- 1Carrera de Biología, Universidad Técnica Particular de Loja, Loja, Ecuador
- 2Laboratorio de Ecología Tropical y Servicios Ecosistémicos, Departamento de Ciencias Biológicas, Universidad Técnica Particular de Loja, Loja, Ecuador
Urbanization is a major driver of stream ecosystem impairment and is typically associated with multiple stressors and species loss. A challenge is to understand how those stressors alter the relationship between biodiversity and ecosystem functioning (B-EF). Amongst the Andean streams of southern Ecuador, we assessed the response of shredder diversity and organic matter breakdown (OMB) to urbanization and identified the urban-associated stressors disrupting the B-EF relationship. A leaf-litter bag experiment during stable flow conditions in 2016 was carried out to quantify total OMB and shredder-mediated OMB, which was estimated to represent the B-EF relationship. We calculated the taxonomic and functional diversity of shredder invertebrates associated with leaf packs. Also, a suite of physicochemical and habitat stressors was measured weekly during the field experiment. Along with the urbanization gradient, both taxonomic and functional diversity of shredders declined while OMB rates decreased. Shredders were absent and their contribution to OMB was null at the most urbanized sites. The B-EF relationship was interrupted through nutrient enrichment, physical habitat homogenization, riparian vegetation disturbance, and leaf-litter availability as a consequence of urbanization. Our results demonstrate how species loss spreads to and affects ecosystem functions in urbanized streams and how environmental stressors alter the B-EF relationship. Better land-use practices are crucial in Andean catchments to guarantee ecosystem services which are the result of successful B-EF relationships.
Introduction
Aquatic ecosystems contain high biodiversity, but at the same time, they exhibit faster species loss than marine or terrestrial ecosystems (Collen et al., 2014; Sánchez-Bayo and Wyckhuys, 2019). Unfortunately, species loss also implies a reduction of ecosystem functions (Cardinale et al., 2006). Species diversity is crucial for the dynamics and basic functioning of ecosystems, and for that reason, the relationship between biodiversity and ecosystem functioning (B-EF) has been well established and is a central research issue (Loreau et al., 2001; Tilman et al., 2014). Also, the B-EF relationships can be altered by environmental gradients, leading to complications in predicting the effects of species loss within ecosystems subjected to environmental stress (McKie et al., 2009). Therefore, it is imperative to understand the mechanisms that interrupt this relationship by way of environmental disturbance gradients such as urbanization.
Urbanization is a complex process involving permanent changes to the landscape such as the transformation of land use from rural (i.e., forest, agriculture) to urban (Antrop, 2004). Also, urbanization is related to numerous stressors that interact synergistically upon streams (Walsh et al., 2005), affecting the hydrology, chemistry, and aquatic communities via multiple pathways (Paul and Meyer, 2001; Walsh et al., 2005). Urbanized streams often show a reduction in channel width and streambed substrate size due to sediment inputs, as well as showing channelization, impervious surfaces and flashy stream flows (Allan, 2004; Walsh et al., 2005). Water quality is degraded via an increase in nutrient concentrations and other pollutants such as pathogens, metals, hydrocarbons, and microplastics from sewage inputs and urban runoff (Guerrero-Latorre et al., 2018; Vásquez et al., 2019; Donoso and Rios-Touma, 2020; Montalvo-Cedillo et al., 2020). Also, the riparian margins of urbanized streams typically suffer native vegetation loss with invasions of exotic plants (Guida-Johnson et al., 2017). When these stream characteristics are altered by urbanization there will be adverse consequences for the macroinvertebrate communities (Roy et al., 2003; de Jesús-Crespo and Ramírez, 2011; Hassett et al., 2018), resulting in species loss (Chadwick et al., 2006; Urban et al., 2006) that can lead to a disruption of the biodiversity–ecosystem function relationship (Meyer et al., 2005; Tilman et al., 2014). Therefore, the interruption of this interaction can turn into a serious issue at the ecosystem level when keystone species are removed or replaced from streams (Iñiguez-Armijos et al., 2016).
Macroinvertebrates can play important roles in stream ecosystem functioning (Wallace and Webster, 1996). For instance, organic matter breakdown (OMB) is mediated by shredder invertebrates, as well as by leaching, microbial conditioning, and physical abrasion (Gessner et al., 1999). Indeed, shredder importance on OMB has been well documented in different regions (Jonsson et al., 2001; Li and Dudgeon, 2009; Dangles et al., 2011). Shredders fragment coarse particulate organic matter (CPOM) into smaller particles to make organic carbon available for other aquatic consumers or ecosystem processes (Gessner et al., 1999; Ramírez and Gutiérrez-Fonseca, 2014). Most of the leaf-shredding invertebrates in Neotropical streams belong to Trichoptera and Plecoptera, and a few taxa are members of Coleoptera, Ephemeroptera, and Diptera (see Table 1 in Ramírez and Gutiérrez-Fonseca, 2014). Indeed, shredders of these orders have been demonstrated to be essential for the functioning of montane streams in the Neotropical region (Encalada et al., 2010; Dangles et al., 2011; Iñiguez-Armijos et al., 2016, 2018b), despite being a species group showing a low richness in the tropics as compared to temperate streams (Boyero et al., 2011). Unfortunately, as an effect of urbanization, most of the sensitive aquatic insects (i.e., Trichoptera, Plecoptera, and Ephemeroptera) are reaching alarming rates of species loss worldwide (Sánchez-Bayo and Wyckhuys, 2019) removing taxa with key functional feeding habits (e.g., Trichoptera shredders), and “breaking down” the linkages of these taxa with certain crucial ecosystem functions such as OMB.
Ecosystem functioning refers to ecological processes determined by the activity of biodiversity (Tilman et al., 2014). Nonetheless, this close relationship can be altered by several environmental stressors. Taking into consideration the Millennium Ecosystem Assessment (MEA, 2005), urbanization has a large effect on the integrity of freshwater ecosystems and its associated environmental stressors (e.g., nutrient enrichment) are seriously affecting stream ecosystem functions such as OMB (Meyer et al., 2005). Naturally, nutrients are important drivers of OMB in the tropics (Tiegs et al., 2019), and nutrient enrichment will disturb this ecosystem function as well as other stream parameters. Several studies have reported that urbanization has negatively affected both decomposer communities and OMB through nutrient enrichment and hydrologic alteration (e.g., Chadwick et al., 2006; Martins et al., 2015; Iñiguez-Armijos et al., 2016). However, because biodiversity and ecosystem functioning respond to stressors in different pathways (Sandin and Solimini, 2009), the joint application of structural and functional metrics to quantify the response of stream ecosystems to a stressor such as urbanization is adequate (Clapcott et al., 2012). Taxonomic metrics can indicate how the organization of aquatic communities responds to environmental stress, while functional biological traits can provide information on multiple-stressor effects and insights into potential mechanisms (Lange et al., 2014). Besides, if ecosystem processes such as OMB rates are quantified, a more comprehensive response to environmental stress gradients can be obtained (Riipinen et al., 2009; Voß et al., 2015), which is challenging when only structural metrics are considered.
In this study, we used shredder diversity (taxonomic and functional diversity) and OMB rates to quantify the effects of urbanization on stream integrity in an Andean catchment influenced by a rapid and unmanaged metropolitan expansion. In particular, we examined (i) how shredder invertebrates are affected by an urbanization gradient, (ii) whether OMB rates are similarly affected by the urbanization gradient, and (iii) which environmental variables altered by urbanization are disturbing the biodiversity–ecosystem function relationship (i.e., shredder-mediated OMB rates). We hypothesized that the loss of shredder diversity due to urbanization also spreads to and affects ecosystem functioning in Andean streams by decreasing organic C processing.
Materials and Methods
Study Area and Sampling Sites
This study was conducted in the city of Loja and its surroundings, the southern Ecuadorian Andes between 2,000 and 2,350 m a.s.l. (Figure 1). The study area is a densely populated headwater catchment (≈ 200,000 inhabitants) of the Zamora River basin with 276 km2 mainly covered by native vegetation and pasture, as well as urbanization (Iñiguez-Armijos et al., 2014). The native vegetation is composed of evergreen mountain forests, humid and dry shrubs, cloud forest, and páramo (a type of tropical alpine grasslands). Historically, native vegetation has been disturbed by human activities and has been restricted to the upper section of the catchment (Ochoa-Cueva et al., 2015; Tapia-Armijos et al., 2015). Pasture land is located in the surroundings of the city and extends to the native vegetation. Pastures are mainly dedicated to livestock and a few small plots for farming while small pine plantations can be also found (Iñiguez-Armijos et al., 2016). The urban area of the city is highly developed, presenting a mixture of residential and commercial land. The city is located along the valley and has grown toward the outskirts. This urban sprawl has transformed land cover around the city from forest to pasture and then to urban over decades, leaving an urbanization gradient within the catchment. Sewage is not treated and agricultural and urban runoff is not managed. Also, the city evidences heavy metal accumulation and solid waste pollution within the environment and river waters (Benítez et al., 2019; Vásquez et al., 2019).
