- 1Melbourne School of Design, Faculty of Architecture, Building and Planning, University of Melbourne, Melbourne, VIC, Australia
- 2Connecticut Institute for Resilience and Climate Adaptation, University of Connecticut, Groton, CT, United States
- 3Urban Ecology and Design Lab and Ecopolitan Design, New Haven, CT, United States
- 4Harvard Forest, Harvard University, Petersham, MA, United States
Interest is growing in designing resilient and ecologically rich urban environments that provide social and ecological benefits. Regenerative and biocentric designs fostering urban ecological habitats including food webs that provide ecosystem services for people and wildlife increasingly are being sought. However, the intentional design of urban landscapes for food webs remains in an early stage with few precedents and many challenges. In this paper, we explore the potential to design (for) urban food webs through collaborations between designers and ecologists. We start by examining the ecology and management of Jamaica Bay in New York City as a case study of an anthropogenic landscape where ecosystems are degraded and the integrity of extant food webs are intertwined with human agency. A subsequent design competition focusing on ecological design and management of this large-scale landscape for animal habitat and ecosystem services for people illustrates how designers approach this anthropogenic landscape. This case study reveals that both designing urban landscapes for food webs and directly designing and manipulating urban food webs are complicated and challenging to achieve and maintain, but they have the potential to increase ecological health of, and enhance ecosystem services in, urban environments. We identify opportunities to capitalize on species interactions across trophic structures and to introduce managed niches in biologically engineered urban systems. The design competition reveals an opportunity to approach urban landscapes and ecological systems creatively through a proactive design process that includes a carefully crafted collaborative approach to constructing ecologically functioning landscapes that can integrate societal demands. As designers increasingly seek to build, adapt, and manage urban environments effectively, it will be critical to resolve the contradictions and challenges associated with human needs, ecosystem dynamics, and interacting assemblages of species. Ecologists and designers are still discovering and experimenting with designing (for) urban food webs and fostering species interactions within them. We recommend generating prototypes of urban food webs through a learning-by-doing approach in urban development projects. Design and implementation of urban food webs also can lead to research opportunities involving monitoring and experiments that identify and solve challenges of food-web construction while supporting and encouraging ongoing management.
1. Introduction
For as long as ecologists have been describing and studying ecosystems1, they have documented the occurrence, structure, interactions, and associated dynamics of species within food webs (Figure 1; McCann, 2012). More than a century of observational and experimental studies in natural ecosystems has revealed that food webs assemble themselves from rich pools of dozens, hundreds, or even thousands of species. A range of strong and weak predator-prey interactions and the flow of energy from producers to consumers to decomposers work in concert to exert both bottom-up and top-down control on the structure and dynamics of food webs (e.g., Ulanowicz et al., 2014).
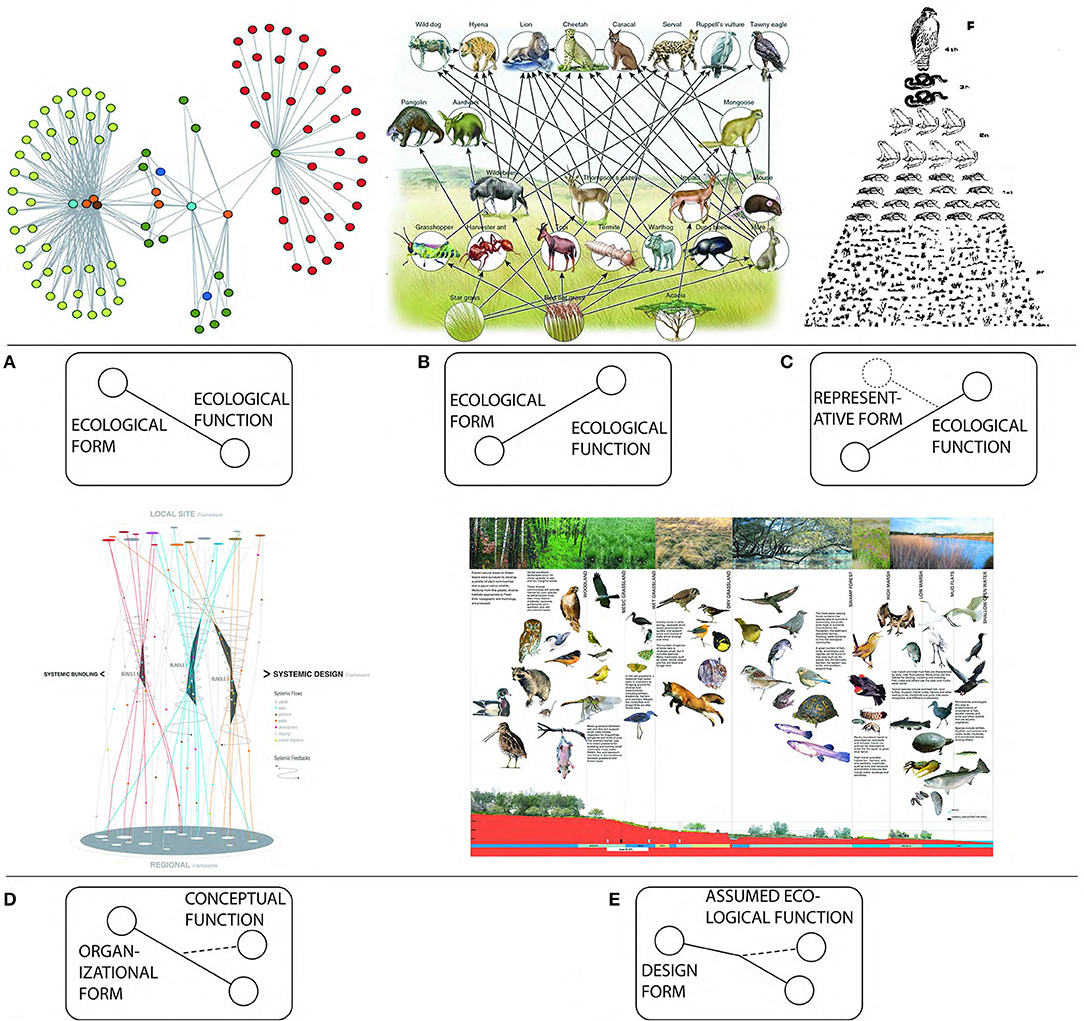
Figure 1. Food webs as envisioned by ecologists and designers place different emphases on their structure (form) and dynamics (function or ecosystem services). The large color panels illustrate portrayal by ecologists of food webs (A,B) and their pedagogical representation (C); and their physical embodiment by designers and landscape architects for the built environment (D,E). The “see-saw” icon below each color panel indicates the relative emphasis on form and function in the associated panel. Form (on the left) or function (on the right) may be emphasized (upper left or right) or de-emphasized (lower left or right) and there is usually (but not always, as in C) a trade-off (see-sawing) between form and function in emphasis by ecologists or designers. For additional discussion and illustration, see, respectively main text section 6.4 and sliders below photographs in Figure 10. (A) Is an abstraction of a food web that illustrates its topology. Colored nodes identify functions (e.g., producer, consumer) but there is no identification of who eats whom, directionality, or interactions. (B) Is an illustration of a food web that emphasizes functions (as interrelationships across organisms and trophic structures) using directional arrows to indicate who eats whom. (C) Is a “food pyramid” like those seen in high-school textbooks that emphasizes trophic transfer and the decreasing abundance of individuals at increasingly higher trophic levels. (D) Illustrates a “Systemic Design” framework for managing interactions and information translated across scales in a hierarchy of nested relationships. Information is bundled into clusters (“Systemic Bundles”) that tie local site conditions to regional perspectives and expose issues surrounding any given site. (E) Is a design-based representation by James Corner Field Operations of an idealized process of ecological succession and food-web development in an urban landfill-turned-public park. Image sources: (A) Image produced with FoodWeb3D, and provided by the Pacific Ecoinformatics and Computational Ecology Lab (http://www.foodwebs.org/; Yoon et al., 2004); (B) Savanna food web from https://savannabiomeassignment.weebly.com/food-web.html; (C) Image in the public domain from Brace (1977); (D) Alan M. Berger and Casey L. Brown, P-REX, published in Berger (2009) and used with permission; (E) James Corner Field Operations, and used with permission.
Ecologists disagree about the effects of urbanization on biodiversity—the pool of species from which food webs assemble. Some studies have reported that biodiversity is reduced and homogenized with increasing human occupation and urbanization (McKinney, 2006; Groffman et al., 2014). Others have found that urban systems have considerable species diversity and support locally rare or endangered native species. For example, changes in productivity and habitat structures in cities may favor specific species of birds (Marzluff and Ewing, 2008), spiders (Shochat et al., 2008), bees (Mata et al., 2019), or amphibians (Hamer and McDonnell, 2008). This urban biodiversity increasingly is valued and deliberately cultivated in more intentionally designed urban green spaces (Aronson et al., 2017). For example, homeowners may deliberately introduce a single trophic level (e.g., “butterfly plants”) to attract herbivores and pollinators (e.g., caterpillars and butterflies), and perhaps unintentionally, their predators (e.g., birds and small mammals) to their yards or gardens (e.g., USDA Natural Resources Conservation Service, 2020). Reconciliation ecology in particular has a unique perspective on fostering biodiversity in human-dominated ecosystems, positing that ecological engineering can shift the abiotic and biotic conditions of more artificial urban analogs to support native and indigenous biodiversity (Rosenzweig, 2003). Through reconciliation, ecologists can make urban environment more suitable for native and indigenous biodiversity and foster wildlife systems that structurally or functionally resemble natural ecosystems and habitats from the region (Lundholm and Richardson, 2010).
A logical next step is to consider entire food webs in urban habitats. We recognize distinctive degrees of structure and composition, utility for people (“ecosystem services”), and intentionality—all at different spatial scales (Figure 2; and see Faeth et al., 2005; McKinney, 2006; Warren et al., 2006)—among urban food webs. For example, many urban food webs occur in the seemingly pre-urban conditions found in semi-natural fragmented forest remnants or urban streams (e.g., Faeth et al., 2011; Adler and Tanner, 2013; Forman, 2014). Others occur unintentionally in small patches or successional landscapes, such as overgrown median strips or accidental wetlands (Nassauer, 2012; Adler and Tanner, 2013; Beninde et al., 2015; Palta et al., 2017).
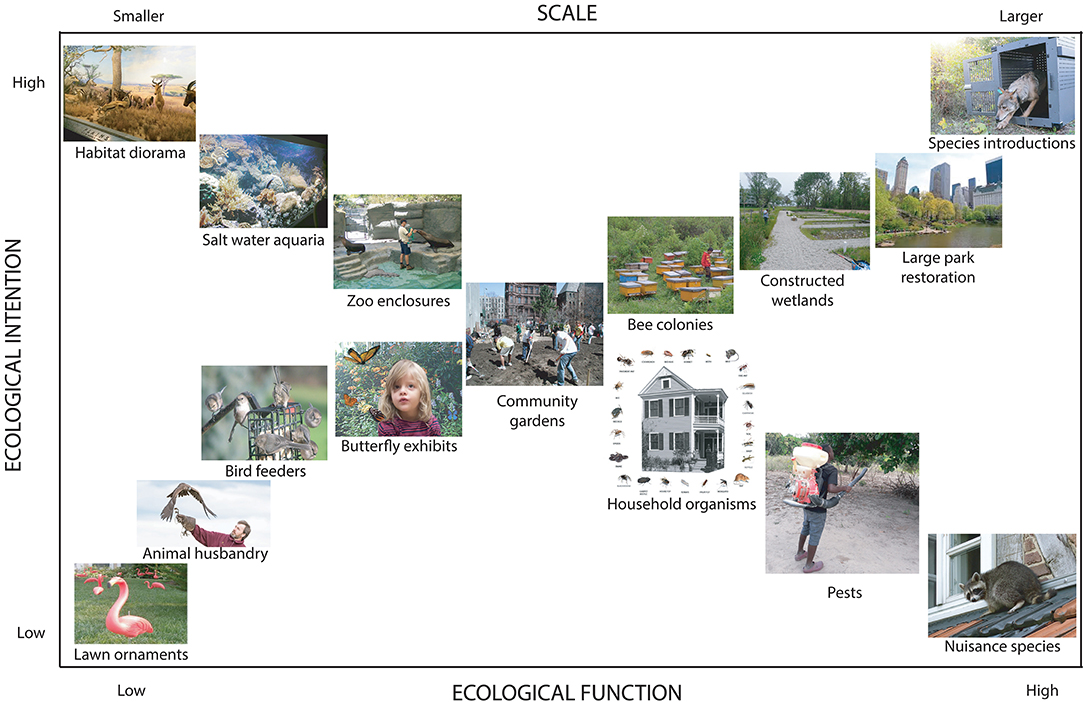
Figure 2. Constructed urban food webs are an outcome of both intentional and unintentional human activities and come in many shapes and sizes. This figure illustrates relationships between ecological functions and human intention to modify the local ecology in real and representational food webs. For designed food webs, we need to combine intentionality with theories of biological succession, invasion, species interactions, and other ecological applications to evaluate and manage the systems over time. Image sources: Lawn ornaments (Bert bert: https://commons.wikimedia.org/wiki/File:Flamingo_1.jpg; CC-BY); Animal husbandry (Government of Victoria: https://www.yarracity.vic.gov.au/events/2018/06/02/hawk-owl-falconry-display; public domain); Bird feeders (Scott Catron: https://commons.wikimedia.org/w/index.php?curid=849195; CC-BY-2.5); Butterfly exhibits (Alexander Felson: photomontage, 2020; used with permission); Community gardens (Alexander Felson: photograph, 2005; used with permission); Habitat diorama (NComparato: https://commons.wikimedia.org/wiki/File:Plains_Diorama.JPG; CC-BY-SA 3.0); Salt water aquaria (Fritz Geller-Grimm; https://commons.wikimedia.org/wiki/File:Aquarium_fg01.jpg; CC-BY-SA-2.5); Zoo enclosures (Fir0002: https://commons.wikimedia.org/wiki/File:Seals@melb_zoo.jpg; CC-BY-SA-3.0); Bee colonies (Okkisafire: http://self.gutenberg.org/articles/eng/File:Beekeeping_at_Kawah_Ijen,_Indonesia.jpg; CC-BY-SA 3.0); Constructed wetlands (Alexander Felson: photograph, 2020; used with permission); Large park restoration (Ingfbruno: https://commons.wikimedia.org/wiki/File:3037-Central_Park-The_Pond.JPG; CC-BY-SA 3.0); Species introductions (US National Park Service: https://www.nps.gov/isro/learn/news/isle-royale-national-park-and-partners-release-two-wolves-on-the-island.htm; public domain); Household organisms (Alexander Felson: photomontage, 2021; used with permission); Pests (Kente l: https://commons.wikimedia.org/wiki/File:Kente_l.jpg; CC BY-SA 4.0); Nuisance species (Carsten Volkwein; https://upload.wikimedia.org/wikipedia/commons/7/7f/Waschbaer_auf_dem_Dach.jpg; CC BY-SA 2.5).
Clearly many species interactions and networks are reshaped by urbanization (Forman, 2014). Remnant urban food webs have been shown to support less diverse ecological communities, with specialist species and rare species being particularly vulnerable. Urbanization can be seen as destabilizing, reshaping ecological stability and (dis)assembling species interactions (Start et al., 2020). We note that our intent with Figure 2 is not to define the only possible sets of food webs in specific combinations of ecological function, ecological intentionality, and space [i.e., the locations of the images in (x,y,z) space in Figure 2] but rather to encourage readers to consider the myriad possibilities about how effects of these different variables may play out in “natural” and designed food webs at different scales.
But urban food webs need to be considered differently from those in “natural” ecosystems. Habitat modifications, species introductions, and dispersal across fragmented and heterogeneous urban landscapes are environmental filters influencing composition of species assemblages and structure of urban food webs (Andrade et al., 2020). Human agency in the deliberate design of urban green spaces and land management practices provides a further set of structuring filters (Hagen et al., 2012). Urban food webs interact with human socioeconomic and cultural systems, including designed landscapes, governance structures, and social networks. The uniquely defined ecological communities and food webs found in urban ecosystems have been described using a variety of terms, from “urban assemblages” (Aronson et al., 2017) and “urban metacommunities” (Andrade et al., 2020) to “ecological networks across environmental gradients” (Tylianakis and Morris, 2017), “ecological networks” (or “meta-networks”) (Mata et al., 2019), “interaction networks” (Start et al., 2020), living shorelines, and green infrastructure (Hostetler et al., 2011), as well as the more concise “urban food webs” (Faeth et al., 2005; Warren et al., 2006; Aronson et al., 2017)2. Each of these terms includes concepts from community, ecosystem, landscape, and urban ecology. While urban food-web research is expanding, the impact of urbanization and human activities on food-web structure remain poorly understood because cities are challenging spaces for ecologists interested in studying and deciphering food-web structure and dynamics (Andrade et al., 2020).
Although ecologists are moving forward slowly, landscape architects and design professionals are more rapidly exploring and incorporating food webs in urban projects through habitat creation, regenerative design, biocentric design, biophilia, urban agriculture, permaculture, urban restoration projects, and commissioned landscape projects, such as large parks or botanical gardens (see examples in section 5 and Czerniak and Hargreaves, 2007; Beck, 2013). Large infrastructure projects, such as stormwater parks, utility corridors, or management zones that encompass or are adjacent to existing habitats and waterways may require habitat mitigation for particular species or provide environmental conditions in which urban food webs can self-assemble and opportunities for more intentionally-designed and constructed urban food webs. However, whether we can design and manage urban food webs remains an open and challenging question.
2. Roadmap
In this paper, we articulate a vision for connecting ecological theory (i.e., concepts, ideas, and generalizations) with landscape architecture and urban design to guide the cultivation and construction of urban food webs. We begin with short précis that briefly reviews the history of how ecologists have thought about design and how designers have engaged with ecologists (section 3). We include in this section a Glossary of Terms (Box 1) used regularly by ecologists and designers but often with very different meanings. We follow this historical overview with a description and analysis of a single case study of the ecology and management of the food web within Jamaica Bay's Gateway National Park within New York City (section 4). This case study illustrates how ideas and issues that will be familiar to ecologists are addressed and reinterpreted by designers. It shows how existing urban food webs are connected to land-use practices and human behaviors through decision making and expressed in design thinking and management. Jamaica Bay also has been the locus of two landscape design competitions that have foregrounded challenges and opportunities in designing, building, and managing urban food webs. The winning designs illustrate how design professionals working independently of ecologists (section 4.2) or in collaboration with ecologists (section 4.3) use a creative process that includes hybrid ecological, recreational, and educational solutions and encourages investment in habitat management for the sake of people and the threatened species.
