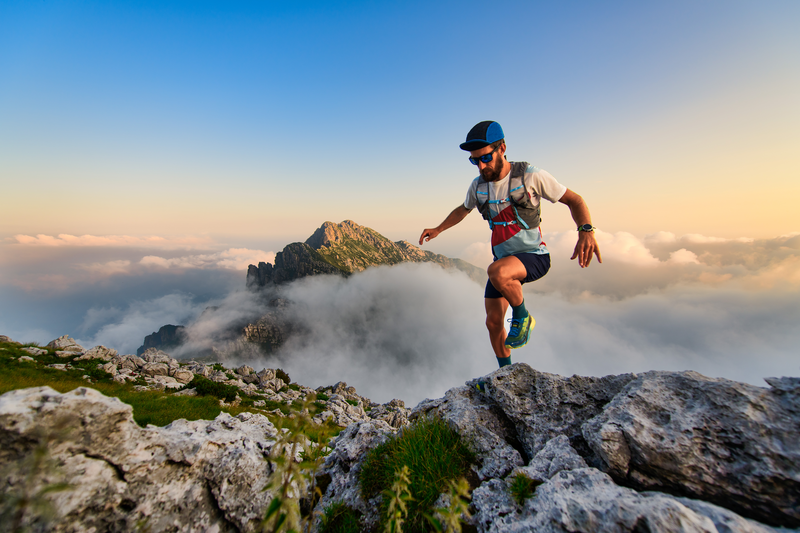
94% of researchers rate our articles as excellent or good
Learn more about the work of our research integrity team to safeguard the quality of each article we publish.
Find out more
ORIGINAL RESEARCH article
Front. Ecol. Evol. , 14 April 2021
Sec. Biogeography and Macroecology
Volume 9 - 2021 | https://doi.org/10.3389/fevo.2021.607023
This article is part of the Research Topic Habitat Modification and Landscape Fragmentation in Agricultural Ecosystems: Implications for Biodiversity and Landscape Multi-Functionality View all 14 articles
Managing regulating ecosystem services delivered by biodiversity in farmland is a way to maintain crop yields while reducing the use of agrochemicals. Because semi-natural habitats provide shelter and food for pest enemies, a higher proportion of semi-natural habitats in the landscape or their proximity to crops may enhance pest control in arable fields. However, the ways in which the spatial arrangement of these habitats affects the delivery of this beneficial ecosystem service to crops remains poorly known. Here, we investigated the relative effects of the amount of grassland in the landscape versus the distance to the nearest grassland on the predation rates of weed seeds and aphids into 52 cereal fields. We found that both seed and aphid predation levels increased with the proportion of grassland in a 500 m radius buffer while the distance to the nearest grassland displayed no effect. We show that increasing from 0 to 50% the proportion of grasslands in a 500 m radius, respectively, increased seed and aphid predation by 38 and 20%. In addition to the strong effect of the proportion of grassland, we found that seed predation increased with the proportion of forest fragments while aphid predation increased with the proportion of organic farming in the landscape. Overall, our results reveal that natural pest control in cereal crops is not related to the distance to the nearest grassland, suggesting that natural enemies are not limited by their dispersal ability. Our study indicates that maintaining key semi-natural habitats, such as grasslands, is needed to ensure natural pest control and support food production in agricultural landscapes.
Agricultural intensification, which manifests through a massive use of pesticides, low crop diversity or landscape simplification, has major impacts on biodiversity (Beketov et al., 2013; Maxwell et al., 2016). Since biodiversity is one of the main determinants of ecosystem productivity (Tilman et al., 2014), this decline may threaten the long-term sustainability of food production (Cardinale et al., 2012). A paradigm shift is therefore needed to meet the double challenge of maintaining – and in some cases, enhancing – food production and minimizing negative environmental impacts (Godfray et al., 2010; Garibaldi et al., 2017). One possible way forward is to increase regulating and supporting ecosystem services in agricultural fields to replace agrochemical inputs (Bommarco et al., 2013).
Among key functions supported by biodiversity, natural pest control, i.e., the suppression of pest species by their predators, is a major one. Natural pest control can benefit farmers by limiting crop yield loss (Maas et al., 2013; Bengtsson, 2015; Schneider et al., 2015; Ali et al., 2018) and pesticide use (Naranjo et al., 2015; Huang et al., 2018). High diversity in natural enemy communities is often associated with high levels of pest suppression (Letourneau et al., 2009). Indeed, a large body of research has focused on understanding the mechanisms operating at multiple spatial scales, from the plant to the landscape, driving the presence of natural enemies and the level of pest suppression in agroecosystems (Bianchi et al., 2006; Rusch et al., 2010, 2016; Chaplin-Kramer et al., 2011). While these studies have demonstrated that landscape heterogeneity is a major predictor of the level of natural pest control, the relative importance of landscape composition (i.e., amount of habitats) and of its configuration (i.e., spatial arrangement of habitats) on this key function remains poorly explored (but see Martin et al., 2019).
Population and community dynamics at the landscape scale can be viewed as a “source-sink” dynamic between habitats, under which both landscape configuration (e.g., fragmentation) and composition (e.g., amount of habitat) are strong drivers of biodiversity dynamics and ecosystem functioning (Haddad et al., 2017). These effects act in concert over multiple spatial scales and are mediated by dispersal abilities of species, rates of colonization and local extinction (Haddad et al., 2015, 2017; Hanski, 2015; Watling et al., 2020). In agricultural landscapes, semi-natural habitats such as woodlands, hedgerows, or grasslands are important sources of natural enemies because they provide alternative sources of food or hosts, refuges from disturbances and overwintering and breeding sites for a large range of species (Landis et al., 2000; Veres et al., 2013; Sarthou et al., 2014). Natural enemies disperse from semi-natural habitats to crops, and their spatial dynamics can be explained by the distribution of source habitats in the landscape through complementation or supplementation processes (Dunning et al., 1992; Rand et al., 2006; Blitzer et al., 2012). The importance of semi-natural habitats for natural pest control mainly comes from studies exploring how the amount of semi-natural habitats in the landscape enhances the activity of natural enemies or the amount of pest control in arable fields (Rusch et al., 2013, 2016; Veres et al., 2013; Haan et al., 2020). However, how the spatial arrangement of semi-natural habitats affects natural pest control remains poorly explored and the few studies investigating the effect of the distance of semi-natural habitats on natural pest control show idiosyncratic effects (Farwig et al., 2009; Thomson and Hoffmann, 2013; González et al., 2017; Lindgren et al., 2018; Aristizabal and Metzger, 2019). In addition, due to their strong correlation in the landscape (Fahrig et al., 2011), the relative importance of composition and configuration of semi-natural habitats on natural enemies and pest control services remains largely unknown (Haan et al., 2020).
Distance of arable crops to semi-natural habitats is expected to be a critical driver of the spillover of natural enemies. The distances are highly species-dependent because dispersal abilities vary from poor dispersal abilities (<1,000 m) for ground-dwelling species such as carabids or spiders, to high dispersal abilities for flying predators, such as ladybirds or syrphids (Schmidt et al., 2005; Saska et al., 2008; Jauker et al., 2009; Maes et al., 2014). Dispersal capacities further interact with the spatial distribution of semi-natural elements: natural enemies with “passive” dispersal (sensu Holt, 1985) forage near their semi-natural habitat source, and natural pest control increases with the proximity to the source habitat (Holzschuh et al., 2010; Haenke et al., 2014). Conversely, natural enemies with “active” dispersion depend less on adjacent natural habitats. As such, identifying the scale of effects of habitat sources for natural enemy communities involved in the delivery of pest control services is of major importance to understand the functioning of agricultural landscapes.
