- 1Grimsö Wildlife Research Station, Department of Ecology, Swedish University of Agricultural Sciences (SLU), Riddarhyttan, Sweden
- 2School for Forest Management, Swedish University of Agricultural Sciences (SLU), Skinnskatteberg, Sweden
- 3Mammal Research Institute, Polish Academy of Sciences, Białowieża, Poland
- 4Department of Natural Sciences, Mid Sweden University, Sundsvall, Sweden
- 5Department of Wildlife, Fish and Environmental Studies, Swedish University of Agricultural Sciences (SLU), Umeå, Sweden
The natural and old-growth forests and their associated biodiversity continues to fade worldwide due to anthropogenic impact in various forms. The boreal forests in Fennoscandia have been subject to intensive clearfelling forestry since the middle of twentieth century. As a result, only a fraction of forests with long temporal continuity remains at the landscape level. In Sweden, some of these primary forests have been formally protected, whereas other forests with known high conservation values are not. Collectively, both protected and not protected known valuable primary forests are included in a nationally delineated network of high conservation value forests (HCVF). In addition to HCVF, older forests that have not been clearfelled since the mid-1900s, i.e., “proxy continuity forests,” have recently been mapped across the entire boreal biome in Sweden. In this paper, we analyze how these proxy continuity forests may strengthen the HCVF network from a green infrastructure perspective. First, we evaluate the spatial overlap between proxy continuity forests and HCVF. Second, we perform a large-scale connectivity analysis, in which we show that adding proxy continuity forests located outside HCVF strongly increases the structural connectivity of the network of protected forests. Finally, by assessing habitat suitability for virtual species specialized in pine, spruce, and broadleaf forests, we find large regional differences in the ability to secure habitat and thereby functional green infrastructure by considering currently unprotected primary forest. We show that, by adding those forests to the network, the area of habitat for low-demanding species dependent on spruce or pine forests can be largely increased. For high-demanding species, additional habitat restoration in the landscape matrix is needed. By contrast, even counting all valuable broadleaf forests available is not enough to provide a suitable habitat for their associated species, which indicates a large need for landscape-scale habitat restoration initiatives, in particular, for broadleaf forests.
Introduction
The loss of natural and old-growth forests and their associated biodiversity continues worldwide due to extensive anthropogenic impact (Ledig, 1992; Haddad et al., 2015; Kormos et al., 2017; Curtis et al., 2018). The area of “intact forest landscapes,” defined as predominantly forested areas showing no or limited signs of human activity and large enough to maintain native biodiversity, is declining and thus their protection is a major conservation concern (Potapov et al., 2008, 2017; Watson et al., 2018; Svensson et al., 2020). In order to fulfill ecological, social, and economic roles of forests, the vital attributes of forest ecosystems must be maintained and, if necessary, restored (Christensen, 2014; Chazdon, 2017). Such attributes include biodiversity, key ecosystem processes, and resilience ensuring forests' capacity to recover from natural and anthropogenic disturbances, including impacts of climate change (e.g., Stanturf et al., 2014; Müller et al., 2019). In addition, to mitigate decades and centuries of degradation caused by intensive forest management, restoration of degraded forests and deforested areas are of great importance (Angelstam, 1998; Aerts and Honnay, 2011; Brancalion et al., 2018). For successful biodiversity conservation, sufficient functional conservation networks are pivotal (sensu Flather et al., 1997).
Among the different forest biomes of the world, boreal forest landscapes constitute important natural assets due to their size, ~30% of the global forest area (Brandt et al., 2013; Gauthier et al., 2015), circumpolar distribution, and a relatively high level of intactness (Potapov et al., 2008; Moen et al., 2014; Kuuluvainen, 2016). However, as demonstrated by Hansen et al. (2013) and, more recently, Curtis et al. (2018), ever-increasing anthropogenic pressure results in continuous and extensive loss of intact boreal forest landscapes with intensive forestry being the major driver of primary forest cover loss in Europe and, in particular, in Fennoscandia (Curtis et al., 2018; Ceccherini et al., 2020). The ecological consequences of this loss can presently not be fully assessed, in particular, in the context of the ongoing climate change (Gauthier et al., 2015).
In Europe, boreal forests cover large areas of the Fennoscandian shield, including Norway, Sweden, Finland, and Northwest Russia, where they have been intensively managed by systematic clearfelling since the middle of the twentieth century (Kuuluvainen, 2009). In Northwest Russia, the proportion of intact forests is still relatively high (Potapov et al., 2008). In Sweden, due to a long history and extensive impact of industrial forest management (Svensson et al., 2019), only a very small fraction of intact boreal landscapes still remain (Potapov et al., 2017; Svensson et al., 2020). Moreover, the main natural disturbance factor, wildfire, has been effectively suppressed in Sweden and other parts of western Fennoscandia since the middle of the nineteenth century (Rolstad et al., 2017) although there have been several incidents of larger wildfires during the most recent years (Gustafsson et al., 2019). As a result of intensive forestry and control of natural disturbances, many boreal species and several types of forest present in naturally dynamic boreal landscapes are threatened, and some successional phases (e.g., old-growth or aging broadleaf succession) are very rare (Berg et al., 1994; Esseen et al., 1997). In total, some 1400 species are included on the Swedish Red List as a direct and indirect consequence of forest harvesting (ArtDatabanken, 2020). These negative biodiversity and ecosystem function trends call for new initiatives that ensure a more favorable conservation status of boreal forests in Sweden and elsewhere in the boreal region in the future (e.g., Naumov et al., 2018; EEA, 2019; Jonsson et al., 2019).
Forestry in Sweden, as in other European countries, is maintained intense based on arguments that maximizing biomass production is justified in the transition toward a bio-economy (Börjesson et al., 2017; Lindahl et al., 2017; Jonsson et al., 2019; Verkerk et al., 2019). At the same time, the protection of natural values in Swedish boreal forests is highlighted in the Swedish forest legislation (Anon, 2018) and follows established international and national policies addressing sustainable use and conservation values. This includes formally protected areas, sites included in the European Union's Natura 2000 network, as well as areas voluntary set aside as a part of forest certification (Swedish Forest Agency, 2019). Similar to the situation in many countries, these conservation efforts collectively represent national implementation of the global Aichi Biodiversity goals (CBD, 2010), in particular, target #7 on sustainable management, biodiversity, and conservation; target #11 on protecting a minimum of 17% of terrestrial areas and ensuring their connectivity;, and target #15 on restoring degraded ecosystems.
At the European Union level, the concept of green infrastructure is being implemented or is in preparation for implementation in the member states (e.g., Slätmo et al., 2019; Chatzimentor et al., 2020). Green infrastructure aims to secure biodiversity, habitat resilience, and ecosystem services at multiple spatial scales (Liquete et al., 2015). One of the main objectives is to support protected areas through safeguarding connectivity of target habitats at multiple spatial scales (Hermoso et al., 2020). All these initiatives require the development of spatially explicit landscape plans concerning how much and where additional set-aside conservation and restoration areas are needed to conserve biodiversity and ecosystem services and to secure sustainable use of boreal landscapes (Snäll et al., 2016; Felton et al., 2017).
The increasing amount and accessibility of comprehensive remote sensing data on forests creates opportunities for more efficient and spatially explicit conservation planning at multiple scales (White et al., 2016; Torresan et al., 2017; Mikusiński et al., 2018). In Sweden, in addition to geographical data on formally protected forests, spatial data on known high conservation value forests (HCVF; Jennings et al., 2003; Anon, 2017) have been compiled. The HCVF-concept was primarily developed to systematically identify biodiversity and ecosystem services delivered by forest ecosystems to aid forest certification schemes (e.g., Senior et al., 2014), but it is also used in the broader sense for delineating forests important for biodiversity conservation. The major characteristics and values of HCVF are (1) diverse horizontal and vertical structure (e.g., mixed tree species composition, occurrence of old trees and large volumes of dead wood, diversified height of trees), (2) ecological functionality (e.g., erosion control, nutrient retention), (3) a long temporal continuity (not clearfelled), and (4) cultural ecosystem services and generically recreational with amenity values and cultural legacies (e.g., Patru-Stupariu et al., 2013; Boucher et al., 2016). In addition, a database delineating forests not clearfelled since the mid-1900s has been developed (proxy continuity forests—pCF) (Ahlcrona et al., 2017; Svensson et al., 2020). Both the HCVF and the pCF databases include forests that are not legally protected and thereby may be logged. Even if some pCF could have been subject to historical selective logging, one can assume that the two databases collectively encompass “primary forests” of boreal Sweden, i.e., naturally regenerated forests with native tree species in which there are no clearly visible signs of human interaction and in which the ecological processes are not significantly disturbed (FRA, 2020). Such forests are, in turn, a main component of “intact forest landscapes” defined above (Potapov et al., 2008).
Primary forests and intact forest landscapes are of profound importance for functional green infrastructures and, as such, essential in conservation planning. The functionality of green infrastructure, i.e., the ability to provide habitat for species and essential ecosystem services is, to a large extent, linked to the spatial configuration of its components (Andersson et al., 2013). For example, Svensson et al. (2020) have lately identified the Scandinavian Mountains Green Belt as a largely contiguous and structurally well-connected belt of intact forest landscapes formed by primary forests and surrounding near-natural forest areas. They concluded that the Scandinavian Mountains Green Belt is of utmost conservation importance on both the European and global scales.
Most recently, a new national-scale land-cover database has been published in Sweden (SEPA, 2019), which provides high spatial (10 ×10 m) and thematic resolution as well as high thematic accuracy. Together with the HCVF and pCF databases, this allows innovative approaches for analyzing the functionality of green infrastructure in providing suitable habitats for species with different habitat requirements and in mapping the premises for broad-scale ecological connectivity.
