- 1Unidad de Botánica, Departamento de Farmacologiìa, Farmacognosia y Botaìnica, Facultad de Farmacia, Universidad Complutense de Madrid, Madrid, Spain
- 2Departamento de Biologiìa y Geologiìa, Fiìsica y Quiìmica Inorgaìnica, Universidad Rey Juan Carlos, Madrid, Spain
Despite the constraining weight of summer drought over plant life which distinguishes Mediterranean high-mountains, and its anticipated exacerbation under the current climate crisis, there is still little knowledge of the underlying drought-endurance mechanisms in Mediterranean high-mountain species, such as osmolyte accumulation. To fill this gap, we studied the role of two of the most frequent osmoregulators in plants, proline and osmotically active carbohydrates (OAC), as pointers of the drought-stress response in seven high-mountain plant species representative of the high-mountain plant communities in Central Spain, along an elevation gradient. Overall, our results are consistent with the escalation of summer drought and suggest the involvement of osmolytes to sustain plant activity in these specialists during the growing season. Proline content showed a steadily increasing pattern in line with the seasonal aggravation of summer drought. The significant rise in mean proline in most species, coinciding with the periods with the greatest decline in soil water content, suggests the recurrent role of proline in the drought-stress response in the studied specialists. The lack of significant differences between elevations and the minimal seasonal variations in the OAC content suggest a fixed OAC content independent of functional type to sustain metabolic functions under summer drought. Moreover, these findings allow inferring the action of both OAC and proline as osmoregulators, allowing to support plant functions in these specialists under atypically dry conditions. Overall, our findings are consistent with proline as a major osmoprotectant strategy over OAC buildup in these specialists, which may be related to an adaptation strategy associated with the briefness of the growing season and the incidence of less favorable conditions in Mediterranean high-mountains.
Introduction
The impact of drought on ecosystem functioning and services depends on both plant-related attributes, including the morphology and phenology of co-occurring species, and the interaction with fine-scale environmental factors that can exacerbate or mitigate climatic conditions (Körner, 2003; Kreyling et al., 2008). Mediterranean-type ecosystems are among those where drought exerts a more critical impact, solely because the highest temperatures, registered during summertime, concur with the period of almost no precipitation (Ruiz-Labourdette et al., 2014; Piper et al., 2016). Mediterranean high-mountains are highly susceptible to the predicted aggravation of climate conditions, particularly during summertime (Bravo et al., 2008; Giorgi and Lionello, 2008). It is not known whether vegetation adapted to the current summer drought levels, will be able to survive the more severe droughts that are anticipated in the future. The incidence of drought in these high-mountain areas is almost counterintuitive since alpine habitats (i.e., temperate-zone areas above the tree line) are well-known for being subject to cold stress but seldom to water limitations (Körner, 2003). In contrast, Mediterranean high-mountain areas experience both summer stress (i.e., co-occurring rainfall shortfalls, high temperatures, and high irradiance) and, as in the case of other mountains, the occasional freezing events during the period in which temperatures are suitable for plant growth (Giménez-Benavides et al., 2007; Sanfuentes et al., 2012). Confirmation of the severe water deficit in these mountains during summer has been attained via direct measurements of topsoil water content (SWC), denoting an aggravation at lower elevations (<5% SWC in July; Giménez-Benavides et al., 2007; Gutiérrez-Girón and Gavilán, 2013; Piper et al., 2016). Summer drought has also been found to be a critical factor for phenology, reproduction, and distribution patterns in several high-mountain specialists in these summits (Giménez-Benavides et al., 2007; García-Camacho and Escudero, 2008; García-Fernández et al., 2013; Gutiérrez-Girón and Gavilán, 2013). The incidence of summer drought during the short active growing season has been determined as a critical ecological and evolutive challenge for plant life in Mediterranean high-mountains (Giménez-Benavides et al., 2011). Moreover, since summer drought is a primary constraint for plant performance and survival in these habitats (Gutiérrez-Girón and Gavilán, 2013), these specialists can be expected to deploy a wide variety of strategies and adaptations (e.g., biochemical) to overcome summer drought.
From a physiological perspective, plant responses to drought involve the synchronization of processes to alleviate cell hyperosmolarity and osmotic imbalance. Osmolyte accumulation is a widespread mechanism in plants targeting the avoidance of water loss and cell turgor (Hayat et al., 2012). However, it is worth stating that some of these metabolic changes are common to several stresses (i.e., salt, drought, and temperature stress), whereas others are specific to one type of stress (Krasensky and Jonak, 2012). Proline (hereafter “Pro”) is an amino acid and one of the main osmolytes accumulated in plants in response to salinity, low temperatures, and drought stress (Hayat et al., 2012; Montesinos-Pereira et al., 2014; Per et al., 2017 and references therein; Roychoudhury et al., 2015). Thus, Pro buildup could be expected to occur in Mediterranean high-mountain plants in response to the seasonal aggravation of summer stress. Pro functions as a stabilizer of cell membranes, added to its alleged ROS scavenging capacity (Hayat et al., 2012). Another well-recognized response to drought and freezing stress in plants is the accumulation of osmotically-active carbohydrates (OAC; i.e., soluble sugars and fructans). OAC accumulation is acknowledged as a physiological response that causes sustained hydraulic function and osmoregulation maintenance, both essential mechanisms increasing plant survival under drought conditions (Gibson, 2005; Sala et al., 2012; O’Brien et al., 2014). For instance, the accumulation of fructans and raffinose-family-oligosaccharides enhances the stability of phospholipidic mono- and bilayers, as they directly insert between polar headgroups (Vereyken et al., 2003; Hincha et al., 2006; Valluru and Van den Ende, 2008). Sucrose buildup is closely related to decreasing membrane permeability, given its interaction with the phosphate in membrane-lipid headgroups to avoid cellular dehydration (Strauss and Hauser, 1986). Fructans have also been shown to play an essential role in the tolerance to drought, freezing and salt stress in plants. Numerous fructan-accumulating species are found in habitats characterized by periods of seasonal drought or frost, similar to those fructan-accumulating transgenic plants evidencing enhanced stress tolerance (Van den Ende, 2013 and references therein). Moreover, raffinose and sucrose enrichment in plastids suggests they play a role as cryoprotectants, as they promote photosystem stabilization against freezing damage and prevent electrolyte leakage in thylakoid membranes (Knaupp et al., 2011; Doltchinkova et al., 2013). Further compatible solutes have been identified as important contributors of osmotic adjustment in certain groups of plants under several abiotic stresses, e.g., glycine betaine (GB), polyols, trehalose (Krasensky and Jonak, 2012). Together with Pro, GB is acknowledged as one of the main osmolytes accumulated in plants during prolonged abiotic stresses, since it significantly contributes to increasing osmotic pressure, balance the vacuolar osmotic potential, and stabilizing the structural and functional integrity of protein complexes and membranes against ROS (Annunziata et al., 2019 and references therein; Krasensky and Jonak, 2012). However, GB primarily accumulates in young tissues, and with much higher levels in halophytes than in glycophytes (only found in few species from the Poaceae family; Annunziata et al., 2019). As for trehalose, it can function as an osmolyte and stabilize proteins and membranes at sufficient levels, though studies have only reported high trehalose levels in some desiccation-tolerant plants (Drennan et al., 1993; Paul et al., 2008). Meanwhile, the available studies show trehalose is present in trace amounts in most angiosperms, showing only relatively moderate increases in its levels under osmotic stress (Krasensky and Jonak, 2012 and references therein).
Dehydration stress in plant cells triggered by the incidence of drought or freezing reveals a close relationship between summer drought and freezing-stress responses in Mediterranean high-mountain specialists, as evidenced by their high endurance to extreme freezing events during their short growing season (Sierra-Almeida et al., 2009; Pescador et al., 2016; Sierra-Almeida et al., 2016). Although plants from Mediterranean high-mountains are distinguished for their resilience to limited water availability in the growing season (Larcher, 2000; Magaña Ugarte et al., 2019), the specific mechanisms underlying the summer-drought response in these specialists remain understudied. Understanding the extent of osmolyte accumulation (e.g., Pro and OAC) and its seasonal variation among species to withstand summer drought is a priority for Mediterranean high-mountain specialists and narrowly distributed species.