Among semi-natural habitats, grasslands are key habitats for many species, including natural enemy species (Bengtsson et al., 2019) and contribute to many ecosystem services in agricultural landscapes (Habel et al., 2013; Bengtsson et al., 2019). Grasslands shelter more natural enemies in early spring than any other type of semi-natural habitats and are therefore a major source of predators in agricultural landscapes (Albrecht et al., 2010; Sarthou et al., 2014; Werling et al., 2014). However, in most studies, grasslands are aggregated with other types of semi-natural habitats, thus their individual effect on pest control services remains poorly understood (Holland et al., 2017). In the few cases where the effect of grasslands was specifically considered, grassland proportion was shown to increase natural enemy abundance or pest predation, and reduce pest abundance in crops (Rusch et al., 2011; Trichard et al., 2013; Alignier et al., 2014; Koh and Holland, 2015; Petit et al., 2017). The distance effect of grasslands on natural pest control services is even less informed, but proximity to grasslands increases pest predation or pest abundance in adjacent crops (French et al., 2001; Badenhausser et al., 2020). However, to our knowledge, the relative importance of grassland proportion in landscape versus distance-decay effects on pest control has never been studied simultaneously in a single study.
The aim of this study is to disentangle the relative effects of the amount of grassland from the effect of distance to the nearest grassland on natural pest control in focal cereal fields. We used predation sentinel cards, and focused on predation rates of two different types of prey, i.e., aphids and weed seeds, which are known to incur cereal yield loss (Ali et al., 2018; Adeux et al., 2019). Both aphids and seeds are predated by a large number of predators, with highly variable dispersal abilities and diets. These include carabid beetles, birds and small rodents for seeds (Bohan et al., 2011; Eraud et al., 2015; Tschumi et al., 2018a, b); ladybirds, carnivorous ground beetles, spiders or syrphid larvae for aphids (Schmidt et al., 2005; Saska et al., 2008; Jauker et al., 2009; Maes et al., 2014). However, in most cases, the identity of natural enemies responsible for pest suppression remain unknown (Furlong and Zalucki, 2010). We hypothesized that if natural pest control services are driven by local and passive diffusion, then the level of pest control should be more affected by the distance to the nearest grassland than by its proportion in the landscape. On the contrary, if natural pest control services are mainly driven by complementation processes mediated by active dispersal from sources habitats to crops, then grassland proportion is more important than the distance to the nearest grassland.
The study took place in 2016 in the Long Term Social-Ecological Research site “Zone Atelier Plaine & Val de Sèvre” (ZAPVS), an agricultural landscape located in central western France (Bretagnolle et al., 2018), in the Nouvelle Aquitaine Region (46.23°N, 0.41 W). Land use is surveyed annually in each of the c. 13,000 fields of the study site (435 km2 managed by c. 450 farms), and is stored in a GIS database (Bretagnolle et al., 2018). The area is mainly used for intensive cereal farming. Grasslands (including meadows and long-term set-a-sides, but excluding alfalfa) represent only 7.1% of the ZAPVS, while cereals, mainly winter wheat varieties, are the dominant crop (37.1% cover). The other main crops consist of oilseed rape (7.1%), sunflower (7.4%) and maize (8.9%). Forests cover about 13.7% of the ZAPVS (including the large Chizé forest, Figure 1). The remaining area is comprized of other crops such as alfalfa, pea, linseed or ryegrass, and urbanized surfaces. Crop fields have an average size of 5.7 ha, ranging from 0.4 to 35.2 ha.
Figure 1. The left panel shows a map of the study area with grassland fields in green and the 52 selected cereal fields in red. The right panel shows the field experimental design. Seed cards were put on the same position as aphid card spaced to 40 centimeters apart. Chizé forest is in brown.
Two landscape gradients of interest were particularly studied: the first gradient, related to landscape configuration, was the distance between the barycentre of sentinel card position and the nearest grassland field (represented by its border), while the second gradient was the proportion of grassland in the landscape at a given buffer size (radius). We selected 52 cereal focal fields (45 winter wheat and 7 winter barley fields) along these two landscape gradients which could influence the predation rates of aphids and weed seeds in these fields (Rusch et al., 2010, 2013). The fields were randomly selected with a moving window aiming at lowering the correlation between two landscape gradients of interest and making them independent (see Fahrig et al., 2011; Sirami et al., 2019). Correlation between the distance to the nearest grassland and the proportion grassland never exceeded 0.52 whatever the radius (Supplementary Figure 1 shows correlations between distance to the nearest grassland and proportion of grassland for the different buffer scales). Distance to closest grassland varied from 25 to 972 m (average: 289.9 m, see Figure 1), while the area of the nearest grassland varied from 0.26 to 7.6 ha (average: 2 ha). In addition to these two landscape metrics, we also calculated the proportions of crops organically farmed, forests and hedgerows, since these landscape metrics are known to affect natural pest control as well as natural enemies presence and abundance (Farwig et al., 2009; Rusch et al., 2010; Muneret et al., 2019). All these landscape metrics were calculated at six different radii ranging from 250 to 1,500 m around the barycentre of sentinel prey card position (see below, Martin et al., 2015; Rusch et al., 2016; Tschumi et al., 2018a). Table 1 shows habitat proportions in the six different buffer sizes. Correlations between organic farming, hedgerow, forest and grassland metric were lower than 0.6 (see Supplementary Figure 2 for all correlations between landscape metrics).
We used sentinel preys to estimate natural pest control potential as it is a standard and efficient method related to predator activity (Lövei and Ferrante, 2017; Boetzl et al., 2020a) and pest regulation (Perez-Alvarez et al., 2019). In each cereal field, we measured natural pest control as the realized predation rate on two common pests: the aphid Acyrthosiphon pisum (Ximenez-Embun et al., 2014) which is commonly used to estimate aphid predation in cereal fields (Winqvist et al., 2011; Ricci et al., 2019), and the weed Viola arvensis (Petit et al., 2017) which is relatively common in cereal fields (Bourgeois et al., 2020). Weed seeds were bought at Herbiseed (Reading, United Kingdom). Aphids colonies were bought at Monster-Souris (Nantes, France) and raised on peas (Pisum sativum) in the laboratory from the beginning to the end of the sentinel card experiment under ambient temperature and natural day-night cycle. Predation rates were quantified using sentinel cards, on which either three dead aphids or 10 weed seeds were glued (Aero’Colle, Cléopâtre) on the rough side of 5 cm × 6 cm sandpaper cards (Boetzl et al., 2020a; McHugh et al., 2020, Figure 1). Cards were placed 24 h in the freezer at −20°C before the experiment to avoid attractive or deterrent effect of predator due to glue evaporation (Boetzl et al., 2020a). Two parallel transects of 21 m were selected per field, distant by at least 10 m from each other to ensure independence between transects. To limit potentially confounding field margin effects, we set up transects at least two tractor bays (i.e., approximately 25–35 m) away from the field border, (Figure 1). On each transect, four cards of each prey type were set on the ground, held a pin (Winqvist et al., 2011; Boetzl et al., 2020a), from the 7th April to 30th June (i.e., from heading to grain ripening of cereals), each being 7 m apart (Figure 1; Ricci et al., 2019; Boetzl et al., 2020b). Seed and aphid cards were put on the same position on the transect and spaced 40 cm apart. Cards were folded in half to provide a tent-like with aphids facing to the ground to limit the deterioration of the aphid or seed gluing by climatic conditions (rain, sun, wind…) as advised by Winqvist et al. (2011). The position of cards in the fields was recorded with a GPS. The barycentre of global card positions in a given focal field was used as the center of buffer and to measure the distance to the nearest grassland. Each field was sampled twice over the spring and summer seasons to account for temporal variation of predation rates throughout the season (Ximenez-Embun et al., 2014). A total 832 cards per prey type were set up (i.e., 8 cards per field and per session). On average, 44.1 days (range: 28–56 days) separated the two sampling sessions for a given field. Seed cards were left 4 days in the field whereas aphid ones were collected after 1 day (24 h) because of much higher predation rates (see results, Ximenez-Embun et al., 2014). This period is standard in studies using predation sentinel cards (Lövei and Ferrante, 2017; Perez-Alvarez et al., 2019; Boetzl et al., 2020a). A total of 817 seeds cards and 818 aphid cards were recovered from the 832 initially installed, 15 and 14 were lost, respectively. In one field, one session was lost for both seed and aphid cards. We counted the number of aphids or seeds remaining on the cards to estimate predation rates, and then removed cards from fields.
