- 1Department of Fish Ecology and Evolution, Center of Ecology, Evolution and Biochemistry, Eawag, Swiss Federal Institute of Aquatic Science and Technology, Dübendorf, Switzerland
- 2Aquatic Ecology and Evolution, Institute of Ecology and Evolution, University of Bern, Bern, Switzerland
- 3Department of Environmental Sciences, University of Basel, Basel, Switzerland
- 4Department of Aquatic Ecology, Eawag, Swiss Federal Institute of Aquatic Science and Technology, Dübendorf, Switzerland
Invasive species can be powerful models for studying contemporary evolution in natural environments. As invading organisms often encounter new habitats during colonization, they will experience novel selection pressures. Threespine stickleback (Gasterosteus aculeatus complex) have recently colonized large parts of Switzerland and are invasive in Lake Constance. Introduced to several watersheds roughly 150 years ago, they spread across the Swiss Plateau (400–800 m a.s.l.), bringing three divergent hitherto allopatric lineages into secondary contact. As stickleback have colonized a variety of different habitat types during this recent range expansion, the Swiss system is a useful model for studying contemporary evolution with and without secondary contact. For example, in the Lake Constance region there has been rapid phenotypic and genetic divergence between a lake population and some stream populations. There is considerable phenotypic variation within the lake population, with individuals foraging in and occupying littoral, offshore pelagic, and profundal waters, the latter of which is a very unusual habitat for stickleback. Furthermore, adults from the lake population can reach up to three times the size of adults from the surrounding stream populations, and are large by comparison to populations globally. Here, we review the historical origins of the threespine stickleback in Switzerland, and the ecomorphological variation and genomic basis of its invasion in Lake Constance. We also outline the potential ecological impacts of this invasion, and highlight the interest for contemporary evolution studies.
Introduction
Colonizing species that invade new environments may experience novel selection pressures and adapt rapidly to local conditions, potentially culminating in divergent phenotypes between distinct habitats in the invaded range (Schluter, 2000; Reznick and Ghalambor, 2001; Sakai et al., 2001; Shine, 2012). In this way, invasive species provide a powerful opportunity to study evolution in action during colonization, population growth, and range expansion. When the invaded range includes unique environments that allow for niche expansion beyond what is observed in the native range, we may also learn about how populations respond to novel ecologically based divergent selection and witness processes akin to the earliest stage of adaptive radiation. Contemporary phenotypic evolution following anthropogenically facilitated invasions often results from selection on standing genetic variation rather than de novo mutations (Hendry et al., 2007; Barrett and Schluter, 2008; Prentis et al., 2008). Therefore, the nature of standing genetic variation in the invading population may have a strong influence on the dynamics of the invasion process.
The ability of a species to invade, colonize, adapt, and diversify can depend on whether the invasion derives from a single introduction, repeated introductions from the same source population, or from multiple distinct source populations (Ehrlich, 1989; Barrett and Husband, 1990; Sakai et al., 2001; Kolbe et al., 2004; Frankham, 2005; Lavergne and Molofsky, 2007; Lucek et al., 2010). Secondary contact between distinct lineages can generate potentially adaptive allelic variation through admixture (Anderson and Stebbins, 1954; Barton, 2001; Seehausen, 2004; Mallet, 2007; Prentis et al., 2008; Abbott et al., 2013; Seehausen and Wagner, 2014; Williams et al., 2014; Roy et al., 2015; Marques et al., 2019b), and this can even result in speciation through recombination of old genetic variants (Marques et al., 2019b). Such speciation can be driven by ecological (Marques et al., 2019b) or non-ecological (Schumer et al., 2015) processes. While single-source invasions are useful to investigate how a population responds to novel selection pressures during colonization of new environments, invasions with multiple genetic origins allow us to study the role of secondary contact and hybridization (or lack thereof) in ecological expansion and diversification.
The threespine stickleback superspecies (Gasterosteus aculeatus species complex, Linnaeus, 1758) is a popular model taxon in ecology and evolutionary biology research (Foster and Bell, 1994; McKinnon and Rundle, 2002; Hendry et al., 2013). Stickleback are known for their propensity to rapidly diversify through habitat dependent divergent selection, and this makes them a particularly useful model for addressing questions about how ecological divergence occurs in invasive species. There is strong evidence for repeated events of adaptive population divergence by stickleback during postglacial colonization of freshwater habitats (McKinnon and Rundle, 2002; Hendry et al., 2009). For such cases, the populations closely resembling the presumed ancestors of derived freshwater stickleback are still extant in the form of marine and anadromous populations with a Holarctic distribution (Baker et al., 2015; Fang et al., 2018). This allows for phenotypic comparisons between freshwater populations and their putative ancestral state. Such comparisons must be made with caution however, as marine populations are also diverging, and can be grouped into several genetically distinct clusters as well (DeFaveri et al., 2012; deFaveri and Merilä, 2014; Fang et al., 2018, 2020a; Morris et al., 2018). Furthermore, geographically adjacent marine and freshwater populations and species do not necessarily share the same common ancestor (Dean et al., 2019; Marques et al., 2019a). Within freshwater, ecotypic diversification occurs frequently along a lake-stream axis of divergence and rarely along a benthic-limnetic axis within lakes, where the latter has been found exclusively in coastal sectors of British Columbia, Canada (Bentzen and McPhail, 1984; Schluter and McPhail, 1992; Foster and Bell, 1994; McPhail, 1994; McKinnon and Rundle, 2002; Gow et al., 2008; Willacker et al., 2010; Østbye et al., 2016). Both the rapid adaptation of stickleback to freshwater, and subsequent ecotypic differentiation within freshwater habitats, has often occurred through selection on standing genetic variation present in oceanic populations (Colosimo et al., 2005; Barrett and Schluter, 2008; Schluter and Conte, 2009; Jones et al., 2012a,b; Terekhanova et al., 2014; Marques et al., 2017b; Bassham et al., 2018; Haenel et al., 2019; Fang et al., 2020a; Rennison et al., 2020). With regards to defensive morphology, freshwater stickleback typically show a reduction in plate numbers compared to the marine form (Foster and Bell, 1994; Barrett et al., 2008; Wootton, 2009), and occasional loss of pelvic spines (Morris et al., 1956; Bell, 1974; Reimchen, 1983; Campbell, 1985; Shapiro et al., 2004; Chan et al., 2010; Lescak and von Hippel, 2011), potentially in response to differences in predation regime between freshwater and the Ocean (Barrett, 2010). Alleles for low plated phenotypes, for example, are at low frequencies in oceanic populations but have increased in frequency in freshwater populations multiple times, independently (Colosimo et al., 2005; Barrett and Schluter, 2008). Other adaptations to freshwater habitats, such as the loss of the pelvic girdle (Chan et al., 2010; Xie et al., 2019) and the increased capacity to synthesize essential fatty acids (i.e., Docosahexaenoic acid) through duplications of the FADS2 gene (Ishikawa et al., 2019) have arisen from de novo mutations.
European threespine stickleback populations in general (Fang et al., 2020a), and Swiss populations in particular (Kottelat and Freyhof, 2007; Lucek et al., 2010; Marques et al., 2019a), provide an interesting setting to observe how secondary contact between lineages can affect ecotype formation in freshwater environments. In Switzerland natural colonizations of freshwater catchments from two divergent lineages represented in Europe (Fang et al., 2018) coincide with recent anthropogenic introductions of other European lineages among and between catchments (Kottelat and Freyhof, 2007; Lucek et al., 2010; Marques et al., 2019a). In Lake Constance, for example, there is ongoing debate about the origin of the lake and stream stickleback populations, and the importance of secondary contact for ecotype formation (Lucek et al., 2010, 2012, 2013, 2014b; Moser et al., 2012; Roesti et al., 2015; Marques et al., 2016, 2019a). In light of this previous work, and recent observations, there is mounting evidence for three major stickleback ecotypes, two of which are observed in the species complex globally, namely an entirely lacustrine form that breeds within the lake, and a stream resident form. The third form may be rather unique: a potamodromous form that lives in the lake but migrates to streams to reproduce, resembling anadromous ecotypes of the oceans. Some of these ecotypes persist in the face of gene flow, with migratory lake ecotypes (i.e., potamodromous ecotype) breeding in sympatry and parapatry with resident stream ecotypes, suggesting that some populations of Lake Constance stickleback are in the incipient stage of ecological speciation, despite their very recent history in the system (Marques et al., 2016).
In the present paper, we review the genetic, genomic, and phenotypic research to date on threespine stickleback in Central Europe, focusing on Switzerland and the invasion of the Lake Constance region. By providing a review of the existing literature on stickleback populations within Lake Constance and drawing on additional stickleback research from elsewhere, we summarize what is known in this system, highlight knowledge gaps and the utility of this system for studying the genomics and ecology of invasion, range expansion, contemporary ecological diversification, and the evolutionary consequences of secondary contact.
How Stickleback Diversify
The G. aculeatus superspecies of threespine stickleback most likely originated in the Western Pacific Ocean (Fang et al., 2020a), and colonized marine habitats around the northern hemisphere during the Pleistocene (Ortí et al., 1994; Mäkinen and Merilä, 2008; Fang et al., 2018, 2020b; Ravinet et al., 2018). It expanded around the Northern Pacific Ocean and through the Bering Sea Strait into the Arctic Ocean, and would have arrived in the Atlantic Ocean between 300 and 50 Kya (Fang et al., 2018, 2020b). After this widespread colonization of the Holarctic Ocean, the ancestors of the Atlantic G. aculeatus complex initially split into colonists of freshwaters in Southern Europe and the Atlantic Ocean population between ~100 and ~25 Kya (Fang et al., 2020b). These Southern European populations colonized freshwater habitats during the Pleistocene, and persisted in freshwater glacial refugia during the Last Glacial Maximum (Mäkinen and Merilä, 2008; DeFaveri et al., 2012; Lucek and Seehausen, 2015; Sanz et al., 2015; Fang et al., 2018), while the Trans-Atlantic clade colonized freshwater habitats in Northern Europe, and Eastern North America much later during the Holocene (Ortí et al., 1994; Mäkinen et al., 2006; Ravinet et al., 2014; Fang et al., 2018, 2020b). Within the Trans-Atlantic clade, multiple geographically structured subclades exist, including Baltic Sea, Barents Sea, North Sea, and mainland European lineages that diverged 27-11 Kya. In the mainland European clade, lineages diverged 17-5 Kya between large river catchments such as the Rhine, Loire, Vistula etc., likely following deglaciation of Central Europe (Fang et al., 2018).
Despite these advances in understanding phylogenetic relationships in the threespine stickleback superspecies, and despite the evidence for many biological species within it (McKinnon and Rundle, 2002; Dean et al., 2019), the diversity of forms is taxonomically treated by most authors under the singular name Gasterosteus aculeatus (Wootton, 1976; Th and Bakker, 1988; Bell, 1995). This “convenient solution” follows a taxonomic history in which over 40 species had been described, often based on phenotypic traits that may well be informative for delimiting two species within a given locale, but not for delimiting local species against all others across the global range (Bertin, 1925; Münzing, 1959, 1963; Penczak, 1966; Miller and Hubbs, 1969; Wootton, 1976; Bell, 1995; Denys et al., 2015). In the European context, for example, Gasterosteus gymnurus was described by Cuvier (Cuvier and Valenciennes, 1828; Cuvier, 1829) based on landlocked stickleback from Northern France and Southern England that had lateral plating restricted to the structural plates. Given the historical West-East separation of these plate morphs across much of Europe (Münzing, 1963), others later applied this name to any stickleback from anywhere in Europe and beyond that shared this plating phenotype (Gordon, 1902; Koch and Heuts, 1943; Kottelat and Freyhof, 2007; Denys et al., 2015). Others recognized that this practice had led to a highly polyphyletic nature of G. gymnurus and lumped it further with the mostly marine fully plated form into a single taxon, G. aculeatus (Wootton, 1976; Paepke, 1983; Bell, 1995; Denys et al., 2015), pending a thorough taxonomic revision that takes biological species into account and that has yet to happen. As a result, the G. aculeatus superspecies currently contains both deeply divergent geographical lineages and reproductively isolated biological species within Europe (Jones et al., 2006; Mäkinen et al., 2006; Mäkinen and Merilä, 2008; DeFaveri et al., 2012; Lucek and Seehausen, 2015; Pérez-Figueroa et al., 2015; Berner et al., 2017; Fang et al., 2018; Dean et al., 2019; Marques et al., 2019a).
Phylogeographic and population genetic studies revealed that freshwater ecotypes and species have evolved many times in parallel from marine ancestors, although some freshwater clades have clearly also expanded their ranges across multiple catchments, such that adjacent catchments often share the same lineage, species or ecotype (Mäkinen et al., 2006; Lucek and Seehausen, 2015; Fang et al., 2018; Ishikawa et al., 2019; Marques et al., 2019a). Evidence from the fossil record in Western North America and Eastern Russia shows that members of the family Gasterosteidae have been colonizing freshwater habitats from the Pacific Ocean since the Miocene, so there have been repeated cycles of colonization, adaptation, and extinction over the evolutionary history of the group (Bell, 1977; Bell and Haglund, 1982; Bell et al., 2006, 2009).
Throughout the northern hemisphere, habitat-specific adaptation in allopatry or parapatry is responsible not only for the parallel evolution of freshwater stickleback from marine or anadromous ancestors (Jones et al., 2006, 2012b), but also for most of the parallel evolution of recurrent ecotypes within freshwaters (Hendry et al., 2009; Willacker et al., 2010), albeit this is more pronounced in the Pacific than in European populations (Fang et al., 2020a). Sympatric pairs of benthic and limnetic stickleback are observed in a handful of British Columbian coastal lakes and nowhere else, despite intensive research (McPhail, 1984; Schluter and McPhail, 1992; Baker et al., 2005). These sympatric pairs are thought to have evolved through serial colonizations from the ocean rather than sympatric speciation from a single source population (Hendry et al., 2009; Bolnick, 2011) and this is deemed the “double-invasion hypothesis” (Schluter and McPhail, 1992; McPhail, 1994; Kassen et al., 1995; Taylor and McPhail, 1999). Models predict that sympatric speciation in stickleback is possible, though unlikely, as pressures from disruptive selection and assortative mating tend to be too weak to result in sympatric speciation (Bolnick, 2004, 2011), and indeed no strong case is known. An incipient sympatric species pair has recently been described from a small lake in Switzerland but this pair evolved within the hybrid zone between the Eastern and the Western European lineages (Marques et al., 2017a), and it is currently unclear whether it emerged in sympatry from a hybrid population or represents persistence of the hybridizing and nearly collapsed pre-existing lineages.
Evolution of lake-stream ecotypes and species pairs in parapatry has occurred much more frequently in stickleback than the evolution of species that can persist in sympatry (Reimchen et al., 1985; Lavin and Mcphail, 1993; Hendry et al., 2002, 2009; Hendry and Taylor, 2004; Berner and Grandchamp, 2009; Deagle et al., 2012; Kaeuffer et al., 2012; Moser et al., 2012; Roesti et al., 2012; Lucek et al., 2013, 2014b). This is not surprising given that much weaker selection is sufficient to retain phenotypic distinctiveness when the opportunity for gene flow is geographically constrained (Doebeli and Dieckmann, 2003). Active matching habitat choice between lake and stream may in some ecotonal situations also facilitate the persistence of differentiation (Edelaar et al., 2008; Edelaar and Bolnick, 2012). Stickleback residing in different habitats often show divergence in foraging traits and performance (Arnegard et al., 2014; Best et al., 2017; Schmid et al., 2019), body size (Hendry et al., 2002; Sharpe et al., 2008), migration behavior (Harvey et al., 1997; Kitano et al., 2012), life history (Moser et al., 2012), nuptial coloration (Hagen and Moodie, 1979; Reimchen, 1989; Jenck et al., 2020), and/or in defense traits and defense performance (Reimchen, 1983, 1992b, 1994, 2000; Lucek et al., 2013).
Strong reproductive isolation rarely evolves between parapatric ecotypes of stickleback, and this is likely the chief reason for the extreme rarity of sympatric species persistence (Räsänen et al., 2012). In the few cases where reproductive isolation has been demonstrated, it can result either as a by-product of divergent adaptation to different habitats or ecological niches that lead to assortative mating preferences (Rundle et al., 2000; Boughman, 2001), or from ecological selection that puts hybrids at a fitness disadvantage and may lead to reinforcement (Rundle and Schluter, 1998; Lackey and Boughman, 2017). Previous work on crosses of sympatric benthic and limnetic stickleback demonstrated that hybrids are not at any intrinsic disadvantage, but they may perform worse than either of the parental types in the parental niche (Schluter, 1994, 1995, 2003; Schluter et al., 1996; Hatfield and Schluter, 1999; Vamosi et al., 2000; Jones et al., 2006; Gow et al., 2007; Arnegard et al., 2014; Laurentino et al., 2020). This opens the possibility that hybrids could invade novel (non-parental) niches if the opportunity arises.
The Environmental Context of the Stickleback Invasion in Lake Constance
Lake Constance is a peri-alpine lake on the northern edge of the European Alps, and is the third largest lake by surface area in Central Europe (after Lakes Balaton and Geneva), and the second by volume (after Geneva). The lake is part of the Rhine catchment and is located at the intersection between Germany, Switzerland, and Austria (Figure 1). Lake Constance consists of a pair of lakes joined by a 4.5 km stretch of river called the Seerhein. Upper Lake Constance is a large (surface area = 472 km2), deep (max. depth = 254 m, mean depth = 101 m) and monomictic lake, while lower Lake Constance is considerably smaller (surface area = 63 km2) and more shallow [max. depth = 46 m, mean depth 13 m; (Petri, 2006)].
