- 1Geography and Environment, Loughborough University, Loughborough, United Kingdom
- 2Joseph Banks Laboratories, School of Life Sciences, University of Lincoln, Lincoln, United Kingdom
Plant competition belowground is a crucial factor that determines plant fitness and shapes plant communities. It has been shown that roots possess the ability to recognize between self and non-self individuals and modify their growth patterns accordingly. Roots may also be further able to discriminate between kin and non-kin roots. Competition is stronger with non-kin than kin neighbors, but whether root growth patterns determined by kin interactions are modified in stressful environments is largely unexplored. In this study we used two different experimental set-ups to confirm whether the clonal species Glechoma hederacea exhibits kin recognition between roots, and how water limitation modifies this response. A split-root design was conducted using a focal clonal fragment of G. hederacea placed onto the ridge that separated two adjacent containers: either square petri-dishes boxes with 200 ml of soil or 400 ml clear pots. Focal plants were randomly allocated to either growing alone or in competition with another G. hederacea ramet (of similar size taken from the same stolon as the focal plant) or a non-kin individual (a ramet selected from a different population). A water limitation treatment was applied to both experiments. Five root parameters were measured during the experiment using boxes: the maximum depth and the maximum width of the deepest root, the depth and the maximum width of the widest root, and the total number of roots visible. All plants were harvested after 36 days and several final root measurements were taken and analyzed using GiaRoots. Our results show that G. hederacea roots have kin recognition mechanisms, showing a significant tendency to avoid growing toward a non-kin neighbor but not avoiding the roots of a kin plant, even though the presence of a kin plant modifies root distribution within the soil. As expected, water limitation significantly affected plant growth and root parameters, but this effect was not related to neighbor kin identity. Taken together, our results confirm the existence of root recognition in this species and suggest that the mechanisms of kin recognition are probably related to a root-derived chemical cues, as recognition took place before physical contact occurred.
Introduction
Plant fitness is strongly determined by competition for resource with neighboring plants (Harper, 1977) and is one of the most important factors shaping plant communities (Callaway and Walker, 1997). From germination, plants must compete for limited space and resources and therefore, identity recognition, i.e., the ability to recognize between “self” and “non-self,” is very important in order to maximize growth and survival. For plants, self-recognition has been documented in structures involved in resource acquisition, such as roots (Mahall and Callaway, 1991; Chen et al., 2012). Studies on root competition have shown that plants that are grown together instead of in isolation put more resources into root growth, while sacrificing resources for reproduction (Gersani et al., 2001); this is known as “tragedy of the commons” (after Hardin, 1968) and suggest that plants have mechanisms for recognizing neighbors as non-self (here defined as any neighbor, regardless of genetic similarity), and modify their growth patterns accordingly (Semchenko et al., 2007).
Roots create nutrient depletion zones around them, so if different roots from the same individual compete with each other for the same limited nutrients, the overall efficiency of resource acquisition decreases. In this scenario, root segregation would be advantageous to maximize resource acquisition and decrease within-individual competition (Mahall and Callaway, 1996; Schenk, 2006). However, if roots were from a competitor plant, an advantageous strategy would be to place roots in the same space and compete for the limited resources, potentially allowing for increased nutrient uptake whilst also denying its competitors resources (Zhang et al., 1999; Gersani et al., 2001).
Studies have further identified that plants might be also able to recognize kin from non-kin roots and modify their resource allocation accordingly (reviewed in Chen et al., 2012). This mechanism is probably a consequence of their inherently limited of propagule dispersal, as new plants tend to establish and grow in close proximity to other genetically related plants (Vekemans and Hardy, 2004). Where there is strong spatial structuring of kin, the fitness of an individual plant is determined not only by its own fitness, but also by that of related neighbors i.e., inclusive fitness (Hamilton, 1964), so selection may act strongly to reduce local competition amongst close relatives (Chen et al., 2012; Dudley et al., 2013). Indeed, empirical data indicate that plants tend to allocate more resources into root growth in order to acquire more nutrients in non-kin interactions compared to kin-kin interactions (Biedrzycki and Bais, 2010; Biedrzycki et al., 2010), and that plants compete more aggressively with non-kin than kin neighbors (Dudley and File, 2007; Murphy and Dudley, 2009). Conversely, fitness may be higher in non-kin groupings because of ecological niche partitioning (Barton and Post, 1986). Here, the theory predicts that relatives overlap more in their niche use and therefore compete more with each other compared with unrelated conspecifics (Young, 1981; Milla et al., 2009). Kin selection and ecological niche partitioning provide alternative outcomes for competition between kin/non-kin. Importantly, differential access to resources may be one potential factor determining the adoption of differing tactics (Novoplansky, 2009).
Regardless of the level of recognition (self, kin, non-kin), the possible mechanisms for identifying the presence of neighbors and their relatedness are still not clearly understood and include direct contact with roots (Mahall and Callaway, 1992, 1996), physiological coordination of own roots (Falik et al., 2003), root exudates (e.g., Semchenko et al., 2014), volatile organic compounds (Bais, 2014), and interactions mediated by other soil components such as mycorrhizal fungi (e.g., File et al., 2012; Simard et al., 2015). Previous studies indicate that there is variation between species in addition to genetic variation in the regulatory mechanisms of recognition, and this is further affected by the local environment in which the plants grow (e.g., Dudley and File, 2007; Murphy and Dudley, 2009). For example, peas grown in N-rich substrate showed root segregation with neighbors, but not in low N substrates (Litav and Harper, 1967). In turn, kin selection has been found to be stronger in N-poor substrates (Pezzola et al., 2020). Plant root system must optimize for the uptake of several limiting resources and it is well-established that the intensity of belowground interactions varies with the availability of soil resources plants compete for (de Vries, 2013; Bisseling and Scheres, 2014), and thus, kin interactions may be modified in stressful or resource-limited environments (Litav and Harper, 1967).
For many plants, drought is an important abiotic stressor that can strongly modify belowground interactions (see Foxx and Fort, 2019; and references there). Under water deficit, root allocation increases, and rooting depth may also increase (Jackson et al., 2000) which will have the potential to influence self-neighbor root interactions. In this study two different experimental set-ups were used to investigate whether the clonal species Glechoma hederacea exhibits kin recognition at the root level, and whether water limitation modifies this response. Specifically, we hypothesized that: (i) plants will increase root growth in the presence of a non-kin neighbor; (ii) water limitation will increase root growth; and (iii) root growth will be greatest in the presence of non-kin when water is limited. Previous research has shown that this species possesses kin recognition and displays an avoidance pattern, avoiding contact with roots of neighboring ramets regardless of its genetic or specific identity (Semchenko et al., 2007). However, a split root design has not been undertaken to determine response to kin and non-kin by the same focal plant. Response patterns have also not been investigated with water limitation included as a stress factor before.
Materials and Methods
Study Species and Plant Origin
Glechoma hederacea (Lamiaceae), is a clonal perennial herb, typically found in fertile, damp, and shaded habitats within the United Kingdom (Hutchings and Price, 1999). It reproduces both through seed production and with the production of numerous monopodial stolons, with flowering taking place from March to June (Hutchings and Price, 1999). Vegetative growth is rapid, and the plant forms a relatively dense carpet in areas where other potential competitor species are limited by shade and disturbance (Grime et al., 1988).