To disentangle the importance of proportion of grassland in the landscape from the importance of the distance to the nearest grassland on aphid and seed predation rates in cereal fields, we used Generalised Linear Models (GLM). For weed seed predation, we used the ratio of the number of weed seed predated on the total number of seeds placed in the fields as response variable and used a Gaussian error distribution. For aphid predation, we used 1- the number of aphids predated on the total number of aphids placed in the fields (i.e., the number of non-predated aphids) due to the very high predation rates of aphids (49% fields have more of 90% aphids predated) and used a Poisson-Gamma distribution from the family Tweedie (Dunn and Smyth, 2018). This distribution handles zero values uniformly with positive and continuous values thanks to a power variance function and a log-link function (Lecomte et al., 2013). This distribution performed better than models such as hurdle or negative-binomial models with zero-inflated data (Lecomte et al., 2013; Saha et al., 2020).
First, we investigated how predation rate (seed or aphid and response variable) was explained by the distance to the nearest grassland or the proportion of grasslands. As the correlation of predation rates between the two sessions was very weak (Pearson correlation test between the two sessions, ρ = 0.09, t = 0.62, df = 49, p-value = 0.54 for seeds, and ρ = 0.15, t = 1.06, df = 49, p-value = 0.29 for aphids), we used the two sessions as independent observations and included the Julian date in the model as a co-variate to account for season. Six models per pest type were fitted using the two landscape variables calculated in six-buffer radius size (Table 1). The six models were compared using the Akaike Information Criterion (AIC; Burnham and Anderson, 2002) and we retained the best model for each response variable, i.e., the model with the lowest AIC. The effect of the interaction between grassland proportion and the distance to the nearest grassland was also tested by adding an interaction term to the best model, and was removed in next models if it was not significant.
Since predator activity may depend on weather, e.g., rainfall potentially modifies predator activity (Zaller et al., 2014), we included weather variables in the models. We added average rainfall per day during the experiment as co-variables to these models, calculated over 4 days for seed predation measures (average 1.76 mm per day, range: 0–8.26 mm) and over 2 days for aphid predation measures (average 1.73 mm per day, range: 0–6 mm), to consider rainfalls from the first day where cards were deposited in the fields to the day when they were retrieved. We did not consider average temperature as an explanatory variable in our models as temperature was strongly correlated to Julian days (Pearson correlation test between Julian days and average temperature on 4 days, ρ = 0.8, t = 13.32, df = 102, p-value < 0.001, Pearson correlation test between Julian days and average temperature over 2 days, ρ = 0.76, t = 11.65, df = 102, p-value < 0.001). All weather metrics were obtained from a weather station located in the city of Niort, within the study zone, and data were downloaded from United States National Oceanic and Atmospheric Administration.
We further tested whether a grassland that is small in surface but close to the focal crop could be equivalent – in terms of source habitat for natural enemies – to a grassland that is large but distant. Thus, in a separate analysis, we incorporated the area of the nearest grassland by replacing the distance to the nearest grassland by the distance to the nearest grassland divided by its area. Finally, because the proportions of hedgerows, forests and organic farming in the landscape are known to affect natural pest control, we explored their potential effects (Farwig et al., 2009; Rusch et al., 2010; Muneret et al., 2019) in additional models. We added these three metrics as co-variates in the best models for aphid and seed predation. Adding these landscape metrics variables allows quantifying their individual effect on predation rates but also their additional effect with grassland effects (both proportion and distance).
All analyses were performed using the software R (R Core Team, 2015), version 3.6.2 with “stats” package for GLMs and AIC estimation, “tweedie” and “statmod” for GLM models with Tweedie distribution (Giner and Smyth, 2016; Dunn, 2017). All explanatory variables were centered and reduced to facilitate the interpretation of coefficients.
Overall, 1998 (of 2454) aphids and 3446 (of 8170) seeds were removed across the two sessions in the 52 focal cereal fields. Seed predation rate was in average of 0.42 ± 0.29 (median: 0.42) while aphid predation rate was 0.81 ± 0.24 (median = 0.88). Seed and aphid predation rates were positively correlated (Pearson correlation, ρ = 0.2, t = 2, df = 100, p-value = 0.048).
The best-fit models were models including explanatory variables calculated in the 500 and 750 m radius (ΔAIC < 2) both for seed and aphid predation rates (Supplementary Figures 3A,B). Effect of grassland was similar between models with landscape variables estimated at 500 and 750 m (Table 2 and Supplementary Table 1), but models with landscape variables calculated at 500 m had lowest AIC for both aphid and seed, thus were chosen. Best models, respectively, explained 15.9 and 15.5% of the variance in seed and aphid predation rates. The proportion of grassland had a positive effect on seed and aphid predation rates, contrasting with the distance to the nearest grassland which had no effect on the predation rates of either prey species (Table 2 and Figures 2A,B,E,F). Predation rates of seeds and aphids, respectively, increased by 42 and 21% along the gradient of grassland proportion (proportion of grassland ranged from 0 to 50% in the 500 m radius). No significant interaction was detected between distance to the nearest grassland and grassland proportion (seeds: Estimate = −0.082, p-value = 0.49, and aphids: Estimate = −0.31, p-value = 0.6; Supplementary Table 2). Including the area of the nearest grassland in the models did not affect the influence of its distance on predation rates for either preys (seeds : Estimate = −0.012, p-value = 0.7, and aphids: Estimate = −0.19, p-value = 0.2; Supplementary Table 3).
Table 2. Summary of generalized linear models for seed and aphid predation rates explained by date (Julian day), average rainfall, and landscape metrics.
Figure 2. Predation rates for seed (A–D) and aphid (E–H) in relation to distance to the nearest grassland (A,E), proportion of grassland (B,F), forest (C,D) and organic farming (D,H) in a buffer size of 500 m. The two sampling sessions are combined in a single analysis (see section “Materials and Methods”). Black lines represent the relationships as predicted by generalized linear models, among which solid lines represent significant effects, whereas non-significant effects are dashed. The gray areas represent the 95% CI.
Adding the proportion of forest, hedgerow and organic farming in the landscape additionally explained 8–10% of the variance in predation rates of seeds and aphids (R2 = 24% for seeds and R2 = 22.3% for aphids). The proportion of forests increased seed predation rates (Table 2 and Figures 2C,G), while the proportion of organic farming increased aphid predation rates (Table 2 and Figures 2D,H). The effect of grassland proportion was partially reduced with the inclusion of other landscape metrics compared with the simpler models (Table 2). In such models, predation rates of seeds and aphids, respectively, increased by 38 and 20% along the gradient of grassland proportion (from 0 to 50% in a 500 m radius, Figures 2B,F). Finally, predation rates of seeds and aphids increased with Julian days and marginally with rainfall (Table 2).
Our study explores the interactive effects of the amount and spatial configuration of grasslands on the predation rates of two types of cereal prey in agricultural landscapes. Our analyses indicate that the proportion of grassland rather that the distance to the nearest grassland increased both seed and aphid predation rates in cereal fields with, respectively, an increase of 38 and 20% from 0 to 50% of grassland in a 500 m-radius buffer. In addition, seed predation increased with the proportion of forests while aphid predation increased with the proportion of organic farming in the surrounding landscape.