In this paper, we use these recently compiled spatial data sets describing primary forests with known and potentially high conservation value in an attempt to explore their abilities to support functional green infrastructure. More specifically, the aim of the study was to analyze and evaluate planning routes toward functional green infrastructure in boreal Sweden through analyses of spatial relationships between unprotected areas and the existing formally protected forests. The research objectives were (1) to estimate the spatial overlap of pCF and HCVF and explore how this overlap varies across the boreal region in Sweden; (2) to quantify the size, number, and distribution of the components of habitat networks for virtual species dependent on coniferous, i.e. spruce (Picea abies) or pine (Pinus sylvestris), or broadleaf forests based on formally protected forests; (3) to examine the potential increase of habitat area for virtual species when all identified primary forests are included and how this affects the functionality of green infrastructure; and (4) to assess how large-scale connectivity patterns varies among the protected primary forests, all primary forests, and all forestlands used as the baseline reference.
Materials and Methods
Study Area
The study area covers northern Sweden, in total a 27.0 million ha terrestrial surface with a forest area equal to 18.9 million ha, corresponding to 67% of all forest land in Sweden (SLU, 2020) and to around 80% of the boreal forests. With the Scandinavian Mountains in the west, 18.4% (i.e., some five million ha) of the terrestrial surface is covered by non-forested alpine land. Flat terrain and sites with poor tree-growth conditions dominate over large areas and support open mires covering more than 4.4 million ha, i.e., 16.4% of the terrestrial surface (SLU, 2020). Of the total studied forest land, 80% is considered as productive (tree growth >1 m3/ha/year on average per rotation cycle) with Scots pine dominating (44.0%) followed by Norway spruce covering 19.7% and mixed coniferous forests covering 12.6% (SLU, 2020). The study region includes all the subalpine mountain birch (Betula pubescens ssp. czerepanovii) forests in Sweden forming the alpine tree line in the western part of the study area and covering, in total, 1.1 million ha (Hedenås et al., 2016).
The history of forestry and other types of land use is extensive with more active forest harvesting since the 1600s and with the more intensive forest exploitation since the mid-1800s (Lundmark et al., 2013). Industrial rotation forest management with clearfelling, soil scarification, and replanting of monoculture genotypes dominates the last 60–70 years. From the middle of 1990s, the introduction of tree retention (i.e., retained solitary trees or trees in patches of about 0.01–0.5 ha on clearfelled areas or as buffer zones along streams, lakes, or mires) and a generally smaller size of single logged areas have, to some degree, ameliorated the environmental impact of forestry (Gustafsson and Perhans, 2010). However, the overall impact of forest management on the level of forest intactness has been massive with the majority of primary forests and intact forest landscapes transformed into intensively managed forests and landscapes (e.g., Jonsson et al., 2019; Svensson et al., 2019, 2020).
To reflect the biogeographical gradient and land use history, our analysis was done for eight subregions (Figure 1); four covering inland-to-mountain conditions (from south to north: Dalarna, Jämtland, Västerbotten west, and Norrbotten west) and four covering coast-to-inland conditions (from south to north: Gävleborg, Västernorrland, Västerbotten east, and Norrbotten east). Hence, we divided the two northernmost regions, Norrbotten and Västerbotten counties that stretch from the Scandinavian Mountains to the Bothnian Sea, into western and eastern parts.
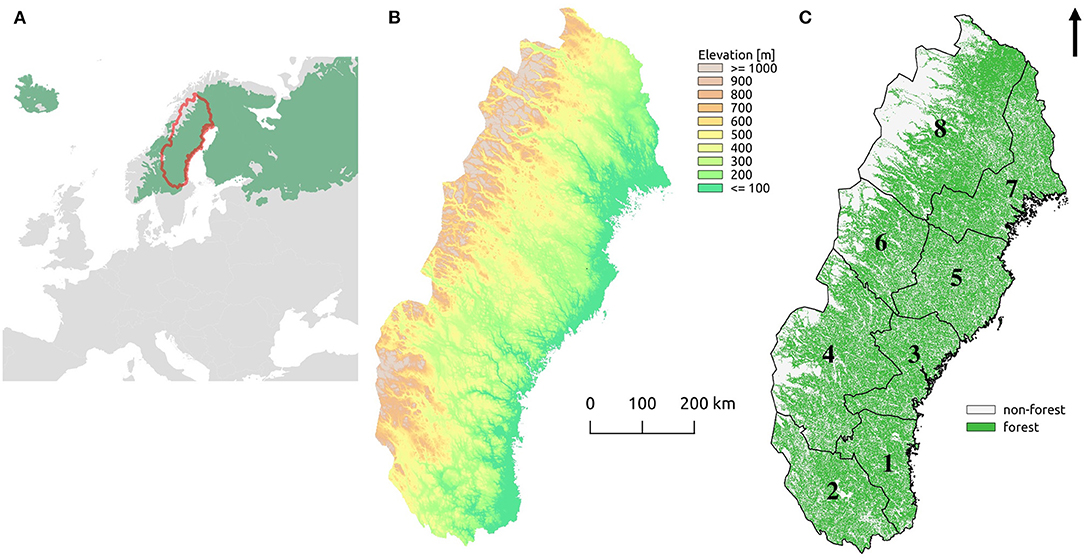
Figure 1. (A) The location of the study region in Europe (red line) with the boreal biome highlighted in green. (B) The elevation (m) above sea level across the study region calculated from the elevation model. (C) Total forest land (green) with the eight subregions; (1) Gävleborg, (2) Dalarna, (3) Västernorrland, (4) Jämtland, (5) Västerbotten east, (6) Västerbotten west, (7) Norrbotten east, (8) Norrbotten west.
Data
We used several different sources of spatial data on forests (details provided in the Supplementary Material). For data on HCVF, we used the national Swedish database provided by the Swedish County Administrations (Anon, 2017). The HCVF were delineated based on forest cover of the national topographic terrain (1:50,000) and road maps (1:100,000), and their high conservation values were validated via field surveys. This database provides the status up to 2016 and includes several categories of long- and short-term formally protected, voluntarily set aside, and unprotected areas combined in two categories: protected and unprotected HCVF. The limitation of the HCVF dataset, or rather HCVF-dataset is that it includes only already known HCVF and, thus, excludes other forest areas not yet identified as valuable.
The pCF-dataset is a complete-coverage continuous raster layer mapped by retrospective analysis of remnant forest patches not being subject to clearfelling at least since the middle of the twentieth century. The pCF-mapping was performed as an automatic change-detection analysis of a time series of satellite images from 1973 to 2016, complemented with aerial photos from the 1950s and 1960s (Ahlcrona et al., 2017; Svensson et al., 2019, 2020). All forest patches 0.5 ha and larger and forest belts 20 m or wider were mapped across the entire boreal biome in Sweden. The inclusion of small patches in the database provides an opportunity to detect small-size, old-growth forest areas that function as stepping-stones linking larger forest patches, i.e., patches that are of interest in habitat distribution analyses. The limitation of the pCF-dataset is that it identifies only potentially valuable forests for conservation with no external validation of their ecological qualities.
Finally, we used the recently published high-resolution (10 m) national land cover database (SEPA, 2019). In this database the forest environments are classified into seven main forest types additionally divided into stands located on upland soils and on wet soils (14 classes in total).
Analyses
First, we combined different raster-based data sets (HCVF, pCF, land cover database) to quantitatively compare their spatial overlap. We used the original spatial resolution of 10 ×10 m of the pCF and national land cover databases and a rasterized HCVF vector layer at the same resolution. Next, we assessed large-scale structural connectivity (i.e., based on their spatial configuration and extent) between different primary forest categories relevant to conservation (HCVF protected, HCVF unprotected, and pCF) considering multiple scenarios. By expanding the area of primary forests from only including protected forest to including also unprotected HCVF and finally also to including pCF, we tested how their combined spatial configuration and extent can support the creation of a functional forest green infrastructure by maximizing the structural connectivity between the focal areas. Finally, we mapped habitats of virtual species (sensu Vos et al., 2001, Mikusiński and Edenius, 2006 and Angelstam et al., 2020; details provided in the Supplementary Material) that are dependent on pine, spruce, or broadleaf forest types with different spatial requirements in terms of the amount and distribution of the habitat available in the landscape perspective (low vs. high demanding). The virtual species approach group ecological profiles of organisms according to the characteristics that are important in their metapopulation response to the habitat amount and distribution in the landscapes. Thereby, in the meta-population context, individual area requirements are viewed as the dominant characteristic of species extinction risk in landscape patches and dispersal distance as the main determinant of the ability to colonize new patches (see also Vos et al., 2001).
To assess the large-scale structural connectivity of primary forests belonging to the two categories of HCVF (protected, unprotected) and pCF as well as composites of them, we used a connectivity model derived from circuit theory (McRae et al., 2008) and implemented in the Circuitscape software v5 (re)written in Julia programming language (http://www.circuitscape.org; Anantharaman et al., 2020). In this part of the study, we aimed at modeling the “global,” i.e., for the whole study area, structural connectivity without focus on particular species or species group (thus, a “species-agnostic” model following Koen et al., 2014). We considered only two classes of land cover when designing the resistance surface used for the Circuitscape modeling: Class 1: clusters of primary forests (based on HCVF-protected, HCVF-unprotected, and pCF in different combinations depending on the scenario) and Class 2: matrix of other land-cover classes, including other (mainly managed) forest areas. To assure sufficient difference in resistance between these two classes, we assigned the value 1 for the pCF clusters and the value 100 for the matrix. The resistance values were chosen to ensure contrast without influencing the connectivity distribution results, and we consider those values reasonable following, e.g., the “one-stage expert approach” (Zeller et al., 2012) and customary approaches to assign increasing resistance values when empirical data is unavailable (Koen et al., 2014).