Plants were sampled in March and July 2017 from two different populations naturally growing within the University of Lincoln Riseholme campus. The first population was located along a stream edge with trees growing alongside (53.267952°N, −0.532236°E), whilst the second was located between a small woodland and grassland (53.270544°N, −0.524995°E). Clonal fragments were selected to have at least three ramets with intact root systems and physically separated from other genets. Clonal fragments were excavated and used directly after collection.
Experimental Set Up
In order to identify root responses to kin and non-kin neighbors, two separate experiments were conducted, both using a split root design. One experiment (referred as Experiment 1: Boxes) used square petri-dish boxes (12 × 12 × 16 cm, L × W × H) that were Sellotaped together and each filled with 200 ml of soil medium comprised of autoclaved sand and perlite (4:1), with addition of 5 g/L of dolomite and 1.5 g/L bone meal (Figure 1A). Boxes were angled at 45 degrees to encourage roots to grow alongside the underside of the petri-dish, so the roots could be traced during growth. The other experiment (referred as Experiment 2: Cups) used 400 ml clear plastic cups; two were Sellotaped together and filled with the same soil mixture as before (Figure 1B). In both experiments’ boxes and cups (referred to as pots hereafter) were placed in foil bags to prevent light penetration from the side.
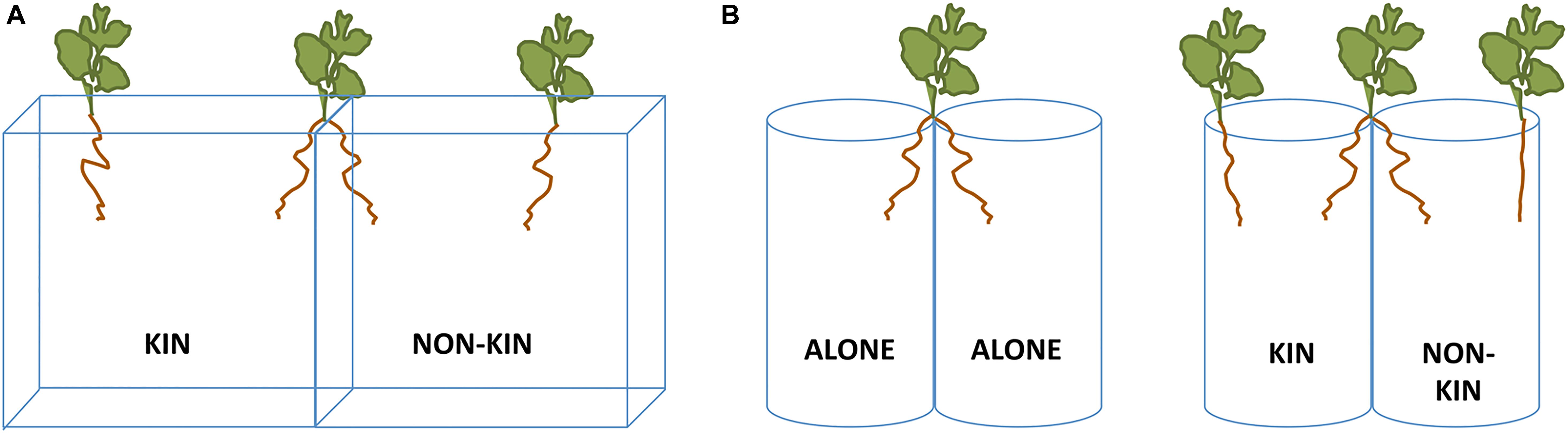
Figure 1. Schematic representation of the experimental treatments used in (A) Experiment 1 (Boxes) and (B) Experiment 2 (Cups).
Both experiments had a similar set up. A focal plant was placed onto the ridge that separated the two adjacent containers, positioning half of the root system onto each side (Figure 1). Roots were trimmed to ensure the two sides had a similar root starting length (3.5 ± 0.1 and 5.9 ± 0.2 cm, for Experiment 1 and 2, respectively). Focal plants were randomly allocated to either growing alone or in competition with another G. hederacea ramet of similar size (Figure 1). In the latter case, one side of the container comprised a kin individual (another ramet taken from the same stolon as the focal plant) or a non-kin individual (a ramet selected from a different population in order to increase genetic difference). All three clonal fragments per pot were matched for size as closely as possible and plants (including roots) were photographed for later initial analysis. Experiment 1 contained 14 replicates per treatment for a total of 56 boxes, and Experiment 2 had 20 replicates per treatment for a total of 80 cups.
Plants in Experiment 1 were grown under greenhouse conditions under natural light levels in July, whilst plants in Experiment 2 were grown in March at the greenhouse facilities at the University of Lincoln for 36 days, under 12:12 h day:night at ∼20°C. A water limitation treatment was used in both experiments to determine how this may modify kin recognition/competition. Control plants were watered daily with 5% of the pot volume (i.e., 20 or 10 ml for cups and boxes respectively). Water volume was determined through testing of the amount of water that fully saturated the pots. Plants in the water limitation treatment received the same amount of water but once every 4 days (evidenced by plant wilting and soil cracking). At 36 days roots were seen to start utilizing the bottom of the pots in both experiments so it was decided to terminate the experiments to avoid undesirable effects due to being pot bound.
Root Measurements and Analysis
In Experiment 1, the growth of the root system was investigated during the experiment. Boxes were visually inspected on day 17 (when roots became first visible against the walls), day 24, and day 35 (day before harvesting), and root growth was traced using different colored pens. Photographs were taken on each occasion and analyzed using ImageJ (2012, version Fiji, Schindelin et al., 2012). Five parameters were measured: the maximum depth and the maximum width of the deepest root (as measured away from the middle wall), the depth and the maximum width of the widest root, and the total number of roots visible (Figure 2).