The relative importance of habitat composition and configuration at the landscape scale to maintain biodiversity and ecosystem functions is highly debated although few studies examined their relative effects on ecosystem functions and services (Watling and Donnelly, 2006; Haddad et al., 2017; Bueno and Peres, 2019; Watling et al., 2020). A recent meta-analysis regrouping 35 study sites from different biomes showed that in most cases, composition (in the form of proportion) was more important than configuration to maintain species richness whatever the taxon identity (Watling et al., 2020). Indeed, both the composition and the configuration of agricultural landscapes shape natural enemy communities and pest damage (Martin et al., 2019; Haan et al., 2020), and more specifically the amount of semi-natural habitats enhances the activity of natural enemies or amount of pest control (Rusch et al., 2013, 2016; Veres et al., 2013; Haan et al., 2020). In most cases, the spatial arrangement had no effect on natural enemies or pest control (Farwig et al., 2009; Thomson and Hoffmann, 2013; González et al., 2017; Lindgren et al., 2018). However, only few studies included both composition and configuration in their analyses (González et al., 2017) as they are strongly correlated in most ecosystems (Fahrig, 2013). Our landscape selection method allowed to disentangle the effect of grassland proportion from the effect of distance to the nearest grassland. Our results suggest that grassland proportion is more important than their distance to crops to increase aphid and seed predation in cereal fields, indicating that the effect of landscape composition on communities also holds for ecosystem functions (Lamy et al., 2016; Duarte et al., 2018). Our results are consistent with previous studies which showed that aphid and seed predation in cereal fields increase with surrounding grassland proportion (Trichard et al., 2013; Alignier et al., 2014; Petit et al., 2017). Similarly, seed and aphid predation in midfield islets did not decrease with distance to grassland (Lindgren et al., 2018). However, none of these studies accounted for both the configuration and the amount of grassland in their experiments, and grasslands were usually aggregated with other types of semi-natural habitats in a large majority of studies (Holland et al., 2017). Only González et al. (2017) integrated both aspects and showed comparable results with stinkbug egg-predation on soybean crops which increases with forest proportion rather their distance to crops. The landscape context of our study region may, however, limit the extrapolation of our results to other farmland landscapes. It should be noted that only three cereal fields were more of 750 m away from a grassland (Supplementary Figure 1), stressing that additional experiments may be needed with cereal fields more than 1 km from closest grassland to confirm our results. In landscape contexts with few grasslands, and therefore higher distances to the nearest grassland, one may expect a higher effect of distance. Moreover, landscapes with high amount of grassland (>15%) were scarce in our study (7 fields on 52), therefore limiting the scope of our conclusions. Conducting this study with the same experimental design over a large range of landscape gradients and in different pedoclimatic contexts would certainly help to consolidate our conclusions. In addition to the effect of grassland proportion, we found that forest proportion increased seed predation rates while organic farming proportion enhanced aphid predation rates, which suggests a complementation effect of habitat for natural enemies. Diverse semi-natural habitats in agricultural landscape provide alternative prey or additional shelter, hence maintain higher diversity of natural enemies (Tscharntke et al., 2007) and pest predation (Letourneau et al., 2009).
The dispersal and behavioral capacity of natural enemies likely determine the relative importance of composition and configuration of semi-natural habitats for natural pest control (Keinath et al., 2017). We found that the scale of effect of grassland proportion on pest control is at spatial extent ranging from 500 to 750 m radius, rather than small spatial extent. This spatial extent is larger than the one found in a previous meta-analysis which showed that pest predation is best explained by landscapes metrics estimated at small scales (250 m, Karp et al., 2018). High dispersal capacity had been predicted as a key trait of natural enemies effectiveness to remove pests (Bianchi et al., 2010). Natural enemies with high dispersal capacity are less susceptible to landscape heterogeneity (Bianchi et al., 2010). Among seed predators, small mammals, ants and carabids have dispersal capacities ranging from 500 to 750 m (Wegner and Merriam, 1990; Holland et al., 2004; Zumeaga et al., 2021). Moreover, small mammals, ants and carabids can benefit from grasslands (Fischer and Schröder, 2014; Petit et al., 2017; Assis et al., 2018) as well as forest patches (Wegner and Merriam, 1990; Holland and Fahrig, 2000; Assis et al., 2018) to maintain their population and may explain why seed predation rates increase with forest proportion. For aphids, spiders and carabids are natural enemies with dispersal capacities that match the 500 – 750 m spatial extent (Holland et al., 2004; Schmidt and Tscharntke, 2005; Maes et al., 2014) and appear as potential candidates involved in aphid predation rates here. Abundances of these species were previously found to increase with grassland proportion in agricultural landscapes (Koh and Holland, 2015; Petit et al., 2017; Badenhausser et al., 2020). There is also some evidence that carabids and aphids control by their natural enemies in cereal crops benefit from the proportion of organic farming in surrounding landscape (Caro et al., 2016; Diekötter et al., 2016; Djoudi et al., 2018; Muneret et al., 2019) which may explain why aphid predation rates increased with amount of fields under organic farming. A camera-trap experiment may help to identify the natural enemies responsible for aphid and seed predation (Petersen and Woltz, 2015); especially because the ground position of aphid cards in our study may have excluded predation from aerial predators such as birds or ladybirds. Including such predators might change the spatial extent of the landscape effects detected in our study.
Finally, we found that meteorological conditions modified predation rates. Predation rates of both preys increased with Julian days, but only aphid predation rates increased with rainfall, though we could not disentangle the respective effects of Julian days and average temperature which were strongly correlated. There is clear evidence that both variables affect predator activity: predator dynamics increase with Julian days (Caro et al., 2016), and predator activity and pest predation increase with average temperature (Korenko et al., 2010). It is therefore likely that the increase of predation rates observed in our study resulted from seasonal effect such as increase in average temperature. Predator activity or abundance was also found to be higher with rainfall (Irmler, 2003; Lundgren et al., 2006; Zaller et al., 2014; Wróbel and Bogdziewicz, 2015), confirming our results on aphid predation rates. However, the absence of relationship between seed predation rate and rainfall may suggest that different natural enemies may contribute to seed and aphid predation and/or that the effect of rainfall is less pronounced on a 4-day (seed predation) than on a 2-day (aphid predation) period. Further studies are now needed to determine how natural enemies of each prey behave under contrasted weather conditions. Similarly, it would be interesting to consider prey abundance or pesticide applications as covariables in our models because these factors can affect natural pest control potential. A high prey abundance can dilute natural pest control by distracting predators from sentinel cards (Lövei and Ferrante, 2017) while pesticide applications can buffer landscape effects (Ricci et al., 2019). These effects need further investigations to fully understand the mechanisms driving natural pest control in agricultural landscapes.
In this study, increasing grassland proportion at landscape scale (500 m buffer radius) by 50% resulted in 38 and 20% increase in seed and aphid predation rates. Conversely, the nearest grassland, whatever its area, displayed no statistical effect on predation rates. These results highlight the importance of maintaining sufficient amount of grassland habitats in agricultural landscapes to enhance natural pest control services, which might help reducing pest pressure and pesticides applications in agricultural landscapes (Naranjo et al., 2015; Huang et al., 2018). However, a more mechanistic understanding of the effect of landscape context on the delivery of natural pest control and its impact on yield and farmers’ income is crucially needed (Schneider et al., 2015; Ali et al., 2018). Pest abundance can also vary with landscape structure, and higher predation rates of pests may not lead to lower abundance and damage (Tscharntke et al., 2016). Consequently, the study of the relationships between pest control potential, as measured in our study, and actual pest abundance deserves more attention. In addition, increasing grassland area at the landscape scale will result in reducing arable crop area; and this might affect farmers’ income. Except for livestock farmers, grasslands have no direct economic benefits, at least under the current European common agriculture policy. Such key topic should now be explored to determine the optimal landscape configuration reducing pest infestation and damage, while maintaining crop yield and farmer profitability (Zhang and Swinton, 2009).
The datasets presented in this study can be found in online repositories. The names of the repository/repositories and accession number(s) can be found below: 10.5281/zenodo.4555503.
AR, SG, and VB designed the study. TP and CC prepared the data and conducted the statistical analysis and wrote the first draft of the manuscript. All authors substantially contributed to revisions.