Box 1. Glossary of Terms used commonly by designers, that may be unfamiliar to ecologists, and whose use and interpretation differs between ecologists and designers.
• Aesthetics: The philosophy of the nature of art, beauty, and taste (Adorno, 1997). Applied in design projects to the creation and appreciation of form, layout, materials, and function. From aisthētós (Greek: “sensible, perceptible”; by extension: “to perceive, take notice of, understand”).
• Design: The process of inventing and creating proposals for buildings, landscapes, and urban artifacts through rigorous inquiry and individual creativity.
• Design competition: An organized competition in which design teams submit proposals responding to a site-specific or conceptual competition brief; winners may or may not be able to actually build the proposed project.
• Design process: Project activities from its initial analysis and conceptualization through design and construction.
• Design thinking: Process of sketching and conceptualizing ideas into design solutions from inquiry through crystallization (Rowe, 1996).
• Parametric design: Computational process that uses algorithmic-based software to generate responsive and immersive three-dimensional models used in (landscape) architecture.
• Working methods of design include iterative approaches to site analysis, conceptual and schematic design, design detailing, material selection, communication with clients and stakeholder, design, and construction (Felson et al., 2013b).
• Green infrastructure: Land and water management using vegetation, water, and modified natural process to restore and sustain hydrologic and habitat functions.
• Ecological infrastructure: Green infrastructure that prioritizes ecosystem functions over engineering infrastructure and re-adapts landscapes and associated ecological processes into multifunctional open spaces.
• Ecosystem: Biotic and abiotic elements and all their interactions in a geographic area.
• Designer ecosystems: ecosystems in which ecological goals have been articulated explicitly, management actions are employed and evaluated to achieve those goals, and where the goals and actions are continually optimized as new information becomes available (Ross et al., 2015).
• Ecosystem functions: Processes that affect biological (or human) communities. Examples include climate control, productivity, energy flow, and nutrient cycling.
• Ecosystem services: Benefits provided by nature to humans, usually economically valued (Millennium Ecosystem Assessment, 2005). Categories include provisioning, regulating, supporting, and cultural ecosystem services.
• Novel ecosystems: Species combinations or environmental configurations that are not thought to have existed previously (Hobbs et al., 2009). For landscape architects, novel ecosystems are common features of design projects.
• Form: The shape or structure of an object.
• Function: The purpose or performance of a structure or system; the relationship from inputs to possible outputs.
• Human agency: The capacity and freedom to make our own choices and to have those choices impact the world around us.
• Intentional(ity): The conscious or subconscious directedness of ones' mind toward a particular task or agenda.
• Landscape: Socio-ecological systems in a bounded area consisting of a mosaic and gradient of more natural to human-modified ecosystems. In art, land that can be seen and comprehended in a single view. From landschap (Dutch: a collection of farms or fenced fields); landschaft (German: land shared by people); also paesaggio (Italian), paysage (French); paisaje (Spanish).
• Landscape architecture: The professional and academic discipline focused on planning, design, management, stewardship, and nurturing of urban, rural, and natural environments, including small-scale trails, streetscapes, plazas, and residences, and regional-scale parklands, urban parks, and campuses.
• Landscape urbanism: A way of thinking about cities through a landscape lens. Includes notions of territory, contemporary city forms, systems; considers landscape, infrastructure, and ecology as equally important components of cities.
• Management: The process of using people and resources to achieve targeted goals. In design, the deployment, effective use, and coordination of finances, technology, and people to efficiently manage the built environment and natural resources.
• Maintenance: Activities required to forestall deterioration of a landscape. Includes mowing, pruning, tree removal, edging, cultivation, fertilization, and pesticide application.
• Material selection: Process of researching and selecting materials—constrained by budget and availability—for distinct areas in a design.
• Urban: Characteristics of a town or city, usually defined by density. In the US, a cluster of areas with 2,500–50,000 people or a single area of ≥50,000 people3.
• Urban ecology: The study of (socio-)ecological processes in cities and urbanized regions.
• Urban food web: Multi-trophic assemblages of interacting species found throughout human-dominated rural, exurban, suburban, and urban built environments. Examples of urban food webs include microbiomes and their hosts in single-family homes, plants, pollinators, and insect herbivores in community gardens, and predators and their prey thriving within planned residential neighborhoods.
In section 5, we build on the case study to discuss approaches already being applied by design professionals interested in designing and managing for food webs. We differentiate design for food webs from intentionally designed food webs in urban environments. We emphasize that designers are already designing (for) food webs even though many ecologists are likely to question the rationale for, or feasibility of, establishing stable urban food webs. We also recognize that designing for food webs through habitat restoration or “rewilding” (e.g., Lorimer et al., 2015; Corlett, 2016; Svenning et al., 2016) is pragmatic and intentional and shares some features with designed food webs proposed by design professionals. The paper concludes with a discussion of the key challenges associated with building bridges between ecologists and design professionals that could help achieve the goal of establishing urban food webs (section 6). We identify roles for ecologists in developing and applying relevant theories needed to intentionally design urban food webs (Pulliam and Johnson, 2002; Grose, 2017) that build on a growing literature establishing ecological principles for landscape design (e.g., Beck, 2013).
3. A Brief History of Intersections Between Ecologists and Designers
Ecologists are increasingly working with designers and other urban practitioners involved in shaping cities (Felson et al., 2013b; Tanner et al., 2014; Pickett et al., 2020). These interactions can be challenging but also have potential to enrich all the partners (e.g., Johnson et al., 2002; Musacchio, 2009; Pickett et al., 2016).
3.1. Ecologists Increasingly Are Considering Design
Ecologists interested in urban areas initially focused on understanding autecology and interactions of non-human populations and communities in cities (e.g., Adams, 2005; Bornkamm, 2007; Sukopp, 2008). These and other early studies relied on a direct translation of classical ecological concepts and theories developed in non-urban systems into cities (Rebele, 1994). Urban ecology has begun focusing on ecology of cities to integrate the built environment and human behavior (Alberti et al., 2009; Pickett et al., 2011; Tanner et al., 2014; Groffman et al., 2017). Concurrently, ecologists are actively revisiting their role in urban research, applied science and design (Felson and Pickett, 2005; Palmer, 2009; Pickett et al., 2020). Most recently, ecologists are embracing ecology for cities (Childers et al., 2015; Pickett et al., 2016) and working directly with designers through the design process.
3.2. The Ebb and Flow of Ecology in Design
Among designers, Ian McHarg heavily influenced the integration of ecology into landscape design, particularly through education (McHarg, 1969). He framed the ecological paradigm around disturbance ecology as a fundamental part of ecosystems and invited ecologists into design schools to teach ecological theory to design students (Spirn, 2000). Many of his students went on to become leaders whose work and that of their students and colleagues influenced the field of landscape architecture worldwide (e.g„ Spirn, 2000; Steiner et al., 2013, 2019; Reed and Lister, 2014; Grose, 2017)4. In the last 50 years, the place and influence of ecology in the design professions has waxed and waned with changing priorities and demands on designers (Steiner et al., 2019). These shifting priorities have reflected the responsiveness of urban design to contemporary cultural attitudes, needs, and interests, all of which can limit opportunities for designers to develop a deeper working knowledge of ecological science than they may have been exposed to during their schooling or receive through continuing education. This is especially evident in the continuing debate about the interplay between form and function in landscape architecture (section 6.4.2).
3.3. Initial Challenges for Integrating Ecology and Design
It has been said that ecologists have been happy if they understand a system while designers are happy if they invent something new (Charlie Canham, 2007 personal communication to Alexander Felson). When they meet to collaborate, ecologists and designers often use identical terms to refer to very different concepts and practices (Figure 3; Box 1; and Ellison and Buckley Borden, 2021). A key point of disassociation is that ecologists consider “ecology” as a single entity and scientific discipline whereas designers often speak of “ecologies” (e.g., Reed and Lister, 2014). Ecologists tend to lack an appreciation for design thinking and rarely consider concerns other than ecological criteria (i.e., issues from ecology as a science), including material constraints, accessibility, and visual cues that influence site design. On the other hand, design professionals, environmental consultants, and other practitioners tend to rely mostly on technical reports, assumptions, and dated ecological knowledge derived from non-urban systems to inform their work (Forman, 2002; Johnson et al., 2002). Although designers have limited time to interpret and synthesize ecological information, working methods of design are flexible enough to incorporate ecological input at different stages (Felson et al., 2013b). These cultural differences, distinct ideologies, vocabularies, methods and tools, scales of activity, and approaches to work need to be addressed in any collaboration between ecologists and designers (Ellison and Buckley Borden, 2021). Working closely with ecologists to define these methods is a way forward to ensure that ecological theory informs design outcomes (Felson et al., 2013b). Landscape ecologists have had the most success in building collaborative bridges with designers, providing connections between ecology, sustainability, aesthetics, and design (Musacchio, 2011). Additional contemporary hurdles that ecologists and designers navigate are highlighted through our case study (section 4) and current ecology-oriented designs (section 5), and elaborated in the final section (section 6).
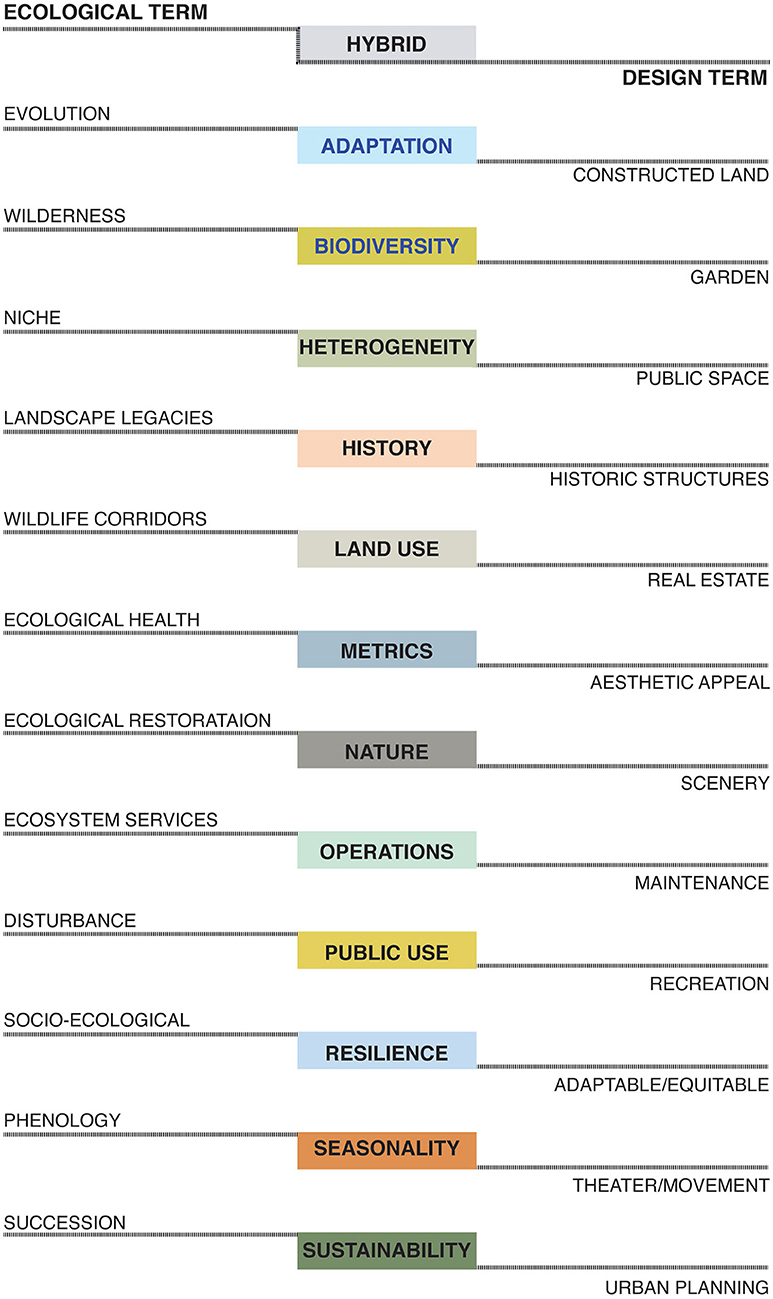
Figure 3. Ecologists (E) and Designers (D) have different interpretations of and use different words for similarly-named conceptual domains. The interpretations or words on the left and right are intentionally offset to suggest differences in understanding between the ecologists and designers (paralleled by the form vs. function “see-saws” in Figure 1 and “sliders” in Figure 10). These differences make it difficult for ecologists and designers to communicate and collaborate effectively. The interpretations and words are connected through their concepts (center) to suggest opportunities for alignment. Identifying and building on areas of shared interest and teaching across disciplines can strengthen collaborations between ecologists and designers. Image by Alexander Felson/UED Lab and used with permission.
4. Case Study: Jamaica Bay, New York
We use a single case study to illustrate the complex and often compromised landscapes in which urban food webs exist and where ecological concerns and design goals intersect and may come into conflict. Government management agencies with limited resources and conflicting demands face daunting challenges when attempting to reconstruct habitats while simultaneously managing individual species, food webs, and human uses. Past land-use practices in and around Jamaica Bay and what is now Gateway National Park have created a landscape under stress with limited management options and opportunities for change. This same location was the site for influential design competitions in 2007 and 2013 to envision and propose reworking of the landscape and environment of Gateway National Park to integrate people with non-human species, biodiversity, and ecosystems (Brash et al., 2011; Orff, 2016). Together, the case study and design competitions illustrate a shared interest in geographic scales and site challenges, suggesting an expanded collaborative role for designers to work with ecologists in urban food web design and management.
4.1. Managing an Urban Food Web
Jamaica Bay is a 50,586-ha (125,000-acre) saline-to-brackish, nutrient-rich estuary located in the borough of Queens in New York City, New York, USA. Jamaica Bay includes the 10,767-ha (20,607-acre) Gateway National Recreation Area of the US National Park Service (NPS) and the 3,683-ha (9,100-acre) Jamaica Bay National Wildlife Refuge (NWR) managed by the US Fish and Wildlife Service (FWS). Positioned at the intersection of the Atlantic Ocean and the Hudson Raritan estuary, the area includes forests, wetlands, grasslands, dunes, marsh islands, mud flats, tidal creeks, and open water. These ecosystems provide critical habitats for migratory birds, insects, and fish. Jamaica Bay is home to more than 330 seasonal and permanent species of regulatory concern or listed status (including 120 bird species and 48 fish species), and is an important stop-over site for migratory waterbirds (Rafferty and Babson, 2015). The extensive habitats of Jamaica Bay historically supported complex food webs, providing refugia from predators, feeding, spawning grounds, and nurseries for finfish and shellfish, and habitat for resident and migrating birds, crustacean species, and transient and resident fish (Gateway National Recreation Area, 2007). The horseshoe crab (Limulus polyphemus) is a central species in the Jamaica Bay food web that connects it to an intercontinental one (Figure 4). Horseshoe crabs are fished recreationally and commercially along the eastern US coast and have been overfished to unsustainable levels in the northern portion of its range (including New York; Walls et al., 2002; Smith et al., 2017). Horseshoe crabs also are an important food source for migratory birds, particularly the IUCN-designated “near-threatened” Red Knot (Calidris canutus) and Semipalmated Sandpiper (C. pusilla); Red Knot populations along their migratory routes are declining in parallel with declines in horseshoe crab populations (Niles et al., 2009).
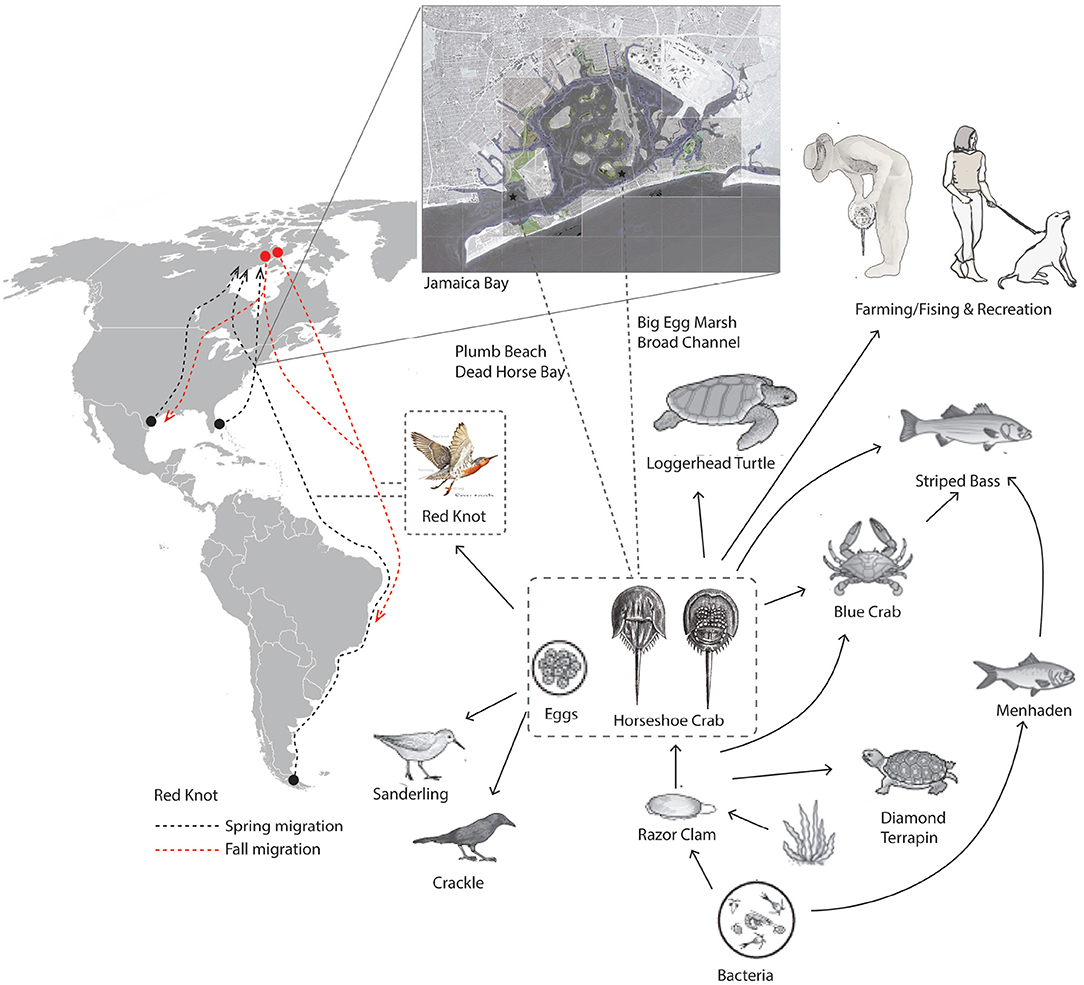
Figure 4. The food web of Jamaica Bay has local, continental, and transcontinental linkages, and includes people and marine and terrestrial species, some of which are of conservation concern. Image by Alexander Felson/UED Lab and used with permission.
