- 1Centre for Sustainable Aquatic Research, Department of Biosciences, Swansea University, Swansea, United Kingdom
- 2Department of Biosciences, University of Durham, Durham, United Kingdom
- 3Reptile Amphibian Fish Conservation Netherlands (RAVON), Nijmegen, Netherlands
- 4Department of Fisheries, University of Rajshahi, Rajshahi, Bangladesh
- 5Centre for Invasion Biology, School of Animal, Plant and Environmental Sciences, University of the Witwatersrand, Johannesburg, South Africa
- 6South African Institute for Aquatic Biodiversity, Makhanda, South Africa
- 7Department of Fisheries and Wildlife, Michigan State University, East Lansing, MI, United States
- 8ETH-Zurich, Stocker Lab, Institute of Environmental Engineering, Department of Civil, Environmental and Geomatics Engineering, Zurich, Switzerland
- 9Laboratório de Ecologia e Conservação, Departamento de Engenharia Ambiental, Universidade Federal do Paraná, Curitiba, Brazil
Aquatic invasive species (AIS) are one of the principal threats to freshwater biodiversity. Exclusion barriers are increasingly being used as a management strategy to control the spread of AIS. However, exclusion barriers can also impact native organisms and their effectiveness is likely to be context dependent. We conducted a quantitative literature review to evaluate the use of barriers to control animal AIS in freshwater ecosystems worldwide. The quantitative aspect of the review was supplemented by case studies that describe some of the challenges, successes, and opportunities for the use of the use of AIS exclusion barriers globally. Barriers have been used since the 1950s to control the spread of AIS, but effort has been increasing since 2005 (80% of studies) and an increasingly diverse range of AIS taxa are now targeted in a wide range of habitat types. The global use of AIS barriers has been concentrated in North America (74% of studies), Australasia (11%), and Europe (10%). Physical barriers (e.g., weirs, exclusion screens, and velocity barriers) have been most widely used (47%), followed by electric (27%) and chemical barriers (12%). Fish were the most targeted taxa (86%), followed by crustaceans (10%), molluscs (3%) and amphibians (1%). Most studies have been moderately successful in limiting the passage of AIS, with 86% of the barriers tested deterring >70% of individuals. However, only 25% of studies evaluated barrier impacts on native species, and development of selective passage is still in its infancy. Most studies have been too short (47% < 1 year, 87% < 5 years) to detect ecological impacts or have failed to use robust before-after-control-impact (BACI) study designs (only 5%). Hence, more effective monitoring is required to assess the long-term effectiveness of exclusion barriers as an AIS management tool. Our global case studies highlight the pressing need for AIS control in many ecoregions, and exclusion barriers have the potential to become an effective tool in some situations. However, the design and operation of exclusion barriers must be refined to deliver selective passage of native fauna, and exclusion barriers should only be used sparingly as part of a wider integrated management strategy.
Introduction
Aquatic invasive species (AIS) are one of the leading causes of the global freshwater biodiversity crisis (Dudgeon et al., 2006; Reid et al., 2019). The impacts of AIS are well documented (Strayer, 2010; Gallardo et al., 2016), including predation pressure (Townsend and Crowl, 1991), competition for resources (Kakareko et al., 2013), habitat degradation (Harvey et al., 2011), hybridisation (Deines et al., 2014), and transmission of disease (Britton, 2005). These negative interactions can result in the extirpation of native species and the biotic homogenisation of river communities across ecoregions (Leprieur et al., 2008a; Vitule et al., 2012; Magalhães et al., 2020), as well as affecting ecosystem functioning (Gozlan et al., 2010; Cucherousset and Olden, 2011). The presence of AIS has also been associated with substantial economic impacts. For instance, invasive fishes and molluscs cost the US over $2 billion annually (Pimentel et al., 2000).
Once established, it is often unfeasible to eradicate AIS, and mitigation efforts typically turn towards limiting their spread (Van der Zanden and Olden, 2008). “Isolation management,” “selective fragmentation,” and “intentional fragmentation,” involve the use of exclusion barriers to impede the movement of AIS, typically within freshwater systems (Novinger and Rahel, 2003; Rahel, 2013; Rahel and McLaughlin, 2018). Originating from methods to protect threatened headwater populations of species such as cutthroat trout (Oncorhynchus clarkii) from invading competitors (Thompson and Rahel, 1998), these approaches tend to operate to inhibit the spread of actively moving individuals, rather than passively dispersing organisms. The use of exclusion barriers can be a useful tool for limiting the impacts of AIS and protecting vulnerable native fauna (Novinger and Rahel, 2003; Rahel, 2013; Rahel and McLaughlin, 2018). However, effectively isolating catchment areas to reduce the spread of invasive species often results in a trade-off with the need to maintain river connectivity for native organisms (Porto et al., 1999; Peterson et al., 2008; Fausch et al., 2009).
Hydrological connectivity is critical to the maintenance of healthy and robust riverine ecosystems (Fullerton et al., 2010; Jackson and Pringle, 2010; Perkin and Gido, 2012). Barrier impacts are perhaps most apparent on diadromous fish, whereby habitat fragmentation and reduced connectivity can severely restrict distributions and reduce population abundance (e.g., Pess et al., 2008). However, river-resident fish also need to undertake longitudinal movements to forage, spawn, seek refugia, and recolonise vacant habitat patches (Meyers et al., 1992; Lucas and Baras, 2008), and multiple lines of evidence suggest even small barriers can have negative impacts on potamodromous fish (Junker et al., 2012; Branco et al., 2017; Coleman et al., 2018). Nor are barrier effects restricted to fish, with documented impacts on crustaceans (Barnett et al., 2020), amphibians (Murphy et al., 2018), reptiles (Bennett et al., 2010), and molluscs (Watters, 1996). Indeed, all biota need to disperse; such movement is essential to the maintenance of metapopulations, enables recolonisation following natural disturbances, and contributes to the maintenance of biodiversity (Fullerton et al., 2010).
A recent global estimate of river connectivity indicated that only 37% of the world’s rivers remain free-flowing (Grill et al., 2019) but this assessment did not include small barriers such as weirs, culverts and bed-sills which are orders of magnitude more abundant (Garcia de Leaniz et al., 2019; Jones et al., 2019; Belletti et al., 2020). Hence, rivers worldwide are already severely fragmented and installing more barriers is wholly undesirable unless the benefits significantly outweigh the costs. Previous reviews on the use of exclusion barriers have primarily focussed on fishes within a limited geographic range, have only considered a narrow range of barrier types, and have taken a strictly qualitative approach (Jackson and Pringle, 2010; Rahel, 2013; Rahel and McLaughlin, 2018; Krieg and Zenker, 2020). Before exclusion barriers are implemented widely, it is important to evaluate the state of the science globally. Environmental managers need to know what works and what does not, for what taxa, and in what instances.
We conducted a comprehensive literature review to quantify global trends in the use of barriers to control animal AIS with the aim of evaluating their effectiveness as a management strategy. Within this aim we had several objectives: (i) assess temporal trends in AIS exclusion barrier research, (ii) review how study effort differed globally, (iii) evaluate the robustness of monitoring to assess AIS exclusion barrier effectiveness, (iv) appraise the types of barrier used, (v) examine the habitat types where AIS barriers have been employed, (vi) determine which AIS taxa have been targeted, and (vii) examine the efficacy of AIS exclusion barriers, both in terms of impeding AIS movement, as well as assessing their effects on native fauna. By combining expertise from six continents (Europe, North America, South America, Africa, Asia, and Australasia), we present several case studies to illustrate global successes and failures, as well as identifying opportunities and limitations in the future application of exclusion barriers. We also provide some principles to optimise the use of AIS barriers, highlight where research on AIS is lacking, and suggest some avenues for future research.
Methods
Literature Search and Relevance Criteria
We followed a semi-systematic approach (Snyder, 2019) to compile literature on the use of AIS exclusion barriers. A quantitative literature search was conducted on Clarivate Analytics Web of Science (WoS), Google Scholar and ProQuest to identify empirical studies involving the use of barriers to control the spread of AIS. The search strings used (Table 1) were designed to capture all barrier types, cover all freshwater habitats, and consider all AIS taxa that could potentially be controlled using barriers (i.e., we relied on the literature searches to identify the taxa targeted rather than making a priori assumptions). The use of different search terms in different databases was designed to capture records missed due to synonyms. No date restrictions were imposed (searches performed on 14th August, 2020).
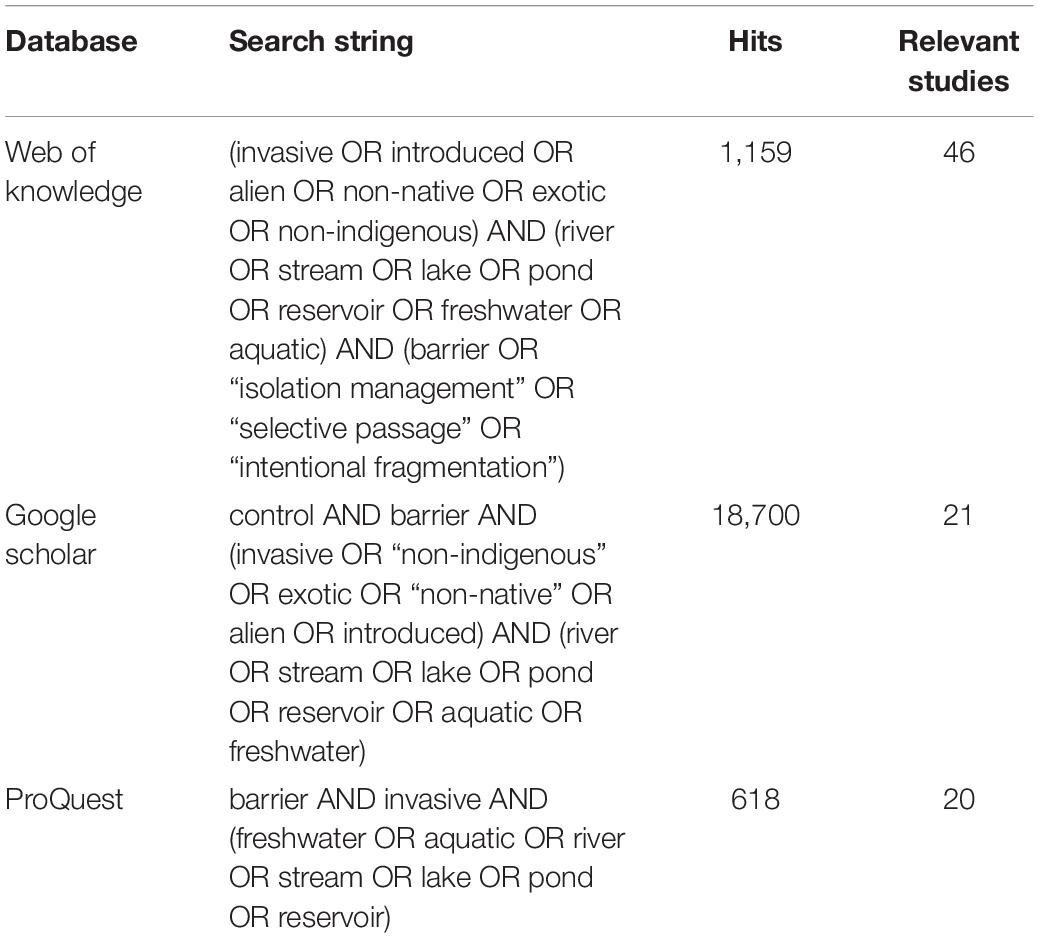
Table 1 | Search string used to find relevant literature in databases, along with number of hits and the relevant studies identified according to predetermined criteria.
All references returned were systematically checked by the lead author for relevance: i.e., involving the intentional use of barriers to control the dispersal of AIS. Papers documenting the effects of pre-existing structures not built specifically to control AIS were not considered (e.g., hydroelectric dams, waterfalls, etc.). Studies covering the use of traps, chemicals, and physiological conditions (temperature, desiccation, etc.) to control AIS were only included when specifically used to impede dispersal. All freshwater non-native animal taxa which could potentially be affected by barriers were considered. These included amphibians, crustaceans, fishes, insects, mammals, molluscs, and reptiles. No restrictions were placed on publication date or journal, and relevant “grey” literature (technical reports and academic theses) was included except where the same results were reported in a peer-reviewed publication. Review papers were excluded except where they reported new results, not published elsewhere. Further relevant studies were obtained by cross-linking references as cited in key studies (“snowballing”) and based on the authors’ knowledge of AIS exclusion barrier studies. This reduced the risk of missing relevant material that was not picked up using database searches. Eighty-two percent of relevant publications were identified using the search strings (Table 1), while the remaining 18% were added through “snowballing” and authors’ additions (Supplementary Table S1).
Processing and Analysis
Papers were categorised according to the barrier type used including: physical (dams/weirs, rock gabions, velocity barriers, exclusion screens, net barriers, and traps), electrical, chemical (pesticides, carbon dioxide, semiochemicals, substrate coatings, and anoxia), acoustic (bubble curtains, water guns, and other sound deterrents), light (e.g., strobe lighting), and temperature barriers. Habitat type where the barrier was used was recorded as river (including canals and floodplain wetlands), stream, lake/pond (including reservoirs), and laboratory (tank and flume experiments). Because information on discharge, stream order or channel width was often missing, streams were differentiated from rivers according to the terminology used by the study authors to describe the habitat type in each paper. The AIS taxa (amphibians, crustaceans, fish, molluscs, etc.), the AIS species, geographic location (country), and publication year were also recorded.
Experimental design is important for interpreting the robustness and implications of study findings so the approach taken by each was categorised as impact-only (I), control-impact (CI), before-after-impact (BAI) or before-after-control-impact (BACI). Study duration is also important to assess the long-term effectiveness of exclusion barriers, so the number of years sampling post-barrier implementation was recorded.
We assessed the effectiveness of exclusion barriers by extracting information on the percentage of AIS intercepted/deterred/killed (i.e., success rate), and compared success rate across AIS taxa, habitat type and barrier type. For these analyses, papers reporting the effects of multiple barriers designs/types, and effects on different species were treated as independent replicates. However, for laboratory experiments testing a range of treatments to identify an optimal barrier design, only the success rate of the best performing design was included.
Exclusion Barrier Research
Temporal Trends
A total of 109 studies were identified that involved the intentional use of barriers to prevent the dispersal of AIS (Supplementary Tables S1, S2), 81% of which were published in peer-reviewed journals. The first studies involving the use of barriers to control AIS were published in the early 1950s (Figure 1A), describing the use of physical, chemical, and electrical barriers to control sea lamprey (Petromyzon marinus), which had recently invaded the Laurentian Great Lakes of North America and negatively impacted fisheries (Applegate, 1950; Applegate and Smith, 1952; McLain et al., 1965). From the early 1970s, barriers were being tested in Sweden and Norway in an effort to slow the invasion of the invasive signal crayfish (Pacificasticus leniusculus) which was displacing native noble crayfish (Astacus astacus) due to competition and disease-transmission (Unestam et al., 1972; Håstein and Gladhaug, 1973). The use of exclusion barriers increased from the late 1990s, especially involving the use of physical barriers to protect local races and subspecies of cutthroat trout from the invasion of introduced salmonids (e.g., Buktenica, 1994; Thompson and Rahel, 1998). In 1999, electric barriers were installed in the Chicago Sanitary and Ship Canal in efforts to prevent several “Asian carp” species (Hypophthalmichthys nobilis and H. molitrix) in the Mississippi River from invading the Laurentian Great Lakes (Moy, 1999). Since 2005 there has been a surge in the number of publications with 81% of studies published since this date, and AIS exclusion barriers are now being used to target an increasingly wide range of species and habitats.