The measure of connectivity was the cumulative current density (CCD) estimated for each pixel of the resistance surface raster. The estimated CCD values resulted from the current flow between all pairs of 24 focal nodes (552 combinations) placed at equal distance along the perimeter of a 50-km buffer zone around the study region. We used the same number and fixed locations of focal nodes for all scenarios to facilitate the comparison of the output CCD maps. To adjust to computational limits, we down-sampled the pCF-raster from a 50 ×50 m to a 500 ×500 m resolution prior to running the Circuitscape algorithm. As we were interested in global (study area) rather than local patterns and as coarsening the input grid usually brings results that closely approximate those generated at fine-scale resolution (see McRae et al., 2008), we foresaw no detectable effects of this coarser resolution on the final results. We applied the same procedure to all scenarios, including the baseline reference with non-forested area representing the matrix (cf. Class 2) and all forest lands representing potentially focal habitats (cf. Class 1). All maps were created with help of the open source software QGIS (QGIS Development Team 2017 Ver. 2.18) and GRASS GIS software (Neteler et al., 2012 Ver. 7.4.0).
We designed habitat suitability index (HSI) models (for review, see Edenius and Mikusiński, 2006; details provided in the Supplementary Material) for defined virtual species using two different selections of forest stands, i.e., (1) protected primary forest and (2) all primary forest delineated by GIS databases. The selection of virtual species should represent broad categories of species habitat demands, so they can function as a focal or umbrella/indicator species (Lambeck, 1997). Because forests are often composed of several tree species, classification of forest types in mapping is usually based on different mixtures of tree species expressed by ranges in their percentages. In the case of the boreal Sweden, Scots pine, Norway spruce, and collectively treated broadleaf trees (dominated by Betula spp.) may be considered as three main compositional components for forest-dependent species. For example, an organism specialized in old pines may find its habitat in both pure pine stands but also in mixed stands with, e.g., spruce, which simultaneously provide habitat for spruce specialists. Therefore, in our application of HSI models, five different forest classes originating from the high-resolution land cover dataset were used as a base for assessing the amount of habitat for three virtual species (Supplementary Table A1):
Pine dependent: Pine forest + mixed coniferous forest + coniferous forest with an admixture of broadleaf trees corresponding to virtual species with natural pine-dominated forest as the main habitat.
Spruce dependent: Spruce forest + mixed coniferous forest + coniferous forest with an admixture of broadleaf trees corresponding to virtual species with natural spruce-dominated forest as the main habitat.
Broadleaf dependent: Broadleaf forest + coniferous forest with an admixture of broadleaf trees corresponding to virtual species with natural broadleaf-dominated forest as the main habitat.
Pine, spruce, and broadleaf forests were treated as optimal (habitat score = 1) for corresponding virtual species, and additional mixed tree species composition types (i.e., mixed coniferous forest and coniferous forest with an admixture of broadleaf trees) were treated as contributing with lower habitat value (habitat score = 0.5). The above habitat scores were used to calculate the effective habitat area for each virtual species.
Two contrasting levels of spatial requirements of virtual species were applied. These were based on the minimum habitat area and the amount of habitat at the landscape level (e.g., Manton et al., 2005; Orlikowska et al., 2020; Supplementary Table A1):
Low-demanding species (LD): Small minimum habitat area (minimum 0.2 ha spatially connected pixels) and low landscape level requirements (minimum 5% in 1 km2 = 5 ha).
High demanding species (HD): Large minimum habitat area (minimum 2 ha spatially connected pixels) and high landscape level requirements (minimum 20% in 2 km2 = 40 ha).
Hence, low-demanding species represent organisms that are able to colonize relatively small and isolated habitat patches, and high-demanding species represent organisms requiring larger contiguous patches of habitat and that are not able to exist in highly fragmented landscapes. In total, HSI models for six different virtual species (the two levels of habitat spatial demands and the three habitat types) were applied for both levels of conservation, i.e., securing just currently protected primary forests (low conservation level) or securing all existing primary forests (high conservation level; Supplementary Table A1). All spatial analyses in this part of the study were performed using the ArcGIS Focal Statistics tool (ESRI Inc., 2015) with a species-specific measure of neighborhood (“moving window”) applied to assess landscape-scale requirements of the species. A more detailed description of the parameters and the entire modeling workflow is included in the Supplementary Material.
The resulting maps of habitat distribution for all defined virtual species were used to compare their habitat networks at the two different conservation levels. The HSI models provide (based on minimum habitat area, habitat type, habitat value, and landscape requirement) the total area of the networks. This area is composed of a number of spatially connected components, approximating a certain functional habitat area. To evaluate the different conservation levels (already protected primary forests vs. all primary forests), we report the total area of the habitat network as well as number and size distribution of the network components (Supplementary Tables A2–A4).
Results
The share of pCF in total was higher in Norrbotten west (75%) and Västerbotten west (69%) and lower in Västernorrland and Gävleborg (both around 40%). There are consistently higher pCF-proportions in the inland mountain subregions, where the share increases northward. The proportion of pCF that does not overlap with HCVF decreases northward; however, eastern subregions have a generally larger proportion of pCF outside HCVF than the western. Consequently, the eastern and southern areas had only a low proportion (below 10%) of protected pCF (Figure 2). The highest level of pCF protection (50%) was found in Norrbotten west. In general, the proportions of unprotected HCVF are low across all subregions and lowest in the coast-inland subregions; i.e., a great majority of HCVF are actually protected in that part of the study region.
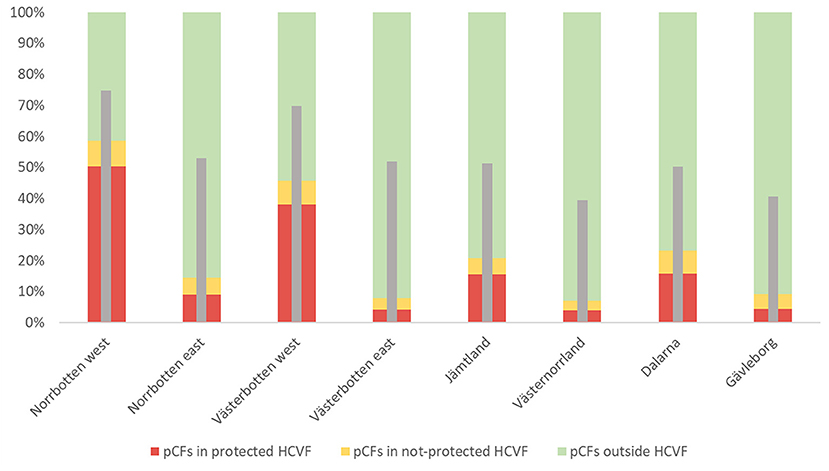
Figure 2. Percentages of pCF located within high conservation value forests [protected HCVF (red), non-protected HCVF (yellow)], or outside HCVF (green), presented for the different subregions. The narrow gray bars depict the percentage of pCF in the total region's forest area (based on the Copernicus Land Monitoring Service data).
Our analysis of the structural connectivity showed divergent patterns both in the context of different levels of conservation ambition and concerning regional differences (Figure 3). We found low connectivity among protected forests with the exception of the mountain foothill forests. The connectivity pattern based on all protected and unprotected HCVF did not reveal any substantial connectivity increases. However, the connectivity map based on all primary forests demonstrates large connectivity improvement in the northern part of the study area and a clear strengthening of the connectivity in the southwest. Finally, given the high fraction of forestland in the region, the “baseline” connectivity map shows high connectivity across almost the entire study area.
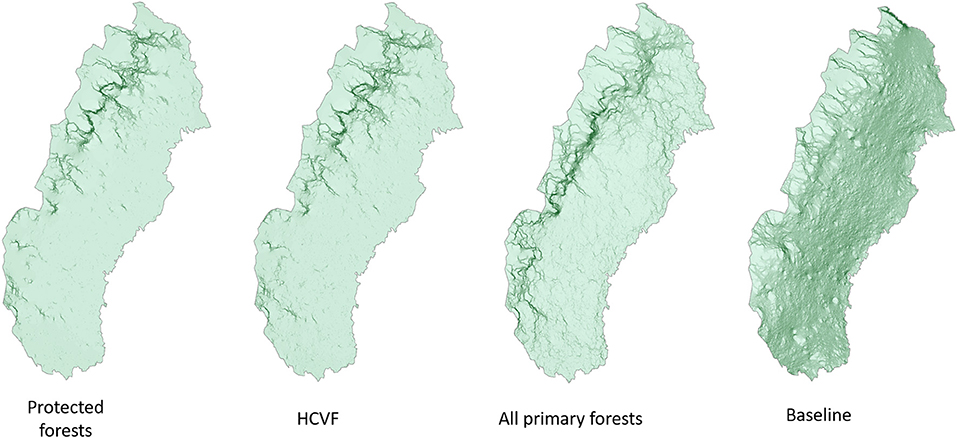
Figure 3. Cumulative current density (CCD) mapping illustrating the global connectivity based for protected primary forests, protected and unprotected high conservation value forests (HCVF), all primary forest, and baseline (all forestland). The color scale from light to dark green depicts areas with subsequently higher current density, indicating better connectivity.