Osmolyte content varies according to the environmental seasonality experienced by plants, which results in higher osmolyte levels during stressful periods (Palacio et al., 2007; Meletiou-Christou and Rhizopoulou, 2017). These accumulation patterns may also vary as a consequence of microclimatic variations driven by elevation, exposition, or other local factors typical of high-mountain environments (Körner, 2003; Bansal and Germino, 2010; Li et al., 2016). Specifically, the present study aims to evaluate (1) the seasonal patterns of Pro and OAC accumulation in Mediterranean high-mountain specialists, and (2) whether this variation changes along an elevation gradient and in consecutive and climatically contrastingly years. More specifically, due to the incidence of summer drought on the short growing season in Mediterranean high-mountain habitats and its seasonal aggravation (Cavieres et al., 2000; Gutiérrez-Girón and Gavilán, 2013) we expect to find an increase in the accumulation of Pro and soluble sugars in these high-mountain specialists alongside the aggravation of summer drought. Similarly, we expect the osmolyte accumulation may differ depending on elevation. Since the drought-severity gradient increases as elevation decreases (Giménez-Benavides et al., 2007; Sierra-Almeida et al., 2009), populations from the lowest end of the elevation gradient could be subjected to most water stress. Thus, we would expect a local advantage to dry settings in the low-elevation populations due to better adaptation to more intense drought stress conditions compared to their high-elevation counterparts.
Materials and Methods
Study Site and Selected Species
The study site was located in the Sierra de Guadarrama National Park (central Spain, 40° 44′ 14″ N, 3° 43′ 48″ W), an E-W running mountain range characterized by a Mediterranean-type climate with an intense dry season during summer, from June to September (less than 10% of total annual rainfall; Gutiérrez-Girón and Gavilán, 2013). Vegetation at the tree line (1,900–2,000 m.a.s.l.) is dominated by Pinus sylvestris, interspersed in a matrix of shrubby species (Cytisus oromediterraneus and Juniperus communis subsp. alpina) and perennial herbs, such as the dominant grass Festuca curvifolia Lag. ex Lange (Fernández-González, 1991). With increasing elevation (from 2,100–2,200 to 2,430 m), the Cytisus-Juniperus shrub community is replaced by patchy xerophytic pasture dominated by Festuca curvifolia (=F. carpetana; Fuente and Sánchez, 2014), cohabiting with several high-mountain specialists (Pescador et al., 2014). The study was set in the Bola del Mundo sector (40.785°N, 3.979°W) across the elevational gradient of this high-mountain grassland community on this summit (from 1,980 to 2,244 m). The sample collection took place during the 2017 and 2018 growing seasons (June–September), in two climatically contrasting years (Figure 1 and Supplementary Figure 1). Average day-time temperatures in 2017 were 1.5°C higher in June and August 2017 than in the same months in 2018. Relatively cold temperatures were registered in the first half of the growing season in 2018, followed by extremely high mean daytime temperatures (>2°C than AEMET monthly reference values) in the second half (Supplementary Figure 1 and Supplementary Table 2). Average SWC varied substantially through the growing season and between years, reaching considerably lower values at the end of the 2017 growing season, compared to the following year (Figure 1). Henceforth 2018 will be regarded as a “standard” year given the low number of days per month with zero rainfall and temperatures above mean monthly values, while 2017 will be classified as a dry year (Supplementary Figure 1).
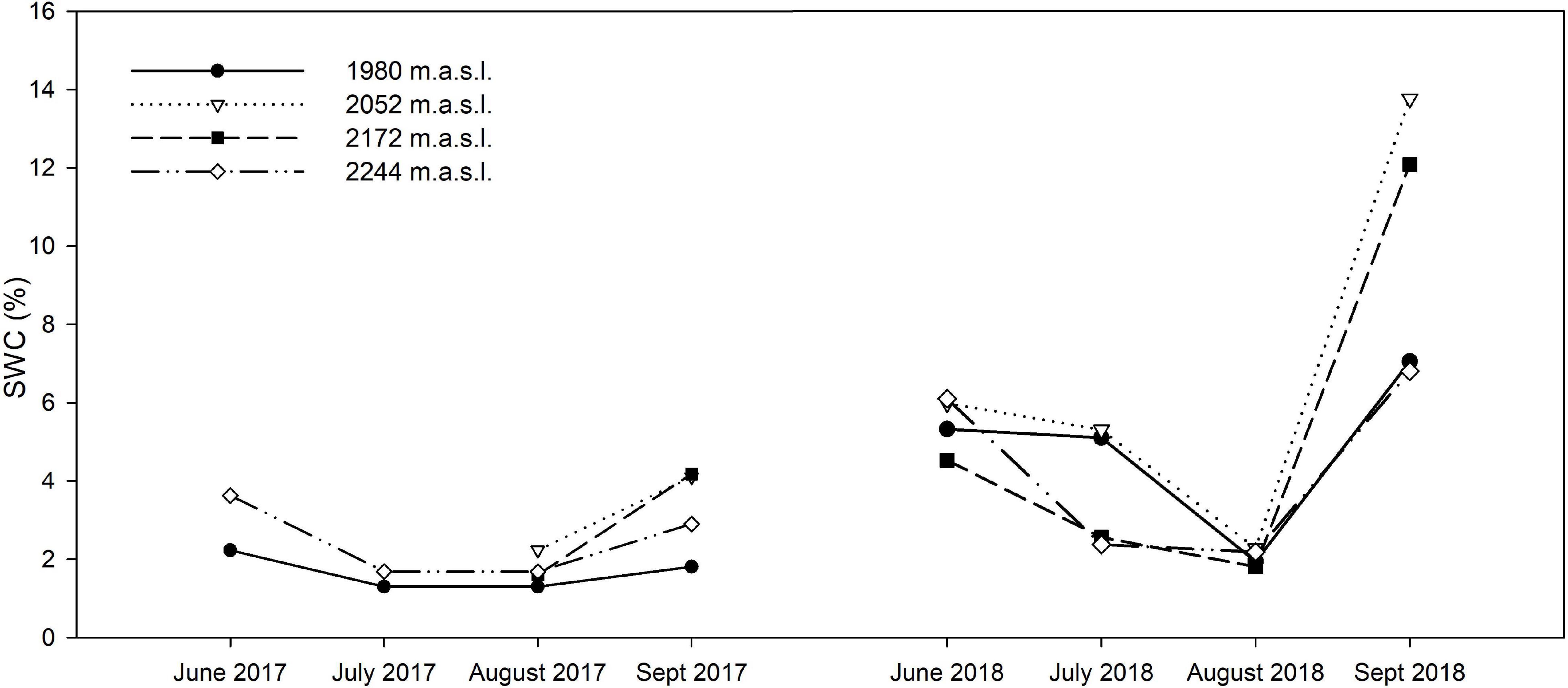
Figure 1. Seasonal variation in SWC along the studied altitudinal gradient through the active growing season of vegetation in the study site. Data corresponds to in-site measurements on both years of study (2017, 2018).
Along the aforementioned elevation gradient, we selected four sites on NW and W facing slopes. One 20×20 m plot was established in each of the four sampling sites on homogeneous pasture areas. The most abundant species were identified in each plot and selected for this study, providing a complete representation of the phylogenetic diversity of this plant community (Pescador et al., 2014). The seven species selected included the chamaephytes Armeria caespitosa (Gómez Ortega) Boiss. in DC, and Juniperus communis subsp. alpina (Suter) Čelak (hereafter J. alpina); and the hemicryptophytes Erysimum penyalarense (Pau) Polatschek, Festuca curvifolia Lag. ex Lange, Jurinea humilis (Desf.) in DC, Hieracium vahlii Froelich. in DC, and Senecio pyrenaicus subsp. carpetanus (Willk.) Rivas-Mart. Further details on the growth form and family of each selected species are provided in Supplementary Table 3. All species are found in the two highest sampling sites (above 2,100 m), while only E. penyalarense, J. alpina, F. curvifolia, and S. carpetanus occur throughout the entire elevation gradient. The selected species encompassed from narrow-endemics, such as E. penyalarense, to widely distributed ones, e.g., J. alpina. All species included in this study are considered as high-mountain specialists (Clavel et al., 2011) as they appear almost exclusively in high-mountain grasslands above the tree line (Fernández-González, 1991; Jiménez-Alfaro et al., 2014).
Field Sampling
We sampled the available populations of the selected plant species in four sites located along a rough altitudinal gradient covering the presence of these oromediterranean pastures above the tree line. These four representative and well conserved remnants of these habitats where located close to the Bola del Mundo summit at the following elevations: 1,980, 2,052, 2,172, and 2,244 m asl. These four localities were sampled in two consecutive years, 2017 and 2018. For valid comparisons of osmolyte buildup between years and elevations, plants were sampled at the same developmental stages in both growing seasons. Available plants were sampled in these two years in June, July, August and September, covering the complete vegetative growth period in these mountains. The list of species per locality were at 1,980 m: E. penyalarense, F. curvifolia, J. alpina, and S. carpetanus; at 2,052 m: E. penyalarense, F. curvifolia, J. alpina, J. humilis, and S. carpetanus; at 2,172 and 2,244 m: A. caespitosa, E. penyalarense, F. curvifolia, H. vahlii, J. alpina, J. humilis, and S. carpetanus.