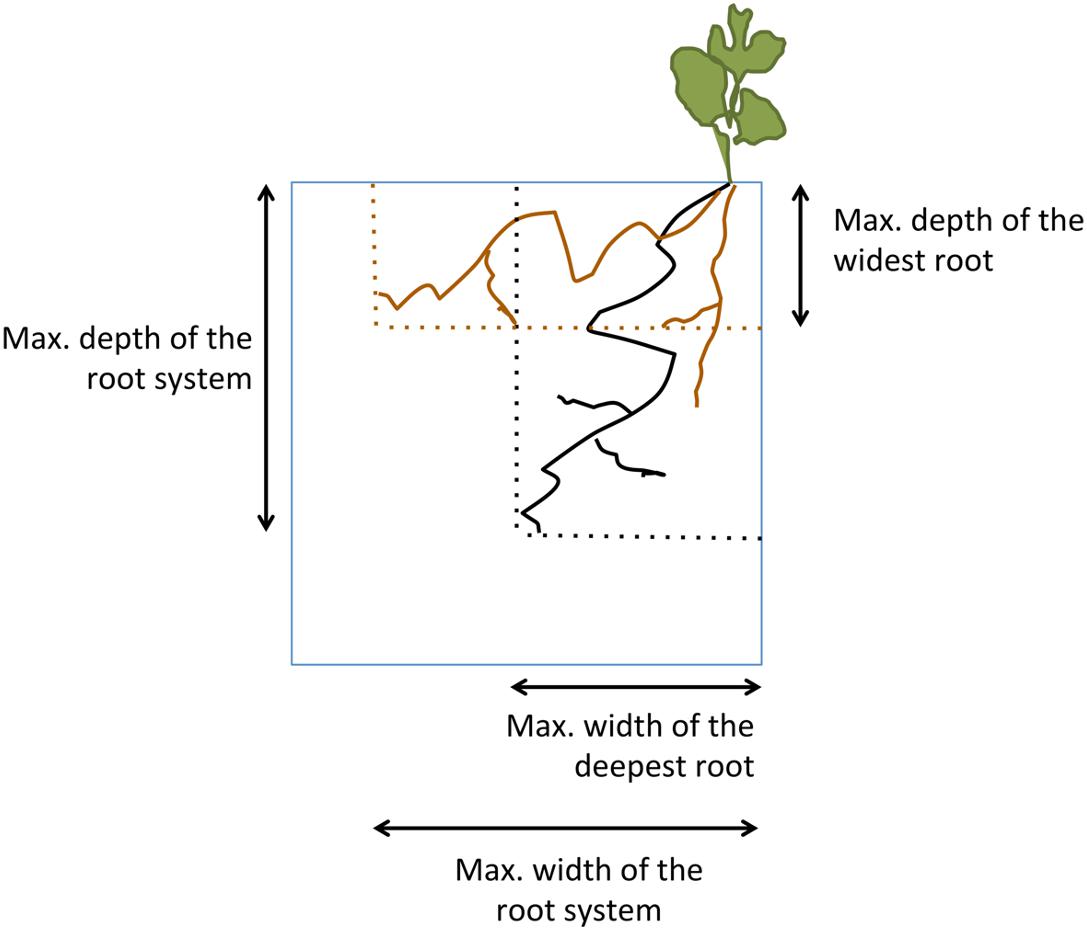
Figure 2. Schematic representation of the plant measurements taken. See section “Materials and Methods” for details.
At harvesting, plants were carefully removed from the containers and pictures of the root systems were again taken for analysis after cleaning the root systems under running water. All photographs were taken using a Nikon D70 and 18–70 mm zoom-Nikkor lens and root components were analyzed using GiaRoots (Galkovskyi et al., 2012). Starting root length was used along with the final root depth at harvest to calculate the increase in root length (cm). In addition, the final number of roots produced, and the root network distribution (i.e., fraction of the root length found in the lower 2/3 of the root network, as defined by the maximum depth of the root system) were calculated in both experiments. The number of connected components was calculated and used as quality control value. An image of a real root system should have a value of 1 connected component. If the root network has one break due to errors in image acquisition and processing, the value would be 2 (Galkovskyi et al., 2012). The number of connected components ranged from 1 to 9 (mean = 2.4) and 1 to 8 (mean = 1.6) for Experiment 1 and 2.
Some plants (eight and two, in Experiment 1 and 2, respectively) died during the experiment and thus these pots were excluded for the analyses. Mortality was not associated with any experimental factor considered (statistical results not shown). Dry weights (DW) were taken separately for aboveground tissues and roots after drying the samples in paper bags for three days at 70°C.
Statistical Analyses
All data were analyzed in R 3.6.2 (R Core Team, 2019) using the packages lmerTest (Kuznetsova et al., 2017) and car (Fox and Weisberg, 2019). Linear mixed-effects models (LMM) were used to investigate the effects of water limitation (control/water-limited), kin treatment (alone/kin/non-kin), and day (17, 24, 35, only in Experiment 1) on the measured root parameters. In Experiment 1, root identity was included as a random effect, nested within pot, whereas in experiment 2, pot was included as a random effect. To investigate differences in aboveground biomass acquisition, analysis compared plants grown alone vs. plants grown with a neighbor. In this case, a linear model was used. Generalized linear mixed effects models were used for count data (number of roots produced). Pots and roots nested within pots were included as random effects in all the models, and also all possible 2-way interactions between factors. All model residuals were checked to make sure the data met the assumptions of the models. Log-transformed data were used for width of the deepest root to ensure models residuals were normally distributed. Post-hoc pairwise tests were used where significant differences between treatments were found using the package emmeans (Lenth, 2020).
Results
Experiment 1: Boxes
Differences Between Dates
Whilst water limitation did not significantly affect any root parameters in G. hederacea clonal fragments growing in the boxes, significant differences between days were detected in all parameters, indicating that the root systems of the plants successfully established and grew within the boxes (Table 1 and Figure 3). After 17 days, the root system had reached a visual depth of 6.1 ± 0.3 cm regardless of whether plants were grown alone or with another individual. By 24 days, roots had grown, on average, 1.3 cm more, and at day 35, roots had grown another 1.5 cm more (Figure 3A). A similar pattern was observed for the width of the deepest root but in this case, significant differences between days were only observed at day 35 (Table 1 and Figure 3B), indicating the deepest root grew away toward the other side of the container. When analyzing the depth of this widest root, significant differences were again only detected at day 35 (Table 1 and Figure 3C).
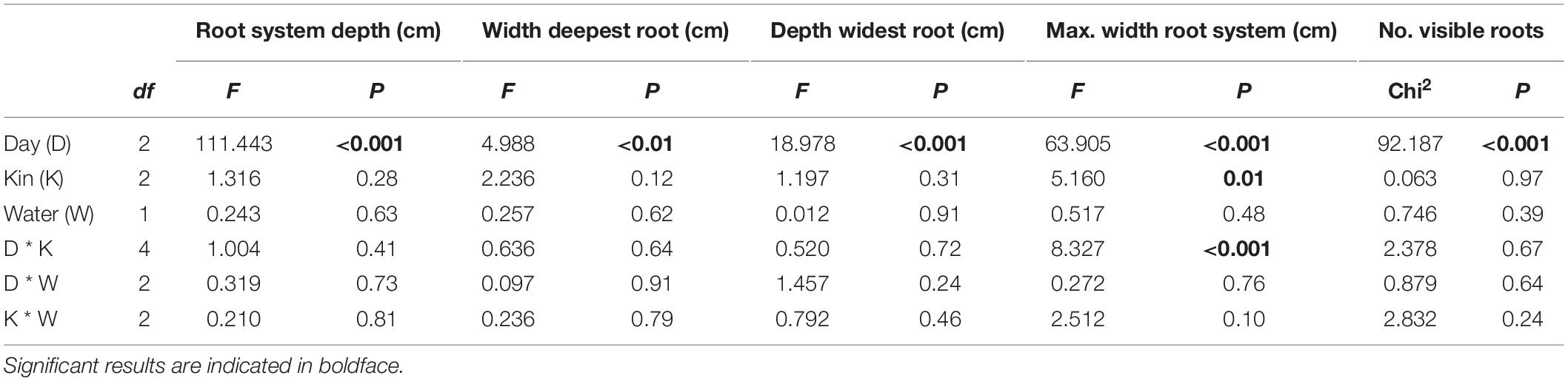
Table 1. ANOVA table of mixed-effects model testing the influence of day, water treatment, kin and their 2-way interactions on Glechoma hederacea root parameters measured in Experiment 1.