The spatial distribution of suitable habitat for the virtual species based on protected primary forests and all primary forests are visualized in Figure 4. First, we found far more habitat area for low- than for high-demanding species. Second, the amount of suitable habitat based on all primary forests greatly exceeds the amount available based on protected primary forests, and this difference was more pronounced for low-demanding species. Third, the habitat networks of virtual species of different forest types were very dissimilar. For low-demanding species, all primary forests added a significant structural increase of habitat, and for high-demanding species, the structural increase associated with all primary forests is quite limited, particularly for broadleaf forest–dependent species. Broadleaf forests and the species that rely on such habitats are largely restricted to the alpine tree line mountain birch forest. The components of the habitat network of the high-demanding spruce specialist were concentrated to the Scandinavian Mountains foothills forest landscapes, and their equivalent for high-demanding pine specialists had concentrations in the north and southwest as well as along the coast.
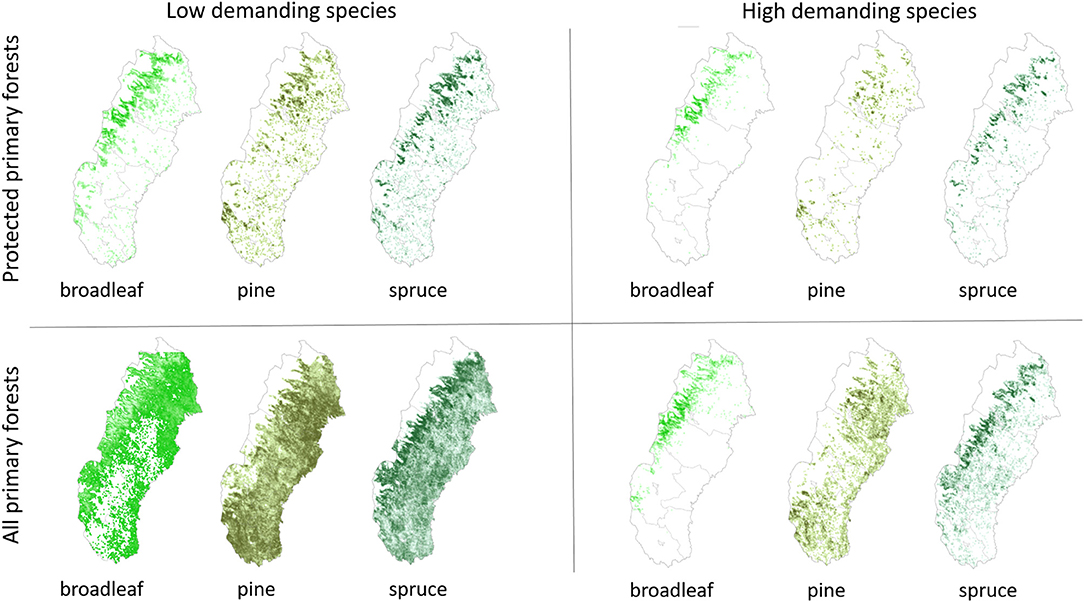
Figure 4. The spatial distribution of suitable habitat for virtual species specialized in three forest types (broadleaf, pine, and spruce) and having two levels of habitat demands (low- and high-demanding species).
The above regional differences for both the absolute (ha) and relative (percentage) increase of potential suitable habitat for the virtual species is presented in Figure 5. The increases were lower for the high-demanding species in the majority of the subregions. The largest increases in potential habitat area were observed for low-demanding pine species in Norrbotten east with 182 kha and in Dalarna with 176 kha. The proportional increase was highest for low-demanding pine species in Västerbotten east (2118%) and in Västernorrland (2058%), low-demanding spruce species in Västernorrland (2110%), and low-demanding broadleaf species in Norrbotten east (1989%).
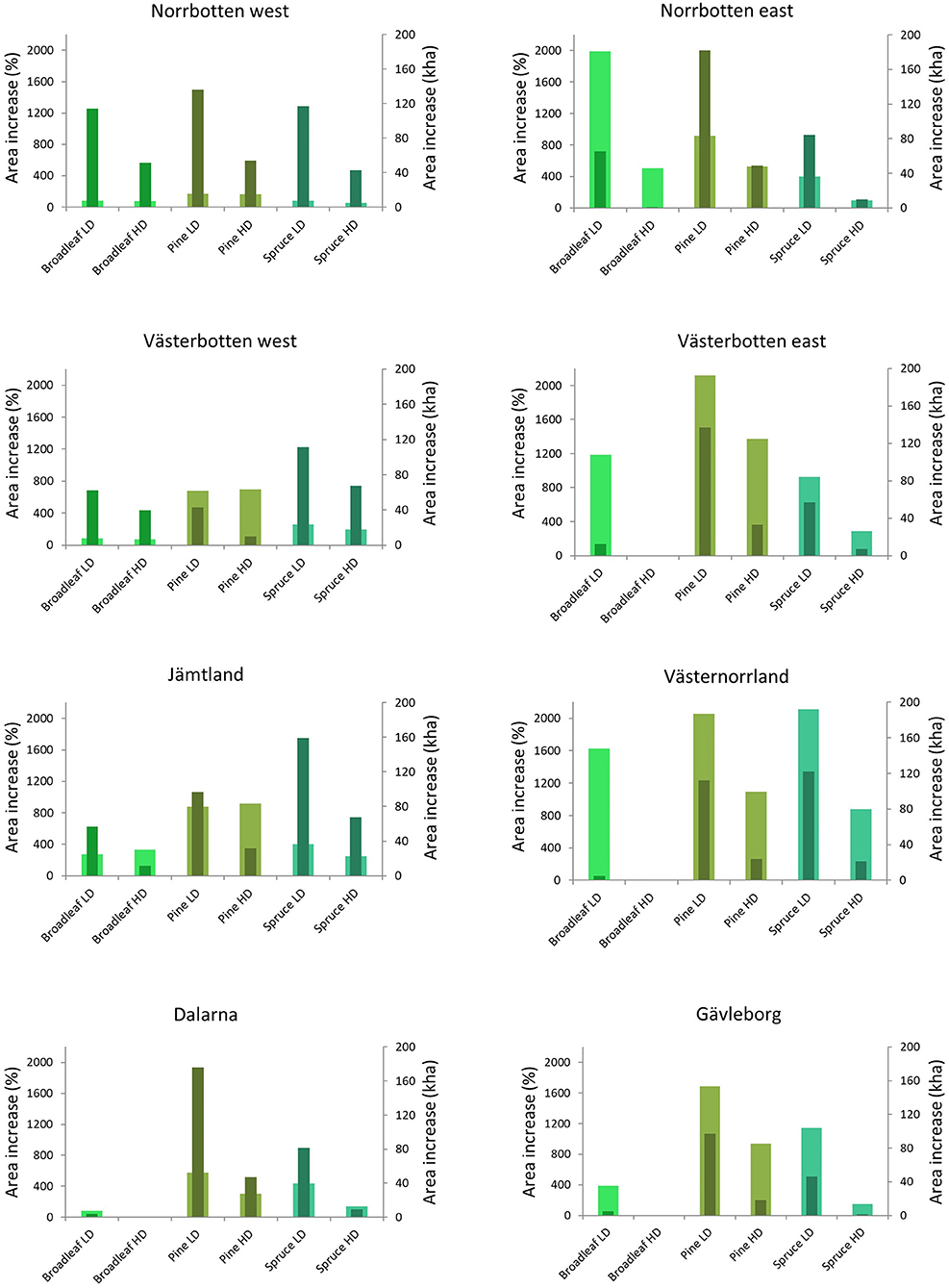
Figure 5. The increase of habitat area when protected primary forests are compared with all primary forests. Wide columns refer to percentages (left axis) and narrow columns to increase expressed in hectares (right axis). LD concern low-demanding virtual species, and HD concern high-demanding virtual species.
The habitat networks of different virtual species varied concerning the size and number of their spatial components, i.e., spatially connected areas that fulfill habitat requirements of the virtual species (Table 1). As expected, the spatial components of habitat networks of low-demanding species were both more numerous and larger than those of high-demanding species regardless of whether protected or all primary forests are considered. The difference in the number of spatial components between low- and high-demanding species was particularly high in the broadleaf species network based on all primary forests (>12 times more in low-demanding species) and in total area of the pine species network based on all primary forests (>6 times more in low-demanding species). A great majority (>94%) of connected spatial components in all networks were more than 100 ha in size (Table 1). Corresponding figures for components with a size over 1,000 ha were between 48.8% (pine, high-demanding species, all primary forests) and 89.4% (pine, low-demanding species, all primary forests). Very large (>10,000 ha) spatial components of habitat networks encompassed from 10.7% (pine, high-demanding species, all primary forests) to 77.5% (pine, low-demanding species, all primary forests). The number and size distribution of the spatial components of habitat networks at the subregional level is presented in Supplementary Tables A2–A4.
