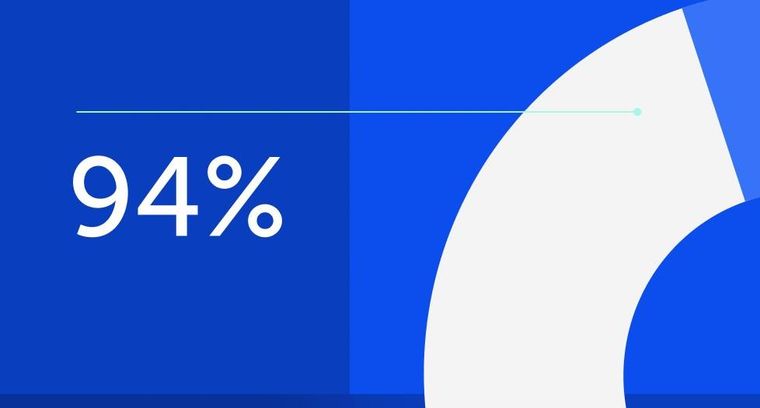
94% of researchers rate our articles as excellent or good
Learn more about the work of our research integrity team to safeguard the quality of each article we publish.
Find out more
ORIGINAL RESEARCH article
Front. Ecol. Evol., 08 June 2021
Sec. Behavioral and Evolutionary Ecology
Volume 9 - 2021 | https://doi.org/10.3389/fevo.2021.569034
This article is part of the Research TopicFactors Affecting Host Selection by Mosquitoes: Implications for the Transmission of Vector-Borne PathogensView all 12 articles
The Asian tiger mosquito Aedes albopictus (Skuse, 1894) is a highly invasive species widely distributed on the Spanish Mediterranean coast and the Balearic archipelago. Most studies involving this species in Spain have been focused on surveillance and control methods. However, micro-evolutionary studies for Ae. albopictus in Spain have been traditionally neglected. Morphological diversity could be the result of long-term evolutionary diversification in responses to selective pressures such as temperature, precipitation, food availability, predation, or competition that may influence flight activity, host-seeking, and blood-feeding behavior. Wing geometric morphometric have been used not only to study micro- and macro-evolution in mosquitoes but also in studies of population structuring and sexual dimorphism. Therefore, the main goal of this study was to investigate the wing shape patterns of Ae. albopictus populations to unveil sexual dimorphism that could provide information about their ecology and behavior. Mosquito eggs were collected using oviposition traps at the main campus of the University of the Balearic Islands (Palma de Mallorca, Spain) and reared under laboratory conditions. In order to study wing shape variation patterns in Ae. albopictus males and females, the left wing of each adult mosquito was removed and analyzed based on 18 landmarks. Our results indicated strong levels of sexual dimorphism between Ae. albopictus males and females. Furthermore, according to the cross-validated reclassification test, males were correctly distinguished from females with an accuracy of 84% and females from males 75%. We observed a significant sexual dimorphism in the wing shape patterns of Ae. albopictus when considering different seasonal patterns (spring vs. autumn). Our results suggested that selective pressures may affect males differently to females. Host-seeking, blood-feeding, and oviposition behavior of females may act as a major driver for wing shape sexual dimorphism. These results should be considered for the development of more effective and targeted mosquito control strategies.
The Asian tiger mosquito Aedes albopictus (Skuse, 1894) is a highly invasive species currently distributed throughout the Mediterranean area including the Balearic archipelago. This mosquito species is native to south-west tropical and subtropical forests in Asia and it has spread through all the continents except Antarctica (Kraemer et al., 2015; Cunze et al., 2016). Due to its ecological plasticity, Ae. albopictus is well adapted to exploit the resources available in urban and peri-urban areas (Bonizzoni et al., 2013).
The introduction of Ae. albopictus to new geographic regions has been facilitated by globalization processes such as transport of commodities and by anthropogenic modifications of natural environments generating suitable breeding sites (Schaffner et al., 2013; Wilke et al., 2020). Worldwide trade of tires and lucky bamboo (Dracaena spp.) have been pointed out as the main pathways for its spreading (Eritja et al., 2017; Sánchez et al., 2017; Wilke et al., 2020) and recent studies have also shown that cars and commercial flights are major drivers in the expansion of Ae. albopictus to new areas (Eritja et al., 2017; Ibañez-Justicia et al., 2017).
Aedes albopictus was first introduced in Europe in Albania in 1979. Since then, it has spread throughout the continent (Gratz, 2004) and it has been recorded in at least 31 European countries (Collantes et al., 2015; Petrić et al., 2018). The most recent recordings include the south of England (Medlock et al., 2017), the north of Portugal (Osório et al., 2018), the region of Bohemia in the Czech Republic (Rettich and Kulma, 2018), and the southern Italian islands of Lampedusa, Linosa and Pantelleria (Di Luca et al., 2017).
In Spain, it was first recorded in Sant Cugat del Vallès in 2004 (Aranda et al., 2006) ten years later Ae. albopictus has spread out along all the east coast of the Iberian Peninsula and the Balearic Islands (Collantes et al., 2015). Nowadays, it can be found in Catalonia, Castellón, Valencia, Alicante, Murcia, Málaga, Andalusia, Basque Country, Extremadura, and the Balearic Islands (Roiz et al., 2008; Bueno-Marí et al., 2009, 2010, 2013, 2014; Delacour-Estrella et al., 2010; Collantes and Delgado, 2011; Miquel et al., 2013; Delacour-estrella et al., 2014; Delacour et al., 2015; Bravo-Barriga et al., 2019). The first detection of Ae. albopictus in the Balearic Islands was in 2012 in Mallorca (Miquel et al., 2013), then in Ibiza in 2014 (Barceló et al., 2015) and in Minorca in 2015 (Bengoa et al., 2016).