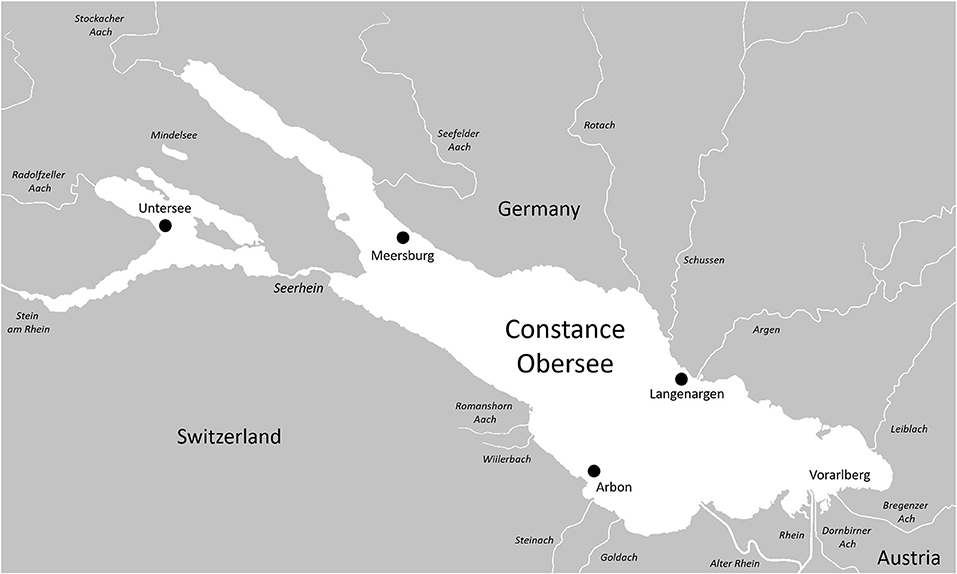
Figure 1. Map of Lake Constance drainage with sampling locations for the 2014 Projet Lac biodiversity sampling campaign. Note the location of Vorarlberg in the southeast of the Lake, where stickleback were first reported by Heller (1871).
The geological history of the lake indicates that it was formed by the process of glacial erosion (Müller and Gees, 1968) through the expansion of the Rhine glacier from the inner Alps into the Central European lowlands during the Würm ice age, roughly 115,000–11,700 Kya (Keller and Krayss, 2000). During this period, Lake Constance was covered by ice and its entire surface became ice-free only ~14,500 ybp (Keller and Krayss, 2000). Thus, all extant freshwater fish species of the lake must have colonized or have been introduced following glacial retreat (Behrmann-Godel et al., 2004). The lake is presently fed by the Alpine Rhine to the south, and drains into the North Sea through the Rhine. Capture of the outflow of Lake Constance by the Rhine formed roughly 7,000–8,000 ybp (Wessels, 1995). Prior to this river capture, Lake Constance drained via the Danube into the Black Sea (Keller and Krayss, 2000). Although this connection no longer exists [with the exception of the underground Danube-Aach system; (Hötzl, 1996)], several freshwater fish species have evidently colonized Lake Constance via the Danube (Nesbø et al., 1999; Bernatchez, 2001; Behrmann-Godel et al., 2004; Gum et al., 2005; Barluenga et al., 2006; Vonlanthen et al., 2007; Hudson et al., 2014; Gouskov and Vorburger, 2016; Lucek et al., 2018).
Naturally oligotrophic, Lake Constance experienced intensive eutrophication beginning in the first half of the 20th century and peaking in the 1980's as a result of human population expansion, agriculture, and industry (Petri, 2006). Total phosphorus concentrations began rapidly increasing from the 1930's primarily from agriculture and sewage runoff. Following concerns of environmental degradation and loss of water quality, the International Commission for the Protection of Lake Constance (IGKB) was formed in 1959 by water management organizations of the bordering countries (Petri, 2006). Efforts to reduce phosphorus concentrations and return the lake to near its original oligotrophic state have eventually been successful in the second decade of the 21st century (Petri, 2006; IGKB, 2018). These rapid shifts in nutrient profiles have had strong impacts on the ecosystem, particularly with regards to primary productivity and oxygen availability in the profundal zone (Numann, 1972; Gaedke and Schweizer, 1993; Sommer et al., 1993; Kümmerlin, 1998; Stich, 2004; Stich and Brinker, 2010), which led to the extinction of at least one endemic fish species (Vonlanthen et al., 2012).
Aside from eutrophication, humans have also facilitated the colonization of many invasive species in the lake. Both the upper and lower Lake Constance have been colonized by considerable numbers of non-indigenous species of fish, crustaceans, and molluscs over the past two centuries, some of which have established large populations (Rey et al., 2005; Alexander et al., 2016). As we discuss in more detail below, threespine stickleback are not native to Lake Constance, but are currently hyper-abundant, representing ~28% of the total fish biomass, and are the second most abundant fish species in the lake (Zimmermann, 2002; Alexander et al., 2016). Large populations of stickleback are known from other large oligotrophic lakes within their natural range, e.g., in Greenland, Alaska, and the West Coast of Canada (Greenbank and Nelson, 1959; Reimchen, 1992a; Bergersen, 1996; Jeppesen et al., 2017), but such a hyper-abundance is rare in quantitative assessments of lakes that are as large and species rich as Constance. As such, understanding the invasion and establishment of the lake Constance population is also of considerable interest for ecosystem management and conservation.
Origins of the Threespine Stickleback in Lake Constance
Switzerland lies at the edge of the natural range of threespine stickleback in Europe (Fang et al., 2018). Threespine stickleback historically had a disjunct and very limited distribution in Switzerland, being only represented by two divergent native clades at opposite ends of the country. The first, part of the mainland European and thus Trans-Atlantic clade, was restricted to Rhine tributaries near Basel (Leuthner, 1877; Fatio, 1882; Schulze, 1892; Göldi, 1914) and outside of Switzerland in the Rhine, and parts of Northern France. The second, part of the Adriatic and thus South European clade, was restricted to Lago Maggiore and its tributaries in the Adriatic catchment, and found otherwise in Northern Italy. Recent work suggests that the low plated stickleback of the upper Rhine belong to the same clade as those of Northern France (Mäkinen et al., 2006), which has been referred to as G. gymnurus, whereas the low plated stickleback of Lago Maggiore belong to the highly divergent yet taxonomically undescribed South European clade (Mäkinen et al., 2006; Cano et al., 2008; Fang et al., 2018, 2020b).
Currently, there are two additional clades that have invaded and colonized Switzerland. First, a lineage colonizing via the middle Rhône that is now dominant in Lake Geneva is a genetically distinctive member of the West European clade (Fang et al., 2018; Marques et al., 2019a). Second, an Eastern European lineage originating from the Baltic Sea Catchment currently dominates Lake Constance (Marques et al., 2019a). However, the history of stickleback in Lake Constance in terms of their time of arrival, source of colonization, and the mode of diversification into lake and stream ecotypes has been debated recently (Roesti et al., 2015; Rösch et al., 2017; Marques et al., 2019a). Notably, some authors proposed a natural postglacial colonization of the Lake Constance basin from the Danube about 9,000 years ago, similar to some other freshwater fish species of which Danube populations inhabit Lake Constance as a consequence of river capture by the Rhine catchment (Nesbø et al., 1999; Bernatchez, 2001; Behrmann-Godel et al., 2004; Gum et al., 2005; Barluenga et al., 2006; Vonlanthen et al., 2007; Hudson et al., 2014; Gouskov and Vorburger, 2016; Lucek et al., 2018). According to this hypothesis, the invading lineage was a stream ecotype that first colonized the tributaries of Lake Constance. These stream populations became isolated from each other because the intermittent lake habitat was presumably ecologically unsuitable (termed “ecological vicariance”). At a later point in time, the lake-adapted population emerged and reconnected previously isolated stream populations through gene flow (Roesti et al., 2015).
Other authors proposed that two or three divergent European lineages of stickleback were introduced into the Lake Constance region in the last 150 years, from catchments south of the Baltic Sea, the upper Rhine, and the Rhône (Lucek et al., 2010; Marques et al., 2019a). In this hypothesis, secondary contact between genetically distinct and previously allopatric lineages resulted in hybridization and variable extents of genetic admixture in different stream populations (Lucek et al., 2010; Roy et al., 2015; Marques et al., 2016, 2019a). The divergent freshwater lineages, including low plated stickleback from Western Europe [i.e., the nominal species referred to as Gasterosteus gymnurus (Cuvier and Valenciennes, 1828; Cuvier, 1829)] and a fully plated stickleback from Eastern Europe, formed a hybrid zone across the Swiss midlands including the western end of Lake Constance. As such, the lake populations in the Constance system are predominantly of East European origin, while stream populations around Lake Constance vary in their genetic composition: ranging from predominantly West European with introgression from the East European-derived lake form, to mainly East European-derived with some genomic islands of putative stream adaptation recruited from West European stickleback. In this latter case, introgression of alleles into the East European lineage has probably facilitated ecological speciation into stream vs. lake ecotypes within the East European-derived lineage (Marques et al., 2019a). We review these different hypotheses in the light of the historical ichthyological evidence, phylogeographic, and genomic data.
Historical ichthyological records describe the fish community of Lake Constance dating back to 1557 (Mangolt, 1557). Herein, Mangolt used 76 different common names to refer to the species found in the lake, though many are duplicates or regional names for the same species, of which there are 27 unique species described in total (Ribi, 1942). This text does not include any mention of the threespine stickleback, however he does describe other small fish such as minnows (“Cyprinus phoxinus” referring to the genus Phoxinus), suggesting that he did not neglect species of the same size as stickleback that have no commercial value. In the same period, an encyclopedia titled Historiae animalium (1551–1558) was published by the Swiss naturalist and physician Conrad Gessner (Gessner, 1558) that attempted to describe the animals of the world in detail. Within the Historiae Piscium & Aquatilium Animantium Natura (1558) volume, Gessner includes a brief account of the threespine and the ninespine stickleback with text and illustrations that are taken from Guillaume Rondelet's “De Piscibus” (Rondelet, 1554; Figure 2) and Albertus Magnus' 13th century text “De Animalibus” (Magnus, 1999; Kitchell Jr. & Resnick translation), referring to them as “Pisiculus aculeatus” and “Pisiculus pungitius,” along with other common names. In the corollary added by Gessner, he states that: “They are found elsewhere in Strasbourg [France], Wittenberg [Germany], and in the Alb River [a tributary of the Rhine in Germany]. None are among us.” (Gessner, 1558), providing strong evidence that G. aculeatus was well-known by natural historians at the time, but absent from Lake Constance. By 1828, Cuvier and Valenciennes write that “Gessner alone says there are [no stickleback] in Switzerland; but we know the opposite” (Cuvier and Valenciennes, 1828), however they do not indicate the regions of Switzerland where stickleback have been found and their information may relate to the native populations from Basel or Lago Maggiore. Further ichthyological descriptions of Lake Constance fish species come from two 19th century fish atlases (Nenning, 1834; von Rapp, 1854) describing 28 and 30 species, respectively [there are presently 42 fish species recognized, of which 11 – including G. aculeatus – are introduced (Alexander et al., 2016)], and again make no mention of the stickleback. Given our thorough investigation, and the early interest in describing the fish species of Lake Constance, it seems unlikely that stickleback would have gone unnoticed for so long had the species been present.
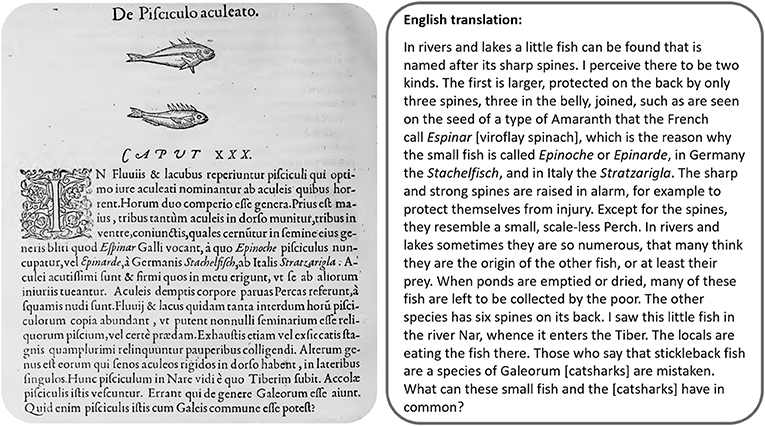
Figure 2. Latin descriptions of the threespine and ninespine stickleback from Guillaume Rondelet's 1554 volume “De Piscibus” accompanied by an English translation.
The earliest report of stickleback in the region documents their appearance upstream of Lake Constance in Austria just over 150 years ago when they were observed in a tributary to the Alpine Rhine in Vorarlberg (Heller, 1871). In the following years, the presence of stickleback is further noted by both German and Austrian statistical reports (Krafft, 1874; Wittmack, 1875) stating that they are observed in the dead arms of the Alpine Rhine (Wittmack, 1875), and breed in Lake Constance from April to June (Krafft, 1874). Even after these previous sightings, Fehling et al. (1881) writes that “In the river area of the Danube, the stickleback is completely missing, even in Lake Constance it has not been found,” suggesting that perhaps the introduced population was at that point in time still isolated in the south-eastern part of the lake and its tributaries. Other documents noted that the stickleback was absent from the upper Danube system until the late 19th century (von Siebold, 1863; Münzing, 1963; Ahnelt, 1986) and most authors agree that the only natural population in Switzerland north of the Alps resided near Basel (Figure 3; Schinz, 1837; Wittmack, 1875; Leuthner, 1877; Fehling et al., 1881; Schulze, 1892; Rauther, 1926; Scheffelt, 1926; Muckle, 1972; Ahnelt, 1986; Ahnelt and Amann, 1994; Ahnelt et al., 1998; Paepke, 2002; Altman et al., 2013), that also served as a popular source location of stickleback for aquarium fish traders in Switzerland (Steinmann, 1936). This historical record implies that stickleback were present in the Lake Constance basin before they established in the upper Danube (Vogt and Hofer, 1909; Gaschott, 1941; Berinkey, 1960; Balon, 1967; Ahnelt, 1986; Cakić et al., 2000; Holcik, 2003; Polačik et al., 2008; Lisjak et al., 2015), making a natural colonization from the Danube unlikely.
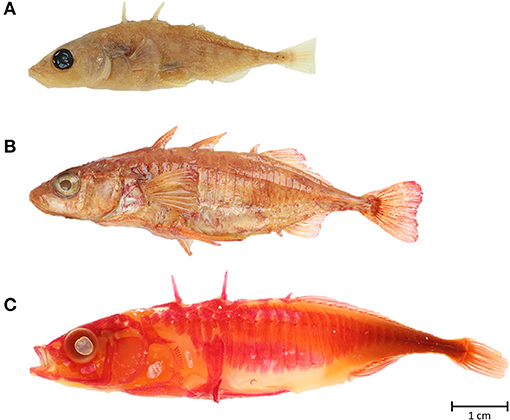
Figure 3. (A) Native low plated specimen belonging to the West European lineage collected in Basel, Switzerland in 1935; (B) A fully plated specimen collected in Lake Constance near Langenargen, Germany in 1963; (C) A large, fully plated pelagic female captured from Lake Constance near Meersburg, Germany during the 2014 Projet Lac survey.
In the mid-19th century, aquarium keeping emerged in central Europe as a means of popularizing natural sciences (Rossmässler, 1857). Early on, stickleback became popular among European aquarium enthusiasts for their colouration and behavioral displays (Schinz, 1837; Prévost, 1861; Fehling et al., 1881), so it would not be surprising that some unwanted pets would be released into the wild. Indeed, in a previous review of stickleback occurrence in Lake Constance, Muckle (1972) describes a conversation with an aquarist who claims that a fish breeder released some stickleback in a stream that flows into Lake Constance near Allensbach in the late 1920's, originating from a pond in Germany inside the autochthonous range of low plated Rhine stickleback. Muckle (1972) also describes “a release in the years 1933 and 1934 by the “friends of aquaria fish Konstanz” group, of fish imported from a pond near Freiburg im Breissgau [Rhine, Germany],” again within the native range of low plated Rhine stickleback. Together with the references above regarding introductions to the eastern end of Lake Constance, these historical records provide direct evidence for the introduction of stickleback to the east and west of the lake, and very probably from multiple distinct source populations. Within the same time period that stickleback were first sighted in Lake Constance, a population from the Western lineage of the middle Rhône that was collected from a small stream called Seillon near Vichy was deliberately released into a stream connected to Lake Geneva in Hermance, Switzerland in 1872 by Professor François-Isaac Mayor of Geneva (Fatio, 1882). A few decades later, further introductions and releases were documented in the Lake Neuchâtel catchment and in the upper Rhône upstream of Lake Geneva in the early 20th century (Blanc, 1922; Bertin, 1925).
More recently, analyses of genomic data have been central to the debate over the source location(s), the phenotype of the founding population(s), and timing of stickleback colonization in Lake Constance (Roesti et al., 2015; Marques et al., 2016, 2019a). The ecological vicariance scenario's (Roesti et al., 2015) proposal of a natural colonization of Lake Constance via the upper Danube drainage, was motivated by the genetic and phenotypic similarity between Lake Constance and contemporary upper Danube populations (Moser et al., 2012; Roesti et al., 2015). However, it is noteworthy that there is evidence that these latter populations were themselves also introduced (Ahnelt, 1986; Ahnelt and Amann, 1994). Based on demographic modeling of population genomic data and assuming that the lake and stream populations originated from a single colonization of the Constance system, a colonization time as far back as ~9,000 years ago was estimated, suggesting an early split between lake and stream ecotypes within the Constance catchment (Roesti et al., 2015). Basal placement of stream populations in a phylogeny, increased linkage disequilibrium, and extended selective sweep signatures in lake stickleback genomes were further interpreted in support of this scenario (Roesti et al., 2015). According to this scenario, the Constance stickleback population would represent a natural range expansion from regions close to the Black Sea to freshwater following Pleistocene glacial retreat 12 Kya (McPhail, 1994; McKinnon and Rundle, 2002). Such a colonization route is in principle, plausible, because geological and biological evidence indicates that the Danube drainage was previously connected to Lake Constance (Nesbø et al., 1999; Keller and Krayss, 2000; Bernatchez, 2001; Behrmann-Godel et al., 2004; Gum et al., 2005; Barluenga et al., 2006; Vonlanthen et al., 2007; Hudson et al., 2014; Gouskov and Vorburger, 2016; Lucek et al., 2018). Today, the only possible corridor connecting the two drainages is the underground Danube-Aach system, which makes contemporary natural colonization by surface water dwelling fishes implausible. However, an early postglacial colonization of threespine stickleback is at odds with the historical ichthyological records described above.
