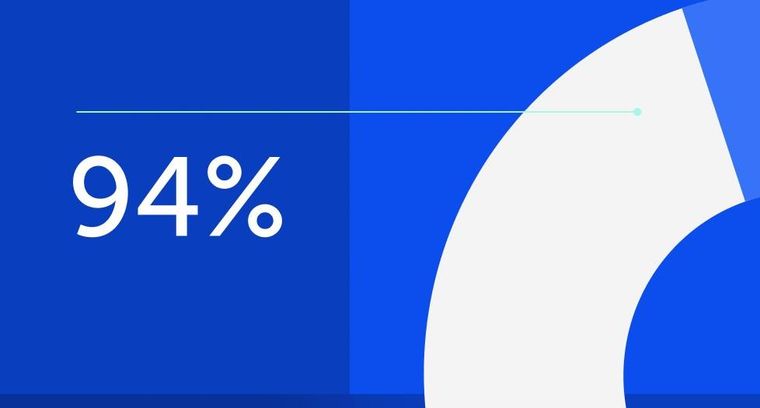
94% of researchers rate our articles as excellent or good
Learn more about the work of our research integrity team to safeguard the quality of each article we publish.
Find out more
ORIGINAL RESEARCH article
Front. Ecol. Evol., 05 March 2021
Sec. Biogeography and Macroecology
Volume 9 - 2021 | https://doi.org/10.3389/fevo.2021.556821
This article is part of the Research TopicAdaptation of Invasive Species to Islands and the Puerto Rican Honey BeeView all 14 articles
Mangifera indica is a widespread economically important tropical fruit. An ongoing study at the Juana Diaz Experimental Station in Puerto Rico aims to understand the factors that influence local pollination success and fruit yields in four fields each hosting a different mango cultivar (Keitt, Kent, Tommy Atkins, and Julie) at different temporal scales. Here we describe the results of insect collection campaigns that evaluated flower visitor communities of these fields (from January to April) in the seasons of 2017 (before Hurricane Maria), 2018 (after Hurricane Maria) and 2019 (2 years after Hurricane Maria). We expected a reduction in diversity, abundance and yields and even changes in composition following the hurricane events of 2017. Over the 3 years, plants were visited by a combined total of 50 insect species, mostly Diptera (also the most abundant), Hymenoptera, Coleoptera, and Lepidoptera. The relative abundances of insect communities changed but overall species richness of insect communities appeared to be recovering by 2019. A clear decline in overall crop yields for two of the four fields (hosting Kent and Tommy Atkins) was seen in 2018 but then recovered in one and surpassed pre-hurricane levels in another in 2019. Mango trees experienced an increase in the abundance for all insect groups in 2019 following the 2018 decline and only one field (hosting Kent) experienced significant species richness declines in 2018. Two of the most dominant insects, Palpada vinetorum (Diptera) and Apis mellifera (Hymenoptera), showed a “reduction-recovery” pattern for the period of 2018–2019 but not so for Cochliomyia minina which was very abundant in 2018 in three out of four cultivars but then returned to pre-hurricane levels in 2019. In 2017, the trees exposed to higher richness and abundance of species experienced higher yields regardless of cultivars but these relationships when present were often weaker in 2018 (post-hurricane) and 2019 and not all cultivars were equally successful at attracting the same levels of diversity and abundance of insects. Our results do support the importance of pollinator diversity and abundance to improve agricultural yields. They also emphasize that within the context of future extreme atmospheric events, that there needs to be an understanding of not only how these pollinator communities may recover from these events but also of how individual pollinators (vs. other factors) may influence plant yields to develop informed management strategies following such events.
Observed declining trends in the diversity and abundance of pollinators, may threaten global economies and our capacity to meet increasing global food demands (Potts et al., 2010a, b; Cameron et al., 2011; Bartomeus et al., 2013; Jevanandam et al., 2013; Aizen et al., 2019). A significant portion of the world’s crop production (35%) depends on animal pollination (Klein and Bernard, 2007) and many of those are dependent on insect pollinator (Rader et al., 2013). In the United States alone, the value of this “pollination service” to the agriculture has been estimated at $43B (Losey and Vaughan, 2006). While managed pollinators like honey bees are main contributors of worldwide agricultural production (Potts et al., 2010b), studies suggest that wild pollinators are also important as they can increase agricultural yield and quality of fruit crops (e.g., Garibaldi et al., 2013; Garratt et al., 2014; Rader et al., 2016). Given the importance that pollination services represent to food and economic security, calls for strategies to conserve, manage and monitor pollinator abundance and biodiversity have been on the rise to reduce potential risks (Intergovernmental Science-Policy Platform on Biodiversity and Ecosystem Services [IPBES], 2016). A core element of the International Pollinator Initiative’s 2013–2018 action plan led by the Food Agriculture Organization (FAO) and the Convention for Biological Diversity (CBD) centers around activities related to monitoring research and assessment on the status and trends of pollinators and pollination (Convention on Biological Diversity, 2018). Information on the diversity and abundance of pollinator species is an important step toward understanding the stability and resilience of crop pollination services (Rader et al., 2013; Rogers et al., 2013). Developing monitoring strategies will be critical when evaluating pollinator management strategies especially in the face of climate variability and the prospect of increases in extreme atmospheric events world-wide.
Predicting the effects of extreme atmospheric events such as hurricanes on the abundance and diversity of insect pollinators is complex. Indeed the literature offers no agreement about the effects of hurricanes on insect communities with some species increasing while others decreased in abundances following these events (Torres, 1992; McGinley and Willig, 1999; Schowalter and Ganio, 1999; Koptur et al., 2002; Gandhi et al., 2007; Spiller et al., 2016). Some responses are modulated by spatial differences in microhabitat (e.g., Schowalter et al., 2017) and others by species interactions within and between trophic levels (e.g., Schowalter, 2012; Novais et al., 2018). From the perspective of diversity changes, it has been argued that while extreme weather events (hurricanes and frosts) often result in immediate reductions of alpha diversity, insect assemblages tend to be fairly resilient returning to pre-disturbance levels within 1–5 years (Marquis et al., 2019; Chen et al., 2020). It should be noted that most studies evaluating the effects of these events on insect communities do not often include pollinators.
Studies addressing the dynamics of insect pollinators following hurricane events have a narrow geographic scope and most are focused on non-agricultural systems. One study explicitly evaluated changes in insect pollinator assemblages in Florida (United States) after hurricanes Frances and Jeanne and showed a decline in species diversity accompanied by a reduction in the reproductive success of Laguncularia racemosa (Landry, 2013). In a second study, the insect pollinator community visiting Ardisia escallonioides following Hurricane Andrew in 1998, changed little in composition and abundance of their generalist pollinators (Pascarella, 1998). A third study, also in Florida, showed that after hurricane Andrew populations of Pegoscapus jimenizeii (fig wasps), a specialized pollinator of Ficus aurea, dropped but were able to recover in only 5 months (Bronstein and Hossaert-McKey, 1995). On the other hand, hurricanes across Yucatan, México, are one of several factors contributing to the decline of feral and managed colonies of stingless bees with generalist species persisting even when some of their food species disappear (Villanueva-Gutierrez et al., 2005; Roubik and Villanueva-Gutierrez, 2009). Clearly more studies are needed to evaluate the potential role of these events on pollinator communities and how these may affect plant reproduction. This would be particularly important for agricultural systems when their production is dependent on animal pollination.
Mangifera indica (mango), is native to Asia but has been widely dispersed in tropical and subtropical areas around the world (Jiron and Hedström, 1985; Galán, 2009). It is an economically important crop worldwide with over 1,000 cultivars (Galán, 2009). The Asian continent is the principal producer (75%) for this crop plant followed by Latin America and the Caribbean region (14%) and Africa (10%) (FAOSTAT, 2000). The scientific literature suggests that flower visitors of M. indica are quite diverse taxonomically and that there is considerable variation among geographic regions. For example, studies in Taiwan and Australia reported that Hymenoptera were the only flower visitors and potential pollinators of M. indica (Anderson et al., 1982; Hsin Sung et al., 2006) whereas in India, Diptera were named as dominant flower visitors (Singh, 1988; Ramírez and Davenport, 2016). Meanwhile, studies in Israel and Africa, showed that the main visitors of mango plants were a mixture of both Hymenoptera and Diptera species (Dag and Gazit, 2000) or Hymenoptera, Diptera, and Coleoptera (Carvalheiro et al., 2010, 2012) while in some areas, Apis bees were important pollinators (Wongsiri and Chen, 1995). The combined data suggest that M. indica flowers can attract a large diversity of floral visitors and therefore has a pollination system that may be generalized (Anderson et al., 1982; De Siqueira et al., 2008; Fajardo et al., 2008; Corredor and García, 2011; Kumar et al., 2012). While generalized pollination systems may offer resilience capacity within the context of environmental change (Waser et al., 1996; González-Varo et al., 2013), this may not always be the case (Memmott et al., 2007) or may not occur at a speed that is necessary in economically important species.