Aedes albopictus alongside with Aedes aegypti (L.) is considered the main vector of dengue and chikungunya viruses causing major disease outbreaks in the Southwestern Indian Ocean, India, and Central Africa (Paupy et al., 2009). This species is also a competent vector for other arboviruses such as Zika, yellow fever, and filarial worms (Paupy et al., 2009; Grard et al., 2014; Gutiérrez-López et al., 2019). In Europe, Ae. albopictus is currently responsible for local outbreaks of chikungunya (Rezza et al., 2007) and dengue (Gould et al., 2010; Lazzarini et al., 2020) due to virus introduction by travelers arriving from endemic areas (Emmanouil et al., 2020). Similarly, local transmission cases of the dengue virus were reported from Italy, Croatia, and France since 2010 (La Ruche et al., 2010; Gjenero-Margan et al., 2011; ECDC, 2020). More recently and for the first time, six dengue autochthonous cases were reported in 2018 and one in 2019 in Spain (ECDC, 2019; Monge et al., 2020) in areas where Ae. albopictus is considered the primary vector.
In the Balearics, Ae. albopictus is considered established but increasing its distribution area every year (Tavecchia et al., 2017; European Centre for Disease Prevention and Control and European Food Safety Authority, 2020). This species breeds preferably in small containers, mostly human-made, that are present in urban and peri-urban areas. Aedes albopictus is known for being anthropophilic and a nuisance species. However, despite its current importance as vector and nuisance species, little information is available about the main drivers responsible for modulating its ecology, behavior, and genetics. Consequently, identifying the possible microevolutionary patterns that may affect the behavior of Ae. albopictus (Suesdek, 2019) is crucial. Environmental factors can affect mosquito bionomy traits such as longevity, body size, reproduction, larval development, and fecundity, among others (Chandrasegaran et al., 2020). Those factors vary across the mosquito spatial distribution and may change its behavior related to its flight activity, host-seeking, or feeding frequency (Bara et al., 2015; Carvajal et al., 2016; Chandrasegaran et al., 2020).
Wing geometric morphometrics (WGM) is a practical tool for describing phenotypic variation in organisms representing individuality by the relative position of morphological landmarks that define the shape of the morphological trait studied (Klingenberg, 2010, 2011, 2016; Sánchez et al., 2017; Multini et al., 2019). In insects, the wing is the preferred structure for morphometric analyses due to its bi-dimensional composition reducing digitizing error (Dujardin, 2008).
This methodology has been proven effective to study micro- and macro-evolution in mosquitoes and also in studies of population structuring and sexual dimorphism (Dujardin, 2011; Louise et al., 2015; Virginio et al., 2015; de Oliveira et al., 2016; Wilke et al., 2016; Lorenz et al., 2017; Multini et al., 2019). The population structuring may be affected by exogenous or endogenous pressures that could affect males and females differentially (Carvajal et al., 2016; de Oliveira et al., 2016). Morphological diversity could be the reflection of biological differences such as shape, that result from long-term evolutionary diversification (Zelditch et al., 2012). This dissimilarity in shape would mean different functional roles or responses to selective pressures (Zelditch et al., 2012).
According to Lorenz and Suesdek (2020), the wing is an important structure for sexual signaling and flight, but it is still unknown if variation in wing patterns have some ecological or behavioral role that directly influences the flight or mating. Hence, the study of sexual dimorphism patterns can provide answers to questions about mating behavior, genetic drift, and how populations react to selective pressures, including host-seeking (Chandrasegaran et al., 2020). Comprehensive knowledge of the morphometrical characteristics of the vector may help in describing the population diversity, morpho-ecological traits and could then be useful for success in control campaigns (Jirakanjanakit et al., 2005; Sendaydiego et al., 2013; Chaiphongpachara et al., 2019).
The goal of this study is to investigate the sexual dimorphism based on wing shape variations in Ae. albopictus populations from the Balearic Islands and to explore if Ae. albopictus male and female populations are driven differently by selective pressures. In addition, the study also assessed the wing shape variation between different seasons.
The sampled area was located in the municipality of Palma (Mallorca, Spain), the most urbanized and populated city on the island (Figure 1). Mallorca has a Mediterranean climate represented by mild wet winters and hot dry summers with mean annual precipitation of about 500 mm and a mean annual temperature of 17°C (Gelabert et al., 2003).
Figure 1. Map of the study area. (A) Spain; (B) Balearic Islands; and (C) Campus of the University of the Balearic Islands. The collection area is highlighted in red.
A total of 72 black plastic container ovitraps (Ø 9 cm, h = 15 cm, 950 mL) were placed to collect Ae. albopictus eggs at the main campus in an experimental plot of the University of the Balearic Islands (UTM 31 S 469347.93 m E 4387387.76 m N). This suburban area is characterized by having residential houses, usually provided with gardens and human-made small containers (plant pots, vases, etc.) which are favorable for Ae. albopictus development. Ovitraps were filled with 500 mL of tap water and 2.5 g of hay as an attraction lure. Wood tongue depressors were placed inside ovitraps as oviposition substrate (ECDC, 2012). Thirteen samplings were performed during autumn 2017 and spring - summer 2018, with six samplings between October 2017 and December 2017, and seven between April 2018 and July 2018. From 21st September to 20th December was considered as Autumn, from 21st December to 20th March was Winter, from 21st March to 20th June was Spring and from 21st June to 20th September was Summer.
Collected eggs were hatched in the laboratory under controlled conditions (27 ± 1°C, 70% relative humidity, 12 h photoperiod), flooding them into tap water in containers until the emergence of adults. The emerged adults were maintained ad libitum in 10% sucrose solution, morphologically identified, sexed, and preserved at −20°C when dead.
In addition to ovitraps, five mosquito adults’ samplings were performed using one BG-Sentinel trap (BG-1 Sentinel, Biogents, Germany) baited with BG-lure (Biogents, Germany) and CO2 (2 Kg of dry ice per day). Adult traps were set for 24 h per sampling between 23/10/2017 and 07/11/2017. By conducting a large sampling effort and different collection methods we greatly reduced the probability of collecting sibling specimens. For the analysis, a total of 50 adults from autumn were collected with the BG-Sentinel trap. The remaining mosquitoes (191) were reared from the egg batches collected by the oviposition traps deployed during spring and summer. To further reduce the probability of analyzing sibling specimens, when the sampling sufficiency was reached (more than 30 mosquitoes per population) adult mosquitoes were chosen randomly for the analyses.
