic grasslands: a comparison of common and rare species. J. Appl. Ecol. 45, 680–687. doi: 10.1111/j.1365-2664.2007.01444.x
Klironomos, J. (2002). Feedback with soil biota contributes to plant rarity and invasiveness in communities. Nature 417, 67–70. doi: 10.1038/417067a
Kruckeberg, A. R. (1954). The ecology of serpentine soils: III. Plant species in relation to serpentine soils. Ecology 35, 267–274.
Kruckeberg, A. R., and Rabinowitz, D. (1985). Biological aspects of endemism in higher-plants. Annu. Rev. Ecol. Syst. 16, 447–479. doi: 10.1146/annurev.es.16.110185.002311
Lyons, K. G., Brigham, C. A., Traut, B., and Schwartz, M. W. (2005). Rare species and ecosystem functioning. Conserv. Biol. 19, 1019–1024. doi: 10.1111/j.1523-1739.2005.00106.x
Mabberley, D. J. (1974). Pachycauly, vessel-elements, islands and evolution of arborescence in herbaceous families. New Phytol. 73, 977–984. doi: 10.1111/j.1469-8137.1974.tb01326.x
Macnair, M. R. (1989). A new species of Mimulus endemic to copper mines in California. Bot. J. Linnean Soc. 100, 1–14. doi: 10.1111/j.1095-8339.1989.tb01707.x
Marhold, K. (2011). Plantaginaceae. Euro+Med Plantbase – the Information Resource for Euro-Mediterranean Plant Diversity. Available online at: http://ww2.bgbm.org/EuroPlusMed/ (accessed August 1, 2014).
Martin, M. D., Chamecki, M., Brush, G. S., Meneveau, C., and Parlange, M. B. (2009). Pollen clumping and wind dispersal in an invasive angiosperm. Am. J. Bot. 96, 1703–1711. doi: 10.3732/ajb.0800407
Martins, N., Goncalves, S., Andrade, P. B., Valentao, P., and Romano, A. (2013a). Changes on organic acid secretion and accumulation in Plantago almogravensis Franco and Plantago algarbiensis Samp. under aluminum stress. Plant Sci. 198, 1–6. doi: 10.1016/j.plantsci.2012.09.001
Martins, N., Goncalves, S., Palma, T., and Romano, A. (2012). Seed germination of two critically endangered plantain species, Plantago algarbiensis and P. almogravensis (Plantaginaceae). Seed Sci. Technol. 40, 144–149. doi: 10.15258/sst.2012.40.1.19
Martins, N., Osório, M. L., Gonçalves, S., Osório, J., Palma, T., and Romano, A. (2013b). Differences in Al tolerance between Plantago algarbiensis and P. almogravensis reflect their ability to respond to oxidative stress. Biometals 26, 427–437. doi: 10.1007/s10534-013-9625-3
Martins, N., Osório, M. L., Gonçalves, S., Osório, J., Palma, T., and Romano, A. (2013c). Physiological responses of Plantago algarbiensis and P. almogravensis shoots and plantlets to low pH and aluminum stress. Acta Physiol. Plant 35, 615–625. doi: 10.1007/s11738-012-1102-z
McGill, B. J. (2012). Trees are rarely most abundant where they grow best. J. Plant Ecol. 5, 46–51. doi: 10.1093/jpe/rtr036
Meharg, A. A. (1994). Integrated tolerance mechanisms - constitutive and adaptive plant-responses to elevated metal concentrations in the environment. Plant Cell Environ. 17, 989–993. doi: 10.1111/j.1365-3040.1994.tb02032.x
Meharg, A. A. (2003). The mechanistic basis of interactions between mycorrhizal associations and toxic metal cations. Mycol. Res. 107, 1253–1265. doi: 10.1017/s0953756203008608
Moore, D. M., Chater, A. O., and Cartier, D. (1976). “Plantaginaceae,” in Flora Europaea, Vol. 4, eds T. G. Tutin, V. H. Heywood, N. A. Burges, D. M. Moore, D. H. Valentine, S. M. Walters, et al. (Cambridge: Cambridge University Press), 38–44.
Mouillot, D., Bellwood, D. R., Baraloto, C., Chave, J., Galzin, R., Harmelin-Vivien, M., et al. (2013). Rare species support vulnerable functions in high-diversity ecosystems. PLoS Biol. 11:e1001569. doi: 10.1371/journal.pbio.1001569
O’Dell, R. E., and Rajakaruna, N. (2011). “Intraspecific variation, adaptation, and evolution,” in Serpentine: Evolution and Ecology in a Model System, eds S. P. Harrison and N. Rajakaruna (Berkeley, CA: University of California Press).
Okubo, A., and Levin, S. A. (1989). A theoretical framework for data-analysis of wind dispersal of seeds and pollen. Ecology 70, 329–338. doi: 10.2307/1937537
Ouborg, N., Vergeer, P., and Mix, C. (2006). The rough edges of the conservation genetics paradigm for plants. J. Ecol. 94, 1233–1248. doi: 10.1111/j.1365-2745.2006.01167.x
Pedrol, J. (2009). “Plantago L,” in Flora Ibérica: Plantaginaceae–Scrophulariaceae, Vol. 13, ed. S. Castroviejo (Madrid: Real Jardín Botánico, CSIC Press), 4–38.
Pinto, M. J., Serrano, H. C., Branquinho, C., and Martins-Loução, M. A. (2013). Éxito en la translocación de una planta con restricciones dispersivas. Conserv. Vegetal. 17, 8–9. Available online at: https://revistas.uam.es/conservacionvegetal/issue/download/70/35 (accessed October 1, 2020).