For each species, young, fully developed leaves were taken from randomly selected healthy, mature individuals to evaluate Pro and OAC concentrations. The number of individuals sampled per species varied depending on their natural abundance each year. Thus, a minimum of 10 individuals was sampled per plot when available, taking five leaves per individual. Mature green leaf samples were selected to guarantee less fluctuating osmolyte levels. Samples were stored immediately in liquid nitrogen to avoid degradation of metabolites during transfer to the lab. All samples for OAC analysis were collected in a brief period afore sunrise, to minimize the effect of diurnal OAC fluctuations. Fully developed green leaf samples collected from contrasting elevations (1,980 and 2,244 m) taken at the end of each growing season were used to study the effect of elevation on OAC accumulation in these species.
SWC was measured in all plots at the time of plant sampling on all dates in 2017 and 2018 at the highest and lowest elevations, and at mid-elevations at all dates in 2018 and only on the last two sampling events in 2017. SWC was measured using a hand-held, time-domain reflectometry sensor (FieldScout TDR 300; Spectrum Technologies, Plainfield, IL, United States), to a depth of 10 cm and taking a mean of ca. 5 measurements per plot in randomly located sites with plant cover. Climate records were obtained from the Puerto de Navacerrada meteorological station in Madrid, run by the Spanish Institute of Meteorology1.
Proline Quantification
The proline content in the leaf tissue was determined using the ninhydrin-based colorimetric method (Abrahám et al., 2010), with minor modifications. Approximately 100 mgFW of leaf samples were thoroughly ground in liquid nitrogen. Next, 3% sulfosalicylic acid was added to each grounded leaf sample, followed by the thorough grinding of plant material. Samples were centrifuged for 5 min at room temperature using a bench-top centrifuge at maximum speed (12,000 ×g). Afterward, 100 μL from the supernatant of each plant extract was added to the new tubes containing the reaction mixture (100 μL of 3% sulfosalicylic acid, 200 μL glacial acetic acid, and 200 μL acidic ninhydrin), and carefully mixed. Tubes were incubated at 96°C for 60 min, and the reaction was terminated on ice. Extraction was performed by adding 1 mL of toluene to each reaction tube. The tubes were then vortexed for 20 s and left to settle until the phase separation could be observed. The chromophore, organic part containing the toluene, was then used for measuring absorbance at 520 nm with a UV-Vis spectrophotometer (UV-1700 PharmaSpec, Shimadzu, Kyoto, Japan) in quartz cuvettes, using toluene as blank. Proline concentration was determined using a standard concentration curve and calculated on a dry weight basis (μmol/gDW).
Quantification of Osmotically-Active Carbohydrates
In this research, the OAC refer to soluble sugars (fructose, glucose, sucrose, raffinose), and fructans (inulin). Samples were analyzed to determine the presence and concentrations of each OAC mentioned above, and total OAC content (OACTOTAL). Sample extraction and determination were performed following the protocol by Weiss and Alt (2017), with minor modifications.
The plant material was grinded into an ultrafine powder which was subsequently freeze-dried to dehydrate and adequately freeze the samples to avoid degradation. Approximately 50 mg of each freeze-dried sample was weighed, transferred into 12 mL Falcon tubes, and mixed with DIO water, and heated at 80°C for 30 min. Three consecutive centrifugation steps and dilution steps were performed to obtain a supernatant. Next the tubes were placed in a horizontal shaker, set at 30 revolutions/min for 1 h. After shaking, precisely 1.5 mL of the supernatant was filtered through an HPLC syringe filter (0.45 μm) into an HPLC vial. A volume of 20 μL per sample was injected. Soluble sugar concentrations were measured using a Carbo Sep CHO-682 Lead Carbohydrate HPLC-Column (300 × 7.8 mm), in a UHPLC Nexera System (Shimadzu Corp. Kyoto, Japan) in 50 min runs. HPLC water was used as the mobile phase. Samples were analyzed in a gradient mode. Sugar concentrations (μg/mgDW) for fructose, glucose, inulin, raffinose, and sucrose were determined by comparison with standards, and OACTOTAL for each sample was calculated with the sum of all quantified carbohydrates.
Statistical Analysis
The effect of the temporal variation throughout the growing season (related to the progression of summer drought) and elevation on Pro content was assessed in seven Mediterranean high-mountain species in two consecutive and climatically contrasting years using Linear Mixed Models (LMMs); via restricted maximum likelihood (REML; Patterson and Thompson, 1971). Given that the data was collected repeatedly over time, the data analyses were performed as follows: (1) an overall analysis, with the additive effect of elevation and date of sampling (within a year) as fixed factors, and species as a random factor; and (2) a separate analysis for each species, including the effect of elevation and date of sampling (between years) as fixed factors, and individual as a random factor since they were submitted to several measurements along time. Mean Pro content per species and individual were modeled with Gaussian error distribution, using the function “lme” from package “nlme” (Pinheiro et al., 2018). The response variables were transformed (logarithmic) to achieve a normal distribution of the residuals when required. Full models were simplified by removing non-significant parameters to obtain more parsimonious models. Fixed factor significance was assessed via posteriori likelihood ratio tests.
Seasonal variations of OAC in leaves, total and by specific carbohydrates, were assessed per species at the beginning and end of each growing season (2017, 2018), and at the highest and lowest elevations using nested ANOVAs. Additional LMMs were implemented with the additive effect of elevation and sampling date (within a year) as fixed factors, and sample identity as the random factor to address and avoid temporal and pseudo-replication. The response variables were inulin, fructose, glucose, raffinose, sucrose, and OACTOTAL. Final model selection was achieved by deleting the non-significant interactions, followed by deleting those variables explaining the less variation.
All statistical analyses were performed with the R statistical package (R Core Team, 2018).
Results
The climatic data retrieved from AEMET and the calculated ombrothermic indexes (Rivas-Martínez, 2007) indicated 2018 was a “standard” year given the low number of days per month with zero rainfall and air temperatures above mean monthly values, while 2017 will be classified as a dry year (Supplementary Figure 1 and Supplementary Table 2). Moreover, the statistical analyses indicated that SWC was not strongly correlated with elevation in our gradient, while denoting a strong negative correlation with the date of sampling, i.e., the progress of the growing season (Supplementary Table 4).
Proline Content
Pro content varied significantly throughout the growing season in both years, whereas the effect of elevation depended on the species. In the overall analysis with species as a random factor, there was a significant accumulation in mean Pro as the growing season advanced (p < 0.05). Conversely, our model showed no significant mean changes in Pro along the elevation gradient (p > 0.05). Our analysis revealed a significant difference in the mean Pro between years, with a higher overall Pro accumulation during the atypically dry year 2017 than under the milder conditions of 2018. The most significant increase in mean Pro occurred between July and August in both years (p < 0.001), as were the lowest SWC measurements. Overall, there was a significant temporal increase in mean Pro per species during the second half of the growing season in both years (Figures 2, 3). Seasonal mean Pro content per species was substantially higher in 2017 than in 2018 in E. penyalarense (295.5 vs. 268.3 μmol/gDW), J. humilis (229.1 vs. 73.3 μmol/gDW), J. alpina (89.4 vs. 71.5 μmol/gDW), and S. carpetanus (357.4 vs. 290.9 μmol/gDW), in contrast to the findings in A. caespitosa (78.1 vs. 111.4 μmol/gDW), F. curvifolia (58.7 vs. 84.7 μmol/gDW), and H. vahlii (102.4 vs. 141.0 μmol/gDW). The seasonal accumulation pattern for mean Pro was recurring in A. caespitosa and E. penyalarense, while no clear accumulation pattern was observed in the remaining high-mountain specialists.