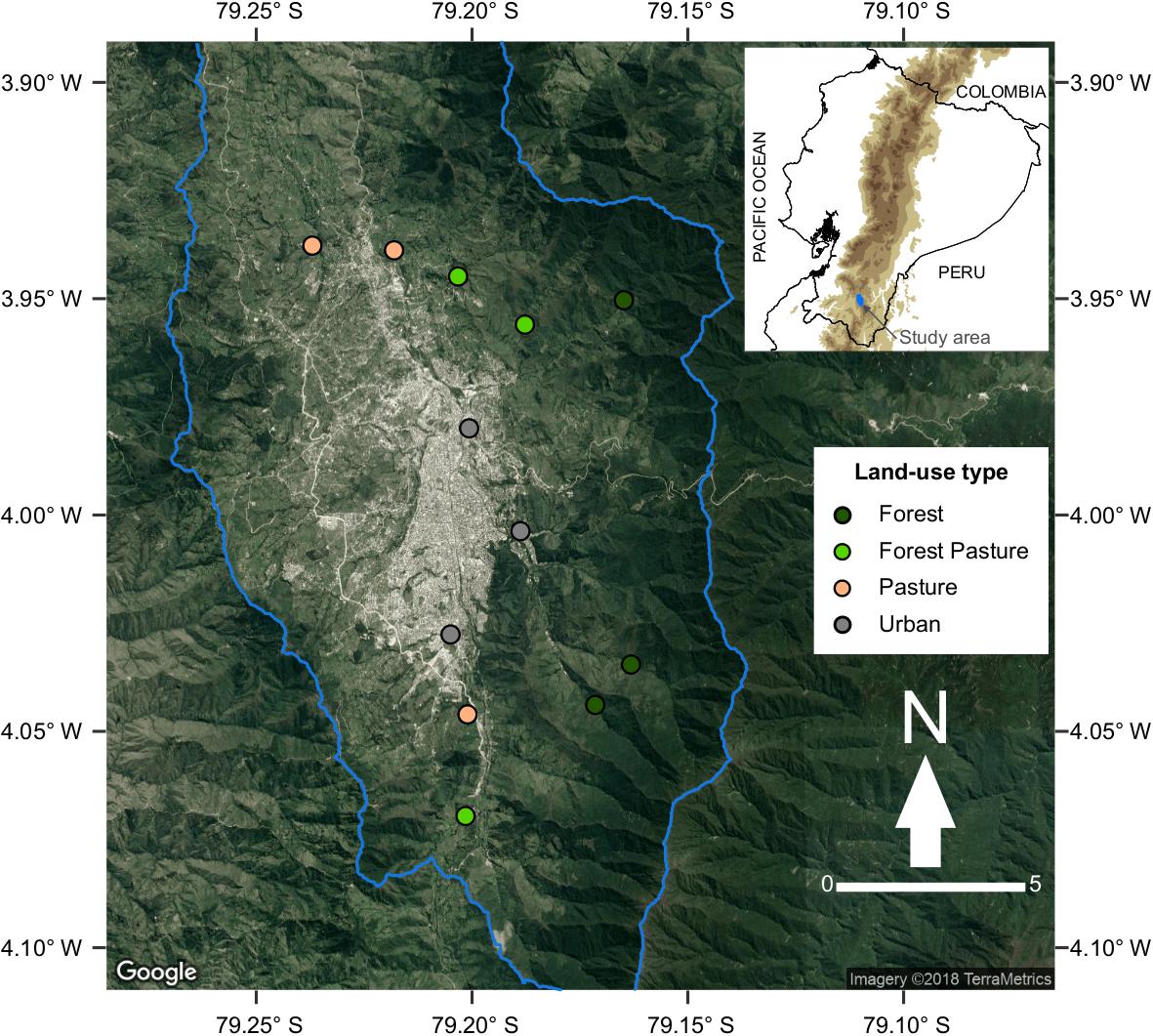
Figure 1. Location of the study area and sampling sites around the city of Loja in the southern Ecuadorian Andes. Colors indicate the land-use gradient studied from forest to urban sites. Modified from Chuquimarca et al. (2019).
Therefore, we selected 12 independent streams along such an urbanization gradient, having three replicated streams at urban (Ur), pasture (Pa), forest mixed with pasture (FP), and forest (Fo) sites, respectively (Figure 1). Also, this urbanization gradient has been demonstrated to be environmentally contrasting among sites and has negatively affected biota (Chuquimarca et al., 2019). All studied streams are similar in size (2nd order) and geology. Fo sites are located in a secondary mature forest, and for that reason they were considered as reference sites. The riparian vegetation is mostly dominated by trees and shrubs of native species. These streams are healthy ecosystems and are used for the water supply of the city of Loja. FP sites are characterized by the presence of native vegetation mixed with cropped grasses of Pennisetum clandestinum, and planted trees of Alnus acuminata, and Eucalyptus globulus along the riparian margins. Cattle ranching is occasional at these sites, and some areas show a narrow minor road parallel to the streams and very few houses in proximity. Pa sites are characterized by several open-canopy reaches dominated by cropped grasses of P. clandestinum with isolated planted trees of A. acuminata, E. globulus, and Salix pyramidalis. The grassland is frequently fertilized with animal manure and is entirely dedicated to cattle ranching. There is no livestock exclusion along the riparian margins, thus cattle normally use these streams as a water source. There are minor settlements and people have small crops for daily subsistence. Pa streams show bank erosion, channel narrowing, water abstraction, and agricultural drainages. In Ur sites, riparian margins are restricted to a thin (2–10 m width) section with planted trees (e.g., A. acuminata, E. globulus, and S. pyramidalis) and exotic ornamental vegetation bordered by impervious surfaces or buildings. Ur streams show concrete channelization, untreated sewage loads, stormwater discharges, and garbage accumulation. At each stream, we placed a sampling site consisting of a 30 m reach located downstream of the target land cover.
Environmental Variables
In the dry season of 2016 (September), during the period of stable flow conditions, a total of 16 environmental variables were measured (Table 1). Water temperature, conductivity, pH, and dissolved oxygen were measured using a YSI 556 multi-probe meter (Yellow Springs, OH, United States); whereas the concentration of dissolved inorganic nitrogen (DIN = nitrate + nitrite + ammonium), dissolved reactive phosphorus (DRP), and turbidity were determined in the laboratory using standard methods (APHA, 2017). Channel width and depth, and current velocity were determined at four to six points along each sampling site using a flowmeter FP311 (Global Water, Rancho Cordova, CA, United States). These variables were measured four times in 7-days intervals throughout the OMB experiment (see below). Substrate size was estimated visually by four randomly placed plots (1 × 1 m) at each sampling site. The percent of silt, sand, gravel, pebble, cobble, boulder, and bedrock was determined according to the Wentworth scale to calculate a substrate index (Harding et al., 2009).
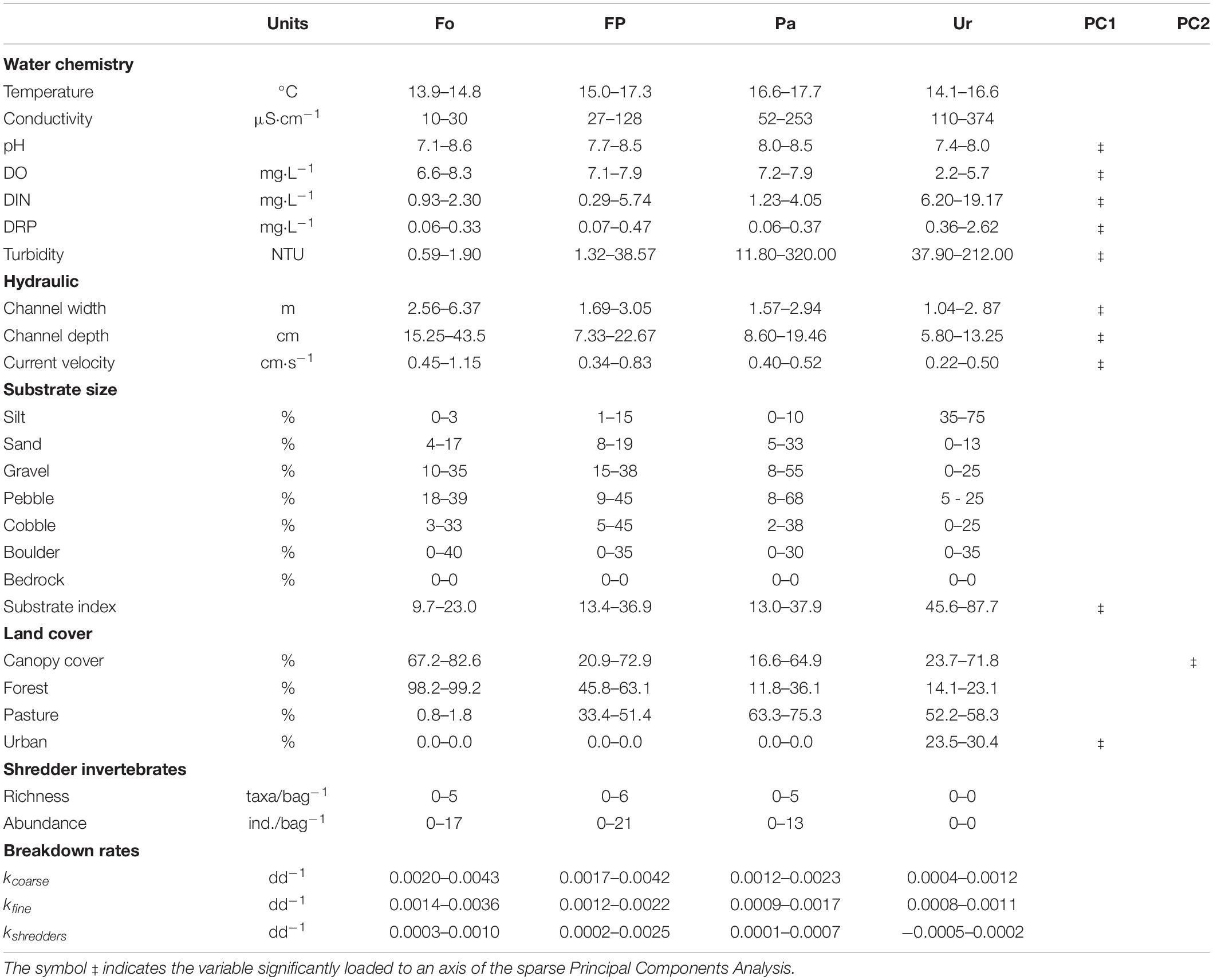
Table 1. Range values (min – max) of the set of environmental variables, shredder diversity, and breakdown rates measured in Andean streams (n = 12) along an urbanization gradient in southern Ecuador.