A recent integrative analysis of the phylogeography and demography of Lake Constance basin stickleback in a broader European-wide context (Marques et al., 2019a) supports the hypothesis involving secondary contact between divergent, previously allopatric lineages. Individuals from the Lake Constance catchment possess mitochondrial haplotypes belonging to at least three divergent stickleback lineages contemporarily found in four main Central European river catchments draining into the Baltic Sea, the North Sea, the Mediterranean Sea, and the Black Sea. This is in stark contrast to the ecological vicariance model, where a single threespine stickleback lineage would have colonized the Black Sea from the Mediterranean Sea through the Bosphorus strait after its formation roughly 7,000 ybp (Gökaşan et al., 1997; Fang et al., 2018), continuing into the Danube, and onward into Lake Constance. If this were the case, we would expect Lake Constance stickleback to exhibit a high frequency of Black Sea haplotypes (Mäkinen and Merilä, 2008; DeFaveri et al., 2012; Lucek and Seehausen, 2015; Sanz et al., 2015; Vila et al., 2017), but such haplotypes are absent in both the Lake Constance catchment as well as in the upper Danube. Instead, the lake population itself is dominated by a haplotype from the Baltic Sea catchment in Poland (Lucek et al., 2010). Further mitochondrial analyses revealed the presence of four additional mitochondrial haplotypes within the Lake Constance catchment, in stream habitats at low to moderate frequencies (Lucek et al., 2010; Moser et al., 2012; Marques et al., 2019a). These haplotypes are otherwise known from populations native to the North Sea, the Rhine catchment, and the Rhône catchment (Mäkinen and Merilä, 2008; Lucek et al., 2010; Marques et al., 2019a). This secondary contact model suggests that introduced stickleback from two or three ancient European lineages met in the Constance basin and have introgressed, facilitating differentiation between lake and stream ecotypes via retention of historical lineage differentiation and/or likely adaptive recruitment of western lineage alleles into stream populations (Marques et al., 2019a). Genome-wide RAD-sequencing data showing admixture between Rhine, Rhône, and Baltic lineages in Lake Constance, a higher Rhine and Rhône ancestry in stream stickleback, and an enrichment of genomic islands of differentiation for Rhine lineage alleles in stream ecotypes support this view (Marques et al., 2019a).
Phenotypic analyses also support the secondary contact scenario. Stickleback native to the Rhine and Rhône originating from natural colonization from the Sea (Lucek et al., 2010), including Rhine populations from Basel (Figure 3), were historically fixed for the low plated phenotype (Fatio, 1882; Münzing, 1963), prior to hybridization and introgression with the introduced Eastern European lineage (Lucek, 2016). In contrast, Baltic Sea freshwater populations were fixed for the fully plated phenotype until recent introductions of low plated fish from Western Europe (Bańbura, 1994) and the likely introduced freshwater populations in the upper and middle Danube which contain a mix of low and fully plated morphs (Ahnelt, 1986). Lake Constance is dominated by fully plated stickleback, while the populations in inlet streams to the North and West of the lake are polymorphic with high frequencies of low plated fish. In contrast, streams to the south of Lake Constance have low frequencies of low plated fish. These phenotypic patterns are in line with the inferences from mitochondrial and genomic data of an origin of lake stickleback from the Baltic region and an admixed origin of stream stickleback north and west of the lake, supporting the secondary contact hypothesis.
In summary, the historical ichthyological evidence, phylogeographic analysis in a European context, and demographic modeling of genomic data, all suggest that the most plausible scenario for the origin of stickleback in Lake Constance is that beginning in the late 1800's stickleback were introduced by aquarium hobbyists or fishermen from multiple sources (Heller, 1871; Fatio, 1882; Steinmann, 1936; Muckle, 1972) that represent at least three different European lineages and a minimum of three different introductions (Marques et al., 2019a). Following these introductions, the lake population then underwent an expansion, becoming abundant by the 1960's (Laurent, 1972; Numann, 1972; Deufel, 1985; Zimmermann, 2002; Alexander et al., 2016), and has experienced fluctuations in density over the past 50 years. This debate, and growing support for the “secondary contact scenario,” reveals the value of integrative analyses of invasion dynamics that include inferences about historical fish occurrences from ichthyological records (where available), historical colonization pathways from geomorphological evidence, and both demographic history and phylogeographic patterns from genomic data.
In the following sections, we review general patterns of ecological and evolutionary diversification in the G. aculeatus species complex, and develop contemporary parallels associated with the invasion of Lake Constance.
Examining the Lake Constance Stickleback Population From a Global Perspective
Their historical and geographic origins notwithstanding, Lake Constance stickleback are unique among central European freshwater populations in that a high number of individuals are foraging in the pelagic zone of the lake, and appear to be phenotypically adapted for a lifestyle in large pelagic environments. A lake-wide fish diversity survey of Swiss lakes, conducted in 2014, demonstrated that lacustrine stickleback are not only hyper-abundant within Lake Constance (Table 1), but are also distributed along a variety of habitats throughout the water body, with some individuals found foraging in the profundal zone as deep as 40 m (Alexander et al., 2016). Similar distribution patterns in freshwater lakes are only known from Lake Michigan, where stickleback invaded the ecosystem on a comparable time scale, occupy qualitatively similar depth ranges (Stedman and Bowen, 1985), and consume a diet that is mostly zooplankton and mysids (Turschak and Bootsma, 2015). During the breeding season, lacustrine stickleback have been observed to spawn both within lakes along the shoreline, and in adjoining streams (Snyder, 1991; Harvey et al., 1997; Lucas et al., 2001). We observe this pattern in Lake Constance as well, though it remains to be investigated whether these resident and potamodromous lacustrine fish constitute a panmictic population or are reproductively isolated. Potamodromous individuals migrate in large numbers several kilometers up into streams in April, where the males build nests and where they breed before they leave the streams again in May/June, much like anadromous marine stickleback. These potamodromous individuals demonstrate genomic differentiation from stream residents in both sympatry and parapatry (Marques et al., 2016), and differ from the stream populations in an array of phenotypic traits. Lake fish have elongated gill rakers and a distinct head shape that allows feeding on small planktonic prey, as opposed to stream resident individuals, which feed predominantly on larger benthic prey (Berner et al., 2010; Lucek et al., 2012, 2013; Moser et al., 2012, 2015; Karvonen et al., 2015; Roesti et al., 2015; Marques et al., 2016, 2019a). Lake and stream ecotypes also differ in defensive morphology, with lake stickleback possessing elongated spines and a set of tall bony lateral plates that cover most of the body, whereas stream resident fish have shorter spines and a reduced plate coverage which they achieve by having either fewer, or shorter, lateral plates (Berner et al., 2010; Moser et al., 2012; Lucek et al., 2013, 2014b; Marques et al., 2016). Lastly, the ecotypes differ in life history, where lake resident and potamodromous fish live longer and start to reproduce on average 1 year later than stream residents (Lucek et al., 2012; Moser et al., 2012, 2015). Lake-stream divergence has been reported elsewhere in Switzerland (and in other locations globally; Table 2) but is much less pronounced in other large Swiss lakes (Lucek et al., 2013, 2014b). This suggests that naturally colonized systems and anthropogenic introductions of threespine stickleback have the underlying process of ecotypic differentiation in common, but that the rate at which phenotypic and genetic divergence occur, and its dimensionality, are system specific.
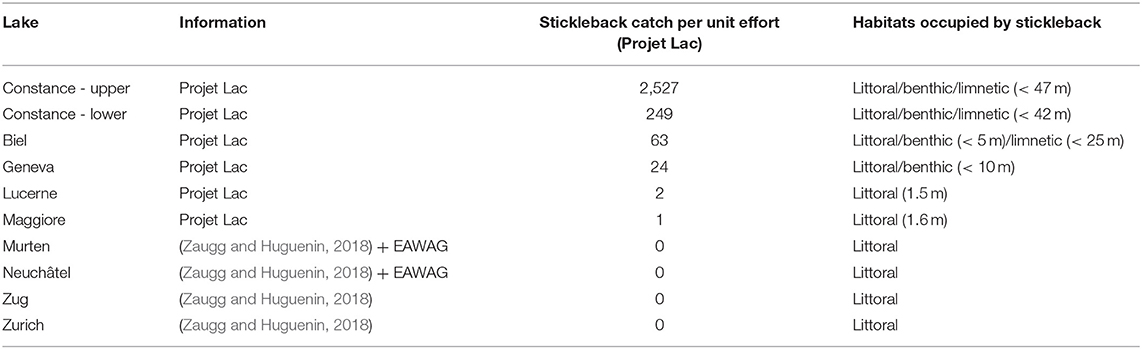
Table 1. Stickleback caught during the Projet Lac sampling campaign, and other Swiss lakes where they are known to occur but were not captured.
In a recent assessment, sticklebacks in Lake Constance represent ~28% of the fish biomass, and accounted for 96% of fish captured in the pelagic zone of the upper lake during the Projet Lac survey (Alexander et al., 2016). Despite being identified as hyper-abundant from the 1960's to the 70's (Numann, 1972), and again in littoral habitats almost two decades ago (Zimmermann, 2002) threespine sticklebacks were first recorded as bycatch in the pelagic zone of Constance by commercial fisheries in 2013 (Rösch et al., 2017). Previous reports (Numann, 1972; Deufel, 1985) indicate that stickleback have been a nuisance to fishermen in the past, with populations reaching high abundances, but then declining rapidly, presumably from parasitic infections (e.g., whitespot disease Ichthyophthirius multifiliis and carp louse Argulus foliaceus), though evidence of this is largely speculative.
The massive recent increase in stickleback abundance coincides with a sharp decline in pelagic whitefish (Coregonus wartmanni, Bloch, 1784) yields, both in the number of individuals caught, and their weight-at-age (Rösch et al., 2017). Previous work has speculated that the invasive stickleback population could have a negative impact on whitefish growth and abundance, and shows that stickleback will prey on whitefish larvae in laboratory foraging experiments (Roch et al., 2018; Ros et al., 2019) or following stocking (Roch et al., 2018). However, the first stickleback population expansion during the eutrophication period in Constance coincides with population size increase in whitefish (Numann, 1972), so the relationship between whitefish and stickleback abundances is either mediated by some other factors in the environment, or it is not causal. It has been proposed that either competition for pelagic zooplankton resources such as Daphnia- that have declined in abundance with the re-oligotrophication of Lake Constance (Straile and Geller, 1998; Stich and Brinker, 2010; Rösch et al., 2017) - or direct predation on whitefish eggs and larvae (Roch et al., 2018; Ros et al., 2019) are responsible for this reduction in yield. Predation by sticklebacks on eggs and juveniles of their own species occurs frequently (Whoriskey and FitzGerald, 1985; Hyatt and Ringler, 1989; Smith and Reay, 1991; Foster and Bell, 1994; Manica, 2002; Mehlis et al., 2010) along with predation on larvae of other fish species (Hynes, 1950; Manzer, 1976; Delbeek and Williams, 1988; Kean-Howie et al., 1988; Gotceitas and Brown, 1993; Nilsson, 2006; Kotterba et al., 2014; Byström et al., 2015), while previous studies on stickleback populations in the Baltic Sea have suggested that intraguild predation on eggs and juvenile fish is responsible for the observed declines in perch (Perca fluviatilis, Linnaeus, 1758) and pike (Esox lucius, Linnaeus, 1758) recruitment (Nilsson, 2006; Bergström et al., 2015; Byström et al., 2015; Nilsson et al., 2019; Eklöf et al., 2020). It is possible that the same is occurring with Lake Constance whitefish populations, although evidence for this is currently lacking. Our analysis of stickleback gut contents (see below) did not detect any whitefish eggs or larvae in wild stickleback, nor did other studies (Lucek et al., 2012; Moser et al., 2012; Roch et al., 2018), though intraspecific egg predation was observed. However, no study to date has sampled lacustrine stickleback during or shortly after whitefish spawning season, so the hypothesis about whether direct predation on whitefish eggs by invasive stickleback is responsible for population declines requires further testing.
For piscivorous predators, increased stickleback abundance may provide a new food source that could be particularly important for overwintering birds. In a similar fashion, the presence of introduced zebra mussels in Lake Constance (Dreissena polymorpha, Pallas, 1771) has resulted in a 4-fold increase in overwintering molluscivorous waterbird densities since their introduction in the 1960's (Werner et al., 2005). Following environmental protection legislation under the EU Birds Directive, a breeding population of great cormorants (Phalacrocorax carbo, Linnaeus, 1758) has established in lower Lake Constance since 1997, and has grown steadily despite population culling (Gaye-Siessegger, 2014). Both cormorant, and great crested grebe (Podiceps cristatus) populations have increased since 2010, and it has been suggested that the abundance of stickleback in the lake is responsible for this change (Werner et al., 2018). Stomach content analysis of great cormorants from lower Lake Constance between 2011 and 2013 during the autumn and winter seasons showed that 19.1% of birds had eaten stickleback, and 24.3% of fish eaten were threespine stickleback. This indicates that cormorants frequently target G. aculeatus, although they only contribute a small proportion of total diet content by weight (Gaye-Siessegger, 2014). A later study (Rey and Becker, 2017) documented an increase in the proportion of cormorant stomachs containing stickleback in comparison to the previous survey, with 39% of individuals hunted in the spring of 2016 containing G. aculeatus. Thus, it is possible that these abundant prey items in the pelagic zone (Eckmann and Engesser, 2018) are particularly beneficial when other species of dietary importance, such as Perca fluviatilis, move to deeper water to overwinter (Wang and Eckmann, 1994; Eckmann and Imbrock, 1996). Many other avian species in the region such as grebes, herons, mergansers, kingfishers, gulls, and terns are known to consume threespine stickleback (Foster and Bell, 1994; Werner et al., 2018), so we may observe an increase in their abundance, or changes in migration patterns as well in response to increased pelagic stickleback densities in the future.
In addition to their high abundance, broad habitat use, and interactions with other species, one of the most compelling characteristics of the Lake Constance stickleback is their exceptional body size. In freshwater, G. aculeatus typically attains between 30 and 80 mm in standard length (SL) (Wootton and Wootton, 1976; Foster and Bell, 1994) and has an average lifespan of 2–4 years (Pennycuick, 1971; Moodie, 1984; Baker, 1994). Stickleback caught in Lake Constance during the Projet Lac survey demonstrated large body sizes and complete defensive complexes (Figure 3), with the largest individual measuring 101 mm in SL (Alexander et al., 2016; Figure 4). Since many individuals captured during this survey were above the typical size range of the species, we compiled data from our own work, along with published sources on body size distributions of freshwater stickleback populations from around the globe (Figure 4). This data shows that their large body size is not necessarily unique for the species, but that Lake Constance individuals are larger than those from most other European freshwater populations. There are two other regions where freshwater stickleback have been measured at comparable body sizes, the Haida Gwaii archipelago (British Columbia, Canada), and Lake Towada, Japan. In Haida Gwaii, stickleback in a small number of very distinctive populations or species (the giant threespined stickleback) have been observed to grow up to 106 mm in SL (Gambling and Reimchen, 2012) and can live to be 8 years old (Reimchen, 1992a), while in an introduced Japanese population in Lake Towada females with a SL > 100 mm have been collected (Mori and Takamura, 2004). Furthermore, marine individuals of threespine stickleback have been reported at sizes up to 110 mm (Muus and Nielsen, 1999), and evidence from the fossil record suggests that such “gigantism” in stickleback has evolved previously, with fossilized individuals measured at 110 mm (Bell, 1984). Large body size hence is repeatedly observed but uncommon in the G. aculeatus species complex, especially in freshwater populations.
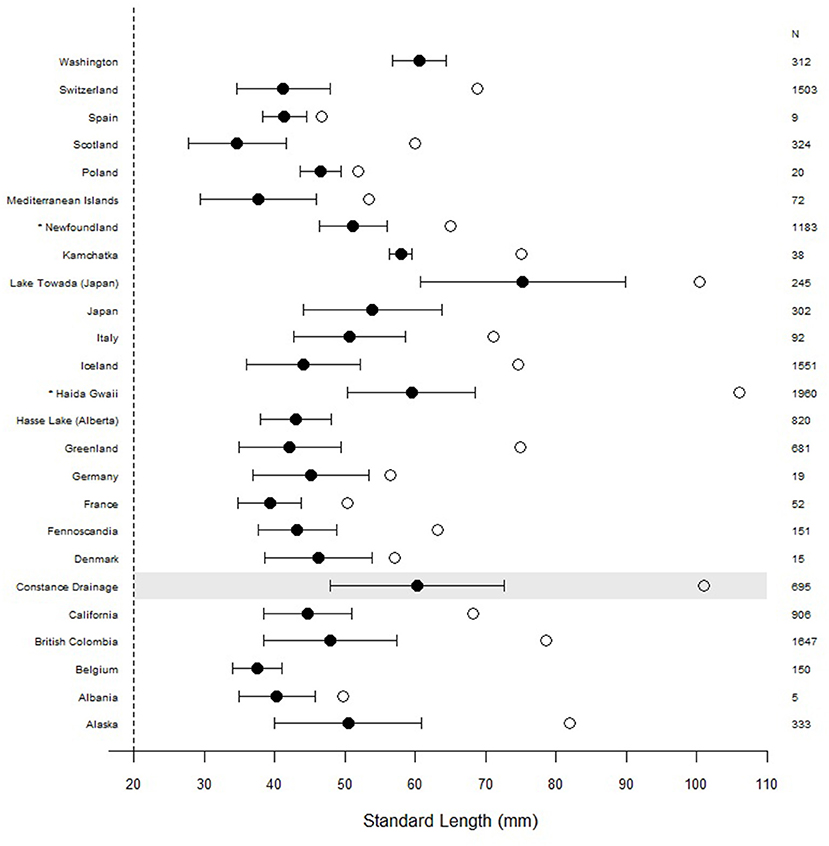
Figure 4. Mean standard length of stickleback captured in the Constance drainage (highlighted in gray) with standard deviation compared to body sizes of other freshwater populations around the globe. Open circles represent maximum body sizes, when available from the data. We obtained body size data from published studies (Supplementary Table 1) and calculated means for each region by pooling all individuals and both sexes. For studies that did not provide raw data, we re-calculated mean and standard deviation values by combining means between studies using standardized methods (Altman et al., 2013). *Haida Gawii populations are represented by the top 10 largest individuals of each sex from 98 populations (Reimchen et al., 2013), and thus the distribution is right-skewed, Newfoundland populations are measured as total length and not SL.