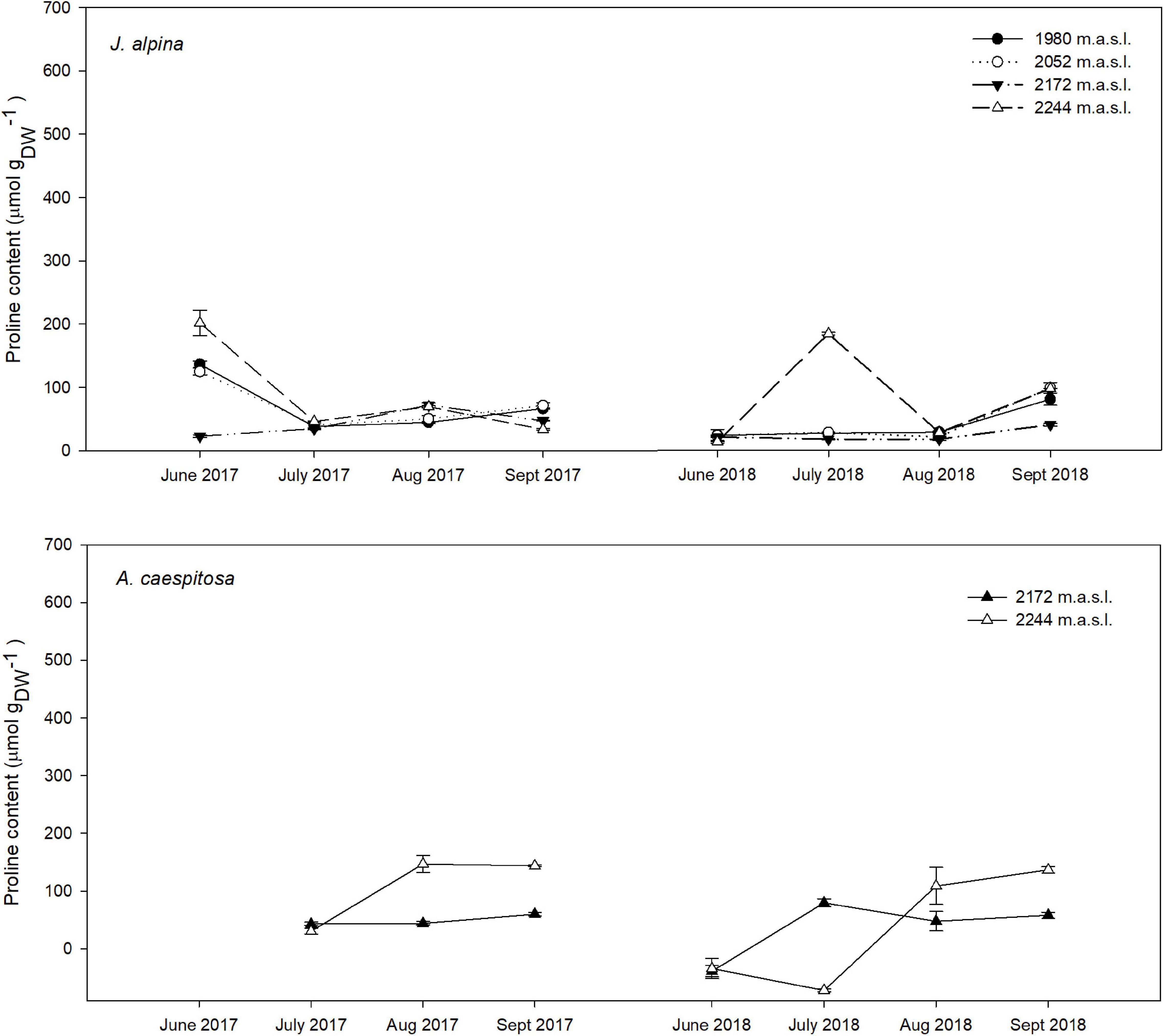
Figure 2. Seasonal variation in Pro content in the studied chamaephytes (dwarf shrubs), J. alpina, and A. caespitosa at each sampling site and throughout their active growing season in 2017, 2018. For each sampling event, n = 10.
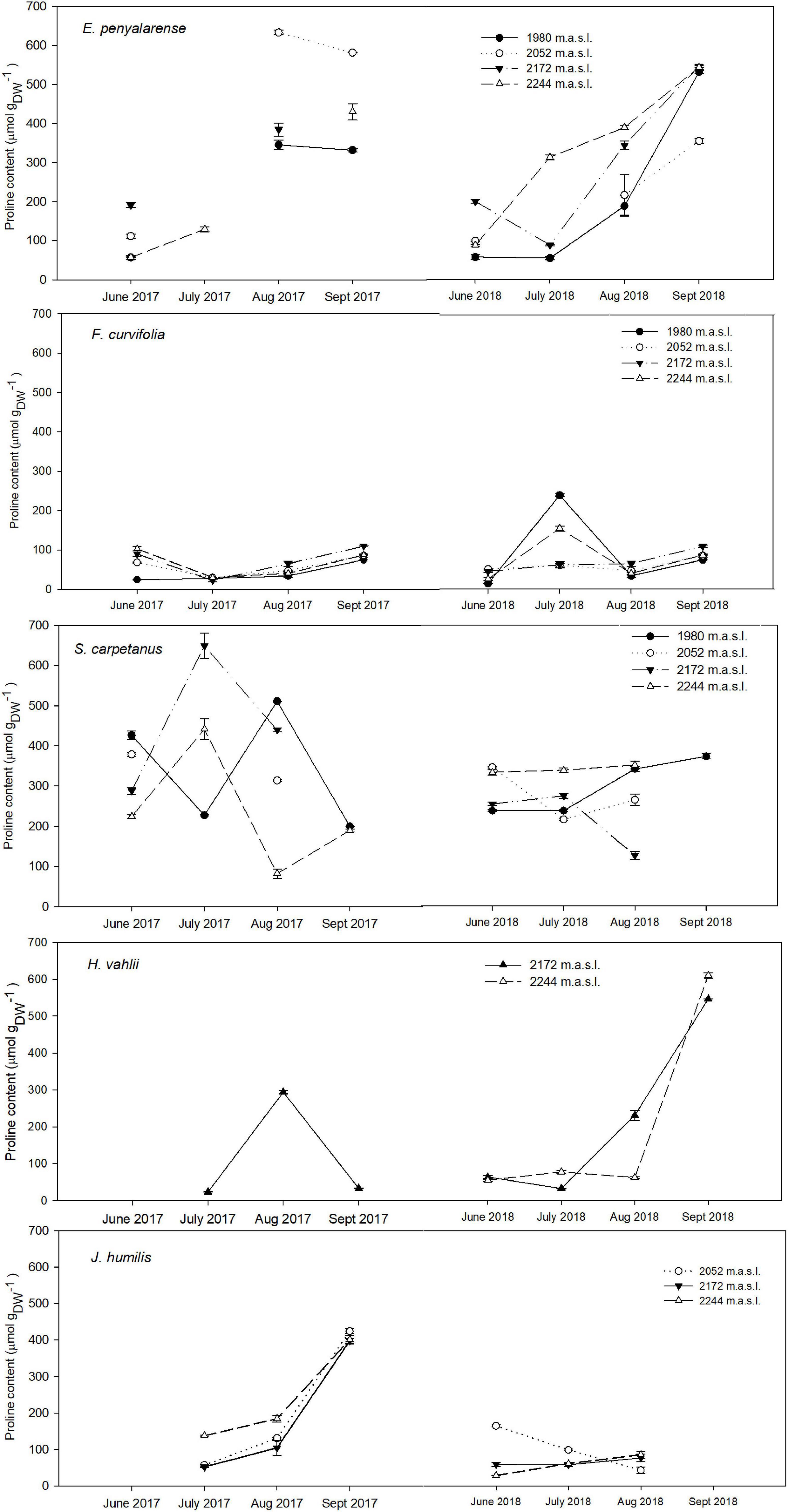
Figure 3. Seasonal variation in Pro content in hemicryptophytes E. penyalarense, F. curvifolia, S. carpetanus, H. vahlii, and J. humilis in both years of study (2017, 2018) throughout the elevation gradient. For each sampling event, n = 10.
In chamaephytes, the Pro buildup showed significant changes throughout the growing season, in parallel with the seasonal decline of SWC (summer drought). In both years of study, Pro content in A. caespitosa significantly increased throughout the growing season (p < 0.001; Figure 2). Regarding J. alpina, Pro content only showed significant seasonal variation in plots located above the optimum elevation for J. alpina (∼2,000 m; p < 0.001) when the SWC suffered rapid declines.
In the case of hemicryptophytes, significant changes in Pro content with the aggravation of seasonal drought were almost unanimous in all models per species, while the effect of elevation diverged among them. In both years of study, Pro content increased significantly in E. penyalarense during the growing season and with elevation (p = 0.021 and 0.001, respectively), with a notable increase in the second half of the active growing season (Figure 3). Similarly, Pro content in J. humilis increased significantly during the growing season in 2017 (p < 0.001); while denoting no significant changes with elevation (p = 0.35). Furthermore, as SWC declined through the growing season, with a sharp decrease between July and August 2018, Pro buildup significantly increased throughout the growing season in H. vahlii (p < 0.001).
Seasonal Variation in OAC Content
Although OACTOTAL diverged between years in the studied species without significant differences between contrasting elevations (2,244 and 1,980 m), all species showed a general pattern whereby they maintained a substantial OACTOTAL during the course of the growing season (Supplementary Table 1). Overall, a significantly higher OACTOTAL was found during an atypically dry growing season (i.e., 2017) compared to a “standard” year (i.e., 2018; p < 0.05), without significant differences between elevations.