In the Caribbean region, the island of Puerto Rico is among the principal producers of M. indica (Central America Data, 2016) and Puerto Rico’s climate is changing. Since 1950, air temperatures have increased by 2°C as well as the frequency and intensity of extreme climatic events (e.g., heat waves, droughts, and tropical storms) (Gould et al., 2015). Indeed, tropical storms and hurricanes have become more common and more intense during the past two decades (Environmental Protection Agency [EPA], 2016) and this trend is expected to continue according to most climate models (see reviews by Gould et al., 2015; Fain et al., 2018). Following hurricane events, flower resources available to pollinators may be reduced through the direct impact to plants (e.g., via mortality or defoliation; Rathcke, 2000) for pollinating insects. Hurricane-driven ecosystem modification may also reduce the availability of suitable habitat (e.g., flooding of ground nesting sites for insects, Savage et al., 2018) and result in declines in pollinating fauna (Landry, 2013). Therefore, both mango trees and their pollinator communities can be affected by the current trends in atmospheric events and these could affect local yields in Puerto Rico. Detecting declines in pollinator abundances and reproductive success of M. indica as a result of these events has yet to be documented in hurricane prone areas. On the other hand, predictions about the effects of hurricanes effects on plant-pollinator interactions are not necessarily straightforward as the impacts on pollinator abundances by these phenomena have not been documented frequently and only for a limited number of taxa.
In 2017, hurricanes Irma (Category 1 on land) and Maria (Category 4 on land) hit the island of Puerto Rico leading to considerable changes in the island’s green landscape and changes in ambient temperature. It was estimated that when Hurricane Maria hit Puerto Rico on 20 September 2017, 23–31 million trees island-wide were severely damaged or killed (Feng et al., 2018) with some areas in Puerto Rico reporting ambient temperature increases of up to 4°C for a full year (U.S. Forest Service, 2018). Such stresses could reduce the ability of plants to produce fruits through a reduction of photosynthetic resources available to produce flowers and fruits and through a reduction of their pollinator communities (Rathcke, 2000). Following the 2017 hurricane season, we took advantage of an ongoing monitoring study of the flower visitors of four cultivars of M. indica on the island of Puerto Rico to address the potential influence of these hurricane events on the flower visitor communities of M. indica cultivars and how hurricane-influenced changes in these communities related to plant reproductive success. We specifically asked if insect communities visiting mango flowers varied significantly across years and how these changes related to plant reproductive success. One hypothesis is that when they occur, hurricanes could lead to a reduced diversity and abundance of local M. indica pollinators and result in reduced yields. At the same time, a current paradigm is that pollinator diversity and not just abundance may help increase pollinator services and plant reproductive success (Gómez et al., 2007; Albrecht et al., 2012). Thus, a minor goal was to evaluate the functional relationships between reproduction and pollinator diversity and abundance. We also sought to determine how these relationships may change following hurricane events and whether they differed among four fields each of which hosted a different mango cultivar. Lastly, we examined the relative importance of Apis mellifera to mango reproduction. Apis mellifera is often seen as a replacement for local species but managed A. mellifera failed to improve yields for M. indica in Africa (Carvalheiro et al., 2010). Furthermore, we know little on how A. mellifera responds to climatic events in the Caribbean Region. Results of this study provide insights into the dynamics of insect communities visiting flowers of M. indicia within the context of extreme weather events (i.e., hurricanes). From a management perspective, our results may help inform proposed strategies for managed pollination following such events.
We conducted our study at the Juana Díaz Agricultural Experiment Station (JDAES) in the island of Puerto Rico (18° 01′N, 66° 31′W) (Figure 1). The Station covers 111.23 ha and was established in 1950 by the University of Puerto Rico, Mayagüez Campus. It is located in the Southern side of the island which lies within a subtropical dry forest life zone (Ewel and Whitmore, 1973). The site has an average monthly temperature that ranges from 22 to 33°C and an annual rainfall of 977.1 mm (Harmsen et al., 2014). According to the USDA Survey, JDAES soils are classified as “Prime” with irrigation systems that are primarily driven by extracting groundwater (Beinroth et al., 2003). Since 1968, the Station has maintained a mango germplasm which today has 84 cultivars that occupy approximately 14.16 ha (Figure 1). Data was collected in four fields within the germplasm area and each field hosted a different cultivar (see below). For simplicity, each field is named after the cultivar it hosted.
Figure 1. (A) Layout of the Juana Díaz Agricultural Experimental Station indicating the location of the mango germplasm and the four fields [Julie (J), Keitt (Ki), Kent (Ke), Tommy Atkins (T)] used in this study. (B) Palpada vinetorum visiting inflorescences of the Julie fields. (C) Location of the Agricultural Experiment Station Juana Díaz, PR. Basemap was obtained from Google Earth Pro, (November 27, 2020) Juana Diaz, Puerto Rico. 18°01’37 73”N, 66° 31’ 23 56” W Maxar Technologies, https://earth.google.com (February 2, 2021).
Mangifera indica L. (Anacardiaceae) is a perennial tree that produces highly branched inflorescences (Coetzer et al., 1995; Goguey, 1997) with flowers that range from 5 to 10 mm in diameter that carry five green sepals and five petals with yellow nectary discs and colors ranging from white to yellow, pink or red (Galán, 2009; Figure 1). Inflorescences carry both hermaphroditic and male flowers. In hermaphroditic flowers the ovary is globose with a single stigma (Ramirez and Davenport, 2010). The Keitt cultivar evaluated in this study is derived from the Mulgoba cultivar, which has a moderate to tall tree height (9.1–40 m), an open canopy, and fruit sizes ranging from 13 to 15 cm (Campbell, 1992). This is the most planted cultivar on the southside of the island. The Kent cultivar evaluated here was derived from the Brooks cultivar and can reach heights of 40 m, has a foliage that looks more compact, and produces fruit sizes ranging from 11 to 13 cm that are a favorite in Latin America (Campbell, 1992). The Tommy Atkins cultivar was derived from the Haden cultivar and produces a dense, rounded top tree with a fruit that can measure between 12 and 14.5 cm and is often preferred for its fruit color (skin orange-yellow, crimson, or dark red blush color) for export (Campbell, 1992). Julie is the smallest of the four cultivars studied. It grows only up to 3.3 m tall and produces smaller fruits relative to the remaining three cultivars. This is the main mango exported from the West Indies to Europe, believed to have been imported from Jamaica to other countries in the Caribbean and a favorite throughout the Caribbean region (Morton, 1987). For any of these cultivars, flowering has historically occurred within the course of 5–6 weeks during the dry months, which in Puerto Rico occurs between the months of January and April although some variation of the onset of flowering may occur depending on the year.
We collected insects visiting the flowers of 10 plants of each of the four M. indica fields (N = 40 plants) three days a week during their annual flowering cycles. In the 2017, and 2018 seasons, flowering occurred from January to April whereas for the 2019 season, flowering started early and ran from the last week of December and continued until April. Trees in the general mango germplasm were planted in rows with a 7 m minimum distance from one planted tree to another and a total area surveyed per cultivar of 0.70 ha. Likewise, cultivars varied in their distance from each other: 64.7 m between the Keitt and Kent cultivars, 477.0 m between Keitt and Tommy Atkins and 150.4 m between Kent and Julie (Figure 1). For each tree in a cultivar, we selected five inflorescences to be the focus of the insect collections (50 inflorescences/cultivar). Inflorescences on a tree were observed for insect visitors that were seen performing legitimate visitations (i.e., when the corolla was open and the visitor was collecting resources (nectar or pollen from it). Once detected, insects were collected by sweeping an entomological net over the inflorescence. Observations and collections were done three days every week: Monday, Wednesday and Friday. Each day, collection was carried out between 09:00 h and 14:00 h following a systematic scheme. The day was divided into 1-h periods with four 15-min intervals each assigned to a given cultivar. Within each 15-min interval, inflorescences for all trees were observed (1.5 min/tree) and insects visiting flowers in legitimate ways were collected in separate bags recording the time and the tree ID on each bag. At each hour period, the order of cultivars was rotated, and the order of trees within a cultivar as well to make sure that cultivars and their respective trees were observed at different daytime periods within the flowering season. All collected insects were taken to the Laboratory to be mounted or preserved in 75% alcohol. All specimens were identified to species except for one which was identified to genus. For each observed tree we also recorded the number of inflorescences per tree during the flowering peak and collected and weighed fruits to generate a value for the number of fruits per tree and fruit yield (kg).
For each field (Julie, Keitt, Kent, Tommy Atkins), an insect data matrix was constructed with the variables year, field, collection week (1, 2, 3, etc.), pooled insect abundance for each M. indica field. This abundance matrix was then analyzed with non-metric multidimensional scaling (NMDS) analysis and time vector overlays (using year as the time variable) (Clarke, 1993; McCune and Grace, 2002) to evaluate potential differences in flower visitor communities among fields and across time periods. This analysis was carried out in PC-ORD version 5.0 (McCune and Mefford, 2006) using the Bray–Curtis similarity index and performing 250 iterations with randomized data to select a dimensionality, and then performing one iteration with the chosen dimensionality to find a stable solution with minimal stress (McCune and Grace, 2002). To explore which species best explained the variance among insect communities in multivariate space, we used Kendall correlation analyses that specifically tested associations between species abundance and the first and second NMDS axes (McCune and Grace, 2002). For these analyses we considered species abundances with a Kendall correlation coefficients of (r) ≥ 0.4 to be significantly correlated with the NMDS multivariate axes and later used the three most dominant ones (and the most consistent in activity across cultivars) to evaluate their association with plant reproductive success (see below). For the purpose of this work, we provide results on total insect species diversity (hereafter richness) and abundance as well as abundances for the three most dominant species as these were the most consistent visitors for all four fields.