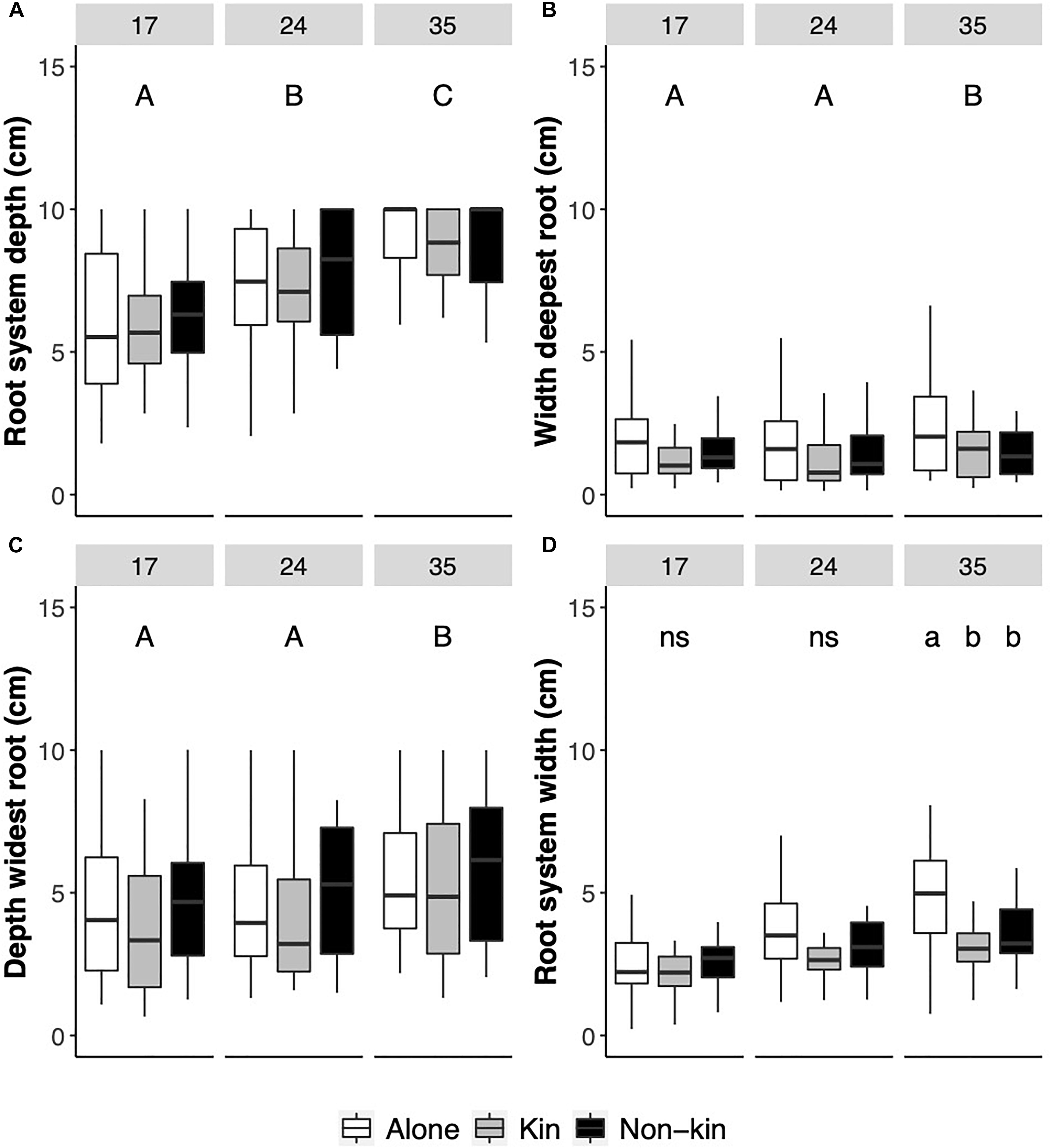
Figure 3. Boxplots of (A) maximum root system depth (cm), (B) Width of the deepest root, (C) Depth of the widest root, and (D) maximum width of the root system of Glechoma hederacea plants grown alone (white boxes) or with a kin (gray boxes) or a non-kin plant (black boxes) measured after 17, 24, and 35 days in Experiment 1. Letters above bars indicate significant post-hoc differences.
Overall, the maximum width of the visible root system showed a significant interaction between kin and days (Table 1 and Figure 3D). For this parameter, differences between kin treatments were only detected at day 35; roots from plants grown alone grew significantly further away toward the end of the box than when grown either with a kin or a non-kin (Figure 3D), suggesting that plants were recognizing the presence of another plant.
The number of visible roots was also significantly only affected by days (Table 1). On average, 3.2 ± 0.2 roots were visible in the boxes after 17 days, and this number increased to 5.5 ± 0.3 and to 6.3 ± 0.3 by 24 and 35 days.
Final Harvest
At the final harvest, neither kin, water limitation, or their interaction significantly affected any root parameter (Table 2). Final root DW ranged between 337.8 and 363.2 mg and was not statistically affected by any of the factors included in the model [F(2, 44.9) = 0.110, p = 0.89; F(1, 44) = 0.174, p = 0.67; and F(2, 44.9) = 1.144, p = 0.32; for the effect of kin, water limitation, and the interaction between factors]. Similarly, aboveground DW ranged between 341.9 and 379.1 mg and no differences due to water limitation or kin treatments were detected [F(1, 92) = 0.003, p = 0.96; F(1,92) = 0.276, p = 0.60; and F(1, 92) = 0.015, p = 0.90; for the effect of kin, water limitation, and the interaction between factors].