The analyses per species revealed that, in a “standard” year, OACTOTAL in E. penyalarense increased in line with the seasonal decrease in SWC. Additionally, a significant seasonal increase in inulin content was measured in this species (Supplementary Table 1). Comparably, OACTOTAL in S. carpetanus increased during the growing season in the lowest elevation, coupled with a significant seasonal increase in fructose and substantial rise in raffinose content. Inulin content maintained high seasonal levels in S. carpetanus without significant differences between elevations (Supplementary Table 1). Similarly, J. alpina maintained high inulin levels through the growing season in high-elevation individuals, contrasting with the substantial seasonal decline in those from the lowest elevation. The OACTOTAL decreased through the growing season in F. curvifolia at both elevations, though high inulin content was maintained all through the growing season without significant differences between contrasting elevations.
During the dry growing season of 2017, OAC content showed minor changes through the growing season and was exempt of significant differences between contrasting elevations. In 2017, E. penyalarense OACTOTAL increased substantially during the growing season, without significant differences between elevations, and a significant seasonal increase in inulin content (Supplementary Table 1). A significant seasonal increase in sucrose, fructose, and raffinose content was also observed in E. penyalarense at the highest elevation. In F. curvifolia, high inulin content was maintained throughout the growing season. Inulin and OACTOTAL significantly declined in S. carpetanus at both elevations (Supplementary Table 1), while raffinose content increased considerably only in the low-elevation individuals. As for J. alpina, a considerable reduction in inulin and significant raffinose buildup was found only in individuals from the highest elevation.
Discussion
In the summits of Sierra de Guadarrama, snow-melting water is the primary source of soil moisture and it decreases progressively as summer advances (Giménez-Benavides et al., 2011; García-Fernández et al., 2013; Gutiérrez-Girón and Gavilán, 2013). Our results support our initial assumption, showing a significant seasonal accumulation in mean Pro in both years in parallel with the seasonal escalation of summer drought. Coupled with the significantly higher Pro accumulation during an atypically dry year (i.e., 2017) than under a “standard” year, our results showed that the most significant increase in mean Pro occurred during the period of maximum plant activity (July–August), and coincided with the most substantial decrease in SWC in the season. These findings suggest the involvement of Pro accumulation in sustaining plant activity during summer drought and reinforce the idea that summer drought is a major driver of plant life in these Mediterranean mountains (Giménez-Benavides et al., 2011).
Plants can increase their tolerance to several stresses (including heat, low temperatures and drought) in response to a gradual exposure to these constraints, a process known as acclimation (Krasensky and Jonak, 2012). In an osmotic-stress scenario, plants can foster a cytoplasmic osmotic adjustment through Pro and OAC accumulation, enabling the maintenance of cell turgor pressure and low leaf water potential to sustain photosynthetic rates, cell expansion, and growth (Chen and Jiang, 2010; Hayat et al., 2012). The significant seasonal increase in mean Pro content observed in consecutive and climatically contrasting years in A. caespitosa and E. penyalarense alongside the escalation of summer drought (Figures 2, 3, respectively), suggests Pro accumulation as an important response mechanism in these specialists to maintain cell turgor when they are subject to conditions that can impose osmotic stress. In this sense, the different responses observed between climatically contrasting years in each species favor the idea that Pro accumulation in these Mediterranean high-mountain specialists may allude to an acclimation response related to the duration and severity of summer drought.
High-mountain plants have evolved and optimized their life strategies to survive the severe prevailing conditions in these habitats, eliciting different enduring mechanisms when the threshold for an environmental variable is exceeded (Bonhert and Sheveleva, 1998; Körner, 2003). In the Mediterranean high-mountains, the picture is more complicated since drought stress, together with freezing events, plays a critical adaptive role given its seasonal aggravation and the interannual variations it undergoes (Giménez-Benavides et al., 2007; Figure 1 and Supplementary Figure 1). Responses to drought and low-temperature stress are clearly linked and widely found in high-mountain vegetation from temperate bioclimates (Magaña Ugarte et al., 2019). The incidence of freezing episodes during the growing season in Mediterranean mountains results in concurrent drought and freezing stresses, with both inflicting osmotic stress on plant cells and tissues (Valladares et al., 2004; Pescador et al., 2016). Exposure to summer drought during the growing season may heighten freezing resistance in these Mediterranean high-mountain specialists, as observed in their Andean counterparts (Sierra-Almeida et al., 2009; Sierra-Almeida et al., 2016). Increased freezing-drought resistance could be achieved through the accumulation of OAC and Pro; two physiological mechanisms related to the freezing-drought response in plants due to their role in sustaining cell growth and guarding cellular components against osmotic stress (Sierra-Almeida et al., 2016; Ahmad and Hameed, 2018). The high inulin and Pro levels found in E. penyalarense and F. curvifolia in our study suggest their role on the osmotic adjustment to protect against the osmotic stress likely imposed by summer drought. Moreover, our findings may provide an explanation for the remarkable freezing resistance described for these two species by Pescador et al. (2016), acting as osmoregulators to maintain cell-membrane integrity and delay ice crystal formation during the sporadic halting events occurring in the growing season (Körner, 2003; Valluru and Van den Ende, 2008). Although it could be argued that occasional freezing events during the growing season could be the primary driver for Pro accumulation, the available climate data indicates that freezing events were uncommon during the period where we detected the highest increase in Pro in most of these specialists (Supplementary Figure 1). Nevertheless, the nature of the study does not allow ruling out the influence of other environmental factors (e.g., temperature variation) on osmolyte accumulation, since both Pro and OAC play a multifunctional role in the plant stress defense (Krasensky and Jonak, 2012). The high Pro and OAC content found in some species toward the end of the growing season could be part of their cold acclimation process, triggered mainly by the low temperatures occurring more frequently late in the growing season. In view of that, and despite the absence of freezing events during our study period, the substantial Pro buildup observed at the end of the growing season in E. penyalarense and A. caespitosa at high elevations (>2,100 m) might imply an acclimation strategy to the annual climate variability in these mountains. This strategy could allow the maintenance of the aboveground portion of the plant through periods with a higher chance of freezing events and cold snaps, enabling a stretched resource-gaining period followed by the potential remobilization of osmoprotectants to meristems and roots to avoid freezing during winter. Thus, these findings suggest the role of Pro accumulation in the freezing-drought response in the studied Mediterranean high-mountain specialists.
Significant differences in the degree of responsiveness to changes in SWC levels were found among these specialists. Supporting our initial assumption, we observed a significant increase in osmolyte contents alongside the seasonal escalation of summer drought and small variations in SWC in most of the studied hemicryptophytes (e.g., E. penyalarense, H. vahlii, J. humilis). Meanwhile, in the other species (e.g., F. curvifolia, J. alpina, S. carpetanus) significant osmolyte buildup was only observed when plants were subjected to severe climatic conditions or extremely reduced SWC. Despite osmolyte accumulation patterns differed among functional groups, these specialists can be classified in terms of their behavior during summer drought as either (1) resilient (i.e., most herbaceous hemicryptophytes), given their increased osmolyte accumulation consistent with summer drought; or (2) robust, comprising the chamaephytes A. caespitosa (dwarf cushion) and J. alpina (shrub), plus two hemicryptophytes: the dominant species F. curvifolia, and perennial herb S. carpetanus. The clear robustness to annual climate variations observed could be related to enhanced life strategies and functional trait differentiation in each biotype. For instance, robustness to climate extremes in chamaephytes is attributed to their atmosphere-decoupling capacity, which allows them to maintain a milder and relatively stable temperature and moisture conditions within their canopy, compared to their surroundings (Körner, 2003). In the case of J. alpina, these findings relate to the superior drought-tolerance reported for junipers, as they present an anisohydric regulation which allows high stomatal conductance and, thus, sustaining high photosynthetic uptake under dry conditions (McDowell et al., 2008; Zweifel et al., 2009). The suggested robustness in S. carpetanus may be linked to long-term feedbacks driven by long-standing environmental coarsening in this mountain range. These feedbacks may have caused the selection of functional traits that confer greater drought endurance, such as smaller leaf areas to reduce the ratio of hydraulic demand to supply (Magaña Ugarte et al., 2020). In F. curvifolia, the inferred robustness could be attributed to its strong competitiveness for resources and dominance in these xerophytic pastures (Pescador et al., 2014). The positive effects exerted by F. curvifolia and its “resource island,” fostered by its dense root system and above-ground biomass, may become negative in the close proximity to the species and within its clumps, enhancing its competitiveness relative to the coexisting species in the community (Pescador et al., 2014).