Our intent was to evaluate differences in total insect abundance, species richness as well as the abundance of dominant species between years and fields and how these variables related to mango reproduction. We first tested for the presence of systematic spatial variation of variables which could influence statistical results when using ordinary least square methods (Dormann et al., 2007). To that effect, we did exploratory analyses of Moran’s I values for all numeric variables in GeoDa (Anselin et al., 2006) first pooling data from all cultivars to check for spatial autocorrelation issues at a landscape level. We then used values for each individual cultivar to test for autocorrelation at a local scale. Moran’s I analyses did reflect spatial autocorrelation issues at the landscape scale but not at local scale (i.e., within a cultivar). Therefore, first we performed “permutational multivariate analysis of variance” (PERMAVOVA) using the Bray-Curtis dissimilarity index to test for multivariate dissimilarities integrating variables related to pollinator species richness and abundance (insect species richness, abundance, and individual abundances of dominant insect species) with year, field and their interaction as main effects. Unlike a regular MANOVA, PERMANOVA is not dependent on the correlation structure among groups (Anderson, 2014). To visualize relationships based on dissimilarity, we generated a dendrogram from a cluster analysis based on the unweighted pair group method with arithmetic mean (UPGMA) and the Bray Curtis dissimilarity index using MSVP v3.22 (Kovach, 2007). We followed PERMANOVA with a series of complementary analyses to evaluate changes in insect community variables and plant reproduction and the relationship among these at the field scale to circumvent spatial autocorrelation issues and using ln transformed variable values to meet the requirement of parametric tests. To evaluate the influence of insect abundance and diversity and the number of inflorescences per tree on the number of fruits/tree and total yield (kg/tree) of each mango field each year, we used generalized linear models to evaluate how the data fitted the models under different distributions (poisson, normal, exponential and negative binomial) and using the lowest AICc value (an adjusted AIC to account for small sample sizes, Cavanaugh, 1997) as our selection criteria for the best model and using a 2 unit minimum criterion for model selection (Burnham and Anderson, 2002). For models under a normal distribution (all but two), ordinary least square models were also run to generate R2 estimates. For each field, we ran repeated measures ANOVAs to test for univariate differences across years in total insect species richness and abundance, fruit yield and the number of inflorescences as well as pairwise Pearson correlation analyses to test for associations between fruit yield with the number of fruits and also between the number inflorescences with fruit yield. We also ran multiple correlation analyses to test for associations between the abundance of each of the three dominant insect species observed for each tree and fruits yield per tree for each field and each year. To account for a potential increase in type II errors from multiple analyses (N = 28 correlations), p-values were corrected using Bonferroni corrections (Hammer et al., 2001). GLM Analyses were run in JMP v. 13.1 (SAS Cary Institute Inc, 2019) while PERMANOVA, Repeated measures ANOVAs and correlations were run in in InfoStat (Di Rienzo et al., 2018).
A grand total of 3,795 individuals distributed across 50 insect species were collected in four mango cultivars (distributed across four fields) across the 3 years of the study (2017: N = 920; 2018: N = 1,318; 2019: N = 1,557). NMS analyses showed that 94.3% of the temporal variation in the insect community composition was explained by a two-dimensional solution with most of the variation (70.4%) explained by Axis 1 (Figure 2). The NMS analysis showed that observations for different the different fields (Julie, Keitt, Kent and Tommy) based on species similarity tended to form clusters around time periods and not around the fields themselves. That is, within a given year observations for the species composition of flower visitors for the different fields were more similar to each other than what the observations for a given field were across the different years (Figure 2A). Also, insect communities in 2017 (before the hurricane events) and 2019 (2 years later) were more similar to each other than what they were to insect communities for all four fields in 2018, 4–6 months after Hurricane Maria hit the island. Kendall correlation analyses showed that the species that most related to the observed variation in the community composition of flower visitors were Palpada albifrons (Diptera: Syrphidae), Palpada vinetorum (Diptera: Syrphidae), Apis mellifera (Hymenoptera: Apidae), Cochliomyia minima (Diptera: Calliphoridae), Psedodorus clavatus (Diptera: Syrphidae), Gonia crassicornis (Diptera: Tachinidae), and Peckia sp. (Diptera: Tachinidae) (Figure 2B and Table 1). Of those, the three most abundant were Palpada vinetorum, Apis mellifera, and Cochliomyia minima (mean observations/field ≥ 7.4 individuals/field/tree). Overall, the abundances for P. vinetorum and A. mellifera were considerably lower in 2018 but that was not the case for Cochliomyia minima which became the dominant species with observed abundances that were six times higher than those observed in 2017 (Figure 3). In 2019, abundance values for Cochliomyia minima had reduced to pre-hurricane levels but those of A. mellifera were significantly lower than abundance values in 2017 (Figure 3). In contrast, following the decline in 2018, P. vinetorum experienced an overall increase in abundance in 2019 and became, once again, the most dominant species by that year. When considering the abundance of dominant species within individual fields, P. vinetorum and A. mellifera exhibited significant declines in 2018 in all fields but returned to pre-hurricane levels in all fields with the exception of Kent field (Figure 3 and Table 2). In that field, the abundance for P. vinetorum increased in 2019 but went above pre-hurricane levels and the abundance for A. mellifera did not recover in 2019 (Figure 3 and Table 2). In contrast, the abundance of C. minima increased for Keitt, Kent and Tommy Atkins fields in 2018 returning to pre-hurricane levels in 2019 with the Julie field experiencing a decline-recovery pattern between 2018 and 2019 (Figure 3 and Table 2).
Figure 2. Non-parametric multidimensional scaling analysis (NMS) based on Bray-Curtis similarity index evaluating differences in insect species composition among years and fields (Julie, Keitt, Kent, Tommy Atkins). (A) Observation groupings based on within year similarities. (B) Correlations lines showing the strongest associations between insect species and multivariate axes based on insect species abundance. See Table 2 for insect acronyms.
Table 1. Kendall correlation coefficients (r) with ordination axes resulting from Non-metric Multidimensional Scaling (NMDS) analyses for seven species of the communities on four mango fields each containing a different cultivar (Julie, Keitt, Kent, Tommy Atkins).
Figure 3. Boxplots illustrating differences in insect abundances per field (Julie, Keitt, Kent, Tommy Atkins) per year for the three most dominant flower visitors. (A) Palpada vinetorum, (B) Apis mellifera, (C) Cochliomyia minima. Repeated Measures analyses performed for each year on each field detected significant differences across years all insect species (Table 2). Different letters within a field indicate significant differences among years.
Table 2. Repeated measures ANOVA analyses testing for annual differences in the average abundance (number of insects observed/tree/day) of dominant insect species for each four fields of M. indica each containing a different cultivar.
PERMANOVA detected significant multivariate dissimilarities defined by the total insect diversity and abundance and the abundance of P. vinetorum, A. mellifera, and C. minima explained by differences across years, fields, and their interaction. Fluctuations in species richness and abundance were not consistent across fields. A dendrogram following a cluster analysis using the same variables as in PERMANOVA showed fields within a year indeed clustered together but that relationships among fields within a year were not consistent based on their similarity in species richness, total insect abundance and the abundance of dominant species (Figure 4). Repeated measures ANOVA results showed significant differences across years in insect species diversity (i.e., richness) and abundance in only two out of four fields (Kent and Tommy Atkins) but the direction of changes was different for each (Figures 5A,B and Table 3). On average, values for species richness were lower in 2018 for the Kent area and these returned to pre-hurricane levels by 2019 while in the Tommy Atkin area, trees experienced annual incremental increases in species richness and an increase in insect abundance in 2018 that remained in 2019. The Julie and Keitt fields did not experience significant changes in neither richness nor abundance across years (Figures 5A,B and Table 3). The variance in species richness also seemed to increase for all fields in 2018 and also remained elevated in 2019 for the Julie, Keitt, and Tommy fields but not for the Kent (Figure 5B).
Figure 4. Dendogram depicting multivariate clusters using Bray Curtis dissimilarity index based on total insect species richness, total abundance, and abundances of each of the three dominant insect pollinators of M. indica (Palpada vinetorum, Apis mellifera, Cochliomyia minima) recorded for four fields (Julie, Keitt, Kent, Tommy Atkins) in 2017, 2018, and 2019 at the Juana Diaz Experimental Station in Puerto Rico.
Figure 5. Boxplots illustrating differences in variables related to the state of pollinator communities experienced by trees (insect richness and abundance) and variables related to tree reproductive effort (number of inflorescences and fruit yield) in M. indica in 2017, 2018, and 2019. (A) Richness, (B) Abundance, (C) Yield (kg), (D) Number of Inflorescences/tree. Different letters within a field indicate significant differences among years following Repeated measures ANOVAs analyses (Table 3).
Table 3. Repeated Measures one-way ANOVAs on reproductive traits and insect community traits recorded annually in four fields of M. indica between 2017 and 2019.