Because Jamaica Bay is both a wildlife sanctuary and a place for recreation, it is managed by several different state and federal agencies. It has been mapped and designated by FWS and the US Federal Emergency Management Agency (FEMA) as a protected beach unit pursuant to the 1982 Coastal Barrier Resources Act (US Public Law 97-348; reauthorized in 2000 as Public Law 106-514). The New York State Department of State has designated Jamaica Bay and adjacent Breezy Point as Significant Coastal Fish and Wildlife Habitats, and the New York Department of Environmental Conservation (DEC) has designated portions of the bay as Critical Environmental Areas.
Despite its multiple layers of legal protection and management, the amount of habitat and its quality within Jamaica Bay has declined with increasing urban development and associated land- (and water-) use changes, including channel dredging, shoreline hardening, jetty and causeway construction, sewage treatment operations, and sewage and stormwater discharge from Queens. Concurrently, sea levels have risen and islands that once hosted emergent salt marshes have become intertidal or subtidal mudflats (National Parks Conservation Association, 2007; Rafferty and Babson, 2015). Horseshoe crab populations are impacted as much by this habitat degradation and sea-level rise as they are by overfishing (Smith et al., 2017), and since 1998 they have been managed by the Atlantic States Marine Fisheries Commission under the regulatory direction of the Horseshoe Crab Fishery Management Plan (Kirchoff et al., 2009).
Working in partnership with several environmental non-profit organizations, NPS has been tagging and monitoring horseshoe crab populations since 2013 to determine the extent of their relationships with shorebird populations and migration patterns (Gateway National Recreation Area, 2007). The Science and Resilience Institute at Jamaica Bay built on the extensive research from Delaware Bay to understand dynamics of the horseshoe crab and Red Knot (e.g., Kirchoff et al., 2009; Niles et al., 2009) and develop management actions, including translocation and fishing restrictions for these species and associated food webs in Jamaica Bay.
A number of restoration projects have been developed and implemented in response to the habitat degradation and loss of salt marshes within Jamaica Bay NWR. A collaborative interagency restoration project including NPS and DEC restored and monitored Big Egg Marsh from 2003 to 2008. This restoration project and others completed with the US Army Corps of Engineers under the Hudson–Raritan Estuary Comprehensive Restoration Plan (U.S. Army Corps of Engineers, 2012) have improved salt-marsh restoration approaches that are being used to enhance planning and implementation in other salt marshes (National Parks Conservation Association, 2007; Rafferty et al., 2011). These restoration projects also established collaborative partnerships focused on biodiversity and food-web development. For example, with each successive restoration project, partners were increasingly willing to adapt the NPS's preference for restoring marshes at higher elevations to support distinct species assemblages and add resiliency of the system to sea-level rise. Research also identified effects of hydrologic modifications, eutrophication, and sea-level rise on salt-marsh loss (U.S. Army Corps of Engineers, 2012).
Ultimately Jamaica Bay faces many complex challenges that require coordinated, collaborative efforts not only by ecologists and designers but also by many other stakeholders and individuals who use and care about its long-term sustainability. Two design competitions began the process of envisioning and developing these efforts.
4.2. The “Envisioning Gateway” Design Competition
In January 2007, the “Envisioning Gateway” international competition invited design teams to propose new visions for the future of the Gateway National Park. Set up as a collaboration between the Van Alen Institute, National Parks Conservation Association, and Columbia University Graduate School of Architecture Planning and Preservation, “Envisioning Gateway” sought to tackle the overlapping challenges of this regional national park. This competition encouraged applicants to focus on history, recreation, ecology, waste management, access and transportation, and economic strategies in creating a vision for landscape adaptation that incorporated the converging fields of design, science, technology, and art5. The winning entries, from a pool of 230 entrants representing 23 countries, articulated a vision for human investment in ecosystems for the sake of the species alongside the value for humans. They also illustrated how landscape designers are well ahead of applied ecologists and restoration ecologists in their willingness to create truly novel ecosystems with embedded assemblages of interacting species, including people.
The overall winning design, “ECOTONES” by Ashley Kelly and Rikako Wakabayashi (Brooklyn, NY, USA), focused on “zones of ecological tension…an urban park that creates a microcosm of shifting habitats, program and landforms.” Their design proposed that “people should be educated that ecosystems are in necessary flux, a cycle increasingly complex with today's global climate shifts” (Figure 5). The second place winner, “Reassembling Ecologies” by North Design Office (Toronto, Canada), focused on “optimal conditions for strengthened aquatic and terrestrial ecologies in and around the Gateway National Recreation Park…[and] the need for a stronger definition of programs and activities for park users and an enhanced structure that allows for sensitive ecologies and recreation to coexist.” Reassembling Ecologies also proposed intertwining salt-marsh habitats with beach-goers and boaters in way that would have requiring a heightened sensitivity among human users to avoid the likely seasonal conflicts between people and nature.
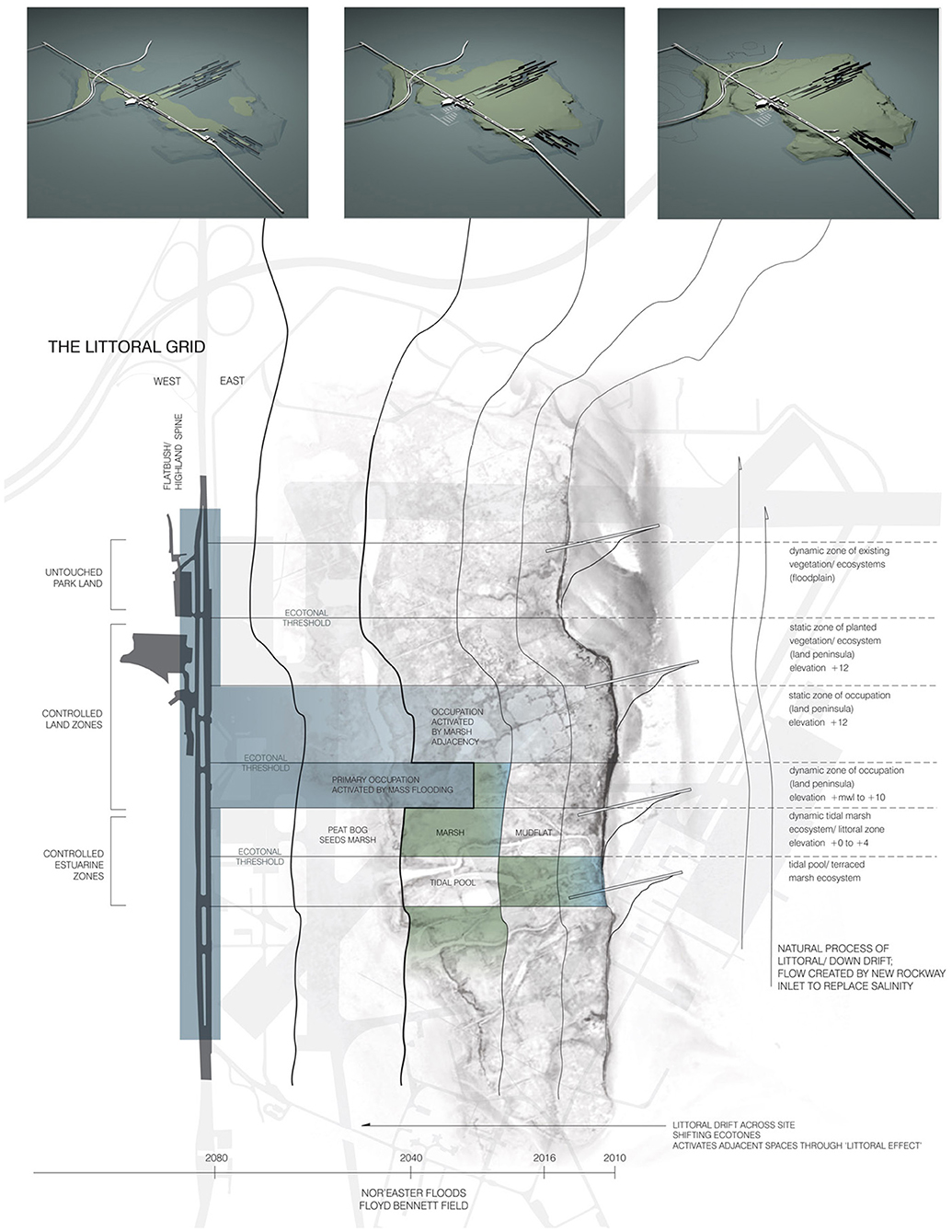
Figure 5. ECOTONES, the winning design of the Van Alen Institute's Envisioning Gateway design competition for Jamaica Bay by Ashley Kelly and Rikako Wakabayashi. Illustration by Ashley Kelly and Rikako Wakabayashi and used with permission.
One honorable mention was awarded to [UN]NATURAL SELECTION by Archipelago Architecture and Landscape Architecture (New York, NY, USA). This proposal highlighted differences between classical (rural) national parks focused on preserving scenic beauty and urban national parks, such as Gateway, which are “all about human contact with the environment and the resulting changes that take place over the centuries. …[I]n the 21st century we are beginning to understand that human health and ecology exist within and not separate from the surrounding environment. Gateway, situated between the nation's greatest concentration of humanity and the ocean, presents a special opportunity to explore the connections and tensions inherent in this amalgam of ocean, land, air and settlement.” A second honorable mention was awarded to Urban Barometer by LOOP|8: Christopher Marcinkoski, Andrew Moddrell (Larchmont, NY, USA), which suggested that Gateway “should be re-designated as a National Eco-urban Research Zone—a territory that both promotes stewardship of existing natural and native resources, but also engages in the active exploration of the relationship between dynamic ecosystems and ongoing anthropologic urbanization.”
“Envisioning Gateway” was a good first step toward addressing the complexity of Jamaica Bay but it didn't take on many ecological challenges. The competition brief provided only a habitat-based framing6. In its formulation, it recast ecological goals in the context of a working landscape in the public realm and de-emphasized species interactions, food webs, and other concerns of academic ecologists.
4.3. The “Rebuild by Design” Competition
Six years after the Envisioning Gateway design competition, “Rebuild by Design” revisited the role of collaborative design thinking for Jamaca Bay. This global competition was launched in 2013 by the US government and the Rockefeller Institute in response to Hurricane Sandy7. Designers were invited to address coastal resilience and flooding from large storms and ongoing sea-level rise. The winning proposal, “THE SHALLOWS: Bay Landscapes as Ecological Infrastructure,” was submitted by the landscape-architecture firm SCAPE and their interdisciplinary team of ecologists, engineers, and designers (including Parsons Brinckerhoff, Philip Orton, SeArc Ecological Consulting, LOT-EK, MTWTF, the Harbor School, and Paul Greenberg) and clearly illustrated an integrated design-thinking approach to addressing challenges of habitat restoration, biodiversity, and large-scale urban food webs.
THE SHALLOWS reimagined how coastal flood infrastructure could simultaneously reduce flood risk and be a social and ecological catalyst. It presented alternative hydrologic regimes and the introduction of a series of architectural habitat generators, including a “habitat breakwater” and “constructed reefs” (SCAPE/Landscape Architecture [Kate Orff], 2015). The breakwater systems were designed to slow erosion and reduce wave action while also supporting the reestablishment of marine ecosystems through the creation of distinct niches. The architectural materials were intended to mimic the distinct “micro-complexity” of the historic reefs of Raritan Bay, creating shelter and foraging areas for finfish, lobsters, and shellfish (SCAPE/Landscape Architecture [Kate Orff], 2015). Like ECOTONES, THE SHALLOWS has not yet been built8.
“Rebuild by Design” represents a much greater emphasis on integrating ecology, design, and broader communities in envisioning and constructing a sustainable future. The results of the Hurricane Sandy competition and others subsequent to it point toward a future of stronger collaborations between ecologists and designers in many urban landscapes.
5. Designing Urban Landscapes with Food Webs in Mind
In section 4, we illustrated the growing interest in visions focused on urban design and construction for biodiversity, food webs, and ecosystem services that blend nature into human environments. Work in this area is being led by designers and landscape architects; ecologists are infrequently included in the design, engineering, or construction teams. We recognize that designing food webs—urban or otherwise—remains aspirational for ecologists (including ourselves) and that standard ecological approaches, such as species (re)introductions or removals, habitat restoration, and rewilding to promote specific assemblages of species are still being developed, studied, and tested. Although ecological theory tends to be included sporadically in the design professionals' toolbox, it is clear that the goal of establishing stable urban food webs is part of the growing interest in biocentric design and construction of biophilic cities. If ecologists can collaborate with designers in creating urban landscapes and in managing them, there is a higher likelihood of creating stable food webs in them. But pursuing such collaborations will require ecologists to commit to working in tandem with informed design professionals.
Collaborations between ecologists and designers to date have led mostly to unintentional (un-designed) food webs (Figure 2 and section 5.1), but in a few, recent instances, these collaborations have led to more intentional, designed ones (section 5.2). In either case, these collaborations have tended to target vegetation, soil, or water (e.g., Calkins, 2012); emphases have been on biodiversity and ecosystem services. Notable examples include the UrBioNet program9 and the annual International Urban Wildlife Conference10.
5.1. Unintentional Urban Food Webs
We distinguish three types of, or approaches to, the rehabilitation, restoration, or planting of vegetation and developing habitats that might eventually support foodwebs. Each of these approaches will be familiar to ecologists. Although the eventual occurrence of food webs in these habitats may be desired, we emphasize that the actual species composition that may arise usually is unintentional.
First, urban food webs with desirable or undesirable species may arise spontaneously (Del Tredici, 2010) as “unintended side effects of land management.” For example, unintentional foodwebs can assemble in urban stormwater retention ponds or catch-basins that create wetlands and attract wildlife or act as refugia for species of conservation concern (e.g., amphibians; Hassall, 2014; Palta et al., 2017). Depending on their location and upland land uses, these “refugia” may actually function as ecological “sinks” (sensu Pulliam, 1988). Similarly, bird feeders set out by urban and suburban gardeners are used to attract birds, but may also attract squirrels, black bears, white-tailed deer, white-footed mice, deer ticks, and spirochetes, all of which can carry a variety of diseases (Goddard and Dougill, 2010; Sterba, 2012). In contrast, forgotten clover-leaves at highway interchanges or abandoned vacant lots, for example, can support multi-trophic food webs that have assembled themselves without any human intervention save for the inadvertent creation of “unused” space (Grewal et al., 2011).
Second, designers may “let nature take its course” by leaving a system alone either intentionally or because of lack of time, knowledge, or resources (see also the “Wildlands” approach of Foster et al., 2010 and the urban re-greening studied by Gallagher et al., 2018). Letting nature take its course builds on the well-established conservation strategy where parcels of land, typically of relatively high ecological value (e.g., waterways, riparian buffers, steep slopes, wetland complexes, or remnant forest patches), are identified and conserved over time. Landscape architects also have a history of embracing aspects of letting nature take its course in their designs. For example, in remediating degraded sites, Julie Bargmann (University of Virginia) embraces their beauty and the need to preserve, rather than erase, their histories. Her D.I.R.T. [Dump It Right There] Studio incorporates an economy of means alongside urban succession to help reshape and regenerate post-industrial sites. The goal of Bargmann's design approach with “the good, bad and the ugly of post-industrial sites is to challenge the persistent pastoral ideal. That beauty emerges through empathy with how that landscape has come to be, what is latent within it and what potential has yet to be revealed. Sort of like the story of the ugly duckling that transforms into a swan11”.
Finally, ecologists and design professionals may intentionally create habitat using plants (and perhaps new soil); it is then expected that any desired fauna will colonize the constructed habitats. Palmer et al. (1997, 2010) has called this expectation the “field of dreams” hypothesis; we call this approach “build-it-and-they-will-come.” The build-it-and-they-will-come approach is used extensively in rehabilitation, restoration, and regeneration projects (Fraser et al., 2015) and there are many examples of successful restoration projects using this approach in natural areas or areas with relatively light human footprints (e.g., Lister, 2007; but see Palmer, 2009). The build-it-and-they-will-come approach is being extended by design professionals who are creating biodversity and hybrid projects incorporating urban design and restoration ecology; THE SHALLOWS (section 4.3) includes many build-it-and-they-will-come aspects. Many other designed landscapes are exemplars of build-it-and-they-will-come habitats: botanical gardens (e.g., the Lady Bird Johnson Wildflower Center in Texas, USA12) and urban nature parks (e.g., Forest Park in Portland, Oregon, USA13) often host mutualists fauna in bipartite networks (e.g., plants and their pollinators) or simplified food webs.
5.2. Intentional, Designed Urban Food Webs
We also distinguish three broad approaches to the intentional design of urban food webs. The first occurs through what we call “ecological theory applied through design,” and includes the use of foundation species (sensu Ellison, 2019) or ecological engineers (sensu Jones et al., 1994) to catalyze food-web development. The second, “research partnerships informing design” involves the co-development and use by designers and ecologists of deliberate experiments to enhance designs and create opportunities for embedded research (Felson et al., 2013a; Ellison et al., 2020). The third approach, “creative and aspirational ecological design” includes biocentric design, urban species introductions, and rewilding (Corlett, 2016; Svenning et al., 2016). As these intentional-design approaches likely will be less familiar to ecologists, for each of them we provide an example and elaborate on their values and constraints. These examples reveal how intentionality can inspire design thinkers to envision innovative strategies in urban settings that respond to these constraints and create novel circumstances geared toward more successful outcomes. In section 6, we identify key challenges that can arise when ecologists, landscape architects, and designers collaborate on intentional-design projects, highlighting that the different discipline-based theoretical concepts of form and function can lead to the parties talking past one another.