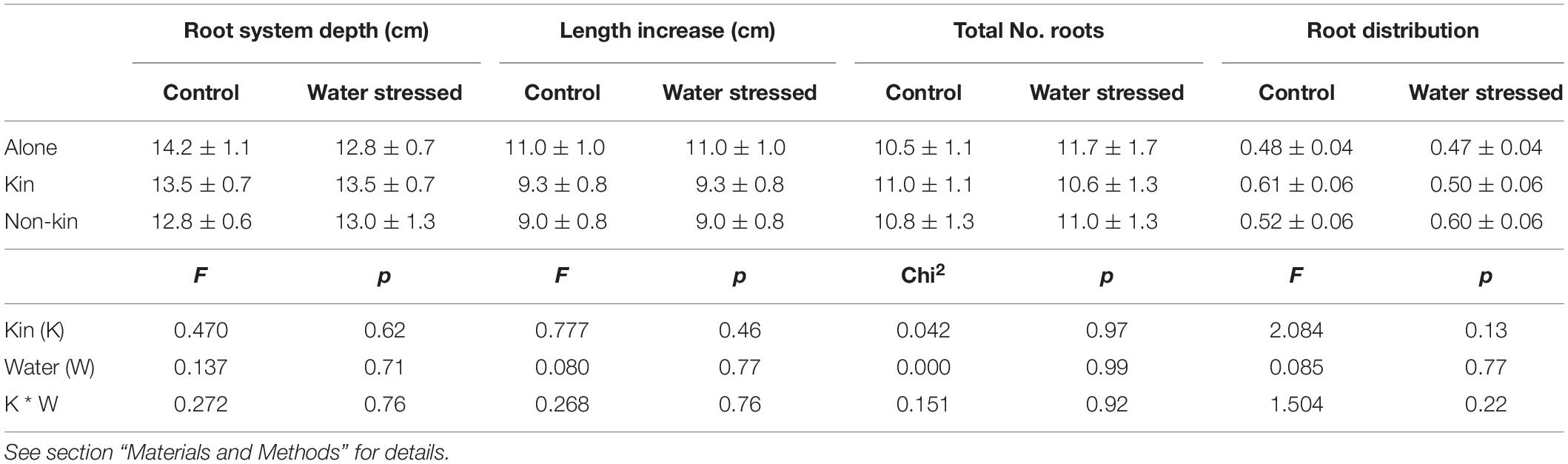
Table 2. Root parameters (means ± SE) measured at the final harvest in Experiment 1 and statistical outputs.
Experiment 2: Cups
In the second experiment, both kin and water limitation treatment affected some of the root parameters analyzed, even though not in an interactive effect manner (Table 3 and Figure 4). Plants grown under water limitation produced on average 3 cm deeper roots than control plants (Figure 4A), although when root length increase was analyzed, no statistically significant differences were detected (Table 3 and Figure 4B). Moreover, the total number of roots produced was also significantly affected by the water treatment as plants under water limitation produced on average four more roots than control plants (Figure 4C). For this parameter, the presence of another plant strongly influenced the total number of roots produced as plants growing with a kin produced on average five more roots than when grown alone, but almost nine more if they were grown with non-kin (Figure 4C). Similarly, kin and water limitation treatments significantly affected root distribution across the space as estimated from the network root distribution (Table 3 and Figure 4D). On average, control plants had a larger proportion of roots in the lower parts of the pot compared to water-limited plants, and the proportion of roots placed in the lower parts of the pots was similar between plants growing alone and with a kin plants, but significantly lower in non-kin pots (Figure 4D).

Table 3. Statistical outputs testing the influence of kin, water treatment, and their 2-way interactions on Glechoma hederaceae root parameters measured in Experiment 2.
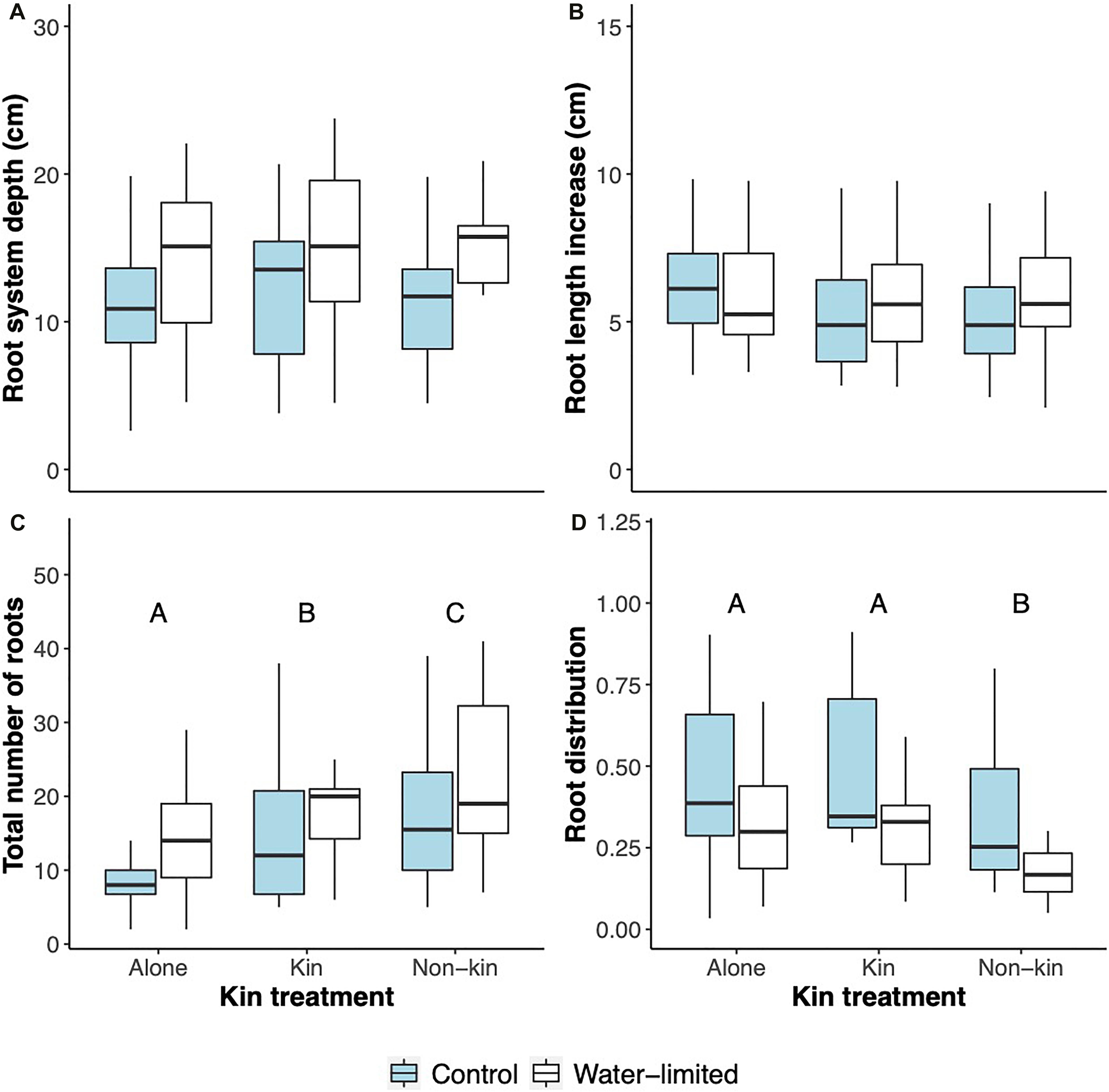
Figure 4. Boxplots of (A) maximum root system depth (cm), (B) root length increase, (C) total number of roots, and (D) proportion of roots in the lower 2/3 of boxes of Glechoma hederacea plants grown alone, with a kin or with a non-kin plant in control (blue boxes) and water-limited (white boxes) conditions in Experiment 2. Letters above bars indicate significant differences.