With the exception of the Julie field in 2018, crop yield was strongly and positively correlated with fruit yields for all fields and all years (Pearson correlations for fruit production (the number of fruits produced per tree) vs. crop yield; Julie 2018: r = 0.39, P = 0.26; all other fields: r = 0.83–0.99, p << 0.01). Results involving crop yield and the number of fruits were always similar in magnitude and direction, thus for the sake of simplicity, we only present results for correlates of plant reproductive output as they relate to crop yield. All fields exhibited declines in the number of inflorescences per tree in 2018 that were followed by significant recoveries in 2019 (Figure 4C and Table 4). Nevertheless, at the field level, the number of inflorescences per tree during peak flowering was never a good predictor of plant reproductive output (Table 4). Instead, plant reproductive output (i.e., crop yield) was positively associated with insect species richness and abundance (Figure 6) but the significance and magnitude of these relationships were also influenced by field and sampling year (Table 4). In 2017, positive relationships between yield, species richness and insect abundance were strong for all fields (Figure 6 and Table 4). In 2018, following the hurricane events, these associations disappeared for the Julie and Tommy Atkins fields and were only present for the Keitt (only for abundance) and Kent (both for species richness and abundance) although they were weaker than they were in 2017 (Table 4). In 2019, relationships between crop yield and species richness and abundance returned for the Julie field and were present in the Keitt field but were absent in the areas with Kent and Tommy Atkins trees. While the Julie and Kent fields had consistent fruit yield values across years, the Kent and Tommy Atkins both experienced an overall reduction in crop yield in 2018 but then recovered to pre-hurricane values in the Kent field and went above pre-hurricane levels in the Tommy Atkins’ field in 2019 (Figure 5D and Table 3). In the Kent field, individuals showed the highest crop yield values of all fields (ave. 407 ± 58.13 kg), followed by the Keitt (ave: 183.25 ± 31.19 kg), Julie (ave: 60.77 ± 7.95 kg), and Tommy Atkins (ave: 77.55 ± 10.63 kg) fields (Figure 4D). The abundance of two of the dominant insect species (P. vinetorum and C. minima) showed positive correlation with reproductive output (crop yields) of mango trees but these relationships were not consistent for all years or fields, nor they were consistent within a single season using conservative alpha values (Table 5). With more relaxed alpha values, significant associations between insect abundances and crop yield were encountered in three instances for P. vinetorum, two for C. minima and none for A. mellifera. Using less conservative alpha values, the number of significant correlations increases to eight for P. vinetorum (distributed across all years), six for C. minima (in 2018 and 2019), and only two for A. mellifera (in 2017 and 2019 only in Julie) (Table 5).
Table 4. Results for simple regression coefficients from general linear model (GLM) analyses evaluating the variation in reproductive success (plant yield) as a function of insect species richness, insect abundance and the number of inflorescences/trees in four field of M. indica in 3 separate years.
Figure 6. Regressions of fruit yield as a function of global species richness and global insect abundance for four fields (Julie, Keitt, Kent, Tommy Atkins) across the 3 years of the study. For each field GLM analyses detected significant relationships between yield and insect richness and abundance in some years but not others (Table 4). Lines represent the line of best fit from significant regressions at p < 0.05.
Table 5. Pearson correlation coefficients for the number of fruits per tree and the abundance of dominant insect species per year per field.
Hurricanes are large-scale weather events with the potential to change insect communities and influence their subsequent dynamics (e.g., Schowalter and Ganio, 1999; McGinley and Willig, 1999; Schowalter et al., 2017). It has been argued that these events may disrupt plant-pollinator interactions with important consequences to food crops (National Research Council et al., 2007; Natalia Escobedo-Kenefic, 2018). While insects carry out most of the pollination function in plants, most of what we know about insect responses to hurricane events comes from other functional insect groups (herbivores, predators, omnivores, detritivores; e.g., Torres, 1992; McGinley and Willig, 1999; Schowalter and Ganio, 1999; Koptur et al., 2002; Spiller et al., 2016; Schowalter et al., 2017; Novais et al., 2018). Indeed, studies evaluating the response of insect pollinators to hurricane events have been few and limited in geographic and taxonomic scope which impairs making generalizations on the short- and long-term effects of these interactions (Bronstein and Hossaert-McKey, 1995; Pascarella, 1998; Roubik and Villanueva-Gutierrez, 2009; Landry, 2013). Here we discuss our results on hurricane-induced changes of pollinator communities of M. indica on the island of Puerto Rico following the passage of Hurricane Maria to: (1) build knowledge of post-hurricane community dynamics of insect pollinators, and contribute to the extensive literature available for hurricane responses of insect communities; (2) understand the role of diversity and abundance of insect communities vs. the role of individual insect species on the reproduction of a worldwide economically important species, and how these relationships may be influenced by extreme weather events; (3) discuss how this information may inform pollinator management in crop systems in areas where catastrophic weather events such as hurricanes are frequent events.
The literature related to post-hurricane dynamics in arthropod communities suggests that while arthropod populations can take different pathways (increase, decrease, or else exhibit complex responses (e.g., Gandhi et al., 2007), under some circumstances these assemblages can be highly resilient and return to pre-hurricane levels in a short period of time (Chen et al., 2020). However, this does not always occur because species responses to hurricanes can depend on the taxon, guild and the environmental context (biotic and abiotic) in which they occur (Schowalter et al., 2017; Novais et al., 2018; Savage et al., 2018). In our system, insect pollinator communities did change after the hurricanes of 2017 but as a whole they were on their way to pre-hurricane levels based on how similar they appeared in multivariate space by 2019. Post-hurricane dynamics of the individual insect pollinator species suggest that these temporal changes in community similarities across years were at least in part driven by changes in their relative abundance and not through the substitution of dominant insect species. At the individual species level, hurricanes clearly reduced the populations of P. vinetorum and A. mellifera (the two most-dominant species before the hurricanes) but A. mellifera abundances was on its way to pre-hurricane levels by 2019 (although not completely) while populations of P. vinetorum increased in numbers that year. In contrast, C. minima abundances spiked dramatically after the hurricane but decreased to pre-hurricane levels by 2019. A probable explanation for the observed differences in species’ post-hurricane abundance dynamics is that hurricane-driven changes in the biotic or abiotic environment influenced the life cycle of different taxa in different ways. For example, many syrphid flies such as P. vinetorum have aquatic larvae that are saprophagous and feed on organic matter and microorganisms (Pérez-Bañon et al., 2003; Sánchez-Galván et al., 2017). We know that the 2017 hurricane season resulted in a large accumulation of debris across the island of which 60% was organic (Lugo, 2018). In managed spaces, disposal of this debris was slow and that material might have created ideal habitats for the growth of syrphid larvae during the wet season. Meanwhile, larvae of Cochliomyia minima (Calliphoridae) most likely feed on dead carcasses (Yusseff-Vanegas, 2014), which were likely abundant after the hurricane events. Following Hurricane Maria and as a result of canopy cover loss, the island of Puerto Rico exhibited increases in temperatures of up to 4°C in some areas (Lugo, 2018).
Differential taxon responses to hurricane-induced abiotic changes may also explain the observed post-hurricane changes in the dominant taxa. It has been shown that exposure to higher than optimal temperatures in Apis mellifera bees may lead to reduced reproductive health in colonies through impaired drone development and reproductive quality, especially if changes are sudden (Rangel and Fisher, 2018 and references therein). High temperature exposures in Apis mellifera may also reduce survival and increase oxidative stress in bees (Li et al., 2019). On the other hand, higher temperatures shorten developmental time in Calliphoridae (Bansode et al., 2016), and some species (including some Cochliomyia) have high thermal tolerances (42C-53C; Richards et al., 2009). If local Cochliomyia have these traits, then it may explain their numerical increases following the hurricanes. Syrphid species, on the other hand, have variable responses (negative and positive) to changes in their abiotic environment but most of this work has come from temperate regions (Radenković et al., 2017; Milić et al., 2019). Clearly more research is needed on the direct and indirect influences of post-hurricane environmental changes to understand the mechanism driving changes of the dominant pollinators of M. indica.
Various studies have emphasized the need to ensure diversity and abundance of wild pollinators and the importance of protecting non-bees as a way to enhance pollination services (Blüthgen and Klein, 2011; Albrecht et al., 2012; Thomson, 2019) and crop production (Rader et al., 2013). The protection of the associated crop biodiversity is seen as an important element to ensure food security and sustainability of agricultural systems (FAO, 2018). Our results do show a clear association between pollinator diversity and abundance with fruit production in M. indica. However, an important result of this study is that fruit yields were highly reduced in one of the fields evaluated even when pollinator diversity (as expressed by species richness) and abundance increased locally. Therefore, the combined results not only suggest that the relationship between pollinator diversity, abundance and production in this crop is complex, but they also suggest that mechanisms other than changes in pollinators’ richness and total abundance themselves will also influence fruit production after hurricanes.