We quantified the percentage of land covered by native forest, pasture, crops, plantations, and urbanization within each catchment of the 12 selected streams. Land cover was obtained upstream of the sampling sites using an open-access GIS and a land-use map for 2016 obtained from another study. Also, we quantified riparian canopy cover (%) at four equidistant points from hemispherical photographs taken at 1.3 m above the water surface. Photographs were acquired with a digital camera equipped with a fish-eye lens and then processed in Gap Light Analyzer Version 2.0.
Ecosystem Functioning
Leaf-litter breakdown was used as a measure of stream ecosystem functioning. We applied the leaf bag technique (Bärlocher, 2005; Benfield et al., 2017) using freshly fallen leaves of Andean alder (Alnus acuminata Kunth), croton (Croton rimbachii Croizat), and eucalyptus (Eucalyptus globulus Labill.). These species are common in the riparian zone of the studied streams, where croton is the only native species while alder and eucalyptus are introduced species. By using different litter types, we considered potential variation in shredder colonization and breakdown rates. We enclosed 4 ± 0.05 g of air-dried leaves in 15 × 15 cm coarse mesh bags (10 mm) to allow access by shredders. Moreover, fine mesh bags (0.5 mm) were also used to exclude shredders and to estimate microbial breakdown. This step allowed us to quantify the contribution of shredders to total breakdown (McKie et al., 2009), as explained below.
At each sampling site, four coarse and four fine mesh bags of each litter type were tied to the substrate with iron bars and incubated for 30 days before retrieval. The timing was selected to achieve 50% of mass loss based on a previous study (Iñiguez-Armijos et al., 2016), which minimizes variation between leaf bags as a result of extended incubation periods (Tiegs et al., 2009). After retrieval, leaf bags were transported individually in zip-lock bags to the laboratory, and leaf material was carefully rinsed to remove sediments and to recover invertebrates. Then, we dried (48 h/50°C), weighed, combusted (4 h/500°C), and reweighed leaf material to obtain ash-free dry mass (AFDM) (Bärlocher, 2005). An extra set of 5 leaf bags of each litter type was similarly treated to correct for mass loss of the final AFDM caused by handling (Bärlocher, 2005).
Over the 30 days, at each sampling site, we recorded water temperature in 1 h intervals using HOBO pendant data loggers (Onset, MA, United States) placed within the mesh bags and secured to the iron bars. This data was used to calculate the sum of degree days (dd–1) during the incubation period to standardize the breakdown rate (k) by temperature, and to reduce differences between stream types. Then, breakdown rates were estimated using an exponential decay model per degree days (k dd–1), as follows:
where W0 is initial air-dried mass, Wt is final AFDM at time t, and t is incubation time in degree-days. Total breakdown rates (ktotal), which is the breakdown in coarse mesh bags, were corrected for microbial breakdown (kcoarse – kfine) to calculate the contribution of shredders invertebrates (kshredders) to this ecosystem process (McKie et al., 2009; Table 1).
Shredder Diversity
All invertebrates recovered from the coarse mesh bags were counted and identified to the lowest taxonomic level (usually genus) using available literature for South America (Dominguez and Fernández, 2009). Then, we assigned the invertebrates to a functional feeding group (Ramírez and Gutiérrez-Fonseca, 2014). For this study, we only selected shredder invertebrates from the macroinvertebrate community to be directly associated with leaf litter breakdown. We are aware that macroinvertebrates can exhibit a dietary shift under polluted conditions, but shedders will not change their food source and are prone to be eliminated when severe pollution is present (Yule et al., 2010). We classified all shredder invertebrates according to three biological traits (size, life cycle duration, respiration) and 14 different modalities based on the literature available (Tachet, 2000). Traits such as food, dietary preference, or reproduction were excluded for being similar among shredders in this study.
For each litter type, we calculated taxonomic richness (taxa per bag–1) and abundance (individuals per bag–1) of shredders in the R environment (R Development Core Team, 2020) using the “vegan” and “BiodiversityR” packages (see Table 1). Also, we estimated functional richness and Rao’s quadratic entropy (RaoQ) based on Gower’s distances of our biological traits using the “FD” package (Laliberté et al., 2014). In particular, we used these metrics for being adequate for testing ecological impairment across environmental gradients. Functional richness measures the amount of multidimensional functional space occupied by the community in multi-trait studies; whereas RaoQ measures the differences among functional entities within a community (Villéger et al., 2008; Laliberté and Legendre, 2010). Since no shredder species were recorded in the most urbanized streams, for practical and representational purposes we added zero values for functional diversity at these sites.
Urbanization Gradient
We produced an urbanization gradient using the environmental variables indicated in Table 1 in a sparse Principal Components Analysis (sPCA) (Zou et al., 2006; Croux et al., 2013) because it facilitates interpretation by reducing the number of variables loading in a principal component. We ran the sPCA using the “pcaPP” package (Filzmoser et al., 2018), and following an open access routine for R available at https://github.com/rbslandau/Function_div, which has been used to obtain a similar environmental stress gradient (Voß and Schäfer, 2017). Before sPCA, the 16 environmental variables were inspected for normality using the Shapiro–Wilk test (“stats” package) and were log or square root-transformed as needed. Logit transformation was used for percentages such as canopy cover. To address collinearity, we applied the Variation Inflation Factor (VIF) using the “usdm” package (Naimi, 2015) and considering a VIF threshold of >7 to exclude variance-inflated variables (Zuur et al., 2007). We used this method because non-linear relationships can also be detected in contrast to correlation analysis (Feld et al., 2016). Consequently, a set of 11 variables was analyzed in the sPCA, obtaining a first principal component (PC1) explaining 53% of total variance between streams and loading 10 out of 11 environmental variables (see Table 1). The scores of the PC1 increased from forested to urbanized streams facilitating environmental interpretation, i.e., increasing scores are related to increased stress. Therefore, we refer to PC1 as the urbanization gradient hereafter.
Response to Urbanization Gradient
Zero-inflated models (ZIM) were applied to determine how taxonomic and functional diversity of shredder invertebrates are affected by the urbanization gradient. Using the “glmmTMB” package (Brooks et al., 2017), ZIMs were built based on Poisson and Negative binomial distributions for taxonomic and functional metrics, respectively. Otherwise, generalized additive models (GAMs) with a gaussian distribution were applied to determine if total (ktotal) and shredders-mediated (kshredders) breakdown rates were similarly affected by the urbanization gradient. We used GAMs because they can fit variation in data structures that have a complex non-linear relationship with response variables (Zuur et al., 2007). Model residuals were tested for normality and homoscedasticity assumptions.
Finally, we applied GAMs to identify which environmental variables associated with the urbanization gradient significantly alter the B-EF relationship. Therefore, we did a variable selection from the environmental variables associated with PC1 following a procedure described in Feld et al. (2016). This step allowed us to select the least statistically redundant predictors and the most ecologically meaningful ones per each group of variables. To represent the B-EF relationship, we used kshredders as an indicator of such interaction considering that this rate reveals the role of shredder invertebrates on OMB during the incubation period. Variables were log or square root-transformed before analysis if needed. We started with a full model including all predictors until obtaining a simplified model selected with Akaike’s information criterion (AIC) (Zuur et al., 2007; Wood, 2017). GAM models were built using the “mgcv” package (Wood, 2017).
Results
Shredder Diversity and Urbanization
A total of 291 shedder invertebrates belonging to nine genera of eight families and four orders were collected across all sites (Supplementary Table S1). No shredders were found at urban sites, whereas the lowest shredder richness was observed at pasture sites with seven taxa and the highest at mixed forest-pasture and forest sites with eight taxa each. Moreover, mixed forest-pasture and forest sites had a greater number of shredder invertebrate individuals than other sites. ZIM models (Table 2), indicated that the effect of the urbanization gradient was negative on both taxonomic richness and the abundance of shredders (Figures 2A,B). Urbanization also negatively affected the functional richness and RaoQ of shredder invertebrates, but the response of functional diversity was a gradual decrease along the urbanization gradient (Figures 2C,D).
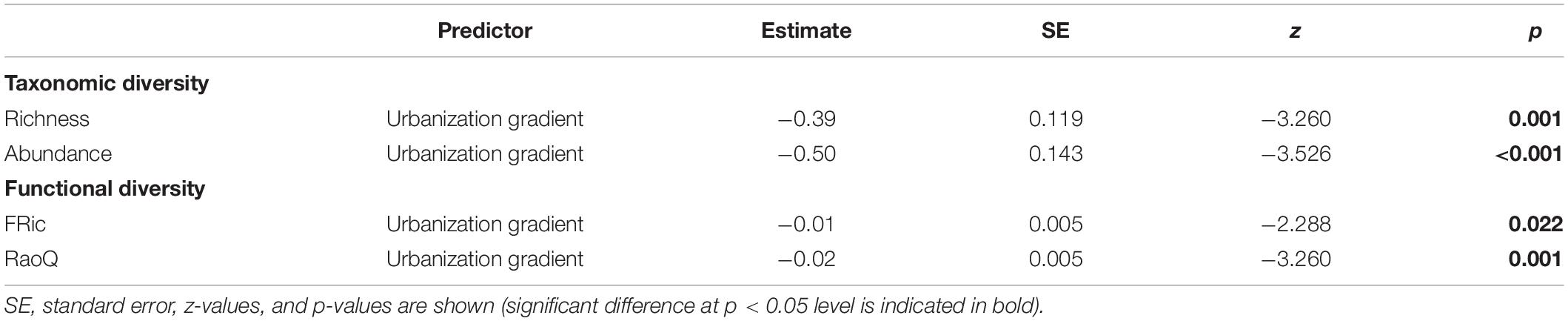
Table 2. Summary of the zero-inflated models (ZIM) showing the response of the taxonomic and functional diversity of shredder invertebrates along an urbanization gradient in Andean streams of southern Ecuador.