The differences in root parameters translated into significant differences in plant mass acquisition aboveground F(2, 74.9) = 0.309, p = 0.73; F(1, 74) = 8.839, p < 0.01; and F(2, 74.9) = 3.740, p = 0.02; for the effect of kin, water limitation, and the interaction between factors; Figure 5A) but not belowground [F(2, 74.9) = 0.583, p = 0.56; F(1, 74) = 1.967, p = 0.16; and F(2, 74.9) = 2.305, p = 0.10; for the effect of kin, water limitation, and the interaction between factors; Figure 5B]. Aboveground, plants growing alone accumulated a similar amount of biomass regardless of the watering treatment but plants growing together with another plant, accumulated more biomass under control conditions, regardless of the identity of the plant (Figure 5A).
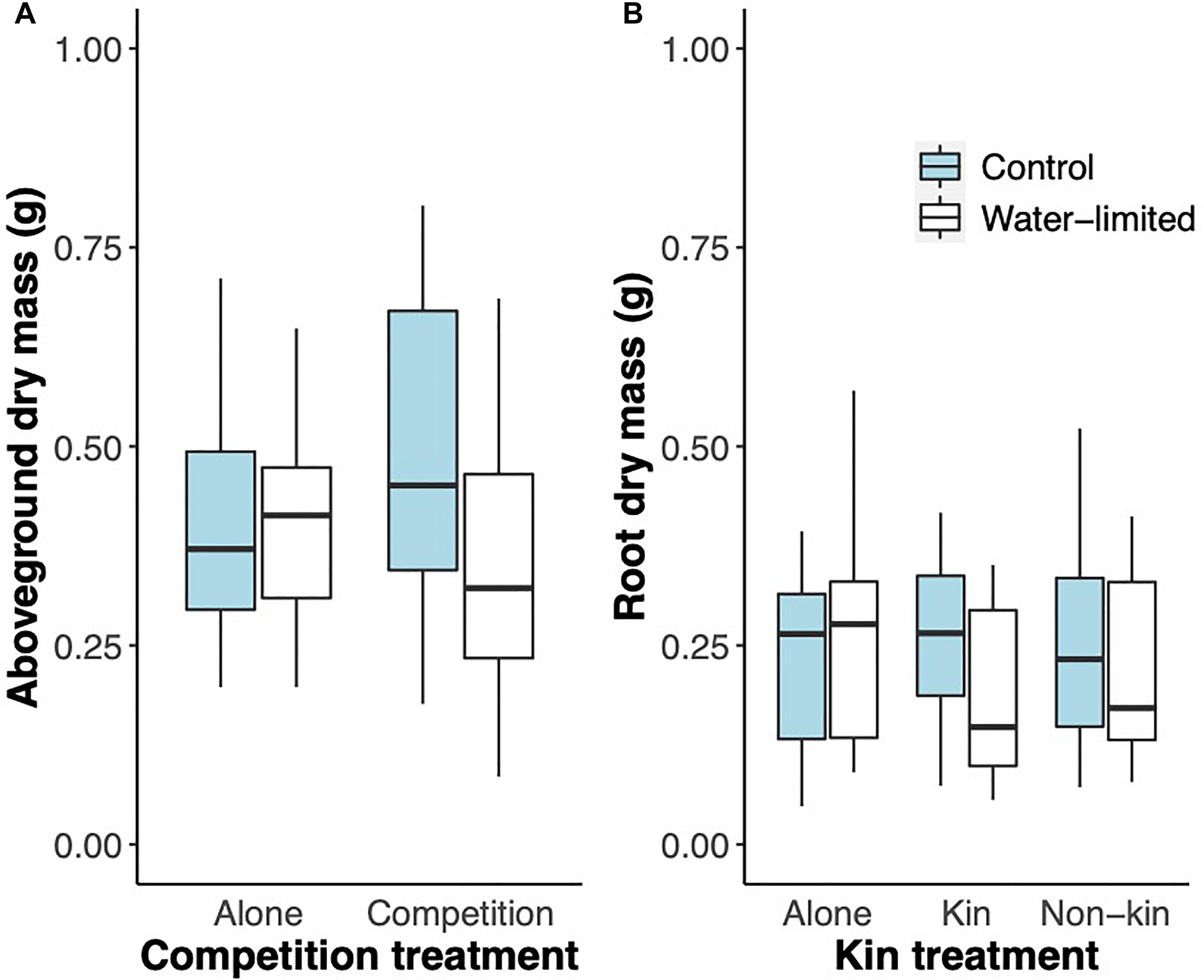
Figure 5. Boxplot of (A) aboveground dry mass and (B) root dry mass (g) accumulated for Glechoma hederacea plants grown for 36 days either alone, or in competition with a kin or a non-kin plant under control (blue boxes) and water-limited (white boxes) conditions in Experiment 2: Cups.
Discussion
Kin recognition and plasticity in roots of clonal herbs may be important traits due to their inherent life growth form. Currently, the majority of available studies have used a split-root experimental approach, growing plants under non-stressful conditions, and measuring root traits after harvesting the plants, demonstrating that plants tend to allocate more resources into root growth in non-kin compared to kin-kin interactions to enhance nutrient acquisition (e.g., Krannitz and Caldwell, 1995; Falik et al., 2006). Previous research investigating kin recognition with roots of G. hederacea has shown that this species displays an avoidance pattern, avoiding contact with roots of neighboring ramets regardless of its genetic or specific identity (Semchenko et al., 2007), a result we partially confirmed in the present study. In experiment 1 roots had a significant tendency to avoid growing toward the neighbor regardless of its identity, in comparison to roots that were grown alone that had a wider root system width. Whereas, in experiment 2, kin identity did not affect the maximum depth of the root system, it significantly affected root number and root distribution, as plants placed a larger proportion of their roots in the top third soil when growing with a non-kin neighbor.
We measured several previously reported plastic root traits known to be affected by water stress, but we found that our watering treatment only significantly affected the plants in experiment 2. The water treatment used in experiment 1 may not have been enough to induce a stress response, whether that be because the different container was able to retain moisture more effectively or due to the difference in the time of year the experiment was conducted. In experiment 2, water-limited plants produced more numerous and deeper roots that were placed on the lower section of the pots, indicating a plastic response to water availability. While we found no interactive effect of water limitation on root kin interactions in our experiments. Differences in aboveground mass accumulation were detected in experiment 2, where water limitation significantly reduced plant growth in competition. Resource limitations such as N limitation are known to affect kin interactions (Bisseling and Scheres, 2014). For example, previous work on nutrient availability found that nutrient availability altered the strength of kin interactions, with competition against non-kin being stronger at low nutrient availability (Pezzola et al., 2020), so we expected the same pattern when the limiting resource was water and thus the lack of interaction with kin is surprising. Our split root set-up did not allow comparison of aboveground responses to kin vs. non-kin roots, so we cannot confidently conclude that water limitation and kin recognition does not have an effect on aboveground mass acquisition and is something to investigate further in the future.