Here we provide several mechanistic hypotheses to explain our results for M. indica, some of which may or may not relate to observed changes in pollinator communities. One possibility is that not all M. indica pollinators are equally effective and that localized declines in the relative abundances of less abundant pollinators other than the dominant pollinator species studied here led to declines in yields after the hurricane for the Kent and Tommy Atkins fields. Even when all fields experienced declines in P. vinetorum and A. mellifera (the dominant pollinators) not all fields experienced declines in fruit yields. Moreover, unexpected significant increases in C. minima abundance in 2018 for three fields (Keitt, Kent, and Tommy Atkins) did not necessarily translate into concomitant increases in fruit yields for those fields in 2018. Several authors have discussed and tested potential mechanisms in which diversity could stabilize pollination services over time (e.g., Winfree and Kremen, 2009; Mukherjee et al., 2019). These include “density compensation” (i.e., the abundance of one pollinating species increases as a result of reductions in the abundance of another species), “response diversity” (i.e., some pollinating species are increase and others decrease by the same environmental change) and “cross-scale resilience” (i.e., different species are responding to the same environmental stressor at different spatial and temporal scales). While our study cannot be used to discriminate the applicability of these mechanisms, results presented here can be used to evaluate some of the premises of these mechanisms as to what extent these may or may not apply. Our data suggest that the hurricane event indeed influenced dominant species differently (P. vinetorum and A. mellifera decreased, while C. minima increased) and that these effects were not observed at the same spatial scales (i.e., abundances of P. vinetorum and A mellifera were reduced for all fields in 2018 but not those of C. minima which instead increased for three of the four fields). Also, fruit yield was reduced for Kent and Tommy even when those fields experienced increases in C. minima abundance. Those fruit yield patterns do not completely align with a hypothesis of “density compensation” effects by C. minima for Kent and Tommy Atkins as their fruit yields decreased even when abundances for this pollinating species increased for these fields. The fact that even with increases in species richness and abundances in 2018, fruit yields declined in the Tommy Atkins field also suggests that this flower visitor may not be as effective at compensating for losses or reduction in pollination services from other species that may have experienced local reductions in abundance The fact that the Julie field experienced a reduction in all dominant pollinators but not a reduction in fruit yields also suggests the possibility that other less frequent pollinators relative to the ones studied here may be acting as stabilizing influences of fruit yields and in a localized fashion. A long-term study with the generalist non-agricultural species Scrophularia californica showed that the relationship between the abundances of the most effective pollinators and reproductive success was non-linear and influenced by variation in spatial and temporal differences in pollinator diversity and abundance (Thomson, 2019). These complex relationships also apply to agricultural systems such as M. indica, whose management for pollinator services and diversity, especially following extreme atmospheric events, would therefore require an understanding of plant-pollinator relationships using both community and species-level approaches.
One important result was that correlations between plant yields and the abundances of individual pollinator species were not consistent across years or fields and that overall A. mellifera showed the least number of significant correlations with yield compared to P. vinetorum and C. minima. Indeed, prior studies have commented on the low attractiveness of mango flowers to honey bees (Free and Williams, 1976) and at least one experimental study that added managed honeybees found no significant increases in fruit production (Carvalheiro et al., 2010). Our results with honeybees are therefore not surprising considering those studies and raises questions about how effective the addition of managed honeybees is as a strategy to improve local pollinator services following hurricane events for crop species like M. indica. Indeed, the study by Carvalheiro et al. (2012) suggest that introducing areas of native vegetation to support pollinator services and improve crop production may be a better management strategy worth pursuing for M. indica management. On the other hand, the relationships between diversity and abundance of insect pollinators with plant yield were not equally strong for all fields and that post-hurricane changes in these relationships were not expressed equally among fields (Figure 6). Each field hosted a different cultivar. Thus, one possibility is that floral characteristics and rewards may differ among cultivars. Mango flowers are minute, and superficially similar among cultivars but could differ in some characteristics which may lead to differential pollinator attraction (Popenoe, 1917). In at least one study, the Keitt cultivar was reported to have low attractiveness relative to Kent and Tommy Atkins (Carvalheiro et al., 2012). We are in the process of analyzing data on floral traits and nectar rewards to address the possibility of differences in floral attractiveness or floral resources among cultivars. Likewise, we have ongoing experiments to evaluate potential differences in pollination effectiveness of dominant pollinators of M. indica to different cultivars to better understand the role of individual pollinator species and fruit production in this system. A last possibility, and equally likely, is that mango cultivars are in different spatial locations across the station and that landscape differences in insect requirements unknown to us may operate to influence the visitation to individual cultivars. Studies with M. mangifera in South Africa have shown that existing flowering resources available within and outside cultivated areas (i.e., natural vegetation) have the potential to influence mango floral visitation by contributing floral resources of shared visitors even outside the mango flowering season (Carvalheiro et al., 2010, 2012; Simba et al., 2018). Carvalheiro et al. (2010), indeed showed that the diversity levels experienced by M. indica trees and their fruit yields were dependent on the distance of trees from natural vegetation patches with more diversity and higher yields exhibited by trees that were closer to natural vegetation. There are patches of natural vegetation about 1 km away from the Juana Diaz Experimental Station and visually the matrix of cultivated trees surrounding the fields with the Julie and Keitt cultivars seems to have a more abundant tree cover than trees from the fields with Kent and Tommy Atkins (Figure 1).
A second non-mutually exclusive alternative for the unexpected declines in fruit production even when pollinator abundances increased following the hurricane may be related to direct hurricane effects on M. indica plants. Hurricane disturbances can damage plants to the point of reducing the resources available to produce flowers and fruits (Rathcke, 2000). A rapid assessment of mango trees at the Juana Diaz Experimental Station following Hurricane Maria, indicated that trees lost between 20 and 90% of their foliage as a result of this event with no tree mortality observed (Cabrera-Asencio, unpublished data). Thus, resource allocation decisions related to the production of leaves vs. fruits may also explain some of the crop yield dynamics but cannot account for all the variation in fruit production. Even when foliage recovery was slow and branch death was still observed in 2019, observed fruit production increased above pre-hurricane levels in 2019 (this study). The lack of tree mortality following hurricane Maria at our site contrasted with a study on mango tree damage in Florida following Hurricane Andrew in 1992 (Department of Health and Human Services, and Department of Agriculture, 1995; Crane and Balerdi, 1996). The Florida mango trees exhibited considerable damage with 58.4% of the trees showing massive damage or mortality which resulted in a 75% reduction in mango production even after 4 years. Furthermore, most mortality occurred in areas where trees were tall and not managed for size. By contrast, trees at the Juana Diaz Experimental Station were managed for size control which may explain different results.
Within season variation in the number of inflorescences did not relate to crop yields and that post hurricane reductions in inflorescence production in mango, while noticeable for all fields, did not necessarily translate into crop yield reductions. Inflorescences of M. indica produce numerous and minute unisexual flowers that reach up thousands of flowers like it has been reported for Tommy Atkins (2,238 flowers/inflorescence, Abourayya et al., 2011). It is also widely known that M. indica in cultivation produces many more flowers than its sets fruits (fruit sets ∼ 10%; Shu, 2009). Thus, one unexplored possibility is that even with the reductions of inflorescences and pollinators, following the hurricanes, the resulting flower:pollinator ratios still allowed to maximize fruit production in some cultivars. Also, the ratio of hermaphroditic to male flowers in andromonoecious plant systems like M. indica can vary in response to environmental stressors (Geetha et al., 2016) in ways that may reflect an optimal use of available resources to reproduction. Thus, another unexplored and not mutually exclusive possibility is that some cultivars of M. indica are also able to adjust floral resources in such a way that resources are used more efficiently toward producing fruits. These ideas would need to be explored thoroughly to understand how hurricane changes in floral resources and pollinators may ultimately influence reproduction in M. indica.
Fruit yields in this economically important species can bounce back (under some conditions) in less than 2 years. In the case of M. indica and assuming low mortality, two conditions may potentiate this recovery. The majority of insects visiting the M. indica cultivars are native to the Caribbean region and probably have, through their evolutionary history, developed adaptations that could make them highly resilient to these systems regardless of taxa. Second, the effects of hurricane-related changes in pollinator faunas on plant reproduction (regardless of the plant system) may depend whether or not pollination systems are generalized or specialized (Dalsgaard et al., 2009) or whether or not plant species have alternative mechanisms of plant reproductive assurance (Jones et al., 2001; Rivera-Marchand and Ackerman, 2006; Pérez et al., 2018). While most cultivars of M. indica depend on animal pollination to set fruit (Anderson et al., 1982; De Siqueira et al., 2008; Fajardo et al., 2008; Corredor and García, 2011; Kumar et al., 2012), it is apparent that globally mangos have a highly generalized pollination system, a condition that is likely favorable where extreme weather events are common such as in the Caribbean. Plant reproductive ecology theory states that the capacity to be pollinated by a large diversity of insects may allow for functional redundancy through shifts in dominant pollinator species, allowing for reproduction to occur in highly variable environments (Waser et al., 1996). A generalist pollination system may explain, at least in part, the naturalization and success of this crop in many areas of Latin America and the Caribbean. Whether such success may continue in the face of climatic change and expected increases in extreme weather events depends on long-term monitoring schemes. The use of information on pollinator diversity and abundance in this system for successful agriculture would certainly require more in-depth information on the role of global insect diversity and abundance vs. the role of individual species themselves.
The raw data supporting the conclusions of this article will be made available by the authors, without undue reservation, to any qualified researcher.
IC-A and EM-A conceived the study, designed the experiments, and co-wrote the manuscript. IC-A conducted the field research and observations, collected the data, and performed the statistical analysis. EM-A contributed to the data analysis and manuscript development strategy. All authors reviewed the manuscript, contributed to the article, and approved the submitted version.