What could be responsible for this pattern of gigantism, and how do Lake Constance stickleback compare to other systems? For Haida Gwaii, Gambling and Reimchen (2012) suggest that large body size has evolved in order to escape gape-limited piscivores that are abundant in the ecosystem, but this is not the case in Lake Towada because there are no predatory fish (Mori and Takamura, 2004). Instead, Mori and Takamura (2004) suggest that either an abundant supply of planktonic prey or greater fish longevity are responsible for the large sizes they observed, although they did not directly measure stickleback ages in their study. In Lake Constance stickleback the majority of lake breeding fish are 2 years of age when they first reproduce (Moser et al., 2012), but fish of 3 years of age are also common and occasionally they are older (Lucek et al., 2012; Moser et al., 2012). There is some experimental evidence that predation pressure on Swiss stickleback populations can select for faster growth rates (Zeller et al., 2012), and that the lake population in Constance grows faster than one of the stream populations when reared on limnetic prey, although they grow at a similar rate when reared on benthic prey (Lucek et al., 2012). This latter experiment suggests that the lake ecotypes are better adapted to grow quickly on limnetic prey than are stream ecotypes. However, whether the larger body sizes of the lake populations are due to adaptations allowing sustained rapid growth under limnetic food resources, or the result of selection for larger body size as a mechanism to escape predation is still unknown.
Understanding variation in dietary niche and metabolism might yield insights into the uniqueness of the Constance population with respect to European and Global populations. In Swiss populations, there is some evidence for differences in the dietary niche between the West European and East European lineages, but insufficient data to quantitatively compare dietary niche variation between the native and introduced range. These lineages have colonized freshwater independently, with populations diverging in the late Pleistocene (Fang et al., 2020b) or early Holocene (Marques et al., 2019a), many thousands of years before their introduction to Swiss lakes and before any known instances of secondary contact. As a result, the lineages have a different evolutionary history of adaptation to freshwater environments. In light of this, previous work has suggested that the Constance population has a more pelagic phenotype and feeds more efficiently on plankton than the population of Lake Geneva (Best et al., 2017), which originates from the West European Lineage (Fang et al., 2018) that invaded Switzerland from the middle Rhône. Indeed, in our analysis of stomach contents from 253 individuals, following similar methods as Lucek et al. (2012), and Anaya-Rojas et al. (2016), and presented here for the first time, we confirm that individuals in both upper and lower Lake Constance are predominantly feeding on plankton (e.g., cladocerans and copepods; Figure 5), and a low proportion of chironomid larvae. We also found some evidence for within-lake dietary niche variation between the upper and lower lake (Figure 5): Individuals caught in the lower lake consumed a higher proportion of bosmina (two-tailed z-test, z = 2.45, p = 0.012; upper lake N = 232; lower lake N = 21) compared to those from the upper lake. Furthermore, within the upper lake, pelagic individuals consumed a higher proportion of bythotrephes than benthic individuals did (two-tailed z-test, z = 2.8, p = 0.005; benthic N = 133, pelagic N = 99). More of such comparative dietary work is needed in other large lakes in order to test how niche variation within populations in large lakes (e.g., Lake Geneva) compares among lineages in the natural and invaded range.
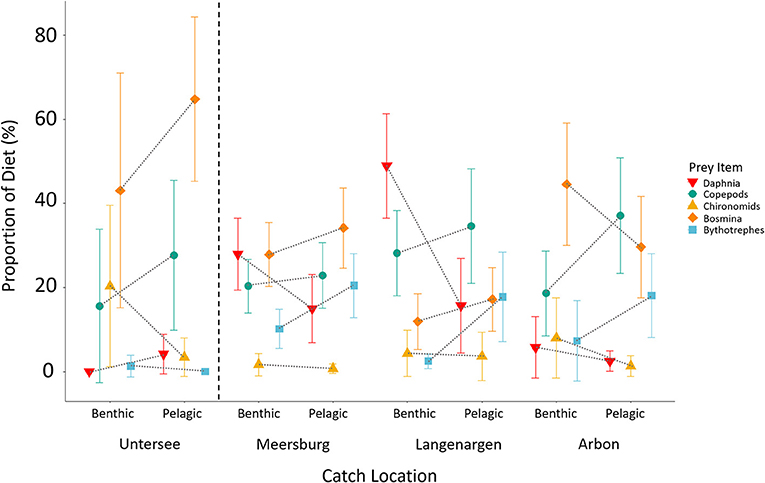
Figure 5. Gut content proportions of the top five most common prey items in Projet Lac stickleback split by region and catch location. Individuals caught in the benthic habitat were those caught <25 m from the lakebed, while individuals caught in the pelagic habitat were captured >25 m. Stickleback caught in Untersee have a higher proportion of Bosmina in their diet, and within Obersee fish caught in deeper pelagic regions had a higher proposition of Bythotrephes in their diet (see main text).
There is also compelling evidence for differences in the extent of metabolic adaptation by the two stickleback lineages to the lower average food quality of freshwater compared to marine prey. During the colonization of freshwater by marine species, organisms need to adapt to an environment where essential fatty acids are low in abundance (Arts et al., 2009). When encountering nutritional constraints, organisms can evolve metabolic or ecological adaptations to overcome this environmental scarcity. In freshwater fish, in vivo biosynthesis of long-chain fatty acids by desaturation of short-chain derivatives is performed by enzymes produced by the Fads2 gene (Castro et al., 2012). Fads2 has recently been identified as a key metabolic gene for several freshwater fish species in that they exhibit multiple independent gene duplications across different lineages, and this suggests that copy number variation is under positive selection for its role in long chain fatty acid biosynthesis (Ishikawa et al., 2019). From the same study, an investigation of copy number variation in European freshwater stickleback populations showed that the Western European lineage possesses higher copy numbers of Fads2 than the population in Lake Constance (Ishikawa et al., 2019), implying that they are capable of more efficient metabolic desaturation and elongation of polyunsaturated fatty acids. It is possible that this has enabled the Western lineage to persist in environments with low food quality such as stream or benthic lake littoral habitats. If organisms are incapable of fatty acid biosynthesis, another evolutionary strategy is to adapt their morphology and behavior to more efficiently prey on food sources that are rich in essential nutrients. A planktonic diet can provide these essential nutrients [i.e., fatty acids; (Smyntek et al., 2008)], although some of the high quality prey items are also evasive (e.g., copepods). Based on the gut content data and the prolific use of the open water habitat, it is possible that Lake Constance stickleback are well-adapted to exploit the abundant zooplankton populations in Lake Constance, and this may help them compensate for the fewer copies of Fads2 that they possess in comparison to the Western lineage.
Putting the Invasion of Lake Constance Into Perspective for Drainages in Switzerland
Invasive species vary widely in both their ability to invade, and their impact following invasion (Williamson and Fitter, 1996; Zenni and Nuñez, 2013). In the case of stickleback in Switzerland, success of establishment varies within the introduced taxon: the population of stickleback in Lake Geneva seems to be much less dominant than the population in Lake Constance, despite similar timescales of invasion. Stickleback are present in many of the large freshwater bodies within Switzerland, including Lakes Biel, Neuchâtel, Lucerne, and Geneva, where they range from exceedingly rare (Lucerne) to locally common (Geneva) but are hyper-abundant only in Lake Constance. Here we will discuss this phenomenon in the context of the two largest lakes, Constance and Geneva. Both are large peri-alpine lakes that harbor introduced populations of stickleback of similar age (Heller, 1871; Fatio, 1882) so why are there striking differences in stickleback abundance between the two? During peak eutrophication in the 1980's stickleback were similarly abundant in both lakes (Laurent, 1966, 1972; Numann, 1972), but this is no longer the case. It is only after re-oligotrophication that Geneva populations have declined, while Constance populations have become hyper-abundant again (Alexander et al., 2016). As the ecosystems are similar in some respects, such as lake depth, size, and community composition, and the time since colonization is roughly the same, differences between the colonizing lineages in phenotype, genetic makeup, or ecology may be responsible for this pattern.
As discussed above, we know that stickleback populations in Switzerland are made up of several divergent European lineages that vary in their evolutionary history in freshwater, and that these lineages differ both phenotypically and genotypically (Mäkinen and Merilä, 2008; Lucek et al., 2010; Moser et al., 2012; Fang et al., 2018, 2020b; Marques et al., 2019a). Stickleback in Geneva are genetically dominated by a freshwater lineage from the middle Rhône (Mäkinen and Merilä, 2008; Marques et al., 2016, 2019a; Fang et al., 2018) while those in Constance most likely originate from the Baltic Sea drainage of Eastern Europe (Lucek et al., 2010). The Geneva population is phenotypically similar to West European stream stickleback, while the Constance population has a pelagic phenotype and is fully plated. Gene flow into Lake Geneva from the East European lineage has recently introduced the fully plated Ectodysplasin (Eda) allele into the population of Lake Geneva where it seems to be under positive selection in the lake but not in the streams (Lucek et al., 2014a). This introgression of the fully plated Eda allele has likely occurred through the large hybrid zone that spans the Swiss plateau between Lake Constance and Lake Geneva, and here we present new data on stickleback populations genotyped for the STN382 allele (Figure 6) as in Lucek et al. (2010) using the protocols of Colosimo et al. (2005). The spread of this allele suggests that genetic contributions from the East European lineage may increasingly permit adaptation to exploitation of pelagic habitats in other invaded lakes as well (Lucek et al., 2014a). Conversely, introgression from the West European lineage (largely from Rhine populations) into Lake Constance has been found among stream populations (Marques et al., 2019a), but not in the lake population. Thus, it is likely that we are observing an inverse scenario of invasion and secondary contact between the two lakes, lineages, and freshwater habitat types.
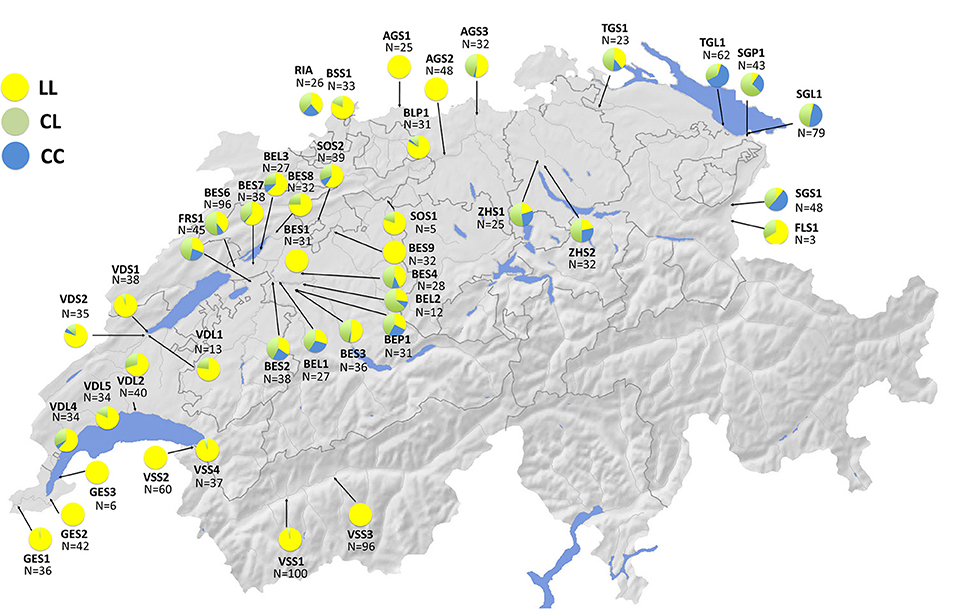
Figure 6. Ectodysplasin (Eda) STN382 allele frequency distributions across Swiss stickleback (n = 1,598) populations (n = 42) generated by KL as in Lucek et al. (2010). Yellow (LL) = homozygous for the low plated allele; Green (CL) = heterozygous individuals; Blue (CC) = homozygous for the complete plated allele. Information on site codes and capture locations can be found in Supplementary Table 2.
When we compare these two lakes, genetic constraints (e.g., limiting genetic variation due to drift at the invasion front or the lack of required adaptive genetic variation for the colonization of a specific habitat) may have limited the invasiveness of each introduced population to colonize multiple habitat types initially, but this constraint was alleviated by hybridization at each invasion front (Lucek et al., 2014a; Marques et al., 2019a). In other words, we have evidence that hybridization between East and West European lineages in Lake Constance has enabled the colonization of stream habitats (Marques et al., 2019a), but we know much less about how introgression of Eastern European alleles might facilitate a habitat expansion of the Lake Geneva population from littoral habitats into the pelagic zone. Further testing of this phenomenon within other Swiss lakes in the hybrid zone (e.g., Lakes Biel and Neuchâtel) could be fruitful, and may reveal similar patterns.
Conclusions
Invasive species allow us to observe the process of evolution on an ecological timescale, as non-native organisms adapt to new environmental conditions. Whether they are ecologically detrimental or not, we can use invasions to explore how organisms from one genetic background perform in an environmental circumstance that differs from that at its origin. Multiple introductions and admixture through hybridization can provide new genetic material for selection to act upon, and teach us about the consequences of secondary contact for adaptation, divergence and associated ecosystem impact. Here we have a system with multiple recent introductions, rapid population divergence, and the potential for large ecosystem effects in an area that has already experienced intense human habitat alteration. The Swiss stickleback system provides a model to study multiple axes of ecological diversification in threespine stickleback, and insights from this system can be applied both to the global threespine stickleback radiation in particular, and to our understanding of invasive species in general. We observe rapid parallel diversification along the lake-stream ecotype axis, and threespine stickleback have become hyper-abundant within the pelagic zone of Lake Constance, now representing one of the most common species in the lake. Whether the lacustrine population has begun to diversify into genetically distinct groups along the benthic-limnetic or lake resident-migratory axes, additional to the lake-stream axis is currently unknown and remains to be investigated. Furthermore, as hybridization has occurred between the same introduced stickleback lineages in other regions of Switzerland, we may see adaptive population divergence and possibly the evolution of invasiveness in other Swiss lakes as well.
Author Contributions
OS started the long-term research on invasion biology of stickleback in Switzerland and on the ecology, genomics of hybridization, and ecotype formation in Lake Constance. OS, BM, CH, and TA conceived of the review. KL, DM, MM, TA, BM, and OS collected data and assisted CH in producing figures and tables. CH analyzed the data and led the writing of the manuscript with assistance from BM, KL, DM, TA, and OS. All authors provided critical feedback and helped shape the research, analysis, and manuscript.
Funding
This study was supported by the Swiss Federal Office for the Environment and Eawag (Eawag Discretionary Funds 2018-2022). Our research was further supported/co-financed by the grant SeeWandel: Life in Lake Constance - the past, present, and future within the framework of the Interreg V programme Alpenrhein-Bodensee-Hochrhein (Germany/Austria/Switzerland/Liechtenstein) which funds are provided by the European Regional Development Fund as well as the Swiss Confederation and cantons. Data collection was partly funded by the Eawag Strategic Project AquaDiverse to OS and Swiss National Science Foundation grants PDFMP3_134657 to OS and 31003A_175614 to BM. The funders had no role in study design, data collection and analysis, decision to publish, or preparation of the manuscript.
Conflict of Interest
The authors declare that the research was conducted in the absence of any commercial or financial relationships that could be construed as a potential conflict of interest.
Acknowledgments
We would like to thank Sophia Hendrikx for her assistance in locating some of the historical ichthyology documents; Jun Kitano, Simone Des Roches, and Tom Reimchen for suggesting references and sharing data; Ralf Thiel and Irena Eidus at the University of Hamburg for sending us preserved museum specimens from Lake Constance; the Natural History Museum of Bern for access to preserved specimens in the Steinmann collection; the Project Lac team led by Pascal Vonlanthen and Guy Periat for sampling Lake Constance; Rebecca Best for compiling the initial dataset for the Projet Lac samples; the Eawag FishEc discussion group for conversations about the structure and flow of the manuscript, as well as Angelina Arquint, Dominique Stadler, Doris Hohmann, Jaime Anaya-Rojas, Aloïs Denervaud, and Corrina Brunner for technical support in processing Projet Lac stickleback samples. We would also like to thank a helpful internet stranger for assistance with translating Latin texts from historical documents, and Kimberley Lemmen for her help producing figures.
Supplementary Material
The Supplementary Material for this article can be found online at: https://www.frontiersin.org/articles/10.3389/fevo.2020.611672/full#supplementary-material
References
Abbott, R., Albach, D., Ansell, S., Arntzen, J. W., Baird, S. J. E., Bierne, N., et al. (2013). Hybridization and speciation. J. Evol. Biol. 26, 229–246. doi: 10.1111/j.1420-9101.2012.02599.x
Adachi, T., Ishikawa, A., Mori, S., Makino, W., Kume, M., Kawata, M., et al. (2012). Shifts in morphology and diet of non-native sticklebacks introduced into Japanese crater lakes. Ecol. Evol. 2, 1083–1098. doi: 10.1002/ece3.234
Ahnelt, H. (1986). Zum Vorkommen des Dreistachligen Stichlings ( Gasterosteus aculeatus, Pisces: Gasterosteidae) im österreichischen Donauraum. Ann. Nat. Hist. Mus. Wien Ser. B Bot. Zool. 88, 309–314.
Ahnelt, H., and Amann, E. (1994). Gasterosteus aculeatus (Pisces, Gasterosteidae) in Österreich–eine Lanze brechen für den Dreistacheligen Stichling. Öst. Fischerei 47, 19–23.
Ahnelt, H., Pohl, H., Hilgers, H., and Splechtna, H. (1998). The threespine stickleback in Austria (Gasterosteus aculeatus L., pisces: gasterosteidae) - morphological variations. Ann. Nat. Hist. Mus. Wien Ser. B Bot. Zool. 100, 395–404.
Alexander, T. J., Vonlanthen, P., Périat, G., Raymond, J., Degiorgi, F., and Seehausen, O. (2016). Artenvielfalt und Zusammensetzung der Fischpopulation im Bodensee. Kastanienbaum: Projet Lac, Eawag.
Altman, D. G., Machin, D., Bryant, T. N., and Gardner, M. (2013). Statistics with Confidence: Confidence Intervals and Statistical Guidelines. 2nd Edn. New Jersey, NJ: John Wiley and Sons.
Anaya-Rojas, J. M., Brunner, F. S., Sommer, N., Seehausen, O., Eizaguirre, C., and Matthews, B. (2016). The association of feeding behaviour with the resistance and tolerance to parasites in recently diverged sticklebacks. J. Evol. Biol. 29, 2157–2167. doi: 10.1111/jeb.12934
Anderson, E., and Stebbins, G. L Jr. (1954). Hybridization as an evolutionary stimulus. Evolution 8, 378–388. doi: 10.1111/j.1558-5646.1954.tb01504.x
Andreou, D., Eizaguirre, C., Boehm, T., and Milinski, M. (2017). Mate choice in sticklebacks reveals that immunogenes can drive ecological speciation. Behav. Ecol. 28, 953–961. doi: 10.1093/beheco/arx074
Arnegard, M. E., McGee, M. D., Matthews, B., Marchinko, K. B., Conte, G. L., Kabir, S., et al. (2014). Genetics of ecological divergence during speciation. Nature 511, 307–311. doi: 10.1038/nature13301
Arts, M. T., Brett, M. T., and Kainz, M. (2009). Lipids in Aquatic Ecosystems. New York, NY: Springer Science & Business Media.