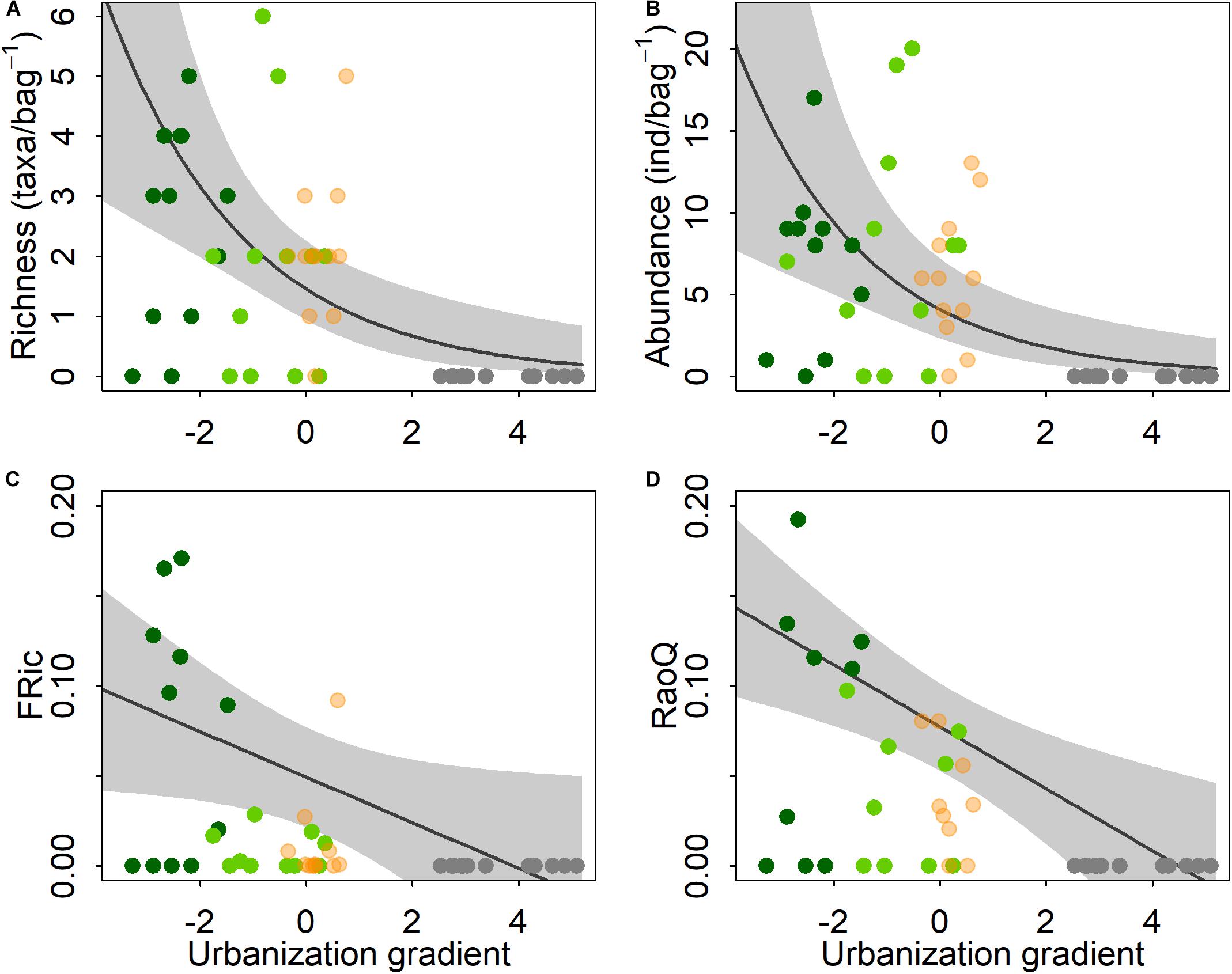
Figure 2. Response of the taxonomic richness (A), abundance (B), functional richness (C), and Rao’s quadratic entropy (D) of shredder invertebrates to an urbanization gradient in Andean streams. Gray line represents the ZIM model fitting and the shadows show the 95% confidence interval. Colored circles represent the forest (dark green), forest-pasture (light green), pasture (salmon), and urban (gray) sites, respectively.
Breakdown Rates and Urbanization
On average, ktotal was 0.0019 dd–1 and the contribution of shredders to OMB was much lower than microorganisms (Table 1 and Supplementary Table S2); indeed, kshredders represented around 21% of total breakdown. GAM models (Table 3), indicated that OMB responded negatively along the urbanization gradient, being faster in forest sites and diminishing toward urbanized sites (Figure 3A). However, the response of OMB mediated by shredders showed a drastic decrease at urban sites (Figure 3B).
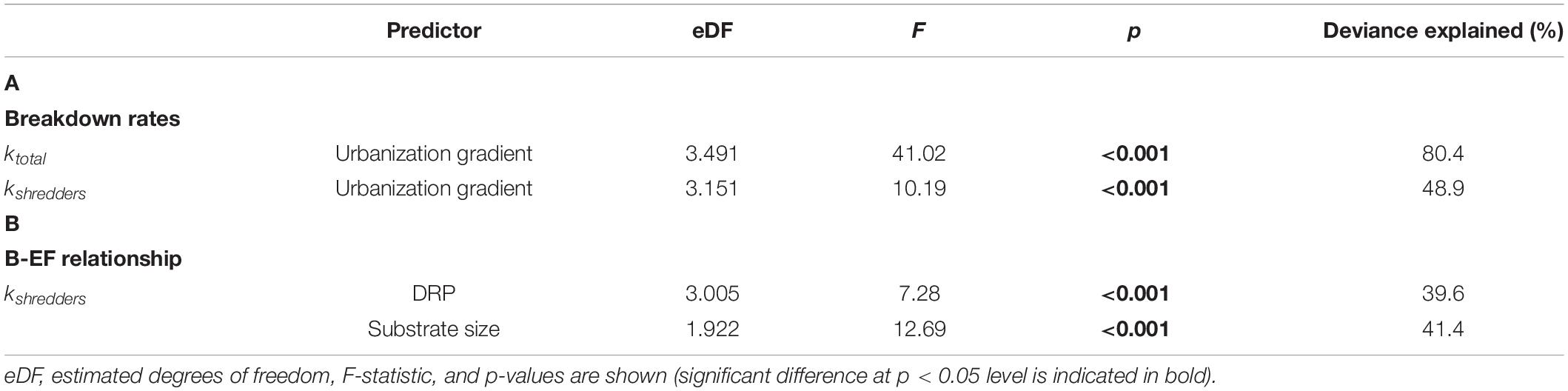
Table 3. Summary of the generalized additive models (GAMs) showing (A) the response of the total and shedder-mediated breakdown rates along an urbanization gradient in Andean streams of southern Ecuador; and (B) the response of the biodiversity-ecosystem function (B-EF) relationship, represented by kshredders, to the environmental stressors associated with urbanization that were identified as the best predictors.
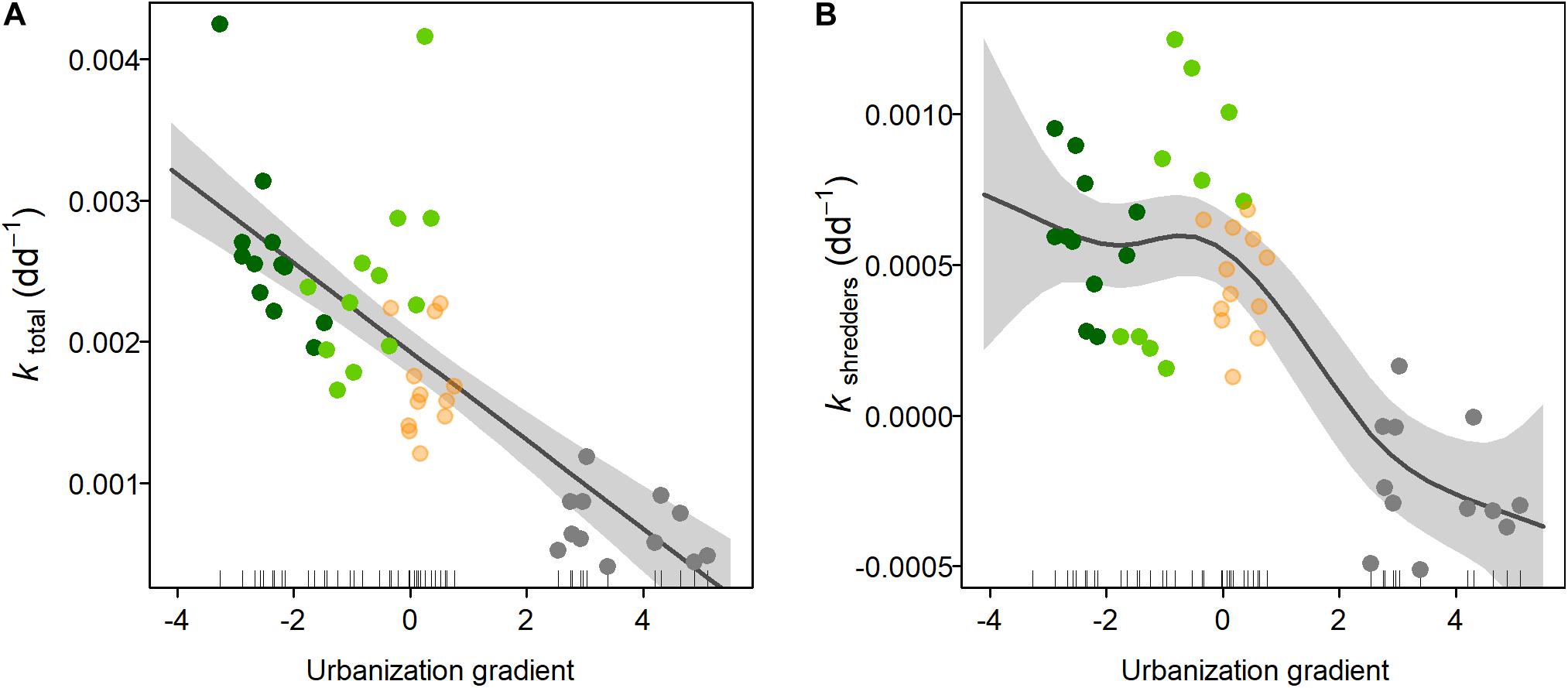
Figure 3. Response of the organic matter breakdown to an urbanization gradient in Andean streams. ktotal (A) is breakdown estimated from coarse mesh bags, whereas kshredders (B) is the difference of the breakdown estimated between coarse and fine mesh bags. Gray line represents the GAM model fitting and the shadows show the 95% confidence interval. Colored circles represent the forest (dark green), forest-pasture (light green), pasture (salmon), and urban (gray) sites, respectively.