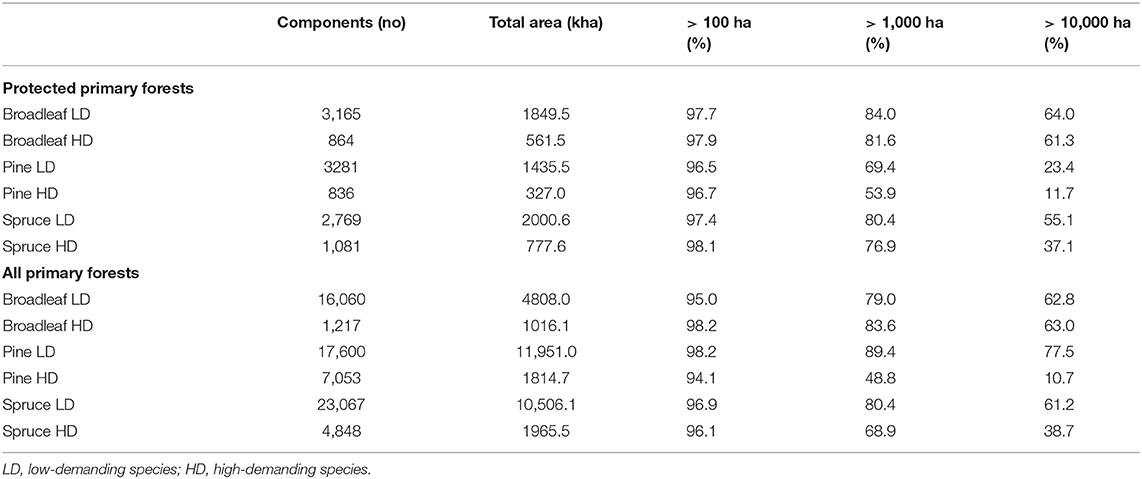
Table 1. Number, area, and size distribution of components of the habitat networks for different virtual species in the entire study region.
Discussion
Primary Forests as Providers of Habitat Networks
Functional habitat networks are the key feature of a green infrastructure (Liquete et al., 2015) with functionality implying the ability to support biodiversity conservation, landscape-scale ecological processes, and provisioning of a range of ecosystem services (Marini et al., 2019). Therefore, accurate mapping and management of habitat networks is central to landscape planning (Opdam et al., 2001). Furthermore, with the extensive transformation of natural and semi-natural forest landscapes, which is the consequence of intensive industrial forest clearfelling, ecosystem and forest landscape restoration is needed with restoration actions to be applied preferentially in places where they are expected to generate a higher level of functionality (Angelstam et al., 2003, 2011, 2020). In this paper, we examined a considerable part of the European Union's boreal forest and northern subalpine biomes by analyzing the spatial distribution of forests with confirmed (i.e., validated) and potential, high conservation value as habitat networks for species with different ecological and spatial requirements. As for all planning, setting a target makes it possible to define the needed management and governance steps forward and the measures that have to be taken to reach the target. Thereby, this study generates a spatial target map that identifies challenges and opportunities for implementing functional green infrastructure with respect to pine, spruce, and broadleaf forest-dependent species with low and high demands on habitat availability and its spatial configuration.
In our study, we first contrasted protected primary forests with all primary forests to assess the potential of unprotected areas to secure and strengthen functional green infrastructure. For generic landscape connectivity, the northwest part of the study area stands out as a largely functional network of protected valuable forest habitats, a fact that has been highlighted earlier (Jonsson et al., 2019; Svensson et al., 2019, 2020; Angelstam et al., 2020). Here, we add the information that currently unprotected primary forests in the southern part of the Scandinavian Mountains Green Belt could secure large-scale, overall north–south connectivity in the subalpine forests. This would increase the ecological resilience of this largely intact forest landscape in the face of climate change and other disturbances (Kuuluvainen et al., 2017). In the remaining part of the study area, the connectivity level of primary forests is drastically lower, particularly if only protected forests are considered. When including all primary forests, the overall connectivity clearly improves in parts of the study area, but it is still very far from a baseline situation with only natural fragmentation. Thereby, protecting all already known forests with high conservation values is not sufficient; their amount, density, and distribution do not provide even a basic large-scale structural connectivity. Hence, remote sensing and field-based inventories need to be intensified to identify and map remaining unknown areas of high conservation value and directed restoration operations need to be planned and executed in areas where there are gaps in forests with high conservation value. We argue that approaches comparing natural fragmentation with fragmentation caused by forestry is particularly needed in landscape planning, above all during mapping of places where restoration is needed to secure connectivity. Since green infrastructure studies on natural fragmentation of habitat appears to be rare (e.g., Haig et al., 2000; Gibson et al., 2017), we emphasize the value of providing a baseline connectivity map as a reference.
In the second step, we assessed primary forests through the eyes of habitat specialists, thereby exploring at a tactical level what is relevant for regional green infrastructure planning (Tittler et al., 2001). Our analysis of habitat networks for virtual species specializing in three different forest habitat types reveals large regional differences in both the available habitat area and the size distribution of components making up particular networks. The present distribution of habitat for species specialized in spruce, pine, and broadleaf forest habitats indicate the need for further protection of forest to increase the total area of the habitat networks and, thus, to improve both their structural and functional connectivity over a large scale. We found large numbers of single spatial components of habitat networks being small and isolated, particularly when including only protected primary forests. Angelstam et al. (2004) assessed the spatial needs for viable local populations of focal forest bird species (supporting 100 breeding females) in a landscape with a generally high amount of suitable habitat to be between 2,000 ha (woodlark Lullula arborea) and 60,000 ha (black woodpecker Dryocopus martius). Corresponding figures for landscapes with low but still acceptable levels of habitat availability were found to be 50,000 and 300,000 ha, respectively, for the two species. In this perspective, the majority of the spatial components of habitat networks identified in our study are very much below these thresholds (see Table 1 and Supplementary Tables A2–A4). This mismatch is clearly smaller if all primary forests are considered, but still, large gaps in networks are evident. This may lead to a situation in which many protected areas are unable to provide functional habitat for the species of conservation interest as demonstrated for several forest bird species (see also Orlikowska et al., 2020). This can be seen as the net result of an intensive forestry that has transformed a naturally dynamic boreal landscape into a heavily fragmented, managed production landscape (e.g., Kouki et al., 2001; Pohjanmies et al., 2017a; Jonsson et al., 2019).
Our large study region encompasses landscapes with different biogeographical conditions (topography, climate, soils, etc.) and with natural variation in forest types, particularly referring to the dominance of either Norway spruce or Scots pine (Nilsson, 1990). This may explain the presence of natural large gaps in habitat networks for virtual species linked to those tree species. Nevertheless, adding unprotected primary forest to our analysis improved the situation for species specialized in both pine and spruce forests quite substantially, particularly for less demanding species.
The situation is different in the case of species linked to broadleaf trees. On the one hand, habitat networks in subalpine landscapes dominated by mountain birch forest are abundant and very extensive for both types of habitat networks, i.e., based on protected primary forests and on all primary forests. Mountain birch forests and the coniferous-to-mountain birch transition zone in the Scandinavian mountain range have low tree-growth capacity, are not subject to forestry, and hence have a high level of naturalness (Jonsson et al., 2019; Svensson et al., 2020). On the other hand, a habitat network suitable for high-demanding species in broadleaf forests is largely absent from inland and coastal, representing a very large share of the study region (see Figure 4).
Broadleaf forest stands in naturally dynamic boreal landscapes are mostly linked to post-fire succession (Esseen et al., 1997). In addition to the current low frequency of wildfires in Fennoscandia in general (Rolstad et al., 2017), the intensive forest management applied in Sweden, aimed at maximizing production of coniferous timber and pulpwood, effectively limits the amount of mature stands with a large proportion of broadleaf trees (Bernes, 2011). Moreover, protecting forests does not ensure, in the absence of fire, the long-term maintenance of broadleaf trees in boreal forests (Bengtsson et al., 2003; Hardenbol et al., 2020). Stands rich in broadleaf trees are, except for the mountain birch forest, largely limited to the vicinity of settlements (Mikusiński et al., 2003) and different linear landscape features (e.g., riparian habitats) that cover relatively small areas and provide limited opportunities for forming effective habitat networks for different species (Ring et al., 2018).
Our analyses are based on the most recent national-scale data on protected and unprotected primary forests with known conservation value, with the pCF database treated as containing forests with the potential of being valuable due to the absence of recent clearfell-based harvesting (Svensson et al., 2019). Moreover, by stratifying these forests into three general types—pine-, spruce-, and broadleaf-dominated—based on the new detailed and high-resolution land cover data set and by analyzing their spatial distribution, we were able to further assess the spatial functionality of green infrastructure in boreal Sweden.
Nevertheless, we acknowledge the limitations in the data used and our approach as a whole. First, the pCF are somewhat overestimating the area of forests that have not been clearfelled, in particular, in the eastern inland and coastal part and along the river valleys, where forestry and other land use have a longer and more extensive history (Svensson et al., 2019). Therefore, the analysis based on all primary forests is optimistic; in reality, the habitat networks of our virtual species are probably less widespread and more fragmented; i.e., the situation is worse than we are able to show. Second, the virtual species used represent only two sets of spatial requirements and provide indicative rather than specific information on attributes for favorable conservation status. This calls for complementary species and species-group assessments. Moreover, and with the same limitations, distinguishing only three types of forests as habitat is also a simplification of the highly variable qualitative habitat preferences of the boreal and subalpine forest species occurring in Sweden (see, e.g., Berg et al., 1994). However, we argue that our evaluation of habitat networks is an important step toward improving our understanding of preconditions for securing functional green infrastructure in boreal Sweden.
Functional Green Infrastructure Based on Forests in Boreal Sweden
The EU strategy on green infrastructure (European Commission, 2013) aims at preserving and enhancing green infrastructure in order to preserve biodiversity and functional ecosystems and the provisioning of services and goods under climate change and ongoing forestry and other land use. It is understood that, with broader geographic scale, the greater the coherence and connectivity of the green infrastructure network, the greater are its conservation benefits. We demonstrate that the existing habitat networks represented collectively by both protected and unprotected pCF are able to support functional green infrastructure over sizable portions of the study region. However, as seen from the perspective of the three different forest types, there are obvious subregional differences in functionality. Thus, there are different habitat-type restoration needs in different regions.