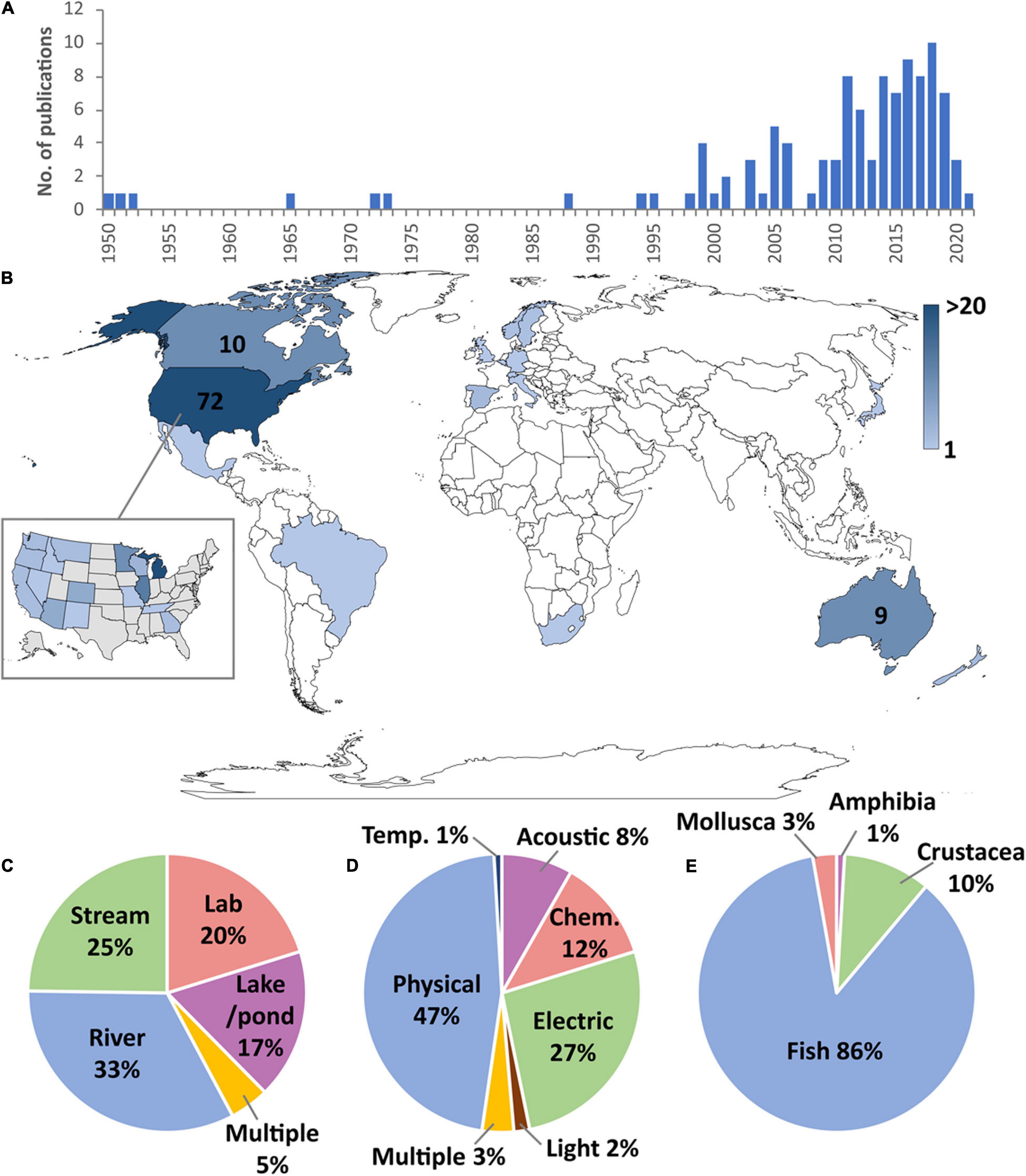
Figure 1. (A) Number of publications involving the use of exclusion barriers by year. (B) Number of publications per country (countries with more than five studies have publication numbers shown and inset shows distribution within the United States). (C) Proportion of studies focussing on different habitat types. (D) Percentage of studies by barrier type. (E) Percentage of studies by AIS taxa.
Global Research Effort
The use of exclusion barriers has been overwhelmingly concentrated in the United States (Figure 1B), accounting for 66% (72 studies) of studies globally. Research has focussed on the Laurentian Great Lakes area, primarily to reduce sea lamprey access to spawning habitat in tributary streams (e.g., Swink, 1999; Johnson et al., 2014; Miehls et al., 2017) and to prevent the intrusion of “Asian carp” species into Lake Michigan (e.g., Ruebush et al., 2012; Parker et al., 2015). The other main focus area within the United States has been in the western states where physical barriers have been installed to prevent non-native salmonids from reaching upstream areas that harbour endangered populations of native trout (e.g., Novinger and Rahel, 2003; Avenetti et al., 2006; Nehring et al., 2018). Canadian examples (10 studies) include the exclusion of common carp (Cyprinus carpio) from bays in Lake Manitoba in order to help restore the quality of marsh habitat (Caskenette et al., 2018). Other studies in Canada involved laboratory trials, including testing chemical alarm cues on sea lamprey (Perrault et al., 2014), and investigating the effects of a vertical electrical barrier on rainbow trout (Oncorhynchus mykiss; Kim and Mandrak, 2019) and common carp (Kim and Mandrak, 2017). Some field studies also assessed the impact of sea lamprey barriers on native fish movements (e.g., Pratt et al., 2009).
In Europe (11 studies), AIS exclusion barriers have largely targeted invasive crayfish (e.g., Dana et al., 2011; Frings et al., 2013; Kerr et al., 2020; Krieg et al., 2021). Apart from crayfish studies, vertical barriers have also been tested to limit the spread of European minnow (Phoxinus phoxinus) in Scandinavia (Holthe et al., 2005), an innovative trap has been designed to intercept Chinese mitten crab (Eriocheir sinensis) as they migrate through a fish pass in Belgium (Schoelynck et al., 2020), and electric barriers have been used to protect endangered populations of endemic Mediterranean trout (Salmo cettii) from introduced brown trout (Salmo trutta) in Italy (Sabatini et al., 2018).
Studies in Australia (nine studies) and New Zealand (three studies) have centred around the control of common carp using exclusion screens and traps (Hillyard et al., 2010; Taylor et al., 2012; Stuart and Conallin, 2018; Tempero et al., 2019). The augmentation of natural barriers is an important part of conservation efforts to protect native galaxiids from invasive salmonids (Lintermans, 2000; Franklin et al., 2018). The only study identified in South America was in Brazil, where atrazine (a herbicide) was used as a chemical barrier to contain invasive guppy (Poecilia reticulata; Araújo et al., 2018). One study has been undertaken in Asia, where pheromone traps were used to capture invasive largemouth bass (Micropterus salmoides) as they moved into spawning areas in a Japanese lake (Fujimoto et al., 2020). A single study was found in Africa, which used a purpose-built rock gabion to exclude spotted bass (Micropterus punctulatus) from the headwaters of a stream in South Africa (Van der Walt et al., 2019).
Habitats Targeted by AIS Exclusion Barriers
Rivers were the habitat type where AIS exclusion barriers have been most frequently used (33% of studies; Figure 1C). Dams and other physical structures are expensive to build and can cause unwanted hydrological alterations and ecological impacts so non-physical solutions such as bubble curtains (Zielinski and Sorensen, 2015), light deterrents (Ruebush et al., 2012), electric fields (Swink, 1999) and CO2 barriers (Cupp et al., 2018) have been favoured. Another widely used technique in rivers is to use exclusion screens and traps to prevent access to spawning habitat on floodplain habitats (Hillyard et al., 2010; Conallin et al., 2016).
Twenty five percent of studies targeted AIS in streams (Figure 1C). The relative simplicity and smaller scale of stream environments means construction of physical barriers is more straightforward, so rock gabions, culverts, and weirs are often the preferred method of AIS exclusion in streams (Buktenica, 1994; Thompson and Rahel, 1998; Avenetti et al., 2006). These are frequently implemented through augmenting existing natural waterfalls (e.g., Lintermans, 2000). Although construction of barriers in streams is not as technologically demanding and less costly compared to rivers, high flow events and bedload movements can still cause failures (Nehring et al., 2018).
Constructing barriers in lakes and ponds (17% of studies; Figure 1C) can be difficult and ecologically disruptive so barrier control efforts have often focussed on targeting access to shorelines, embayments and inlets to prevent spawning (French et al., 1999; Maceina et al., 1999; Caskenette et al., 2018). Laboratory experiments (20% of studies; Figure 1C) are useful for testing proof of concept (Thwaites et al., 2010; Zielinski et al., 2014; Kerr et al., 2020) but field studies are essential to evaluate their efficacy in situ.
Barrier Types
Physical barriers, including weirs, culverts, rock gabions, velocity barriers, traps, and exclusion screens, accounted for 47% of studies (Figure 1D). Weirs and culverts work by targeting the jumping/climbing/swimming ability of AIS versus native species to prevent upstream movement. For example, in Iberia, vertical barriers of 0.8 m were 100% effective in blocking goldfish (Carassius auratus) but allowed passage of >95% of native barbel (Luciobarbus sp.; Morán-López and Tolosa, 2017). Lipped weirs have been used to prevent upstream passage of non-leaping sea lamprey in the Laurentian Great Lakes, while allowing passage of jumping salmonids, but unfortunately they block many other non-jumping native fishes (Pratt et al., 2009). Culverts can effectively prevent upstream movement of invasive salmonids (Thompson and Rahel, 1998) but likely also isolate native fish populations. Rock gabions also work by excluding fish with poor jumping ability, although interstitial spaces sometimes allow passage of smaller bodied individuals which can result in ineffective exclusion of AIS (Thompson and Rahel, 1998). Velocity barriers (e.g., sloping weirs that create localised high current velocity zones) can be useful in preventing movement of AIS where they lack the locomotive ability to move upstream, although their efficiency has only been tested under laboratory conditions thus far (Frings et al., 2013; Kerr et al., 2020). Physical traps have been integrated with fish passes and function by capturing jumping fish only (Stuart et al., 2006) or by intercepting crawling crustaceans (Schoelynck et al., 2020). The other type of physical barrier which has been widely used are exclusion screens and nets, which aim to intercept larger-bodied invasive fish, although they are generally ineffective for early life stages (Martinez and Foreman, 2001; Hillyard et al., 2010; Tempero et al., 2019). An advantage of physical barriers is that they tend to last longer term (multiple decades), although traps and screens require regular maintenance (Daniel et al., 2014). The majority of physical barriers are designed to prevent upstream passage only, with the exception of some bidirectional exclusion screens and traps (e.g., Tempero et al., 2019; Schoelynck et al., 2020).
Electric barriers were the second most widely studied type of barrier (27%; Figure 1D). Electric fields are used to deter, incapacitate, or kill AIS attempting to pass (depending on voltage and frequency settings, body size, and how far AIS penetrate the electric field). They can be highly effective in blocking some AIS (Savino et al., 2001; Holliman et al., 2015; Layhee et al., 2016), but steel-hulled boats can temporarily disrupt electric fields (Parker et al., 2015), and power outages can be an issue, particularly where AIS accumulate below electric barriers (Parker et al., 2016). For example, despite only suffering 0.001% downtime over a 12-year period (due to lightning strikes, power failures, improper maintenance, and equipment malfunction), an electric barrier on the Gila River (Arizona) was breached by several AIS (Clarkson, 2004). The need for a constant power supply can also prohibit the use of electric barriers in remote locations.
A wide range of chemical barriers (12% of studies; Figure 1D) have been used. Perhaps the simplest type of chemical barrier is the use of pesticides to block AIS from entering target areas (Araújo et al., 2018). While some pesticides are taxon-specific and can be quite effective, others can have negative impacts on the wider ecosystem (e.g., Birceanu et al., 2014). The use of chemosensory alarm cues may present a low cost, non-toxic alternative (Wagner et al., 2011) but their utility for blocking AIS upstream movement has rarely been evaluated in the field, and may not provide the necessary restriction of movement (e.g., Luhring et al., 2016). Using copper as a contact-deterrent substrate barrier shows promise in its ability to block the spread of the invasive New Zealand mudsnail (Potamopyrgus antipodarum; Hoyer and Myrick, 2012). Carbon dioxide barriers can reduce invasive fish passage and can be useful because they do not interfere with shipping and river flow (Cupp et al., 2018). The use of pheromones to increase efficiency of barrier-integrated traps to intercept AIS has also been trialled (e.g., sex pheromones alone, Fujimoto et al., 2020; or in combination with an alarm cue, Hume et al., 2015). At sufficient concentrations, ozone can kill as wide range of AIS, and a recent review suggested its potential use as an AIS exclusion barrier (Buley et al., 2017), though its effectiveness is unproven.
Studies evaluating acoustic barriers (8%; Figure 1D) generally involved the use of bubble curtains as a behavioural deterrent (Taylor et al., 2005; Zielinski et al., 2014; Zielinski and Sorensen, 2015), although broadband sound in water guns has also been tested (Romine et al., 2015). Light barriers (2%; Figure 1D) have involved the use of strobe lights to deter passage of invasive fish, with limited success (Miehls et al., 2017). The use of barriers incorporating multiple deterrents have only rarely been used (3% of studies) to control AIS spread (e.g., Altenritter et al., 2019).
AIS Targeted by Exclusion Barriers
Research investigating the use of barriers to control AIS spread has overwhelmingly focussed on fish (86% of studies; Figure 1E) using a wide range of barrier types (physical, electric, acoustic, chemical, and light). Sea lamprey was the most studied species (22 studies), followed by “Asian carp,” especially silver carp and bighead carp (H. molitrix and H. nobilis; 20 studies), and common carp (19 studies). Invasive salmonids have been the focus of 14 studies: brook trout (Salvelinus fontinalis; 5 studies), rainbow trout (6 studies), and brown trout (3 studies). Fish may have received a relatively large amount of research attention because they are generally completely restricted to underwater movement and can therefore be targeted more easily using barriers than some other groups which can move overland.
After fishes, crustaceans were the next most studied group (10% of studies), principally focussed on signal crayfish (6 studies), but also red swamp crayfish (Procambarus clarkii; 1 study), Chinese mitten crab (1 study), and exotic-disease-infected native crayfish (2 studies). However, there is some evidence that invasive crustaceans can disperse terrestrially, or at least in damp areas (Thomas et al., 2019), and thereby bypass instream obstacles. Also, most studies have not been of sufficient duration to assess the long-term effectiveness of crustacean barriers.