The seasonal aggravation of drought could induce an increased osmolyte accumulation in plants as an adaptive response, particularly toward the dry-lower end of the elevational gradient (Sierra-Almeida et al., 2009). Moreover, water limitation is probably exacerbated in the thin, sandy-textured soils where F. curvifolia grasslands occur (Pescador et al., 2015). Contrary to our initial assumption, the absence of overall significant differences in osmolyte buildup between populations from contrasting elevations suggests the lack of enhanced endurance to summer drought in low-elevation populations relative to those from the high end. These summits are characterized by the combined effect of two opposed severity gradients operating along the elevation gradient: drought stress which decreases with elevation, and low-temperature stress which increases with elevation; which could neutralize the species net responses to changes in soil moisture availability (Pescador et al., 2015). The latter could provide an explanation of the weak response found in the studied species along the elevation gradient compared to the temporal gradient.
Fructans (e.g., inulin) and raffinose accumulation in vegetative tissues have been shown to contribute to osmotic adjustment in several species by acting as osmotic regulators or maintaining membrane integrity under stressful conditions (Taji et al., 2002; Valluru and Van den Ende, 2008; Krasensky and Jonak, 2012). Resembling the high drought-induced raffinose accumulation found in transgenic A. thaliana (Taji et al., 2002), the seasonal raffinose buildup found solely in low-elevation individuals of E. penyalarense and S. carpetanus in 2017 (Supplementary Table 1) could be a protective mechanism to delay or prevent the negative impact of more severe drought stress at the lowest elevation, compared to higher elevations (Sierra-Almeida et al., 2009). The exposure to constraining conditions over the course of several days could have resulted in high accumulation of raffinose and Pro (Krasensky and Jonak, 2012). Additionally, the maintenance of high inulin levels throughout the growing season in all species at both ends of the elevation gradient is consistent with the findings by Amiard et al. (2003); Vereyken et al. (2003), and Hincha et al. (2006), advocating for its pivotal role in protecting against drought stress via membrane stabilization. Furthermore, the minor reduction observed in the contents of soluble sugars and inulin in these specialists could suggest a requirement for a fixed level in the OAC, unconstrained by functional type, to sustain metabolic functions under summer drought in this elevation gradient (Martínez-Vilalta et al., 2016). In all the studied species, the OAC levels remained relatively constant and high throughout the season, similar to the low seasonal variability of soluble sugars and their slight increase in the summer months reported by Martínez-Vilalta et al. (2016) in a series of Mediterranean lowland species. The latter suggests a widespread OAC pattern in the Mediterranean flora.
The capacity of individual species to adjust to water availability likely drives their presence in a community. Our findings provide suggestive evidence of how summer drought could impose certain limits in these species. For instance, the substantial increase in Pro content in J. humilis, only during a dry year (i.e., 2017), compared with the lower Pro buildup recorded the following year under milder, wetter conditions (Figure 3). Relatedly, J. humilis showed an extended growing season during the atypically dry conditions of 2017 (personal observation), unlike in the milder growing season of 2018 (Supplementary Figure 2). Exposure to a longer growing season in 2017, coupled with the intensification of topsoil desiccation due to a substantially earlier snowmelt date and dry winter, could have prevented the efficient use of the limited water supply (Giménez-Benavides et al., 2007). Moreover, a limited rooting volume explored could have exposed J. humilis to more negative soil water potentials than other species with larger, denser root systems (e.g., F. curvifolia, J. alpina; McDowell et al., 2008) ultimately constraining water. Another example is the observed species occurrence (withdrawal of the aboveground portion and random re-sprouting) and high osmolyte accumulation in E. penyalarense when subject to very low SWC. The observed onset of partial biomass recovery and leaf regrowth following extreme environmental conditions could be attributed to the availability of stored reserves, suggesting the role of the high pre-disturbance OACTOTAL in E. penyalarense’s recovery (Van der Heyden and Stock, 1996; Wyka, 1999; Morvan-Bertrand et al., 2001). In contrast, the low mean Pro generally maintained in J. alpina at all elevations and as the summer drought intensified, coupled with the seldom seasonal changes in OACTOTAL, resembles the notably unaltered behavior observed in other junipers undergoing severe drought (Zweifel et al., 2009; Herrero and Zamora, 2014). This high drought-tolerance can be partly explained by their ability to sustain very low-water potentials due to their cavitation−resistant xylem, low leaf/root area ratio, large water potential gradient from soil to leaf, and their relatively short stature (McDowell et al., 2008). The observed upholding of OACTOTAL levels is in keeping with the inferred survival strategy of hydraulic adjustment for junipers, by means of leaf area reductions to maintain hydraulic function and non-structural carbohydrate pools above critical levels (Limousin et al., 2013). Similarly, we observed the maintenance of low mean Pro content in F. curvifolia in climatically differing years (Figure 3). This could relate to the behavior of the roots of F. curvifolia capturing soil resources and its dense aboveground biomass plausibly trapping both nutrients and water from overflow and/or precipitation (Pescador et al., 2015). Nevertheless, the action of other osmoregulators is not discarded in this graminoid, particularly that of GB. The latter as GB is one of the main osmolytes accumulated in Poaceae to counter osmotic stress (Annunziata et al., 2019). Overall, these findings suggest enhanced hardiness to summer drought in these two species, compared to the other specialists studied. Moreover, the observed capacity of F. curvifolia and J. alpina to restore low Pro levels following the incidence of extreme climatic episodes in June 2017 (a combination of severe drought and heat) underlines their hardiness to extreme climatic events during their growing season. Nevertheless, further research is needed to assess the tolerance of these species to drought stress and determine whether osmolyte accumulation would suffice to avoid detrimental effects on plant fitness and survival in these specialists, given the predicted exacerbation of summer stress in the region.
Our approach, while not allowing for outlining single drought-stress effects, provides support of some of the roles played by the studied osmolytes on plant functions such as osmoregulation. For instance, it is worth noting that the highest increase in mean Pro in some of these species (e.g., E. penyalarense, S. carpetanus, H. vahlii) coincided with the periods with a sharp decline in SWC (July–August; Figure 1), and with their period of reproductive activity (Fernández-González, 1991). Our findings are therefore consistent with suggesting OAC and Pro act simultaneously as osmoregulators in the studied species, with Pro appearing as the primary osmoprotectant strategy, out of the ones studied, in these Mediterranean high-mountain specialists. The proclivity for Pro accumulation observed in these high-mountain plants in preference to OAC buildup could be related to an adaptation strategy associated with the briefness of the growing season and the incidence of less favorable conditions in these habitats. During less favorable conditions, plants could be seeking to optimize photosynthate investment into tissue growth, reproduction, and storage rather than osmoregulation, allowing this task to be generally undertaken by Pro, as observed in A. thaliana under severe water deficit (Hummel et al., 2010). The high Pro accumulation could be further associated with its redox potential and role as a stabilizer in other metabolic processes following stress (Montesinos-Pereira et al., 2014; Ahmad and Hameed, 2018), contributing to counteract the simultaneous effect of various abiotic stressors shaping plant life in high-mountain environments (Körner, 2003).
Data Availability Statement
The original contributions presented in the study are included in the article/Supplementary Material, further inquiries can be directed to the corresponding author/s.
Author Contributions
RM and RG performed the material preparation and data collection. RM, AE, and RG performed the data analysis. RM wrote the manuscript with all authors commenting and proofreading the current and previous versions of the manuscript. AE and RG performed editorial guidance and supervision. All authors read and approved the final manuscript version.
Funding
This research was funded by the Madrid Autonomous Region Government (REMEDINAL-TE CM, S2018/EMT-4338; REMEDINAL-TE CM, S2018/EMT-4338); and the Ministry of Economy of the Spanish Government (ROOTS, CGL2015-66809-P).
Conflict of Interest
The authors declare that the research was conducted in the absence of any commercial or financial relationships that could be construed as a potential conflict of interest.
The handling editor declared a past co-authorship with one of the authors AE.
Acknowledgments
We would like to acknowledge the support of the Phenotypes project. We thank the research group SYSTEMOL and S. Elvira Ph.D., for their assistance with laboratory work. Likewise, thanks are due to technician P. Alonso Valente for her help with HPLC analyses. Special thanks to Prudence Brooke-Turner for performing the English revision. We also acknowledge the staff of the Peñalara Natural Reserve for granting permission to work in the area.
Supplementary Material
The Supplementary Material for this article can be found online at: https://www.frontiersin.org/articles/10.3389/fevo.2021.576122/full#supplementary-material
Footnotes
References
Abrahám, E., Hourton-Cabassa, C., Erdei, L., and Szabados, L. (2010). “Methods for determination of proline in plants,” in Methods in Molecular Biology. Plant Stress Tolerance. Methods and Protocols, ed. R. Sunkar (Totowa: Humana Press), 318–331.
Ahmad, S., and Hameed, M. (2018). Beating cold by being tough: impact of elevation on leaf characteristics in Phleum himalaicum Mez. endemic to Himalaya. Acta Physiol. Plant. 40, 1–17.