Baker, J. A. (1994). Life History Variation in Female Threespine Stickleback. The Evolutionary Biology of the Threespine Stickleback. Available online at: https://ci.nii.ac.jp/naid/10015006230/ (accessed December 9, 2019).
Baker, J. A., Wund, M. A., Heins, D. C., King, R. W., Reyes, M. L., and Foster, S. A. (2015). Life-history plasticity in female threespine stickleback. Heredity 115, 322–334. doi: 10.1038/hdy.2015.65
Baker, J. A., Cresko, W. A., Foster, S. A., and Heins, D. C. (2005). Life-history differentiation of benthic and limnetic ecotypes in a polytypic population of threespine stickleback (Gasterosteus aculeatus). Evol. Ecol. Res. 7, 121–131.
Balon, E. K. (1967). Three-spined stickleback–Gasterousteus aculeatus Linnaeus, 1758 in the Danube near Bratislava. Ac. Rer. Nat. Mus. Nat. Slov. 13, 127–134.
Bańbura, J. (1994). Lateral plate morph differentiation of freshwater and marine populations of the three-spined stickleback, Gasterosteus aculeatus, in Poland. J. Fish Biol. 44, 773–783. doi: 10.1111/j.1095-8649.1994.tb01254.x
Barluenga, M., Sanetra, M., and Meyer, A. (2006). Genetic admixture of burbot (Teleostei: Lota lota) in lake constance from two European glacial refugia. Mol. Ecol. 15, 3583–3600. doi: 10.1111/j.1365-294X.2006.03045.x
Barrett, R., and Schluter, D. (2008). Adaptation from standing genetic variation. Trends Ecol. Evol. 23, 38–44. doi: 10.1016/j.tree.2007.09.008
Barrett, R. D. H. (2010). Adaptive evolution of lateral plates in three-spined stickleback Gasterosteus aculeatus: a case study in functional analysis of natural variation. J. Fish Biol. 77, 311–328. doi: 10.1111/j.1095-8649.2010.02640.x
Barrett, R. D. H., Rogers, S. M., and Schluter, D. (2008). Natural selection on a major armor gene in threespine stickleback. Science 322, 255–257. doi: 10.1126/science.1159978
Barrett, S. C. H., and Husband, B. C. (1990). Variation in outcrossing rates in Eichhornia paniculata: the role of demographic and reproductive factors. Plant Species Biol. 5, 41–55. doi: 10.1111/j.1442-1984.1990.tb00191.x
Barton, N. H. (2001). The role of hybridization in evolution. Mol. Ecol. 10, 551–568. doi: 10.1046/j.1365-294x.2001.01216.x
Bassham, S., Catchen, J., Lescak, E., and von Hippel, F. A. (2018). Repeated selection of alternatively adapted haplotypes creates sweeping genomic remodeling in stickleback. Genetics 209, 921–939.doi: 10.1534/genetics.117.300610
Behrmann-Godel, J., Gerlach, G., and Eckmann, R. (2004). Postglacial colonization shows evidence for sympatric population splitting of Eurasian perch (Perca fluviatilis L.) in lake constance. Mol. Ecol. 13, 491–497. doi: 10.1046/j.1365-294X.2003.02082.x
Bell, M. A. (1974). Reduction and Loss of the Pelvic Girdle in Gasterosteus (Pisces): A Case of Parallel Evolution. Los Angeles, CA: Natural History Museum.
Bell, M. A. (1977). A late miocene marine threespine stickleback, Gasterosteus aculeatus aculeatus, and its zoogeographic and evolutionary significance. Copeia 1977, 277–282. doi: 10.2307/1443909
Bell, M. A. (1984). Gigantism in threespine sticklebacks: implications for causation of body size evolution. Copeia 1984, 530–534. doi: 10.2307/1445211
Bell, M. A. (1995). Intraspecific systematics of Gasterosteus aculeatus populations: implications for behavioral ecology. Behaviour 132, 1131–1152. doi: 10.1163/156853995X00496
Bell, M. A., and Haglund, T. R. (1982). Fine-scale temporal variation of the Miocene stickleback Gasterosteus doryssus. Paleobiology 8, 282–292. doi: 10.1017/S0094837300006990
Bell, M. A., Stewart, J. D., and Park, P. J. (2009). The world's oldest fossil threespine stickleback fish. Copeia 2009, 256–265. doi: 10.1643/CG-08-059
Bell, M. A., Travis, M. P., and Max Blouw, D. (2006). Inferring natural selection in a fossil threespine stickleback. Paleobiology 32, 562–577. doi: 10.1666/05026.1
Bentzen, P., and McPhail, J. D. (1984). Ecology and evolution of sympatric sticklebacks (Gasterosteus): specialization for alternative trophic niches in the Enos Lake species pair. Can. J. Zool. 62, 2280–2286. doi: 10.1139/z84-331
Bergersen, R. (1996). Sticklebacks from Greenland. J. Fish Biol. 48, 799–801. doi: 10.1111/j.1095-8649.1996.tb01474.x
Bergström, U., Olsson, J., Casini, M., Eriksson, B. K., Fredriksson, R., Wennhage, H., et al. (2015). Stickleback increase in the Baltic Sea – a thorny issue for coastal predatory fish. Estuar. Coast. Shelf Sci. 163, 134–142. doi: 10.1016/j.ecss.2015.06.017
Berinkey, L. (1960). The stickleback (Gasterosteus aculeatus L.), a new fish species from Hungary. Verte. Hung. Musei Histori. Nat. Hung. 2, 1–10.
Bernatchez, L. (2001). The evolutionary history of brown trought (Salmo trutta L.) inferred from phylogeographic, nested clade, and mismatch analyses of mitochondrial DNA variation. Evolution 55, 351–379. doi: 10.1554/0014-3820(2001)055[0351:TEHOBT]2.0.CO;2
Berner, D., Ammann, M., Spencer, E., Rüegg, A., Lüscher, D., and Moser, D. (2017). Sexual isolation promotes divergence between parapatric lake and stream stickleback. J. Evol. Biol. 30, 401–411. doi: 10.1111/jeb.13016
Berner, D., and Grandchamp, A. C. (2009). Variable progress toward ecological speciation in parapatry: stickleback across eight lake-stream transitions. Evolution 63, 1740–1753. doi: 10.1111/j.1558-5646.2009.00665.x
Berner, D., Roesti, M., Hendry, A. P., and Salzburger, W. (2010). Constraints on speciation suggested by comparing lake-stream stickleback divergence across two continents. Mol. Ecol. 19, 4963–4978. doi: 10.1111/j.1365-294X.2010.04858.x
Bertin, L. (1925). Recherches Bionomiques, Biométriques et Systématiques sur les Épinoches Gastérostéidés), Par Léon. Bertin: E. Blondel La Rougery.
Best, R. J., Anaya-Rojas, J. M., Leal, M. C., Schmid, D. W., Seehausen, O., and Matthews, B. (2017). Transgenerational selection driven by divergent ecological impacts of hybridizing lineages. Nat. Ecol. Evol. 1, 1757–1765. doi: 10.1038/s41559-017-0308-2
Blanc, H. (1922). L'apparition dans le lac Léman de l'Epinoche à queue lisse (Gasterosteus aculeatus L. varietas : G. gymnurus). Actes Soc. Helvétiq. Sci. Nat. 103, 252–253.
Blouw, D. M. (1996). Evolution of offspring desertion in a stickleback fish. Écoscience 3, 18–24. doi: 10.1080/11956860.1996.11682310
Blouw, D. M., and Hagen, D. W. (1990). Breeding ecology and evidence of reproductive isolation of a widespread stickleback fish (Gasterosteidae) in Nova Scotia, Canada. Biol. J. Linn. Soc. Lond. 39, 195–217. doi: 10.1111/j.1095-8312.1990.tb00512.x
Bolnick, D. I. (2004). Can intraspecific competition drive disruptive selection? An experimental test in natural populations of sticklebacks. Evolution 58, 608–618. doi: 10.1111/j.0014-3820.2004.tb01683.x
Bolnick, D. I. (2011). Sympatric speciation in threespine stickleback: why not? Int. J. Ecol. 2011:942847. doi: 10.1155/2011/942847
Boughman, J. W. (2001). Divergent sexual selection enhances reproductive isolation in sticklebacks. Nature 411, 944–948. doi: 10.1038/35082064
Byström, P., Bergström, U., Hjälten, A., Ståhl, S., Jonsson, D., and Olsson, J. (2015). Declining coastal piscivore populations in the Baltic Sea: where and when do sticklebacks matter? Ambio 44(Suppl. 3), 462–471. doi: 10.1007/s13280-015-0665-5
Cakić, P. D., Lenhardt, M., and Petrović, Z. (2000). The first record of Gasterosteus aculeatus L. 1758 (Pisces: Gasterosteidae) in the Yugoslav section of Danube. Acta Biol. Iugoslavica-Serija E Ichtyol. 32, 79–82.
Campbell, R. N. (1985). Morphological variation in the three-spined stickleback (Gasterosteus aculeatus) in Scotland. Behaviour 93, 161–168. doi: 10.1163/156853986X00838
Cano, J. M., Mäkinen, H. S., Leinonen, T., Freyhof, J., and Merilä, J. (2008). Extreme neutral genetic and morphological divergence supports classification of Adriatic three-spined stickleback (Gasterosteus aculeatus) populations as distinct conservation units. Biol. Conserv. 141, 1055–1066. doi: 10.1016/j.biocon.2008.01.015
Castro, L. F. C., Monroig, Ó., Leaver, M. J., Wilson, J., Cunha, I., and Tocher, D. R. (2012). Functional desaturase Fads1 (Δ5) and Fads2 (Δ6) orthologues evolved before the origin of jawed vertebrates. PLoS ONE 7:e31950. doi: 10.1371/journal.pone.0031950
Chan, Y. F., Marks, M. E., Jones, F. C., Villarreal, G. Jr, Shapiro, M. D., Brady, S. D., et al. (2010). Adaptive evolution of pelvic reduction in sticklebacks by recurrent deletion of a Pitx1 enhancer. Science 327, 302–305. doi: 10.1126/science.1182213
Colosimo, P. F., Hosemann, K. E., Balabhadra, S., Villarreal, G. Jr, Dickson, M., Grimwood, J., et al. (2005). Widespread parallel evolution in sticklebacks by repeated fixation of ectodysplasin alleles. Science 307, 1928–1933. doi: 10.1126/science.1107239
Cresko, W. A., Amores, A., Wilson, C., Murphy, J., Currey, M., Phillips, P., et al. (2004). Parallel genetic basis for repeated evolution of armor loss in Alaskan threespine stickleback populations. Proc. Natl. Acad. Sci. U.S.A. 101, 6050–6055. doi: 10.1073/pnas.0308479101
Cuvier, G., and Valenciennes, M. (1828). Histoire Naturelle des Poissons. Paris: Chez F. G. Levrault.
Cuvier, G. (1829). Le règne Animal Distribué D'après son Organisation Pour servir à L'histoire Naturelle des Animaux et D'introduction à L'anatomie Comparée. Paris: Deterville.
Deagle, B. E., Jones, F. C., Chan, Y. F., Absher, D. M., Kingsley, D. M., and Reimchen, T. E. (2012). Population genomics of parallel phenotypic evolution in stickleback across stream-lake ecological transitions. Proc. Biol. Sci. 279, 1277–1286. doi: 10.1098/rspb.2011.1552
Dean, L. L., Magalhaes, I. S., Foote, A., D'Agostino, D., McGowan, S., and MacColl, A. D. C. (2019). Admixture between ancient lineages, selection, and the formation of sympatric stickleback species-pairs. Mol. Biol. Evol. 36, 2481–2497. doi: 10.1093/molbev/msz161
deFaveri, J., Jonsson, P. R., and Merilä, J. (2013). Heterogeneous genomic differentiation in marine threespine sticklebacks: adaptation along an environmental gradient. Evolution 67, 2530–2546. doi: 10.1111/evo.12097
deFaveri, J., and Merilä, J. (2013). Variation in age and size in Fennoscandian three-spined sticklebacks (Gasterosteus aculeatus). PLoS ONE 8:e80866. doi: 10.1371/journal.pone.0080866
deFaveri, J., and Merilä, J. (2014). Local adaptation to salinity in the three-spined stickleback? J. Evol. Biol. 27, 290–302. doi: 10.1111/jeb.12289
DeFaveri, J., Zanella, L. N., Zanella, D., Mrakovčić, M., and Merilä, J. (2012). Phylogeography of isolated freshwater three-spined stickleback Gasterosteus aculeatus populations in the Adriatic Sea basin. J. Fish Biol. 80, 61–85. doi: 10.1111/j.1095-8649.2011.03147.x
Delbeek, J. C., and Williams, D. D. (1988). Feeding selectivity of four species of sympatric stickleback in brackish-water habitats in eastern Canada. J. Fish Biol. 32, 41–62. doi: 10.1111/j.1095-8649.1988.tb05334.x
Denys, G. P. J., GeiGer, M. F., Persat, H., Keit,H, P., and Dettai, A. (2015). Invalidity of Gasterosteus gymnurus (Cuvier, 1829) (Actinopterygii, Gasterosteidae) according to integrative taxonomy. Cybium 39, 37–45. doi: 10.26028/cybium/2015-391-005
Deufel, J. (1985). Am Beispiel des Bodensees: Lohnt sich der Einstaz fremder Fischarten? (Taking Lake Constance as an example: is stocking foreign fish species worth it?) Fisch Fang 4:1985.
Doebeli, M., and Dieckmann, U. (2003). Speciation along environmental gradients. Nature 421, 259–264. doi: 10.1038/nature01274
Doucette, L. I., Skúlason, S., and Snorrason, S. S. (2003). Risk of predation as a promoting factor of species divergence in threespine sticklebacks (Gasterosteus aculeatus L.). Biol. J. Linn. Soc. Lond. 82, 189–203. doi: 10.1111/j.1095-8312.2004.00323.x
Eckmann, R., and Engesser, B. (2018). Reconstructing the build-up of a pelagic stickleback (Gasterosteus aculeatus) population using hydroacoustics. Fish. Res. 210, 189–192. doi: 10.1016/j.fishres.2018.08.002
Eckmann, R., and Imbrock, F. (1996). Distribution and diel vertical migration of Eurasian perch (Perca fluviatilis L.) during winter. Ann. Zool. Fennici 33, 679–686.
Edelaar, P., and Bolnick, D. I. (2012). Non-random gene flow: an underappreciated force in evolution and ecology. Trends Ecol. Evol. 27, 659–665. doi: 10.1016/j.tree.2012.07.009
Edelaar, P., Siepielski, A. M., and Clobert, J. (2008). Matching habitat choice causes directed gene flow: a neglected dimension in evolution and ecology. Evolution 62, 2462–2472. doi: 10.1111/j.1558-5646.2008.00459.x
Ehrlich, P. (1989). Attributes of invaders and the invading processes vertebrates. Biol. Invasions 315–328.
Eizaguirre, C., Lenz, T. L., Sommerfeld, R. D., Harrod, C., Kalbe, M., and Milinski, M. (2011). Parasite diversity, patterns of MHC II variation and olfactory based mate choice in diverging three-spined stickleback ecotypes. Evol. Ecol. 25, 605–622. doi: 10.1007/s10682-010-9424-z
Eklöf, J. S., Sundblad, G., Erlandsson, M., Donadi, S., Hansen, J. P., Eriksson, B. K., et al. (2020). A spatial regime shift from predator to prey dominance in a large coastal ecosystem. Commun Biol. 3:459. doi: 10.1038/s42003-020-01180-0
Fang, B., Kemppainen, P., Momigliano, P., Feng, X., and Merilä, J. (2020a). On the causes of geographically heterogeneous parallel evolution in sticklebacks. Nat Ecol Evol. 4, 1105–1115. doi: 10.1038/s41559-020-1222-6
Fang, B., Merilä, J., Matschiner, M., and Momigliano, P. (2020b). Estimating uncertainty in divergence times among three-spined stickleback clades using the multispecies coalescent. Mol. Phylogenet. Evol. 142:106646. doi: 10.1016/j.ympev.2019.106646
Fang, B., Merilä, J., Ribeiro, F., Alexandre, C. M., and Momigliano, P. (2018). Worldwide phylogeny of three-spined sticklebacks. Mol. Phylogenet. Evol. 127, 613–625. doi: 10.1016/j.ympev.2018.06.008
Fatio, V. (1882). “Faunes des Vertebres de la Suisse. 4, Poissons 1. Genke et Bue,” in Geographical Variation in the Life History Tactics of Some Species of Freshwater Fish. In'Fish Reproduction: Strategies and Tactics', eds G. W. Potts, and R. J. Wootton (London: Academic Press), 171–186. doi: 10.5962/bhl.title.124573
Fehling, H. V., Fraas, O., Krauss, F. V, and Zech, P. V. (1881). Württembergische Naturwissenschaftliche Jahreshefte. Stuttgart: E. Schweizerbart'sche Verlagshandlung
Feller, A. F., Seehausen, O., Lucek, K. J. O., and Marques, D. A. (2016). Habitat choice and female preference in a polymorphic stickleback population. Evol. Ecol. Res. 17, 419–435. doi: 10.7892/boris.79067
Feulner, P. G. D., Chain, F. J. J., Panchal, M., Huang, Y., Eizaguirre, C., Kalbe, M., et al. (2015). Genomics of divergence along a continuum of parapatric population differentiation. PLoS Genet. 11:e1004966. doi: 10.1371/journal.pgen.1004966
Flamarique, I. N., Cheng, C. L., Bergstrom, C., and Reimchen, T. E. (2013). Pronounced heritable variation and limited phenotypic plasticity in visual pigments and opsin expression of threespine stickleback photoreceptors. J. Exp. Biol. 216, 656–667. doi: 10.1242/jeb.078840
Foster, S. A., and Bell, M. A. (1994). The Evolutionary Biology of the Threespine Stickleback. Oxford: Oxford University Press.
Frankham, R. (2005). Resolving the genetic paradox in invasive species. Heredity 94:385. doi: 10.1038/sj.hdy.6800634
Gaedke, U., and Schweizer, A. (1993). The first decade of oligotrophication in Lake Constance. Oecologia 93, 268–275. doi: 10.1007/BF00317681
Gambling, S. J., and Reimchen, T. E. (2012). Prolonged life span among endemic Gasterosteus populations. Can. J. Zool. 90, 284–290. doi: 10.1139/z11-133
Gaschott, O. (1941). Die Stichlinge (Gasterosteidae). In Handbuch der Binnenfischerei Mitteleuropas III A. eds R. Demoll, and H. N. Maier (Stuttgart: Schweizerbart), 129–141.