Three studies were identified that involved the use of barriers to control invasive molluscs: metallic substrates that deterred the dispersal of a gastropod (Hoyer and Myrick, 2012), thermal treatments (hot water immersion) can kill adhered zebra mussels (Dreissena polymorpha) and quagga mussels (Dreissena rostriformis bugensis) on boat hulls as they pass through canal locks (Beyer et al., 2011), and barrier matts that created anoxic conditions to prevent movements of Asian clam (Corbicula fluminea; Wittmann et al., 2012). Only one study investigated the use of barriers to control the spread of amphibians: plastic screens were erected around a pond in Bermuda, effectively preventing invasive cane toad (Rhinella marina) from breeding, resulting in their eradication from an island (Wingate, 2011). We did not find any studies that used barriers to exclude invasive reptiles (aquatic snakes, turtles, crocodilians, etc.) or mammals, probably due to their propensity for overland dispersal and the fact that most are lake or wetland species, occupying large areas of flooded or open-water habitat.
Monitoring
Sixty-six percent of studies employed an impact-only (no control) approach, 18% a control-impact method, 11% were before-after-impact studies, while only 5% followed a before-after control-impact (BACI) design (Figure 2A). BACI approaches (sampling before and after interventions, while also sampling a biologically relevant control system) are optimal for assessing the ecological effects of mitigation works (Smith et al., 1993; Martin et al., 2012). While running BACI experiments can be resource intensive, they are preferential because they have greater statistical power; less robust experimental designs are more likely to risk missing or misinterpreting important AIS barrier effects.
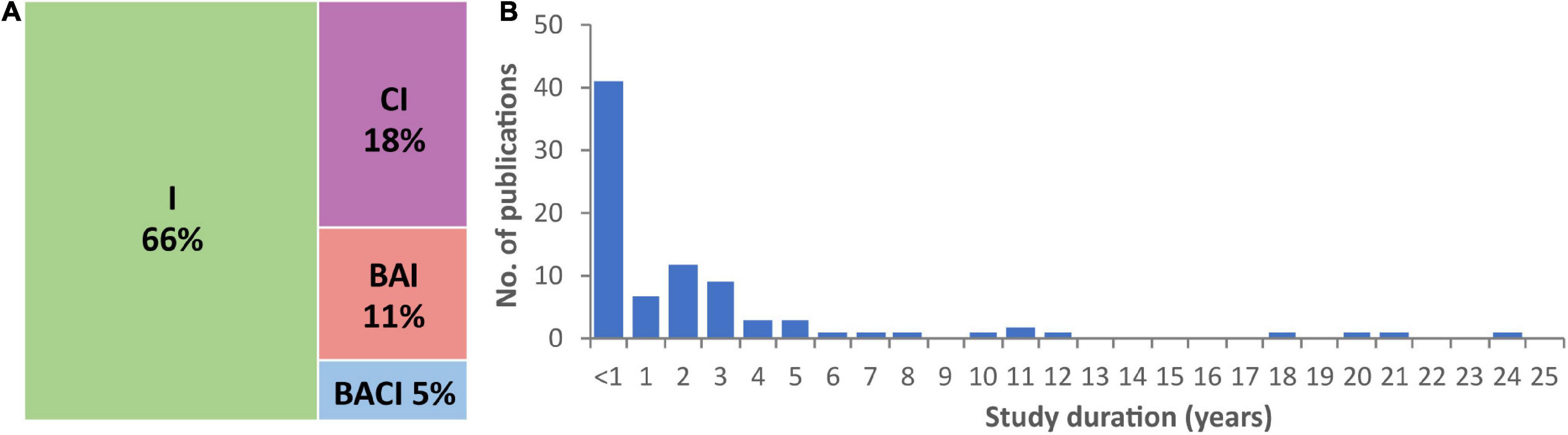
Figure 2. (A) Frequency (%) of exclusion barrier studies reviewed by experimental design. I, impact only; CI, control impact; BAI, before after impact; BACI, before after control impact. (B) Duration of exclusion barrier studies.
Most studies examining the effectiveness of barriers for controlling AIS were of short duration (Figure 2B): 47% tested barrier efficacy for <1 year and 87% for ≤5 years. While short term experiments can provide useful insights, long term studies are needed to evaluate the effectiveness of AIS barriers under a broader range of conditions. For example, physical barriers can fail during high flow events (Nehring et al., 2018), some taxa can disperse terrestrially (Thomas et al., 2019), electrical barriers can suffer power-outages (Clarkson, 2004), and AIS can become habituated to behavioural deterrents (Vetter et al., 2015) as well as chemical barriers (Schneider et al., 2018). Promising results from short term studies need to be treated with caution. For example, big decreases in common carp biomass were observed over 3 years following the installation of a selective barrier in a lake in New Zealand, but biomass increased to above pre-intervention levels in the following 2 years (Tempero et al., 2019). However, some of the longer-term studies do show promise. For example, a 21-year study showed a tenfold increase in bull trout (Salvelinus confluentus) abundance in response to AIS removal and isolation management (Buktenica et al., 2013), and a selective trap remained successful at removing common carp over an 11-year period (Stuart and Conallin, 2018).
Effectiveness of Barriers in Controlling AIS
Overall, exclusion barrier studies have been largely successful in reducing the spread of AIS (Figure 3A). Of the 92 studies reporting success rates, 33 (36%) excluded 100% of individuals attempting to pass the barrier, and a further 50% of studies reported 70–99% exclusion rates. While it could be argued that a success rate less than 100% equates to failure, substantially reducing the number of AIS dispersing can make control by other methods more feasible and reduce the cost of management. Conversely, partial removal of AIS can result in increased population growth rates through compensatory mechanisms, as occurred for common carp at Delta Marsh, Lake Manitoba, Canada (Caskenette et al., 2018), and has also been reported for zebra mussel in Wales (Rolla et al., 2020). Only 10% of studies reported a success rate of less than 50%, although this could potentially be a result of publication bias against unsuccessful barriers.
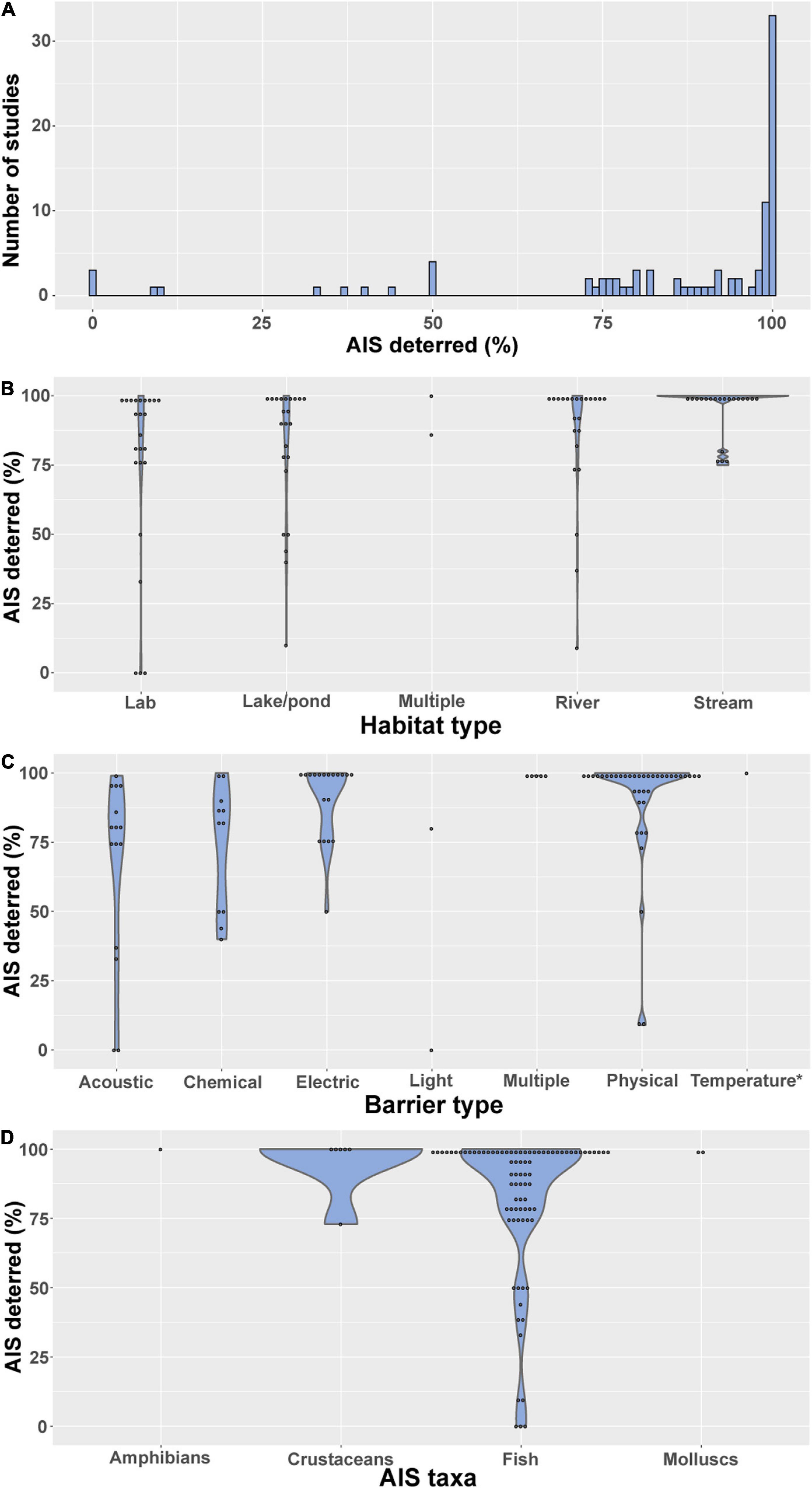
Figure 3. Success rate (percentage of AIS individuals excluded) by (A) number of studies, (B) habitat type, (C) barrier type, (D) AIS taxa.
Success rate differed between habitat type (Figure 3B) and barrier type (Figure 3C), but there was little evidence that success rate differed markedly between AIS taxa targeted (Figure 3D). Exclusion barriers tended to show higher success rates in streams compared to lakes/ponds (Figure 3B), likely due to the relative simplicity of installing barriers in streams. Barriers tested under laboratory conditions had relatively low success rates compared to those used in streams (Figure 3B), perhaps because the former were mainly testing novel barrier designs. Physical and electric barriers showed markedly higher success rates than both acoustic and chemical barriers (Figure 3C). However, acoustic and chemical cues are generally used with the aim of allowing selective passage, as opposed to physical and electric barriers which are often non-selective, so it is perhaps unreasonable to make direct comparisons. It was difficult to compare success rate between AIS taxa due to the lack of studies for all groups except fish (Figure 3D).
Effects of AIS Exclusion Barriers on Native Fauna
A substantial number of barrier interventions originate for specific conservation projects of target species, in particular taxa such as galaxids, cutthroat trout and native crayfishes. However, barriers to AIS often also impact the movements of many other native non-target species. Only 25% of studies assessed the effects of AIS barriers on the dispersal of native fauna, indicating that the term “selective fragmentation” (Rahel and McLaughlin, 2018) is rarely accurate. Physical barriers such as weirs and rock gabions often allow downstream passage but generally prohibit upstream movement, and therefore tend to isolate native species upstream (e.g., Novinger and Rahel, 2003; Buktenica et al., 2013). Isolating populations makes them more vulnerable to extinction due to inbreeding depression and stochastic environmental events (Hilderbrand and Kershner, 2000; Fagan, 2002). Vertical barriers have been designed that can successfully allow passage of native fish by exploiting the inferior leaping behaviour of invasive species (Holthe et al., 2005; Morán-López and Tolosa, 2017). For example, low-head lipped weirs can prevent movements of non-jumping sea lamprey while allowing passage of recreationally important salmonids, but unfortunately, they also impede the upstream movement of many small-bodied native fish (Porto et al., 1999; Dodd et al., 2003). Velocity barriers that block invasive crayfish but allow passage of fish have also been designed (Frings et al., 2013; Kerr et al., 2020; Krieg et al., 2021) but their effect on native species movement has not been robustly tested in the field. Exclusion screens are often designed to prevent passage of larger bodied invasive species but allow free movement of smaller-bodied native species. However, at the moment these are ineffective at preventing the movement of early life stages of AIS so their long-term effectiveness is questionable (Tempero et al., 2019).
Electric barriers are generally non-selective and, depending on settings, can result in behavioural deterrence, incapacitation, injury and sometimes even mortality of any native organism attempting to pass (McLain et al., 1965). However, electricity effects do vary with body size so there is potential to provide selective passage by varying voltage and frequency (Layhee et al., 2016) but reducing settings too far will inevitably reduce their effectiveness for blocking AIS (Parker et al., 2015). Chemical barriers are often more target-specific. For example, pheromones can be used to guide target AIS into traps which have no effect on non-target species (Johnson et al., 2009; Hume et al., 2015). However, the use of broad-spectrum toxic chemicals (Araújo et al., 2018) will inevitably affect a wide range of species, and broad physiological effects of carbon dioxide (Cupp et al., 2018) or temperature (Beyer et al., 2011) barriers likely mean these are of limited use for providing selective passage.
Some success has been observed with the use of acoustic bubble curtains to target the relatively specialised hearing system of invasive carp relative to the wider community (Zielinski et al., 2014; Zielinski and Sorensen, 2016), but again these studies did not directly quantify effects on native species. Indeed, where the effects of acoustic deterrents on native species have been robustly evaluated, the impact was more severe than on the target AIS taxa (Rivera et al., 2018). No studies evaluating the use of light to deter AIS also assessed the impact on native species (Ruebush et al., 2012; Stamplecoskie et al., 2012; Miehls et al., 2017). Zielinski et al. (2020) recently proposed a fish pass solution incorporating a sorting process to selectively target traits of non-native fish to impede passage, while promoting passage of native species. However, the effectiveness of this design in providing selective passage has not yet been tested.
Global Case Studies
Africa: Controlling Invasive Fish Using Barrier Enhancement and Piscicide
Africa has experienced many invasive fish introductions, particularly among former British colonies (De Moor and Bruton, 1988; Weyl et al., 2017). The country of first arrival for many of these species was South Africa, which today is one of the world’s fish invasion hotspots (Leprieur et al., 2008b). Management of invasive fish within South Africa has pivoted over the last 40 years from promoting their spread, to attempting to control invasive populations through environmental legislation (Woodford et al., 2017). Due to limited capacity within conservation agencies both in South Africa and elsewhere on the continent, there have to date been only two successful operations of invasive fish control using barriers in African rivers, only one of which employed a purpose-built barrier. Both interventions took place in the Olifants-Doring catchment (Western Cape Province, South Africa), a hotspot for threatened endemic freshwater stream fishes (Weyl et al., 2014). The catchment is invaded by North American black basses (Micropterus spp.) in 81% of its reaches, with native fish confined to headwaters above natural barriers to bass invasion (Van der Walt et al., 2016).
In the first operation, on the Rondegat River, a 4 km reach invaded by smallmouth bass (M. dolomieu) was confined between a natural upstream barrier (a waterfall) and a pre-existing water-abstraction weir (Supplementary Figure S1). In 2012 and 2013, the invaded reach between the barriers was treated with rotenone to eradicate the bass, with potassium permanganate being used to neutralise the piscicide downstream of the weir, as the river drained into a reservoir with an economically important bass fishery (Weyl et al., 2014). The abstraction weir was further augmented with basket gabions to create a permanent barrier to re-invasion by bass from the reservoir. Post-treatment monitoring of the fish and macroinvertebrates revealed rapid re-colonisation of the treated reach by native fauna, with no evidence of reinvasion by bass (Weyl et al., 2014; Bellingan et al., 2019).