A total of 241 left wings, 123 from females and 118 from males emerged in the laboratory and collected from the field in different seasons (Table 1) were dissected and mounted between a slide and a coverslip (without mounting medium) fixing the coverslip with adhesive tape (Vidal et al., 2012; Beriotto et al., 2021). Wings were photographed at 40x magnification using a Zeiss Scope A.1 stereoscopic microscope attached to a camera AxioCamICc1 (Zeiss, Germany) by one of the authors (JLM) to minimize error measurements (Fruciano, 2016). The images were processed using ZEN 2.3 lite program (Blue edition). Eighteen landmarks (LMs) were selected to study the geometric morphometry of Ae. albopictus wings according to Bookstein (1997); Vidal et al. (2011), and Vidal and Suesdek (2012). The coordinates of the 18 landmarks represented by vein intersections (Figure 2) were obtained using tpsDig2 2.30 (Rohlf, 2005; Vidal and Suesdek, 2012; Sendaydiego et al., 2013). These landmarks were selected for being homologous and found in all representatives of the Culicidae family (Lorenz et al., 2017; Beriotto et al., 2021).
Table 1. Season and sampling period, number of samplings, sampling method and number of males and females of Ae. albopictus that were collected for wing geometric morphometrics.
Figure 2. Left wing of Ae. albopictus (female) mounted between a microscope slide and coverslip showing the 18 landmarks selected for the geometric morphometrics analysis.
The wing geometric morphometric analysis was conducted using MorphoJ 1.06d software (Oracle Corporation) that provides a wide range of tests for two- or three-dimensional landmark data (Klingenberg, 2011). Classifiers about sex and season information were generated for each specimen to examine morphometric differences. Firstly, allometric effect of the wing size on the wing shape was estimated using multivariate regression analysis of the Procrustes coordinates on the centroid size with a permutation test of 10,000 randomizations, and, finally, removed from the subsequent analyses (Klingenberg, 2016). Due to the different sample methods, we focused on wing shape variation patterns disregarding size variations, coordinates, and orientation of the landmarks (Klingenberg, 2010; Börstler et al., 2014; Virginio et al., 2015). After that, to extract shape information, landmarks were subjected to the generalized least-squares Procrustes superimposition algorithm aligning by the principal axes in order to standardize the shape of wings and determine the wing shape coordinates (partial warps) for sex (Virginio et al., 2015; Carvajal et al., 2016) and then for season classifiers (Vidal et al., 2012). After that, a covariance matrix was performed to assess the linear relationship between the independent and the dependent variables. Principal Component Analysis (PCA) was carried out using a covariance matrix for all populations. Then, a Canonical Variate Analysis (CVA) with a permutation of 10,000 randomizations was carried out to test the pairwise distances between males and females, and between seasons (Klingenberg, 2010; Carvajal et al., 2016). In the visualization of the CVA, every possible shape corresponds to a shape point in the morphospace and every direction in the morphospace corresponds to a specific shape change (Klingenberg, 2010). Subsequently, thin-plate splines were obtained by the regression analysis of CVA scores against wing shape variation (Bookstein, 1989; Wilke et al., 2016). Each specimen was then reclassified using the cross-validated classification test of the discriminant analysis based on Mahalanobis distances to determine the degree of wing shape dissimilarity between sex and seasons (Vidal et al., 2012; Wilke et al., 2016). Finally, the Mahalanobis distances resulted from the CVA analysis with season classifier were used to group clusters defined by unweighted pair group method with arithmetic mean (UPGMA, Sneath and Sokal, 1973) to assess the similarity of the wing shape patterns (Carvajal et al., 2016). UPGMA was achieved using PAST software.
The allometry test predicted 0.81% (P = 0.0790) of the wing shape variation. The allometric effect was not considered as it only explained a negligible proportion of the variance.
The Procrustes superimposition analysis using the classifier sex resulted in significant differences in the wing shape patterns of males and females. In the wireframe, it was observed that male wings were narrow and lengthened, whereas females were wider and shorter. The CVA showed significant differences in the wing shape patterns between males and females (Figure 3A) and, in the principal-partial warps from the PCA analysis, it was observed that the most variable anatomical landmarks were landmarks 2, 12, 17, and 18 (Figure 3B). The Procrustes distance of discriminant function showed a value of 0.0288 and a Mahalanobis distance of 3.5673 (P < 0.05) (Table 2).
Figure 3. Comparison between male and female specimens. (A) CVA represented in the morphospace to compare the wing shape variation patterns between males (blue) and females (red). X-axis: First canonical variate; Y-axis: Frequency of each wing shape. (B) Wireframe graph from the PCA displaying the mean value of wing shape variation in the male (blue) and female (red) populations.
Table 2. Pairwise cross-validated reclassification rates, Mahalanobis distances and P-value of males and females Ae. albopictus specimens.
Female specimens yielded high values in the cross-validated classification test from the discriminant function, yielding 94.31% of accuracy when compared to male specimens (Table 2). Conversely, males also yielded high cross-validated classification values, resulting in 93.22% accuracy when compared to females (Figure 3 and Table 2).
The CVA considering seasonality showed significant differences in wing shape between seasons of the year for both sexes (Figure 4). Discriminant analysis revealed that the dissimilarity in wing shape separately for males and females over the seasons was statistically significant (P < 0.05) except between these groups: Females-Spring vs. Females-Summer (P = 0.2275), Males-Autumn vs. Males-Spring (P = 0.1542), Males-Autumn vs. Males-Summer (P = 0.2900) and Males- Spring vs. Males-Summer (P = 0.1151). From the 482 total comparisons carried out with the 241 wings, 350 of them resulted in the cross-validated classification values based on Mahalanobis distances yielding scores higher than 70%, indicating significant differences in wing shape (Table 2). In general, Ae. albopictus collected during the summer yielded lower reclassification values (38–79%) suggesting a higher overlap in wing shape patterns between males and females in this specific season.