This research was funded through the 2013–2014 BiodivERsA/FACCE-JPI joint call for research proposals (project ECODEAL), with the national funders ANR, BMBF, FORMAS, FWF, MINECO, NWO, and PT-DLR; the ANR IMAGHO (ANR-18-CE32-0002) and the INRAE MP SMACH project “ESPACE.” TP is partially funded by the Nouvelle Aquitaine Région (HARMONIE project).
The authors declare that the research was conducted in the absence of any commercial or financial relationships that could be construed as a potential conflict of interest.
We would like to express our thanks to Jean-Luc Gautier, Edoardo Tedesco, and Thierry Fanjas-Mercere for their help with sentinel cards experiment.
The Supplementary Material for this article can be found online at: https://www.frontiersin.org/articles/10.3389/fevo.2021.607023/full#supplementary-material
Adeux, G., Vieren, E., Carlesi, S., Bàrberi, P., Munier-Jolain, N., and Cordeau, S. (2019). Mitigating crop yield losses through weed diversity. Nat. Sustain. 211, 1018–1026. doi: 10.1038/s41893-019-0415-y
Albrecht, M., Schmid, B., Obrist, M. K., Schüpbach, B., Kleijn, D., and Duelli, P. (2010). Effects of ecological compensation meadows on arthropod diversity in adjacent intensively managed grassland. Biol. Conserv. 143, 642–649. doi: 10.1016/j.biocon.2009.11.029
Ali, A., Desneux, N., Lu, Y., and Wu, K. (2018). Key aphid natural enemies showing positive e ff ects on wheat yield through biocontrol services in northern China. Agric. Ecosyst. Environ. 266, 1–9. doi: 10.1016/j.agee.2018.07.012
Alignier, A., Raymond, L., Deconchat, M., Menozzi, P., Monteil, C., Sarthou, J. P., et al. (2014). The effect of semi-natural habitats on aphids and their natural enemies across spatial and temporal scales. Biol. Control 77, 76–82. doi: 10.1016/j.biocontrol.2014.06.006
Aristizabal, N., and Metzger, J. P. (2019). Landscape structure regulates pest control provided by ants in sun coffee farms Natalia Aristizábal. J. Appl. Ecol. 56, 21–30. doi: 10.1111/1365-2664.13283
Assis, D. S., Dos Santos, I. A., Ramos, F. N., Barrios-Rojas, K. E., Majer, J. D., and Vilela, E. F. (2018). Agricultural matrices affect ground ant assemblage composition inside forest fragments. PLoS One 13:e197697. doi: 10.1371/journal.pone.0197697
Badenhausser, I., Gross, N., Mornet, V., Roncoroni, M., Saintilan, A., and Rusch, A. (2020). Increasing amount and quality of green infrastructures at different scales promotes biological control in agricultural landscapes. Agric. Ecosyst. Environ. 290:106735. doi: 10.1016/j.agee.2019.106735
Beketov, M. A., Kefford, B. J., Schafer, R. B., and Liess, M. (2013). Pesticides reduce regional biodiversity of stream invertebrates. Proc. Natl. Acad. Sci. U.S.A. 110, 11039–11043. doi: 10.1073/pnas.1305618110
Bengtsson, J. (2015). Biological control as an ecosystem service: partitioning contributions of nature and human inputs to yield. Ecol. Entomol. 40, 45–55. doi: 10.1111/een.12247
Bengtsson, J. B., Ullock, J. M. B., Goh, B. E., Verson, C. E., Verson, T. E., Onnor, T. O. C., et al. (2019). Grasslands — more important for ecosystem services than you might think. Ecosphere 10:e02582. doi: 10.1002/ecs2.2582
Bianchi, F. J. J., Booij, C. J. H., and Tscharntke, T. (2006). Sustainable pest regulation in agricultural landscapes: a review on landscape composition, biodiversity and natural pest control. Proc. Biol. Sci. 273, 1715–1727. doi: 10.1098/rspb.2006.3530
Bianchi, F. J. J. A., Schellhorn, N. A., Buckley, Y. M., and Possingham, H. P. (2010). Spatial variability in ecosystem services: simple rules for predator-mediated pest suppression. Ecol. Appl. 20, 2322–2333. doi: 10.1890/09-1278.1
Blitzer, E. J., Dormann, C. F., Holzschuh, A., Klein, A. M., Rand, T. A., and Tscharntke, T. (2012). Spillover of functionally important organisms between managed and natural habitats. Agric. Ecosyst. Environ. 146, 34–43. doi: 10.1016/j.agee.2011.09.005
Boetzl, F. A., Konle, A., and Krauss, J. (2020a). Aphid cards – Useful model for assessing predation rates or bias prone nonsense? J. Appl. Entomol. 144, 74–80. doi: 10.1111/jen.12692
Boetzl, F. A., Schuele, M., Krauss, J., and Steffan-Dewenter, I. (2020b). Pest control potential of adjacent agri-environment schemes varies with crop type and is shaped by landscape context and within-field position. J. Appl. Ecol. 57, 1482–1493. doi: 10.1111/1365-2664.13653
Bohan, D. A., Boursault, A., Brooks, D. R., and Petit, S. (2011). National-scale regulation of the weed seedbank by carabid predators. J. Appl. Ecol. 48, 888–898. doi: 10.1111/j.1365-2664.2011.02008.x
Bommarco, R., Kleijn, D., and Potts, S. G. (2013). Ecological intensification: harnessing ecosystem services for food security. Trends Ecol. Evol. 28, 230–238. doi: 10.1016/j.tree.2012.10.012
Bourgeois, B., Gaba, S., Plumejeaud, C., and Bretagnolle, V. (2020). Weed diversity is driven by complex interplay between multi-scale dispersal and local filtering: multi-scale drivers of weed diversity. Proc. R. Soc. B Biol. Sci. 287:20201118. doi: 10.1098/rspb.2020.1118rspb20201118
Bretagnolle, V., Berthet, E., Gross, N., Gauffre, B., Plumejeaud, C., Houte, S., et al. (2018). Biodiversity, ecosystem services and citizen science: the value of long term monitoring in farmland landscapes for sustainable agriculture. Sci. Total Environ. 627, 822–834.