Following the success of the Rondegat River rehabilitation, a smaller-scale operation was undertaken in the nearby Thee River, where a new rock gabion weir was constructed to exclude spotted bass, and manual removal techniques deployed to remove the bass upstream of the weir. This project experienced several setbacks and reinvasions, but after 3 years of non-detection of bass and the recolonisation of the managed reach by native cyprinids and galaxiids, the eradication was declared a success (Van der Walt et al., 2019).
Moving into the future, the success of the Rondegat and Thee projects demonstrates that physical barriers could be exceptionally effective tools for native fish conservation in other South African rivers, if used as part of an integrated alien species eradication programme. A critical finding of research on black bass invasions, which are by far the most damaging to native biodiversity in South African streams (Ellender and Weyl, 2014), is that a vertical barrier of only 0.5 m is sufficient to block upstream migration (Van der Walt et al., 2016). The provincial implementing agency responsible for the Rondegat and Thee project (CapeNature) plans to rehabilitate reaches of other rivers within the Western Cape Province through a combination of piscicides and either new or enhanced artificial barriers. Such projects could provide critical conservation interventions for threatened fishes like the Twee River redfin (Sedercypris erubescens) and several endemic lineages of Galaxias, which today persist in mountain stream refugia above bass barriers that represent a tiny fraction of their historic range (Ellender et al., 2017). Despite the potential for such barrier-assisted interventions to result in major conservation gains, on-going funding and human capacity challenges within provincial government remain major constraints to future success in alien fish management, both in South Africa and across the continent at large (Woodford et al., 2017; Weyl et al., 2017).
South America: In Dire Need of AIS Control
The use of barriers as a management tactic to exclude non-native species or to prevent their further dispersal is rarely assessed in South America. Research on non-native species is still dominated by species records and descriptive patterns, at least for Brazil (Frehse et al., 2016). The few restricted examples of barriers to dispersal include non-intentionally designed structures such as road culverts that potentially halt the widespread invasion of exotic salmonids into areas where they threaten native galaxiid fishes (Garcia de Leaniz et al., 2010), and dams without structures allowing for the upstream passage of fish (e.g., Garrone-Neto et al., 2014).
Specific cases of threatened native species are currently being examined from the perspective of using barriers to prevent the spread of non-native species posing a major threat. In Argentina, the naked characin (Gymnocharacinus bergii), which is classified as critically endangered by the IUCN (Cussac et al., 2019), has the potential to benefit greatly from the implementation of barriers preventing non-native salmonid predators from reaching their upstream habitats. However, such barriers are not yet in use.
Rivers and streams are increasingly being transformed by dams and reservoirs, which have contributed to the spread of several non-native species. For example, the invasive golden mussel (Limnoperna fortunei), probably benefited from the large number of dams and reservoirs in the region, which act as steppingstones and seed areas for subsequent range expansion (Oliveira et al., 2015). Disrupting natural drainage patterns can also lead to unwanted dispersal. For example, in response to increasing water demand, the Brazilian government intends to divert water from the Tocantins River (Amazon River Basin) to the Preto River (São Francisco River Basin). This inter-basin connection will lead to the exchange of several aquatic non-native species, potentially causing the extinction of threatened native species, leading to taxonomic homogenisation (Daga et al., 2020). Furthermore, dam construction often comes with the removal of natural barriers and so contributes to the dispersal of non-native species, ultimately leading to the homogenisation of biotas (Vitule et al., 2012; Skóra et al., 2015; Santos et al., 2019; Daga et al., 2020).
In Brazil, the introduction of non-native species for recreational angling is often encouraged by sports fishermen and even state agencies (Vitule et al., 2009; Pelicice et al., 2017; Brito et al., 2020). A good example is the Brazilian tiger fish (Salminus basiliensis; Supplementary Figure S2), which is a powerful predator in Neotropical rivers and a flagship species, threatened in its native ranges. This has led to confounding practices that have promoted its introduction in other regions where it has been causing severe impacts (Daga et al., 2016). For instance, without the translocations by sport fishermen, this fish would never be able to pass the Iguaçu Falls (Supplementary Figure S2), and it has now invaded much of the Iguaçu basin (Vitule et al., 2014; Daga et al., 2016). Evidence of fish introductions in nearly 60 reservoirs indicate that fish farming and sport fishing are major contributors to unwanted dispersal. This activity, together with other vectors, such as the release of ornamental species, use of invasive species for fishing bait, and fish stocking, will predictably reduce the effectiveness of AIS exclusion barriers. Hence, any future use of barriers to control non-native species in South America must be accompanied by intensive environmental education and robust enforcement of legislation.
Europe: Managing the Movement of Invasive Crayfish With Physical Barriers
Crayfish barriers have been installed in several regions across Europe: the River Buåa at the Norway-Sweden border (Johnsen et al., 2008); mountain streams of southern Spain (three barriers, Dana et al., 2011); the Clyde in Scotland (two barriers at a single location, C. Bean pers. comm.); the Pont, England (two barriers at separate locations); and a variety of barriers have recently been tested in Switzerland (Krieg et al., 2021). The Spanish barriers were for red swamp crayfish, while the remainder were for signal crayfish. However, their effectiveness remains questionable as crayfish managed to bypass the barrier in the Buåa (Johnsen et al., 2008) and both barriers in the Pont were damaged severely by high flows within 9 months of deployment. Barrier efficiency in the Clyde remains unknown due to a lack of systematic study, although the paired barrier design provides an option for crayfish extermination between the barriers if only the lower one is ascended. In Spain, vertical 1.5–2 m barriers, which incorporate overhanging crests, a central V-notch and vertical wing walls, have prevented red swamp crayfish invasion upstream (Dana et al., 2011) but they inevitably impact connectivity for native biota. The Swiss study reported some success in preventing the upstream movement of signal crayfish in a variety of natural and artificial channels, but the various designs were not tested for long enough, with study duration varying from hours to a maximum of 3 months (Krieg et al., 2021).
Ideally, barriers for non-native decapods should be selective, allowing native biota (especially fish) passage, but this has proved difficult, with evidence that conventional fish ladders can facilitate invasive crayfish dispersal upstream past barriers (Welsh and Loughman, 2015). Frings et al. (2013) found that signal crayfish could pass barrier slopes up to 48° provided there was sufficient roughness. Kerr et al. (2020) found velocity barriers created by a Crump weir in a flume tank prevented the passage of signal crayfish under conditions that allowed some passage of native fishes, but no field trials have been undertaken yet. It is also possible that invasive crayfish can bypass in-stream structures altogether by moving overland (Thomas et al., 2019), although field studies do indicate in-stream barriers can limit spread (Supplementary Figure S3; Bubb, 2004; Robinson et al., 2019) and the addition of terrestrial barriers may help prevent overland movement (Krieg et al., 2021). In summary, it is possible that smooth-surfaced, lipped weirs at sites with steep artificial wing walls, that are never submerged, will minimise upstream passage of invasive decapods, but these conditions will also likely prevent passage of stream fish biota, especially small benthic species (Tummers et al., 2016).
New Zealand: Competing Interests in Controlling Introduced Salmonids
Introduced salmonids (mainly brown trout and rainbow trout) have established in almost all New Zealand rivers and streams with suitable habitat (Jones and Closs, 2018), supporting a world-renowned recreational fishery. However, this colonisation has resulted in the widespread decline of native fish, especially several endemic species of non-migratory galaxiids. Trout prey on all size classes of galaxiid, as well as competing aggressively for food and space (McDowall, 2006; Supplementary Figure S4).
Largely because of pressures from trout, most non-migratory galaxiids are restricted to areas upstream of natural waterfalls. In a small number of cases, mitigation works have been undertaken to remove salmonids from invaded reaches and install barriers to prevent reinvasion (Franklin et al., 2018). This has generally involved augmenting existing waterfalls with weirs or culverts to prevent trout from recolonising during high flow events (Supplementary Figure S4). These projects have largely been successful in protecting galaxiid populations within isolated reaches, although galaxiid population sizes are often very small, and the long-term effects of isolation are unknown.
Unfortunately, the aims of galaxiid conservation efforts often conflict with those of fisheries managers. New Zealand Fish & Game, the organisation responsible for managing sports fisheries, are reluctant to support trout removal projects even in very small streams, even though they are usually of little recreational value (Jones and Closs, 2018). The Department of Conservation, which is responsible for protecting galaxiids, has been constrained by a lack of effective legislation. Ironically, until recently, the only native fish species unequivocally protected by legislation was extinct, while trout and salmon were specifically protected by New Zealand law (McDowall, 2006). This conflict between recreational fisheries and conservation goals has hampered restoration efforts and contributed to the decline of the native fauna. However, the 2019 Indigenous Freshwater Fish Bill now affords native fish greater protection and will hopefully pave the way to more robust conservation management.
North America: Sea Lamprey and the “Connectivity Conundrum”
Sea lamprey control employs physical barriers to concentrate larvae in the lower sections of Great Lakes tributaries by blocking upstream movement of adults. Larvae can then be effectively and efficiently targeted with pesticides, and without the presence of barriers the cost of sea lamprey control in the Laurentian Great Lakes would rapidly become untenable (Hume et al., 2020a). Of the 1,000 downstream-most barriers present in tributaries, only 77 (8%) were constructed or subsequently adapted to block sea lamprey (Zielinski et al., 2019) while the rest were originally built for other purposes (Moody et al., 2017). The most common barriers employed to block sea lamprey upstream movement are of fixed-crest designs, and those with an overhanging lip and just 30 cm differential between the barrier crest and surface of the tail water are highly effective because sea lamprey do not jump (Zielinski et al., 2019). However, these barriers also block native and desirable fish species, particularly those with limited jumping capacity (Porto et al., 1999; Dodd et al., 2003; McLaughlin et al., 2006). Some barriers have an adjustable crest or are operated seasonally, and this permits passage of fishes that do not overlap with sea lamprey migration timing (Vélez-Espino et al., 2011). But in reality, adjustable and seasonally operated barriers are fraught with uncertainty regarding migration phenology (Klingler et al., 2003). Other barrier types have been tested in a limited capacity in regards to blocking sea lamprey upstream movement, but with the exception of a single combined electrical-fixed crest weir barrier, none have been implemented at the management scale (Zielinski et al., 2019).
The need for barriers to restrict sea lamprey spread creates tension among stakeholders who differentially value sea lamprey control and passage of native and desirable fish (McLaughlin et al., 2013); recently characterized as the “connectivity conundrum” (Zielinski et al., 2020). Many factors must be considered in sea lamprey barrier removal or mitigation decisions (e.g., barrier age, public safety, costs, and ownership) and the process requires consideration of multiple, often conflicting, value sets and objectives (McLaughlin et al., 2013; Jensen and Jones, 2018; Lin and Robinson, 2019). Yet this tension is proving to be fertile ground for developing and testing novel approaches to AIS control that improves existing barrier function, and could permit selective passage1. For example, applications of sea lamprey semiochemicals could be used to guide adults toward barrier-integrated traps (Hume et al., 2015) or intercept them prior to or following barrier encounter (Hume et al., 2020c). Exploiting sea lamprey response to semiochemicals and their behavioural tendencies at barriers could also be employed to encourage climbing of studded ramps when upstream progress is halted (Hume et al., 2020b). Combining engineered solutions with behaviourally-relevant considerations may yet help solve the connectivity conundrum.
Asia: A Long Way to Go
A wide range of AIS have been introduced to Asian countries, particularly cyprinids, catfishes, cichlids, snakeheads, and salmonids, many of which have established self-sustaining populations (Galib and Mohsin, 2010; Luo et al., 2019). Introductions have mainly occurred due to both intentional and accidental releases from the aquaculture and ornamental trades (Knight, 2010; Xiong et al., 2015). Despite the presence of so many non-native species, evidence of severe impacts on native communities is scarce (De Silva et al., 2006). Although there are reports of hybridisation issues (Li et al., 2008) and habitat alteration (Chen, 1989) associated with AIS, insufficient research has been undertaken to evaluate AIS impacts in Asia. In most Asian countries, particularly in the south and east, the emphasis is on increased fish production through non-native aquaculture and fisheries, and there is generally little concern about the adverse impacts of AIS on native ecosystems. This may explain why control measures have rarely been considered, with only one record of AIS exclusion barriers being used in Asia (Fujimoto et al., 2020). Moreover, complex interconnecting water networks in many Asian countries (Galib et al., 2018), especially during the monsoon season, are extremely challenging environments for barrier implementation. Government bans on aquaculture of a few non-native species have been imposed in some countries (e.g., African catfish Clarias gariepinus in Bangladesh, S. Galib, pers. obs.; tilapias in Thailand, Tomojiri et al., 2019) but the use of barriers to control AIS in this region seems unlikely in the near future.
Important Considerations in the Application of AIS Exclusion Barriers
Management of AIS cannot be achieved by exclusion barriers alone, it requires a coordinated approach and integrated management. This includes effective policy, education and enforcement to prevent the release of non-native species outside their natural range; predictive tools to classify and rank those species at greatest risk of becoming invaders; surveillance to identify invasions prior to establishment, and effective management measures to eradicate or control AIS once established (Koehn and MacKenzie, 2004; Van der Zanden and Olden, 2008). The policy context is key to the effective control of AIS, and governments worldwide need to fully address the threat posed by AIS and take appropriate action to reduce their impacts. Exclusion barriers have a role to play once AIS have become established, either by limiting secondary spread, especially upstream, or impeding reinvasion of sections of habitat containing focal native species (Fausch et al., 2009; Neeson et al., 2015).
The landscape context is a crucial consideration in the application of AIS exclusion barriers. Where existing barriers (typically physical ones such as dams and weirs) occur within an invaded catchment, the distribution, type and permeability of those barriers provides a tool for the management of within-catchment invasion (Rahel and McLaughlin, 2018). Almost all river fauna have one or more life stages which facilitate downstream movement, often by way of passive drift in water currents, and contribute to dispersal and recolonisation (Lechner et al., 2014). Thus, the use of existing barriers to manage AIS is mostly limited to inhibiting their spread upstream. A GIS framework of the spatial distribution and characteristics of existing barriers may be developed to assess management options within a catchment in combination with barrier network algorithms (King and O’Hanley, 2016) so that the spread of AIS may be mapped and limited adequately (Dana et al., 2011; Clarkson et al., 2012).
A key challenge for the effective use of exclusion barriers is identifying a suitable location for barrier placement. It is often difficult to pinpoint the exact location of an invasion front because individuals at the invasion front are present at low-density and are therefore difficult to detect. In recent years environmental DNA (eDNA) has started to be used as an AIS monitoring tool due to its high sensitivity, non-invasive sampling, and cost effectiveness (Herder et al., 2014; Clusa and García-Vázquez, 2018; Geerts et al., 2018). The method is based on the detection of species from extracellular DNA, or cell debris, that species leave behind in the environment (Valentini et al., 2009). It can be very useful for detecting the presence of low-density AIS. For example, “Asian carp” (Hypopthalmichthys spp.) were detected in the immediate vicinity of Lake Michigan using eDNA, and it subsequently took 93 person-days of electric fishing to manually confirm their presence (Jerde et al., 2011). Reliably locating invasion fronts should help river managers use AIS barriers more effectively. Environmental DNA also represents a useful tool to monitor AIS barrier efficiency (Robinson et al., 2019).