Figure 4. Comparison of specimens by season. Morphological space produced by CVA of Ae. albopictus males, females and males vs. females collected in autumn 2017 (red), spring 2018 (green), and summer 2018 (blue). Females: Summer-Spring (P = 0.2275), Autumn-Summer (P = 0.0269), Autumn-Spring (P = 0.0002); Males: Summer-Spring (P = 0.1151), Autumn-Summer (P = 0.2900), Autumn-Spring (P = 0.1542); Males vs. Females: Spring (P < 0.001), Summer (P = 0.0006), Autumn (P < 0.001).
Constructed UPGMA tree based on Mahalanobis distances highlighted the segregation between Ae. albopictus sex and seasons, showing a Cophenic correlation of 0.9767 (Figure 5). Male and female groups were segregated into two separate clusters. In males, spring and summer samples were clustered together, whereas Autumn was clustered apart from the others. Results indicate that the Autumn male population has a higher dissimilarity when compared to the other populations. Conversely, Autumn and Spring were clustered together in female population, and summer was clustered apart.
Figure 5. Mahalanobis distances of seasonal mosquito groups (Spring, Summer, and Autumn) represented in an unweighted pair group method with arithmetic mean.
Since the introduction of Ae. albopictus in the Balearic Islands in 2012 (Miquel et al., 2013), it has increased its abundance (Tavecchia et al., 2017) and has become a public health concern in many places of the Mediterranean coast of Spain (Collantes et al., 2015). In Europe, emerging and re-emerging outbreaks of diseases transmitted by mosquito vectors are increasing (Martinet et al., 2019; Emmanouil et al., 2020). For that reason, it is essential to know the bioecology of vector species as well as the preferred breeding sites, host preference, and dispersal capacity among other characteristics.
Our results showed significant wing sexual dimorphism between male and female Ae. albopictus specimens collected either as eggs or adults in a suburban area of Palma city. Male wings were narrower and lengthened, whereas females were wider and shorter. In our study, the highest differences observed in the wireframe were found at the central contraction zones of the wing (LM 2, 10, 17, and 18) most likely due to the robust and mechanical stability of the wings. These wing morphometrics differences could be a consequence of different selective pressures such as sex-specific behaviors (i.e., host-seeking or mating) (Vidal et al., 2012; Chandrasegaran et al., 2020). In fact, shape variation in sexual dimorphism studies is mainly concentrated in the landmarks located between the middle and distal regions of the wing (Lorenz et al., 2017). Similar results of sexual dimorphism based on wing shape using WGM were observed in Ae. aegypti species collected in urban areas in the Philippines (Sendaydiego et al., 2013; Carvajal et al., 2016). Similarly, such sex-specific variation in wing shape patterns has been also confirmed within ten mosquito species belonging to the genus Culex, Aedes, and Anopheles in southeastern Brazil (Virginio et al., 2015).
Furthermore, our results indicated that the wing pattern variation was associated with seasonality, finding that shape overlapping occurred on mosquitoes of both sexes collected in spring. On the contrary, female mosquitoes collected during autumn showed lower levels of wing shape pattern similarity in comparison to summer and spring. Our findings indicate that different conditions (extrinsic or intrinsic) of each season may significantly affect Ae. albopictus wing shape in male and female specimens and possibly other morphological and physiological traits (Virginio et al., 2015). Vidal et al. (2012) and Louise et al. (2015) used the same methodology to demonstrate that short periods of time (less than a year) are enough to result in detectable wing shape changes (Suesdek, 2019).
Our results suggest that microevolutionary changes affected the wing shape patterns of the Ae. albopictus populations analyzed in this study. Wing shape pattern evolution may express flight activity changing behavior, intrinsic to the host-seeking process, host preference, vectorial capacity, and habitat suitability, among others (Morales Vargas et al., 2013; Suesdek, 2019; Chandrasegaran et al., 2020).
The evolution of populations within species and how this process impacts vector biology and epidemiology is not the only consequence of biotic factors (Suesdek, 2019; Chandrasegaran et al., 2020). Abiotic local factors, such as temperature, humidity, landscape, and presence of breeding sites could influence microevolution (Chandrasegaran et al., 2020).
There are several ecological differences between males and females of Culicidae that may explain the difference in wing shape of both sexes (Virginio et al., 2015; Christe et al., 2019). Usually, males remain near breeding sites, feeding on sugar sources, such as flowers, or resting in vegetation waiting for females to mate. In addition, females must seek hosts to obtain blood to perform oogenesis and look for aquatic habitats for oviposition. These ecological selective pressures could be responsible for the morphological variations between sex. Other studies such as de Oliveira Christe et al. (2016) and Christe et al. (2019) also demonstrated that males and females of Aedes fluviatilis (Lutz, 1904) react to selective pressures such as anthropogenic changes in the environment differently. Further, Nasci (1986) studied the wing length between host-seeking and non-host-seeking females of Ae. aegypti and found differences. These results concur with our results, indicating that wing shape patterns are sex-specific and reflect the mechanisms employed by each sex to cope with selective pressures. Landscape heterogeneity was not measured in this study; however, wing shape variations may be attributed to host and habitat availability causing local population changes (Carvajal et al., 2016; Christe et al., 2019). In fact, urbanization can act as a driver of microevolution events that can be observed in the wing shape of Culex quinquefasciatus in São Paulo (Brazil) (Wilke et al., 2017). Multini et al. (2019) studied the population structure of Anopheles cruzii (Dyar and Knab, 1908) populations for 3 years from three locations with different urbanization levels (urban, peri-urban and sylvatic), finding different wing morphometrics patterns between years and collection sites. Their results suggested that urban disturbances may affect mosquito biology that is reflected with a phenotypic change in the wing. In this sense, evidence of habitat-related morphometric variation was found in urban parks in Sao Paulo, Brazil, where De Carvalho et al. (2017) observed heterogeneity in the wing shape of Culex nigripalpus (Theobald, 1901) females. In the case of Ae. albopictus, habitat disturbances may lead to the creation of new breeding sites (mostly human-made) compared to natural breeding sites, allowing the rapid spread of the population increasing the contact between mosquito vectors and human hosts. However, this important evolutionary force remains mostly unexplored (Medlock et al., 2015).