We maintain that habitat distribution and density maps capturing the amount, spatial distribution (including the level of connectivity), and quality support large-scale green infrastructure planning. More detailed analyses of valuable forest networks could be applied at subnational or water catchment levels with the use of additional data concerning, e.g., distribution of species or habitats of conservation interest and available conservation planning software (Mikusiński et al., 2007; Snäll et al., 2016). Such detailed analyses should also include identification of local restoration needs that seem to be principally required for habitat networks based on broadleaf trees (e.g., Hof and Hjältén, 2018). Even if challenging, measures to create habitats dominated by broadleaf trees have been proven to be possible and successful (e.g., Hämäläinen et al., 2020).
Supporting biodiversity and ecosystem services through adding protected habitat is a central aspect in green infrastructure planning and implementation. Clearly, the most pressing green infrastructure challenge is the lack of broadleaf forest habitats, and restoration and re-creation is needed on a landscape scale throughout north Sweden. Although the potential for increasing functional connectivity of the already protected forests is large, it is unlikely to represent a realistic scenario for green infrastructure development. It has to be assumed that active forest management will continue into the future and that clearfelling will be a continued practice. It should be emphasized that the green infrastructure concept includes and does not exclude land use.
The approach taken in this study allows for identifying green infrastructure gaps to identify possibilities to restore certain types of habitat in certain places based on what is available and to define types and characteristics of missing habitats that need to be re-created. Thereby, our study contributes to direct green infrastructure planning and management guidelines with regional resolution. The increased capacity for each case assessed can be matched toward a green infrastructure target and included in regional green infrastructure plans to be reflected on the national plans.
The work on securing green infrastructure based on HCVF is evidently in conflict with other interests in boreal Sweden (Pohjanmies et al., 2017b; Jonsson, 2019). Simplified and intensively managed monocultures with timber, pulp, and biomass production as a main aim are not able to sustain biodiversity and multiple ecosystem services (Gamfeldt et al., 2013; Halme et al., 2013; Pohjanmies et al., 2017a). The current level of protection of HCVF in boreal Sweden, except for its northwestern part, is highly insufficient to support functional green infrastructure (Jonsson et al., 2019; Angelstam et al., 2020). Moreover, the level of protected area is far from the quantitative target #11 of the Aichi Biodiversity goals and does not satisfy the national Environmental Quality Objective “Sustainable Forests” (Swedish Parliament, 1998). As the conflict over the current and future use of Sweden's boreal forests intensifies (e.g., Sténs and Mårald, 2020), the current situation is at a value chain crossroad between strengthened nature conservation and intensified wood production in boreal forests.
Data Availability Statement
The raw data supporting the conclusions of this article will be made available by the authors, without undue reservation.
Author Contributions
GM, JS, and BGJ delineated the general idea of the paper. GM provided the first draft. EHO and JWB performed all the spatial analyses. All authors contributed to the final text of the article and approved the submitted version.
Funding
This work has been supported by the Swedish Environmental Protection Agency Grants No. NV-03501-15 and NV-03728-17.
Conflict of Interest
The authors declare that the research was conducted in the absence of any commercial or financial relationships that could be construed as a potential conflict of interest.
Acknowledgments
We appreciate all the constructive comments provided by the reviewers.
Supplementary Material
The Supplementary Material for this article can be found online at: https://www.frontiersin.org/articles/10.3389/fevo.2020.595730/full#supplementary-material
References
Aerts, R., and Honnay, O. (2011). Forest restoration, biodiversity and ecosystem functioning. BMC Ecol. 11:29. doi: 10.1186/1472-6785-11-29
Ahlcrona, E., Giljam, C., and Wennberg, S. (2017). Kartering av kontinuitetsskog i boreal region. Metria AB på uppdrag av Naturvårdsverket, 79.
Anantharaman, R., Hall, K., Shah, V., and Edelman, A. (2020). Circuitscape in julia: high performance connectivity modelling to support conservation decisions. JuliaCon Proc. 1:58. doi: 10.21105/jcon.00058
Andersson, K., Angelstam, P., Elbakidze, M., Axelsson, R., and Degerman, E. (2013). Green infrastructures and intensive forestry: need and opportunity for spatial planning in a Swedish rural-urban gradient. Scand. J. Forest Res. 28, 143–165. doi: 10.1080/02827581.2012.723740
Angelstam, P., Andersson, K., Axelsson, R., Elbakidze, M., Jonsson, B. -G., and Roberge, J. -M. (2011). Protecting forest areas for biodiversity in Sweden 1991-2010: policy implementation process and outcomes on the ground. Silva Fenn. 45, 1111–1133. doi: 10.14214/sf.90
Angelstam, P., Manton, M., Green, M., Jonsson, B. G., Mikusiński, G., Svensson, J., et al. (2020). Sweden does not meet agreed national and international forest biodiversity targets: a call for adaptive landscape planning. Landscape Urban Plan. 202:103838. doi: 10.1016/j.landurbplan.2020.103838
Angelstam, P., Mikusiński, G., Rönnback, B.-I., Östman, A., Lazdinis, M., Roberge, J.-M., et al. (2003). Two-dimensional gap analysis: a tool for efficient conservation planning and biodiversity policy implementation. Ambio 32, 527–534. doi: 10.1579/0044-7447-32.8.527
Angelstam, P., Roberge, J. M., Lõhmus, A., Bergmanis, M., Brazaitis, G., Dönz-Breuss, M., et al. (2004). Habitat modelling as a tool for landscape-scale conservation: a review of parameters for focal forest birds. Ecol. Bull. 51, 427–453. Available online at: https://www.jstor.org/stable/20113327
Angelstam, P. K. (1998). Maintaining and restoring biodiversity in European boreal forests by developing natural disturbance regimes. J. Veget. Sci. 9, 593–602. doi: 10.2307/3237275
Anon (2017). Skogliga värdekärnor i Sverige – sammanfattande beskrivning av dataurval och nuläge 2015-2016. Bilaga 2a till Naturvårdsverkets och Skogsstyrelsens redovisning av regeringsuppdrag om Värdefulla skogar, 2017-01-30.
Anon (2018). Forestry Act 1979:429. Skogsvårdslag SFS no. 1979:429, revised to SFS 1993:553 and updated to SFS 2018:1413.
Bengtsson, J., Angelstam, P., Elmqvist, T., Emanuelsson, U., Folke, C., Ihse, M., et al. (2003). Reserves, resilience and dynamic landscapes. Ambio 32, 389–396. doi: 10.1579/0044-7447-32.6.389
Berg, Å., Ehnström, B., Gustafsson, L., Hallingbäck, T., Jonsell, M., and Weslien, J. (1994). Threatened plant, animal, and fungus species in Swedish forests: distribution and habitat associations. Cons. Biol. 8, 718–731. doi: 10.1046/j.1523-1739.1994.08030718.x
Bernes, C. (2011). Biodiversity in Sweden. Vol Monitor 22. Stockholm: Swedish Environmental Protection Agency.
Börjesson, P., Hansson, J., and Berndes, G. (2017). Future demand for forest-based biomass for energy purposes in Sweden. For. Ecol. Manag. 383, 17–26. doi: 10.1016/j.foreco.2016.09.018
Boucher, J., Hebert, C., Ibarzabal, J., and Bauce, E. (2016). High conservation value forests for burn-associated saproxylic beetles: an approach for developing sustainable post-fire salvage logging in boreal forest. Insect Conserv. Divers. 9, 402–415. doi: 10.1111/icad.12175
Brancalion, P. H. S., Bello, C., Chazdon, R. L., Galetti, M., Jordano, P., Lima, R. A. F., et al. (2018). Maximizing biodiversity conservation and carbon stocking in restored tropical forests. Conser. Lett. 11:12454. doi: 10.1111/conl.12454
Brandt, J. P., Flannigan, M. D., Maynard, D. G., Thompson, I. D., and Volney, W. J. A. (2013). An introduction to Canada's boreal zone: ecosystem processes, health, sustainability, and environmental issues. Environ. Rev. 21, 207–226. doi: 10.1139/er-2013-0040
CBD (2010). Convention on Biological Diversity Strategic plan for biodiversity 2011–2020 and the Aichi targets. Convention on Biological Diversity, Montreal. Available online at: https://www.cbd.int/sp/targets/ (accessed March 22, 2020).
Ceccherini, G., Duveiller, G., Grassi, G., Lemoine, G., Avitabile, V., Pilli, R., et al. (2020). Abrupt increase in harvested forest area over Europe after (2015). Nature 583, 72–77. doi: 10.1038/s41586-020-2438-y
Chatzimentor, A., Apostolopoulou, E., and Mazaris, A. D. (2020). A review of green infrastructure research in Europe: challenges and opportunities. Landscape Urban Plan. 198:103775. doi: 10.1016/j.landurbplan.2020.103775
Chazdon, R. L. (2017). Landscape restoration, natural regeneration, and the forests of the future. Ann. Mo. Bot. Gard. 102, 251–257. doi: 10.3417/2016035
Christensen, N. L. Jr. (2014). An historical perspective on forest succession and its relevance to ecosystem restoration and conservation practice in North America. For. Ecol. Manag. 330, 312–322. doi: 10.1016/j.foreco.2014.07.026
Curtis, P. G., Slay, C. M., Harris, N. L., Tyukavina, A., and Hansen, M. C. (2018). Classifying drivers of global forest loss. Science 361, 1108–1111. doi: 10.1126/science.aau3445
Edenius, L., and Mikusiński, G. (2006). Utility of habitat suitability models as biodiversity assessment tools in forest management. Scand. J. Forest Res. 21, 62–72. doi: 10.1080/14004080500486989
EEA (2019). The European Environment — State and Outlook (2020). Knowledge for transition to a sustainable Europe. Copenhagen: EEA.