Studies that have investigated belowground kin interactions have been criticized for pitfalls related to the experimental design (Klemens, 2008). This includes experiments that keep a constant volume of substrate and amount of nutrients per plant (Gersani et al., 2001; Maina et al., 2002; Falik et al., 2003, 2006). Our results show differences between our approaches used in the two separate experiments, which differed in the volume of soil available to plants, the type of container used, as well as the timing when clonal fragments were collected from the same locations. In experiment 2, clonal fragments were collected in March when plants where flowering, whereas in experiment 1 plants were collected in July. Thus, the differences observed between the two experiments may be linked to the recognition mechanisms used by G. hederacea, which are still not fully understood. Besides direct physical contact between roots, plants can also detect neighbor’s presence by root exudates and/or volatile organic compounds (Schenk et al., 1999; Bais et al., 2006) which are known to be linked to plant phenology (Chaparro et al., 2013), with higher amounts being released during flowering times (Hamlen et al., 1972). Thus, further research should focus to identify how the mechanisms used in root recognition may change during plant phenology.
In this study we used clonal fragments as the kin individuals. This was advantageous to us in that we could take individuals straight from wild populations and of similar size. G. hederacea produces horizontal above-ground stems that root in the soil and provide a connection between the mother plant and its offspring for 1–2 years in natural conditions (Klimešová and de Bello, 2009). In this as in all clonal species, ramets are usually considered descendants from a genet and therefore considered kin offspring. However, there is some debate as whether our results should be considered under self-recognition rather than kin recognition. It has been argued that self/non-self recognition is part of the more specific mechanism focusing on kin/non-kin recognition (Chen et al., 2012). We think that self recognition of an unconnected ramet should be part of kin selection and recognition. Nevertheless, future experiments should be carried out to parameterize which level of relatedness between a mother plant and kin (self, mother-offspring, sibling, half-sibling) is needed to have kin selection occurring.
We should emphasize that root responses to abiotic stress may not only be mediated by the presence of neighboring roots. Root phenotypic plasticity allows plants to adapt to resource availability and competition as well as to other soil components including mycorrhizal fungi or soil bacteria (Ballare et al., 1990; Dudley and Schmitt, 1995; Schlichting and Smith, 2002; Sultan, 2003), and plant responses are ultimately regulated through a common network of signal-transduction pathways (see Pineda et al., 2013, and references there). Below-ground interactions are therefore complex and may include both strong and weak effects, sometimes in opposing directions. Further work is needed to integrate combinations of these mechanisms and processes to disentangle their ecological relevance.
Data Availability Statement
The raw data supporting the conclusions of this article will be made available by the authors, without undue reservation. The data is available at https://doi.org/10.6084/m9.figshare.13042058.v4.
Author Contributions
CS and SV conceived the study. EG collected the data. SV analyzed the data. SV, CS, and EG wrote the manuscript. All authors edited the final draft.
Funding
This study was funded by the University of Lincoln, School of Life Sciences MBio research project (EG).
Conflict of Interest
The authors declare that the research was conducted in the absence of any commercial or financial relationships that could be construed as a potential conflict of interest.
The reviewer, JV, declared a past co-authorship with one of the authors, SV, to the handling editor.
Acknowledgments
We thank MBio students who helped with experiment set ups and two reviewers for insightful comments.
References
Bais, H. P., Weir, T. L., Perry, L. G., Gilroy, S., and Vivanco, J. M. (2006). The role of root exudates in rhizosphere interactions with plants and other organisms. Annu. Rev. Plant Biol. 57, 233–266. doi: 10.1146/annurev.arplant.57.032905.105159
Bais, H. (2014). Shedding light on kin recognition response in plants. New. Phytol. 205, 4–6. doi: 10.1111/nph.13155
Ballare, C., Scopel, A., and Sanchez, R. (1990). Far-red radiation reflected from adjacent leaves: an early signal of competition in plant canopies. Science 247, 329–332. doi: 10.1126/science.247.4940.329
Barton, N. H., and Post, R. J. (1986). Sibling competition and the advantage of mixed families. J. Theor. Biol. 120, 381–387. doi: 10.1016/s0022-5193(86)80033-9
Biedrzycki, M., and Bais, H. (2010). Kin recognition: another biological function for root secretions. Plant Signal. Behav. 5, 401–402. doi: 10.4161/psb.5.4.10795
Biedrzycki, M., Jilany, T., Dudley, S., and Bais, H. (2010). Root exudates mediate kin recognition in plants. Commun. Integr. Biol. 3, 1–8.
Bisseling, T., and Scheres, B. (2014). Nutrient computation for root architecture. Science 346, 300–301. doi: 10.1126/science.1260942
Callaway, R. M., and Walker, L. R. (1997). Competition and facilitation: a synthetic approach to interactions in plant communities. Ecology 78, 1958–1965.doi: 10.1890/0012-9658(1997)078%5B1958:cafasa%5D2.0.co;2
Chaparro, J. M., Badri, D. V., Bakker, M. G., Sugiyama, A., Manter, D. K., and Vivanco, J. M. (2013). Root exudation of phytochemicals in Arabidopsis follows specific patterns that are developmentally programmed and correlate with soil microbial functions. PLoS One 8:e55731. doi: 10.1371/journal.pone.0055731
Chen, B. J. W., During, H. J., and Anten, N. P. R. (2012). Detect thy neighbor: identity recognition at the root level in plants. Plant Sci. 195, 157–167. doi: 10.1016/j.plantsci.2012.07.006
de Vries, J. (2013). Modelling the Effect of Competition Through Soil Resources on Root Architecture. MSc thesis, Utrecht University, Utrecht.
Dudley, S. A., and File, A. L. (2007). Kin recognition in an annual plant. Biol. Lett. 3, 435–438. doi: 10.1098/rsbl.2007.0232
Dudley, S. A., Murphy, G. P., and File, A. L. (2013). Kin recognition and competition in plants. Funct. Ecol. 27, 898–906. doi: 10.1111/1365-2435.12121
Dudley, S. A., and Schmitt, J. (1995). Genetic differentiation in morphological responses to simulated foliage shade between populations of Impatiens capensis from open and woodland sites. Func. Ecol. 9, 655–666. doi: 10.2307/2390158
Falik, O., de Kroon, H., and Novoplansky, A. (2006). Physiologically-mediated self/nonself root discrimination in Trifolium repens has mixed effects on plant performance. Plant Signal. Behav. 1, 116–121. doi: 10.4161/psb.1.3.2639
Falik, O., Reides, P., Gersani, M., and Novoplansky, A. (2003). Self/non-self discrimination in roots. J. Ecol. 91, 525–531. doi: 10.1046/j.1365-2745.2003.00795.x
File, A. L., Klironomos, J., Maherali, H., and Dudley, S. A. (2012). Plant kin recognition enhances abundance of symbiotic microbial partner. PLoS One 7:e45648. doi: 10.1371/journal.pone.0045648
Foxx, A. J., and Fort, F. (2019). Root and shoot competition lead to contrasting competitive outcomes under water stress: a systematic review and meta-analysis. PLoS One 14:e0220674. doi: 10.1371/journal.pone.0220674
Galkovskyi, T., Mileyko, Y., Bucksch, A., Moore, B., Symonova, O., Price, C. A., et al. (2012). GiA Roots: software for the high throughput analysis of plant root system architecture. BMC Plant Biol. 12:116. doi: 10.1186/1471-2229-12-116
Gersani, M., O’Brien, E. E., Maina, G. M., and Abramsky, Z. (2001). Tragedy of the commons as a result of root competition. J. Ecol. 89, 660–669. doi: 10.1046/j.0022-0477.2001.00609.x
Grime, J. P., Hodgson, J. G., and Hunt, R. (1988). Comparative Plant Ecology: A Functional Approach to Common British Species. London: Unwin-Hyman.