Bueno, A. S., and Peres, C. A. (2019). Patch-scale biodiversity retention in fragmented landscapes: Reconciling the habitat amount hypothesis with the island biogeography theory. J. Biogeogr. 46, 621–632. doi: 10.1111/jbi.13499
Burnham, K. P., and Anderson, D. R. (2002). Model Selection and Multimodel Inference: A Practical Information-Theoretic Approach, 2nd Edn. Berlin: Springer, doi: 10.1016/j.ecolmodel.2003.11.004
Cardinale, B. J., Duffy, J. E., Gonzalez, A., Hooper, D. U., Perrings, C., Venail, P., et al. (2012). Biodiversity loss and its impact on humanity. Nature 489, 326–326. doi: 10.1038/nature11373
Caro, G., Marrec, R., Gauffre, B., Roncoroni, M., Augiron, S., and Bretagnolle, V. (2016). Multi-scale effects of agri-environment schemes on carabid beetles in intensive farmland. Agric. Ecosyst. Environ. 229, 48–56. doi: 10.1016/j.agee.2016.05.009
Chaplin-Kramer, R., O’Rourke, M. E., Blitzer, E. J., and Kremen, C. (2011). A meta-analysis of crop pest and natural enemy response to landscape complexity. Ecol. Lett. 14, 922–932. doi: 10.1111/j.1461-0248.2011.01642.x
Diekötter, T., Wamser, S., Dörner, T., Wolters, V., and Birkhofer, K. (2016). Organic farming affects the potential of a granivorous carabid beetle to control arable weeds at local and landscape scales. Agric. For. Entomol. 18, 167–173. doi: 10.1111/afe.12150
Djoudi, E. A., Marie, A., Mangenot, A., Puech, C., Aviron, S., Plantegenest, M., et al. (2018). Farming system and landscape characteristics differentially affect two dominant taxa of predatory arthropods. Agric. Ecosyst. Environ. 259, 98–110. doi: 10.1016/j.agee.2018.02.031
Duarte, G. T., Santos, P. M., Cornelissen, T. G., Ribeiro, M. C., and Paglia, A. P. (2018). The effects of landscape patterns on ecosystem services: meta-analyses of landscape services. Landsc. Ecol. 33, 1247–1257. doi: 10.1007/s10980-018-0673-5
Dunn, P. K., and Smyth, G. K. (2018). Generalized Linear Models With Examples in R. Berlin: Springer, doi: 10.1007/978-1-4419-0118-7
Dunning, J. B., Danielson, B. J., Pulliam, H. R., and Ecology, I. (1992). Nordic society oikos ecological processes that affect populations in complex landscapes. Oikos 65, 169–175. doi: 10.2307/3544901
Eraud, C., Cadet, E., Powolny, T., Gaba, S., Bretagnolle, F., and Bretagnolle, V. (2015). Weed seeds, not grain, contribute to the diet of wintering skylarks in arable farmlands of Western France. Eur. J. Wildl. Res. 61, 151–161. doi: 10.1007/s10344-014-0888-y
Fahrig, L. (2013). Rethinking patch size and isolation effects: the habitat amount hypothesis. J. Biogeogr. 40, 1649–1663. doi: 10.1111/jbi.12130
Fahrig, L., Baudry, J., Brotons, L., Burel, F. G., Crist, T. O., Fuller, R. J., et al. (2011). Functional landscape heterogeneity and animal biodiversity in agricultural landscapes. Ecol. Lett. 14, 101–112. doi: 10.1111/j.1461-0248.2010.01559.x
Farwig, N., Bailey, D., Bochud, E., Herrmann, J. D., Kindler, E., Reusser, N., et al. (2009). Isolation from forest reduces pollination, seed predation and insect scavenging in Swiss farmland. Landsc. Ecol. 24, 919–927. doi: 10.1007/s10980-009-9376-2
Fischer, C., and Schröder, B. (2014). Predicting spatial and temporal habitat use of rodents in a highly intensive agricultural area. Agric. Ecosyst. Environ. 189, 145–153. doi: 10.1016/j.agee.2014.03.039
French, B. W., Elliott, N. C., Berberet, R. C., and Burd, J. D. (2001). Effects of riparian and grassland habitats on ground beetle (Coleoptera: Carabidae) assemblages in adjacent wheat fields. Environ. Entomol. 30, 225–234. doi: 10.1603/0046-225x-30.2.225
Furlong, M. J., and Zalucki, M. P. (2010). Exploiting predators for pest management: the need for sound ecological assessment. Entomol. Exp. Appl. 135, 225–236. doi: 10.1111/j.1570-7458.2010.00988.x
Garibaldi, L. A., Gemmill-Herren, B., D’Annolfo, R., Graeub, B. E., Cunningham, S. A., and Breeze, T. D. (2017). Farming approaches for greater biodiversity, livelihoods, and food security. Trends Ecol. Evol. 32, 68–80. doi: 10.1016/j.tree.2016.10.001
Giner, G., and Smyth, G. K. (2016). statmod: probability calculations for the inverse Gaussian distribution. arXiv [Preprint] doi: 10.32614/rj-2016-024
Godfray, H. C. J., Crute, I. R., Haddad, L., Lawrence, D., Muir, J. F., Nisbett, N., et al. (2010). The future of the global food system. Philos. Trans. R. Soc. B Biol. Sci. 365, 2769–2777. doi: 10.1098/rstb.2010.0180
González, E., Salvo, A., and Valladares, G. (2017). Arthropod communities and biological control in soybean fields: forest cover at landscape scale is more influential than forest proximity. Agric. Ecosyst. Environ. 239, 359–367. doi: 10.1016/j.agee.2017.02.002
Haan, N. L., Zhang, Y., and Landis, D. A. (2020). Predicting landscape configuration effects on agricultural pest suppression. Trends Ecol. Evol. 35, 175–186. doi: 10.1016/j.tree.2019.10.003
Habel, J. C., Dengler, J., Janišová, M., Török, P., Wellstein, C., and Wiezik, M. (2013). European grassland ecosystems: threatened hotspots of biodiversity. Biodivers. Conserv. 22, 2131–2138. doi: 10.1007/s10531-013-0537-x
Haddad, N. M., Brudvig, L. A., Clobert, J., Davies, K. F., Gonzalez, A., Holt, R. D., et al. (2015). Habitat fragmentation and its lasting impact on Earth’s ecosystems. Sci. Adv. 1, 1–10. doi: 10.1126/sciadv.1500052
Haddad, N. M., Gonzalez, A., Brudvig, L. A., Burt, M. A., Levey, D. J., and Damschen, E. I. (2017). Experimental evidence does not support the Habitat Amount Hypothesis. Ecography 40, 48–55. doi: 10.1111/ecog.02535
Haenke, S., Kovács-Hostyánszki, A., Fründ, J., Batáry, P., Jauker, B., Tscharntke, T., et al. (2014). Landscape configuration of crops and hedgerows drives local syrphid fly abundance. J. Appl. Ecol. 51, 505–513. doi: 10.1111/1365-2664.12221
Hanski, I. (2015). Habitat fragmentation and species richness. J. Biogeogr. 42, 989–993. doi: 10.1111/jbi.12478
Holland, J., and Fahrig, L. (2000). Effect of woody borders on insect density and diversity in crop fields: a landscape-scale analysis. Agric. Ecosyst. Environ. 78, 115–122. doi: 10.1016/S0167-8809(99)00123-1
Holland, J. M., Begbie, M., Birkett, T., Southway, S., Thomas, S. R., Alexander, C. J., et al. (2004). The spatial dynamics and movement of Pterostichus melanarius and P. madidus (carabidae) between and within arable fields in the UK. Int. J. Ecol. Environ. Sci. 30, 35–53.