Funding for conservation is always limited, especially in developing countries, so the cost of exclusion barriers will inevitably limit their widespread implementation. Some small-scale barriers can be fairly inexpensive; for example, US$3,200 for a carp exclusion barrier (Daniel et al., 2014), a portable trapping system for sea lamprey cost US$4,800 (Johnson et al., 2016), a bubble curtain US$5,000 (Zielinski and Sorensen, 2015), and a crayfish barrier US$6,500 (Mouser et al., 2019). However, costs depend very much on scale and complexity; for example, a large concrete barrier to protect Apache trout (Oncorhynchus apache) in Arizona cost US$3 million (Avenetti et al., 2006) and one of the electric array systems in the Chicago Shipping and Sanitary Canal had built costs in excess of US$9 million (Shea and Dettmers, 2009). These figures do not include maintenance and monitoring costs which can also be considerable (Clarkson, 2004). However, the economic benefits of AIS control can significantly outweigh the costs. For example, Sturtevant and Cangelosi (2000) estimated that the sea lamprey control programs brought a net economic benefit of US$2–4 billion per year to the Laurentian Great Lakes region.
Finally AIS autecology needs to be taken into account, both in terms of predicting their future distributions and their interactions with native species and environments. The AIS impacts on native species and environments need to be balanced against any potential isolation impacts exclusion barriers may have on native species. Where barriers are constructed, novel habitat may be created, which can create colonisation opportunities for other AIS, and river modifications can create new invasion pathways (Johnson et al., 2008; Liew et al., 2016). Also, non-native species are often deliberately introduced to dam impoundments for aquaculture, or commercial or recreational fisheries (Gozlan et al., 2010). A careful approach should therefore be taken by freshwater managers when the decision is made to construct barriers to ensure they do not facilitate the spread of AIS.
Conclusions and Future Development of Barrier Management for AIS Control
The intentional use of exclusion barriers to prevent or reduce passage of AIS is gaining attention, with over 80% of relevant studies published in the last 15 years. While the implementation of AIS barriers holds worldwide significance, two-thirds of existing studies have been undertaken in the United States (and these were concentrated in the Laurentian Great Lakes region and western states), so results from a greater range of geographical conditions are needed. Fishes have been the focus of the vast majority of studies, perhaps because they are the group most effectively targeted using barriers, though there are examples of barriers being used to successfully control crustaceans, molluscs and amphibians, suggesting there may be some promise in using exclusion barriers to target other AIS taxa. There are instances where exclusion barriers have been applied successfully in lentic waterbodies, as well as lotic habitats, demonstrating their potential utility across a range of environments.
Overall, most of the studies report some success in the use of exclusion barriers for controlling the spread of AIS in the short term, with a mean success rate of 84%. However, few studies have lasted long enough, or have followed a rigorous experimental design, to demonstrate such benefits conclusively. Long term studies with more robust experimental designs (i.e., BACI) are sorely needed to test whether exclusion barriers are an effective long-term management tool in the conservation of native fauna. It is also probable that there has been a publication bias against unsuccessful studies and this information is important for those conceptualising barrier designs.
The impact of AIS exclusion barriers on native fauna has rarely been evaluated, with less than a quarter of studies investigating passage rates of native species. Where barriers prevent dispersal of native species, the size of isolated populations needs to be considered very carefully or populations will experience gradual decline (Muhlfeld et al., 2012). For example, Hilderbrand and Kershner (2000) estimated that over 25 km of stream length would be needed to support the long-term persistence of a low abundance population of cutthroat trout. Minimum viable population sizes have rarely been considered when implementing exclusion barriers, which have often been used as a last-ditch effort to protect populations from extinction. Further research is required to establish minimum viable population sizes for a greater range of native species to better inform the long-term effectiveness of exclusion barriers.
Avoiding the harmful effects of isolation requires that exclusion barriers be species-selective. There is some promise in targeting traits of AIS compared to native communities. For example, differences in jumping behaviour, swimming capacity, body size, hearing sensitivity, and response to chemical cues have all been exploited in the design of selective barriers. However, testing on native species has been limited, and there are examples of native fauna being more impacted than the target AIS (e.g., Vélez-Espino et al., 2011; Rivera et al., 2018). Also, selective barrier designs that have worked under laboratory conditions can fail when tested in the field (e.g., Zielinski and Sorensen, 2015). Targeting traits to allow selective passage may be relatively straightforward in low diversity communities (Holthe et al., 2005; Morán-López and Tolosa, 2017) but is likely to be increasingly difficult in more biodiverse tropical communities. Overall, research on selective passage is in its infancy and more innovative solutions are required.
Some of the terminology used in the literature, such as “intentional fragmentation” and “selective fragmentation” (Rahel, 2013; Rahel and McLaughlin, 2018), is somewhat misleading. Fragmentation is the process by which a habitat is transformed into an increasing number of smaller patches, isolated from one another by habitats dissimilar to the original (Fahrig, 2003). Habitat fragmentation may be an unintended consequence of the use of AIS exclusion barriers, but never the underlying aim. The objective is to block or reduce AIS dispersal, and thereby benefit native fauna and protect habitats. Equally, “isolation management” should not be a goal, as isolation may negatively impact the long-term viability of native populations (Novinger and Rahel, 2003). Given that the global emphasis is on removing river barriers to restore connectivity, ecologists should be wary of using phrases such as “intentional fragmentation” which might not accurately reflect the underlying aims to non-expert stakeholders. We therefore suggest the term “AIS exclusion barriers” is more precise, especially since such barriers may be chemical, electrical, and acoustic, as well as physical.
With dam construction increasing in developing countries (Grill et al., 2019; Reid et al., 2019), dam removals gathering pace in Europe and North America (Bellmore et al., 2017), and climate change removing former thermal barriers to AIS movement (Hesselschwerdt and Wantzen, 2018), new invasion pathways are opening up for AIS globally. Meanwhile intentional introductions are still ongoing (Thomaz et al., 2015). While prevention is always the best way of limiting the harmful effects of AIS, exclusion barriers can become part of the AIS control toolbox. However, current barrier designs do not guarantee selective passage, so their use needs to be considered carefully to avoid impacting native fauna. Integrated AIS management plans are needed to mitigate the risk to aquatic ecosystems globally.
Ethics Statement
Written informed consent was obtained from the relevant individual(s) for the publication of any potentially identifiable images or data included in this article.
Author Contributions
ML, JT, and PJ conceptualised the study. PJ and JT led authorship, data collection, and analysis. All authors contributed to global case studies and critically contributed to writing of manuscript. All authors contributed to the article and approved the submitted version.
Funding
PJ, JT, CGL, and ML were supported by the European Union project AMBER (Adaptive Management of Barriers in European Rivers; https://amber.international/), which received funding from the EU Horizon 2020 Research and Innovation Programme under grant agreement No. 689682. SG was supported by a Commonwealth Scholarship from the UK government. DW was supported by National Research Foundation incentive funding (Grant 103581).
Conflict of Interest
The authors declare that the research was conducted in the absence of any commercial or financial relationships that could be construed as a potential conflict of interest.
Supplementary Material
The Supplementary Material for this article can be found online at: https://www.frontiersin.org/articles/10.3389/fevo.2021.611631/full#supplementary-material
Supplementary Figure 1 | An abstraction weir (A) used to prevent reinvasion of smallmouth bass (B) following eradication using piscicide.
Supplementary Figure 2 | Iguaçu Falls (A), a natural barrier to Brazilian tiger fish (S. brasiliensis) (B), a top predator in Neotropical rivers.
Supplementary Figure 3 | Barrier effect of a 1 m high obstacle (waterfall) on the upstream movement of non-native signal crayfish Pacifastacus leniusculus in the River Wharfe, northern England (based on Bubb, 2004).
Supplementary Figure 4 | (A) Waterfall augmented with a weir and culvert on the Swin Burn, New Zealand to protect Galaxias anomalus from brown trout (Photo credit: D. Jack), (B) A 30 cm brown trout captured immediately downstream from the barrier on the Swin Burn with six juvenile G. anomalus in its stomach (Photo credit: D. Jack).
Footnotes
References
Altenritter, M. E., Pescitelli, S. M., Whitten, A. L., and Casper, A. F. (2019). Implications of an invasive fish barrier for the long-term recovery of native fish assemblages in a previously degraded northeastern Illinois River system. River Res. Appl. 35, 1044–1052. doi: 10.1002/rra.3457
Applegate, V. C. (1950). Natural history of the sea lamprey (Petromyzon marinus) in Michigan. Spec. Sci. Rep. Fish. 55:237.
Applegate, V. C., and Smith, B. R. (1952). Use of electricity in the control of sea lampreys. Spec. Sci. Rep. Fish. 92:52.
Araújo, C. V., Silva, D. C., Gomes, L. E., Acayaba, R. D., Montagner, C. C., Moreira-Santos, M., et al. (2018). Habitat fragmentation caused by contaminants: atrazine as a chemical barrier isolating fish populations. Chemosphere 193, 24–31. doi: 10.1016/j.chemosphere.2017.11.014
Avenetti, L. D., Robinson, A. T., and Cantrell, C. J. (2006). Short-term effectiveness of constructed barriers at protecting Apache trout. North Am. J. Fish. Manag. 26, 213–216. doi: 10.1577/m04-092.1
Barnett, Z. C., Adams, S. B., Ochs, C. A., and Garrick, R. C. (2020). Crayfish populations genetically fragmented in streams impounded for 36–104 years. Freshw. Biol. 65, 768–785. doi: 10.1111/fwb.13466
Belletti, B., Garcia de Leaniz, C., Jones, J. A. H., Bizzi, S., Börger, L., Segura, G., et al. (2020). More than one million barriers fragment Europe’s rivers. Nature 588, 436–441.
Bellingan, T. A., Hugo, S., Woodford, D. J., Gouws, J., Villet, M. H., and Weyl, O. L. F. (2019). Rapid recovery of macroinvertebrates in a South African stream treated with rotenone. Hydrobiologia 834, 1–11. doi: 10.1007/s10750-019-3885-z
Bellmore, R. J., Duda, J. J., Craig, L. S., Greene, S. L., Torgersen, C. E., Collins, M. J., et al. (2017). Status and trends of dam removal research in the United States. Wiley Interdiscip. Rev. Water 4:e1164. doi: 10.1002/wat2.1164
Bennett, A. M., Keevil, M., and Litzgus, J. D. (2010). Spatial ecology and population genetics of northern map turtles (Graptemys geographica) in fragmented and continuous habitats in Canada. Chelonian Conserv. Biol. 9, 185–195. doi: 10.2744/ccb-0824.1
Beyer, J., Moy, P., and De Stasio, B. (2011). Acute upper thermal limits of three aquatic invasive invertebrates: hot water treatment to prevent upstream transport of invasive species. Environ. Manag. 47, 67–76. doi: 10.1007/s00267-010-9573-4
Birceanu, O., Sorensen, L. A., Henry, M., McClelland, G. B., Wang, Y. S., and Wilkie, M. P. (2014). The effects of the lampricide 3-trifluoromethyl-4-nitrophenol (TFM) on fuel stores and ion balance in a non-target fish, the rainbow trout (Oncorhynchus mykiss). Comp. Biochem. Physiol. Part C Toxicol. Pharmacol. 160, 30–41. doi: 10.1016/j.cbpc.2013.10.002
Branco, P., Amaral, S. D., Ferreira, M. T., and Santos, J. M. (2017). Do small barriers affect the movement of freshwater fish by increasing residency? Sci. Total Environ. 581, 486–494. doi: 10.1016/j.scitotenv.2016.12.156
Brito, M. F. G., Daga, V. S., and Vitule, J. R. S. (2020). Fisheries and biotic homogenization of freshwater fish in the Brazilian semiarid region. Hydrobiologia 847, 3877–3895. doi: 10.1007/s10750-020-04236-8
Britton, J. (2005). Dispersal of the invasive topmouth gudgeon, Pseudorasbora parva in the UK: a vector for an emergent infectious disease. Fish. Manag. Ecol. 12, 411–414. doi: 10.1111/j.1365-2400.2005.00466.x
Bubb, D. H. (2004). Spatial Ecology of White-Clawed Crayfish Austropotamobius pallipes and Signal Crayfish Pacifastacus leniusculus in Upland Rivers. Ph.D. thesis, Durham University, Durham.
Buktenica, M. W. (1994). Bull Trout Restoration and Brook Trout Eradication at Crater Lake National Park, Oregon. Washington, DC: National Park Service.
Buktenica, M. W., Hering, D. K., Girdner, S. F., Mahoney, B. D., and Rosenlund, B. D. (2013). Eradication of nonnative Brook Trout with electrofishing and antimycin-A and the response of a remnant Bull Trout population. North Am. J. Fish. Manag. 33, 117–129. doi: 10.1080/02755947.2012.747452
Buley, R. P., Hasler, C. T., Tix, J. A., Suski, C. D., and Hubert, T. D. (2017). Can ozone be used to control the spread of freshwater aquatic invasive species? Manag. Biol. Invasions 8:13. doi: 10.3391/mbi.2017.8.1.02
Caskenette, A., Enders, E. C., Watkinson, D., and Wrubleski, D. (2018). Partial exclusion of spawning Cyprinus carpio to improve coastal marsh habitat may come at the cost of increased carp population growth. Ecol. Model. 385, 58–64. doi: 10.1016/j.ecolmodel.2018.07.005
Chen, H. D. (1989). Impact of aquaculture on the ecosystem of Donghu Lake. Wuhan. Acta Hydrobiol. Sin. 13, 359–368.
Clarkson, R. W. (2004). Effectiveness of electrical fish barriers associated with the central arizona project. North Am. J. Fish. Manag. 24, 94–105. doi: 10.1577/m02-146
Clarkson, R. W., Marsh, P. C., and Dowling, T. E. (2012). Population prioritization for conservation of imperilled warmwater fishes in an arid-region drainage. Aquat. Conserv. 22, 498–510. doi: 10.1002/aqc.2257
Clusa, L., and García-Vázquez, E. (2018). A simple, rapid method for detecting seven common invasive fish species in Europe from environmental DNA. Aquat. Conserv. 28, 619–629. doi: 10.1002/aqc.2890
Coleman, R. A., Gauffre, B., Pavlova, A., Beheregaray, L. B., Kearns, J., Lyon, J., et al. (2018). Artificial barriers prevent genetic recovery of small isolated populations of a low-mobility freshwater fish. Heredity 120, 515–532. doi: 10.1038/s41437-017-0008-3
Conallin, A., Smith, B., Thwaites, L., Walker, K., and Gillanders, B. (2016). Exploiting the innate behaviour of common carp, Cyprinus carpio, to limit invasion and spawning in wetlands of the River Murray, Australia. Fish. Manag. Ecol. 23, 431–449. doi: 10.1111/fme.12184
Cucherousset, J., and Olden, J. D. (2011). Ecological impacts of nonnative freshwater fishes. Fisheries 36, 215–230. doi: 10.1080/03632415.2011.574578
Cupp, A. R., Smerud, J. R., Tix, J. A., Schleis, S. M., Fredricks, K. T., Erickson, R. A., et al. (2018). Field evaluation of carbon dioxide as a fish deterrent at a water management structure along the Illinois River. Manag. Biol. Invasions 9, 299–308. doi: 10.3391/mbi.2018.9.3.12
Cussac, V., Quiroga, S., Kacoliris, F., Povedano, H., Crichigno, S., Becker, L., et al. (2019). Gymnocharacinus bergii. The IUCN Red List of Threatened Species 2019: e.T40695A119048712. Available online at: http://dx.doi.org/10.2305/IUCN.UK.2019-2.RLTS.T40695A119048712.en (accessed August 29, 2017).