Urbanization and the Biodiversity and Ecosystem Function Relationship
After the selection process for environmental variables associated with the urban gradient (i.e., PC1) for GAM modeling, DRP, pH, turbidity, and substrate size were retained. DIN and dissolved oxygen were highly correlated with DRP; whereas channel width and depth, and current velocity were highly correlated with substrate size. We excluded urban land cover for being a variance-inflated variable. GAM modeling indicated that DRP and substrate size were the most meaningful variables and had a strong negative effect on kshredders, i.e., B-EF relationship (Figure 4), each explaining 39 and 41% of the deviance explained, respectively (Table 3).
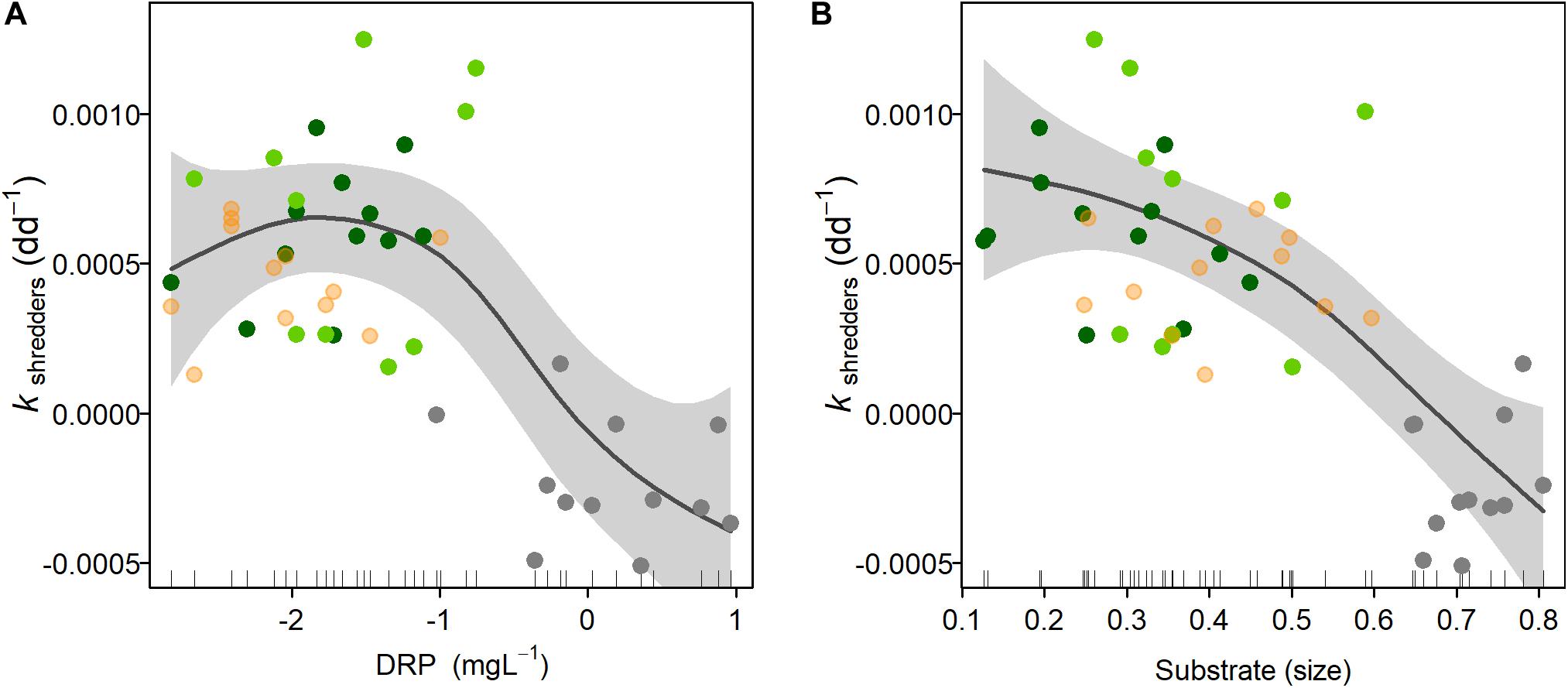
Figure 4. Response of the biodiversity-ecosystem function (B-EF) relationship against two environmental variables associated with the urbanization gradient. The B-EF relationship is represented by kshredders, while dissolved reactive phosphorus (DRP) (A) and substrate size (B) were selected as the most meaningful variables. Gray line represents the GAM model fitting and the shadows show the 95% confidence interval. Colored circles represent the forest (dark green), forest-pasture (light green), pasture (salmon), and urban (gray) sites, respectively.
Discussion
Although the relationship of B-EF has been extensively studied in temperate ecosystems (Tilman et al., 2014), it is still necessary to understand how environmental disturbance gradients can alter this relationship in neotropical streams. In this study, we assessed the response of taxonomic and functional diversity of shredder invertebrates as well as OMB rates along an urbanization gradient in Andean streams. We detected that the diversity of shredder invertebrates decreases from natural to more urbanized sites and that shredders are absent in urban streams. We also found that OMB rates are negatively affected along the urbanization gradient, slowing down this ecosystem process toward urban sites and that urban-associated variables representing water chemistry (e.g., DRP) and physical habitat (e.g., substrate size) of the streams are altering the relationship between shredders and OMB.
DRP is produced by phosphate inputs to stream ecosystems from wastewater coming from sewage systems of the urban areas (Paul and Meyer, 2001). In our study, DRP concentrations were low at forest sites but increased considerably toward urban sites (up to 25-fold greater). At the same time, we observed a decrease in the taxonomic and functional diversity of shredder invertebrates and a slowdown in OMB along the urbanization gradient. Although a moderate increase in the concentration of nutrients such as P can stimulate OMB by shredders in temperate streams (Woodward et al., 2012), in montane Andean streams the increasing of P has been shown to slow down this OMB (Iñiguez-Armijos et al., 2016). Other stream parameters such as dissolved oxygen and DIN were negative and positively associated with DRP, respectively. This correlation can be explained by the same land-use intensification along the urbanization gradient, which facilitates the incorporation of organic and inorganic pollutants that interact synergistically and reduce the stream ecosystem integrity (Paul and Meyer, 2001). The increase in N and P concentrations from sewage (e.g., human waste and detergents) and higher water temperatures caused by light penetration can decrease the dissolved oxygen (Allan, 2004). Nutrient enrichment and warmer temperatures favor microbial activity which increases respiration rates and decreases the availability of oxygen in the streams (Walsh et al., 2005). High nutrient concentrations and water temperature, along with reduced dissolved oxygen, combined, can interact reducing or eliminating altogether shredder invertebrates (Couceiro et al., 2006; Iñiguez-Armijos et al., 2016). This pattern is supported by our findings, but we also observed that the reduction in OMB by shredders can be attributed to an abrupt loss of their taxonomic and functional diversity toward more urbanized sites as an effect of nutrient enrichment, water temperature and low availability of dissolved oxygen. Our evidence makes it clear that the aforementioned water chemistry variables have a negative effect on this B-EF relationship, but we believe that other urban-associated stressors are also disruptive.