Holland, J. M., Douma, J. C., Crowley, L., James, L., Kor, L., Stevenson, D. R. W., et al. (2017). Semi-natural habitats support biological control, pollination and soil conservation in Europe. A review. Agron. Sustain. Dev. 37:31. doi: 10.1007/s13593-017-0434-x
Holt, R. D. (1985). Population dynamics in two-patch environments: Some anomalous consequences of an optimal habitat distribution. Theor. Popul. Biol. 28, 181–208. doi: 10.1016/0040-5809(85)90027-9
Holzschuh, A., Steffan-Dewenter, I., and Tscharntke, T. (2010). How do landscape composition and configuration, organic farming and fallow strips affect the diversity of bees, wasps and their parasitoids? J. Anim. Ecol. 79, 491–500. doi: 10.1111/j.1365-2656.2009.01642.x
Huang, J., Zhou, K., Zhang, W., Deng, X., Van Der Werf, W., Lu, Y., et al. (2018). Uncovering the economic value of natural enemies and true costs of chemical insecticides to cotton farmers in China. Environ. Res. Lett. 13:064027. doi: 10.1088/1748-9326/aabfb0
Irmler, U. (2003). The spatial and temporal pattern of carabid beetles on arable fields in northern Germany (Schleswig-Holstein) and their value as ecological indicators. Agric. Ecosyst. Environ. 98, 141–151. doi: 10.1016/S0167-8809(03)00076-8
Jauker, F., Diekötter, T., Schwarzbach, F., and Wolters, V. (2009). Pollinator dispersal in an agricultural matrix: Opposing responses of wild bees and hoverflies to landscape structure and distance from main habitat. Landsc. Ecol. 24, 547–555. doi: 10.1007/s10980-009-9331-2
Karp, D. S., Chaplin-kramer, R., Martin, E. A., Karp, D. S., Chaplin-kramer, R., Meehan, T. D., et al. (2018). Crop pests and predators exhibit inconsistent responses to surrounding landscape composition. Proc. Natl. Acad. Sci. U.S.A. 115, E7863–E7870. doi: 10.1073/pnas.1800042115
Keinath, D. A., Doak, D. F., Hodges, K. E., Prugh, L. R., Fagan, W., Sekercioglu, C. H., et al. (2017). A global analysis of traits predicting species sensitivity to habitat fragmentation. Glob. Ecol. Biogeogr. 26, 115–127. doi: 10.1111/geb.12509
Koh, I., and Holland, J. D. (2015). Grassland plantings and landscape natural areas both in fl uence insect natural enemies. Agric. Ecosyst. Environ. 199, 190–199. doi: 10.1016/j.agee.2014.09.007
Korenko, S., Pekár, S., and Honěk, A. (2010). Predation activity of two winter-active spiders (Araneae: Anyphaenidae, Philodromidae). J. Therm. Biol. 35, 112–116. doi: 10.1016/j.jtherbio.2009.12.004
Lamy, T., Liss, K. N., Gonzalez, A., and Bennett, E. M. (2016). Landscape structure affects the provision of multiple ecosystem services. Environ. Res. Lett. 11:124017. doi: 10.1088/1748-9326/11/12/124017
Landis, D. A., Wratten, S. D., and Gurr, G. M. (2000). Habitat management to conserve natural enemies of arthropod pests in agriculture. Annu. Rev. Entomolal 45, 175–201. doi: 10.1146/annurev.ento.45.1.175
Lecomte, J. B., Benoît, H. P., Ancelet, S., Etienne, M. P., Bel, L., and Parent, E. (2013). Compound Poisson-gamma vs. delta-gamma to handle zero-inflated continuous data under a variable sampling volume. Methods Ecol. Evol. 4, 1159–1166. doi: 10.1111/2041-210X.12122
Letourneau, D. K., Jedlicka, J. A., Bothwell, S. G., and Moreno, C. R. (2009). Effects of natural enemy biodiversity on the suppression of arthropod herbivores in terrestrial ecosystems. Annu. Rev. Ecol. Evol. Syst. 40, 573–592. doi: 10.1146/annurev.ecolsys.110308.120320
Lindgren, J., Lindborg, R., and Cousins, S. A. O. (2018). Local conditions in small habitats and surrounding landscape are important for pollination services, biological pest control and seed predation. Agric. Ecosyst. Environ. 251, 107–113. doi: 10.1016/j.agee.2017.09.025
Lövei, G. L., and Ferrante, M. (2017). A review of the sentinel prey method as a way of quantifying invertebrate predation under field conditions. Insect Sci. 24, 528–542. doi: 10.1111/1744-7917.12405
Lundgren, J. G., Shaw, J. T., Zaborski, E. R., and Eastman, C. E. (2006). The influence of organic transition systems on beneficial ground-dwelling arthropods and predation of insects and weed seeds. Renew. Agric. Food Syst. 21, 227–237. doi: 10.1079/raf2006152
Maas, B., Clough, Y., and Tscharntke, T. (2013). Bats and birds increase crop yield in tropical agroforestry landscapes. Ecol. Lett. 16, 1480–1487. doi: 10.1111/ele.12194
Maes, S., Massart, X., Grégoire, J. C., and De Clercq, P. (2014). Dispersal potential of native and exotic predatory ladybirds as measured by a computer-monitored flight mill. BioControl 59, 415–425. doi: 10.1007/s10526-014-9576-9
Martin, E. A., Clough, Y., Bommarco, R., Garratt, M. P. D., Holzschuh, A., Kleijn, D., et al. (2019). The interplay of landscape composition and configuration : new pathways to manage functional biodiversity and agroecosystem services across Europe. Ecol. Lett. 22, 1083–1094. doi: 10.1111/ele.13265
Martin, E. A., Reineking, B., Seo, B., and Steffan-Dewenter, I. (2015). Pest control of aphids depends on landscape complexity and natural enemy interactions. PeerJ 3:e1095. doi: 10.7717/peerj.1095
Maxwell, S. L., Fuller, R. A., Brooks, T. M., and Watson, J. E. M. (2016). The ravages of guns, nets and bulldozers. Nature 536, 143–145. doi: 10.1038/536143a
McHugh, N. M., Moreby, S., Lof, M. E., Van der Werf, W., and Holland, J. M. (2020). The contribution of semi-natural habitats to biological control is dependent on sentinel prey type. J. Appl. Ecol. 57, 914–925. doi: 10.1111/1365-2664.13596
Muneret, L., Auriol, A., Thiéry, D., and Rusch, A. (2019). Organic farming at local and landscape scales fosters biological pest control in vineyards. Ecol. Appl. 29, 1–15. doi: 10.1002/eap.1818
Naranjo, S. E., Ellsworth, P. C., and Frisvold, G. B. (2015). Economic value of biological control in integrated pest management of managed plant systems. Annu. Rev. Entomol. 60, 621–645. doi: 10.1146/annurev-ento-010814-021005
Perez-Alvarez, R., Nault, B. A., and Poveda, K. (2019). Effectiveness of augmentative biological control depends on landscape context. Sci. Rep. 9, 1–15. doi: 10.1038/s41598-019-45041-1
Petersen, L. F., and Woltz, J. M. (2015). Diel variation in the abundance and composition of the predator assemblages feeding on aphid-infested soybean. BioControl 60, 209–219. doi: 10.1007/s10526-014-9631-6
Petit, S., Trichard, A., Biju-Duval, L., McLaughlin, B., and Bohan, D. A. (2017). Interactions between conservation agricultural practice and landscape composition promote weed seed predation by invertebrates. Agric. Ecosyst. Environ. 240, 45–53. doi: 10.1016/j.agee.2017.02.014
R Core Team (2015). R A Language Environment Statical Computing. Vienna: R Foundation Statical Computing.