Daga, V. S., Azevedo-Santos, V. M., Pelicice, F. M., Fearnside, P. M., Perbiche-Neves, G., Paschoal, L. R. P., et al. (2020). Water diversion in Brazil threatens biodiversity. Ambio 49, 165–172. doi: 10.1007/s13280-019-01189-8
Daga, V. S., Debona, T., Abilhoa, V., Gubiani, ÉA., and Vitule, J. R. S. (2016). Non-native fish invasions of a Neotropical ecoregion with high endemism: a review of the Iguaçu River. Aquat. Invasions 11, 209–223. doi: 10.3391/ai.2016.11.2.10
Dana, E. D., García-de-Lomas, J., González, R., and Ortega, F. (2011). Effectiveness of dam construction to contain the invasive crayfish Procambarus clarkii in a Mediterranean mountain stream. Ecol. Eng. 37, 1607–1613. doi: 10.1016/j.ecoleng.2011.06.014
Daniel, A. J., Morgan, D. K., and Ling, N. (2014). Get Out, Stay Out! Restoring a Small New Zealand Floodplain Lake: Removal and Exclusion of Carp. In Carp Management in Australia-State of Knowledge. Canberra, ACT: Invasive Animals Cooperative Research Centre, 132–139.
De Moor, I. J., and Bruton, M. N. (1988). Atlas of alien and translocated indigenous aquatic animals in southern Africa. South African National Scientific Programmes Report 144. Pretoria: Council for Scientific and Industrial Research.
De Silva, S., Nguyen, T. T. T., Abery, N. W., and Amarasinghe, U. S. (2006). An evaluation of the role and impacts of alien finfish in Asian inland aquaculture. Aquac. Res. 37, 1–17. doi: 10.1111/j.1365-2109.2005.01369.x
Deines, A. M., Bbole, I., Katongo, C., Feder, J. L., and Lodge, D. M. (2014). Hybridisation between native Oreochromis species and introduced Nile tilapia O. niloticus in the Kafue River. Zambia. Afr. J. Aquat. Sci. 39, 23–34. doi: 10.2989/16085914.2013.864965
Dodd, H. R., Hayes, D. B., Baylis, J. R., Carl, L. M., Goldstein, J. D., McLaughlin, R. L., et al. (2003). Low-head sea lamprey barrier effects on stream habitat and fish communities in the Great Lakes Basin. J. Great Lakes Res. 29(Suppl. 1), 386–402. doi: 10.1016/s0380-1330(03)70502-4
Dudgeon, D., Arthington, A. H., Gessner, M. O., Kawabata, Z. I., Knowler, D. J., Lévêque, C., et al. (2006). Freshwater biodiversity: importance, threats, status and conservation challenges. Biol. Rev. 81, 163–182.
Ellender, B. R., Wasserman, R. J., Chakona, A., Skelton, P. H., and Weyl, O. L. F. (2017). A review of the biology and status of cape fold ecoregion freshwater fishes. Aquat. Conserv. 27, 867–879. doi: 10.1002/aqc.2730
Ellender, B. R., and Weyl, O. L. F. (2014). A review of current knowledge, risk and ecological impacts associated with non-native freshwater fish introductions in South Africa. Aquat. Invasions 9, 117–132. doi: 10.3391/ai.2014.9.2.01
Fagan, W. F. (2002). Connectivity, fragmentation, and extinction risk in dendritic metapopulations. Ecology 83, 3243–3249. doi: 10.1890/0012-9658(2002)083[3243:cfaeri]2.0.co;2
Fahrig, L. (2003). Effects of habitat fragmentation on biodiversity. Annu. Rev. Ecol. Evol. Syst. 34, 487–515.
Fausch, K. D., Rieman, B. E., Dunham, J. B., Young, M. K., and Peterson, D. P. (2009). Invasion versus isolation: trade-offs in managing native salmonids with barriers to upstream movement. Conserv. Biol. 23, 859–870. doi: 10.1111/j.1523-1739.2008.01159.x
Franklin, P. A., Gee, E., Baker, C. F., and Bowie, S. (2018). New Zealand Fish Passage Guidelines–For Structures Up to 4 Metres. Hamilton, NZ: NIWA.
Frehse, F. A., Braga, R. R., Nocera, G. A., and Vitule, J. R. S. (2016). Non-native species and invasion biology in a megadiverse country: scientometric analysis and ecological interactions in Brazil. Biol. Invasions 18, 3713–3725. doi: 10.1007/s10530-016-1260-9
French, J. R., Wilcox, D. A., and Nichols, S. J. (1999). Passing of northern pike and common carp through experimental barriers designed for use in wetland restoration. Wetlands 19, 883–888. doi: 10.1007/bf03161790
Frings, R. M., Vaeßen, S. C., Groß, H., Roger, S., Schüttrumpf, H., and Hollert, H. (2013). A fish-passable barrier to stop the invasion of non-indigenous crayfish. Biol. Conserv. 159, 521–529. doi: 10.1016/j.biocon.2012.12.014
Fujimoto, Y., Yambe, H., Takahashi, K., and Sato, S. (2020). Bile from reproductively mature male largemouth bass Micropterus salmoides attracts conspecific females and offers a practical application to control populations. Manag. Biol. Invasions 11, 415–427. doi: 10.3391/mbi.2020.11.3.05
Fullerton, A. H., Burnett, K. M., Steel, E. A., Flitcroft, R. L., Pess, G. R., Feist, B. E., et al. (2010). Hydrological connectivity for riverine fish: measurement challenges and research opportunities. Freshw. Biol. 55, 2215–2237. doi: 10.1111/j.1365-2427.2010.02448.x
Galib, S. M., Lucas, M. C., Chaki, N., Fahad, F. H., and Mohsin, A. B. M. (2018). Is current floodplain management a cause for concern for fish and bird conservation in Bangladesh’s largest wetland? Aquat. Conserv. 28, 98–114. doi: 10.1002/aqc.2865
Galib, S. M., and Mohsin, A. B. M. (2010). Exotic ornamental fishes of Bangladesh. Bangladesh J. Progress. Sci. Technol. 8, 255–258.
Gallardo, B., Clavero, M., Sánchez, M. I., and Vilà, M. (2016). Global ecological impacts of invasive species in aquatic ecosystems. Glob. Change Biol. 22, 151–163. doi: 10.1111/gcb.13004
Garcia de Leaniz, C., Berkhuysen, A., and Belletti, B. (2019). Beware small dams as well as large. Nature 570, 164–164. doi: 10.1038/d41586-019-01826-y
Garcia de Leaniz, C., Gajardo, G., and Consuegra, S. (2010). From best to pest: changing perspectives on the impact of exotic salmonids in the Southern Hemisphere. Syst. Biodiv. 8, 447–459. doi: 10.1080/14772000.2010.537706
Garrone-Neto, D., Haddad, V. Jr., and Gadig, O. B. F. (2014). Record of ascending passage of potamotrygonid stingrays through navigation locks: implications for the management of non-native species in the Upper Paraná River basin, southeastern Brazil. Manag. Biol. Invasions 5, 113–119. doi: 10.3391/mbi.2014.5.2.04
Geerts, A. N., Boets, P., Van den Heede, S., and Goethals, P. (2018). A search for standardized protocols to detect alien invasive crayfish based on environmental DNA (eDNA): a lab and field evaluation. Ecol. Indic. 84, 564–572. doi: 10.1016/j.ecolind.2017.08.068
Gozlan, R. E., Britton, J. R., Cowx, I., and Copp, G. H. (2010). Current knowledge on non-native freshwater fish introductions. J. Fish Biol. 76, 751–786. doi: 10.1111/j.1095-8649.2010.02566.x
Grill, G., Lehner, B., Thieme, M., Geenen, B., Tickner, D., Antonelli, F., et al. (2019). Mapping the world’s free-flowing rivers. Nature 569, 215–221.
Harvey, G. L., Moorhouse, T. P., Clifford, N. J., Henshaw, A. J., Johnson, M. F., Macdonald, D. W., et al. (2011). Evaluating the role of invasive aquatic species as drivers of fine sediment-related river management problems: the case of the signal crayfish (Pacifastacus leniusculus). Progress Phys. Geogr. 35, 517–533. doi: 10.1177/0309133311409092
Håstein, T., and Gladhaug, O. (1973). The occurrence of the crayfish plague in Norway and attempts to prevent further spread of the disease. Freshw. Crayfish 1, 181–184.
Herder, J. E., Valentini, A., Bellemain, E., Dejean, T., van Delft, J. J. C. W., Thomsen, P. F., et al. (2014). Environmental DNA – A Review of the Possible Applications for the Detection of (Invasive) Species. Report 2013-104. Nijmegen: Stichting RAVON.
Hesselschwerdt, J., and Wantzen, K. M. (2018). Global warming may lower thermal barriers against invasive species in freshwater ecosystems–a study from lake constance. Sci. Total Environ. 645, 44–50. doi: 10.1016/j.scitotenv.2018.07.078
Hilderbrand, R. H., and Kershner, J. L. (2000). Conserving inland cutthroat trout in small streams: how much stream is enough? North Am. J. Fish. Manag. 20, 513–520. doi: 10.1577/1548-8675(2000)020¡0513:cictis¿2.3.co;2
Hillyard, K. A., Smith, B. B., Conallin, A. J., and Gillanders, B. M. (2010). Optimising exclusion screens to control exotic carp in an Australian lowland river. Mar. Freshw. Res. 61, 418–429. doi: 10.1071/mf09017
Holliman, F. M., Killgore, K. J., and Shea, C. (2015). Development of Operational Protocols for Electric Barrier Systems on the Chicago Sanitary and Ship Canal: Induction of Passage-Preventing Behaviors in Small Sizes of Silver Carp (No. ERDC/TN-ANSRP-15-1). Vicksburg, MS: US Army Engineer Research and Development Center.
Holthe, E., Lund, E., Finstad, B., Thorstad, E. B., and McKinley, R. S. (2005). A fish selective obstacle to prevent dispersion of an unwanted fish species, based on leaping capabilities. Fish. Manag. Ecol. 12, 143–147. doi: 10.1111/j.1365-2400.2004.00436.x
Hoyer, S. A., and Myrick, C. A. (2012). Can copper-based substrates be used to protect hatcheries from invasion by the New Zealand mudsnail? North Am. J. Aquac. 74, 575–583. doi: 10.1080/15222055.2012.685213
Hume, J. B., Almeida, P. R., Bucjley, C. M., Criger, L. A., Madenjian, C. P., Robinson, K. F., et al. (2020a). Managing native and non-native sea lamprey (Petromyzon marinus) through anthropogenic change: a prospective assessment of key threats and uncertainties. J. Great Lakes Res. doi: 10.1016/j.jglr.2020.08.015
Hume, J. B., Lucas, M. C., Reinhardt, U., Hrodey, P. J., and Wagner, C. M. (2020b). Sea lamprey (Petromyzon marinus) transit of a ramp equipped with studded substrate: implications for fish passage and invasive species control. Ecol. Eng. 155:105957. doi: 10.1016/j.ecoleng.2020.105957
Hume, J. B., Luhring, T. M., and Wagner, C. M. (2020c). Push, pull, or push-pull? An alarm cue better guides sea lamprey towards capture devices than a mating pheromone during the reproductive migration. Biol. Invasions 22, 2129–2142. doi: 10.1007/s10530-020-02242-4
Hume, J. B., Meckley, T. D., Johnson, N. S., Luhring, T. M., Siefkes, M. J., and Wagner, C. M. (2015). Application of a putative alarm cue hastens the arrival of invasive sea lamprey (Petromyzon marinus) at a trapping location. Can. J. Fish. Aquat. Sci. 72, 1799–1806. doi: 10.1139/cjfas-2014-0535
Jackson, C. R., and Pringle, C. M. (2010). Ecological benefits of reduced hydrologic connectivity in intensively managed landscapes. BioScience 60, 37–46. doi: 10.1525/bio.2010.60.1.8
Jensen, A. J., and Jones, M. L. (2018). Forecasting the response of Great Lakes sea lamprey (Petromyzon marinus) to barrier removals. Can. J. Fish. Aquat. Sci. 75, 1415–1426.
Jerde, C. L., Mahon, A. R., Chadderton, W. L., and Lodge, D. M. (2011). “Sight-unseen” detection of rare aquatic species using environmental DNA. Conserv. Lett. 4, 150–157. doi: 10.1111/j.1755-263x.2010.00158.x
Johnsen, S. I., Jansson, T., Høye, J. K., and Taugbøl, T. (2008). Vandringssperre for Signalkreps i Bua a, Eda Kommun, Sverige – Overva King av signalkreps og Krepsepest Situasjonen. NINA Rapport 356, Trondheim: NINA, 15.
Johnson, N. S., Miehls, S., O’Connor, L. M., Bravener, G., Barber, J., Thompson, H., et al. (2016). A portable trap with electric lead catches up to 75% of an invasive fish species. Sci. Repor. 6:28430. doi: 10.1038/srep28430
Johnson, N. S., Thompson, H. T., Holbrook, C., and Tix, J. A. (2014). Blocking and guiding adult sea lamprey with pulsed direct current from vertical electrodes. Fish. Res. 150, 38–48. doi: 10.1016/j.fishres.2013.10.006
Johnson, N. S., Yun, S., Thompson, H. T., Brant, C. O., and Li, W. (2009). A synthesized pheromone induces upstream movement in female sea lamprey and summons them into traps. Proc. Natl. Acad. Sci. 106, 1021–1026. doi: 10.1073/pnas.0808530106
Johnson, P. T., Olden, J. D., and Vander Zanden, M. J. (2008). Dam invaders: impoundments facilitate biological invasions into freshwaters. Front. Ecol. Environ. 6:357–363. doi: 10.1890/070156
Jones, J., Börger, L., Tummers, J., Jones, P. E., Lucas, M., Kerr, J., et al. (2019). A comprehensive assessment of stream fragmentation in Great Britain. Sci. Total Environ. 673, 756–762. doi: 10.1016/j.scitotenv.2019.04.125
Jones, P. E., and Closs, G. P. (2018). The Introduction of Brown Trout to New Zealand and Their Impact on Native Fish Communities. In Brown Trout: Biology, Ecology and Management. Hoboken, NJ: John Wiley and Sons Ltd, 545–567.