5.2.1. Ecological Theory Applied Through Design
Designers have some history working directly with research ecologists (section 3), but one obstacle has been identifying ecologists willing to work with designers on projects that may have limited value as research sites or for conservation (Felson, 2013). Designers frequently subcontract environmental consultants to delineate critical habitats (e.g., wetlands) and secure environmental permits, but they also have a history of working with professional or academic geographers and landscape ecologists (Deming and Swaffield, 2011; Musacchio, 2011). Ecologists are increasingly developing theory in areas that seek to bridge with design and management. Ecological landscaping, for example, takes a systems approach for investigating biotic and abiotic conditions, biodiversity, and ecosystem patterns and processes in urban landscapes (Byrne and Grewal, 2008). Plant scientists are increasingly engaging in critical questions about novel urban plant communities, such as ruderal landscapes (Del Tredici, 2010) and hybrid native-exotic vegetation, and testing ways in which such communities can support a rich native invertebrate fauna that also fosters predatory vertebrates (Hitchmough, 2008)14. Urban reconciliation with wildlife has focused on living roofs and green walls (Francis and Lorimer, 2011). Ornithologists working with designers have contributed expertise on addressing bird-friendly urban design and building systems to address particular target species of concern (City of Calgary, 2011; Holloway, 2018). More recently, entomologists, herpetologists, icthyologists, and marine scientists are collaborating with designers on designed rehabilitation and restoration projects (e.g., Ellison et al., 2020). These collaborations are certainly benefiting the design professions while creating new avenues for ecological inquiry.
Oyster-tecture (Figure 6) is an example of a design that incorporated ecological ideas and concepts to create an ecological infrastructure. The design for Oyster-tecture was created by the landscape architecture firm SCAPE for the 2010 “Rising Currents” exhibition at the Museum of Modern Art (Orff, 2010). Configured as an infrastructural armature, Oyster-tecture proposed using an ecological engineer to anchor the design. The oyster reefs would simultaneously take up nutrients, remove toxins from New York's Gowanus Canal, physically attenuate waves, and increase habitat around Governor's Island.
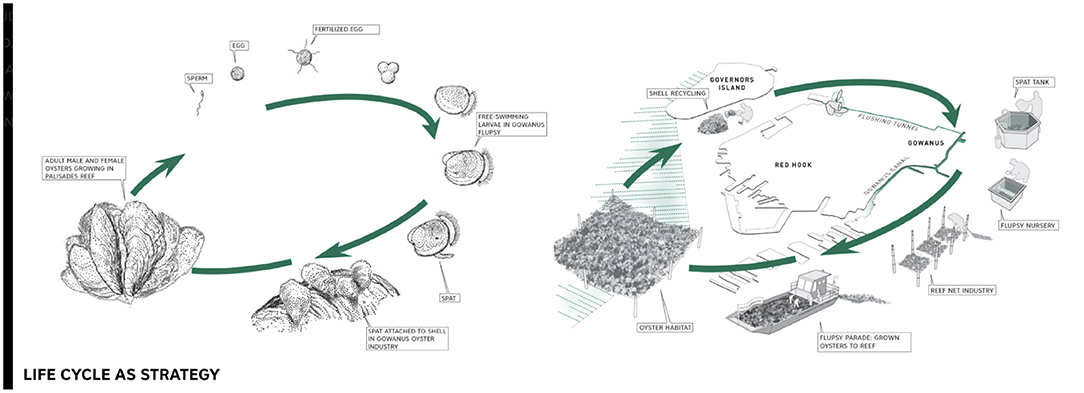
Figure 6. The proposed design for Oyster-Tecture is an example of Ecological Theory Applied through Design. Illustration courtesy SCAPE/Kate Orff, developed for the Musem of Modern Art's ‘Rising Currents’ exhibit (2009).
The design in this project occurs at the interface and integration of the species lifecycle with the urban systems and fabric. The design reinterprets and restructures the lifecycle of the organism into different zones of the city to perform an ecological function. At the same time, the organism contributes to the aesthetic and form-making of the proposed interventions. The organism's lifecycle is overlaid and nested into the urban infrastructure and remnant ecosystems of the city (Steiner et al., 2013; Orff, 2016). The outcome is a spatially and temporally designed lifecycle attuned to the hydrology and industrial pollution of the city. The oyster would reprogram the harbor geographically, respond to anthropogenic impacts to the harbor, and take advantage of urban planning and technology to bring the oyster as an ecosystem engineer up to the scale necessary to initiate remediation of the harbor ecosystem (Orff, 2010). Although this design has not been built, it contributes to the Gowanus By Design initiative15.
Like the habitat-based build-it-and-they-will-come approach (section 5.1), Oyster-tecture focused on a single species. But unlike a plant-based creation, Oyster-tecture had as an explicit goal that an ecological engineer would catalyze the assembly of a stable food web. This design proposal went on to influence multiple subsequent built projects, including SCAPE's Jamaica Bay project and the Rebuild by Design Living Breakwaters project in Staten Island, New York, both of which incorporated food-web considerations (see section 4.3 and Orff, 2016). Although ecological designs such as Oyster-tecture seek to leverage ecological processes, they are distinct from conservation or restoration efforts. They accept anthropogenic impacts, stress innovative solutions, and direct attention and resources to cities where the majority of people live and where investments increasingly are being targeted for improving wildlife habitats while providing education about ecology to broader audiences.
5.2.2. Research Partnerships Informing Design
Research partnerships informed the design of the Elliott Bay Seawall in Seattle (Washington, USA), built in 2017. The landscape architecture and urban design firm, James Corner Field Operations, led a large multi-disciplinary team of structural, coastal, storm water, and geotechnical engineers, habitat engineers, and an artist to combine infrastructure with habitat creation as part of a 1,128-m (3,700-ft) public waterfront and seawall replacement (Figure 7)16. The three primary project goals were to enhance nearshore marine habitat that would support the algae and detritus fed on by the preferred prey of juvenile Pacific salmon (Oncorhynchus spp.), re-establish an interrupted migration route from natal streams out to the ocean, and create a high-quality pedestrian promenade (Sawyer et al., 2020). The team built an elevated seafloor and “fish-smart” seawalls (Cordell et al., 2017; Morris et al., 2018) faced with textured substrates. The elevated seafloor included an engineered pocket beach, a saltmarsh eco-bench, an offshore rock-kelp-reef system, and a riparian zone all functioning together as an integrated habitat. The seawalls simulated the physical configuration of natural shallow-water habitat, increasing invertebrate colonization (e.g., barnacles) and abundance of epibenthic invertebrate prey preferred by juvenile salmon (Toft et al., 2013; Coombes et al., 2015; Cordell et al., 2017; Strain et al., 2018). Above this belowground corridor is a pedestrian promenade that includes glass surfaces and perforated panels to allow light to penetrate and support growth of algae and other plants. The promenade also serves as an integrated public realm that engages visitors in understanding marine food webs. This is also an example of an innovative “broader impact” that ecologists doing research on designed food webs could realize through collaborating with designers.
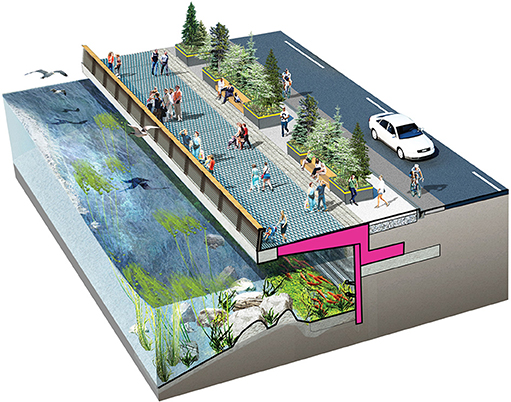
Figure 7. The Elliott Bay Seawall is an example of a Research Partnership Informing Design project. It includes an elevated seafloor and bench re-established a long-interrupted salmon migration route. The light penetration and water depth conditions mimic a natural shoreline and provide a refuge from predation. The precast concrete seawall face panels and habitat shelves and cantilevered light-penetrating system panels support the salmon migratory path. Overall, the restoration activities have increased substrate complexity and epibenthic invertebrate taxa richness. The project team for the seawall includes WSP (formerly Parsons) (Prime Firm, Program Manager, Design Manager, Permitting, Environmental Documentation, Procurement, Utilities Engineering, Geotechnical Engineering, Landscape Architecture, and Structural Engineering), Magnusson Klemencic Associates (Lead Engineer for Public Realm and Habitat Design Integration), Perteet and SvR (Civil and Drainage Engineering), Moffatt and Nichol (Coastal Engineering), COWI North America and Exeltech Consulting, Inc. (Structural Engineering), Shannon and Wilson, Inc. (Geotechnical Engineering), Hart Crowser (Habitat Engineering), Harrison Design Landscape Architecture (Local Landscape Architect), Haddad/Drugan (Artist), and Jacobs (Construction Management). Image used with permission from James Corner Field Operations.
5.2.3. Creative and Aspirational Ecological Design
Alongside the two previous nascent approaches to intentional design of food webs, more creative and aspirational designs are emerging that build on investigations of roles that humans and urbanization play in eco-evolutionary dynamics (Alberti, 2015; Lambert and Donihue, 2020). The recognition that strong selection occurs in urban areas in response to rapid environmental changes, and that ecosystem services, stability, and species interactions are affected on short time scales raise questions about evolutionary consequences and dynamics in designed projects. Comprehensive designs of novel ecosystems are one avenue to addressing these (and other) constraints and focus on the likelihood of success of alterations to habitats or landscapes (Hobbs et al., 2014; Kareiva et al., 2018).
An example of a collaborative creative and aspirational project is the East River Marsh Planter—developed by Ken Smith Landscape Architect with the first author—that was proposed for installation along the bulkhead at 34th Street in New York City (Figure 8; Amidon, 2006). This project was built, but in a different configuration17.
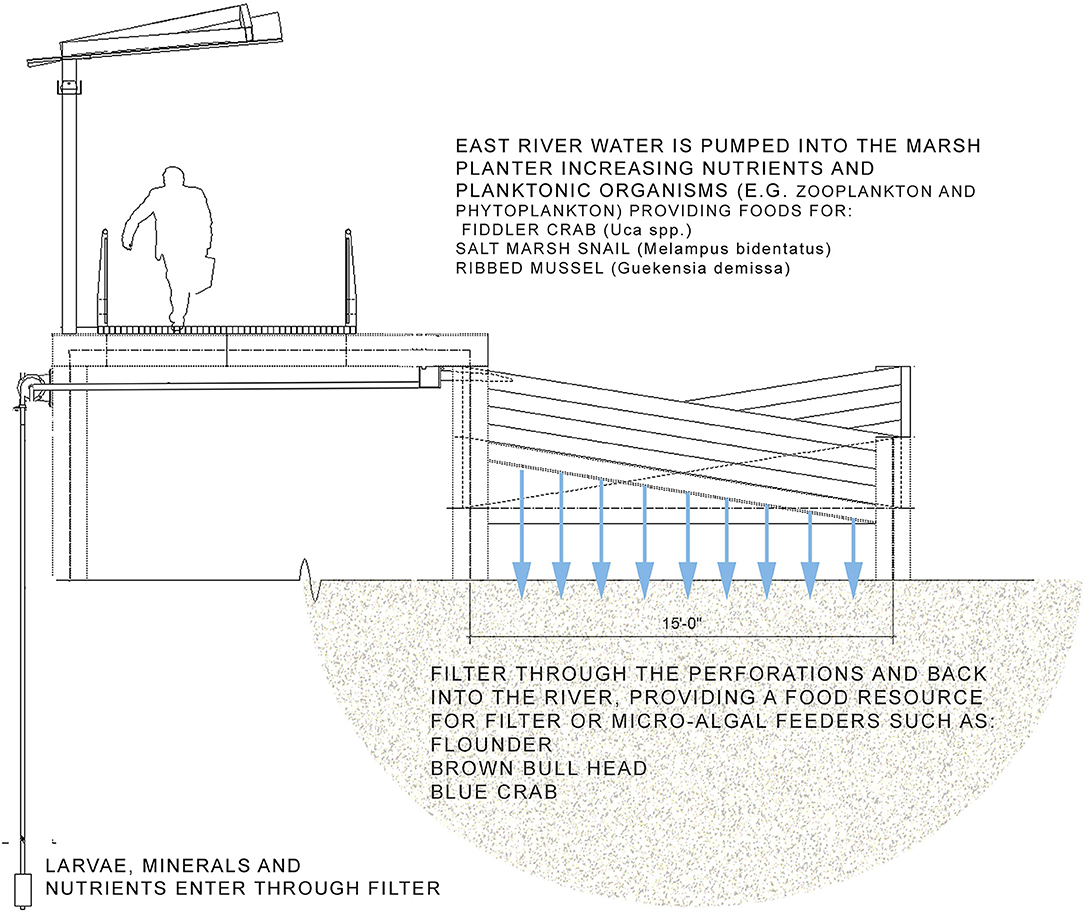
Figure 8. The proposed design for the East River Marsh Planter is an example of Creative and Aspirational Ecological Design. Design by Ken Smith Landscape Architect; drawing by Alexander Felson and used with permission.
The original design was intended to be a food-web catalyst and illustrated a design approach to influencing multiple trophic structures. Its key design concept is the insertion of an artificial, technologically managed “interlayer”: pumps bring in water with organisms from low trophic levels that are inserted into a series of perforated “planters”—elevated boxes planted with native saltmarsh cordgrass (Spartina alterniflora). Populations of these organisms are grown and then reintroduced in an in situ “production line” to foster food-web assembly in the East River. In an engaging, educational display of ecological dynamics, the pumps would flood the planters every morning and evening, co-incident with people's daily commute.
The design of the East River Marsh Planter aesthetically combines ecological principles and technology. The planter was intended to use engineered soils including hydrogel—a polymer that holds water—to act as an artificial mud layer and allay concerns about desiccation in an elevated artificial marsh. The macroinvertebrate larvae and minerals pumped from the East River supplied nutrient-rich water for the planters while seeding them with species occupying a low trophic level, yet one higher than the plants (“resources”) where build-it-and-they-will-come projects usually stop. The nutrient-rich water and the associated invertebrate larvae supported a set of intermediate-level consumers, including deposit-feeding fiddler crabs (Uca spp.) and salt-marsh snails (Melampus bidentatus) located in cells within the planters. A freshwater emitter would periodically flush out detritus and plankton from the planters through perforations in their bases, providing nutrient-rich food for higher-level consumers, including blue crab (Callinectes sapidus), flounder (Pseudopleuronectes americanus), and brown bullhead (Ameiurus nebulosus). As mesocosms, the planters would enable ecological studies of food-web development and evaluation of the ecological value of habitat design and restoration strategies. Finally, the ecological mesocosms would be situated along the routes of >30,000 daily commuters, presenting ecosystem services to broader audiences in an aesthetically pleasing way.
The marsh planter is an example of a designer ecosystem that attempts to balance site constraints with human social and economic needs. The designers recognized that site conditions and pressures on organisms have changed, and they sought to maximize ecosystem services in a human-modified environment. The planters themselves are an integral part of a new ferry terminal; they mark the entrance to the terminal (economic service) and the design provides educational opportunities to daily commuters (social service). Although designer ecosystems and classically managed systems, such as Jamaica Bay (section 4) each blend human social, political, and economic demands with technology, creativity, and innovation to support multiple species and provide ecosystem services, designer ecosystems are much more flexible in the species that are used (e.g., not necessarily native) and are more likely to include regular human interventions to enhance ecosystem services and facilitate system longevity.
Some rewilding projects also are creative and aspirational. Rewilding takes re-introductions to a new level by replacing locally extinct species with either translocated populations of the same species or ecological “proxies” that can replace the ecosystem services of extinct species (“trophic rewilding,” sensu Svenning et al., 2016). Interest in rewilding is growing rapidly, but is still much debated (Donlan et al., 2005) and criticized by ecologists (Caro, 2007; Oliveira-Santos and Fernandez, 2010; Lorimer et al., 2015). Yet, rewilding projects are moving forward.
Trophic rewilding privileges restoring trophic interactions, not necessarily involving original fauna (Svenning et al., 2016). Most examples of trophic rewilding involve reintroduction of missing herbivores (reviewed by Svenning et al., 2016). These can be succeeded by sequential reintroductions of extirpated animal species, including carnivores, that foster restoration of ecological interactions with the remaining flora and fauna (Fernandez et al., 2017). The reintroduction of wolves into Yellowstone National Park is the best example of re-establishment of a trophic cascade following reintroduction (“rewilding”) of a top predator (Dobson, 2014). An example of urban rewilding is the reintroduction of two seed dispersers, the red-humped agouti (Dasyprocta leporina) and the howler monkey (Alouatta guariba) into the Tijuca National Park18, a 3,953-ha forest fragment located within the city limits of Rio de Janeiro, Brazil (Fernandez et al., 2017). Because the Tijuca forest is surrounded by the city, re-introduced fauna cannot disperse to other forest fragments (Fernandez et al., 2017). Twenty-five dung-beetle species have been observed using the howler-monkey dung (i.e., a trophic effect) and there is the hope that the agoutis and monkeys will foster forest regeneration (Fernandez et al., 2017).
6. Challenges and Opportunities in Designing (for) Urban Food Webs
The examples in sections 4 and 5 are intended to suggest ways forward for bringing ecologists and design professionals together in collaborative efforts to deliberately design and study habitats or landscapes for urban food webs. There are many theoretical, practical, and cultural obstacles to designing, building, and maintaining urban food webs and building collaborations between ecologists and design professionals. In this section we identify the key challenges and opportunities to address them.
6.1. A New Frontier for Research and Collaboration
Recognizing existing remnant food webs, cultivating them through architectural design, and exploring ways to construct novel urban food webs will require new research and practical development. Ecologists will need to identify how human activities have already altered food webs (e.g., Christie et al., 2010). Design professionals will need to work with ecologists to translate findings from ecological theory into projects that can be built and maintained efficiently and cost-effectively. Designers are particularly keen to apply ecological theory at spatial and temporal scales relevant to people living in cities (Jabareen, 2013; Mosbach, 2013; Steiner et al., 2013). Designers also bring aesthetic considerations and methods of translating form into (ecological) functions and services (and vice-versa). Ecologists and designers alike need to understand each other's vocabulary and aesthetics and work outside their individual comfort zones to collaborate effectively on the design, construction, and monitoring of, and research into, urban food webs (see section 6.4).