Rand, T. A., Tylianakis, J. M., and Tscharntke, T. (2006). Spillover edge effects: the dispersal of agriculturally subsidized insect natural enemies into adjacent natural habitats. Ecol. Lett. 9, 603–614. doi: 10.1111/j.1461-0248.2006.00911.x
Ricci, B., Lavigne, C., Alignier, A., Aviron, S., Biju-Duval, L., Bouvier, J. C., et al. (2019). Local pesticide use intensity conditions landscape effects on biological pest control. Proc. R. Soc. B Biol. Sci. 286:20182898. doi: 10.1098/rspb.2018.2898
Rusch, A., Bommarco, R., Jonsson, M., Smith, H. G., and Ekbom, B. (2013). Flow and stability of natural pest control services depend on complexity and crop rotation at the landscape scale. J. Appl. Ecol. 50, 345–354. doi: 10.1111/1365-2664.12055
Rusch, A., Chaplin-Kramer, R., Gardiner, M. M., Hawro, V., Holland, J., Landis, D., et al. (2016). Agricultural landscape simplification reduces natural pest control: a quantitative synthesis. Agric. Ecosyst. Environ. 221, 198–204. doi: 10.1016/j.agee.2016.01.039
Rusch, A., Valantin-Morison, M., Sarthou, J. P., and Roger-Estrade, J. (2011). Multi-scale effects of landscape complexity and crop management on pollen beetle parasitism rate. Landsc. Ecol. 26, 473–486. doi: 10.1007/s10980-011-9573-7
Rusch, A., Valentin-Morison, M., Sarthou, J.-P., and Roger-Estrade, J. (2010). Biological Control of Insect Pests in Agroecosystems: Effects of Crop Management, Farming system, and Seminatural Habitats at the Landscape Scale: A Review, 1 Edn. Amsterdam: Elsevier Inc, doi: 10.1016/S0065-2113(10)09006-1
Saha, D., Alluri, P., Dumbaugh, E., and Gan, A. (2020). Application of the Poisson-Tweedie distribution in analyzing crash frequency data. Accid. Anal. Prev. 137:105456. doi: 10.1016/j.aap.2020.105456
Sarthou, J. P., Badoz, A., Vaissière, B., Chevallier, A., and Rusch, A. (2014). Local more than landscape parameters structure natural enemy communities during their overwintering in semi-natural habitats. Agric. Ecosyst. Environ. 194, 17–28. doi: 10.1016/j.agee.2014.04.018
Saska, P., Van Der Werf, W., De Vries, E., and Westerman, P. R. (2008). Spatial and temporal patterns of carabid activity-density in cereals do not explain levels of predation on weed seeds. Bull. Entomol. Res. 98, 169–181. doi: 10.1017/S0007485307005512
Schmidt, M. H., Roschewitz, I., Thies, C., and Tscharntke, T. (2005). Differential effects of landscape and management on diversity and density of ground-dwelling farmland spiders. J. Appl. Ecol. 42, 281–287. doi: 10.1111/j.1365-2664.2005.01014.x
Schmidt, M. H., and Tscharntke, T. (2005). The role of perennial habitats for Central European farmland spiders. Agric. Ecosyst. Environ. 105, 235–242. doi: 10.1016/j.agee.2004.03.009
Schneider, G., Krauss, J., Riedinger, V., Holzschuh, A., and Steffan-dewenter, I. (2015). Biological pest control and yields depend on spatial and temporal crop cover dynamics. J. Appl. Ecol. 52, 1283–1292. doi: 10.1111/1365-2664.12471
Sirami, C., Gross, N., Bosem, A., Bertrand, C., Carrié, R., and Hass, A. (2019). Increasing crop heterogeneity enhances multitrophic diversity across agricultural regions. Proc. Natl. Acad. Sci. U.S.A. 116, 16442–16447. doi: 10.1073/pnas.1906419116
Thomson, L. J., and Hoffmann, A. A. (2013). Spatial scale of benefits from adjacent woody vegetation on natural enemies within vineyards. Biol. Control 64, 57–65. doi: 10.1016/j.biocontrol.2012.09.019
Tilman, D., Isbell, F., and Cowles, J. M. (2014). Biodiversity and Ecosystem Functioning. Annu. Rev. Ecol. Evol. Syst. 45, 471–493. doi: 10.1146/annurev-ecolsys-120213-091917
Trichard, A., Alignier, A., Biju-Duval, L., and Petit, S. (2013). The relative effects of local management and landscape context on weed seed predation and carabid functional groups. Basic Appl. Ecol. 14, 235–245. doi: 10.1016/j.baae.2013.02.002
Tscharntke, T., Bommarco, R., Clough, Y., Crist, T. O., Kleijn, D., Rand, T. A., et al. (2007). Conservation biological control and enemy diversity on a landscape scale. Biol. Control 43, 294–309. doi: 10.1016/j.biocontrol.2007.08.006
Tscharntke, T., Karp, D. S., Chaplin-kramer, R., Batáry, P., Declerck, F., Gratton, C., et al. (2016). When natural habitat fails to enhance biological pest control – Five hypotheses. Biol. Conserv. 204, 449–458. doi: 10.1016/j.biocon.2016.10.001
Tschumi, M., Ekroos, J., Hjort, C., Smith, H. G., and Birkhofer, K. (2018a). Predation-mediated ecosystem services and disservices in agricultural landscapes. Ecol. Appl. 28, 2109–2118. doi: 10.1002/eap.1799
Tschumi, M., Ekroos, J., Hjort, C., Smith, H. G., and Birkhofer, K. (2018b). Rodents, not birds, dominate predation-related ecosystem services and disservices in vertebrate communities of agricultural landscapes. Oecologia 188, 863–873. doi: 10.1007/s00442-018-4242-z
Veres, A., Petit, S., Conord, C., and Lavigne, C. (2013). Does landscape composition affect pest abundance and their control by natural enemies? A review. Agric. Ecosyst. Environ. 166, 110–117. doi: 10.1016/j.agee.2011.05.027
Watling, J. I., Arroyo-Rodríguez, V., Pfeifer, M., Baeten, L., Banks-Leite, C., Cisneros, L. M., et al. (2020). Support for the habitat amount hypothesis from a global synthesis of species density studies. Ecol. Lett. 23, 674–681. doi: 10.1111/ele.13471
Watling, J. I., and Donnelly, M. A. (2006). Fragments as islands: a synthesis of faunal responses to habitat patchiness. Conserv. Biol. 20, 1016–1025. doi: 10.1111/j.1523-1739.2006.00482.x
Wegner, J., and Merriam, G. (1990). Use of spatial elements in a farmland mosaic by a Woodland Rodent. Biol. Conserv. 54, 263–276. doi: 10.1016/0006-3207(90)90056-U
Werling, B. P., Dickson, T. L., Isaacs, R., Gaines, H., Gratton, C., and Gross, K. L. (2014). Perennial grasslands enhance biodiversity and multiple ecosystem services in bioenergy landscapes. Proc. Natl. Acad. Sci. U.S.A. 111, 1652–1657. doi: 10.1073/pnas.1309492111
Winqvist, C., Bengtsson, J., Aavik, T., Berendse, F., Clement, L. W., Eggers, S., et al. (2011). Mixed effects of organic farming and landscape complexity on farmland biodiversity and biological control potential across Europe. J. Appl. Ecol. 48, 570–579. doi: 10.1111/j.1365-2664.2010.01950.x
Wróbel, A., and Bogdziewicz, M. (2015). It is raining mice and voles: which weather conditions influence the activity of Apodemus flavicollis and Myodes glareolus? Eur. J. Wildl. Res. 61, 475–478. doi: 10.1007/s10344-014-0892-2
Ximenez-Embun, M. G., Zaviezo, T., and Grez, A. (2014). Seasonal, spatial and diel partitioning of Acyrthosiphon pisum (Hemiptera: Aphididae) predators and predation in alfalfa fields. Biol. Control 69, 1–7. doi: 10.1016/j.biocontrol.2013.10.012
Zaller, J. G., Simmer, L., Santer, N., Tataw, J. T., Formayer, H., Murer, E., et al. (2014). Future rain fall variations reducea bundances of aboveground arthropods in model agroecosystems with different soil types. Front. Environ. Sci. 2:44. doi: 10.3389/fenvs.2014.00044
Zhang, W., and Swinton, S. M. (2009). Incorporating natural enemies in an economic threshold for dynamically optimal pest management. Ecol. Modell. 220, 1315–1324. doi: 10.1016/j.ecolmodel.2009.01.027
Keywords: ecosystem services, agricultural intensification, predation, agroecology, natural enemy, aphid, weed
Citation: Perrot T, Rusch A, Coux C, Gaba S and Bretagnolle V (2021) Proportion of Grassland at Landscape Scale Drives Natural Pest Control Services in Agricultural Landscapes. Front. Ecol. Evol. 9:607023. doi: 10.3389/fevo.2021.607023
Received: 16 September 2020; Accepted: 24 March 2021;
Published: 14 April 2021.
Edited by:
Georg K. S. Andersson, Lund University, SwedenReviewed by:
Berta Caballero Lopez, Museu de les Ciències Naturals de Barcelona, SpainCopyright © 2021 Perrot, Rusch, Coux, Gaba and Bretagnolle. This is an open-access article distributed under the terms of the Creative Commons Attribution License (CC BY). The use, distribution or reproduction in other forums is permitted, provided the original author(s) and the copyright owner(s) are credited and that the original publication in this journal is cited, in accordance with accepted academic practice. No use, distribution or reproduction is permitted which does not comply with these terms.
*Correspondence: Thomas Perrot, VGhvbWFzLnBlcnJvdEBpbnJhZS5mcg==
Disclaimer: All claims expressed in this article are solely those of the authors and do not necessarily represent those of their affiliated organizations, or those of the publisher, the editors and the reviewers. Any product that may be evaluated in this article or claim that may be made by its manufacturer is not guaranteed or endorsed by the publisher.
Research integrity at Frontiers
Learn more about the work of our research integrity team to safeguard the quality of each article we publish.