To the best of our knowledge, this is the first geometric morphometric work with Ae. albopictus conducted in Spain. We have observed a significant sexual dimorphism variation in the wing shape patterns of Ae. albopictus. Furthermore, we have detected microevolutionary changes in wing shape between female populations from autumn in comparison to spring and summer. However, its impact on Ae. albopictus ecology and behavior remain unknown. Nevertheless, we can only assume that the morphometric results show us morphological differences between groups being a limitation when compared with the entire population and address functional and control strategies of the species (Richtsmeier et al., 2002). Further research increasing the number of samples per season or even include different populations must be considered to address these functional implications.
The raw data supporting the conclusions of this article will be made available by the authors, without undue reservation.
JL-M designed and performed the experiment. MM designed and directed the experiment. CB assisted with the wing mounting. AB contributed to the design of the figures and the performance of the analysis. JL-M, AB, CB, and MM wrote the manuscript. All authors agreed to be accountable for the content of the work.
The authors declare that the research was conducted in the absence of any commercial or financial relationships that could be construed as a potential conflict of interest.
We would like the acknowledge the students Toni Sureda and Tania Navarro for their contribution to the field and laboratory work. Also, we would like to thank the editor and the reviewers for their time and comments which have greatly improved the manuscript.
Aranda, C., Eritja, R., and Roiz, D. (2006). First record and establishment of the mosquito Aedes albopictus in Spain. Med. Vet. Entomol. 20, 150–152. doi: 10.1111/j.1365-2915.2006.00605.x
Bara, J., Rapti, Z., Cáceres, C. E., and Muturi, E. J. (2015). Effect of larval competition on extrinsic incubation period and vectorial capacity of Aedes albopictus for dengue virus. PLoS One 10:e0126703. doi: 10.1371/journal.pone.0126703
Barceló, C., Bengoa, M., Monerris, M., Molina, R., Delacour-Estrella, S., Lucientes, J., et al. (2015). First record of Aedes albopictus (Skuse, 1894) (Diptera; Culicidae) from Ibiza (Balearic Islands; Spain). J. Eur. Mosq. Control Assoc. 33, 1–4.
Bengoa, M., Delacour-Estrella, S., Barceló, C., Paredes-Esquivel, C., Leza, M., Lucientes, J., et al. (2016). First record of Aedes albopictus (Skuse, 1894) (Diptera. Culicidae) from Minorca (Balearic Islands, Spain). J. Eur. Mosq. Control Assoc. 34, 5–9.
Beriotto, A. C., Garzón, M. J., and Schweigmann, N. (2021). Is There a Minimum Number of Landmarks That Optimizes the Geometric Morphometric Analysis of Mosquito (Diptera, Culicidae) Wings? J. Med. Entomol. 58, 576–587.
Bonizzoni, M., Gasperi, G., Chen, X., and James, A. A. (2013). The invasive mosquito species Aedes albopictus: current knowledge and future perspectives. Trends Parasitol. 29, 460–468. doi: 10.1016/j.pt.2013.07.003
Bookstein, F. L. (1989). Principal warps: thin-plate splines and the decomposition of deformations. IEEE Trans. Pattern Anal. Mach. Intell. 11, 567–585.
Bookstein, F. L. (1997). Morphometric Tools for Landmark Data: Geometry and Biology. Cambridge: Cambridge University Press. doi: 10.1017/CBO9780511573064
Börstler, J., Lühken, R., Rudolf, M., Steinke, S., Melaun, C., Becker, S., et al. (2014). The use of morphometric wing characters to discriminate female Culex pipiens and Culex torrentium. J. Vector Ecol. 39, 204–212.
Bravo-Barriga, D., Gouveia, A. A., Parreira, R., Jiménez-Vidal, D., Pérez-Martín, J. E., Martin-Cuervo, M., et al. (2019). First detections of Aedes albopictus (tiger mosquito) in the region of Extremadura, west of Spain. Gac. Sanit. 33, 299–300.
Bueno-Marí, R., Acosta-Aleixandre, R., and Serna-Mompeán, J. P. (2014). Primeras capturas de Aedes albopictus (Skuse, 1894) (Diptera: Culicidae) en el Área Metropolitana de Valencia. Arquivos Entomoloxicos 12, 103–108.
Bueno-Marí, R., Bernués-Bañeres, A., Muñoz-Rodríguez, M., and Jiménez-Peydró, R. (2013). Primera cita de Aedes albopictus (Skuse, 1894) en la provincia de Valencia (Diptera, Culicidae). Bol. Asoc. Esp. Entomol. 37, 375–378.
Bueno-Marí, R., Chordá-Olmos, F. A., Bernués-Bañeres, A., and Jiménez-Peydró, R. (2009). Aportaciones al conocimiento de los mosquitos. Pirineos 164, 49–68. doi: 10.3989/pirineos.2009.v164.29
Bueno-Marí, R., Chordá-Olmos, F. A., Bernués-Bañeres, A., and Jiménez-Peydró, R. (2010). Detección de Aedes albopictus (Skuse, 1894) en Torrevieja (Alicante, España). Bol. Asoc. Esp. Entomol. 33, 529–532.
Carvajal, T. M., Hernandez, L. F. T., Ho, H. T., Cuenca, M. G., Orantia, B. C., Estrada, C. R., et al. (2016). Spatial analysis of wing geometry in dengue vector mosquito, Aedes aegypti (L.) (diptera: Culicidae), populations in metropolitan Manila, Philippines. J. Vector Borne Dis. 53, 127–135.