The authors declare that the research was conducted in the absence of any commercial or financial relationships that could be construed as a potential conflict of interest.
We wish to acknowledge the Agricultural Experimental Station of the University of Puerto Rico, Mayagüez Campus (UPRM) and the Department of Environmental Sciences of the University of Puerto Rico, Rio Piedras Campus (UPRRP), for support in the development and implementation of this research project. We also would like to thank James D. Ackerman for his thoughtful comments on an earlier version of this manuscript. Frontiers and the University of Puerto Rico supported the publication of this article.
Abourayya, M. S., Kassim, N. E., El-Sheikh, M. H., and Rakha, A. M. (2011). Comparative study between inflorescences characteristics, pollen viability, germination, and dimensions of Tommy Atkins, Kent, and Keitt mango cultivars. Life Sci. J. 8, 100–105.
Aizen, M. A., Aguiar, S., Biesmeijer, J. C., Garibaldi, L. A., Inouye, D. W., Jung, C., et al. (2019). Global agricultural productivity is threatened by increasing pollinator dependence without a parallel increase in crop diversification. Global Change Biol. 25, 3516–3527. doi: 10.1111/gcb.14736
Albrecht, M., Schmid, B., Hautier, Y., and Müller, C. B. (2012). Diverse pollinator communities enhance plant reproductive success. Proc. R. Soc. B: Biol. Sci. 279, 4845–4852. doi: 10.1098/rspb.2012.1621
Anderson, D. L., Sedgley, M., Short, J. R. T., and Allwood, A. J. (1982). Insect pollination of mango in Northern Australia. Aust. J. Agric. Res. 33, 541–548. doi: 10.1071/ar9820541
Anderson, M. J. (2014). Permutational Multivariate Analysis of Variance (PERMANOVA). Hoboken, NJ: Wiley.
Anselin, L., Syabri, I., and Kho, Y. (2006). GeoDa : an introduction to spatial data analysis. Geograph. Anal. 38, 5–22. doi: 10.1111/j.0016-7363.2005.00671.x
Bansode, S. A., More, V. R., and Zambare, S. P. (2016). Effect of different constant temperature on the life cycle of a fly of forensic importance Lucilia cuprina. Entomol. Ornithol. Herpetol. 5, 2161–2983.
Bartomeus, I., Ascher, J. S., Gibbs, J., Danforth, B. N., Wagner, D. L., Hedtke, S. M., et al. (2013). Historical changes in northeastern US bee pollinators related to shared ecological traits. Proc. Natl. Acad. Sci. U S A. 110, 4656–4660. doi: 10.1073/pnas.1218503110
Beinroth, F. H., Engel, R. J., Lugo, J. L., Santiago, C. L., Ríos, S., and Brannon, G. (2003). Updated taxonomic classification of the soils of puerto rico, 2002. Agr. Exp. de P.R. 303:73.
Blüthgen, N., and Klein, A. M. (2011). Functional complementarity and specialisation: the role of biodiversity in plant–pollinator interactions. Basic Appl. Ecol. 12, 282–291. doi: 10.1016/j.baae.2010.11.001
Bronstein, J. L., and Hossaert-McKey, M. (1995). Hurricane Andrew and a Florida fig pollination mutualism: resilience of an obligate interaction. Biotropica 27, 373–381. doi: 10.2307/2388922
Burnham, K. P., and Anderson, D. R. (2002). Model Selection and Multimodel Inference: a Practical Information-theoretic Approach, 2nd Edn. New York, NY: Springer-Verlag.
Cameron, S. A., Lozier, J. D., Strange, J. P., Koch, J. B., Cordes, N., Solter, L. F., et al. (2011). Patterns of widespread decline in North American bumble bees. Proc. Natl. Acad. Sci. U S A. 108, 662–667. doi: 10.1073/pnas.1014743108
Campbell, R. J. (1992). Mangos: A Guide to Mangos in Florida (No. Ph0756). Coral Gables: FL: Fairchild Tropical Gardens.
Carvalheiro, L. G., Seymour, C. L., Nicolson, S. W., and Veldtman, R. (2012). Creating patches of native flowers facilitates crop pollination in large agricultural fields: mango as a case study. J. Appl. Ecol. 49, 1373–1383. doi: 10.1111/j.1365-2664.2012.02217.x
Carvalheiro, L. G., Seymour, C. L., Veldtman, R., and Nicolson, S. W. (2010). Pollination services decline with distance from natural habitat even in biodiversity-rich areas. J. Appl. Ecol. 47, 810–820. doi: 10.1111/j.1365-2664.2010.01829.x
Cavanaugh, J. E. (1997). Unifying the derivations for the Akaike and corrected Akaike information criteria. Statist. Probabil. Lett. 33, 201–208. doi: 10.1016/s0167-7152(96)00128-9
Central America Data (2016). Más Países Exportan Mangó a EE.UU. Available online at: https://www.centralamericadata.com/es/article/home/Ms_pases_exportan_mango_a_EEUU (accessed February 5, 2021).
Chen, X., Adams, B. J., Platt, W. J., and Hooper-Bùi, L. M. (2020). Effects of a tropical cyclone on salt marsh insect communities and post-cyclone reassembly processes. Ecography 43, 843–847.
Clarke, K. R. (1993). Non-parametric multivariate analysis of changes in community structure. Aus. J. Ecol. 18, 117–143. doi: 10.1111/j.1442-9993.1993.tb00438.x
Coetzer, L. A., Oosthuye, S. A., Wishart, D. L., and Robbertse, P. J. (1995). Influence of pruning on the flower sex ratio in some mango cultivars. s.a. mango growers assoc. Yearbook 15, 26–30.
Convention on Biological Diversity (2018). Convention and Sustainable Use of Pollinators. Rio de Janeiro: Convention on Biological Diversity.
Corredor, J. P., and García, L. J. (2011). Fenología, biología floral y visitantes florales en los cultivares de mango (Mangifera indica) Hilacha y Tommy Atkins en el valle del alto Magdalena (Colombia). Corpoica. Tecnol. Agropecu. 12, 21–32. doi: 10.21930/rcta.vol12_num1_art:212
Crane, J. H., and Balerdi, C. F. (1996). Effect of hurricane andrew on mango trees in Florida and their recovery. Acta Hort. 455, 323–330. doi: 10.17660/actahortic.1997.455.42
Dag, A., and Gazit, S. (2000). Mango pollinators in Israel. J. Appl.Hort. 2, 39–43. doi: 10.37855/jah.2000.v02i01.12
Dalsgaard, B., González, A. M. M., Olesen, J. M., Ollerton, J., Timmermann, A., Andersen, L. H., et al. (2009). Plant–hummingbird interactions in the West Indies: floral specialisation gradients associated with environment and hummingbird size. Oecologia 159, 757–766. doi: 10.1007/s00442-008-1255-z
De Siqueira, K. M. M., Kiill, L. H. P., Martins, C. F., Lemos, I. B., Monteiro, S. P., and Feirosa, E. A. (2008). Comparative study of pollination of Mangifera indica L. in conventional and organic crops in the region of the Subme’dio Sao Francisco valley. Revista Brasileira de Fruticultura 30, 303–310.
Department of Health and Human Services, and Department of Agriculture (1995). Ten-year Comprehensive Plan for the National Nutrition Monitoring and Related Research Program. Washington, D.C: Department of Health and Human Services.
Di Rienzo, J. A., Casanoves, F., Balzarini, M., Gonzalez, L., Tablada, M., and Robledo, C. W. (2018). InfoStat. Software Estadístico. Córdoba: Universidad Nacional de Córdoba.
Dormann, F. C., McPherson, J. M., Araújo, B. M., Bivand, R., Bolliger, J., Carl, G., et al. (2007). Methods to account for spatial autocorrelation in the analysis of species distributional data: a review. Ecography 30, 609–628. doi: 10.1111/j.2007.0906-7590.05171.x
Environmental Protection Agency [EPA] (2016). Climate change indicators in the United States, 2016, 4th Edn. Washington, D.C: Environmental Protection Agency.
Ewel, J. J., and Whitmore, J. L. (1973). The Ecological Life Zones of Puerto Rico and the US Virgin Islands. Washington, D.C: USDA. USDA Forest Service, Institute of Tropical Forestry, Research Paper ITF-018, 18.
Fain, S. J., Quiñones, M., Álvarez-Berríos, N. L., Parés-Ramos, I. K., and Gould, W. A. (2018). Climate change and coffee: assessing vulnerability by modeling future climate suitability in the Caribbean island of Puerto Rico. Clim. Change 146, 175–186. doi: 10.1007/s10584-017-1949-5
Fajardo, A. C., Medina, R. J., Opina, O. S., and Cervancia, C. R. (2008). Insect pollinators and floral visitors of mango (Mangifera indica L. C.V. Carabao). Phil. Agricult. Sci. 91, 372–382.
Feng, Y., Negron-Juarez, R. I., Patricola, C. M., Collins, W. D., Uriarte, M., Hall, J. S., et al. (2018). Rapid remote sensing assessment of impacts from Hurricane Maria on forests of Puerto Rico. PeerJ Inc. 6:e26597v1.