6.2. Ecological Considerations
6.2.1. Biodiversity and Habitats Are Parts of, But Not a Proxy for, Urban Food Webs
Individual species are parts of food webs (Figures 1A–C) and recent efforts to identify and enhance biodiversity in cities (e.g., Kowarik, 2011; Uchida et al., 2021) are a first step in its appreciation not only by design professionals but also by the broader public. Yet, knowing there are, for example, many species of ants on Broadway (Pećarević et al., 2010) or that there are unique scaling relationships for urban biodiversity (Uchida et al., 2021) does not describe functional roles of species in a food web. Urban food webs also may have lower species diversity and occur in weakly or unconnected habitat patches (e.g., Start et al., 2020). Such patches in designed and maintained landscapes may function as habitat sinks or ecological traps for species in cities, but such sinks rely on ex-urban populations for replenishment. Ecologists need to identify combinations of species in multiple trophic levels that can survive and function in the fragmented landscapes of cities (e.g., Start et al., 2020) as a prelude to designing habitats that could support urban food webs (Roudavski, 2020).
(Re)building or restoring urban food webs also will require habitat rehabilitation co-incident with, or followed by, management of introduced or undesired species. Such efforts are already underway in many parts of the world. For example, the blue-banded bee (Amegilla cingulata) in Melbourne, Australia, is being actively supported using planted habitats, but the designers and managers are already considering how to construct and maintain metanetworks of bee subpopulations (Mata et al., 2019). Although their approach does not yet include additional trophic dynamics (e.g., introducing neon cuckoo bees or bird species that prey on blue-banded bees), this build-it-and-they-will-come approach recognizes that food webs are often anchored by a few key species. Establishing and managing urban food webs will require additional research and analysis on how specific species interact with the rest of the altered regional and urban systems.
6.2.2. Urban Food Webs Integrate Human and Ecological Concerns
All of the documentation and analysis of non-urban food webs is unlikely to be captured in cities where human behavior and urbanization reshape the networks of species interactions and food webs can unravel and disassemble (McCann, 2012; Start et al., 2020). Urban environments will be influenced and constrained by geometry and fragmentation of the urban habitat matrix. Such influences, constraints, and limitations range from effects of urban forms (buildings, street layouts, and landscaping) on local microclimates to hydrological alteration and interruption of migration corridors at larger spatiotemporal scales. The sizes, forms, and networks of urban parks, waterways, gardens, lawns, and street tree populations have interacting impacts on food-web structure, dynamics, and the services they provide. Remnant urban food webs are species-poor, sparse patches surrounded by inhospitable environments (Start et al., 2020).
Urban food webs occur in human-dominated landscapes and people often are apex predators (as in Jamaica Bay). Yet, there are few examples outside of fin- and shellfisheries (e.g., Pauly et al., 2000; Moreno, 2001; de Boer and Prins, 2002; Reitz, 2004; Essington et al., 2006; Braje et al., 2007; Graham et al., 2017) of ecological studies of “natural” food webs that include people as predators, even though people routinely kill (although rarely eat) herbivores and predators in cities. Ecologists need to study and understand how human behaviors and desires (e.g., controlling or eliminating insects, rodents, or other “pests”) affect urban food-web dynamics and ecosystem services. Results of such studies, perhaps included in experiments within designed landscapes, could help identify critical factors that define or constrain the management of urban food webs. Ecologists also need to educate and communicate to homeowners and park managers that different landscaping practices will have different effects on food webs. For example, embracing snags and downed wood as part of our parklands and introducing artificial nesting environments may sustain food webs whereas raking, removing, and composting leaves or chipping and burning coarse woody debris removes critical habitat and trophic connections (e.g., Hoyle et al., 2017).
6.2.3. Temporal Dynamics May Be Unappreciated or Arrested in Urban Food Webs
Like plant ecologists studying succession, animal ecologists have long appreciated that food webs assemble and change through time as individual species progress through their life-histories, some species go (locally) extinct, or other species colonize habitats that are already occupied (Figure 1D; MacArthur and Wilson, 1967; Drake, 1990a,b, 1991; Ellison and Gotelli, 2021). Ironically, most ecological research on food webs has emphasized their static description (see reviews in McCann, 2012; Ellison and Gotelli, 2021), but that does not negate the fact that food webs are dynamic, constantly changing entities (e.g., de Ruiter et al., 2005; Pilosof et al., 2017). Ironically, many restoration projects (regardless of defined baseline) seek to manage conditions to maintain a specific, temporally invariant species composition.
Similarly, a specific and temporally invariant species composition may be desired by city planners, designers, or clients for designed habitats and urban food webs, Maintaining these habitats or restored sites to preserve a particular design or assemblage of species is analogous to arresting ecological succession (Del Tredici, 2007). Design and restoration goals often contrast with ecological reality; the former's emphasis on planning, cultivation, neatness, rigidity, and status contrasts with the latter's emphasis on spontaneity, wildness, messiness, adaptive, and flux and change (Del Tredici, 2007). Design practitioners similarly focus on maintenance, whereas ecologists focus on management. For designers, operations and maintenance is an ongoing, underfunded challenge, whereas for ecologists, management is an expected, fundamental component of habitat or trophic restoration. A central challenge for ecologists working with designers is to identify and co-design resilient urban ecosystems while promoting effective and adaptable experimental management strategies appropriate for urban sites and human subjects.
6.2.4. Urban Food Webs as Experimental Systems
Restoration ecologists have attempted with mixed results to reintroduce (or rewild) species (see section 5.2.3; and Caro, 2007; Svenning et al., 2016; Perino et al., 2019). Some reintroductions seek to restore ecosystem functions or services (e.g., the reintroduction of the golden lion tamarin, Leontopithecus rosalia, in northern Rio de Janeiro; Kierulff et al., 2012; IUCN/SSC, 2013). Other species may be relocated or introduced into new locations where they can survive. For example, in the construction of the Railyard Park in Santa Fe, a population of prairie dogs that lived on the railyard was deemed a problem for the planned park; the prairie dogs were relocated to a new location where they would not come into conflict with people (Chacón, 2013). The deliberate introduction of species into urban natural areas has a long history (e.g., starlings and sparrows [Wing, 1943]; arthropods for biological control [Vickery and Kevan, 1983; Aslan et al., 2014; Evans, 2016]) but these are not considered as reintroductions or rewilding. Ecologists have a new research opportunity to consider introductions of predators into urban environments as a way to study (re)introductions of species into food webs in the “unnatural” systems represented by cities where ecological “disasters” or trophic cascades may be of less concern than they might be in more “natural” (i.e., non-urban) systems (e.g., Aslan et al., 2014; Egerer and Kowarik, 2020).
6.3. Design Considerations
6.3.1. The Need for Maintaining a Messy Aesthetic
Aesthetic and functional goals are important considerations for designers. The relationship between aesthetics and ecology as it relates to landscape design, planning, and management can be well-aligned or in opposition to one another. Aesthetic goals of, for example, scenic beauty or appreciation of nature may map closely or clash with indicators of ecological quality (Gobster et al., 2007). Embracing “messy order” as an aesthetic that allows some geometry and refinement of spaces while allowing for organic growth to occur will help create habitat to support urban food webs (Nassauer, 1995). Yet designed landscapes, even messy ones, need to be maintained in their desired states (see also section 6.2.3). Parks departments are always challenged to keep up with maintenance and many designers posit maintenance issues as the major factor leading to failure of landscape designs (Aronson et al., 2017; Hoyle et al., 2017; Riley et al., 2018). Controlling and managing multiple species is difficult enough in restoration projects (Fraser et al., 2015); keeping food webs in a desired state will be difficult for already overextended maintenance crews. Designers need to create robust systems that have management strategies embedded in the design. This may be achievable by increasing redundancy within urban food webs but ecologists still do not know whether or how often food webs contain functionally replaceable taxa, and which taxa they might be (e.g., Chalcraft and Resetarits, 2003; Petchey and Gaston, 2006; Hagen et al., 2012; Gray et al., 2016). Maintenance staff will need additional training to increase their habitat management expertise.
6.3.2. Using Design to Change Human Perceptions of Food Webs
People rarely think about predation, and when they do, perceptions are generally negative (e.g., Archer-Lean et al., 2015; Bencin et al., 2016; Miranda et al., 2016; Eshete et al., 2018). Including food webs in cities will require overcoming these negative perceptions. Zoos are well-known examples of designed environments that prioritize persistent form over ecological dynamics and function. Visitors witness single or interacting species in artificial, static conditions that are modeled to look “natural” in immersive exhibits but where actual predator-prey interactions cannot occur (Figure 9). What are reasonable designs that could induce favorable perceptions of predation and inculcate an aesthetic desire for stable food webs (Roudavski, 2020)? Will these designs align aesthetics with ecological function (Gobster et al., 2007)? Designers must also consider the challenges of designing and managing urban food webs and the “cans of worms” that could be unleashed with proposals that use animals or trophic structures as material components (Roudavski, 2020).
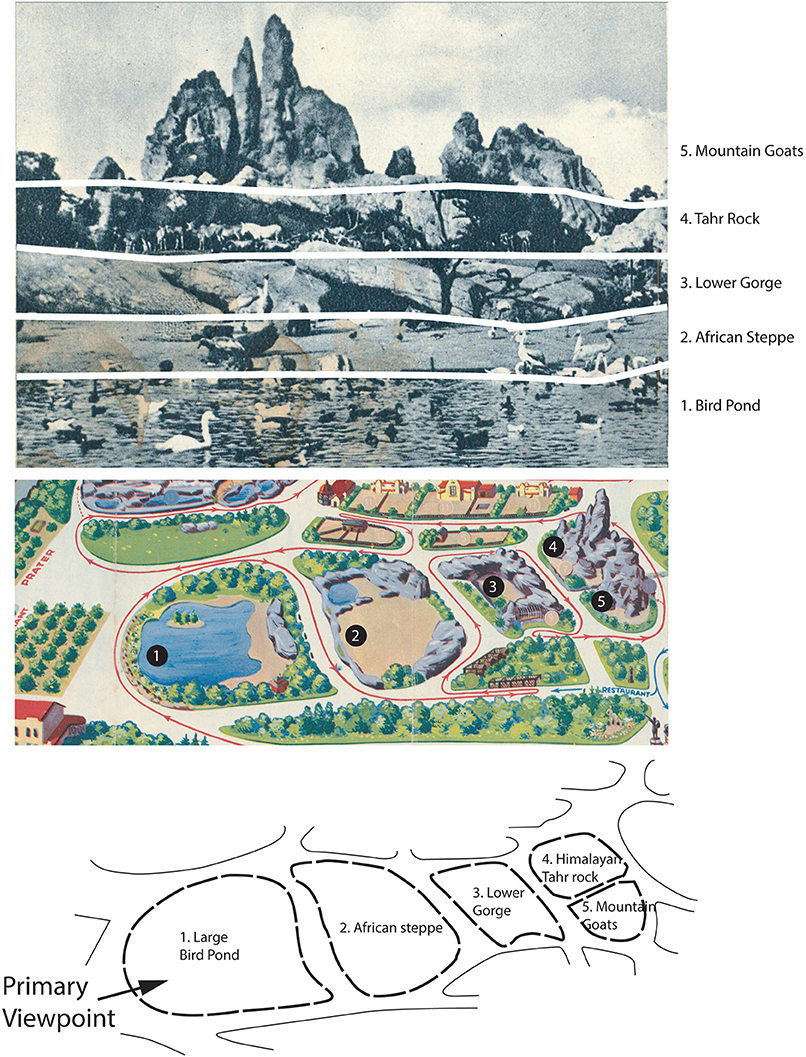
Figure 9. Zoo enclosures are artificial, theatrical spaces where animals are put on display. Only the form of the food webs to which the animal belongs is illustrated; its sole function is to represent nature. The Hagenbeck Tierpark Zoo near Hamburg, Germany (constructed 1907) was the first zoo to replace cages with open enclosures and moats and berms to manage animal. The African exhibits illustrate potentially interacting species whose functions in their ecological community are entirely lost. People standing at the primary viewpoint (indicated in the bottom panel) see an assemblage of species that each occupy their own enclosure. This is an example of form-driven aesthetics created by perspectival viewpoints. The theatrical arrangement follows functional considerations related to vantage points and perspective (top and middle panels). Image sources: (Top) Carl Hagenbeck's Tierpark, Hamburg. 1935. Die Entwicklung des Hauses Carl Hegenbeck (https://www.davidrumsey.com/luna/servlet/detail/RUMSEY~8~1~299571~90070511:Text--Die-Entwicklung-des-Hauses-Ca; CC BY-NC-SA 3.0); Diagram by Alexander Felson; (Middle) Plan, Carl Hagenbecks Tierpark, Altona/Stellingen/Hamburg, (https://www.davidrumsey.com/luna/servlet/detail/RUMSEY~8~1~299572~90070510:Plan,-Carl-Hagenbecks-Tierpark; CC BY-NC-SA 3.0); (Bottom) Diagram by Alexander Felson and used with permission.
6.4. Urban Food Webs Require Finding Common Ground That Recognizes and Resolves Differences in Language and Vocabulary
The aforementioned technical challenges of designing (for) urban food webs are complicated by the differences between ecologists and designers in aesthetic priorities, desired ecosystem services, and perspectives about how the world “works” (Gobster et al., 2007). In short, ecologists and designers speak very different languages (Johnson et al., 2002; Ellison and Buckley Borden, 2021). In our experience, the crux of these differences lies in the different meanings, values, and cause-and-effect relationships between form and function that are used and understood by ecologists and designers.
Our intent here is not to review the 150 years of ecological research on food webs (reviewed by McCann, 2012; Ellison and Gotelli, 2021) or the similarly long debate among architects and designers on the relationship between form and function. Rather, we first identify how ecologists define and describe the form of food webs and measure their function. We then turn to the aesthetic and intellectual challenges designers face when using ecological knowledge to design landscapes intended to support specific ecological functions (e.g., Figures 1D,E; Mozingo, 1998; Lister, 2007; Musacchio, 2011; Nassauer, 2012; Forman, 2014, 2016; Orff, 2016; Parris et al., 2018). We emphasize that designers' more fluid perspectives on the relationship between form and function differs strongly from ecologists' generally inflexible expectation that form derives from function (for a notable exception, see Gould and Lewontin, 1979).
6.4.1. Form and Function in Ecological Food Webs
Ecologists describe the “form” of a food web (which ecologists also call its “structure”) with illustrations of its network topology (i.e., food-web diagrams: Figures 1A–C). Structural properties of food webs (and of networks in general), such as the number of species (“nodes”), the number of trophic levels, the sizes or traits of individual species, and the degree to which species are linked to one another (via “edges”), are system-independent metrics that ecologists use to compare forms of different food webs and create general theories of food-web structure (Lau et al., 2017). The edges of food-web diagrams illustrate predator-prey interactions or the flow of energy and nutrients between species, and within and across trophic levels. These edges define the ecological “functions” or “services” of a food web: processes, such as production (e.g., Baiser et al., 2013), consumption or predation (Paine, 1966; Valls et al., 2015), and energy flow (e.g., Borrett, 2014).
Ecologists generally assume that the form (structure or topology) of a food web is a direct consequence of its ecological functions (predator-prey relationships and energy flow between individual species: Figures 1A–C and the set of images from lower left to upper right in Figure 2). This idea parallels Sullivan's 19th-century maxim for designers that “form ever follows function” (Sullivan, 1896). However, an ecologist's “form” is not related to the physical form of a designer's habitat or landscape, but only defines the topology of the food web itself. In support of this hypothesized “one-way street” on which function defines form, ecological studies of food webs that emphasize function (bottom-up or top-down control or fluxes of energy and nutrients) generally pay little attention to the underlying topology (form) of food webs beyond identifying particular functional groups of organisms (e.g., primary producers, herbivores, carnivores, or decomposers).
6.4.2. Form and Function in Landscape Architecture and Design
Landscape architects have gone through multiple cycles of signifying “natural” ecological relationships in designed landscapes as “forms” (Figure 10; Treib, 1989; Girot, 2016). For example, in the 17th-century French style of André Le Nôtre and others, form (as formal composition), not function, was the fundamental driver of landscape design (form; Figure 10B). This evolved in the 18th-century English Picturesque style into complicated overlays of formal composition through the design of paths and vantages points, with scenes imitating nature (Figure 10C). In a more “form-follows-function” approach, 20th-century Modernist architects stripped buildings of ornament and other formal devices while embracing and foregrounding the functional aspects of buildings (Figures 10D,E). Landscape architecture reinterpreted the modernist framework with complex geometries and imposed controls that allowed designers to use plants and soil as materials, and permitted organic growth and messiness (section 6.3.1; Nassauer, 2012).