Pinto-Cruz, C. (2010). Temporary Ponds Vegetation and Dynamics: SW Portugal. Lisboa: Instituto Superior de Agronomia, Universidade Tecnica de Lisboa. Available online at: http://hdl.handle.net/10400.5/2862 (accessed May 1, 2021).
Purvis, A., Gittleman, J. L., Cowlishaw, G., and Mace, G. M. (2000). Predicting extinction risk in declining species. Proc. R. Soc. Lond. B 267, 1947–1952. doi: 10.1098/rspb.2000.1234
Rahn, K. (1996). A phylogenetic study of the Plantaginaceae. Bot. J. Linnean Soc. 120, 145–198. doi: 10.1111/j.1095-8339.1996.tb00484.x
RCM115-A/2008 (2008). Resolução do Conselho de Ministros n.° 115-A/2008, aprovação do Plano Sectorial da Rede Natura 2000 (PSRN2000). Diário da República, 1a série, n139, de 21 de Julho de 2008. Available online at: https://data.dre.pt/eli/resolconsmin/115-a/2008/07/21/p/dre/pt/html (accessed May 1, 2021).
Rønsted, N., Chase, M. W., Albach, D. C., and Bello, M. A. (2002). Phylogenetic relationships within Plantago (Plantaginaceae): evidence from nuclear ribosomal ITS and plastid trnL-F sequence data. Bot. J. Linnean Soc. 139, 323–338. doi: 10.1046/j.1095-8339.2002.00070.x
Sampaio, G. A. S. F. (1909). Flora vascular de Odemira. Coimbra: Imprensa da Universidade de Coimbra, 128.
Serrano, H. C. (2015). Ecology of a Rare and Endemic Plantago species. Lisboa: Faculdade de Ci ncias, Universidade de Lisboa. Available online at: http://hdl.handle.net/10451/22721 (accessed October 1, 2020).
Serrano, H. C., Antunes, C., Pinto, M. J., Máguas, C., Martins-Loução, M. A., and Branquinho, C. (2015). The ecological performance of metallophyte plants thriving in geochemical islands is explained by the Inclusive Niche Hypothesis. J. Plant Ecol. 8, 41–50. doi: 10.1093/jpe/rtu007
Serrano, H. C., Cotrim, H., Pinto, M. J., Martins-Loucao, M. A., and Branquinho, C. (2017). Metal hyperaccumulation patterns within Plantago phylogeny (Plantaginaceae). Plant Soil 411, 227–241. doi: 10.1007/s11104-016-3024-9
Serrano, H. C., Pinto, M. J., Cotrim, H., Branquinho, C., and Martins-Loução, M. A. (2014). A investigação ecológica como base de estratégias de conservção. O/El Botanico, Revista anual da/de la AIMJB. 8, 37–39. Available online at: https://issuu.com/elbotanico/docs/revista8_web (accessed May 1, 2021).
Serrano, H. C., Pinto, M. J., Martins-Loução, M. A., and Branquinho, C. (2011). How does an Al-hyperaccumulator plant respond to a natural field gradient of soil phytoavailable Al? Sci. Total Environ. 409, 3749–3756. doi: 10.1016/j.scitotenv.2011.06.036
Smith, T. B., Kinnison, M. T., Strauss, S. Y., Fuller, T. L., and Carroll, S. P. (2014). Prescriptive evolution to conserve and manage biodiversity. Annu. Rev. Ecol. Evol. Syst. 45, 1–22. doi: 10.1146/annurev-ecolsys-120213-091747
van der Heijden, M. G. A., Bardgett, R. D., and van Straalen, N. M. (2008). The unseen majority: soil microbes as drivers of plant diversity and productivity in terrestrial ecosystems. Ecol. Lett. 11, 296–310. doi: 10.1111/j.1461-0248.2007.01139.x
Wamelink, G. W. W., Goedhart, P. W., and Frissel, J. Y. (2014). Why some plant species are rare. PLoS One 9:e102674. doi: 10.1371/journal.pone.0102674
Zaady, E., Gutterman, Y., and Boeken, B. (1997). The germination of mucilaginous seeds of Plantago coronopus, Reboudia pinnata, and Carrichtera annua on cyanobacterial soil crust from the Negev Desert. Plant and Soil 190, 247–252. doi: 10.1023/A:1004269031844
Keywords: aluminum, ecological niche, geochemical islands, hyperaccumulation, Mediterranean, phylogeny, Plantago almogravensis
Citation: Serrano HC, Pinto MJ, Branquinho C and Martins-Loução MA (2021) Ecology as a Tool to Assist Conservation of a Rare and Endemic Mediterranean Plantago Species. Front. Ecol. Evol. 9:614700. doi: 10.3389/fevo.2021.614700
Received: 06 October 2020; Accepted: 10 May 2021;
Published: 16 June 2021.
Edited by:
Terry D. Macfarlane, Conservation and Attractions (DBCA), AustraliaReviewed by:
Attila D. Sándor, University of Agricultural Sciences and Veterinary Medicine of Cluj-Napoca, RomaniaNina Rønsted, National Tropical Botanical Garden, United States
Copyright © 2021 Serrano, Pinto, Branquinho and Martins-Loução. This is an open-access article distributed under the terms of the Creative Commons Attribution License (CC BY). The use, distribution or reproduction in other forums is permitted, provided the original author(s) and the copyright owner(s) are credited and that the original publication in this journal is cited, in accordance with accepted academic practice. No use, distribution or reproduction is permitted which does not comply with these terms.
*Correspondence: Maria Amélia Martins-Loução, bWFsb3VjYW9AZmMudWwucHQ=