Concerning substrate size, riparian land-use change, and bank erosion, all produce streambed sedimentation in urbanized streams, reducing substrate size and its heterogeneity affecting negatively upon macroinvertebrate diversity (Couceiro et al., 2010). After the loss of macroinvertebrate taxa such as shredders due to the alteration of substrate size and type, their ecological role will be also affected. In our study, we found that the more the urbanization gradient increases, the less the substrate size which is largely dominated by sand, silt, and mud. This consequence can explain the strong negative effect of substrate size on OMB mediated by shredders and their loss of taxonomic and functional diversity. Size and type of the substrate determine the presence of several members of the macroinvertebrate community in Andean stream ecosystems since the substrate provides habitat, refuge, and food (Miserendino and Pizzolon, 2004). Therefore, as the heterogeneity and size of the substrate decrease, there would be a loss of taxonomic and functional diversity of leaf-shredding invertebrates, thus affecting their contribution to OMB at the ecosystem level. Other hydraulic parameters such as channel width and depth, and current velocity were negatively correlated to substrate size. In the Andes, streams in natural areas are wide and deep and show high current velocity and turbulent flow, while the streambed presents differing substrate sizes and contains several shredder invertebrate taxa (Iñiguez-Armijos et al., 2018a; Vimos-Lojano et al., 2020). On the contrary, the studied streams at urban sites presented a laminar flow and the slowest current velocity as a consequence of sediment deposition in the streambed dominated by small particle sizes such as silt and mud. However, during rainy events, we could observe flashy storm flows in these urban sites, which is a classical hydrologic pattern exhibited in urban streams (Walsh et al., 2005). Also, these streams were more narrow due to channelization/streambed erosion, and have been documented as lacking shredder invertebrates (Iñiguez-Armijos et al., 2016). All these aspects have been observed in other tropical streams resulting as well in the elimination of shredders and their food source at the most urbanized streams (Yule et al., 2010, 2015). The alteration of these hydraulic variables, as we have described here, has negatively affected the OMB in tropical streams (e.g., Martins et al., 2015); and in our case, we believe that it has resulted in the loss of the taxonomic and functional diversity of shredder invertebrates, their availability, as well as their capacity for organic matter processing in Andean stream ecosystems.
Although we did not measure litter inputs and litter quality, we believe that shredders were also affected by the availability and quality of allochthonous organic matter along the urbanization gradient. Leaf litter inputs and retention of leaf packs in the streams are dependent on riparian land use and in-stream conditions such as substrate and current velocity (Hagen et al., 2010). The homogenization of streambed substrate and flashy stream flows may be preventing the accumulation of leaf packs toward the most urbanized sites which also contributes to shredder loss. Leaf packs are crucial for shredders as they provide habitat and food (Richardson, 1992). On the other hand, urban streams are typically poorly shaded and warmer, and particularly when they are channelized, a shift in basal food sources can occur whereby primary consumers such as shredders are replaced by scrapers. The energy supply of food webs in these kinds of urban streams is extensively supported by autochthonous sources (e.g., by algal growth) contrasting with the forested streams supplied by allochthonous organic matter (Yule et al., 2010, 2015). Conversely, our urban streams showed a canopy cover similar to forest-pasture streams, but riparian vegetation was dominated by invasive eucalypt trees (E. globulus), which in turn suggests that these urban streams are not poorly shaded but are of poor-quality litter canopy instead. From a shredders’ perspective, leaves of E. globulus are poor-quality (tough and high in tannins) and low-quantity litter which even affect the shredder fitness (Canhoto and Graça, 1999; Correa-Araneda et al., 2015), and along eucalypt-canopied streams their role on OMB can be diminished due to lower colonization (Ferreira et al., 2015). Therefore, depending on identity/quality of litter and the decomposer community, invasive trees may not provide leaf litter that similarly supports the functioning of stream ecosystems as do native trees (Kuglerová et al., 2017). It is also possible that there is lower retention of organic matter due to flashy flows and lack of cobbles and boulders to trap leaf litter. Further research should consider all these aspects related to riparian vegetation loss to have more evidence of the lack of shredders at these urban sites.
Decades of research were needed to understand the leading role of biodiversity in the dynamics and functioning of ecosystems, and to quantify the positive effect of species diversity on ecosystem processes (Tilman et al., 2014). Macroinvertebrates play important roles in the functioning of stream ecosystems, among others, as shredder invertebrates contribute to the C cycle by feeding on CPOM (Ramírez and Gutiérrez-Fonseca, 2014). However, anthropogenic environmental stress has negatively affected the taxonomic and functional diversity of macroinvertebrates, reflecting negatively on the ecosystem functioning of temperate streams (Voß and Schäfer, 2017). In our study on Andean stream ecosystems, we observed that the urbanization gradient notably reduced both taxonomic and functional diversity of shredders which also influenced OMB rates mediated by this FFG. Indeed, at the most urbanized sites, we did not find shredders indicating a null contribution of macroinvertebrates on organic matter processing in these urban streams in the Andes.
The importance of shredders in stream ecosystems is also given as they are key organisms for energy transfer at the low levels of food webs (Ramírez and Gutiérrez-Fonseca, 2014; Merritt et al., 2017). In montane streams the relevance of shredders can be even greater due to leaf litter forming the major energy source (Wipfli et al., 2007). When shredders reduce the size of CPOM, they allow other organisms to have nutrients downstream as well as for the accomplishment of other ecosystem processes (Woodward, 2009; Vaughn, 2010; Benfield et al., 2017). Our findings suggest that there could be an alteration in the transfer of organic C in urban Andean streams. Nevertheless, more evidence is needed to understand the effect of shedder loss on organic C fluxes in these tropical mountain stream ecosystems.
We demonstrate that urbanization affected the B-EF relationships in Andean streams via nutrient enrichment and substrate homogenization causing the loss of taxonomic and functional diversity in shredders and OMB decline. The loss of keystone species mostly results in a reduction of ecosystem functions (Cardinale et al., 2006; Vaughn, 2010). Also, species loss is associated with the loss of functional biological traits related to ecosystem functioning (Flynn et al., 2009). However, it remains to be understood how the biological traits of shredders important for OMB are affected by stressors associated with urbanization in Andean streams. Finally, by losing ecosystem functions, several ecosystem services are also compromised. It is crucial to implement better land-use practices in Andean catchments to guarantee environmental services at lower altitudes.
Data Availability Statement
The raw data supporting the conclusions of this article will be made available by the authors, without undue reservation.
Author Contributions
CI-A contributed to the conception and design of the study. VR-C and CI-A performed the fieldwork and laboratory analysis. WZ-S and VR-C organized the database. WZ-S, FG, and CI-A performed the statistical analysis and wrote the first draft of the manuscript. All authors contributed to manuscript revision, read, and approved the submitted version.
Funding
This work was funded by the Universidad Técnica Particular de Loja through a reinvestment program for research granted to the research team Laboratorio de Ecología Tropical y Servicios Ecosistémicos (EcoSs-Lab).
Conflict of Interest
The authors declare that the research was conducted in the absence of any commercial or financial relationships that could be construed as a potential conflict of interest.
Acknowledgments
We thank the Ministerio del Ambiente del Ecuador for granting access to the field sites and the necessary collecting permits (MAE-DNB-CM- 2015-0016). We also thank Nathaly Luna and Diego Palma for their field assistance. This manuscript has been released as a pre-print at bioRxiv (Zúñiga-Sarango et al., 2020). We also acknowledge the helpful comments by two reviewers to an early version of the manuscript and to thank Gregory Gedeon for text revision.
Supplementary Material
The Supplementary Material for this article can be found online at: https://www.frontiersin.org/articles/10.3389/fevo.2020.592404/full#supplementary-material
References
Allan, J. D. (2004). Landscapes and riverscapes: the influence of land use on stream ecosystems. Annu. Rev. Ecol. Evol. Syst. 35, 257–284. doi: 10.1146/annurev.ecolsys.35.120202.110122
Antrop, M. (2004). Landscape change and the urbanization process in Europe. Landsc. Urban Plan. 67, 9–26. doi: 10.1016/s0169-2046(03)00026-4
APHA (2017). Standard Methods for the Examination of Water and Wastewater, 23rd Edn, eds R. B. Baird, A. D. Eaton, and E. W. Rice (Washington DC: American Public Health Association).
Bärlocher, F. (2005). “Leaf mass loss estimated by litter bag technique,” in Methods to Study Litter Decomposition, eds M. A. S. Graça, F. Bärlocher, and M. O. Gessner (Dordrecht: Springer), 37–42. doi: 10.1007/1-4020-3466-0_6
Benfield, E. F., Fritz, K. M., and Tiegs, S. D. (2017). “Decomposition of leaf material,” in Methods in Stream Ecology: Ecosystem Function, Vol. 2, eds F. R. Hauer and G. A. Lamberti (London: Academic Press), 71–82.