Gaye-Siessegger, J. (2014). The great Cormorant (Phalacrocorax carbo) at lower lake constance/Germany: dietary composition and impact on commercial fisheries. Knowl. Manag. Aquat. Ecosyst. 04:12. doi: 10.1051/kmae/2014015
Gessner, C. (1558). “De piscium et aquatilium animantium natura (On fish and other aquatic animals),” in Bibliopolio Andreæ Cambieri. (Zurich: Henricus Laurentius), 8–9.
Giles, N. (1983). The possible role of environmental calcum levels during the evolution of phenotypic diversity in outer hebridean populations of the three-spined stickleback, Gasterosteus aculeatus. J. Zool. 199, 535–544. doi: 10.1111/j.1469-7998.1983.tb05104.x
Gökaşan, E., Demirbag, E., Oktay, F. Y., Ecevitog, B., Simşek, M., Yüce, H., et al. (1997). On the origin of the Bosphorus. Mar. Geol. 140, 183–199. doi: 10.1016/S0025-3227(97)00022-4
Göldi, E. A. (1914). Die Tierwelt der Schweiz in der Gegenward und in der Vergangenheit. Bern: A. Francke. doi: 10.5962/bhl.title.132942
Gordon, W. J. (1902). Our Country's Fishes and How to Know Them: A Guide to All the Fishes of Great Britain. London: Simpkin, Hamilton, Kent & Co.
Gotceitas, V., and Brown, J. A. (1993). Risk of predation to fish larvae in the presence of alternative prey: effects of prey size and number. Marine Ecol. Prog. Series 98, 215–215. doi: 10.3354/meps098215
Gouskov, A., and Vorburger, C. (2016). Postglacial recolonizations, watershed crossings and human translocations shape the distribution of chub lineages around the Swiss Alps. BMC Evol. Biol. 16:185. doi: 10.1186/s12862-016-0750-9
Gow, J. L., Peichel, C. L., and Taylor, E. B. (2007). Ecological selection against hybrids in natural populations of sympatric threespine sticklebacks. J. Evol. Biol. 20, 2173–2180. doi: 10.1111/j.1420-9101.2007.01427.x
Gow, J. L., Rogers, S. M., Jackson, M., and Schluter, D. (2008). Ecological predictions lead to the discovery of a benthic–limnetic sympatric species pair of threespine stickleback in Little Quarry Lake, British Columbia. Can. J. Zool. 86, 564–571. doi: 10.1139/Z08-032
Greenbank, J., and Nelson, P. R. (1959). Life history of the threespine stickleback Gasterosteus aculeatus Linnaeus in Karluk Lake and Bare Lake. Kodiak Island, AK: US Government Printing Office.
Gum, B., Gross, R., and Kuehn, R. (2005). Mitochondrial and nuclear DNA phylogeography of European grayling (Thymallus thymallus): evidence for secondary contact zones in central Europe. Mol. Ecol. 14, 1707–1725. doi: 10.1111/j.1365-294X.2005.02520.x
Guo, B., DeFaveri, J., Sotelo, G., Nair, A., and Merilä, J. (2015). Population genomic evidence for adaptive differentiation in Baltic Sea three-spined sticklebacks. BMC Biol. 13:19. doi: 10.1186/s12915-015-0130-8
Gygax, M., Rentsch, A. K., Rudman, S. M., and Rennison, D. J. (2018). Differential predation alters pigmentation in threespine stickleback (Gasterosteus aculeatus). J. Evol. Biol. 31, 1589–1598. doi: 10.1111/jeb.13354
Haenel, Q., Roesti, M., Moser, D., and MacColl, A. D. C. (2019). Predictable genome-wide sorting of standing genetic variation during parallel adaptation to basic versus acidic environments in stickleback fish. Evolution 3, 28–42. doi: 10.1002/evl3.99
Hagen, D. W., and Moodie, G. E. E. (1979). Polymorphism for breeding colors in Gasterosteus aculeatus. I. their genetics and geographic distribution. Evolution 33, 641–648. doi: 10.1111/j.1558-5646.1979.tb04717.x
Haglund, T. R., Buth, D. G., and Blouw, D. M. (1990). Allozyme variation and the recognition of the “white stickleback.” Biochem. Syst. Ecol. 18, 559–563. doi: 10.1016/0305-1978(90)90129-4
Haley, A. L., Dalziel, A. C., and Weir, L. K. (2019). A comparison of nuptial coloration and breeding behaviour in white and common marine threespine stickleback (Gasterosteus aculeatus) ecotypes. Evol. Ecol. Res. 20, 145–166.
Hanson, D., Hu, J., Hendry, A. P., and Barrett, R. D. H. (2017). Heritable gene expression differences between lake and stream stickleback include both parallel and antiparallel components. Heredity 119, 339–348. doi: 10.1038/hdy.2017.50
Harvey, C. J., Ruggerone, G. T., and Rogers, D. E. (1997). Migrations of three-spined stickleback, nine-spined stickleback, and pond smelt in the Chignik catchment, Alaska. J. Fish Biol. 50, 1133–1137. doi: 10.1111/j.1095-8649.1997.tb01639.x
Hatfield, T., and Schluter, D. (1999). Ecological speciation in sticklebacks: environment-dependent hybrid fitness. Evolution 53, 866–873. doi: 10.1111/j.1558-5646.1999.tb05380.x
Head, M. L., Kozak, G. M., and Boughman, J. W. (2013). Female mate preferences for male body size and shape promote sexual isolation in threespine sticklebacks. Ecol. Evol. 3, 2183–2196. doi: 10.1002/ece3.631
Hendry, A. P., Bolnick, D. I., Berner, D., and Peichel, C. L. (2009). Along the speciation continuum in sticklebacks. J. Fish Biol. 75, 2000–2036. doi: 10.1111/j.1095-8649.2009.02419.x
Hendry, A. P., Nosil, P., and Rieseberg, L. H. (2007). The speed of ecological speciation. Funct. Ecol. 21, 455–464. doi: 10.1111/j.1365-2435.2007.01240.x
Hendry, A. P., Peichel, C. L., Matthews, B., Boughman, J. W., and Nosil, P. (2013). Stickleback research: the now and the next. Evol. Ecol. Res. 15, 111–141.
Hendry, A. P., and Taylor, E. B. (2004). How much of the variation in adaptive divergence can be explained by gene flow? An evaluation using lake-stream stickleback pairs. Evolution 58, 2319–2331. doi: 10.1111/j.0014-3820.2004.tb01606.x
Hendry, A. P., Taylor, E. B., and McPhail, J. D. (2002). Adaptive divergence and the balance between selection and gene flow: lake and stream stickleback in the Misty system. Evolution 56, 1199–1216. doi: 10.1111/j.0014-3820.2002.tb01432.x
Holcik, J. (2003). Changes in the fish fauna and fisheries in the Slovak section of the Danube River: a review. Ann. Limnol. Int. J. Lim. 39, 177–195. doi: 10.1051/limn/2003015
Hosoki, T., Mori, S., Nishida, S., Kume, M., Sumi, T., and Kitano, J. (2019). Diversity of gill raker number and diets among stickleback populations in novel habitats created by the 2011 Tohoku earthquake and tsunami. Evol. Ecol. Res. 20, 213–230.
Hötzl, H. (1996). Origin of the Danube-Aach system. Environ. Geol. 27, 87–96. doi: 10.1007/s002540050034
Hudson, A. G., Vonlanthen, P., and Seehausen, O. (2014). Population structure, inbreeding and local adaptation within an endangered riverine specialist: the nase (Chondrostoma nasus). Conserv. Genet. 15, 933–951. doi: 10.1007/s10592-014-0590-3
Hyatt, K. D., and Ringler, N. H. (1989). Egg cannibalism and the reproductive strategies of threespine sticklebacks (Gasterosteus aculeatus) in a coastal British Columbia lake. Can. J. Zool. 67, 2036–2046. doi: 10.1139/z89-290
Hynes, H. B. N. (1950). The food of fresh-water sticklebacks (Gasterosteus aculeatus and Pygosteus pungitius), with a review of methods used in studies of the food of fishes. J. Anim. Ecol. 19, 36–58. doi: 10.2307/1570
IGKB, I. G. F., and den, B. (2018). Jahresbericht der Internationalen Gewässerschutzkommission für den Bodensee: Limnologischer Zustand des Bodensees Nr. 42 (2016–2017). Available online at: https://www.igkb.org/fileadmin/user_upload/dokumente/publikationen/gruene_berichte/42_gb42gesamtbericht.pdf.
Ishikawa, A., Kabeya, N., Ikeya, K., Kakioka, R., Cech, J. N., Osada, N., et al. (2019). A key metabolic gene for recurrent freshwater colonization and radiation in fishes. Science 364, 886–889. doi: 10.1126/science.aau5656
Jamieson, I. G., Blouw, D. M., and Colgan, P. W. (1992a). Field observations on the reproductive biology of a newly discovered stickleback (Gasterosteus). Can. J. Zool. 70, 1057–1063. doi: 10.1139/z92-148
Jamieson, I. G., Blouw, D. M., and Colgan, P. W. (1992b). Parental care as a constraint on male mating success in fishes: a comparative study of threespine and white sticklebacks. Can. J. Zool. 70, 956–962. doi: 10.1139/z92-136
Jenck, C. S., Lehto, W. R., Ketterman, B. T., Sloan, L. F., Sexton, A. N., and Tinghitella, R. M. (2020). Phenotypic divergence among threespine stickleback that differ in nuptial coloration. Ecol. Evol. 10, 2900–2916. doi: 10.1002/ece3.6105
Jeppesen, E., Lauridsen, T. L., Christoffersen, K. S., Landkildehus, F., Geertz-Hansen, P., Amsinck, S. L., et al. (2017). The structuring role of fish in Greenland lakes: an overview based on contemporary and paleoecological studies of 87 lakes from the low and the high Arctic. Hydrobiologia 800, 99–113. doi: 10.1007/s10750-017-3279-z
Jones, F. C., Brown, C., Pemberton, J. M., and Braithwaite, V. A. (2006). Reproductive isolation in a threespine stickleback hybrid zone. J. Evol. Biol. 19, 1531–1544. doi: 10.1111/j.1420-9101.2006.01122.x
Jones, F. C., Chan, Y. F., Schmutz, J., Grimwood, J., Brady, S. D., Southwick, A. M., et al. (2012a). A genome-wide SNP genotyping array reveals patterns of global and repeated species-pair divergence in sticklebacks. Curr. Biol. 22, 83–90. doi: 10.1016/j.cub.2011.11.045
Jones, F. C., Grabherr, M. G., Chan, Y. F., Russell, P., Mauceli, E., Johnson, J., et al. (2012b). The genomic basis of adaptive evolution in threespine sticklebacks. Nature 484, 55–61. doi: 10.1038/nature10944
Kaeuffer, R., Peichel, C. L., Bolnick, D. I., and Hendry, A. P. (2012). Parellel and nonparallel aspects of ecologcal, phenotypic, and genetic divergence across replicate population pairs of lake and stream stickleback. Evolution 66, 402–418. doi: 10.1111/j.1558-5646.2011.01440.x
Karvonen, A., Lucek, K., Marques, D. A., and Seehausen, O. (2015). Divergent macroparasite infections in parapatric swiss lake-stream pairs of threespine stickleback (Gasterosteus aculeatus). PLoS ONE 10:e0130579. doi: 10.1371/journal.pone.0130579
Kassen, R., Schluter, D., and McPhail, J. D. (1995). Evolutionary history of threespine sticklebacks (Gasterosteus spp.) in British Columbia: insights from a physiological clock. Can. J. Zool. 73, 2154–2158. doi: 10.1139/z95-252
Kean-Howie, J. C., Pearre, S., and Dickie, L. M. (1988). Experimental predation by sticklebacks on larval mackerel and protection of fish larvae by Zooplankton alternative prey. J. Exp. Mar. Biol. Ecol. 124, 239–259. doi: 10.1016/0022-0981(88)90174-8
Keller, O., and Krayss, E. (2000). Die Hydrographie des Bodenseeraums in Vergangenheit und Gegenwart. Ber. St. Gallen Nat.wiss. Ges. 89, 39–56.
Kitano, J., Bolnick, D. I., Beauchamp, D. A., Mazur, M. M., Mori, S., Nakano, T., et al. (2008). Reverse evolution of armor plates in the threespine stickleback. Curr. Biol. 18, 769–774. doi: 10.1016/j.cub.2008.04.027
Kitano, J., Ishikawa, A., Kume, M., and Mori, S. (2012). Physiological and genetic basis for variation in migratory behavior in the three-spined stickleback, Gasterosteus aculeatus. Ichthyol. Res. 59, 293–303. doi: 10.1007/s10228-012-0289-8
Kitano, J., Mori, S., and Peichel, C. L. (2007). Sexual dimorphism in the external morphology of the threespine stickleback (Gasterosteus aculeatus). Copeia 2007, 336–349. doi: 10.1643/0045-8511(2007)7[336:SDITEM]2.0.CO;2
Koch, H. J., and Heuts, M. J. (1943). Régulation osmotique, cycle sexuel et migration de reproduction chez les épinoches. Arch. Int. Physiol. 53, 253–266. doi: 10.3109/13813454309144822
Kolbe, J. J., Glor, R. E., Rodríguez Schettino, L., Lara, A. C., Larson, A., and Losos, J. B. (2004). Genetic variation increases during biological invasion by a Cuban lizard. Nature 431, 177–181. doi: 10.1038/nature02807
Kottelat, M., and Freyhof, J. (2007). Handbook of European Freshwater Fishes. Available online at: http://agris.fao.org/agris-search/search.do?recordID=US201300126031 (accessed September 05, 2020).
Kotterba, P., Kühn, C., Hammer, C., and Polte, P. (2014). Predation of threespine stickleback (Gasterosteus aculeatus) on the eggs of Atlantic herring (Clupea harengus) in a Baltic Sea lagoon. Limnol. Oceanogr. 59, 578–587. doi: 10.4319/lo.2014.59.2.0578
Krafft, C. (1874). Die Neuesten Erhebungen über den Zustände der Fisherei in den im Reichsrathe Vertretenen Königreichen und Ländern und an Oesterreichish-Ungarischen Meeresküsten (The Most Recent Surveys on the Conditions of Fishing in the Reichsrathe, Represented Kingdoms, and the Austro-Hungarian Seacoast). Weins: Kaiserlich-Königlichen hof – und Staatsdruckerei.
Kristjansson, B. K., Skulason, S., and Noakes, D. L. G. (2002). Morphological segregation of Icelandic threespine stickleback (Gasterosteus aculeatus L). Biol. J. Linn. Soc. Lond. 76, 247–257. doi: 10.1046/j.1095-8312.2002.00063.x
Kümmerlin, R. E. (1998). Taxonomical response of the phytoplankton community of upper lake constance (Bodensee-Obersee) to eutrophication and re-oligotrophication. Adv. Limnol. Stuttgart. 53, 109–117.
Lackey, A. C. R., and Boughman, J. W. (2017). Evolution of reproductive isolation in stickleback fish. Evolution 71, 357–372. doi: 10.1111/evo.13114
Laurent, P. J. (1966). Modifications biologiques récentes dans quelques lacs subalpins. Extrait. Bull. 18, 47–56.
Laurent, P. J. (1972). Lac Léman: effects of exploitation, eutrophication, and introductions on the salmonid community. J. Fish. Res. Bd. Can. 29, 867–875. doi: 10.1139/f72-130
Laurentino, T. G., Moser, D., Roesti, M., Ammann, M., Frey, A., Ronco, F., et al. (2020). Genomic release-recapture experiment in the wild reveals within-generation polygenic selection in stickleback fish. Nat. Commun. 11:1928. doi: 10.1038/s41467-020-15657-3
Lavergne, S., and Molofsky, J. (2007). Increased genetic variation and evolutionary potential drive the success of an invasive grass. Proc. Natl. Acad. Sci. U.S.A. 104, 3883–3888. doi: 10.1073/pnas.0607324104
Lavin, P. A., and Mcphail, J. D. (1993). Parapatric lake and stream sticklebacks on northern Vancouver Island: disjunct distribution or parallel evolution? Can. J. Zool. 71, 11–17. doi: 10.1139/z93-003
Lescak, E. A., and von Hippel, F. A. (2011). Selective predation of threespine stickleback by rainbow trout. Ecol. Freshw. Fish 20, 308–314. doi: 10.1111/j.1600-0633.2011.00497.x
Leuthner, F. (1877). Die mittelrheinische Fischfauna mit besonderer Berücksichtigung des Rheins bei Basel, nebst einer mit kurzen Diagnosen versehenen systematischen Übersicht zur Bestimmung der rheinischen Fische. Basel; Geneva; Lyon: H. Georg
Lisjak, D., Zanella, D., Mustafić, P., Caleta, M., Buj, I., Mihinjač, T., et al. (2015). First record of three-spined stickleback (Gasterosteus aculeatus Linnaeus, 1758) in the Daube basin of Croatia. Croat. J. Fish. 73, 70–72. doi: 10.14798/73.2.824
Lucas, M. C., Baras, E., Thom, T. J., Duncan, A., and Slavk, O. (2001). Migration of Freshwater Fishes. Oxford: Blackwell Science Ltd. doi: 10.1002/9780470999653
Lucek, K. (2016). Cryptic invasion drives phenotypic changes in central European threespine stickleback. Conserv. Genet. 17, 993–999. doi: 10.1007/s10592-016-0837-2
Lucek, K., Keller, I., Nolte, A. W., and Seehausen, O. (2018). Distinct colonization waves underlie the diversification of the freshwater sculpin (Cottus gobio) in the Central European Alpine region. J. Evol. Biol. 31, 1254–1267. doi: 10.1111/jeb.13339
Lucek, K., Lemoine, M., and Seehausen, O. (2014a). Contemporary ecotypic divergence during a recent range expansion was facilitated by adaptive introgression. J. Evol. Biol. 27, 2233–2248. doi: 10.1111/jeb.12475
Lucek, K., Roy, D., Bezault, E., Sivasundar, A., and Seehausen, O. (2010). Hybridization between distant lineages increases adaptive variation during a biological invasion: stickleback in Switzerland. Mol. Ecol. 19, 3995–4011. doi: 10.1111/j.1365-294X.2010.04781.x
Lucek, K., and Seehausen, O. (2015). Distinctive insular forms of threespine stickleback (Gasterosteus aculeatus) from western Mediterranean islands. Conserv. Genet. 16, 1319–1333. doi: 10.1007/s10592-015-0742-0
Lucek, K., Sivasundar, A., Kristjánsson, B. K., Skúlason, S., and Seehausen, O. (2014b). Quick divergence but slow convergence during ecotype formation in lake and stream stickleback pairs of variable age. J. Evol. Biol. 27, 1878–1892. doi: 10.1111/jeb.12439
Lucek, K., Sivasundar, A., Roy, D., and Seehausen, O. (2013). Repeated and predictable patterns of ecotypic differentiation during a biological invasion: lake-stream divergence in parapatric Swiss stickleback. J. Evol. Biol. 26, 2691–2709. doi: 10.1111/jeb.12267
Lucek, K., Sivasundar, A., and Seehausen, O. (2012). Evidence of adaptive evolutionary divergence during biological invasion. PLoS ONE 7:e49377. doi: 10.1371/journal.pone.0049377
Macdonald, J. F., Bekkers, J., Macisaac, S. M., and Blouw, D. M. (1995). Experiments on embryo survivorship, habitat selection, and competitive ability of a stickleback fish (Gasterosteus) which nests in the rocky intertidal zone. Behaviour 132, 1207–1221. doi: 10.1163/156853995X00531
Magalhaes, I. S., D'Agostino, D., Hohenlohe, P. A., and MacColl, A. D. C. (2016). The ecology of an adaptive radiation of three-spined stickleback from North Uist, Scotland. Mol. Ecol. 25, 4319–4336. doi: 10.1111/mec.13746
Magalhaes, I. S., Whiting, J. R., D'Agostino, D., Hohenlohe, P. A., Mahmud, M., Bell, M. A., et al. (2020). Intercontinental genomic parallelism in multiple adaptive radiations. Nat. Ecol. Evol. doi: 10.1038/s41559-020-01341-8
Magnus, A. (1999). “Book twenty-four: On aquatic animals,” in On Animals: A Medieval Summa Zoologica, Vol. 2, eds K. F. Kitchell Jr and I. M. Resnik. (Baltimore, MD: The Johns Hopkins University Press).