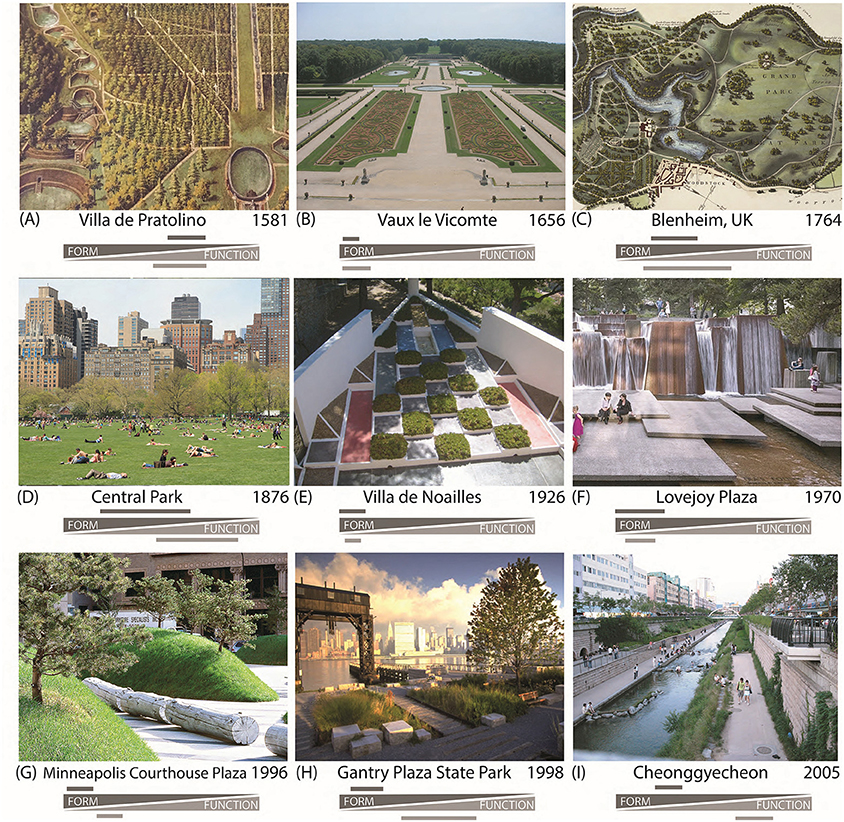
Figure 10. Representative shifts in the attitude and aesthetics of form and function in landscape architecture (images) and the relative importance of form and function from ecological and design standpoints (“sliders” below the images). The color photographs illustrate particular designed landscapes arranged in chronological order. The “sliders” underneath them reflect the relative emphasis of form (dark gray) and function (light gray); the bars above and below the sliders reflect the qualitative magnitude (Form: left to right = large to small; Function: right to left = small to large) and apparent range (length of the bar). (A) Abstraction and form influenced a managed forest and waterway converted into a series of pools in a partially constructed natural fountain in the Italian garden at the Villa di Pratolino. Functions, such as direct path connections and flow of water influenced the aesthetically-driven form of the large forested area and waterway. (B) André le Nôtre's French garden, Vaux-le-Vicomte, abstracted nature and carved geometric forms onto the landscape using vegetation, water, and paths. The symmetric landscapes represented power and created a sense of theater; ecological function was irrelevant. (C) The extensive landscaped park, woodlands, and formal gardens of the English Picturesque Blenheim Palace (Oxfordshire, England) included clumps of forest arranged around large sinuous water bodies across hills to create a series of naturalist views. The idea of nature and organic form was crafted through extensive grading and manipulation to imitate nature visually rather than functionally. (D) Frederick Law Olmstead's Central Park in New York imported the concepts from the English garden into an urban area and initiated large park designs across US cities. The park introduced the picturesque and naturalistic style. (E) In the Garden at Villa de Noailles, Gabriel Guevrekian created a highly geometric and artificially-conceived landscape influenced by Cubist painting. The project was, in part, a critical response to the Modernist assertion that form following function should drive design. (F) The Lovejoy Plaza (formerly Ira Keller Fountain) by Lawrence Halprin Associates with Angela Danadjieva is part of the Portland Open Space Sequence. The project introduced a metaphorical mountain cascade that falls through a series of fissured concrete geometric forms to create crags and eddies as part of a waterfall and adjacent riparian zone. The forms have been aestheticized and turned into stepping and seating platforms. The sound of the water and the cascading pattern and microclimates mimic the natural mountain cascade. (G) Martha Schwartz's Minneapolis Courthouse Plaza created a highly orchestrated and form-driven metaphorical landscape of drumlins and cut logs to represent, respectively, the memory of geological forms and the state's history of timbering. (H) Gantry Plaza State Park by Thomas Balsey Associates reclaimed and remediated an industrial waterfront. This project used an organic aesthetic organized around industrial ruins to create both ecosystem services and programmatic values. (I) Cheonggyecheon is a public recreational retrofit of a former highway infrastructure. The 10.9-kilometer-long (6.8 mi) urban renewal project is built where a stream once flowed. Image sources: (A) Villa Pratolino (https://commons.wikimedia.org/wiki/File:Pratolino_utens.jpg; public domain); (B) Esther Westerveld (https://commons.wikimedia.org/wiki/File:Kasteel_van_Vaux-le-Vicomte_-_Maincy_06.jpg; CC-BY-2.0); (C) British Library (https://en.wikipedia.org/wiki/File:A_new_and_accurate_plan_of_Blenheim_Palace_-_L'Art_de_Cr%C3%A9er_les_Jardins_(1835),_pl._1_-_BL.jpg; public domain); (D) Ingfbruno (https://commons.wikimedia.org/wiki/File:3015-Central_Park-Sheep_Meadow.JPG; CC-BY-SA-3.0); (E) SiefkinDR (https://commons.wikimedia.org/wiki/File:Cubist_Garden_Villa_de_Noailles_Hyeres.JPG; CC-BY-SA-3.0); (F) Hagar66 (https://commons.wikimedia.org/wiki/File:Portland-Ira_Keller_Fountain.jpg; CC-BY-SA-3.0); (G) City of Minneapolis (https://msp.world/minneapolis-courthouse-plaza-minneapolis-mn-usa/; public domain); (H) https://www.newyork-architects.com/en/thomas-balsley-associates-new-york/project/gantry-plaza-state-park#image-10; used with permission; (I) madmarv00 (https://commons.wikimedia.org/wiki/File:Korea-Seoul-Cheonggyecheon-01.jpg; CC-BY-2.0).
Since the simultaneous publication of Design with Nature (McHarg, 1969) and the emergence of a broad environmental consciousness, landscape architects increasingly embraced environmental (“ecological”) systems and strategies. In the last 50 years, designers have alternately moved toward and away from this embrace as they have struggled to define their role and determine or prioritize methods for integrating ecology with design (Spirn, 2000; Johnson et al., 2002). This back-and-forth is illustrated by comparing San Jose's Guadalupe Park (1991–2005)19 with the rooftop garden atop New York's Museum of Modern Art (2002–2005)20. Guadalupe Park redesigned an urban river floodplain into a park (form) that provided flood control (ecosystem service). Although many areas of the park are designed to flood, the area of the former floodplain that rarely floods today includes land forms constructed to look like a miniature field of drumlins or a ski slalom. These landforms have little to no ecological function but they aesthetically express a glacial history and the flow of water. In contrast, MOMA's rooftop garden is entirely artificial. This form has no ecological function, but instead reflects engineering constraints (the load the room could bear) and a strong and particular aesthetic: camouflage from surrounding buildings.
In 21st-century practice, the intertwined interrelationships between form and function are deeply embedded in design but form is rarely, if ever, derived directly from function. Although designers seeking certain functions may explore what types of form produce them (“function follows form”), if a particular form is desired or specified by a client, a designer may seek the functions to produce it (“form follows function”). In practice, designers deal with both form and function. For example, landscape urbanism (Waldheim, 2016) and parametric design (Cantrell and Mekies, 2018) claim to explore ecological processes or applications and their relationships to form, representation, and materiality. Both focus on surfaces, materiality, and aesthetics, and provide interacting analysis of these through process diagrams and graphical layouts that ecologists would find challenging to interpret or in which to connect form and function (Figure 1D).
6.4.3. Toward a Common Language
The intentional design of urban food webs links ecological theory with the performative and functional properties of built environments. In urban planning and landscape architecture, form, aesthetics, and desired services (functions) may drive the design, but only a subset of organisms can be cultivated in, or otherwise inhabit, a designed landscape. These “simplified” food webs can provide new research opportunities for ecologists, such as testing hypotheses about assembly and temporal dynamics of food webs and the ecosystem services they provide (sections 6.2.3, 6.2.4). The design process itself requires intentionality in the formulation of a desired reality (i.e., creation of a designed habitat or landscape; Figure 10). Designers working alone may make uninformed assumptions about individual species or ecological processes. Designers and ecologists working together with a common language will improve one another's ability to incorporate complicated challenges and resolve inconsistencies or conflicts among design elements in urban ecosystems (Felson et al., 2013a). Opportunities to intentionally shape urban food webs arise from the recognition that populations of different organisms may respond to different cues and configurations within newly structured conditions in a designed, urbanized environment. If food-web form can be coupled directly to its functionality (e.g., Ulanowicz et al., 2014), food webs will be more appreciated because of their utility to society. If such services can persist costs-effectively over the lifetime of a project, they could prove attractive to the clients who might have to pay an initial premium to incorporate a designed food web into a project. For example, identification of foundation or keystone species within food webs (or sub-webs) that could yield desired services (Baiser et al., 2013) could be a logical and reasonably-priced starting point for designing a food web into a particular project.
6.5. The Importance of Human Intention in Urban Food Webs
People add aesthetic and functional criteria that influence the design of landscapes and will apply similar criteria to designing (for) food webs. Standard design practice introduces vegetation. Although food webs often follow, any that do are essentially unintentional (via the “build it and they will come” approach; section 5.1). In contrast, deliberately designing food webs using species introductions or other bio-manipulations of food webs (e.g., fostering trophic cascades or lengthening food chains), translocations, or other direct interventions with fauna remains mostly untested, especially in urban landscapes. Permitting and regulatory constraints and public concerns about managing or manipulating wildlife remain formidable hurdles. Urban food webs are unlikely to be self-sufficient and will require regular maintenance, including resource inputs and re-introductions, to preserve and sustain their intended forms and functions. To ensure that designed urban food webs meet the demands of clients and public users, they must be monitored and, if contracts and designs permit, managed, augmented, or adapted. By working within design constraints, including ongoing designed experiments, and taking on central roles in managing these systems, ecologists may be able to direct, accelerate, or slow the process of food-web assembly and development.
Human intention also will distinguish designed urban food webs from those embedded in classical ecological restoration projects. The former should provide services (primarily utilitarian, but also aesthetic) for people and urban wildlife, whereas the latter usually are intended to recreate a Prelapsarian “nature” that pre-dates European colonization or does not include humans at all (Jordan and Lubick, 2011). As urban ecological restoration projects become more common (e.g., Ingram, 2008; Clarkson and Kirby, 2016), they increasingly are using different baselines, creating novel ecosystems, and anticipating and grappling with both rapid evolutionary dynamics and human behavior in their design and execution (Alberti, 2015; Lambert and Donihue, 2020). Whereas successful restoration projects ideally become self-maintaining, many require extensive inputs or ongoing maintenance. For designed urban food webs, management and maintenance will be required. Building some capacity for self-reassembly or succession into urban food webs may reduce maintenance, a key design-criterion for successful and sustainable urban landscapes.
Finally, we note that intentionality in the process of designing food webs differs from that of species re-introductions, including re-population of historic ranges and rewilding of sites (Donlan et al., 2005; Caro, 2007; Lorimer et al., 2015; Corlett, 2016; Svenning et al., 2016; Felson and Dugapolski, 2017; Perino et al., 2019). For example, re-introduction of an endangered species requires an RFP, a scope of work, permitting, and site evaluation long before species are re-introduced and monitored for many years thereafter. With urban food webs, design and construction considerations are as crucial as the evaluation and permitting, but the latter are considered rarely, if at all. Given the critical role that people play in supporting, residing in, and learning from our urban habitats and ecological areas, coupling the process of food-web creation with the design process (Felson et al., 2013b) and exploring form and function of urban food webs should be seen as opportunities to study and achieve greater ecological reconciliation and resilience (Felson, 2013).
7. Conclusion
As landscape architects are expanding their role as designers and builders at site-to-city-to-regional scales, they are looking for research and analysis to guide the form, function, sustainability, and resiliency of their projects. Even though “biodiversity planning” may be part of the design process, such planning rarely extends beyond different kinds of plants or their pollinators; complete, or at least stable food webs rarely are considered explicitly. Designers increasingly are seeking to develop ecological habitats that embrace ecological theory and foster biological richness and abundance. The opportunity exists for ecologists to work together with designers to move the design process to the next level: designing environments for supporting food webs and designing food webs directly.
To move forward, ecologists and designers will need to reconsider form and function and how these might play out in an urban context in relation to constructed habitats that foster food webs. The predominant focus on function for ecologists contrasts with the varied philosophical interpretations of principles of aesthetics and a more fluid relationship between form and function that continues to emerge in design through technological advances, adaptations of existing concepts, and development of new theoretical frameworks. Even though these disparate stances may make it difficult for ecologists and designers to find common ground, their dual approaches must come together in the intentional design of urban food webs.
Rather than simply providing ecological theory for designers to interpret and translate into practice, ecologists should participate directly in the design process (Felson et al., 2013b) and co-develop strategies based on emerging ecological theory and ongoing research to create novel food webs and relevant design strategies that work for cities (Felson et al., 2013a). These collaborations will help designers expand their own range of aesthetic and ecologically functional spaces. Establishing habitat designs and associated food webs that are responsive to stakeholders will help to inform the intentional design of urban food webs that make ecological processes visible, lead to the development of new types of green spaces, contribute to new aesthetics for parks and recreation, and permit urban land uses that may be more valuable than a particular restored ecosystem or one recreated for one or a few specific ecosystem services provided by food webs (Felson and Pickett, 2005; Gobster et al., 2007; Aronson et al., 2017). Finally, collaborations that lead to successful design, construction, and installation of urban food webs will of necessity expand beyond ecologists and landscape architects to include urban planners, civil engineers, transportation planners, and municipal authorities, among many others. The case study of Jamaica Bay (section 4) and new directions in designed landscapes (section 5) lay out the possibility of a future in which ecologists are integral participants in the design and construction of urban landscapes where ecological research is a central part of the long-term and ongoing management of urban food webs and the landscapes they help create and support.
Ethics Statement
Written informed consent was obtained from the minor's legal guardian for the publication of the potentially identifiable image in Figure 2 of this article.
Author Contributions
AF and AE contributed equally to the conceptualization, writing, and editing of the manuscript. Both authors contributed to the article and approved the submitted version.
Funding
AE's initial work on these ideas was supported by an Edward P. Bass Distinguished Visiting Environmental Fellowship from Yale University's Institute for Biospheric Studies. AF's work on this project was supported in part through funding from the Related Company for the Tuxedo Reserve research project, developed as a master-plan project with EDAW and later AECOM, and from Rutgers University.
Conflict of Interest
The authors declare that the research was conducted in the absence of any commercial or financial relationships that could be construed as a potential conflict of interest.
Acknowledgments
We thank the Ecological Society of America, the Yale School of the Environment, the University of California at Davis, AECOM, the Water Forum, the Sacramento Area Flood Control Agency, the American River Parkway Foundation, and the Sacramento County Parks for their contributions to the Earth Stewardship Initiative, focusing on the American River Parkway. Paul Craze, several reviewers, and our colleagues at Yale and the Harvard Forest provided useful feedback on an early version of this manuscript. This manuscript has been released as a pre-print at PeerJ Preprints (Felson and Ellison, 2017).
Footnotes
1. ^Terms defined in the Glossary are set in bold-face type at first mention.
2. ^A search run in Web of Science on 22 November 2020 retrieved 31,243 papers published between 1900 and 2020 on “food web” or “food webs” but only seven published on “urban food web” or “urban food webs.”
3. ^https://www.census.gov/programs-surveys/geography/guidance/geo-areas/urban-rural.html.
4. ^The nexus of this continuing work is The Ian L. McHarg Center for Urbanism and Ecology in the Stuart Weitzman School of Design at the University of Pennsylvania: https://mcharg.upenn.edu/.
5. ^The description framing the competition conveyed this critical intersection of human and natural concerns: “Gateway presents a significant regional resource with incredible infrastructural, ecological and cultural value in the New York metropolitan region, hosting endangered birds, fish and shellfish breeding grounds, marinas, playfields, and cultural relics. It is also the site of combined sewer outfalls, treated wastewater effluent, abandoned buildings, degraded habitat, drowned marshes, former landfills and vast asphalt runways.” See https://www.vanalen.org/projects/envisioning-gateway/#resources.
6. ^https://www.vanalen.org/projects/envisioning-gateway/#sitebrief.
7. ^http://www.rebuildbydesign.org/our-work/sandy-projects.
8. ^The low likelihood of winning designs in design competitions actually being built may dissuade ecologists from working with designers on them. But in the design community, winning a design competition is a lot like an ecologist getting a paper accepted and published in Nature or Science!
9. ^https://sites.rutgers.edu/urbionet/.
10. ^https://www.urban-wildlife.org/.
11. ^https://landscapeaustralia.com/articles/interview-dirt-studios-julie -bargmann-1/.
12. ^https://www.wildflower.org/.
13. ^https://forestparkconservancy.org/forest-park/.
14. ^https://dirt.asla.org/2012/08/22/another-winner-the-london-olympics-landscape/.
15. ^https://www.gowanusbydesign.org.
16. ^https://www.asla.org/2017awards/320768.html.
17. ^https://www.architecturalrecord.com/articles/14187-pier-35-by-shop-architects-and-ken-smith-workshop.
18. ^https://www.icmbio.gov.br/parnatijuca/.
References
Adams, L. W. (2005). Urban wildlife ecology and conservation: a brief history of the discipline. Urban Ecosyst. 8, 139–156. doi: 10.1007/s11252-005-4377-7
Adler, F. R., and Tanner, C. J. (2013). Urban Ecosystems: Ecological Principles for the Built Environment. Cambridge: Cambridge University Press.
Alberti, M. (2015). Eco-evolutionary dynamics in an urbanizing planet. Trends Ecol. Evol. 30, 114–126. doi: 10.1016/j.tree.2014.11.007
Alberti, M., Marzluff, J. M., Shulenberger, E., Bradley, G., Ryan, C., and ZumBrunnen, C. (2009). Integrating humans into ecology: opportunities and challenges for studying urban ecosystems. BioScience 53, 1169–1179. doi: 10.1641/0006-3568(2003)053[1169:IHIEOA]2.0.CO;2
Amidon, J., (Ed.). (2006). Ken Smith Landscape Architects/Urban Projects. New York, NY: Princeton Architectural Press.
Andrade, R., Franklin, J., Larson, K. L., Swan, C. M., Lerman, S. B., Bateman, H. L., et al. (2020). Predicting the assembly of novel communities in urban ecosystems. Landsc. Ecol. 36, 1–15. doi: 10.1007/s10980-020-01142-1
Archer-Lean, C., Wardell-Johnson, A., Conroy, G., and Carter, J. (2015). Representations of the dingo: contextualising iconicity. Australas. J. Environ. Manage. 22, 181–196. doi: 10.1080/14486563.2014.985268
Aronson, M. F. J., Lepczyk, C. A., Evans, K. L., Goddard, M. A., Lerman, S. B., MacIvor, J. S., et al. (2017). Biodiversity in the city: key challenges for urban green space management. Front. Ecol. Environ. 15, 189–196. doi: 10.1002/fee.1480
Aslan, C. E., Aslan, A., Croll, D., Tershy, B., and Zavaleta, E. (2014). Building taxon substitution guidelines on a biological control foundation. Restor. Ecol. 22, 437–441. doi: 10.1111/rec.12096
Baiser, B., Whitaker, N., and Ellison, A. M. (2013). Modeling foundation species in food webs. Ecosphere 4:art146. doi: 10.1890/ES13-00265.1
Bencin, H., Kioko, J., and Kiffner, C. (2016). Local people's perceptions of wildlife species in two distinct landscapes of northern Tanzania. J. Nat. Conserv. 34, 82–92. doi: 10.1016/j.jnc.2016.09.004
Beninde, J., Veith, M., and Hochkirch, A. (2015). Biodiversity in cities needs space: a meta–analysis of factors determining intra–urban biodiversity variation. Ecol. Lett. 18, 581–592. doi: 10.1111/ele.12427
Bornkamm, R. (2007). Spontaneous development of urban woody vegetation on differing soils. Flora 202, 695–704. doi: 10.1016/j.flora.2007.05.004
Borrett, S. R. (2014). Systems ecology: a network perspective and retrospective. Ecol. Modell. 293, 1–220. doi: 10.1016/j.ecolmodel.2014.10.005
Brace, J. (1977). Teaching Conservation in Developing Nations. Washington, DC: US Government Printing Office.