Benítez, Á., Medina, J., Vásquez, C., Loaiza, T., Luzuriaga, Y., and Calva, J. (2019). Lichens and bromeliads as bioindicators of heavy metal deposition in Ecuador. Diversity 11:28. doi: 10.3390/d11020028
Boyero, L., Pearson, R. G., Dudgeon, D., Graça, M. A. S., Gessner, M. O., Albariño, R. J., et al. (2011). Global distribution of a key trophic guild contrasts with common latitudinal diversity patterns. Ecology 92, 1839–1848. doi: 10.1890/10-2244.1
Brooks, M. E., Kristensen, K., van Benthem, K. J., Magnusson, A., Berg, C. W., Nielsen, A., et al. (2017). glmmTMB balances speed and flexibility among packages for zero-inflated generalized linear mixed modeling. R J. 9, 378–400. doi: 10.32614/rj-2017-066
Canhoto, C., and Graça, M. A. S. (1999). Leaf barriers to fungal colonization and shredders (Tipula lateralis) consumption of decomposing Eucalyptus globulus. Microb. Ecol. 37, 163–172. doi: 10.1007/s002489900140
Cardinale, B. J., Srivastava, D. S., Duffy, J. E., Wright, J. P., Downing, A. L., Sankaran, M., et al. (2006). Effects of biodiversity on the functioning of trophic groups and ecosystems. Nature 443, 989–992. doi: 10.1038/nature05202
Chadwick, M. A., Dobberfuhl, D. R., Benke, A. C., Huryn, A. D., Suberkropp, K., and Thiele, J. E. (2006). Urbanization affects stream ecosystem function by altering hydrology, chemistry, and biotic richness. Ecol. Appl. 16, 1796–1807. doi: 10.1890/1051-0761(2006)016[1796:uasefb]2.0.co;2
Chuquimarca, L., Gaona, F. P., Iñiguez-Armijos, C., Benítez, Á., Chuquimarca, L., Gaona, F. P., et al. (2019). Lichen responses to disturbance: clues for biomonitoring land-use effects on riparian Andean ecosystems. Divers 11:73. doi: 10.3390/D11050073
Clapcott, J. E., Collier, K. J., Death, R. G., Goodwin, E. O., Harding, J. S., Kelly, D., et al. (2012). Quantifying relationships between land-use gradients and structural and functional indicators of stream ecological integrity. Freshw. Biol. 57, 74–90. doi: 10.1111/j.1365-2427.2011.02696.x
Collen, B., Whitton, F., Dyer, E. E., Baillie, J. E. M., Cumberlidge, N., Darwall, W. R. T., et al. (2014). Global patterns of freshwater species diversity, threat and endemism. Glob. Ecol. Biogeogr. 23, 40–51. doi: 10.1111/geb.12096
Correa-Araneda, F., Boyero, L., Figueroa, R., Sánchez, C., Abdala, R., Ruiz-García, A., et al. (2015). Joint effects of climate warming and exotic litter (Eucalyptus globulus Labill.) on stream detritivore fitness and litter breakdown. Aquat. Sci. 77, 197–205. doi: 10.1007/s00027-014-0379-y
Couceiro, S. R. M., Hamada, N., Forsberg, B. R., and Padovesi-Fonseca, C. (2010). Effects of anthropogenic silt on aquatic macroinvertebrates and abiotic variables in streams in the Brazilian Amazon. J. Soils Sediments 10, 89–103. doi: 10.1007/s11368-009-0148-z
Couceiro, S. R. M., Hamada, N., Luz, S. L. B., Forsberg, B. R., and Pimentel, T. P. (2006). Deforestation and sewage effects on aquatic macroinvertebrates in urban streams in Manaus, Amazonas, Brazil. Hydrobiologia 575, 271–284. doi: 10.1007/s10750-006-0373-z
Croux, C., Filzmoser, P., and Fritz, H. (2013). Robust sparse principal component analysis. Technometrics 55, 202–214. doi: 10.1080/00401706.2012.727746
Dangles, O., Crespo-Pérez, V., Andino, P., Espinosa, R., Calvez, R., and Jacobsen, D. (2011). Predicting richness effects on ecosystem function in natural communities: insights from high elevation streams. Ecology 92, 733–743. doi: 10.1890/10-0329.1
de Jesús-Crespo, R., and Ramírez, A. (2011). Effects of urbanization on stream physicochemistry and macroinvertebrate assemblages in a tropical urban watershed in Puerto Rico. J. North Am. Benthol. Soc. 30, 739–750. doi: 10.1899/10-081.1
Dominguez, E., and Fernández, H. R. (2009). Macroinvertebrados Bentónicos Sudamericanos. Sistemática y Biología. Tucumán: Fundación Miguel Lillo.
Donoso, J. M., and Rios-Touma, B. (2020). Microplastics in tropical Andean rivers: a perspective from a highly populated Ecuadorian basin without wastewater treatment. Heliyon 6:e04302. doi: 10.1016/j.heliyon.2020.e04302
Encalada, A. C., Calles, J., Ferreira, V., Canhoto, C. M., and Graça, M. A. S. (2010). Riparian land use and the relationship between the benthos and litter decomposition in tropical montane streams. Freshw. Biol. 55, 1719–1733. doi: 10.1111/j.1365-2427.2010.02406.x
Feld, C. K., Segurado, P., and Gutiérrez-Cánovas, C. (2016). Analysing the impact of multiple stressors in aquatic biomonitoring data: a “cookbook” with applications in R. Sci. Total Environ. 573, 1320–1339. doi: 10.1016/j.scitotenv.2016.06.243
Ferreira, V., Larrañaga, A., Gulis, V., Basaguren, A., Elosegi, A., Graça, M. A. S., et al. (2015). The effects of eucalypt plantations on plant litter decomposition and macroinvertebrate communities in Iberian streams. For. Ecol. Manage. 335, 129–138. doi: 10.1016/j.foreco.2014.09.013
Flynn, D. F. B., Gogol-Prokurat, M., Nogeire, T., Molinari, N., Richers, B. T., Lin, B. B., et al. (2009). Loss of functional diversity under land use intensification across multiple taxa. Ecol. Lett. 12, 22–33. doi: 10.1111/j.1461-0248.2008.01255.x
Gessner, M. O., Chauvet, E., and Dobson, M. (1999). A perspective on leaf litter breakdown in streams. Oikos 85, 377–384. doi: 10.2307/3546505
Guerrero-Latorre, L., Romero, B., Bonifaz, E., Timoneda, N., Rusiñol, M., Girones, R., et al. (2018). Quito’s virome: metagenomic analysis of viral diversity in urban streams of Ecuador’s capital city. Sci. Total Environ. 645, 1334–1343. doi: 10.1016/j.scitotenv.2018.07.213
Guida-Johnson, B., Faggi, A. M., and Zuleta, G. A. (2017). Effects of urban sprawl on riparian vegetation: is compact or dispersed urbanization better for biodiversity? River Res. Appl. 33, 959–969. doi: 10.1002/rra.3138
Hagen, E. M., McTammany, M. E., Webster, J. R., and Benfield, E. F. (2010). Shifts in allochthonous input and autochthonous production in streams along an agricultural land-use gradient. Hydrobiologia 655, 61–77. doi: 10.1007/s10750-010-0404-7
Harding, J., Clapcott, J., Quinn, J., Hayes, J., Joy, M., Storey, R., et al. (2009). Stream Habitat Assessment Protocols for Wadeable Rivers and Streams of New Zealand. Christchurch: Canterbury University Press.
Hassett, B. A., Sudduth, E. B., Somers, K. A., Urban, D. L., Violin, C. R., Wang, S.-Y., et al. (2018). Pulling apart the urbanization axis: patterns of physiochemical degradation and biological response across stream ecosystems. Freshw. Sci. 37, 653–672. doi: 10.1086/699387
Iñiguez-Armijos, C., Hampel, H., and Breuer, L. (2018a). Land-use effects on structural and functional composition of benthic and leaf-associated macroinvertebrates in four Andean streams. Aquat. Ecol. 52, 77–92. doi: 10.1007/s10452-017-9646-z
Iñiguez-Armijos, C., Luzuriaga, L. R., and Marín, D. (2018b). New records and functional role of Anchytarsus palpalis champion, 1897 (Coleoptera: Ptilodactylidae) in Ecuador. Coleopt. Bull. 72, 157–160. doi: 10.1649/0010-065X-72.1.157
Iñiguez-Armijos, C., Leiva, A., Frede, H.-G., Hampel, H., and Breuer, L. (2014). Deforestation and benthic indicators: how much vegetation cover is needed to sustain healthy Andean streams? PLoS One 9:e105869. doi: 10.1371/journal.pone.0105869
Iñiguez-Armijos, C., Rausche, S., Cueva, A., Sánchez-Rodríguez, A., Espinosa, C., and Breuer, L. (2016). Shifts in leaf litter breakdown along a forest-pasture-urban gradient in Andean streams. Ecol. Evol. 6, 4849–4865. doi: 10.1002/ece3.2257
Jonsson, M., Malmqvist, B., and Hoffsten, P.-O. (2001). Leaf litter breakdown rates in boreal streams: does shredder species richness matter? Freshw. Biol. 46, 161–171. doi: 10.1046/j.1365-2427.2001.00655.x
Kuglerová, L., García, L., Pardo, I., Mottiar, Y., and Richardson, J. S. (2017). Does leaf litter from invasive plants contribute the same support of a stream ecosystem function as native vegetation? Ecosphere 8:e01779. doi: 10.1002/ecs2.1779
Laliberté, E., and Legendre, P. (2010). A distance-based framework for measuring functional diversity from multiple traits. Ecology 91, 299–305. doi: 10.1890/08-2244.1
Laliberté, E., Legendre, P., and Shipley, B. (2014). FD: Measuring Functional Diversity from Multiple Traits, and other Tools for Functional Ecology. R package version 1.0-12.
Lange, K., Townsend, C. R., and Matthaei, C. D. (2014). Can biological traits of stream invertebrates help disentangle the effects of multiple stressors in an agricultural catchment? Freshw. Biol. 59, 2431–2446. doi: 10.1111/fwb.12437
Li, A. O. Y., and Dudgeon, D. (2009). Shredders: species richness, abundance, and role in litter breakdown in tropical Hong Kong streams. J. North Am. Benthol. Soc. 28, 167–180. doi: 10.1899/08-043.1
Loreau, M., Naeem, S., Inchausti, P., Bengtsson, J., Grime, J. P., Hector, A., et al. (2001). Biodiversity and ecosystem functioning: current knowledge and future challenges. Science 294, 804–808. doi: 10.1126/science.1064088
Martins, R. T., Melo, A. S., Gonçalves, J. F., and Hamada, N. (2015). Leaf-litter breakdown in urban streams of Central Amazonia: direct and indirect effects of physical, chemical, and biological factors. Freshw. Sci. 34, 716–726. doi: 10.1086/681086
McKie, B. G., Schindler, M., Gessner, M. O., and Malmqvist, B. (2009). Placing biodiversity and ecosystem functioning in context: environmental perturbations and the effects of species richness in a stream field experiment. Oecologia 160, 757–770. doi: 10.1007/s00442-009-1336-7
Merritt, R. W., Cummins, K. W., and Berg, M. P. (2017). “Trophic relationships of macroinvertebrates,” in Methods in Stream Ecology, Volume 1: Ecosystem Structure, eds F. R. Hauer and G. A. Lamberti (London: Academic Press), 413–433. doi: 10.1016/b978-0-12-416558-8.00020-2
Meyer, J. L., Paul, M. J., and Taulbee, W. K. (2005). Stream ecosystem function in urbanizing landscapes. J. North Am. Benthol. Soc. 24, 602–612. doi: 10.1899/04-021.1
Miserendino, M. L., and Pizzolon, L. A. (2004). Interactive effects of basin features and land-use change on macroinvertebrate communities of headwater streams in the Patagonian Andes. River Res. Appl. 20, 967–983. doi: 10.1002/rra.798
Montalvo-Cedillo, C., Jerves-Cobo, R., and Domínguez-Granda, L. (2020). Determination of pollution loads in spillways of the combined sewage network of the city of Cuenca, Ecuador. Water 12:2540. doi: 10.3390/w12092540
Naimi, B. (2015). Usdm: Uncertainty analysis for Species Distribution Models. R package version 1.1-18.