Free, J. B., and Williams, I. H. (1976). Insect pollination of Anacardium occidentale L., Mangifera indica L., Blighia sapida koening and persea americana mill. Trop. Agric. 53, 125–139.
Galán, S. V. (2009). El cultivo del mango. Situación Botanica 2a. Ediciones Mundi-Prensa Libros. México. 33–35Google Scholar
Gandhi, K. J., Gilmore, D. W., Katovich, S. A., Mattson, W. J., Spence, J. R., and Seybold, S. J. (2007). Physical effects of weather events on the abundance and diversity of insects in North American forests. Environ. Rev. 15, 113–152. doi: 10.1139/a07-003
Garibaldi, L. A., Steffan-Dewenter, I., Winfree, R., Aizen, M. A., Bommarco, R., Cunningham, S. A., et al. (2013). Wild pollinators enhance fruit set of crops regardless of honey bee abundance. Science 339, 1608–1611. doi: 10.1126/science.1230200
Garratt, M. P. D., Breeze, T. D., Jenner, N., Polce, C., Biesmeijer, J. C., and Potts, S. G. (2014). Avoiding a bad apple: insect pollination enhances fruit quality and economic value. Agricult. Ecosystems Environ. 184, 34–40. doi: 10.1016/j.agee.2013.10.032
Geetha, G. A., Shivashankara, K. S., and Reddy, Y. T. N. (2016). Varietal variations in temperature response for hermaphrodite flower production and fruit set in mango (Mangifera indica L). South African J. Botany 106, 196–203. doi: 10.1016/j.sajb.2016.07.011
Goguey, T. (1997). Architectural Approach of the mechanisms of canopy growth and flowering of mango trees. Acta Horticult. 455, 124–134. doi: 10.17660/actahortic.1997.455.17
Gómez, J. M., Bosch, J., Perfectti, F., Fernández, J., and Abdelaziz, M. (2007). Pollinator diversity affects plant reproduction and recruitment: the tradeoffs of generalization. Oecologia 153, 597–605. doi: 10.1007/s00442-007-0758-3
González-Varo, J. P., Biesmeijer, J. C., Bommarco, R., Potts, S. G., Schweiger, O., Smith, H. G., et al. (2013). Combined effects of global change pressures on animal-mediated pollination. Trends Ecol. Evol. 28, 524–530. doi: 10.1016/j.tree.2013.05.008
Gould, W., Fain, S. J., Pares, I. K., McGinley, K., Perry, A., and Steele, R. F. (2015). Caribbean Regional Climate Sub Hub Assessment of Climate Change Vulnerability and adaptation and Mitigation Strategies. Washington, D.C: United States Department of Agriculture.
Hammer, Ø, Harper, D. A., and Ryan, P. D. (2001). PAST: paleontological statistics software package for education and data analysis. Palaeontol. Electron. 4:9.
Harmsen, E. W., Tosado, P., and Mecikalski, J. (2014). Calibration of selected pyrometers and satellite derived solar radiation in Puerto Rico. Int. J. Renew. Energy Technol. 5, 43–54. doi: 10.1504/IJRET.2014.059660
Hsin Sung, I., Ming-Yin, L. G., Chin-Hsing, C., Ann-Shiou, C., and Wen-Shyong, C. (2006). Pollinators and their behaviors on mango flowers in Southern Taiwan. Formosan Entomol. 26, 161–170.
Intergovernmental Science-Policy Platform on Biodiversity and Ecosystem Services [IPBES] (2016). Summary for Policymakers of the Assessment Report of the Intergovernmental Science-Policy Platform on Biodiversity and Ecosystem Services on Pollinators, Pollination and Food Production. Bonn: IPBES, 1–30.
Jevanandam, N., Goh, A. G., and Corlett, R. T. (2013). Climate warming and the potential extinction of fig wasps, the obligate pollinators of figs. Biol. Lett. 9:20130041. doi: 10.1098/rsbl.2013.0041
Jiron, L. F., and Hedström, I. (1985). Pollination ecology of mango (Mangifera indica L.) (Anacardiaceae) in the neotropic region. Turrialba 35, 269–277.
Jones, K. E., Barlow, K. E., Vaughan, N., Rodríguez-Durán, A., and Gannon, M. R. (2001). Short-term impacts of extreme environmental disturbance on the bats of Puerto Rico. Animal Conserv. Forum 4, 59–66. doi: 10.1017/s1367943001001068
Klein, A.-M., and Bernard, E. (2007). Vaissiere, James H Cane, ingolf steffan-dewenter, saul a cunningham, claire kremen, and teja tscharntke. importance of pollinators in changing landscapes for world crops. Proc. R. Soc. B-Biol. Sci. 274, 303–313. doi: 10.1098/rspb.2006.3721
Koptur, S., Rodriguez, M. C., Oberbauer, S. F., Weekley, C., and Herndon, A. (2002). Herbivore-free time? damage to new leaves of woody plants after hurricane andrew 1. Biotropica 34, 547–554. doi: 10.1111/j.1744-7429.2002.tb00573.x
Kovach, W. L. (2007). MVSP - A MultiVariate Statistical Package for Windows, ver. 3.1. Pentraeth: Kovach Computing Services.
Kumar, P., Baskaran, S., Sundaravadivelan, C., Kuberan, T., and Anburaj, J. (2012). Influence of environmental factor son insect pollination activity of mangifera indica linn. Asian J. Plant Sci. Res. 2, 692–698.
Landry, C. L. (2013). Changes in pollinator assemblages following hurricanes affect the mating system of Laguncularia racemosa (Combretaceae) in Florida, USA. J. Trop. Ecol. 29, 209–216. doi: 10.1017/s0266467413000266
Li, X., Ma, W., Shen, J., Long, D., Feng, Y., Su, W., et al. (2019). Tolerance and response of two honeybee species Apis cerana and Apis mellifera to high temperature and relative humidity. PLoS One 14:e0217921. doi: 10.1371/journal.pone.0217921
Losey, J. E., and Vaughan, M. (2006). The economic value of ecological services provided by insects. Bioscience 56, 311–323. doi: 10.1641/0006-3568(2006)56[311:tevoes]2.0.co;2
Lugo, A. E. (2018). Social-Ecological-Technological Effects of Hurricane María on Puerto Rico: Planning for Resilience under Extreme Events. Berlin: Springer.
Marquis, R. J., Lill, J. T., Forkner, R. E., Le Corff, J., Landosky, J. M., and Whitfield, J. B. (2019). Declines and resilience of communities of leaf chewing insects on missouri oaks following spring frost and summer drought. Front. Ecol. Evol. 7:396. doi: 10.3389/fevo.2019.00396
McCune, B., and Grace, J. (2002). Analysis of Ecological Communities. MJM Software Design. Oregon, OR: Gleneden beach.
McCune, B., and Mefford, M. J. (2006). PC-ORD. Multivariate Analysis of Ecological Data, Version 5.0 for Windows. MJM Software. Oregon, OR: Gleneden Beach.
Memmott, J., Craze, P. G., Waser, N. M., and Price, M. V. (2007). Global warming and the disruption of plant-pollinator interactions. Ecol. Lett. 10, 710–717. doi: 10.1111/j.1461-0248.2007.01061.x
McGinley, M. A., and Willig, M. R. (1999). “The response of animals to disturbance and their roles in patch generation,” in Ecosystems of Disturbed Ground, ed. L. Walker (Amsterdam: Elsevier), 633–657.
Milić, D., Radenković, S., Radišić, D., Andrić, A., Nikolić, T., and Vujić, A. (2019). Stability and changes in the distribution of Pipiza hoverflies (Diptera, Syrphidae) in Europe under projected future climate conditions. PLoS One 14:e0221934. doi: 10.1371/journal.pone.0221934
Mukherjee, R., Deb, R., and Devy, S. M. (2019). Diversity matters: effects of density compensation in pollination service during rainfall shift. Ecol. Evol. 9, 9701–9711. doi: 10.1002/ece3.5500
Natalia Escobedo-Kenefic (2018). Background Document for the Biodiversity and Ecosystem Services Network (BES-Net) Caribbean Regional Trialogue on Pollinators, Food Security and Climate Resilience. Biodiversity and Ecosystem Services Network. Available online at: https://www.besnet.world/sites/default/files//event/document/Background%20document%20online_English.pdf (accessed February 5, 2021).
National Research Council, Board on Agriculture, Committee on the Nutrient Requirements of Small Ruminants, and Division on Earth and Life Studies (2007). Nutrient Requirements of Small Ruminants: Sheep, Goats, Cervids, and New World Camelids. Washington, D.C: National Research Council.
Novais, S., Macedo-Reis, L. E., Cristóbal-Pérez, E. J., Sánchez-Montoya, G., Janda, M., Neves, F., et al. (2018). Positive effects of the catastrophic Hurricane Patricia on insect communities. Sci. Rep. 8:15042.