Junker, J., Peter, A., Wagner, C. E., Mwaiko, S., Germann, B., Seehausen, O., et al. (2012). River fragmentation increases localized population genetic structure and enhances asymmetry of dispersal in bullhead (Cottus gobio). Conserv. Genet. 13, 545–556. doi: 10.1007/s10592-011-0306-x
Kakareko, T., Kobak, J., Grabowska, J., Jermacz, Ł, Przybylski, M., Poznańska, M., et al. (2013). Competitive interactions for food resources between invasive racer goby Babka gymnotrachelus and native European bullhead Cottus gobio. Biol. Invasions 15, 2519–2530. doi: 10.1007/s10530-013-0470-7
Kerr, J., Vowles, A., Crabb, M., and Kemp, P. (2020). Selective fish passage: restoring habitat connectivity without facilitating the spread of a non-native species. J. Environ. Manag. 279:110908. doi: 10.1016/j.jenvman.2020.110908
Kim, J., and Mandrak, N. E. (2017). Effects of vertical electric barrier on the behaviour of common carp. Manag. Biol. Invasions 8, 497–505. doi: 10.3391/mbi.2017.8.4.04
Kim, J., and Mandrak, N. E. (2019). Effects of a vertical electric barrier on the behaviour of Rainbow Trout. Aquat. Ecosyst. Health Manag. 22, 183–192.
King, S., and O’Hanley, J. R. (2016). Optimal fish passage barrier removal – revisited. River Res. Appl.32, 418–428. doi: 10.1002/rra.2859
Klingler, G. L., Adams, J. V., and Heinrich, J. W. (2003). Passage of four teleost species prior to sea lamprey (Petromyzon marinus) migration in eight tributaries of Lake Superior, 1954 to 1979. J. Great Lakes Res. 29, 403–409. doi: 10.1016/s0380-1330(03)70503-6
Knight, J. D. M. (2010). Invasive ornamental fish: a potential threat to aquatic biodiversity in peninsular India. J. Threat. Taxa. 2, 700–704. doi: 10.11609/JoTT.o2179.700-4
Koehn, J. D., and MacKenzie, R. F. (2004). Priority management actions for alien freshwater fish species in Australia. N. Z. J. Mar. Freshw. Res. 38, 457–472. doi: 10.1080/00288330.2004.9517253
Krieg, R., King, A., and Zenker, A. (2021). Barriers against invasive crayfish species in natural waters and fish passes-practical experience. Glob. Ecol. Conserv. 25:e01421. doi: 10.1016/j.gecco.2020.e01421
Krieg, R., and Zenker, A. (2020). A review of the use of physical barriers to stop the spread of non-indigenous crayfish species. Rev. Fish Biol. Fish. 30, 423–435. doi: 10.1007/s11160-020-09606-y
Layhee, M. J., Sepulveda, A. J., Shaw, A., Smuckall, M., Kapperman, K., and Reyes, A. (2016). Effects of electric barrier on passage and physical condition of juvenile and adult rainbow trout. J. Fish Wildl. Manag. 7, 28–35. doi: 10.3996/042015-jfwm-039
Lechner, A., Keckeis, H., Schludermann, E., Humphries, P., McCasker, N., and Tritthart, M. (2014). Hydraulic forces impact larval fish drift in the free-flowing section of a large European river. Ecohydrology 7, 648–658. doi: 10.1002/eco.1386
Leprieur, F., Beauchard, O., Blanchet, S., Oberdorff, T., and Brosse, S. (2008a). Fish invasions in the world’s river systems: when natural processes are blurred by human activities. PLoS Biol. 6:e28. doi: 10.1371/journal.pbio.0060028
Leprieur, F., Beauchard, O., Hugueny, B., Grenouillet, G., and Brosse, S. (2008b). Null model of biotic homogenization: a test with the European freshwater fish fauna. Divers. Distrib. 14, 291–300. doi: 10.1111/j.1472-4642.2007.00409.x
Li, F., Zhang, J. J., Yuan, Y. F., Feng, H., Zhang, J. Y., and Yang, X. Z. (2008). Present situation and problems on fish introduction in Yellow River system. J. Anhui Agric. Sci. 36, 15024–15026.
Liew, J. H., Tan, H. H., and Yeo, D. C. (2016). Dammed rivers: impoundments facilitate fish invasions. Freshw. Biol. 61, 1421–1429. doi: 10.1111/fwb.12781
Lin, H. Y., and Robinson, K. F. (2019). How do migratory fish populations respond to barrier removal in spawning and nursery grounds? Theor. Ecol. 12, 379–390. doi: 10.1007/s12080-018-0405-0
Lintermans, M. (2000). Recolonization by the mountain galaxias Galaxias olidus of a montane stream after the eradication of rainbow trout Oncorhynchus mykiss. Mar. Freshw. Res. 51, 799–804. doi: 10.1071/mf00019
Luhring, T. M., Meckley, T. D., Johnson, N. S., Siefkes, M. J., Hume, J. B., and Wagner, C. M. (2016). A semelparous fish continues upstream migration when exposed to alarm cue, but adjusts movement speed and timing. Anim. Behav. 121, 41–51. doi: 10.1016/j.anbehav.2016.08.007
Luo, D., Wei, H., Chaichana, R., Yang, D., Gu, D., Mu, X., et al. (2019). Current status and potential risks of established alien fish species in China. Aquat. Ecosyst. Health Manag. 22, 371–384. doi: 10.1080/14634988.2019.1693226
Maceina, M. J., Slipke, J. W., and Grizzle, J. M. (1999). Effectiveness of three barrier types for confining grass carp in embayments of Lake Seminole, Georgia. North Am. J. Fish. Manag. 19, 968–976. doi: 10.1577/1548-8675(1999)019¡0968:eotbtf¿2.0.co;2
Magalhães, A. L. B., Daga, V. S., Bezerra, L. A. V., Vitule, J. R. S., Jacobi, C. M., and Silva, L. G. M. (2020). All the colors of the world: biotic homogenization-differentiation dynamics of freshwater fish communities on demand of the Brazilian aquarium trade. Hydrobiologia 847, 3897–3915. doi: 10.1007/s10750-020-04307-w
Martin, C. J., Allen, B. J., and Lowe, C. G. (2012). Environmental impact assessment: detecting changes in fish community structure in response to disturbance with an asymmetric multivariate BACI sampling design. Bull. South. Calif. Acad. Sci. 111, 119–131. doi: 10.3160/0038-3872-111.2.119
Martinez, P. J., and Foreman, C. (2001). Observations on the Effectiveness and Maintenance of the Highline Lake Fish Barrier Net. Grand Junction, CO: Colorado Division of Wildlife.
McDowall, R. M. (2006). Crying wolf, crying foul, or crying shame: alien salmonids and a biodiversity crisis in the southern cool-temperate galaxioid fishes? Rev. Fish Biol. Fish. 16, 233–422. doi: 10.1007/s11160-006-9017-7
McLain, A. L., Smith, B. R., and Moore, H. H. (1965). Experimental control of sea lampreys with electricity on the south shore of Lake Superior, 1953-60. Great Lakes Fish Commun. Tech. Rep. 10:48.
McLaughlin, R. L., Porto, L., Noakes, D. L., Baylis, J. R., Carl, L. M., Dodd, H. R., et al. (2006). Effects of low-head barriers on stream fishes: taxonomic affiliations and morphological correlates of sensitive species. Can. J. Fish. Aquat. Sci. 63, 766–779. doi: 10.1139/f05-256
McLaughlin, R. L., Smyth, E. R. B., Castro-Santos, T., Jones, M. L., Koops, M. A., Pratt, T. C., et al. (2013). Unintended consequences and trade-offs of fish passage. Fish Fish. 14, 580–604. doi: 10.1111/faf.12003
Meyers, L. S., Thuemler, T. F., and Kornely, G. W. (1992). Seasonal movements of brown trout in northeast Wisconsin. North Am. J. Fish. Manag. 12, 433–441. doi: 10.1577/1548-8675(1992)012¡0433:smobti¿2.3.co;2
Miehls, S. M., Johnson, N. S., and Hrodey, P. J. (2017). Test of a nonphysical barrier consisting of light, sound, and bubble screen to block upstream movement of sea lampreys in an experimental raceway. North Am. J. Fish. Manag. 37, 660–666. doi: 10.1080/02755947.2017.1308892
Moody, A. T., Neeson, T. M., Wangen, S., Dischler, J., Diebel, M. W., Milt, A., et al. (2017). Pet project or best project? Online decision support tools for prioritizing barrier removals in the Great Lakes and beyond. Fish 42, 57–65. doi: 10.1080/03632415.2016.1263195
Morán-López, R., and Tolosa, O. U. (2017). Relative leaping abilities of native versus invasive cyprinids as criteria for selective barrier design. Biol. Invasions 19, 1243–1253. doi: 10.1007/s10530-016-1328-6
Mouser, J., Ashley, D. C., Aley, T., and Brewer, S. K. (2019). Subterranean invasion by gapped ringed crayfish: effectiveness of a removal effort and barrier installation. Diversity 11:3. doi: 10.3390/d11010003
Moy, P. B. (1999). “Development of an aquatic nuisance species dispersal barrier,” in WRPMD’99: Preparing for the 21st Century, Chicago, IL: U.S. Army Corps of Engineers, 1–6.
Muhlfeld, C., D’Angelo, V., Kalinowski, T. S., Landguth, L. E., Downs, C., Tohtz, J., et al. (2012). A fine-scale assessment of using barriers to conserve native stream salmonids: a case study in Akokala Creek, Glacier National Park, USA. Open Fish Sci. J. 5, 9–20. doi: 10.2174/1874401x01205010009
Murphy, M. O., Jones, K. S., Price, S. J., and Weisrock, D. W. (2018). A genomic assessment of population structure and gene flow in an aquatic salamander identifies the roles of spatial scale, barriers, and river architecture. Freshw. Biol. 63, 407–419. doi: 10.1111/fwb.13071
Neeson, T. M., Ferris, M. C., Diebel, M. W., Doran, P. J., O’Hanley, J. R., and McIntyre, P. B. (2015). Enhancing ecosystem restoration efficiency through spatial and temporal coordination. Proc. Natl. Acad. Sci. 112, 6236–6241. doi: 10.1073/pnas.1423812112
Nehring, R. B., Alves, J., Nehring, J. B., and Felt, B. (2018). Elimination of Myxobolus cerebralis in placer creek, a native cutthroat trout stream in Colorado. J. Aquat. Anim. Health 30, 264–279. doi: 10.1002/aah.10039
Novinger, D. C., and Rahel, F. J. (2003). Isolation management with artificial barriers as a conservation strategy for cutthroat trout in headwater streams. Conserv. Biol. 17, 772–781. doi: 10.1046/j.1523-1739.2003.00472.x
Oliveira, M., Campos, M., Paolucci, E., Mansur, M., and Hamilton, S. (2015). “Colonization and spread of Limnoperna fortunei in South America,” In Limnoperna Fortunei. Invading Nature – Springer Series in Invasion Ecology, ed. D. boltovskoy (New York, NY: Springer International Publishing), 10, 24.
Parker, A. D., Glover, D. C., Finney, S. T., Rogers, P. B., Stewart, J. G., and Simmonds, R. L. Jr. (2015). Direct observations of fish incapacitation rates at a large electrical fish barrier in the Chicago Sanitary and Ship Canal. J. Great Lakes Res. 41, 396–404. doi: 10.1016/j.jglr.2015.03.004
Parker, A. D., Glover, D. C., Finney, S. T., Rogers, P. B., Stewart, J. G., and Simmonds, R. L. Jr. (2016). Fish distribution, abundance, and behavioral interactions within a large electric dispersal barrier designed to prevent Asian carp movement. Can. J. Fish. Aquat. Sci. 73, 1060–1071. doi: 10.1139/cjfas-2015-0309
Pelicice, F. M., Azevedo-Santos, V. M., Vitule, J. R. S., Orsi, M. L., Lima Junior, D. P., Magalhães, A. L. B., et al. (2017). Neotropical freshwater fishes imperilled by unsustainable policies. Fish Fish. 18, 1119–1133.
Perkin, J. S., and Gido, K. B. (2012). Fragmentation alters stream fish community structure in dendritic ecological networks. Ecol. Appl. 22, 2176–2187. doi: 10.1890/12-0318.1
Perrault, K., Imre, I., and Brown, G. E. (2014). Behavioural response of larval sea lamprey (Petromyzon marinus) in a laboratory environment to potential damage-released chemical alarm cues. Can. J. Zool. 92, 443–447. doi: 10.1139/cjz-2013-0095
Pess, G. R., McHenry, M. L., Beechie, T. J., and Davies, J. (2008). Biological impacts of the Elwha River dams and potential salmonid responses to dam removal. Northwest Sci. 82, 72–90. doi: 10.3955/0029-344x-82.s.i.72
Peterson, D. P., Rieman, B. E., Dunham, J. B., Fausch, K. D., and Young, M. K. (2008). Analysis of trade-offs between threats of invasion by nonnative brook trout (Salvelinus fontinalis) and intentional isolation for native westslope cutthroat trout (Oncorhynchus clarkii lewisi). Can. J. Fish. Aquat. Sci. 65, 557–573. doi: 10.1139/f07-184
Pimentel, D., Lach, L., Zuniga, R., and Morrison, D. (2000). Environmental and economic costs of nonindigenous species in the United States. BioScience 50, 53–65. doi: 10.1641/0006-3568(2000)050[0053:eaecon]2.3.co;2
Porto, L. M., McLaughlin, R. L., and Noakes, D. L. G. (1999). Low-head barrier dams restrict the movements of fishes in two Lake Ontario streams. North Am. J. Fish. Manag. 19, 1028–1036. doi: 10.1577/1548-8675(1999)019¡1028:lhbdrt¿2.0.co;2
Pratt, T. C., O’connor, L. M., Hallett, A. G., McLaughlin, R. L., Katopodis, C., Hayes, D. B., et al. (2009). Balancing aquatic habitat fragmentation and control of invasive species: enhancing selective fish passage at sea lamprey control barriers. Trans. Am. Fish. Soc. 138, 652–665. doi: 10.1577/t08-118.1
Rahel, F. J. (2013). Intentional fragmentation as a management strategy in aquatic systems. BioScience 63, 362–372. doi: 10.1525/bio.2013.63.5.9
Rahel, F. J., and McLaughlin, R. L. (2018). Selective fragmentation and the management of fish movement across anthropogenic barriers. Ecol. Appl. 28, 2066–2081. doi: 10.1002/eap.1795
Reid, A. J., Carlson, A. K., Creed, I. F., Eliason, E. J., Gell, P. A., Johnson, P. T., et al. (2019). Emerging threats and persistent conservation challenges for freshwater biodiversity. Biol. Rev. 94, 849–873.
Rivera, J. M., Glover, D. C., Kocovsky, P. M., Garvey, J. E., Gaikowski, M. P., Jensen, N. R., et al. (2018). Water guns affect abundance and behavior of bigheaded carp and native fish differently. Biol. Invasions 20, 1243–1255. doi: 10.1007/s10530-017-1624-9
Robinson, C. V., Garcia, de Leaniz, C., and Consuegra, S. (2019). Effect of artificial barriers on the distribution of the invasive signal crayfish and Chinese mitten crab. Sci. Rep. 9:7230.