Braje, T. J., Kennett, D. J., Erlandson, J. M., and Culleton, B. J. (2007). Human impacts on nearshore shellfish taxa: a 7,000 year record from Santa Rosa Island, California. Am. Antiq. 72, 735–756. doi: 10.2307/25470443
Brash, A., Handel, J., and Orff, K., (Eds.). (2011). Gateway: Visions for an Urban National Park. New York, NY: Princeton Architectural Press.
Byrne, L. B., and Grewal, P. (2008). Introduction to ecological landscaping: a holistic description and framework to guide the study and management of urban landscape parcels. Cities Environ. 1:article3. doi: 10.15365/cate.1232008
Calkins, M. (2012). The Sustainable Sites Handbook: A Complete Guide. Hoboken, NJ: John Wiley & Sons.
Cantrell, B., and Mekies, A. (2018). Codify: Parametric and Computational Design in Landscape Architecture. Abingdon: Routledge.
Caro, T. (2007). The Pleistocene re-wilding gambit. Trends Ecol. Evol. 22, 281–283. doi: 10.1016/j.tree.2007.03.001
Chacón, D. J. (2013). Prairie Dog Costs Evoke Mixed Reaction. Available online at: https://www.santafenewmexican.com/news/local_news/prairie-dog-costs-evoke-mixed-reaction/article_a78a4aa4–5045-5ed2–9def-e40cbde17db3.html
Chalcraft, D. R., and Resetarits, W. J. (2003). Predator identity and ecological impacts: functional redundancy or functional diversity? Ecology 84, 2407–2418. doi: 10.1890/02-0550
Childers, D. L., Cadenasso, M. L., Grove, J. M., Marshall, V., McGrath, B., and Pickett, S. T. A. (2015). An ecology for cities: a transformational nexus of design and ecology to advance climate change resilience and urban sustainability. Sustainability 7, 3774–3791. doi: 10.3390/su7043774
Christie, F. J., Cassis, G., and Hochuli, D. F. (2010). Urbanization affects the trophic structure of arboreal communities. Urban Ecosyst. 13, 169–180. doi: 10.1007/s11252-009-0115-x
City of Calgary (2011). Bird-Friendly Urban Design Guidelines: Integrating Natural Systems With Human Activities. Available online at: http://www.animalarchitecture.org/wp-content/uploads/2013/11/CalgaryBirdingGuidelines.pdf
Clarkson, B. D., and Kirby, C. L. (2016). Ecological restoration in urban environments in New Zealand. Ecol. Manage. Restor. 17, 180–190. doi: 10.1111/emr.12229
Coombes, M. A., La Marca, E. C., Naylor, L. A., and Thompson, R. C. (2015). Getting into the groove: opportunities to enhance the ecological value of hard coastal infrastructure using fine-scale surface textures. Ecol. Eng. 77:314–323. doi: 10.1016/j.ecoleng.2015.01.032
Cordell, J. R., Toft, J. D., Munsch, S. H., and Goff, M. (2017). “Benches, beaches, and bumps: how habitat monitoring and experimental science can inform urban seawall design,” in Living Shorelines: The Science and Management of Nature-Based Coastal Protection eds C. M. Bikovic, M. M. Mitchell, M. K. La Peyre, and J. D. Toft (Boca Raton, FL: CRC Press), 421–438. doi: 10.1201/9781315151465-25
Corlett, R. T. (2016). Restoration, reintroduction, and rewilding in a changing world. Trends Ecol. Evol. 31, 453–462. doi: 10.1016/j.tree.2016.02.017
Czerniak, J., and Hargreaves, G., (Eds.). (2007). Large Parks. Princeton, NJ: Princeton Architectural Press.
de Boer, W. F., and Prins, H. H. T. (2002). Human exploitation and benthic community structure on a tropical intertidal flat. J. Sea Res. 48, 225–240. doi: 10.1016/S1385-1101(02)00160-0
de Ruiter, P. C., Wolters, V., and Moore, J. C. (2005). Dynamic Food Webs: Multispecies Assemblages, Ecosystem Development and Environmental Change. Burlingon, MA: Academic Press.
Del Tredici, P. (2007). “The role of horticulture in a changing world,” in Botanical Progress, Horticultural Innovations and Cultural Changes, ed M. Conan and W. J. Kress (Washington, DC: Dumbarton Oaks Research Library and Collection), 265–272.
Del Tredici, P. (2010). Spontaneous urban vegetation: reflections of change in a globalized world. Nat. Cult. 5, 299–315. doi: 10.3167/nc.2010.050305
Deming, E. M., and Swaffield, S. (2011). Landscape Architecture Research, Inquiry, Strategy, Design. Hoboken, NJ: John Wiley & Sons, Inc.
Dobson, A. P. (2014). Yellowstone wolves and the forces that structure natural systems. PLoS Biol. 12:e1002025. doi: 10.1371/journal.pbio.1002025
Donlan, J., Greene, H. W., Berger, J., Bock, C., Bock, J. H., Burney, D. A., et al. (2005). Re-wilding North America. Nature 436, 913–914. doi: 10.1038/436913a
Drake, J. A. (1990a). Communities as assembled structures: do rules govern patterns? Trends Ecol. Evol. 5, 159–164. doi: 10.1016/0169-5347(90)90223-Z
Drake, J. A. (1990b). The mechanics of community assembly and succession. J. Theor. Biol. 147, 213–233. doi: 10.1016/S0022-5193(05)80053-0
Drake, J. A. (1991). Community-assembly mechanics and the structure of an experimental species ensemble. Am. Nat. 137, 1–26. doi: 10.1086/285143
Egerer, M., and Kowarik, I. (2020). Confronting the modern Gordian knot of urban beekeeping. Trends Ecol. Evol. 35, 956–959. doi: 10.1016/j.tree.2020.07.012
Ellison, A. M. (2019). Foundation species, non-trophic interactions, and the value of being common. iScience 13, 254–268. doi: 10.1016/j.isci.2019.02.020
Ellison, A. M., and Buckley Borden, D. (2021). Constructive friction creates a third space for art/science collaborations. SocArXiv. doi: 10.31235/osf.io/ms3gq
Ellison, A. M., Felson, A. J., and Friess, D. A. (2020). Mangrove rehabilitation and restoration as experimental adaptive management. Front. Mar. Sci. 7:327. doi: 10.3389/fmars.2020.00327
Ellison, A. M., and Gotelli, N. J. (2021). Scaling in Ecology With a Model System, Volume 64 of Monographs in Population Biology. Princeton, NJ: Princeton University Press.
Eshete, G., Sillero-Zubiri, C., Cieraad, E., Musters, C. J. M., De Snoo, G. R., De Iongh, H. H., et al. (2018). Does livestock predation reflect in negative local perceptions of Ethiopian wolves in SouthWollo? Trop. Ecol. 59, 11–19. Available online at: http://www.tropecol.com/pdf/open/PDF_59_1/2/2%20Eshete%20et%20al.pdf
Essington, T. E., Beaudreau, A. H., and Wiedenmann, J. (2006). Fishing through marine food webs. Proc. Natl. Acad. Sci. U.S.A. 103, 3171–3175. doi: 10.1073/pnas.0510964103
Evans, E. W. (2016). Biodiversity, ecosystem functioning, and classical biological control. Appl. Entomol. Zool. 51, 173–184. doi: 10.1007/s13355-016-0401-z
Faeth, S. H., Bang, C., and Saari, S. (2011). Urban biodiversity: patterns and mechanisms. Ann. N. Y. Acad. Sci. 1223, 69–81. doi: 10.1111/j.1749-6632.2010.05925.x
Faeth, S. H., Warren, P. S., Shochat, E., and Marussich, W. A. (2005). Trophic dynamics in urban communities. BioScience 55, 399–407. doi: 10.1641/0006-3568(2005)055[0399:TDIUC]2.0.CO;2
Felson, A. J. (2013). “The role of designers in creating wildlife habitat in the built environment,” in Designing Wildlife Habitat, ed J. Beardsley (Cambridge, MA: Harvard University Press), 215–240.
Felson, A. J., Bradford, M. A., and Terway, T. M. (2013a). Promoting Earth Stewardship through urban design experiments. Front. Ecol. Environ. 11, 362–367. doi: 10.1890/130061
Felson, A. J., and Dugapolski, J. (2017). “Re-wilding the suburbs. Housing taxonomy,” in XXL-XS: New Directions in Ecological Design, eds M. Joachim and M. Silver (New York, NY: Actar Publishers), 195–197.
Felson, A. J., and Ellison, A. M. (2017). Designer food webs: from build-it-and-they-will-come to intentionality-in-design. PeerJ 5:e3375v1. doi: 10.7287/peerj.preprints.3375
Felson, A. J., Pavao-Zuckerman, M., Carter, T., Montalto, F., Shuster, B., Springer, N., et al. (2013b). Mapping the design process for urban ecology researchers. BioScience 63, 854–865. doi: 10.1525/bio.2013.63.11.4
Felson, A. J., and Pickett, S. T. A. (2005). Designed experiments: new approaches to understanding urban ecosystems. Front. Ecol. Environ. 3, 549–556. doi: 10.1890/1540-9295(2005)003[0549:DENATS]2.0.CO;2
Fernandez, F. A. S., Rheingantz, M. L., Genes, L., Kenup, C. F., Galliez, M., Cezimbra, T., et al. (2017). Rewilding the Atlantic Forest: restoring the fauna and ecological interactions of a protected area. Perspect. Ecol. Conserv. 15, 308–314. doi: 10.1016/j.pecon.2017.09.004
Forman, R. T. T. (2002). “The missing catalyst: design and planning with ecology roots,” in Ecology and Design: Frameworks for Learning, eds B. R. Johnson and K. Hill (Washington, DC: Island Press), 85–109.
Forman, R. T. T. (2016). Urban ecology principles: are urban ecology and natural area ecology really different? Landsc. Ecol. 31, 1653–1662. doi: 10.1007/s10980-016-0424-4
Foster, D. R., Donahue, B. M., Kittredge, D. B., Lambert, K. F., Hunter, M. L., Hall, B. R., et al. (2010). Wildlands and Woodlands: A Vision for the New England Landscape. Petersham, MA: Harvard Forest.
Francis, R. A., and Lorimer, J. (2011). Urban reconciliation ecology: the potential of living roofs and walls. J. Environ. Manage. 92, 1429–1437. doi: 10.1016/j.jenvman.2011.01.012
Fraser, L. H., Harrower, W. L., Garris, H. W., Davidson, S., Hebert, P. D. N., Howie, R., et al. (2015). A call for applying trophic structure in ecological restoration. Restor. Ecol. 23, 503–507. doi: 10.1111/rec.12225
Gallagher, F., Goodey, N. M., Hagmann, D., Singh, J. P., Holzapfel, C., Litwhiler, M., et al. (2018). Urban re-greening: a case study in multi-trophic biodiversity and ecosystem functioning in a post-industrial landscape. Diversity 10:119. doi: 10.3390/d10040119
Gateway National Recreation Area (2007). An Update on the Disappearing Salt Marshes of Jamaica Bay, New York. Available online at: https://www.nytimes.com/packages/pdf/nyregion/city_room/20070802_FinalJamaicaBayReport.pdf
Girot, C. (2016). The Course of Landscape Architecture: A History of Our Designs on the Natural World. London: Thames & Hudson.
Gobster, P. H., Nassauer, J. I., Daniel, T. C., and Fry, G. (2007). The shared landscape: what does aesthetics have to do with ecology? Landsc. Ecol. 22, 959–972. doi: 10.1007/s10980-007-9110-x
Goddard, M. A., Dougill, A. J., and Benton, T. G. (2010). Scaling up from gardens: biodiversity conservation in urban environments. Trends Ecol. Evol. 25, 90–98. doi: 10.1016/j.tree.2009.07.016
Gould, S. J., and Lewontin, R. C. (1979). The spandrels of San Marco and the Panglossian paradigm: a critique of the adaptationist programme. Proc. R. Soc. B: Biol. Sci. 205, 581–598. doi: 10.1098/rspb.1979.0086
Graham, N. A. J., McClanahan, T. R., MacNeil, M. A., Wilson, S. K., Cinner, J. E., Huchery, C., et al. (2017). Human disruption of coral reef trophic structure. Curr. Biol. 27, 231–236. doi: 10.1016/j.cub.2016.10.062
Gray, C., Hildrew, A. G., Lu, X., Ma, A., McElroy, D., Monteith, D., et al. (2016). Recovery and nonrecovery of freshwater food webs from the effects of acidification. Adv. Ecol. Res. 55, 475–534. doi: 10.1016/bs.aecr.2016.08.009
Grewal, S. S., Cheng, Z., Masih, S., Woboldt, M., Huda, N., Knight, A., et al. (2011). An assessment of soil nematode food webs and nutrient pools in community gardens and vacant lots in two post-industrial American cities. Urban Ecosyst. 14, 181–194. doi: 10.1007/s11252-010-0146-3
Groffman, P. M., Cadenasso, M. L., Cavender-Bares, J., Childers, D. L., Grimm, N. B., Grove, J. M., et al. (2017). Moving towards a new urban systems science. Ecosystems 20, 38–43. doi: 10.1007/s10021-016-0053-4
Groffman, P. M., Cavender-Bares, J., Bettez, N. D., Grove, J. M., Hall, S. J., Heffernan, J. B., et al. (2014). Ecological homogenization of urban USA. Front. Ecol. Environ. 12, 74–81. doi: 10.1890/120374
Grose, M., (Ed.). (2017). Constructed Ecologies–Critical Reflections on Ecology With Design. London: Routledge.
Hagen, M., Kissling, W. D., Rasmussen, C., Aguiar, M. A. D., Brown, L. E., Carstensen, D. W., et al. (2012). Biodiversity, species interactions and ecological networks in a fragmented world. Adv. Ecol. Res. 46, 89–102. doi: 10.1016/B978-0-12-396992-7.00002-2
Hamer, A. J., and McDonnell, M. J. (2008). Amphibian ecology and conservation in the urbanising world: a review. Biol. Conserv. 141, 2432–2449. doi: 10.1016/j.biocon.2008.07.020
Hassall, C. (2014). The ecology and biodiversity of urban ponds. Wiley Interdiscpl. Rev. Water 1, 187–206. doi: 10.1002/wat2.1014
Hitchmough, J. (2008). New approaches to ecologically based, designed urban plant communities in Britain: do these have any relevance in the USA? Cities Environ. 1: Article 10. doi: 10.15365/cate.12102008
Hobbs, R. J., Higgs, E., Hall, C. M., Bridgewater, P. F. S. III C, Ellis, E. C., et al. (2014). Managing the whole landscape: historical, hybrid, and novel ecosystems. Front. Ecol. Environ. 12, 557–564. doi: 10.1890/130300
Hobbs, R. J., Higgs, E., and Harris, J. A. (2009). Novel ecosystems: implications for conservation and restoration. Trends Ecol. Evol. 24, 599–605. doi: 10.1016/j.tree.2009.05.012
Holloway, M. (2018). By Design: An Architectural Awakening Could Save Billions of Birds. Available online at: https://www.audubon.org/magazine/fall-2018/by-design-architectural-awakening-could-save
Hostetler, M., Allen, W., and Meurk, C. (2011). Conserving urban biodiversity? Creating green infrastructure is only the first step. Landsc. Urban Plan. 100, 369–371. doi: 10.1016/j.landurbplan.2011.01.011
Hoyle, H., Jorgensen, A., Warren, P., Dunnett, N., and Evans, K. (2017). “Not in their front yard”: the opportunities and challenges of introducing perennial urban meadows: a local authority stakeholder perspective. Urban For. Urban Green. 25, 139–149. doi: 10.1016/j.ufug.2017.05.009
Ingram, M. (2008). Urban ecological restoration. Restor. Ecol. 26, 175–177. doi: 10.3368/er.26.3.175
IUCN/SSC (2013). Guidelines for Reintroductions and Other Conservation Translocations. Gland: IUCN Species Survival Commission.
Jabareen, Y. (2013). Planning the resilient city: concepts and strategies for coping with climate change and environmental risk. Cities 31, 220–229. doi: 10.1016/j.cities.2012.05.004
Johnson, B. R., Silbernagel, J., Hostetler, M., Mills, A., Ndubisi, F., Fife, E., et al. (2002). “The nature of dialogue and the dialogue of nature: designers and ecologists in collaboration,” in Ecology and Design: Frameworks for Learning, eds B. R. Johnson and K. Hill (Washington DC: Island Press), 305–355.
Jones, C. G., Lawton, J. H., and Shachak, M. (1994). Organisms as ecosystem engineers. Oikos 69, 373–386. doi: 10.2307/3545850
Jordan, W., and Lubick, G. M. (2011). Making Nature Whole: A History of Ecological Restoration. Washington, DC: Island Press.
Kareiva, P., Marvier, M., and Silliman, B., (Eds.). (2018). Effective Conservation Science: Data Not Dogma. Oxford: Oxford University Press.
Kierulff, M. C. M., Ruiz-Miranda, C., Prócopio de Oliveira, P., Beck, B. B., Martins, A., Dietz, J. M., et al. (2012). The golden lion tamarin (Leontopithecus rosalia): a conservation success history. Int. Zoo Yearb. 46, 36–45. doi: 10.1111/j.1748-1090.2012.00170.x
Kirchoff, K., Stokes, L., Cuevas, A. B., Chan, L., Filipovic, M., Goodrich, G., et al. (2009). Gateway National Recreation Area Long-Term Resource Management Under a Changing Climate. New York, NY: Columbia University, School of International & Public Affairs.