Pascarella, J. B. (1998). Hurricane disturbance, plant-animal interactions, and the reproductive success of a tropical shrub 1. Biotropica 30, 416–424. doi: 10.1111/j.1744-7429.1998.tb00075.x
Pérez, M. E., Meléndez-Ackerman, E. J., and Monsegur-Rivera, O. A. (2018). Breeding system and pollination of Gesneria pauciflora (Gesneriaceae), a threatened Caribbean species. Flora 242, 8–15. doi: 10.1016/j.flora.2018.02.009
Pérez-Bañon, C., Rotheray, G., Hancock, G., Marcos-García, M. A., and Zumbado, M. A. (2003). Immature stages and breeding sites of some neotropical saprophagous syrphids (Diptera: Syrphidae). Annals Entomol. Soc. Am. 96, 458–471. doi: 10.1603/0013-8746(2003)096[0458:isabso]2.0.co;2
Popenoe, W. (1917). The Pollination of the Mango (No. 542). Washington, D.C: US Department of Agriculture.
Potts, S. G., Biesmeijer, J. C., Kremen, C., Neumann, P., Schweiger, O., and Kunin, W. E. (2010a). Global pollinator declines: trends, impacts and drivers. Trends Ecol. Evol. 25, 345–353. doi: 10.1016/j.tree.2010.01.007
Potts, S. G., Roberts, S. P. M., Dean, R., Marris, G., Brown, M. A., Jones, R., et al. (2010b). Declines of managed honey bees and beekeepers in Europe. J. Apicult. Res. 49, 15–22. doi: 10.3896/ibra.1.49.1.02
Radenković, S., Schweiger, O., Milić, D., Harpke, A., and Vujić, A. (2017). Living on the edge: forecasting the trends in abundance and distribution of the largest hoverfly genus (Diptera: Syrphidae) on the Balkan Peninsula under future climate change. Biol. Conserv. 212, 216–229. doi: 10.1016/j.biocon.2017.06.026
Rader, R., Bartomeus, I., Garibaldi, L. A., Garratt, M. P., Howlett, B. G., Winfree, R., et al. (2016). Non-bee insects are important contributors to global crop pollination. Proc. Natl. Acad. Sci. U S A. 113, 146–151.
Rader, R., Edwards, W., Westcott, D. A., Cunningham, S. A., and Howlett, B. G. (2013). Diurnal effectiveness of pollination by bees and flies in agricultural Brassica rapa: implications for ecosystem resilience. Basic Appl. Ecol. 14, 20–27. doi: 10.1016/j.baae.2012.10.011
Ramirez, F., and Davenport, T. L. (2010). Mango (Mangifera indica L.) flowering physiology. Sci. Horticult. 126, 65–72. doi: 10.1016/j.scienta.2010.06.024
Ramírez, F., and Davenport, T. L. (2016). Mango (Mangifera indica L.) pollination: a review. Sci. Horticult. 203, 158–168. doi: 10.1016/j.scienta.2016.03.011
Rangel, J., and Fisher, A. (2018). Factors affecting the reproductive health of honey bee (Apis mellifera) drones—a review. Apidologie 50, 759–778. doi: 10.1007/s13592-019-00684-x
Rathcke, B. J. (2000). Hurricane causes resource and pollination limitation of fruit set in a bird-pollinated shrub. Ecology 81, 1951–1958. doi: 10.1890/0012-9658(2000)081[1951:hcrapl]2.0.co;2
Richards, C. S., Price, B. W., and Villet, M. H. (2009). Thermal ecophysiology of seven carrion-feeding blowflies in Southern Africa. Entomol. Exp. et Appl. 131, 11–19. doi: 10.1111/j.1570-7458.2009.00824.x
Rivera-Marchand, B., and Ackerman, J. D. (2006). Bat pollination breakdown in the Caribbean columnar cactus Pilosocereus royenii. Biotropica 38, 635–642. doi: 10.1111/j.1744-7429.2006.00179.x
Rogers, S. R., Tarpy, D. R., and Burrack, H. J. (2013). Multiple criteria for evaluating pollinator performance in highbush blueberry (Ericales: Ericaceae) agroecosystems. Environ. Entomol. 42, 1201–1209. doi: 10.1603/en12303
Roubik, D. W., and Villanueva-Gutierrez, R. (2009). Invasive Africanized honey bee impact on native solitary bees: a pollen resource and trap nest analysis. Biol. J. Linnean Soc. 98, 152–160. doi: 10.1111/j.1095-8312.2009.01275.x
Sánchez-Galván, I. R., Ferrer, J., Galante, E., and Marcos-García, M. A. (2017). Bacteria and hoverflies (Diptera: Syrphidae) in tree hollows from the Iberian mediterranean forest. Environ. Entomol. 46, 137–142.
SAS Institute Inc (2019). Certified Professional Prep Guide: Advanced Programming Using SAS 9.4. Cary, NC
Savage, A. M., Youngsteadt, E., Ernst, A. F., Powers, S. A., Dunn, R. R., and Frank, S. D. (2018). Homogenizing an urban habitat mosaic: arthropod diversity declines in New York City parks after SuperStorm Sandy. Ecol. Appl. 28, 225–236. doi: 10.1002/eap.1643
Schowalter, T. D. (2012). Insect responses to major landscape-level disturbance. Annual Rev. Entomol. 57, 1–20. doi: 10.1146/annurev-ento-120710-100610
Schowalter, T. D., and Ganio, L. M. (1999). Invertebrate communities in a tropical rain forest canopy in Puerto Rico following Hurricane Hugo. Ecol. Entomol. 24, 191–201. doi: 10.1046/j.1365-2311.1999.00186.x
Schowalter, T. D., Willig, M. R., and Presley, S. J. (2017). Post-hurricane successional dynamics in abundance and diversity of canopy arthropods in a tropical rainforest. Environ. Entomol. 46, 11–20.
Shu, Z. H. (2009). Sex distribution, sex ratio and natural pollination percentage of mango (Mangifera indica L.). Acta Hortic. 820, 205–212. doi10.17660/ActaHortic.2009.820.22
Simba, L. D., Foord, S. H., Thébault, E., van Veen, F. F., Joseph, G. S., and Seymour, C. L. (2018). Indirect interactions between crops and natural vegetation through flower visitors: the importance of temporal as well as spatial spillover. Agricult. Ecosystems Environ. 253, 148–156. doi: 10.1016/j.agee.2017.11.002
Singh, G. (1988). Insect Pollinators of mango and their role in fruit setting. Acta Horticult. 231, 629–632. doi: 10.17660/actahortic.1989.231.22
Spiller, D. A., Schoener, T. W., and Piovia-Scott, J. (2016). Predators suppress herbivore outbreaks and enhance plant recovery following hurricanes. Ecology 97:10.
Thomson, D. M. (2019). Effects of long-term variation in pollinator abundance and diversity on reproduction of a generalist plant. J. Ecol. 107, 491–502. doi: 10.1111/1365-2745.13055
Torres, J. A. (1992). Lepidoptera outbreaks in response to successional changes after the passage of Hurricane Hugo in Puerto Rico. J. Trop. Ecol. 8, 285–298. doi: 10.1017/s0266467400006544
U.S. Forest Service (2018). In the Aftermath of Hurricane Maria- Part 3: El Yunque National Forest. Available online at: https://www.fs.fed.us/inside-fs/aftermath-hurricane-maria-part-3-el-yunque-national-forest (accessed February 5, 2021).
Villanueva-Gutierrez, R., Roubik, D. W., and Colli-Ucán, W. (2005). Extinction of Melipona beecheii and traditional beekeeping in the Yucatán peninsula. Bee World 86, 35–41.
Waser, N. M., Chittka, L., Price, M. V., Williams, N. M., and Ollerton, J. (1996). Generalization in pollination systems, and why it matters. Ecology 77, 1043–1060. doi: 10.2307/2265575
Winfree, R., and Kremen, C. (2009). Are ecosystem services stabilized by differences among species? a test using crop pollination. Proc. Biol. Sci. 276, 229–237. doi: 10.1098/rspb.2008.0709
Wongsiri, S., and Chen, P. P. (1995). Effects of agricultural development on honey bees in thailand. Bee World 76, 3–5.
Keywords: Caribbean, pollinator, disturbance, insect diversity, resilience, Diptera, Apis
Citation: Cabrera-Asencio I and Meléndez-Ackerman EJ (2021) Community and Species-Level Changes of Insect Species Visiting Mangifera indica Flowers Following Hurricane María: “The Devil Is in the Details”. Front. Ecol. Evol. 9:556821. doi: 10.3389/fevo.2021.556821
Received: 28 April 2020; Accepted: 25 January 2021;
Published: 05 March 2021.
Edited by:
Roksana Majewska, North-West University, South AfricaReviewed by:
Claire Kremen, University of British Columbia, CanadaCopyright © 2021 Cabrera-Asencio and Meléndez-Ackerman. This is an open-access article distributed under the terms of the Creative Commons Attribution License (CC BY). The use, distribution or reproduction in other forums is permitted, provided the original author(s) and the copyright owner(s) are credited and that the original publication in this journal is cited, in accordance with accepted academic practice. No use, distribution or reproduction is permitted which does not comply with these terms.
*Correspondence: Irma Cabrera-Asencio, aXJtYS5jYWJyZXJhYXNlbmNpb0B1cHIuZWR1
Disclaimer: All claims expressed in this article are solely those of the authors and do not necessarily represent those of their affiliated organizations, or those of the publisher, the editors and the reviewers. Any product that may be evaluated in this article or claim that may be made by its manufacturer is not guaranteed or endorsed by the publisher.
Research integrity at Frontiers
Learn more about the work of our research integrity team to safeguard the quality of each article we publish.