Hamilton, W. D. (1964). The genetical evolution of social behaviour. I. J. Theor. Biol. 7, 1–16. doi: 10.1016/0022-5193(64)90038-4
Hamlen, R. A., Lukezic, F. L., and Bloom, J. R. (1972). Influence of age and stage of development on the neutral carbohydrate components in root exudates from alfalfa plants grown in a gnotobiotic environment. Can. J. Plant Sci. 52, 633–642. doi: 10.4141/cjps72-097
Hutchings, M. J., and Price, E. A. C. (1999). Glechoma hederacea L. (Nepeta glechoma Benth. N. hederacea (L.) Trev.). J. Ecol. 87, 347–364. doi: 10.1046/j.1365-2745.1999.00358.x
Jackson, R. B., Sperry, J. S., and Dawson, T. E. (2000). Root water uptake and transport: using physiological processes in global predictions. Trends Plant Sci. 5, 482–488. doi: 10.1016/s1360-1385(00)01766-0
Klimešová, J., and de Bello, F. (2009). CLO-PLA: the database of clonal and bud bank traits of Central European flora. J. Veget. Sci. 20, 511–516. doi: 10.1111/j.1654-1103.2009.01050.x
Krannitz, P. G., and Caldwell, M. M. (1995). Root growth responses of three Great Basin perennials to intraspecific and interspecific contact with other roots. Flora 190, 161–167. doi: 10.1016/s0367-2530(17)30647-3
Kuznetsova, A., Brockhoff, P. B., and Christensen, R. H. B. (2017). lmerTest package: tests in linear mixed effects models. J. Stat. Soft. 82, 1–26.
Lenth, R. (2020). emmeans: Estimated Marginal Means, Aka Least-Squares Means. R Package Version 1.4.5. Available online at: https://CRAN.R-project.org/package=emmeans (accessed June 2020).
Litav, M., and Harper, J. L. (1967). A method for studying spatial relationships between the root systems of two neighbouring plants. Plant Soil 26, 389–392. doi: 10.1007/bf01880190
Mahall, B. E., and Callaway, R. M. (1991). Root communication among desert shrubs. Proc. Natl. Acad. Sci. U.S.A. 88, 874–876. doi: 10.1073/pnas.88.3.874
Mahall, B. E., and Callaway, R. M. (1992). Root communication mechanisms and intracommunity distributions of two Mojave Desert shrubs. Ecology 73, 2145–2151. doi: 10.2307/1941462
Mahall, B. E., and Callaway, R. M. (1996). Effects of regional origin and genotype on intraspecific root communication in the desert shrub Ambrosia dumosa (Asteraceae). Am. J. Bot. 83, 93–98. doi: 10.1002/j.1537-2197.1996.tb13879.x
Maina, G. G., Brown, J. S., and Gersani, M. (2002). Intra-plant versus inter-plant root competition in beans: avoidance, resource matching or tragedy of the commons. Plant Ecol. 160, 235–247.
Milla, R., Forero, D. M., Escudero, A., and Iriondo, J. M. (2009). Growing with siblings: a common ground for cooperation or for fiercer competition among plants? Proc. R. Soc. Lond. B 276, 2531–2540. doi: 10.1098/rspb.2009.0369
Murphy, G. P., and Dudley, S. A. (2009). Kin recognition: competition and cooperation in Impatiens (Balsaminaceae). Am. J. Bot. 96, 1990–1996. doi: 10.3732/ajb.0900006
Novoplansky, A. (2009). Picking battles wisely: plant behaviour under competition. Plant Cell Environ. 32, 726–741. doi: 10.1111/j.1365-3040.2009.01979.x
Pezzola, E., Pandolfi, C., and Mancuso, S. (2020). Resource availability affects kin selection in two cultivars of Pisum sativum. Plant Growth Regul. 90, 321–329. doi: 10.1007/s10725-019-00562-7
Pineda, A., Dicke, M., Pieterse, C. M., and Pozo, M. J. (2013). Beneficial microbes in a changing environment: are they always helping plants to deal with insects? Funct. Ecol. 27, 574–586.
Schenk, H. J., Callaway, R. M., and Mahall, B. E. (1999). Spatial root segregation: are plants territorial? Adv. Ecol. Res. 28, 145–180.
Schindelin, J., Arganda-Carreras, I., Frise, E., Kaynig, V., Longair, M., Pietzsch, T., et al. (2012). Fiji: an open-source platform for biological-image analysis. Nat. Methods 9, 676–682.
Schlichting, C., and Smith, H. (2002). Phenotypic plasticity: linking molecular mechanisms with evolutionary outcomes. Evol. Ecol. 16, 189–211.
Semchenko, M., John, E. A., and Hutchings, M. J. (2007). Effects of physical connection and genetic identity of neighbouring ramets on root-placement patterns in two clonal species. New Phytol. 176, 644–654.
Semchenko, M., Saar, S., and Lepik, A. (2014). Plant root exudates mediate neighbour recognition and trigger complex behavioural changes. New Phytol. 204, 631–637.
Simard, S. W., Asay, A. K., Beiler, K. J., Bingham, M. A., Deslippe, J. R., He, X., et al. (2015). “Resource transfer between plants through ectomycorrhizal networks,” in Mycorrhizal Networks, Ecological Studies, Vol. 224, ed. T. R. Horton (Cham: Springer), 133–176.
Sultan, S. (2003). Phentypic plasticity in plants: a case study in ecological development. Evol. Dev. 5, 25–33.
Vekemans, X., and Hardy, O. J. (2004). New insights from fine-scale spatial genetic structure analyses in plant populations. Mol. Ecol. 13, 921–935.
Keywords: plant competition, clonal plant, identity recognition, kin recognition, root competition, water stress, root recognition
Citation: Goddard EL, Varga S, John EA and Soulsbury CD (2020) Evidence for Root Kin Recognition in the Clonal Plant Species Glechoma hederacea. Front. Ecol. Evol. 8:578141. doi: 10.3389/fevo.2020.578141
Received: 30 June 2020; Accepted: 24 September 2020;
Published: 19 October 2020.
Edited by:
John Richard Pannell, University of Lausanne, SwitzerlandReviewed by:
Harsh Bais, University of Delaware, United StatesJulia Sanchez Vilas, Cardiff University, United Kingdom
Copyright © 2020 Goddard, Varga, John and Soulsbury. This is an open-access article distributed under the terms of the Creative Commons Attribution License (CC BY). The use, distribution or reproduction in other forums is permitted, provided the original author(s) and the copyright owner(s) are credited and that the original publication in this journal is cited, in accordance with accepted academic practice. No use, distribution or reproduction is permitted which does not comply with these terms.
*Correspondence: Ellen L. Goddard, ZS5nb2RkYXJkQGxib3JvLmFjLnVr; ZWxsZW5nb2RkYXJkOTVAZ21haWwuY29t