Ochoa-Cueva, P., Fries, A., Montesinos, P., Rodríguez-Díaz, J. A., and Boll, J. (2015). Spatial estimation of soil erosion risk by land-cover change in the Andes of southern Ecuador. Land Degrad. Dev. 26, 565–573. doi: 10.1002/ldr.2219
Paul, M. J., and Meyer, J. L. (2001). Streams in the urban landscape. Annu. Rev. Ecol. Syst. 32, 333–365. doi: 10.1146/annurev.ecolsys.32.081501.114040
R Development Core Team (2020). R: A Language and Environment for Statistical Computing. Vienna: R Foundation for Statistical Computing.
Ramírez, A., and Gutiérrez-Fonseca, P. E. (2014). Functional feeding groups of aquatic insect families in Latin America: a critical analysis and review of existing literature. Rev. Biol. Trop. 62, 155–167. doi: 10.15517/rbt.v62i0.15785
Richardson, J. S. (1992). Food, microhabitat, or both? Macroinvertebrate use of leaf accumulations in a montane stream. Freshw. Biol. 27, 169–176. doi: 10.1111/j.1365-2427.1992.tb00531.x
Riipinen, M. P., Davy-Bowker, J., and Dobson, M. (2009). Comparison of structural and functional stream assessment methods to detect changes in riparian vegetation and water pH. Freshw. Biol. 54, 2127–2138. doi: 10.1111/j.1365-2427.2008.01964.x
Roy, A. H., Rosemond, A. D., Paul, M. J., Leigh, D. S., and Wallace, J. B. (2003). Stream macroinvertebrate response to catchment urbanization (Georgia, USA). Freshw. Biol. 48, 329–346. doi: 10.1046/j.1365-2427.2003.00979.x
Sánchez-Bayo, F., and Wyckhuys, K. A. G. (2019). Worldwide decline of the entomofauna: a review of its drivers. Biol. Conserv. 232, 8–27. doi: 10.1016/J.BIOCON.2019.01.020
Sandin, L., and Solimini, A. (2009). Freshwater ecosystem structure-function relationships: from theory to application. Freshw. Biol. 54, 2017–2024. doi: 10.1111/j.1365-2427.2009.02313.x
Tapia-Armijos, M. F., Homeier, J., Espinosa, C. I., Leuschner, C., and de la Cruz, M. (2015). Deforestation and forest fragmentation in South Ecuador since the 1970s – Losing a hotspot of biodiversity. PLoS One 10:e0133701. doi: 10.1371/journal.pone.0133701
Tiegs, S. D., Akinwole, P. O., and Gessner, M. O. (2009). Litter decomposition across multiple spatial scales in stream networks. Oecologia 161, 343–351. doi: 10.1007/s00442-009-1386-x
Tiegs, S. D., Costello, D. M., Isken, M. W., Woodward, G., Mcintyre, P. B., Gessner, M. O., et al. (2019). Global patterns and drivers of ecosystem functioning in rivers and riparian zones. Sci. Adv. 5:eaav0486. doi: 10.1126/sciadv.aav0486
Tilman, D., Isbell, F., and Cowles, J. M. (2014). Biodiversity and ecosystem functioning. Annu. Rev. Ecol. Evol. Syst. 45, 471–493.
Urban, M. C., Skelly, D. K., Burchsted, D., Price, W., and Lowry, S. (2006). Stream communities across a rural-urban landscape gradient. Divers. Distrib. 12, 337–350. doi: 10.1111/j.1366-9516.2005.00226.x
Vásquez, C., Calva, J., Morocho, R., Donoso, D. A., and Benítez, Á. (2019). Bryophyte communities along a tropical urban river respond to heavy metal and arsenic pollution. Water 11:813. doi: 10.3390/w11040813
Vaughn, C. C. (2010). Biodiversity losses and ecosystem function in freshwaters: emerging conclusions and research directions. Bioscience 60, 25–35. doi: 10.1525/bio.2010.60.1.7
Villéger, S., Mason, N. W. H., and Mouillot, D. (2008). New multidimensional functional diversity indices for a multifaceted framework in functional ecology. Ecology 89, 2290–2301. doi: 10.1890/07-1206.1
Vimos-Lojano, D., Hampel, H., Vázquez, R. F., and Martínez-Capel, F. (2020). Community structure and functional feeding groups of macroinvertebrates in pristine Andean streams under different vegetation cover. Ecohydrol. Hydrobiol. 20, 357–368. doi: 10.1016/j.ecohyd.2020.04.004
Voß, K., Fernández, D., and Schäfer, R. B. (2015). Organic matter breakdown in streams in a region of contrasting anthropogenic land use. Sci. Total Environ. 527–528, 179–184. doi: 10.1016/j.scitotenv.2015.04.071
Voß, K., and Schäfer, R. B. (2017). Taxonomic and functional diversity of stream invertebrates along an environmental stress gradient. Ecol. Indic. 81, 235–242. doi: 10.1016/j.ecolind.2017.05.072
Wallace, J. B., and Webster, J. R. (1996). The role of macroinvertebrates in stream ecosystem function. Annu. Rev. Entomol. 41, 115–139. doi: 10.1146/annurev.en.41.010196.000555
Walsh, C. J., Roy, A. H., Feminella, J. W., Cottingham, P. D., Groffman, P. M., and Morgan, R. P. (2005). The urban stream syndrome: current knowledge and the search for a cure. J. North Am. Benthol. Soc. 24, 706–723. doi: 10.1899/04-028.1
Wipfli, M. S., Richardson, J. S., and Naiman, R. J. (2007). Ecological linkages between headwaters and downstream ecosystems: transport of organic matter, invertebrates, and wood down headwater channels. J. Am. Water Resour. Assoc. 43, 72–85. doi: 10.1111/j.1752-1688.2007.00007.x
Wood, S. N. (2017). Generalized Additive Models: An Introduction with R, 2nd Edn. London: Chapman and Hall.
Woodward, G. (2009). Biodiversity, ecosystem functioning and food webs in fresh waters: assembling the jigsaw puzzle. Freshw. Biol. 54, 2171–2187. doi: 10.1111/j.1365-2427.2008.02081.x
Woodward, G., Gessner, M. O., Giller, P. S., Gulis, V., Hladyz, S., Lecerf, A., et al. (2012). Continental-scale effects of nutrient pollution on stream ecosystem functioning. Science 336, 1438–1440. doi: 10.1126/science.1219534
Yule, C. M., Boyero, L., and Marchant, R. (2010). Effects of sediment pollution on food webs in a tropical river (Borneo, Indonesia). Mar. Freshw. Res. 61, 204–213. doi: 10.1071/MF09065
Yule, C. M., Gan, J. Y., Jinggut, T., and Lee, K. V. (2015). Urbanization affects food webs and leaf-litter decomposition in a tropical stream in Malaysia. Freshw. Sci. 34, 702–715. doi: 10.1086/681252
Zou, H., Hastie, T., and Tibshirani, R. (2006). Sparse principal component analysis. J. Comput. Graph. Stat. 15, 265–286. doi: 10.1198/106186006X113430
Zúñiga-Sarango, W., Gaaona, F. P., Reyes-Castillo, V., and Iñiguez-Armijos, C. (2020). Disrupting the biodiversity – ecosystem function relationship: response of shredders and leaf breaakdown to urbanization in Andean streams. bioRxiv doi: 10.1101/2020.07.28.223867
Keywords: shredders diversity, leaf breakdown, tropical Andes, urbanized streams, biodiversity-ecosystem function relationship
Citation: Zúñiga-Sarango W, Gaona FP, Reyes-Castillo V and Iñiguez-Armijos C (2020) Disrupting the Biodiversity–Ecosystem Function Relationship: Response of Shredders and Leaf Breakdown to Urbanization in Andean Streams. Front. Ecol. Evol. 8:592404. doi: 10.3389/fevo.2020.592404
Received: 07 August 2020; Accepted: 14 October 2020;
Published: 28 October 2020.
Edited by:
Mingbo Yin, Fudan University, ChinaReviewed by:
Yonghong Bi, Chinese Academy of Sciences, ChinaCatherine Mary Yule, University of the Sunshine Coast, Australia
Copyright © 2020 Zúñiga-Sarango, Gaona, Reyes-Castillo and Iñiguez-Armijos. This is an open-access article distributed under the terms of the Creative Commons Attribution License (CC BY). The use, distribution or reproduction in other forums is permitted, provided the original author(s) and the copyright owner(s) are credited and that the original publication in this journal is cited, in accordance with accepted academic practice. No use, distribution or reproduction is permitted which does not comply with these terms.
*Correspondence: Carlos Iñiguez-Armijos, Y2FpbmlndWV6QHV0cGwuZWR1LmVj; Y2FpbmlndWV6YUBnbWFpbC5jb20=