Chaiphongpachara, T., Sriwichai, P., Samung, Y., Ruangsittichai, J., Morales Vargas, R. E., Cui, L., et al. (2019). Geometric morphometrics approach towards discrimination of three member species of Maculatus group in Thailand. Acta Trop. 192, 66–74. doi: 10.1016/j.actatropica.2019.01.024
Chandrasegaran, K., Lahondère, C., Escobar, L. E., and Vinauger, C. (2020). Linking mosquito ecology, traits, behavior, and disease transmission. Trends Parasitol. 36, 393–403. doi: 10.1016/j.pt.2020.02.001
Christe, R. O., Marrelli, M. T., Vidal, P. O., Vendrami, D. P., and Wilke, A. B. B. (2019). Assessment of the microgeographic population structure of Aedes fluviatilis (Diptera: Culicidae) using wing geometric morphometrics. Entomol. Gen. 39, 183–191. doi: 10.1127/entomologia/2019/0763
Collantes, F., Delacour, S., Alarcón-Elbal, P. M., Ruiz-Arrondo, I., Delgado, J. A., Torrell-Sorio, A., et al. (2015). Review of ten-years presence of Aedes albopictus in Spain 2004–2014: known distribution and public health concerns. Parasit. Vectors 8:655. doi: 10.1186/s13071-015-1262-y
Collantes, F., and Delgado, J. A. (2011). Primera cita de Aedes (Stegomyia) albopictus (Skuse, 1894) en la Región de Murcia. An. Biol. 33, 99–101.
Cunze, S., Kochmann, J., Koch, L. K., and Klimpel, S. (2016). Aedes albopictus and its environmental limits in Europe. PLoS One 11:e0162116. doi: 10.1371/journal.pone.0162116
De Carvalho, G. C., Vendrami, D. P., Marrelli, M. T., and Wilke, A. B. B. (2017). Wing variation in Culex nigripalpus (Diptera: Culicidae) in urban parks. Parasit. Vectors 10:423. doi: 10.1186/s13071-017-2348-5
de Oliveira, R., Barretto, A., Wilke, B., Oliveira, P., and Toledo, M. (2016). Wing sexual dimorphism in Aedes fluviatilis (Diptera: Culicidae). Infect. Genet. Evol. 45, 434–436. doi: 10.1016/j.meegid.2016.10.007
Delacour, S., Barandika, J. F., Hidalgo-Triana, N., García-Pérez, A. L., Collantes, F., Ruiz-Arrondo, I., et al. (2015). Early detection of tiger mosquito, Aedes albopictus (Skuse, 1894), in the Basque Country (Spain). An. Biol. 37, 25–30. doi: 10.6018/analesbio.37.3
Delacour-Estrella, S., Bravo-Minguet, D., Alarcón-Elbal, P., Bengoa, M., Casanova, A., Melero-Alcibar, R., et al. (2010). Detección de Aedes (Stegomyia) albopictus (Skuse, 1894) (Diptera: Culicidae) en Benicàssim. Primera cita para la provincia de Castellón (España). Bol. Soc. Entomol. Aragonesa 47:440.
Delacour-estrella, S., Collantes, F., Ruiz-Arrondo, I., Alarcón-Elbal, P. M., Delgado, J. A., Eritja, R., et al. (2014). Primera cita de mosquito tigre, Aedes albopictus (Diptera, Culicidae), para Andalucía y primera corroboración de los datos de la aplicación Tigatrapp. An. Biol. 36, 93–96. doi: 10.6018/analesbio.36.16
de Oliveira Christe, R., Wilke, A. B. B., Vidal, P. O., and Marrelli, M. T. (2016). Wing sexual dimorphism in Aedes fluviatilis (Diptera: Culicidae). Infect. Genet. Evol. 45, 434–436.
Di Luca, M., Toma, L., Severini, F., Boccolini, D., D’Avola, S., Todaro, D., et al. (2017). First record of the invasive mosquito species Aedes (Stegomyia) albopictus (Diptera: Culicidae) on the southernmost Mediterranean islands of Italy and Europe. Parasit. Vectors 10:543. doi: 10.1186/s13071-017-2488-7
Dujardin, J. (2011). “Modern morphometrics of medically important insects,” in Genetics and Evolution of Infectious Disease, ed. M. Tibayrenc (Amsterdam: Elsevier), 473–501. doi: 10.1016/B978-0-12-384890-1.00016-9
Dujardin, J. P. (2008). Morphometrics applied to medical entomology. Infect. Genet. Evol. 8, 875–890. doi: 10.1016/j.meegid.2008.07.011
ECDC (2012). Guidelines for the surveillance of invasive mosquitoes in Europe. Euro Surveill. 17:20265. doi: 10.1186/1756-3305-6-209
Emmanouil, M., Evangelidou, M., Papa, A., and Mentis, A. (2020). Importation of dengue, Zika and chikungunya infections in Europe: the current situation in Greece. New Microbes New Infect. 35:100663.
Eritja, R., Palmer, J., Roiz, D., Sanpera-Calbet, I., and Bartumeus, F. (2017). Direct Evidence of Adult Aedes albopictus Dispersal by Car. Sci. Rep. 7:14399. doi: 10.1038/s41598-017-12652-5
European Centre for Disease Prevention and Control and European Food Safety Authority (2020). Mosquito Maps [Internet]. Stockholm: ECDC.
Gelabert, B., Fornós, J. J., and Gómez-Pujol, L. (2003). Geomorphological characteristics and slope processes associated with different basins: Mallorca (Western Mediterranean). Geomorphology 52, 253–267.
Gjenero-Margan, I., Aleraj, B., Krajcar, D., Lesnikar, V., Klobućar, A., Pem-Novosel, I., et al. (2011). Autochthonous dengue fever in Croatia, August- September 2010. Euro Surveill. 16:19805. doi: 10.2807/ese.16.09.19805-en
Gould, E. A., Gallian, P., De Lamballerie, X., and Charrel, R. N. (2010). First cases of autochthonous dengue fever and chikungunya fever in France: From bad dream to reality! Clin. Microbiol. Infect. 16, 1702–1704. doi: 10.1111/j.1469-0691.2010.03386.x
Grard, G., Caron, M., Mombo, I. M., Nkoghe, D., Mboui Ondo, S., Jiolle, D., et al. (2014). Zika Virus in Gabon (Central Africa) - 2007: A new threat from Aedes albopictus? PLoS Negl. Trop. Dis. 8:e2681. doi: 10.1371/journal.pntd.0002681
Gratz, N. G. (2004). Critical review of the vector status of Aedes albopictus. Med. Vet. Entomol. 18, 215–227. doi: 10.1111/j.0269-283X.2004.00513.x
Gutiérrez-López, R., Bialosuknia, S. M., Ciota, A. T., Montalvo, T., Martínez-de la Puente, J., Gangoso, L., et al. (2019). Vector competence of Aedes caspius and Ae. albopictus mosquitoes for Zika virus, Spain. Emerg. Infect. Dis. 25, 346–348.