ESRI Inc. (2015). ArcGIS 10.3.1 for Desktop, Redlands, CA: Environmental Systems Research Institute.
Esseen, P. -A., Ehnström, B., Ericson, L., and Sjöberg, K. (1997). Boreal forests. Ecol. Bull. 46, 16–47.
European Commission (2013). Green Infrastructure (GI) — Enhancing Europe's Natural Capital. Communication From the Commission to the European Parliament, the Council, the European Economic and Social Committee and the Committee of the Regions. Brussels: European Commission Environment
Felton, A., Ranius, T., Roberge, J.-M., Öhman, K., Lamas, T., Hynynen, J., et al. (2017). Projecting biodiversity and wood production in future forest landscapes: 15 key modeling considerations. J. Environ. Manage. 197, 404–414. doi: 10.1016/j.jenvman.2017.04.001
Flather, C. H., Wilson, K. R., Dean, D. J., and McComb, W. C. (1997). Identifying gaps in conservation networks: of indicators and uncertainty in geographic-based analyses. Ecol. Appl. 7, 531–542. doi: 10.1890/1051-0761(1997)007[0531:IGICNO]2.0.CO;2
FRA (2020). General Forest Resources Assessment 2020. Terms and Definitions. Forest Resources Assessment Working Paper 188. Rome: Food and Agriculture Organization of the United Nations.
Gamfeldt, L., Snäll, T., Bagchi, R., Jonsson, M., Gustafsson, L., Kjellander, P., et al. (2013). Higher levels of multiple ecosystem services are found in more diverse forests. Nature Comm. 4:1340. doi: 10.1038/ncomms2328
Gauthier, S., Bernier, P., Kuuluvainen, T., Shvidenko, A. Z., and Schepaschenko, D. G. (2015). Boreal forest health and global change. Science 349, 819–822. doi: 10.1126/science.aaa9092
Gibson, L. A., Cowan, M. A., Lyons, M. N., Palmer, R., Pearson, D. J., and Doughty, P. (2017). Island refuges: conservation significance of the biodiversity patterns resulting from ‘natural' fragmentation. Biol. Conserv. 212, 349–356. doi: 10.1016/j.biocon.2017.06.010
Gustafsson, L., Berglind, M., Granström, A., Grelle, A., Isacsson, G., Kjellander, P., et al. (2019). Rapid ecological response and intensified knowledge accumulation following a north European mega-fire. Scand. J. Forest Res. 34, 234–253. doi: 10.1080/02827581.2019.1603323
Gustafsson, L., and Perhans, K. (2010). Biodiversity conservation in Swedish forests: ways forward for a 30-year-old multi-scaled approach. Ambio 39, 546–554. doi: 10.1007/s13280-010-0071-y
Haddad, N. M., Brudvig, L. A., Clobert, J., Davies, K. F., Gonzalez, A., Holt, R. D., et al. (2015). Habitat fragmentation and its lasting impact on Earth's ecosystems. Sci. Adv. 1:1500052. doi: 10.1126/sciadv.1500052
Haig, A. R., Matthes, U., and Larson, D. W. (2000). Effects of natural habitat fragmentation on the species richness, diversity, and composition of cliff vegetation. Can. J. Bot. 78, 786–797. doi: 10.1139/b00-047
Halme, P., Allen, K. A., Aunins, A., Bradshaw, R. H. W., Brumelis, G., Cada, V., et al. (2013). Challenges of ecological restoration: lessons from forests in northern Europe. Biol. Conserv. 167, 248–256. doi: 10.1016/j.biocon.2013.08.029
Hämäläinen, K., Junninen, K., Halme, P., and Kouki, J. (2020). Managing conservation values of protected sites: how to maintain deciduous trees in white-backed woodpecker territories. For. Ecol. Manag. 461:117946. doi: 10.1016/j.foreco.2020.117946
Hansen, M. C., Potapov, P. V., Moore, R., Hancher, M., Turubanova, S. A., Tyukavina, A., et al. (2013). High-resolution global maps of 21st-century forest cover change. Science 342, 850–853. doi: 10.1126/science.1244693
Hardenbol, A. A., Junninen, K., and Kouki, J. (2020). A key tree species for forest biodiversity, European aspen (Populus tremula), is rapidly declining in boreal old-growth forest reserves. For. Ecol. Manag. 462:118009. doi: 10.1016/j.foreco.2020.118009
Hedenås, H., Christensen, P., and Svensson, J. (2016). Changes in vegetation cover and composition in the Swedish mountain region. Ecol. Monit. Assess. 188:452. doi: 10.1007/s10661-016-5457-2
Hermoso, V., Moran-Ordonez, A., Lanzas, M., and Brotons, L. (2020). Designing a network of green infrastructure for the EU. Landscape Urban Plan. 196:103732. doi: 10.1016/j.landurbplan.2019.103732
Hof, A. R., and Hjältén, J. (2018). Are we restoring enough? Simulating impacts of restoration efforts on the suitability of forest landscapes for a locally critically endangered umbrella species. Restor. Ecol. 26, 740–750. doi: 10.1111/rec.12628
Jennings, S., Nussbaum, R., Judd, N., Evans, T., Iacobelli, T., Jarvie, J., et al. (2003). The High Conservation Value Forest Toolkit. Edition I. Oxford: ProForest, 12, 1–62.
Jonsson, B. G., Svensson, J., Mikusiński, G., Manton, M., and Angelstam, P. (2019). European Union's last intact forest landscapes are at a value chain crossroad between multiple use and intensified wood production. Forests 10:564. doi: 10.3390/f10070564
Koen, E. L., Bowman, J., Sadowski, C., and Walpole, A. A. (2014). Landscape connectivity for wildlife: development and validation of multispecies linkage maps. Methods Ecol. Evol. 5, 626–633. doi: 10.1111/2041-210X.12197
Kormos, C. F., Mackey, B., Della Sala, D. A., Kumpe, N., Jaeger, T., Mittermeier, R. A., et al. (2017). “Primary forests: definition, status and future prospects for global conservation,” in Encyclopedia of the Anthropocene eds D. A. DellaSala, and M. I. Goldstein (Amsterdam: Elsevier), 31–41. doi: 10.1016/B978-0-12-809665-9.09711-1
Kouki, J., Löfman, S., Martikainen, P., Rouvinen, S., and Uotila, A. (2001). Forest fragmentation in Fennoscandia: linking habitat requirements of wood associated threatened species to landscape and habitat changes. Scand. J. Forest Res. 16, 27–37. doi: 10.1080/028275801300090564
Kuuluvainen, T. (2009). Forest management and biodiversity conservation based on natural ecosystem dynamics in Northern Europe: the complexity challenge. Ambio 38, 309–315. doi: 10.1579/08-A-490.1
Kuuluvainen, T. (2016). Ecosystem Management of the Boreal Forest. Oxford Research Encyclopedia of Environmental Science (Oxford: Oxford University Press). doi: 10.1093/acrefore/9780199389414.013.15
Kuuluvainen, T., Hofgaard, A., Aakala, T., and Jonsson, B. G. (2017). North Fennoscandian mountain forests: history, composition, disturbance dynamics and the unpredictable future. For. Ecol. Manag. 385, 140–149. doi: 10.1016/j.foreco.2016.11.031
Lambeck, R. J. (1997). Focal species: a multi-species umbrella for nature conservation. Conserv. Biol. 11, 849–856. doi: 10.1046/j.1523-1739.1997.96319.x
Ledig, F. T. (1992). Human impacts on genetic diversity in forest ecosystems. Oikos 63, 87–108. doi: 10.2307/3545518
Lindahl, K. B., Stens, A., Sandström, C., Johansson, J., Lidskog, R., Ranius, T., et al. (2017). The Swedish forestry model: more of everything? Forest Policy Econ. 77, 44–55. doi: 10.1016/j.forpol.2015.10.012
Liquete, C., Kleeschulte, S., Dige, G., Maes, J., Grizetti, B., Olah, B., et al. (2015). Mapping green infrastructure based on ecosystem services and ecological networks: a Pan-European case study. Environ. Sci. Policy 54, 268–280. doi: 10.1016/j.envsci.2015.07.009
Lundmark, A., Josefsson, T., and Östlund, L. (2013). The history of clear-cutting in northern Sweden – driving forces and myths in boreal silviculture. For. Ecol. Manag. 307:112–122. doi: 10.1016/j.foreco.2013.07.003
Manton, M., Angelstam, P., and Mikusiński, G. (2005). Modelling habitat suitability for deciduous forest focal species – a sensitivity analysis using different satellite land cover data. Landscape Ecol. 20, 827–839. doi: 10.1007/s10980-005-3703-z
Marini, L., Bartomeus, I., Rader, R., and Lami, F. (2019). Species–habitat networks: a tool to improve landscape management for conservation. J. Appl. Ecol. 56, 923–928. doi: 10.1111/1365-2664.13337
McRae, B. H., Dickson, B. G., Keitt, T. H., and Shah, V. B. (2008). Using circuit theory to model connectivity in ecology, evolution, and conservation. Ecology 89, 2712–2724. doi: 10.1890/07-1861.1
Mikusiński, G., Angelstam, P., and Sporrong, U. (2003). Distribution of deciduous stands in villages located in coniferous forest landscapes in Sweden. Ambio 32, 520–526. doi: 10.1579/0044-7447-32.8.520
Mikusiński, G., Bubnicki, J. W., Churski, M., Czeszczewik, D., Walankiewicz, W., and Kuijper, D. (2018). Is the impact of loggings in the last primeval lowland forest in Europe underestimated? The conservation issues of Białowieża Forest. Biol. Conserv. 227, 266–274. doi: 10.1016/j.biocon.2018.09.001