Amiard, V., Morvan-Bertrand, A., Billard, J., Huault, C., Keller, F., and Prudhomme, M. (2003). Fructans, but not the sucrose-galactosides, raffinose and foliose, are affected by drought stress in perennial ryegrass. Plant Physiol. 132, 2218–2229. doi: 10.1104/pp.103.022335
Annunziata, M. G., Ciarmiello, L. F., Woodrwo, P., Dell’Aversana, E., and Carillo, P. (2019). Spatial and temporal profile of glycine betaine accumulation in plants under abiotic stresses. Front. Plant Sci. 10:230. doi: 10.3389/fpls.2019.00230
Bansal, S., and Germino, M. J. (2010). Unique responses of respiration, growth, and non-structural carbohydrate storage in sink tissue of conifer seedlings to an elevation gradient at timberline. Environ. Exper. Bot. 69, 313–319. doi: 10.1016/j.envexpbot.2010.05.002
Bonhert, H. J., and Sheveleva, E. (1998). Plant stress adaptations - making metabolism move. Curr. Opin. Plant Biol. 1, 267–274. doi: 10.1016/s1369-5266(98)80115-5
Bravo, D., Araújo, M., Lasanta, T., and Moreno, J. (2008). Climate change in Mediterranean mountains during the 21st century. Ambio J. Hum. Environ. 37, 280–285. doi: 10.1579/0044-7447(2008)37[280:ccimmd]2.0.co;2
Cavieres, L. A., Peñaloza, A., and Kalin, M. (2000). Altitudinal vegetation belts in the high-Andes of Central Chile (33 S). Rev. Chil. Hist. Nat. 73:8. doi: 10.4067/S0716-078X2000000200008
Chen, H., and Jiang, J.-G. (2010). Osmotic adjustment and plant adaptation to environmental changes related to drought and salinity. Environ. Rev. 18, 309–319. doi: 10.1139/a10-014
Clavel, J., Julliard, R., and Devictor, V. (2011). Worldwide decline of specialists species: towards a global functional homogenization? Front. Ecol. Environ. 9, 222–228. doi: 10.1890/080216
Doltchinkova, V., Angelova, P., Ivanova, E., Djilianov, D., and Moyankova, D. (2013). Surface electric charge of thylakoid membranes from genetically modified tobacco plants under freezing stress. J. Photochem. Photobiol. B Biol. 119, 22–30. doi: 10.1016/j.jphotobiol.2012.12.004
Drennan, P. M., Smith, M. T., Goldsworthy, D., and van Staden, J. (1993). The occurence of trehalose in the leaves of the desiccation-tolerant angiosperm Myrothamnus flabellifolius Welw. J. Plant Physiol. 142, 493–496. doi: 10.1016/s0176-1617(11)81257-5
Fernández-González, F. (1991). La vegetación del valle del Paular (Sierra de Guadarrama, Madrid), I. Lazaroa 12, 153–272.
Fuente, V., and Sánchez, D. (2014). Festuca carpetana (Poaceae), a new species for the Iberian flora. Lazaroa 34, 133–137.
García-Camacho, R., and Escudero, A. (2008). Reproduction of an early-flowering Mediterranean mountain narrow-endemic (Armeria caespitosa) in a contracting mountain island. Plant Biol. 11, 515–524. doi: 10.1111/j.1438-8677.2008.00151.x
García-Fernández, A., Iriondo, J. M., Bartels, D., and Escudero, A. (2013). Response to artificial drying until drought-induced death in different elevation populations of a high-mountain plant. Plant Biol. 15, 93–100. doi: 10.1111/j.1438-8677.2012.00638.x
Gibson, S. I. (2005). Control of plant development and gene expression by sugar signaling. Curr. Opin. Plant Biol. 8, 93–102. doi: 10.1016/j.pbi.2004.11.003
Giménez-Benavides, L., Escudero, A., and Iriondo, J. (2007). Reproductive limits of a late-flowering high-mountain Mediterranean plant along an elevational climate gradient. New Phytol. 173, 367–382. doi: 10.1111/j.1469-8137.2006.01932.x
Giménez-Benavides, L., García-Camacho, R., Iriondo, J., and Escudero, A. (2011). Selection on flowering time in Mediterranean high-mountain plants under global warming. Evol. Ecol. 25, 777–794. doi: 10.1007/s10682-010-9440-z
Giorgi, F., and Lionello, P. (2008). Climate change projections for the Mediterranean region. Glob. Planet Chang. 63, 90–104. doi: 10.1016/j.gloplacha.2007.09.005
Gutiérrez-Girón, A., and Gavilán, R. G. (2013). Plant functional strategies and environmental constraints in Mediterranean high mountain grasslands in central Spain. Plant Ecol. Diver. 6, 435–446. doi: 10.1080/17550874.2013.783641
Hayat, S., Hayat, Q., Nasser-Alyemeni, M., Wani, A. S., Pichtel, J., and Ahmad, A. (2012). Role of proline under changing environments. Plant Signal Behav. 7, 1456–1466. doi: 10.4161/psb.21949
Herrero, A., and Zamora, R. (2014). Plant responses to extreme climatic events: a field test of resilience capacity at the southern range edge. PLoS One 9:e87842. doi: 10.1371/journal.pone.0087842
Hincha, D., Popova, A., and Cacela, C. (2006). “Effects of sugars on the stability of lipid membranes during drying,” in Advances in Planar Lipid Bilayers and Liposomes, Vol. 3, (Amsterdam: Elsevier), 189–217. doi: 10.1016/s1554-4516(05)03006-1
Hummel, I., Pantin, F., Sulpice, R., Pisques, M., Rolland, G., Dauzat, M., et al. (2010). Arabidopsis plants acclimate to water deficit at low cost through changes of carbon usage: an integrated perspective using growth, metabolite, enzyme and gene expression analysis. Plant Physiol. 154, 357–372. doi: 10.1104/pp.110.157008
Jiménez-Alfaro, B., Gavilán, R. G., Escudero, A., Iriondo, J. M., and Fernández-González, F. (2014). Decline of dry grassland specialists in mediterranean high-mountain communities influenced by recent climate warming. J. Veg. Sci. 25, 1394–1404. doi: 10.1111/jvs.12198
Knaupp, M., Mishra, K. B., Nedbal, L., and Heyer, A. G. (2011). Evidence for a role of raffinose in stabilizing photosystem II during freeze-thaw cycles. Planta 234, 477–486. doi: 10.1007/s00425-011-1413-0
Körner, C. (2003). Alpine Plant Life: Functional Plant Ecology of High Mountain Ecosystems, 2nd Edn, Berlín: Springer.
Krasensky, J., and Jonak, C. (2012). Drought, salt, and temperature stress0induced metabolic rearrangements and regulatory networks. J. Exp. Bot. 63, 1593–1608. doi: 10.1093/jxb/err460
Kreyling, J., Wenigmann, M., Beierkuhnlein, C., and Jentsch, A. (2008). Effects of extreme weather events on plant productivity and tissue die-back are modified by community composition. Ecosystems 11, 752–763.
Larcher, W. (2000). Temperature stress and survival ability of Mediterranean sclerophyllous plants. Plant Biosyst. 134, 279–295. doi: 10.1080/11263500012331350455
Li, N., He, N., Yu, G., Wang, Q., and Sun, J. (2016). Leaf non-structural carbohydrates regulated by plant functional groups and climate: evidences from a tropical to cold-temperate forest transect. Ecol. Indic. 62, 122–131.
Limousin, J. M., Bickford, C. P., Dickman, L. T., Pangle, R. E., Hudson, P. J., Boutz, A. L., et al. (2013). Regulation and acclimation of leaf gas-exchange in a piñon-juniper woodland exposed to three different precipitation regimes. Plant Cell Environ. 36, 1812–1825.
Magaña Ugarte, R., Escudero, A., and Gavilán, R. (2019). Metabolic and physiological responses of Mediterranean high-mountain and Alpine plants to combined abiotic stresses. Physiol. Plant. 165, 403–412.
Magaña Ugarte, R., Escudero, A., Sánchez-Mata, D., and Gavilán, R. (2020). Changes in foliar functional traits of S. pyrenaicus subsp. carpetanus under the ongoing climate change: a retrospective survey. Plants 9:395. doi: 10.3390/plants9030395
Martínez-Vilalta, J., Sala, A., Asensio, D., Galiano, L., Hoch, G., Palacio, S., et al. (2016). Dynamics of non-structural carbohydrates in terrestrial plants: a global synthesis. Ecol. Monogr. 86, 495–516. doi: 10.1002/ecm.1231
McDowell, N., Pockman, W. T., Allen, C. D., Breshears, D. D., Cobb, N., Kolb, T., et al. (2008). Mechanisms of plant survival and mortality during drought: why do some plants survive while others succumb to drought? New Phytol. 178, 719–739. doi: 10.1111/j.1469-8137.2008.02436.x
Meletiou-Christou, M., and Rhizopoulou, S. (2017). Leaf functional traits of four evergreen species growing in Mediterranean environmental conditions. Acta Physiol. Plant. 39:34.