Ibañez-Justicia, A., Gloria-Soria, A., Den Hartog, W., Dik, M., Jacobs, F., and Stroo, A. (2017). The first detected airline introductions of yellow fever mosquitoes (Aedes aegypti) to Europe, at Schiphol International airport, the Netherlands. Parasit. Vectors 10:603. doi: 10.1186/s13071-017-2555-0
Jirakanjanakit, N., Dujardin, J., and Pathom, N. (2005). Discrimination of Aedes aegypti (Diptera: Culicidae) laboratory lines based on wing geometry. Southeast Asian J. Trop. Med. Public Health 36, 858–861.
Klingenberg, C. P. (2010). Evolution and development of shape: integrating quantitative approaches. Nat. Rev. Genet. 11, 623–635.
Klingenberg, C. P. (2011). MorphoJ: an integrated software package for geometric morphometrics. Mol. Ecol. Resour. 11, 353–357.
Klingenberg, C. P. (2016). Size, shape, and form: concepts of allometry in geometric morphometrics. Dev. Genes Evol. 226, 113–137.
Kraemer, M. U. G., Sinka, M. E., Duda, K. A., Mylne, A. Q. N., Shearer, F. M., Barker, C. M., et al. (2015). The global distribution of the arbovirus vectors Aedes aegypti and Ae. albopictus. eLife 4:e08347. doi: 10.7554/eLife.08347
La Ruche, G., Souarès, Y., Armengaud, A., Peloux-Petiot, F., Delaunay, P., Desprès, P., et al. (2010). First two autochthonous dengue virus infections in metropolitan France, september 2010. Euro Surveill. 15:19676. doi: 10.2807/ese.15.39.19676-en
Lazzarini, L., Barzon, L., Foglia, F., Manfrin, V., Pacenti, M., Pavan, G., et al. (2020). First autochthonous dengue outbreak in Italy, August 2020. Euro Surveill. 25:2001606.
Lorenz, C., Almeida, F., Almeida-Lopes, F., Louise, C., Pereira, S. N., Petersen, V., et al. (2017). Geometric morphometrics in mosquitoes: What has been measured? Infect. Genet. Evol. 54, 205–215. doi: 10.1016/j.meegid.2017.06.029
Lorenz, C., and Suesdek, L. (2020). The use of wing shape for characterising macroevolution in mosquitoes (Diptera: Culicidae). Infect. Genet. Evol. 77:104052. doi: 10.1016/j.meegid.2019.104052
Louise, C., Vidal, P. O., and Suesdek, L. (2015). Microevolution of Aedes aegypti. PLoS One 10:e0137851. doi: 10.1371/journal.pone.0137851
Martinet, J. P., Ferté, H., Failloux, A. B., Schaffner, F., and Depaquit, J. (2019). Mosquitoes of north-western Europe as potential vectors of arboviruses: a review. Viruses 11:1059. doi: 10.3390/v11111059
Medlock, J. M., Hansford, K. M., Versteirt, V., Cull, B., Kampen, H., Fontenille, D., et al. (2015). An entomological review of invasive mosquitoes in Europe. Bull. Entomol. Res. 105, 637–663. doi: 10.1017/S0007485315000103
Medlock, J. M., Vaux, A. G., Cull, B., Schaffner, F., Gillingham, E., Pfluger, V., et al. (2017). Detection of the invasive mosquito species Aedes albopictus in southern England. Lancet Infect. Dis. 17:140. doi: 10.1016/S1473-309930024-5
Miquel, M., del Río, R., Borrás, D., Barceló, C., Esquivel, C. P., Lucientes, J., et al. (2013). First detection of Aedes albopictus (Diptera: Culicidae) in the Balearic Islands (Spain) and assessment of its establishment according to the ECDC guidelines. J. Eur. Mosq. Control Assoc. 31, 8–11.
Monge, S., García-Ortúzar, V., Hernández, B. L., Pérez, M. Á. L., Delacour-Estrella, S., Sánchez-Seco, M. P., et al. (2020). Characterization of the first autochthonous dengue outbreak in Spain (August–September 2018). Acta Trop. 205:105402.
Morales Vargas, R. E., Phumala-Morales, N., Tsunoda, T., Apiwathnasorn, C., and Dujardin, J. P. (2013). The phenetic structure of Aedes albopictus. Infect. Genet. Evol. 13, 242–251. doi: 10.1016/j.meegid.2012.08.008
Multini, L. C., Wilke, A. B. B., and Marrelli, M. T. (2019). Urbanization as a driver for temporal wing-shape variation in Anopheles cruzii (Diptera: Culicidae). Acta Trop. 190, 30–36. doi: 10.1016/j.actatropica.2018.10.009
Nasci, R. S. (1986). The size of emerging and host-seeking Aedes aegypti and the relation of size to blood-feeding success in the field. J. Am. Mosq. Control Assoc. 2, 61–62.
Osório, H. C., Zé-Zé, L., Neto, M., Silva, S., Marques, F., Silva, A. S., et al. (2018). Detection of the invasive mosquito species Aedes (Stegomyia) albopictus (diptera: Culicidae) in Portugal. Int. J. Environ. Res. Public Health 15:820. doi: 10.3390/ijerph15040820
Paupy, C., Delatte, H., Bagny, L., Corbel, V., and Fontenille, D. (2009). Aedes albopictus, an arbovirus vector: from the darkness to the light. Microbes Infect. 11, 1177–1185. doi: 10.1016/j.micinf.2009.05.005
Petrić, M., Lalić, B., Pajović, I., Micev, S., Durdević, V., and Petrić, D. (2018). Expected changes of Montenegrin climate, impact on the establishment and spread of the Asian tiger mosquito (Aedes albopictus), and validation of the model and model-based field sampling. Atmosphere 9:453. doi: 10.3390/atmos9110453
Rettich, F., and Kulma, M. (2018). The invasive mosquito Aedes albopictus (Diptera, culicidae) firstly recorded in bohemia, Czech Republic. Epidemiol. Mikrobiol. Imunol. 67, 32–35.