Rolla, M., Consuegra, S., Hall, D. J., and Garcia de Leaniz, C. (2020). Seasonal and spatial variation in growth and abundance of zebra mussel (Dreissena polymorpha) in a recently invaded artificial lake: Implications for management. Front. Ecol. Evol. 8:159. doi: 10.3389/fevo.2020.00159
Romine, J. G., Jensen, N. R., Parsley, M. J., Gaugush, R. F., Severson, T. J., Hatton, T. W., et al. (2015). Response of bighead carp and silver carp to repeated water gun operation in an enclosed shallow pond. North Am. J. Fish. Manag. 35, 440–453. doi: 10.1080/02755947.2015.1012279
Ruebush, B. C., Sass, G. G., Chick, J. H., and Stafford, J. D. (2012). In-situ tests of sound-bubble-strobe light barrier technologies to prevent range expansions of Asian carp. Aquat. Invasions 7, 37–48. doi: 10.3391/ai.2012.7.1.005
Sabatini, A., Podda, C., Frau, G., Cani, M. V., Musu, A., Serra, M., et al. (2018). Restoration of native Mediterranean brown trout Salmo cettii Rafinesque, 1810 (Actinopterygii: Salmonidae) populations using an electric barrier as a mitigation tool. Eur. Zool. J. 85, 137–149. doi: 10.1080/24750263.2018.1453554
Santos, D. A., Affonso, I. P., Message, H. J., Okada, E. K., Gomes, L. C., Bornatowski, H., et al. (2019). Societal perception, impacts and judgment values about invasive freshwater stingrays. Biol. Invasions 21, 3593–3606. doi: 10.1007/s10530-019-02071-0
Savino, J. F., Jude, D. J., Kostich, M. J., Jude, D. J., and Kostich, M. J. (2001). “Use of electrical barriers to deter movement of round goby,” in Proceedings of the Behavioral Technologies for Fish Guidance: American Fisheries Society Symposium, Bethesda, MD, 26, 171–182.
Schneider, E. V., Hasler, C. T., and Suski, C. D. (2018). Fish behavior in elevated CO2: implications for a movement barrier in flowing water. Biol. Invasions 20, 1899–1911. doi: 10.1007/s10530-018-1669-4
Schoelynck, J., Van Loon, P., Heirmans, R., Jacobs, S., and Keirsebelik, H. (2020). Design and testing of a trap removing Chinese mitten crabs (Eriocheir sinensis, H. Milne Edwards, 1853) from invaded river systems. River Res. Appl. doi: 10.1002/rra.3635
Shea, C., and Dettmers, D. J. (2009). Chicago Sanitary and Ship Canal Aquatic Nuisance Species Dispersal Barriers. Washington, DC: US Army Corps of Engineers.
Skóra, F., Abilhoa, V., Padial, A. A., and Vitule, J. R. S. (2015). Darwin’s hypotheses to explain colonization trends: evidence from a quasi-natural experiment and a new conceptual model. Divers. Distrib. 21, 583–594. doi: 10.1111/ddi.12308
Smith, E. P., Orvos, D. R., and Cairns, J. Jr. (1993). Impact assessment using the before-after-control-impact (BACI) model: concerns and comments. Can. J. Fish. Aquat. Sci. 50, 627–637. doi: 10.1139/f93-072
Snyder, H. (2019). Literature review as a research methodology: an overview and guidelines. J. Bus. Res. 104, 333–339. doi: 10.1016/j.jbusres.2019.07.039
Stamplecoskie, K. M., Binder, T. R., Lower, N., Cottenie, K., McLaughlin, R. L., and McDonald, D. G. (2012). Response of migratory sea lampreys to artificial lighting in portable traps. North Am. J. Fish. Manag. 32, 563–572. doi: 10.1080/02755947.2012.675963
Strayer, D. L. (2010). Alien species in fresh waters: ecological effects, interactions with other stressors, and prospects for the future. Freshw. Biol. 55, 152–174. doi: 10.1111/j.1365-2427.2009.02380.x
Stuart, I. G., and Conallin, A. J. (2018). Control of Globally Invasive Common Carp: An 11-Year Commercial Trial of the Williams’ Cage. North Am. J. Fish. Manag. 38, 1160–1169. doi: 10.1002/nafm.10221
Stuart, I. G., Williams, A., McKenzie, J., and Holt, T. (2006). Managing a migratory pest species: a selective trap for common carp. North Am. J. Fish. Manag. 26, 888–893. doi: 10.1577/m05-205.1
Sturtevant, R., and Cangelosi, A. (2000). The Great Lakes at the Millennium: Priorities for Fiscal 2001. Washington, DC: Northeast Midwest Institute.
Swink, W. D. (1999). Effectiveness of an electrical barrier in blocking a sea lamprey spawning migration on the Jordan River, Michigan. North Am. J. Fish. Manag. 19, 397–405. doi: 10.1577/1548-8675(1999)019¡0397:eoaebi¿2.0.co;2
Taylor, A. H., Tracey, S. R., Hartmann, K., and Patil, J. G. (2012). Exploiting seasonal habitat use of the common carp, Cyprinus carpio, in a lacustrine system for management and eradication. Mar. Freshw. Res. 63, 587–597. doi: 10.1071/mf11252
Taylor, R. M., Pegg, M. A., and Chick, J. H. (2005). Response of bighead carp to a bioacoustic behavioural fish guidance system. Fish. Manag. Ecol. 12, 283–286. doi: 10.1111/j.1365-2400.2005.00446.x
Tempero, G. W., Hicks, B. J., Ling, N., Morgan, D., Daniel, A. J., Özkundakci, D., et al. (2019). Fish community responses to invasive fish removal and installation of an exclusion barrier at Lake Ohinewai, Waikato. N. Z. J. Mar. Freshw. Res. 53, 397–415. doi: 10.1080/00288330.2019.1579101
Thomas, J. R., Masefield, S., Hunt, R., Wood, M. J., Hart, A. G., Hallam, J., et al. (2019). Terrestrial emigration behaviour of two invasive crayfish species. Behav. Process. 167:103917. doi: 10.1016/j.beproc.2019.103917
Thomaz, S. M., Kovalenko, K. E., Havel, J. E., and Kats, L. B. (2015). Aquatic invasive species: general trends in the literature and introduction to the special issue. Hydrobiologia 746, 1–12. doi: 10.1007/s10750-014-2150-8
Thompson, P. D., and Rahel, F. J. (1998). Evaluation of artificial barriers in small Rocky Mountain streams for preventing the upstream movement of brook trout. North Am. J. Fish. Manag. 18, 206–210. doi: 10.1577/1548-8675(1998)018¡0206:eoabis¿2.0.co;2
Thwaites, L. A., Smith, B. B., Decelis, M., Fleer, D., and Conallin, A. (2010). A novel push trap element to manage carp (Cyprinus carpio L.): a laboratory trial. Mar. Freshw. Res. 61, 42–48. doi: 10.1071/mf09011
Tomojiri, D., Musikasinthorn, P., and Iwata, A. (2019). Food habits of three non-native cichlid fishes in the lowermost Chao Phraya River basin. Thailand. J. Freshw. Ecol. 34, 419–432. doi: 10.1080/02705060.2019.1585392
Townsend, C. R., and Crowl, T. A. (1991). Fragmented population structure in a native New Zealand fish: an effect of introduced brown trout? Oikos 61, 347–354. doi: 10.2307/3545242
Tummers, J. S., Hudson, S., and Lucas, M. C. (2016). Evaluating the effectiveness of restoring longitudinal connectivity for stream fish communities: towards a more holistic approach. Sci. Total Environ. 569-570, 850–860. doi: 10.1016/j.scitotenv.2016.06.207
Unestam, T., Nestell, C. G., and Abrahamsson, S. (1972). An electrical barrier for preventing migration of freshwater crayfish in running water. A method to stop the spread of crayfish plague. Rep. Inst. Freshw. Res. Drottningholm 52, 199–203.
Valentini, A., Pompanon, F., and Taberlet, P. (2009). DNA barcoding for ecologists. Trends Ecol. Evol. 24, 110–117. doi: 10.1016/j.tree.2008.09.011
Van der Walt, J. A., Marr, S. M., Wheeler, M. J., Impson, N. D., Garrow, C., and Weyl, O. L. (2019). Successful mechanical eradication of spotted bass (Micropterus punctulatus (Rafinesque, 1819)) from a South African river. Aquat. Conserv. 29, 303–311. doi: 10.1002/aqc.3035
Van der Walt, J. A., Weyl, O. L. F., Woodford, D. J., and Radloff, F. G. T. (2016). Spatial extent and consequences of black bass (Micropterus spp.) invasion in a Cape Floristic Region river basin. Aquat. Conserv. 26, 736–748. doi: 10.1002/aqc.2589
Van der Zanden, M. J., and Olden, J. D. (2008). A management framework for preventing the secondary spread of aquatic invasive species. Can. J. Fish. Aquat. Sci. 65, 1512–1522. doi: 10.1139/f08-099
Vélez-Espino, L. A., McLaughlin, R. L., Jones, M. L., and Pratt, T. C. (2011). Demographic analysis of trade-offs with deliberate fragmentation of streams: control of invasive species versus protection of native species. Biol. Conserv. 144, 1068–1080. doi: 10.1016/j.biocon.2010.12.026
Vetter, B. J., Cupp, A. R., Fredricks, K. T., Gaikowski, M. P., and Mensinger, A. F. (2015). Acoustical deterrence of silver carp (Hypophthalmichthys molitrix). Biol. Invasions 17, 3383–3392. doi: 10.1007/s10530-015-0964-6
Vitule, J. R. S., Bornatowski, H., Freire, C. A., and Abilhoa, V. (2014). Extralimital introductions of Salminus brasiliensis (Cuvier, 1816) (Teleostei, Characidae) for sport fishing purposes: a growing challenge for the conservation of biodiversity in neotropical aquatic ecosystems. BioInvasions Rec. 3, 291–296. doi: 10.3391/bir.2014.3.4.11
Vitule, J. R. S., Freire, C. A., and Simberloff, D. (2009). Introduction of non-native freshwater fish can certainly be bad. Fish Fish. 10, 98–108. doi: 10.1111/j.1467-2979.2008.00312.x
Vitule, J. R. S., Skóra, F., and Abilhoa, V. (2012). Homogenization of freshwater fish faunas after the elimination of a natural barrier by a dam in Neotropics. Divers. Distrib. 18, 111–120. doi: 10.1111/j.1472-4642.2011.00821.x
Wagner, C. M., Stroud, E. M., and Meckley, T. D. (2011). A deathly odor suggests a new sustainable tool for controlling a costly invasive species. Can. J. Fish. Aquat. Sci. 68, 1157–1160. doi: 10.1139/f2011-072
Watters, G. T. (1996). Small dams as barriers to freshwater mussels (Bivalvia, Unionoida) and their hosts. Biol. Conserv. 75, 79–85. doi: 10.1016/0006-3207(95)00034-8
Welsh, S. A., and Loughman, Z. J. (2015). Upstream dispersal of an invasive crayfish aided by a fish passage facility. Manag. Biol. Invasions 6, 287–294. doi: 10.3391/mbi.2015.6.3.07
Weyl, O. L. F., Ellender, B. R., Ivey, P., Jackson, M. C., Tweddle, D., Wasserman, R. J., et al. (2017). “Africa: brown trout introductions, establishment, current status, impacts and conflicts,” in Brown Trout: Biology, Ecology and Management, ed. J. Lobon Cervia (Hoboken, NJ: Wiley), 621–641.
Weyl, O. L. F., Finlayson, B., Impson, N. D., Woodford, D. J., and Steinkjer, J. (2014). Threatened endemic fishes in South Africa’s cape floristic region: a new beginning for the rondegat river. Fisheries 39, 270–279. doi: 10.1080/03632415.2014.914924
Wingate, D. B. (2011). The successful elimination of cane toads, Bufo marinus, from an island with breeding habitat off Bermuda. Biol. Invasions 13, 1487–1492. doi: 10.1007/s10530-010-9925-2
Wittmann, M. E., Chandra, S., Reuter, J. E., Schladow, S. G., Allen, B. C., and Webb, K. J. (2012). The control of an invasive bivalve, Corbicula fluminea, using gas impermeable benthic barriers in a large natural lake. Environ. Manag. 49, 1163–1173. doi: 10.1007/s00267-012-9850-5
Woodford, D. J., Ivey, P., Jordaan, M. S., Kimberg, P. K., Zengeya, T., and Weyl, O. L. (2017). Optimising invasive fish management in the context of invasive species legislation in South Africa. Bothalia Afr. Biodivers. Conserv. 47, 1–9.
Xiong, W., Sui, X., Liang, S.-H., and Chen, Y. (2015). Non-native freshwater fish species in China. Rev. Fish Biol. Fish. 25, 651–687. doi: 10.1007/s11160-015-9396-8
Zielinski, D. P., McLaughlin, R., Castro-Santos, T., Paudel, B., Hrodey, P., and Muir, A. (2019). Alternative sea lamprey barrier technologies: history as a control tool. Rev. Fish. Sci. Aquac. 27, 438–457. doi: 10.1080/23308249.2019.1625300
Zielinski, D. P., McLaughlin, R. L., Pratt, T. C., Goodwin, R. A., and Muir, A. M. (2020). Single-stream recycling inspires selective fish passage solutions for the connectivity conundrum in aquatic ecosystems. Bioscience 70, 871–886. doi: 10.1093/biosci/biaa090
Zielinski, D. P., and Sorensen, P. W. (2015). Field test of a bubble curtain deterrent system for common carp. Fish. Manag. Ecol. 22, 181–184. doi: 10.1111/fme.12108
Zielinski, D. P., and Sorensen, P. W. (2016). Bubble curtain deflection screen diverts the movement of both Asian and common carp. North Am. J. Fish. Manag. 36, 267–276. doi: 10.1080/02755947.2015.1120834
Keywords: selective fragmentation, isolation management, intentional fragmentation, selective passage, non-native species, alien species, introduced species, exclusion barrier
Citation: Jones PE, Tummers JS, Galib SM, Woodford DJ, Hume JB, Silva LGM, Braga RR, Garcia de Leaniz C, Vitule JRS, Herder JE and Lucas MC (2021) The Use of Barriers to Limit the Spread of Aquatic Invasive Animal Species: A Global Review. Front. Ecol. Evol. 9:611631. doi: 10.3389/fevo.2021.611631
Received: 29 September 2020; Accepted: 18 January 2021;
Published: 09 February 2021.
Edited by:
Balázs Deák, Hungarian Academy of Science, HungaryReviewed by:
Ádám Lovas-Kiss, Hungarian Academy of Sciences, HungaryLaurie Alexander, Office of Research and Development, United States Environmental Protection Agency, United States
Copyright © 2021 Jones, Tummers, Galib, Woodford, Hume, Silva, Braga, Garcia de Leaniz, Vitule, Herder and Lucas. This is an open-access article distributed under the terms of the Creative Commons Attribution License (CC BY). The use, distribution or reproduction in other forums is permitted, provided the original author(s) and the copyright owner(s) are credited and that the original publication in this journal is cited, in accordance with accepted academic practice. No use, distribution or reproduction is permitted which does not comply with these terms.
*Correspondence: Peter E. Jones, p.e.jones@swansea.ac.uk
†These authors have contributed equally to this work