Kowarik, I. (2011). Novel urban ecosystems, biodiversity, and conservation. Environ. Pollut. 159, 1974–1983. doi: 10.1016/j.envpol.2011.02.022
Lambert, M., and Donihue, C. (2020). Urban biodiversity management using evolutionary tools. Nat. Ecol. Evol. 4, 903–910. doi: 10.1038/s41559-020-1193-7
Lau, M. K., Borrett, S. R., Baiser, B., Gotelli, N. J., and Ellison, A. M. (2017). Ecological network metrics: opportunities for synthesis. Ecosphere 8:e01900. doi: 10.1002/ecs2.1900
Lister, N.-M. (2007). “Sustainable large parks: ecological design or designer ecology?” in Large Parks, eds J. Czerniak and G. Hargreaves (Princeton, NJ: Princeton Architectural Press), 31–51.
Lorimer, J., Sandom, C., Jepson, P., Doughty, C., Barua, M., and Kirby, K. J. (2015). Rewilding: science, practice, and politics. Annu. Rev. Environ. Resour. 40, 39–62. doi: 10.1146/annurev-environ-102014-021406
Lundholm, J. T., and Richardson, P. J. (2010). Habitat analogues for reconciliation ecology in urban and industrial environments. J. Appl. Ecol. 47, 966–975. doi: 10.1111/j.1365-2664.2010.01857.x
MacArthur, R. H., and Wilson, E. O. (1967). The Theory of Island Biogeography. Princeton, NJ: Princeton University Press.
Marzluff, J. M., and Ewing, K. (2008). “Restoration of fragmented landscapes for the conservation of birds: a general framework and specific recommendations for urbanizing landscapes,” in Urban Ecology: An International Perspective on the Interaction Between Humans and Nature, eds J. M. Marzluff, E. Shulenberger, W. Endlicher, M. Alberti, G. Bradley, C. Ryan, et al., (New York, NY: Springer Science+Business Media, LLC), 739–756. doi: 10.1007/978-0-387-73412-5_48
Mata, L., Garrard, G., Fidler, F., Ives, C., Maller, M., Wilson, J., et al. (2019). Punching above their weight: the ecological and social benefits of pop-up parks. Front. Ecol. Environ. 17, 341–347. doi: 10.1002/fee.2060
McKinney, M. L. (2006). Urbanization as a major cause of biotic homogenization. Biol. Conserv. 127, 247–260. doi: 10.1016/j.biocon.2005.09.005
Millennium Ecosystem Assessment (2005). Ecosystems and Human Well-being: Synthesis. Washington, DC: Island Press.
Miranda, E. B. P., Ribeiro, R. P. J., and Strüssmann, C. (2016). The ecology of human-anaconda conflict: a study using internet videos. Trop. Conserv. Sci. 9, 43–77. doi: 10.1177/194008291600900105
Moreno, C. A. (2001). Community patterns generated by human harvesting on chilean shores: a review. Aquat. Conserv. Mar. Freshw. Ecosyst. 11, 19–30. doi: 10.1002/aqc.430
Morris, R. L., Porter, A. G., Figueira, W. F., Coleman, R. A., Fobert, E. K., and Ferrari, R. (2018). Fish-smart seawalls: a decision tool for adaptive management of marine infrastructure. Front. Ecol. Environ. 16, 278–287. doi: 10.1002/fee.1809
Mosbach, C. (2013). Catherine Mosbach's “Submersive” Experiments in Landscape Architecture. Available online at: https://www.foreground.com.au/culture/the-delightful-dialogues-of-catherine-mosbachs-landscape-architecture/
Mozingo, L. A. (1998). The aesthetics of ecological design: seeing science as culture. Landsc. J. 16, 46–59. doi: 10.3368/lj.16.1.46
Musacchio, L. R. (2009). “Pattern: process metaphors for metropolitan landscapes,” in Ecology of Cities and Towns: A Comparative Approach, eds M. J. McDonnell, A. K. Hahs, and J. H. Breuste (Dordrecht: Springer), 484–502. doi: 10.1017/CBO9780511609763.029
Musacchio, L. R. (2011). The grand challenge to operationalize landscape sustainability and the design-in-science paradigm. Landsc. Ecol. 26, 1–5. doi: 10.1007/s10980-010-9562-2
Nassauer, J. I. (1995). Messy ecosystems, orderly frames. Landsc. J. 14, 161–170. doi: 10.3368/lj.14.2.161
Nassauer, J. I. (2012). Landscape as medium and method for synthesis in urban ecological design. Landsc. Urban Plan. 106, 221–229. doi: 10.1016/j.landurbplan.2012.03.014
National Parks Conservation Association (2007). Gateway National Recreation Area: A Resource Assessment. Fort Collins, C: National Parks Conservation Service, Center for State of the Parks.
Niles, L. J., Bart, J., Sitters, H. P., Dey, A. D., Clark, K. E., Atkinson, P. W., et al. (2009). Effects of horseshoe crab harvest in Delaware Bay on Red Knots: are harvest restrictions working? BioScience 59, 153–164. doi: 10.1525/bio.2009.59.2.8
Oliveira-Santos, L. G. R., and Fernandez, F. A. D. S. (2010). Pleistocene rewilding, Frankenstein ecosystems, and an alternative conservation agenda. Conserv. Biol. 24, 4–5. doi: 10.1111/j.1523-1739.2009.01379.x
Orff, K. (2010). “Oyster-tecture,” in Rising Currents: Projects for New York Waterfronts, ed B. Bergdoll (New York, NY: Museum of Modern Art), 90–99.
Paine, R. T. (1966). Food web complexity and species diversity. Am. Nat. 100, 65–75. doi: 10.1086/282400
Palmer, M. A. (2009). Reforming watershed restoration: science in need of application and applications in need of science. Estuar. Coasts 32, 1–17. doi: 10.1007/s12237-008-9129-5
Palmer, M. A., Ambrose, R. F., and Poff, N. L. (1997). Ecological theory and community restoration ecology. Restor. Ecol. 5, 291–300. doi: 10.1046/j.1526-100X.1997.00543.x
Palmer, M. A., Menninger, H. L., and Bernhardt, E. (2010). River restoration, habitat heterogeneity and biodiversity: a failure of theory or practice? Freshw. Biol. 55, 205–222. doi: 10.1111/j.1365-2427.2009.02372.x
Palta, M. M., Grimm, N. B., and Groffman, P. M. (2017). “Accidental” urban wetlands: ecosystem functions in unexpected places. Front. Ecol. Environ. 15, 248–256. doi: 10.1002/fee.1494
Parris, K. M., Amati, M., Bekessy, S. A., Dagenais, D., Fryd, O., Hahs, A. K., et al. (2018). The seven lamps of planning for biodiversity in the city. Cities 83, 44–53. doi: 10.1016/j.cities.2018.06.007
Pauly, D., Christensen, V., Froese, R., and Palomares, M. L. (2000). Fishing down aquatic food webs. Am. Sci. 88, 46–51. doi: 10.1511/2000.1.46
Pećarević, M., Danoff-Burg, J., and Dunn, R. R. (2010). Biodiversity on Broadway: enigmatic diversity of the societies of ants (Formicidae) on the streets of New York City. PLoS ONE 5:e13222. doi: 10.1371/journal.pone.0013222
Perino, A., Pereira, H. M., Navarro, L. M., Fernández, N., Bullock, J. M., Ceausu, S., et al. (2019). Rewilding complex ecosystems. Science 364:eaav5570. doi: 10.1126/science.aav5570
Petchey, O. L., and Gaston, K. J. (2006). Functional diversity: back to basics and looking forward. Ecol. Lett. 9, 741–758. doi: 10.1111/j.1461-0248.2006.00924.x
Pickett, S. T. A., Cadenasso, M. L., Childers, D. L., Mcdonnell, M. J., and Zhou, W. (2016). Evolution and future of urban ecological science: ecology in, of, and for the city. Ecosyst. Health Sustain. 2:e01229. doi: 10.1002/ehs2.1229
Pickett, S. T. A., Cadenasso, M. L., Grove, J. M., Boone, C. G., Irwin, E., Groffman, P. M., et al. (2011). Urban ecological systems: foundations and a decade of progress. J. Environ. Manage. 92, 331–362. doi: 10.1016/j.jenvman.2010.08.022
Pickett, S. T. A., Grove, M., LaDeau, S. L., Rosi, E. J., and Cadenasso, M. L. (2020). “Urban ecology as an integrative science and practice,” in Urban Ecology: Its Nature And Challenges, ed P. Barbosa (Wallingford: CABI Publishing), 122–143. doi: 10.1079/9781789242607.0122
Pilosof, S. M.A P M P, and Kéfi, S. (2017). The multilayer nature of ecological networks. Nat. Ecol. Evol. 1:0101. doi: 10.1038/s41559-017-0101
Pulliam, H. R. (1988). Sources, sinks, and population regulation. Am. Nat. 132, 652–661. doi: 10.1086/284880
Pulliam, H. R., and Johnson, B. R. (2002). “Ecology's new paradigm: what does it offer designers and planners?” in Ecology and Design: Frameworks for Learning, eds B. R. Johnson and K. Hill (Washington, DC: Island Press), 51–84.
Rafferty, P., and Babson, A. (2015). “Restoring the Jamaica Bay Wetlands, Gateway National Recreation Area, New York: case study on a project of the Gateway National Recreation Area,” in Coastal Adaptation Strategies: Case Studies (Fort Collins, CO: US Department of the Interior, National Park Service), 27–28.
Rafferty, P., Castagna, J., and Adamo, D. (2011). Restoration journal: building partnerships to restore an urban marsh ecosystem at gateway national recreation area. Park Sci. 27, 34–41. Available online at: https://irma.nps.gov/DataStore/DownloadFile/616075
Rebele, F. (1994). Urban ecology and special features of urban ecosystems. Glob. Ecol. Biogeogr. 4, 173–187. doi: 10.2307/2997649
Reed, C., and Lister, N. M., (Eds.). (2014). Projective Ecologies. New York, NY: Actar/Hravard Graduate School of Design.
Reitz, E. J. (2004). “Fishing down the food web”: a case study from St. Augustine, Florida, USA. Am. Antiq. 69, 63–83. doi: 10.2307/4128348
Riley, C. B., Perry, K. I., Ard, K., and Gardiner, M. M. (2018). Asset or liability? Ecological and sociological tradeoffs of urban spontaneous vegetation on vacant land in shrinking cities. Sustainability 10:2139. doi: 10.3390/su10072139
Rosenzweig, M. L. (2003). Win-Win Ecology: How the Earth's Species Can Survive in the Midst of Human Enterprise. New York, NY: Oxford University Press.
Ross, M. R. V., Bernhardt, E. S., Doyle, M. W., and Heffernan, J. B. (2015). Designer ecosystems: incorporating design approaches into applied ecology. Annu. Rev. Environ. Resour. 40, 419–443. doi: 10.1146/annurev-environ-121012-100957
Roudavski, S. (2020). “Multispecies cohabitation and future design,” in Proceedings of Design Research Society (DRS) 2020 International Conference: Synergy, eds S. Boess, M. Cheung, and R. Cain (London: Design Research Society), 731–750. doi: 10.21606/drs.2020.402
Sawyer, A. C., Toft, J. D., and Cordell, J. R. (2020). Seawall as salmon habitat: eco-engineering improves the distribution and foraging of juvenile Pacific salmon. Ecol. Eng. 151:105856. doi: 10.1016/j.ecoleng.2020.105856
SCAPE/Landscape Architecture [Kate Orff] (2015). The Shallows: Volume I. Bay Landscapes as Ecological Infrastructure. New York: SCAPE.
Shochat, E., Stefanov, W. L., Whitehouse, M. E. A., and Faeth, S. H. (2008). “Urbanization and spider diversity: influences of human modification of habitat structure and productivity,” in Urban Ecology: An International Perspective on the Interaction Between Humans and Nature, eds J. M. Marzluff, E. Shulenberger, W. Endlicher, M. Alberti, G. Bradley, C. Ryan, et al., (New York, NY: Springer Science+Business Media, LLC), 455–472. doi: 10.1007/978-0-387-73412-5_30
Smith, D. R., Brockmann, H. J., Beekey, M. A., King, T. L., Millard, M. J., and Zaldivar-Rae, J. (2017). Conservation status of the American horseshoe crab (Limulus polyphemus): a regional assessment. Rev. Fish Biol. Fish. 27, 135–175. doi: 10.1007/s11160-016-9461-y
Spirn, A. W. (2000). “Ian McHarg, landscape architecture, and environmentalism: ideas and methods in context,” in Environmentalism in Landscape Architecture, ed M. Conan (Washington, DC: Dumbarton Oaks Research Library and Collection), 97–114.
Start, D., Barbour, M. A., and Bonner, C. (2020). Urbanization reshapes a food web. J. Anim. Ecol. 89, 808–816. doi: 10.1111/1365-2656.13136
Steiner, F., Simmons, M., Gallagher, M., Ranganathan, J., and Robertson, C. (2013). The ecological imperative for environmental design and planning. Front. Ecol. Environ. 11, 355–361. doi: 10.1890/130052
Steiner, F. R., Weller, R., M'Closkey, K., and Fleming, B., (Eds.). (2019). Design With Nature Now. Cambridge, MA: Lincoln Institute of Land Policy.
Sterba, J. (2012). Nature Wars: The Incredible Story of How Wildlife Comebacks Turned Backyards Into Battlegrounds. New York, NY: Crown Publishers.
Strain, E. M. A., Olabarria, C., Mayer-Pinto, M., Cumbo, V., Morris, R. L., Bugnot, A. B., et al. (2018). Eco-engineering urban infrastructure for marine and coastal biodiversity: which interventions have the greatest ecological benefit? J. Appl. Ecol. 55, 426–441. doi: 10.1111/1365-2664.12961
Sukopp, H. (2008). “The city as a subject for ecological research,” in Urban Ecology: An International Perspective on the Interaction Between Humans and Nature, eds J. M. Marzluff, E. Shulenberger, W. Endlicher, M. Alberti, G. Bradley, C. Ryan, et al., (New York, NY: Springer Science+Business Media LLC), 281–298. doi: 10.1007/978-0-387-73412-5_17
Sullivan, L. H. (1896). The tall office building artistically considered. Lippincotts Mag. 1896, 403–409.
Svenning, J.-C., Pedersen, P. B. M., Donlan, C. J., Ejrnæs, R., Faurby, S., Galetti, M., et al. (2016). Science for a wilder Anthropocene: synthesis and future directions for trophic rewilding research. Proc. Natl. Acad. Sci. U.S.A. 113, 898–906. doi: 10.1073/pnas.1502556112
Tanner, C. J., Adler, F. R., Grimm, N. B., Groffman, P. M., Levin, S. A., Munshi-South, J., et al. (2014). Urban ecology: advancing science and society. Front. Ecol. Environ. 12, 574–581. doi: 10.1890/140019
Toft, J. D., Ogston, A. S., Heerhartz, S. M., Cordell, J. R., and Flemer, E. E. (2013). Ecological response and physical stability of habitat enhancements along an urban armored shoreline. Ecol. Eng. 57, 97–108. doi: 10.1016/j.ecoleng.2013.04.022
Treib, M., (Ed.). (1989). Modern Landscape Architecture: A Critical Reivew. Cambridge, MA: The MIT Press.
Tylianakis, J. M., and Morris, R. J. (2017). Ecological networks across environmental gradients. Annu. Rev. Ecol. Evol. Syst. 48, 25–48. doi: 10.1146/annurev-ecolsys-110316-022821
U.S. Army Corps of Engineers (2012). Army Corps Restores Marsh Islands in Jamaica Bay N.Y. Available online at: http://www.nan.usace.army.mil/Media/NewsStories.aspx
Uchida, K., Blakey, R. B., Burger, J. R., Cooper, D. S., Niesner, C. A., and Blumstein, D. T. (2021). Urban biodiversity and the importance of scale. Trends Ecol. Evol. 36, 123–131. doi: 10.1016/j.tree.2020.10.011
Ulanowicz, R. E., Hot, R. D., and Barfield, M. (2014). Limits on ecosystem trophic complexity: insights from ecological network analysis. Ecol. Lett. 17, 127–136. doi: 10.1111/ele.12216
USDA Natural Resources Conservation Service (2020). Wildlife Habitat. Available online at: https://www.nrcs.usda.gov/wps/portal/nrcs/detail/national/newsroom/features/?cid=nrcs143_023553
Valls, A., Coll, M., and Christensen, V. (2015). Keystone species: toward an operational concept for marine biodiversity conservation. Ecol. Monogr. 85, 29–47. doi: 10.1890/14-0306.1
Vickery, V. R., and Kevan, D. K. M. (1983). A Monograph of the orthopteroid insects of Canada and adjacent regions. Lyman Entomol. Museum Memoir 13, 1–1462.
Waldheim, C. (2016). Landscape as Urbanism: A General Theory. Princeton, NJ: Princeton University Press.
Walls, E. A., Berkson, J., and Smith, S. A. (2002). The horseshoe crab, Limulus polyphemus: 200 million years of existence 100 years of study. Rev. Fish. Sci. 10, 39–73. doi: 10.1080/20026491051677
Warren, P., Tripler, C., Bolger, D., Faeth, S., Huntly, N., Lepczyk, C., et al. (2006). Urban food webs: predators, prey, and the people who feed them. Bull. Ecol. Soc. Am. 87, 387–393. doi: 10.1890/0012-9623(2006)87[387:UFWPPA]2.0.CO;2
Keywords: urban ecology, urban food webs, landscape architecture, ecological design, species assemblages, trophic structures, design process
Citation: Felson AJ and Ellison AM (2021) Designing (for) Urban Food Webs. Front. Ecol. Evol. 9:582041. doi: 10.3389/fevo.2021.582041
Received: 10 July 2020; Accepted: 23 March 2021;
Published: 30 April 2021.
Edited by:
Mary L. Cadenasso, University of California, Davis, United StatesReviewed by:
Frederick R. Adler, The University of Utah, United StatesLoren B. Byrne, Roger Williams University, United States
Copyright © 2021 Felson and Ellison. This is an open-access article distributed under the terms of the Creative Commons Attribution License (CC BY). The use, distribution or reproduction in other forums is permitted, provided the original author(s) and the copyright owner(s) are credited and that the original publication in this journal is cited, in accordance with accepted academic practice. No use, distribution or reproduction is permitted which does not comply with these terms.
*Correspondence: Aaron M. Ellison, aellison@fas.harvard.edu