Rezza, G., Nicoletti, L., Angelini, R., Romi, R., Finarelli, A., Panning, M., et al. (2007). Infection with chikungunya virus in Italy: an outbreak in a temperate region. Lancet 370, 1840–1846. doi: 10.1016/S0140-673661779-6
Richtsmeier, J. T., Burke Deleon, V., and Lele, S. R. (2002). The promise of geometric morphometrics. Am. J. Phys. Anthropol. 119, 63–91.
Rohlf, F. J. (2005). TpsDig, Digitize Landmarks and Outlines. Version 2.0. Stony Brook, NY: Stony Brook University.
Roiz, D., Eritja, R., Molina, R., Melero-Alcibar, R., and Lucientes, J. (2008). Initial distribution assessment of Aedes albopictus (Diptera: Culicidae) in the Barcelona, Spain, area. J. Med. Entomol. 45, 347–352.
Sánchez, E., Castillo, D., and Liria, J. (2017). Pupal shape and size dimorphism in Aedes albopictus (Skuse, 1894) (Diptera: Culicidae). J. Threat. Taxa 9, 10314–10319. doi: 10.11609/jot.3059.9.6.10314-10319
Schaffner, F., Medlock, J. M., and Van Bortel, W. (2013). Public health significance of invasive mosquitoes in Europe. Clin. Microbiol. Infect. 19, 685–692. doi: 10.1111/1469-0691.12189
Sendaydiego, J. P., Torres, M. A. J., and Demayo, C. G. (2013). Describing wing geometry of Aedes aegypti using landmark-based geometric morphometrics. Int. J. Biosci. Biochem. Bioinformatics 3, 379–383.
Sneath, P. H., and Sokal, R. R. (1973). Numerical Taxonomy. The Principles and Practice of Numerical Classification, 1st Edn. San Francisco, CA: W. H. Freeman.
Suesdek, L. (2019). Microevolution of medically important mosquitoes–A review. Acta Trop. 191, 162–171.
Tavecchia, G., Miranda, M. A., Borrás, D., Bengoa, M., Barceló, C., Paredes-Esquivel, C., et al. (2017). Modelling the range expansion of the Tiger mosquito in a Mediterranean Island accounting for imperfect detection. Front. Zool. 14:39. doi: 10.1186/s12983-017-0217-x
Vidal, P. O., Carvalho, E., and Suesdek, L. (2012). Temporal variation of wing geometry in Aedes albopictus. Mem. Inst. Oswaldo Cruz 107, 1030–1034. doi: 10.1590/S0074-02762012000800011
Vidal, P. O., Peruzin, M. C., and Suesdek, L. (2011). Wing diagnostic characters for Culex quinquefasciatus and Culex nigripalpus (Diptera, Culicidae). Rev. Bras. Entomol. 55, 134–137. doi: 10.1590/S0085-56262011000100022
Vidal, P. O., and Suesdek, L. (2012). Comparison of wing geometry data and genetic data for assessing the population structure of Aedes aegypti. Infect. Genet. Evol. 12, 591–596. doi: 10.1016/j.meegid.2011.11.013
Virginio, F., Oliveira Vidal, P., and Suesdek, L. (2015). Wing sexual dimorphism of pathogen-vector culicids. Parasit. Vectors 8:159. doi: 10.1186/s13071-015-0769-6
Wilke, A. B. B., Benelli, G., and Beier, J. C. (2020). Beyond frontiers: On invasive alien mosquito species in America and Europe. PLoS Negl. Trop. Dis. 14:e0007864. doi: 10.1371/journal.pntd.0007864
Wilke, A. B. B., de Carvalho, G. C., and Marrelli, M. T. (2017). Microgeographic Population Structuring of Culex quinquefasciatus (Diptera: Culicidae) From São Paulo, Brazil. J. Med. Entomol. 54, 1582–1588. doi: 10.1093/jme/tjx149
Wilke, A. B. B., De Oliveira Christe, R., Multini, L. C., Vidal, P. O., Wilk-Da-silva, R., De Carvalho, G. C., et al. (2016). Morphometric wing characters as a tool for mosquito identification. PLoS One 11:e0161643. doi: 10.1371/journal.pone.0161643
Keywords: Aedes albopictus, sexual dimorphism, wing shape, host-seeking, mosquito
Citation: López-Mercadal J, Barretto Bruno Wilke A, Barceló C and Miranda MA (2021) Evidence of Wing Shape Sexual Dimorphism in Aedes (Stegomyia) albopictus in Mallorca, Spain. Front. Ecol. Evol. 9:569034. doi: 10.3389/fevo.2021.569034
Received: 02 June 2020; Accepted: 14 May 2021;
Published: 08 June 2021.
Edited by:
Jenny C. Dunn, University of Lincoln, United KingdomReviewed by:
Flávia Virginio, Butantan Institute, BrazilCopyright © 2021 López-Mercadal, Barretto Bruno Wilke, Barceló and Miranda. This is an open-access article distributed under the terms of the Creative Commons Attribution License (CC BY). The use, distribution or reproduction in other forums is permitted, provided the original author(s) and the copyright owner(s) are credited and that the original publication in this journal is cited, in accordance with accepted academic practice. No use, distribution or reproduction is permitted which does not comply with these terms.
*Correspondence: Júlia López-Mercadal, anVsaWEubG9wZXpAdWliLmVz
Disclaimer: All claims expressed in this article are solely those of the authors and do not necessarily represent those of their affiliated organizations, or those of the publisher, the editors and the reviewers. Any product that may be evaluated in this article or claim that may be made by its manufacturer is not guaranteed or endorsed by the publisher.
Research integrity at Frontiers
Learn more about the work of our research integrity team to safeguard the quality of each article we publish.