Mikusiński, G., and Edenius, L. (2006). Assessment of spatial functionality of old forest in Sweden as habitat for virtual species. Scand. J. Forest Res. 21, 73–83. doi: 10.1080/14004080500487045
Mikusiński, G., Pressey, R. L., Edenius, L., Kujala, H., Moilanen, A., Niemelä, J., et al. (2007). Conservation planning in forest landscapes of Fennoscandia and an approach to the challenge of Countdown (2010). Conserv. Biol. 21, 1445–1454. doi: 10.1111/j.1523-1739.2007.00833.x
Moen, J., Rist, L., Bishop, K., Chapin, I. I. I. F. S., Ellison, D., et al. (2014). Eye on the Taiga: removing global policy impediments to safeguard the boreal forest. Conserv. Lett. 7, 408–418. doi: 10.1111/conl.12098
Müller, J., Noss, R. F., Thorn, S., Bässler, C., Leverkus, A. B., and Lindenmayer, D. (2019). Increasing disturbance demands new policies to conserve intact forest. Conserv. Lett. 12:12449. doi: 10.1111/conl.12449
Naumov, V., Manton, M., Elbakidze, M., Rendenieks, Z., Priednieks, J., Uhlianets, S., et al. (2018). How to reconcile wood production and biodiversity conservation? The Pan-European boreal forest history gradient as an “experiment”. J. Environ. Manag. 218, 1–13. doi: 10.1016/j.jenvman.2018.03.095
Neteler, M., Bowman, M., Landa, M., and Metz, M. (2012). GRASS GIS: a multi-purpose Open Source GIS. Environ. Modell. Softw. 31, 124–130. doi: 10.1016/j.envsoft.2011.11.014
Opdam, P., Foppen, R., and Vos, C. (2001). Bridging the gap between ecology and spatial planning in landscape ecology. Landscape Ecol. 16, 767–779. doi: 10.1023/A:1014475908949
Orlikowska, E. H., Svensson, J., Roberge, J. -M., Blicharska, M., and Mikusiński, G. (2020). Hit or miss? Evaluating the effectiveness of Natura 2000 for conservation of forest bird habitat in Sweden. Global Ecol. Conserv. 22:00939. doi: 10.1016/j.gecco.2020.e00939
Patru-Stupariu, I., Angelstam, P., Elbakidze, M., Huzui, A., and Andersson, K. (2013). Using forest history and spatial patterns to identify potential high conservation value forests in Romania. Biodivers. Conserv. 22, 2023–2039. doi: 10.1007/s10531-013-0523-3
Pohjanmies, T., Triviño, M., Le Tortorec, E., Mazziotta, A., Snäll, T., and Mönkkönen, M. (2017a). Impacts of forestry on boreal forests: an ecosystem services perspective. Ambio 46, 743–755. doi: 10.1007/s13280-017-0919-5
Pohjanmies, T., Triviño, M., Le Tortorec, E., Salminen, H., and Mönkkönen, M. (2017b). Conflicting objectives in production forests pose a challenge for forest management. Ecosyst. Serv. 28, 298–310. doi: 10.1016/j.ecoser.2017.06.018
Potapov, P., Hansen, M. C., Laestadius, L., Turubanova, S., Yaroshenko, A., Thies, C., et al. (2017). The last frontiers of wilderness: tracking loss of intact forest landscapes from 2000 to (2013). Sci. Adv. 3:1600821. doi: 10.1126/sciadv.1600821
Potapov, P., Yaroshenko, A., Turubanova, S., Dubinin, M., Laestadius, L., Thies, C., et al. (2008). Mapping the world's intact forest landscapes by remote sensing. Ecol. Soc. 13:51. doi: 10.5751/ES-02670-130251
Ring, E., Widenfalk, O., Jansson, G., Hölmstrom, H., Högbom, L., and Sonesson, J. (2018). Riparian forests along small streams on managed forest land in Sweden. Scand. J. Forest Res. 33, 133–146. doi: 10.1080/02827581.2017.1338750
Rolstad, J., Blanck, Y. L., and Storaunet, K. O. (2017). Fire history in a western Fennoscandian boreal forest as influenced by human land use and climate. Ecol. Monogr. 87, 219–245. doi: 10.1002/ecm.1244
Senior, M. J. M., Brown, E, Villalpando, P., and Hill, J. K. (2014). Increasing the scientific evidence base in the “high conservation value” (HCV) approach for biodiversity conservation in managed tropical landscapes. Conserv. Lett. 8, 361–367. doi: 10.1111/conl.12148
SEPA (2019). Nationella Marktäckedata (NMD). Swedish Environmental Protection Agency. Available online at https://www.naturvardsverket.se/Sa-mar-miljon/Kartor/Nationella-Marktackedata-NMD/ (accessed March 16, 2020).
Slätmo, E., Nilsson, K., and Turunen, E. (2019). Implementing green infrastructure in spatial planning in Europe. Land 8:62. doi: 10.3390/land8040062
SLU (2020). Forest Statistics (2020). Official Statistics of Sweden. Swedish University of Agricultural Sciences Umeå. ISSN 0280-0543. 158pp.
Snäll, T., Lehtomäki, J., Arponen, A., Elith, J., and Moilanen, A. (2016). Green infrastructure design based on spatial conservation prioritization and modeling of biodiversity feautures and ecosystem services. Environ. Manag. 57, 251–256. doi: 10.1007/s00267-015-0613-y
Stanturf, J. A., Palik, B. J., and Dumroese, R. K. (2014). Contemporary forest restoration: a review emphasizing function. For. Ecol. Manag. 331, 292–323. doi: 10.1016/j.foreco.2014.07.029
Sténs, A., and Mårald, E. (2020). “Forest property rights under attack”: actors, networks and claims about forest ownership in the Swedish press 2014–2017. Forest Policy Econ. 111:102038. doi: 10.1016/j.forpol.2019.102038
Svensson, J., Andersson, J., Sandström, P., Mikusiński, G., and Jonsson, B. G. (2019). Landscape trajectory of natural boreal forest loss as an impediment to green infrastructure. Conserv. Biol. 33, 152–163. doi: 10.1111/cobi.13148
Svensson, J., Bubnicki, J. W., Jonsson, B.-G., Andersson, J., and Mikusiński, G. (2020). Conservation significance of intact forest landscapes in the Scandinavian mountains green belt. Landscape Ecol. 35, 2113–2131. doi: 10.1007/s10980-020-01088-4
Swedish Forest Agency (2019). Statistik om formellt skyddad skogsmark, frivilliga avsättningar, hänsynsytor samt improduktiv skogsmark. Report 2019/18, Jönköping: Skogsstyrelsen.
Swedish Parliament (1998). Svenska miljömål. Miljöpolitik för ett hållbart Sverige. Government Proposition No. 1997/98:145, Stockholm: Swedish Parliament.
Tittler, R., Messier, C., and Burton, P. J. (2001). Hierarchical forest management planning and sustainable forest management in the boreal forest. Forest. Chron. 77, 998–1005. doi: 10.5558/tfc77998-6
Torresan, C., Berton, A., Carotenuto, F., Di Gennaro, S. F., Gioli, B., Matese, A., et al. (2017). Forestry applications of UAVs in Europe: a review. Int. J. Remote Sens. 38, 2427–2447. doi: 10.1080/01431161.2016.1252477
Verkerk, P. J., Fitzgerald, J. B., Datta, P., Dees, M., Hengeveld, G. M., Lindner, M., et al. (2019). Spatial distribution of the potential forest biomass availability in Europe. For. Ecosyst. 6:5. doi: 10.1186/s40663-019-0163-5
Vos, C. C., Verboom, J., Opdam, P. F. M., and Ter Braak, C. J. F. (2001). Toward ecologically scaled landscape indices. Am. Nat. 157, 24–41. doi: 10.1086/317004
Watson, J. E. M., Evans, T., Venter, O., Williams, B., Tulloch, A., Stewart, C., et al. (2018). The exceptional value of intact forest ecosystems. Nat. Ecol. Evol. 2, 599–610. doi: 10.1038/s41559-018-0490-x
White, J. C., Coops, N. C., Wulder, M. A., Vastaranta, M., Hilker, T., and Tompalski, P. (2016). Remote sensing technologies for enhancing forest inventories: a review. Can. J. Remote Sens. 42, 619–641. doi: 10.1080/07038992.2016.1207484
Keywords: continuity forests, primary forests, virtual species, Sweden, connectivity, green infrastructure, Scandinavian Mountains Green Belt
Citation: Mikusiński G, Orlikowska EH, Bubnicki JW, Jonsson BG and Svensson J (2021) Strengthening the Network of High Conservation Value Forests in Boreal Landscapes. Front. Ecol. Evol. 8:595730. doi: 10.3389/fevo.2020.595730
Received: 17 August 2020; Accepted: 08 December 2020;
Published: 21 January 2021.
Edited by:
Anouschka R. Hof, Wageningen University and Research, NetherlandsReviewed by:
Marie-Josee Fortin, University of Toronto, CanadaNicole Fenton, Université du Québec en Abitibi Témiscamingue, Canada
Copyright © 2021 Mikusiński, Orlikowska, Bubnicki, Jonsson and Svensson. This is an open-access article distributed under the terms of the Creative Commons Attribution License (CC BY). The use, distribution or reproduction in other forums is permitted, provided the original author(s) and the copyright owner(s) are credited and that the original publication in this journal is cited, in accordance with accepted academic practice. No use, distribution or reproduction is permitted which does not comply with these terms.
*Correspondence: Grzegorz Mikusiński, Z3J6ZWdvcnoubWlrdXNpbnNraUBzbHUuc2U=