Mäkinen, H. S., Cano, J. M., and Merilä, J. (2006). Genetic relationships among marine and freshwater populations of the European three-spined stickleback (Gasterosteus aculeatus) revealed by microsatellites. Mol. Ecol. 15, 1519–1534. doi: 10.1111/j.1365-294X.2006.02871.x
Mäkinen, H. S., and Merilä, J. (2008). Mitochondrial DNA phylogeography of the three-spined stickleback (Gasterosteus aculeatus) in Europe—evidence for multiple glacial refugia. Mol. Phylogenet. Evol. 46, 167–182. doi: 10.1016/j.ympev.2007.06.011
Mangolt, G. (1557). “Von den Gattungen/namen/natur vnd Eigenschafft der vischen Bodensees/Zu welcher Zyt des Jars Jeder leiche vnd deshalb arg zu schühen sey,” in Die Fischbenennungen des Unterseegebiets-Mit dem erstmaligen Abdruck des Fischbüchleins von Gregor Mangolt nach den Originalhandschriften, ed A. Ribi (Rüschikon: Baublatt), 1942.
Manica, A. (2002). Filial cannibalism in teleost fish. Biol. Rev. Camb. Philos. Soc. 77, 261–277. doi: 10.1017/S1464793101005905
Manzer, J. I. (1976). Distribution, food, and feeding of the threespine stickleback in Great Central Lake, vancouver Island, with comments on competition for food with juvenile sockeye salmon. Fish. Bull. 74, 647–668.
Marques, D. A., Lucek, K., Haesler, M. P., Feller, A. F., Meier, J. I., Wagner, C. E., et al. (2017a). Genomic landscape of early ecological speciation initiated by selection on nuptial colour. Mol. Ecol. 26, 7–24. doi: 10.1111/mec.13774
Marques, D. A., Lucek, K., Meier, J. I., Mwaiko, S., Wagner, C. E., Excoffier, L., et al. (2016). Genomics of rapid incipient speciation in sympatric threespine stickleback. PLoS Genet. 12:e1005887. doi: 10.1371/journal.pgen.1005887
Marques, D. A., Lucek, K., Sousa, V. C., Excoffier, L., and Seehausen, O. (2019a). Admixture between old lineages facilitated contemporary ecological speciation in Lake Constance stickleback. Nat. Commun. 10:4240. doi: 10.1038/s41467-019-12182-w
Marques, D. A., Meier, J. I., and Seehausen, O. (2019b). A combinatorial view on speciation and adaptive radiation. Trends Ecol. Evol. 34, 531–544. doi: 10.1016/j.tree.2019.02.008
Marques, D. A., Taylor, J. S., Jones, F. C., Di Palma, F., Kingsley, D. M., and Reimchen, T. E. (2017b). Convergent evolution of SWS2 opsin facilitates adaptive radiation of threespine stickleback into different light environments. PLoS Biol. 15:e2001627. doi: 10.1371/journal.pbio.2001627
McKinnon, J. S., and Rundle, H. D. (2002). Speciation in nature: the threespine stickleback model systems. Trends Ecol. Evol. 17, 480–488. doi: 10.1016/S0169-5347(02)02579-X
McPhail, J. D. (1984). Ecology and evolution of sympatric sticklebacks (Gasterosteus): morphological and genetic evidence for a species pair in Enos Lake, British Columbia. Can. J. Zool. 62, 1402–1408. doi: 10.1139/z84-201
McPhail, J. D. (1994). Speciation and the Evolution of Reproductive Isolation in the Sticklebacks Gasterosteus) of Southwestern British Columbia. The Evolutionary Biology of the Threespine Stickleback. Available online at: https://ci.nii.ac.jp/naid10015636633/ (accessed December 2, 2019).
Mehlis, M., Bakker, T. C. M., Engqvist, L., and Frommen, J. G. (2010). To eat or not to eat: egg-based assessment of paternity triggers fine-tuned decisions about filial cannibalism. Proc. Biol. Sci. 277, 2627–2635. doi: 10.1098/rspb.2010.0234
Miller, R. R., and Hubbs, C. L. (1969). Systematics of Gasterosteus aculeatus, with particular reference to intergradation and introgression along the pacific coast of North America: a commentary on a recent contribution. Copeia 1969, 52–69. doi: 10.2307/1441696
Millet, A., Kristjánsson, B. K., Einarsson, A., and Räsänen, K. (2013). Spatial phenotypic and genetic structure of threespine stickleback (Gasterosteus aculeatus) in a heterogeneous natural system, Lake Mývatn, Iceland. Ecol. Evol. 3, 3219–3232. doi: 10.1002/ece3.712
Moodie, G. E. E. (1984). Status of the giant (Mayer Lake) stickleback, Gasterosteus sp., on the queen charlotte Islands, British Columbia. Can. Field Nat. 98:115.
Mori, S., and Takamura, N. (2004). Changes in morphological characteristics of an introduced population of the threespine stickleback Gasterosteus aculeatus in Lake Towada, northern Japan. Ichthyol. Res. 51, 295–300. doi: 10.1007/s10228-004-0232-8
Morris, D., Tinbergen, N., and Hoogland, R. (1956). The spines of sticklebacks (Gasterosteus and Pygosteus) as means of defence against predators (Perca and Esox). Behaviour 10, 205–236. doi: 10.1163/156853956X00156
Morris, M. R. J., Bowles, E., Allen, B. E., Jamniczky, H. A., and Rogers, S. M. (2018). Contemporary ancestor? Adaptive divergence from standing genetic variation in Pacific marine threespine stickleback. BMC Evol. Biol. 18:113. doi: 10.1186/s12862-018-1228-8
Moser, D., Kueng, B., and Berner, D. (2015). Lake-stream divergence in stickleback life history: a plastic response to trophic niche differentiation? Evol. Biol. 42, 328–338. doi: 10.1007/s11692-015-9327-6
Moser, D., Roesti, M., and Berner, D. (2012). Repeated lake-stream divergence in stickleback life history within a Central European lake basin. PLoS ONE 7:e50620. doi: 10.1371/journal.pone.0050620
Muckle, R. (1972). “Der dreistachlige stichling (Gasterosteus aculeatus L.) im Bodensee,” in Schriften des Vereins für Geschichte des Bodensees und seiner Umgebung, Vol. 90, 249–257.
Müller, G., and Gees, R. A. (1968). Origin of the lake constance basin. Nature 217:836. doi: 10.1038/217836a0
Münzing, J. (1959). Biologie, variabilität und Genetik von Gasterosteus aculeatus L. (Pisces) Untersuchungen Im Elbegebiet. Int. Revue ges. Hydrobiol. Hydrogr. 44, 317–382. doi: 10.1002/iroh.19590440119
Münzing, J. (1963). The evolution of variation and distributional patterns in european populations of the three-spined stickleback, Gasterosteus aculeatus. Evolution 17, 320–332. doi: 10.1111/j.1558-5646.1963.tb03285.x
Muus, B. J., and Nielsen, J. G. (1999). Sea Fish. Denmark: Scandinavian Fishing Year Book, Hedehusene.
Nelson, J. S., and Harris, M. A. (1987). Morphological characteristics of an introduced threespine stickleback, Gasterosteus aculeatus, from Hasse Lake, Alberta: a first occurrence in the interior plains of North America. Environ. Biol. Fishes 18, 173–181. doi: 10.1007/BF00000357
Nenning, S. T. (1834). Die Fische des Bodensees nach ihrer aussern erscheinung / herausgegeben von Dr. Konstanz: St. Nenning. doi: 10.5962/bhl.title.6889
Nesbø, C. L., Fossheim, T., Vollestad, L. A., and Jakobsen, K. S. (1999). Genetic divergence and phylogeographic relationships among european perch (Perca fluviatilis) populations reflect glacial refugia and postglacial colonization. Mol. Ecol. 8, 1387–1404. doi: 10.1046/j.1365-294x.1999.00699.x
Nilsson, J. (2006). Predation of Northern Pike (Esox lucius L.) eggs: a possible cause of regionally poor recruitment in the baltic sea. Hydrobiologia 553, 161–169. doi: 10.1007/s10750-005-1949-8
Nilsson, J., Flink, H., and Tibblin, P. (2019). Predator–prey role reversal may impair the recovery of declining pike populations. J. Anim. Ecol. 242:62. doi: 10.1111/1365-2656.12981
Numann, W. (1972). The bodensee: effects of exploitation and eutrophication on the salmonid community. J. Fish. Res. Bd. Can. 29, 833–847. doi: 10.1139/f72-127
Ólafsdóttir, G. Á., and Snorrason, S. S. (2007). Morphological and genetic divergence of intralacustrine stickleback morphs in Iceland: a case for selective differentiation? J. Evol. Biol. doi: 10.1111/j.1420-9101.2006.01250.x
Ólafsdóttir, G. Á., and Snorrason, S. S. (2009). Parallels, nonparallels, and plasticity in population differentiation of threespine stickleback within a lake. Biol. J. Linnean Soc. 98, 803–813. doi: 10.1111/j.1095-8312.2009.01318.x
Ortí, G., Bell, M. A., Reimchen, T. E., and Meyer, A. (1994). Global survey of mitochondrial DNA sequences in the threespine stickleback: evidence for recent migrations. Evolution 48, 608–622. doi: 10.1111/j.1558-5646.1994.tb01348.x
Østbye, K., Harrod, C., Gregersen, F., Klepaker, T., Schulz, M., Schluter, D., et al. (2016). The temporal window of ecological adaptation in postglacial lakes: a comparison of head morphology, trophic position and habitat use in Norwegian threespine stickleback populations. BMC Evol. Biol. 16:102. doi: 10.1186/s12862-016-0676-2
Paccard, A., Hanson, D., Stuart, Y. E., von Hippel, F. A., Kalbe, M., Klepaker, T., et al. (2019). Repeatability of adaptive radiation depends on spatial scale: regional versus global replicates of stickleback in lake versus stream habitats. J. Hered. 111, 43–56. doi: 10.1093/jhered/esz056
Paccard, A., Wasserman, B. A., Hanson, D., Astorg, L., Durston, D., Kurland, S., et al. (2018). Adaptation in temporally variable environments: stickleback armor in periodically breaching bar-built estuaries. J. Evol. Biol. 31, 735–752. doi: 10.1111/jeb.13264
Paepke, H.-J. (2002). Gasterosteus Aculeatus Linnaeus, 1758. The Freshwater Fishes of Europe. Wiebelsheim: AULA-Verlag.
Penczak, T. (1966). Comments on the taxonomy of the three-spined stickleback, Gasterosteus aculeatus Linnaeus. Ohio J. Sci. 66, 81–87.
Pennycuick, L. (1971). Differences in the parasite infections in three-spined sticklebacks (Gasterosteus aculeatus L.) of different sex, age and size. Parasitology 63, 407–418. doi: 10.1017/S0031182000079932
Pérez-Figueroa, A., Fernández, C., Amaro, R., Hermida, M., and San Miguel, E. (2015). Population structure and effective/census population size ratio in threatened three-spined stickleback populations from an isolated river basin in northwest Spain. Genetica 143, 403–411. doi: 10.1007/s10709-015-9839-0
Petri, M. (2006). “Water quality of lake constance,” in The Rhine, ed T. P. Knepper (Berlin: Springer Berlin Heidelberg), 127–138. doi: 10.1007/698_5_018
Pichugin, M. Y., Pavlov, D. S., and Savvaitova, K. A. (2008). Life cycle and structure of populations of three-spined stickleback Gasterosteus aculeatus (Fam. Gasterosteidae) in rivers of northwestern Kamchatka (with reference to the Utkholok River). J. Ichthyol. 48, 151–161. doi: 10.1134/S0032945208020021
Polačik, M., Trichkova, T., Janáč, M., Vassilev, M., and Jurajda, P. (2008). The ichthyofauna of the shoreline zone in the longitudinal profile of the Danube River, Bulgaria. Acta Zool. Bulg. 60, 77–88.
Prentis, P. J., Wilson, J. R. U., Dormontt, E. E., Richardson, D. M., and Lowe, A. J. (2008). Adaptive evolution in invasive species. Trends Plant Sci. 13, 288–294. doi: 10.1016/j.tplants.2008.03.004
Prévost, F. (1861). Des animaux D'appartement et de Jardin: Oiseaux, Poissons, Chiens, Chats. Paris: F. Savy.
Raeymaekers, J. A. M., Maes, G. E., Audenaert, E., and Volckaert, F. A. M. (2005). Detecting Holocene divergence in the anadromous–freshwater three-spined stickleback (Gasterosteus aculeatus) system. Mol. Ecol. 14, 1001–1014. doi: 10.1111/j.1365-294X.2005.02456.x
Räsänen, K., Delcourt, M., Chapman, L. J., and Hendry, A. P. (2012). Divergent selection and then what not: the conundrum of missing reproductive isolation in Misty Lake and stream stickleback. Int. J. Ecol. 2012:902438. doi: 10.1155/2012/902438
Ravinet, M., Harrod, C., Eizaguirre, C., and Prodöhl, P. A. (2014). Unique mitochondrial DNA lineages in Irish stickleback populations: cryptic refugium or rapid recolonization? Ecol. Evol. 4, 2488–2504. doi: 10.1002/ece3.853
Ravinet, M., Prodöhl, P. A., and Harrod, C. (2013). Parallel and nonparallel ecological, morphological and genetic divergence in lake-stream stickleback from a single catchment. J. Evol. Biol. 26, 186–204. doi: 10.1111/jeb.12049
Ravinet, M., Yoshida, K., Shigenobu, S., Toyoda, A., Fujiyama, A., and Kitano, J. (2018). The genomic landscape at a late stage of stickleback speciation: High genomic divergence interspersed by small localized regions of introgression. PLoS Genet. 14:e1007358. doi: 10.1371/journal.pgen.1007358
Reimchen, T. E. (1983). Structural relationships between spines and lateral plates in threespine stickleback (Gasterosteus aculeatus). Evolution 37:931. doi: 10.2307/2408408
Reimchen, T. E. (1989). Loss of nuptial color in threespine sticklebacks (Gasterosteus aculeatus). Evolution 43, 450–460. doi: 10.1111/j.1558-5646.1989.tb04239.x
Reimchen, T. E. (1992a). Extended longevity in a large-bodied stickleback, Gasterosteus, population. Canadian field-naturalist. Ottawa 106, 122–125.
Reimchen, T. E. (1992b). Injuries on stickleback from attacks by a toothed predator (Oncorhynchus) and implications for the evolution of lateral plates. Evolution 46, 1224–1230. doi: 10.1111/j.1558-5646.1992.tb00631.x
Reimchen, T. E. (2000). Predator handling failures of lateral plate morphs in Gasterosteus aculeatus: functional implications for the ancestral plate condition. Behaviour 137, 1081–1096. doi: 10.1163/156853900502448
Reimchen, T. E., Stinson, E. M., and Nelson, J. S. (1985). Multivariate differentiation of parapatric and allopatric populations of threespine stickleback in the Sangan River watershed, Queen Charlotte Islands. Can. J. Zool. 63, 2944–2951. doi: 10.1139/z85-441
Reimchen, T. E., Bergstrom, C., and Nosil, P. (2013). Natural selection and the adaptive radiation of Haida Gwaii stickleback. Evol. Ecol. Res. 15, 241–269.
Reimchen, T. E. (1994). “Predators and morphological evolution in threespine stickleback,” in The Evolutionary Biology of the Threespine Stickleback, eds M. A. Bell and S. A. Foster (Oxford, UK: Oxford University Press), 240–276.
Rennison, D. J., Delmore, K. E., Samuk, K., Owens, G. L., and Miller, S. E. (2020). Shared patterns of genome-wide differentiation are more strongly predicted by geography than by ecology. Am. Nat. 195, 192–200. doi: 10.1086/706476
Rennison, D. J., Stuart, Y. E., Bolnick, D. I., and Peichel, C. L. (2019). Ecological factors and morphological traits are associated with repeated genomic differentiation between lake and stream stickleback. Philos. Trans. R. Soc. Lond. B Biol. Sci. 374:20180241. doi: 10.1098/rstb.2018.0241
Rennison, D. J. (2016). Detecting the Drivers of Divergence: Identifying and Estimating Natural Selection in Threespine Stickleback. Vancouver, BC: University of British Columbia.