Montesinos-Pereira, D., Barrameda-Medina, Y., Romero, L., Ruiz, J., and Sánchez-Rodríguez, E. (2014). Genotype differences in the metabolism of proline and polyamides under moderate drought in tomato plants. Plant Biol. 16, 1050–1057.
Morvan-Bertrand, A., Boucaud, J., Le Saos, J., and Prud’homme, M. P. (2001). Roles of the fructans from leaf sheaths and from the elongating leaf bases in the regrowth following defoliation of Lolium perenne L. Planta 213, 109–120. doi: 10.1007/s004250000478
O’Brien, M., Leuzinger, S., Philipson, C. D., Tay, J., and Hector, A. (2014). Drought survival of tropical tree seedlings enhanced by non-structural carbohydrate levels. Nat. Clim. Chang. 4, 710–714. doi: 10.1038/nclimate2281
Palacio, S., Maestro, M., and Montserrat-Martí, G. (2007). Seasonal dynamics of non-structural carbohydrates in two species of Mediterranean sub-shrubs with different leaf phenology. Environ. Exp. Bot. 59, 34–42. doi: 10.1016/j.envexpbot.2005.10.003
Patterson, H., and Thompson, R. (1971). Recovery of inter-black information when block sizes are unequal. Biometrika 58, 545–554. doi: 10.1093/biomet/58.3.545
Paul, M. J., Primavesi, L. F., Jhurreea, D., and Zhang, Y. H. (2008). Trehalose metabolism and signaling. Annu. Rev. Plant Biol. 59, 417–441. doi: 10.1146/annurev.arplant.59.032607.092945
Per, T. S., Khan, N. A., Reddy, P. S., Masood, A., Hasanuzzaman, M., Khan, M. I. R., et al. (2017). Approaches in modulating proline metabolism in plants for salt and drought stress tolerance: phytohormohnes, mmineral nutrients and transgenics. Plant Physiol. Biochem. 115, 126–140. doi: 10.1016/j.plaphy.2017.03.018
Pescador, D., Chacón, J., de la Cruz, M., and Escudero, A. (2014). Maintaining distances with the engineer: patterns of coexistence in plant communities beyond the patch-bare dichotomy. New Phytol. 204, 140–148. doi: 10.1111/nph.12899
Pescador, D., Sierra-Almeida, A., Torres, P. J., and Escudero, A. (2016). Summer freezing resistance: a critical factor for plant community assemblies in mediterranean high mountains. Front. Plant Sci. 7:194. doi: 10.3389/fpls.2016.00194
Pescador, D. S., de Bello, F., Valladares, F., and Escudero, A. (2015). Plant trait variation along an altitudinal gradient in Mediterranean high mountain grasslands: controlling the species turnover effect. PLoS One 10:e0118876. doi: 10.1371/journal.pone.0118876
Pinheiro, J., Bates, D., DebRov, S., Sarkar, D., and R Core Team (2018). nmle: Linear and Nonlinear Mixed Effects Models (Version 3.1-137). Available online at: https://CRAN.R-project.org/package=nlme (accessed January 12, 2019).
Piper, F. I., Viñegla, B., Linares, J. C., Camarero, J., Cavieres, L. A., and Fajardo, A. (2016). Mediterranean and temperate treelines are controlled by different environmental drivers. J. Ecol. 104, 691–702. doi: 10.1111/1365-2745.12555
R Core Team (2018). R: A Language and Environment for Statistical Computing (Version 1.1.456). Vienna: R Foundation for Statistical Computing.
Rivas-Martínez, S. (2007). Mapas de series, geoseries y geopermaseries de vegetación de España [Memoria del mapa de vegetacion potencial de Espana]. Parte I Itinera Geobot. 17:436.
Roychoudhury, A., Banerjee, A., and Lahiri, V. (2015). Metabolic and molecular-genetic regulation of proline signaling and its cross-talk with major effectors mediates abiotic stress tolerance in plants. Turk. J. Bot. 39, 887–910. doi: 10.3906/bot-1503-27
Ruiz-Labourdette, D., Génova, M., Schmitz, M. F., Urrutia, R., and Pineda, F. D. (2014). Summer rainfall variability in European Mediterranean mountains from the sixteenth to the twentieth century reconstructed from tree rings. Int. J. Biometeorol. 58, 1627–1639. doi: 10.1007/s00484-013-0766-4
Sala, A., Woodruff, D. R., and Meinzer, F. C. (2012). Carbon dynamics in trees: feast or famine? Tree Physiol. 32, 764–775. doi: 10.1093/treephys/tpr143
Sanfuentes, C., Sierra-Almeida, A., and Cavieres, L. A. (2012). Effect of the increase in temperature in the photosynthesis of a high-andeans species at two elevations. Gayana Bot. 69, 37–45.
Sierra-Almeida, A., Cavieres, L. A., and Bravo, L. A. (2009). Freezing resistance varies within the growing season and with elevation in high-Andean species of central Chile. New Phytol. 182, 461–469. doi: 10.1111/j.1469-8137.2008.02756.x
Sierra-Almeida, A., Reyes-Bahamonde, C., and Cavieres, L. (2016). Drought increases the freezing resistance of high-elevation plants of the Central Chilean Andes. Oecologia 181, 1011–1023. doi: 10.1007/s00442-016-3622-5
Strauss, G., and Hauser, H. (1986). Stabilization of lipid bilayer vesicles by sucrose during freezing. Proc. Natl. Acad. Sci. U.S.A. 83, 2422–2426. doi: 10.1073/pnas.83.8.2422
Taji, T., Ohsumi, C., Iuchi, S., Seki, M., Kasuga, M., Kobayashi, M., et al. (2002). Important roles of drought- and cold-inducible genes for galactinol synthase in stress tolerance in Arabidopsis thaliana. Plant J. 29, 417–426. doi: 10.1046/j.0960-7412.2001.01227.x
Valladares, F., Vilagrosa, A., Peñuelas, J., Ogaya, R., Camarero, J. J., Corcuera, L., et al. (2004). “Estrés hídrico: ecofisiología y escalas de la sequía,” in Ecología del bosque Mediterráneo en un Mundo Cambiante, 2nd Edn, ed. U. Y. Valladares (Madrid: Ministerio de Medio Ambiente, EGRAF), 192.
Valluru, R., and Van den Ende, W. (2008). Plant fructans in stress environments: emerging concepts and future perspectives. J. Exp. Bot. 59, 2905–2916. doi: 10.1093/jxb/ern164
Van den Ende, W. (2013). Multifunctional fructans and raffinose family oligosaccharides. Front. Plant Sci. 4:247. doi: 10.3389/fpls.2013.00247
Van der Heyden, F., and Stock, W. D. (1996). Regrowth of a semiarid shrub following simulated browsing: the role of reserve carbon. Funct. Ecol. 10, 647–653. doi: 10.2307/2390175
Vereyken, I., Albert van Kuik, J., Evers, T., Rijken, P., and de Kruijff, B. (2003). Structural requirements of the fructan-lipid interaction. Biophys. J. 84, 3147–3154. doi: 10.1016/s0006-3495(03)70039-3
Weiss, K., and Alt, M. (2017). Determination of single sugars, including inulin, in plants and feed materials by high-performance liquid chromatography and refraction index detection. Fermentation 3:20.
Wyka, T. (1999). Carbohydrate storage and use in an alpine population of the perennial herb, Oxytropis sericea. Oecologia 120:208.
Keywords: summer drought, proline, climate change, osmotically-active carbohydrates, Mediterranean high-mountains
Citation: Magaña Ugarte R, Escudero A and Gavilán RG (2021) Assessing the Role of Selected Osmolytes in Mediterranean High-Mountain Specialists. Front. Ecol. Evol. 9:576122. doi: 10.3389/fevo.2021.576122
Received: 25 June 2020; Accepted: 13 January 2021;
Published: 28 January 2021.
Edited by:
Angela Sierra-Almeida, University of Concepcion, ChileReviewed by:
Claudia Reyes, University of Concepcion, ChileValentino Casolo, University of Udine, Italy
Copyright © 2021 Magaña Ugarte, Escudero and Gavilán. This is an open-access article distributed under the terms of the Creative Commons Attribution License (CC BY). The use, distribution or reproduction in other forums is permitted, provided the original author(s) and the copyright owner(s) are credited and that the original publication in this journal is cited, in accordance with accepted academic practice. No use, distribution or reproduction is permitted which does not comply with these terms.
*Correspondence: Rosina Magaña Ugarte, rosinamu@gmail.com