Rey, P., Mürle, U., Ortlepp, J., Mörtl, M., Scheifhacken, N., Werner, S., et al (2005). Wirbellose Neozoen im Bodensee. Karlsruhe: Landesanstalt für Umweltschutz Baden-Württemberg, 1–44. Available online at: https://kops.uni-konstanz.de/handle/123456789/40055
Rey, P., and Becker, A. (2017). Der Kormoran am Bodensee. Konstanz: Internationale Bevollmächtigtenkonferenz für die Bodenseefischerei (IBKF).
Reznick, D. N., and Ghalambor, C. K. (2001). The population ecology of contemporary adaptations: what empirical studies reveal about the conditions that promote adaptive evolution. Genetica 112–113, 183–198. doi: 10.1007/978-94-010-0585-2_12
Ribi, A. (1942). Die Fischbenennungen des Unterseegebietes: mit dem erstmaligen Abdruck des Fischbüchleins von Gregor Mangolt nach den Original-Handschriften 1557. Rüschlikon: Baublatt A.G.
Roch, S., von Ammon, L., Geist, J., and Brinker, A. (2018). Foraging habits of invasive three-spined sticklebacks (Gasterosteus aculeatus) – impacts on fisheries yield in upper lake constance. Fish. Res. 204, 172–180. doi: 10.1016/j.fishres.2018.02.014
Roesti, M., Hendry, A. P., Salzburger, W., and Berner, D. (2012). Genome divergence during evolutionary diversification as revealed in replicate lake-stream stickleback population pairs. Mol. Ecol. 21, 2852–2862. doi: 10.1111/j.1365-294X.2012.05509.x
Roesti, M., Kueng, B., Moser, D., and Berner, D. (2015). The genomics of ecological vicariance in threespine stickleback fish. Nat. Commun. 6:8767. doi: 10.1038/ncomms9767
Rondelet, G. (1554). Libri de Piscibus Marinis: in quibus verae Piscium effigies expressae sunt (Book of marine fish: wherein true fish images are expressed). Lyon: Mathias Bonhomme. doi: 10.5962/bhl.title.64229
Ros, A., Dunst, J., Gugele, S., and Brinker, A. (2019). Anti-predator mechanisms in evolutionarily predator-naïve vs. adapted fish larvae. Ecosphere 10:e02699. doi: 10.1002/ecs2.2699
Rösch, R., Baer, J., and Brinker, A. (2017). Impact of the invasive three-spined stickleback (Gasterosteus aculeatus) on relative abundance and growth of native pelagic whitefish (Coregonus wartmanni) in upper lake constance. Hydrobiologia 824, 243–254. doi: 10.1007/s10750-017-3479-6
Roy, D., Lucek, K., Walter, R. P., and Seehausen, O. (2015). Hybrid “superswarm” leads to rapid divergence and establishment of populations during a biological invasion. Mol. Ecol. 24, 5394–5411. doi: 10.1111/mec.13405
Rundle, H. D., Nagel, L., Wenrick Boughman, J., and Schluter, D. (2000). Natural selection and parallel speciation in sympatric sticklebacks. Science 287, 306–308. doi: 10.1126/science.287.5451.306
Rundle, H. D., and Schluter, D. (1998). Reinforcement of stickleback mate preferences: Sympatry breeds contempt. Evolution 52, 200–208. doi: 10.1111/j.1558-5646.1998.tb05153.x
Ryan, P. M. (1984). Age, Growth, and Food of Threespine Sticklebacks (Gasterosteus aculeatus) in Three Lakes of Central Newfoundland, Canada. St. John's, NL: Department of Fisheries and Oceans, Fisheries Research Branch.
Sakai, A. K., Allendorf, F. W., Holt, J. S., Lodge, D. M., Molofsky, J., With, K. A., et al. (2001). The population biology of invasive species. Annu. Rev. Ecol. Syst. 32, 305–332. doi: 10.1146/annurev.ecolsys.32.081501.114037
Samuk, K., Iritani, D., and Schluter, D. (2014). Reversed brain size sexual dimorphism accompanies loss of parental care in white sticklebacks. Ecol. Evol. 4, 3236–3243. doi: 10.1002/ece3.1175
Sanz, N., Araguas, R. M., Vidal, O., and Viñas, J. (2015). Glacial refuges for three-spined stickleback in the Iberian Peninsula: mitochondrial DNA phylogeography. Freshw. Biol. 60, 1794–1809. doi: 10.1111/fwb.12611
Scheffelt, E. (1926). Geschichte und Zusammensetzung der Bodensee-Fischfauna. Schrif. Verei. Geschich. Boden. Seiner Umgebung 45, 351–380.
Schinz, H. R. (1837). Verzeichniss der in der Schweiz vorkommenden wirbelthiere, Neue Denkschriften der Allgemeinen Schweizerischen Gesellschaft. 1. 1837. Neuchâtel: in der Buchdruckerei von Petitpierre, 1837.
Schluter, D. (1994). Experimental evidence that competition promotes divergence in adaptive radiation. Science 266, 798–801. doi: 10.1126/science.266.5186.798
Schluter, D. (1995). Adaptive radiation in sticklebacks: trade-offs in feeding performance and growth. Ecology 76, 82–90. doi: 10.2307/1940633
Schluter, D. (2003). Frequency dependent natural selection during character displacement in sticklebacks. Evolution 57, 1142–1150. doi: 10.1111/j.0014-3820.2003.tb00323.x
Schluter, D., and Conte, G. L. (2009). Genetics and ecological speciation. Proc. Natl. Acad. Sci. U.S.A. 106(Suppl. 1), 9955–9962. doi: 10.1073/pnas.0901264106
Schluter, D., and McPhail, J. D. (1992). Ecological character displacement and speciation in sticklebacks. Am. Nat. 140, 85–108. doi: 10.1086/285404
Schluter, D., Rambaut, A., Clarke, B. C., and Grant, P. R. (1996). Ecological speciation in postglacial fishes. Philos. Trans. R. Soc. Lond. B Biol. Sci. 351, 807–814. doi: 10.1098/rstb.1996.0075
Schmid, D. W., McGee, M. D., Best, R. J., Seehausen, O., and Matthews, B. (2019). Rapid divergence of predator functional traits affects prey composition in aquatic communities. Am. Nat. 193, 331–345. doi: 10.1086/701784
Schulze, E. (1892). Fauna Piscium Germaniae: Verzeichnis der Fische der Stromgebiete der Donau, des Rheines, der Ems, Weser, Elbe, Oder. Weichsel: des Pregels und der Memel. Hartungsche Verlagsdruckerei. doi: 10.5962/bhl.title.13339
Schumer, M., Cui, R., Rosenthal, G. G., and Andolfatto, P. (2015). Reproductive isolation of hybrid populations driven by genetic incompatibilities. PLoS Genet. 11:e1005041. doi: 10.1371/journal.pgen.1005041
Seehausen, O. (2004). Hybridization and adaptive radiation. Trends Ecol. Evol. 19, 198–207. doi: 10.1016/j.tree.2004.01.003
Seehausen, O., and Wagner, C. E. (2014). Speciation in freshwater fishes. Annu. Rev. Ecol. Evol. Syst. 45, 621–651. doi: 10.1146/annurev-ecolsys-120213-091818
Shapiro, M. D., Marks, M. E., Peichel, C. L., Blackman, B. K., Nereng, K. S., Jónsson, B., et al. (2004). Genetic and developmental basis of evolutionary pelvic reduction in threespine sticklebacks. Nature 428, 717–723. doi: 10.1038/nature02415
Sharpe, D. M. T., Räsänen, K., Berner, D., and Hendry, A. P. (2008). Genetic and environmental contributions to the morphology of lake and stream stickleback: implications for gene flow and reproductive isolation. Evol. Ecol. Res. 10, 849–866.
Shine, R. (2012). Invasive species as drivers of evolutionary change: cane toads in tropical Australia. Evol. Appl. 5, 107–116. doi: 10.1111/j.1752-4571.2011.00201.x
Smith, C., and Reay, P. (1991). Cannibalism in teleost fish. Rev. Fish Biol. Fisheries 1, 41–64. doi: 10.1007/BF00042661
Smyntek, P. M., Teece, M. A., Schulz, K. L., and Storch, A. J. (2008). Taxonomic differences in the essential fatty acid composition of groups of freshwater zooplankton relate to reproductive demands and generation time. Freshw. Biol. 53, 1768–1782. doi: 10.1111/j.1365-2427.2008.02001.x
Snyder, R. J. (1991). Migration and life histories of the threespine stickleback: evidence for adaptive variation in growth rate between populations. Environ. Biol. Fishes 31, 381–388. doi: 10.1007/BF00002363
Sommer, U., Gaedke, U., and Schweizer, A. (1993). The first decade of oligotrophication of lake constance. Oecologia 93, 276–284. doi: 10.1007/BF00317682
Spence, R., Wootton, R. J., Barber, I., Przybylski, M., and Smith, C. (2013). Ecological causes of morphological evolution in the three-spined stickleback. Ecol. Evol. 3, 1717–1726. doi: 10.1002/ece3.581
Spence, R., Wootton, R. J., Przybylski, M., Zieba, G., Macdonald, K., and Smith, C. (2012). Calcium and salinity as selective factors in plate morph evolution of the three-spined stickleback (Gasterosteus aculeatus). J. Evol. Biol. 25, 1965–1974. doi: 10.1111/j.1420-9101.2012.02585.x
Stedman, R. M., and Bowen, C. A. (1985). Introduction and spread of the threespine stickleback (Gasterosteus aculeatus) in lakes huron and michigan. J. Great Lakes Res. 11, 508–511. doi: 10.1016/S0380-1330(85)71794-7
Stich, H. B. (2004). Back again: the reappearance of Diaphanosoma brachyurum in lake constance. Archiv für Hydrobiologie 159, 423–431. doi: 10.1127/0003-9136/2004/0159-0423
Stich, H. B., and Brinker, A. (2010). Oligotrophication outweighs effects of global warming in a large, deep, stratified lake ecosystem. Glob. Chang. Biol. 16, 877–888. doi: 10.1111/j.1365-2486.2009.02005.x
Straile, D., and Geller, W. (1998). “Influence of weather conditions on the seasonal plankton development in a large and deep lake (L. Constance): IV. The response of crustacean zooplankton to variations in water temperature and algal growth in spring and early summer,” in NATO Advanced Science Institutes Series 2: Environment: Management of Lakes and Reservoirs During Global Climate Change, Vol. 42 (Dordrecht: Kluwer Academic Publishers), 323. doi: 10.1007/978-94-011-4966-2_6
Stuart, Y. E., Veen, T., Weber, J. N., Hanson, D., Ravinet, M., Lohman, B. K., et al. (2017). Contrasting effects of environment and genetics generate a continuum of parallel evolution. Nat. Ecol. Evol. 1:158. doi: 10.1038/s41559-017-0158
Taylor, E. B., and McPhail, J. D. (1999). Evolutionary history of an adaptive radiation in species pairs of threespine sticklebacks (Gasterosteus): insights from mitochondrial DNA. Biol. J. Linn. Soc. Lond. 66, 271–291. doi: 10.1111/j.1095-8312.1999.tb01891.x
Terekhanova, N. V., Logacheva, M. D., and Penin, A. A. (2014). Fast evolution from precast bricks: genomics of young freshwater populations of threespine stickleback Gasterosteus aculeatus. PLoS Genet. 10:e1004696. doi: 10.1371/journal.pgen.1004696
Th, C. M., and Bakker Sevenster, P. (1988). Plate morphs of gasterosteus aculeatus linnaeus (pisces: gasterosteidae): comments on terminology. Copeia 1988, 659–663. doi: 10.2307/1445384
Turschak, B. A., and Bootsma, H. A. (2015). Lake Michigan trophic structure as revealed by stable C and N isotopes. J. Great Lakes Res. 41, 185–196. doi: 10.1016/j.jglr.2015.04.004
Vamosi, S. M., Hatfield, T., and Schluter, D. (2000). A test of ecological selection against young-of-the-year hybrids of sympatric sticklebacks. J. Fish Biol. 57, 109–121. doi: 10.1111/j.1095-8649.2000.tb00779.x
Vila, M., Hermida, M., Fernández, C., Perea, S., Doadrio, I., Amaro, R., et al. (2017). Phylogeography and conservation genetics of the ibero-balearic three-spined stickleback (Gasterosteus aculeatus). PLoS ONE 12:e0170685. doi: 10.1371/journal.pone.0170685
Vogt, C., and Hofer, B. (1909). Die Süßwasserfische Mittel-Europas. ed W. Grote (Leipzig: W. Engelmann Verlag), 558.
von Siebold, C. T. E. (1863). Die süsswasserfische von Mitteleuropa. Leipzig: W. Engelmann. doi: 10.5962/bhl.title.61814
Vonlanthen, P., Bittner, D., Hudson, A. G., Young, K. A., Müller, R., Lundsgaard-Hansen, B., et al. (2012). Eutrophication causes speciation reversal in whitefish adaptive radiations. Nature 482, 357–362. doi: 10.1038/nature10824
Vonlanthen, P., Excoffier, L., Bittner, D., Persat, H., Neuenschwander, S., and Largiadèr, C. R. (2007). Genetic analysis of potential postglacial watershed crossings in Central Europe by the bullhead (Cottus gobio L.). Mol. Ecol. 16, 4572–4584. doi: 10.1111/j.1365-294X.2007.03531.x
Wang, N., and Eckmann, R. (1994). Distribution of perch (Perca fluviatilis L.) during their first year of life in lake constance. Hydrobiologia 277, 135–143. doi: 10.1007/BF00007295
Werner, S., Mortl, M., Bauer, H.-G., and Rothhaupt, K.-O. (2005). Strong impact of wintering waterbirds on zebra mussel (Dreissena polymorpha) populations at lake constance, Germany. Freshw. Biol. 50, 1412–1426. doi: 10.1111/j.1365-2427.2005.01411.x
Werner, S., Bauer, H.-G., Heine, G., Jacoby, H., and Stark, H. (2018). 55 Jahre Wasservogelzählung am Bodensee: Bestandsentwicklung der Wasservögel von 1961/62 bis 2015/16. Available online at: https://pure.mpg.de/pubman/faces/ViewItemOverviewPage.jsp?itemId=item_3033525. (accessed September 05, 2020).
Wessels, M. (1995). “Bodensee-Sedimente als Abbild von Umweltänderungen im Spät-und Postglazial. Göttingen: Göttinger Arbeiten zur Geologie und Paläontologie Nr. 66.
Whoriskey, F. G., and FitzGerald, G. J. (1985). Sex, cannibalism and sticklebacks. Behav. Ecol. Sociobiol. 18, 15–18.
Willacker, J. J., von Hippel, F. A., Wilton, P. R., and Walton, K. M. (2010). Classification of threespine stickleback along the benthic-limnetic axis. Biol. J. Linn. Soc. Lond. 101, 595–608. doi: 10.1111/j.1095-8312.2010.01531.x
Williams, W. I., Friedman, J. M., Gaskin, J. F., and Norton, A. P. (2014). Hybridization of an invasive shrub affects tolerance and resistance to defoliation by a biological control agent. Evol. Appl. 7, 381–393. doi: 10.1111/eva.12134
Williamson, M., and Fitter, A. (1996). The varying success of invaders. Ecology 77, 1661–1666. doi: 10.2307/2265769
Wittmack, L. (1875). Beiträge zur Fisherei-Statistik des Deutschen Reichs sowie eines Theiles von Oesterreich-Ungarn und der Schweiz im Auftrage des Deutschen Fisherei-Vereins bearbeitet (Contributions to the Fishery Statistics of the German Reich as Well as Part of Austria-Hungary and Switzerland, Worked on Behalf of the German Fisheries Association). Berlin: Das Bureau des Deutschen Fisherei-Vereins.
Wootton, R. J. (2009). The Darwinian stickleback Gasterosteus aculeatus: a history of evolutionary studies. J. Fish Biol. 75, 1919–1942. doi: 10.1111/j.1095-8649.2009.02412.x
Wootton, R. J. (1976). Biology of the Sticklebacks. Available online at: http://agris.fao.org/agris-search/search.do?recordID=US201300561636 (accessed September 12, 2020).
Xie, K. T., Wang, G., Thompson, A. C., Wucherpfennig, J. I., Reimchen, T. E., MacColl, A. D. C., et al. (2019). DNA fragility in the parallel evolution of pelvic reduction in stickleback fish. Science 363, 81–84. doi: 10.1126/science.aan1425
Zaugg, B., and Huguenin, K. (2018). Fauna Helvetica 7 - Pisces Atlas. Neuchâtel: Centre Suisse de Cartographie de la Faune.
Zeller, M., Lucek, K., Haesler, M. P., Seehausen, O., and Sivasundar, A. (2012). Signals of predation-induced directional and disruptive selection in the threespine stickleback. Evol. Ecol. Res. 14, 193–205.
Zenni, R. D., and Nuñez, M. A. (2013). The elephant in the room: the role of failed invasions in understanding invasion biology. Oikos 122, 801–815. doi: 10.1111/j.1600-0706.2012.00254.x
Keywords: adaptive radiation, contemporary evolution, lake constance, invasive species, stickleback
Citation: Hudson CM, Lucek K, Marques DA, Alexander TJ, Moosmann M, Spaak P, Seehausen O and Matthews B (2021) Threespine Stickleback in Lake Constance: The Ecology and Genomic Substrate of a Recent Invasion. Front. Ecol. Evol. 8:611672. doi: 10.3389/fevo.2020.611672
Received: 29 October 2020; Accepted: 28 December 2020;
Published: 21 January 2021.
Edited by:
Baran Yoğurtçuoğlu, Hacettepe University, TurkeyReviewed by:
Phillip Joschka Haubrock, Senckenberg Research Institute and Natural History Museum Frankfurt, GermanyWindsor Aguirre, DePaul University, United States
Copyright © 2021 Hudson, Lucek, Marques, Alexander, Moosmann, Spaak, Seehausen and Matthews. This is an open-access article distributed under the terms of the Creative Commons Attribution License (CC BY). The use, distribution or reproduction in other forums is permitted, provided the original author(s) and the copyright owner(s) are credited and that the original publication in this journal is cited, in accordance with accepted academic practice. No use, distribution or reproduction is permitted which does not comply with these terms.
*Correspondence: Cameron M. Hudson, Y2FtZXJvbi5odWRzb24mI3gwMDA0MDtlYXdhZy5jaA== orcid.org/0000-0003-3298-4510