- 1Department of Neurobiology, University of Konstanz, Konstanz, Germany
- 2Department of Biosciences, Paris Lodron University of Salzburg, Salzburg, Austria
- 3Institute of Evolutionary Ecology and Conservation Genomics, Ulm University, Ulm, Germany
Social vespid wasps regularly forage on flowers with a generalist pollination system. However, little is known about communication between wasps and their host plants. The aim of this study was to investigate the role of olfactory and visual floral signals of Hedera helix and Heracleum sphondylium, both frequently visited by Vespula and Dolichovespula wasps for the collection of nectar. A combination of chemical, electrophysiological and spectral analyses and behavioral experiments was used to identify attractive floral signals. We identified 37 and 41 EAD-active substances (mainly terpenoids and aromatics) in H. helix and H. sphondylium, respectively. The most abundant floral compounds were 4-oxoisophorone in H. helix and linalool in H. sphondylium, followed by (E)-linalool oxide furanoid in both plants. The olfactory signals were attractive for wasps; however, a combination of olfactory and visual signals made both plants more attractive than olfactory signals alone and, in the case of H. helix, also than visual traits alone. Visual traits were not attractive by themselves. Wasps were also attracted by a synthetic solution resembling the floral scent of H. helix. Our study contributes to a better understanding of the foraging behavior of vespid wasps and describes, for the first-time, floral signals that attract vespine wasps to generalist flowers.
Introduction
Most plants are pollinated by animals, with various animal groups being involved (Ollerton et al., 2011). These include vertebrates such as mice, birds, and bats and a high taxonomic diversity of insects. Overall, insects are the most important pollinators (Ollerton, 2017). In contrast to well-known bees, butterflies and hoverflies as effective pollinators (Ollerton, 2017), wasps have been studied little in terms of their pollination performance. Although, wasps are mainly known for their predatory behavior (Raveret Richter, 2000), they frequently visit flowers to forage for nectar in easily accessible flowers (Schremmer, 1962; Akre et al., 1981) and can be highly effective pollinators (Ollerton et al., 2003, 2007; Narbona and Dirzo, 2010; Shuttleworth and Johnson, 2012; Wiemer et al., 2012; Zych et al., 2019).
Pollinators are attracted to flowers by visual and olfactory signals (Dobson, 2006; Raguso, 2008a). The relative importance of the floral traits and their specific characteristics (e.g., specific floral volatiles and colors; Ayasse et al., 2003; Burger et al., 2010, 2011; Schäffler et al., 2015) varies among pollination systems (Ômura and Honda, 2005; Balkenius et al., 2006; Dötterl et al., 2011; Fandino et al., 2019). Whereas the number of studies into the relative importance of olfactory and visual signals is increasing in various insects (Raguso, 2008a; Dötterl and Vereecken, 2010; Barragán-Fonseca et al., 2020), comparably little is known about this topic in wasps, despite mainly olfactory signals being known as important chemical mediators in specialized plant-wasp pollination systems. Such systems include those that signal the presence of prey (Orchidaceae: (Nazarov, 1995; Brodmann et al., 2008, 2009; Scrophulariaceae: Brodmann et al., 2012), a mating partner (Orchidaceae: Ayasse et al., 2003; Schiestl et al., 2003; Schiestl, 2005; Ayasse, 2006), or other associations in fig wasps (Song et al., 2001; Grison-Pigé et al., 2002), spider-hunting wasps (Orchidaceae: Johnson, 2005; Hyacinthaceae: Shuttleworth and Johnson, 2009a; Apocynaceae: Shuttleworth and Johnson, 2009b), or vespid wasps (Apocynaceae: Burger et al., 2017).
In addition to visiting flowers specialized for wasp pollinators, Vespine wasps feed on plant species with a generalist pollination system (ecologically and functionally generalized, Ollerton et al., 2007). Among such plants are the Apiaceae and Araliaceae that are frequently descried as important food plants for vespid wasps (Schremmer, 1962; Fateryga, 2010). However, to the best of our knowledge, no investigation has been carried out into the single or combined olfactory and visual signals that nectar-seeking vespid wasps use to find flowers of such generalist plants.
The aim of this study was to investigate the role of olfactory and visual signals used by Vespula and Dolichovespula wasps to locate Hedera helix and Heracleum sphondylium flowers, both frequently visited for the collection of nectar. We have studied the communication between vespine wasps (Vespula germanica FABRICIUS 1793, Vespula vulgaris LINNAEUS 1758; Dolichovespula sylvestris SCOPOLI 1763) (Figure 1) and the two plant species Hedera helix L. (Araliaceae) and Heracleum sphondylium L. (Apiaceae). In detail, we have performed chemical, electrophysiological, and spectral analyses, plus behavioral experiments. We have addressed the following questions. (1) Do both olfactory and visual signals of H. helix and H. sphondylium play a role in the attraction of Vespine wasps and what is their relative importance? (2) Are the floral colors, as perceived by wasps, distinguishable from background vegetation against which they are viewed? (3) Which components of the floral scent elicit antennal responses in Vespula wasps? (4) Does the (synthetically mixed) scent of physiologically active compounds of H. helix attract Vespula wasps?
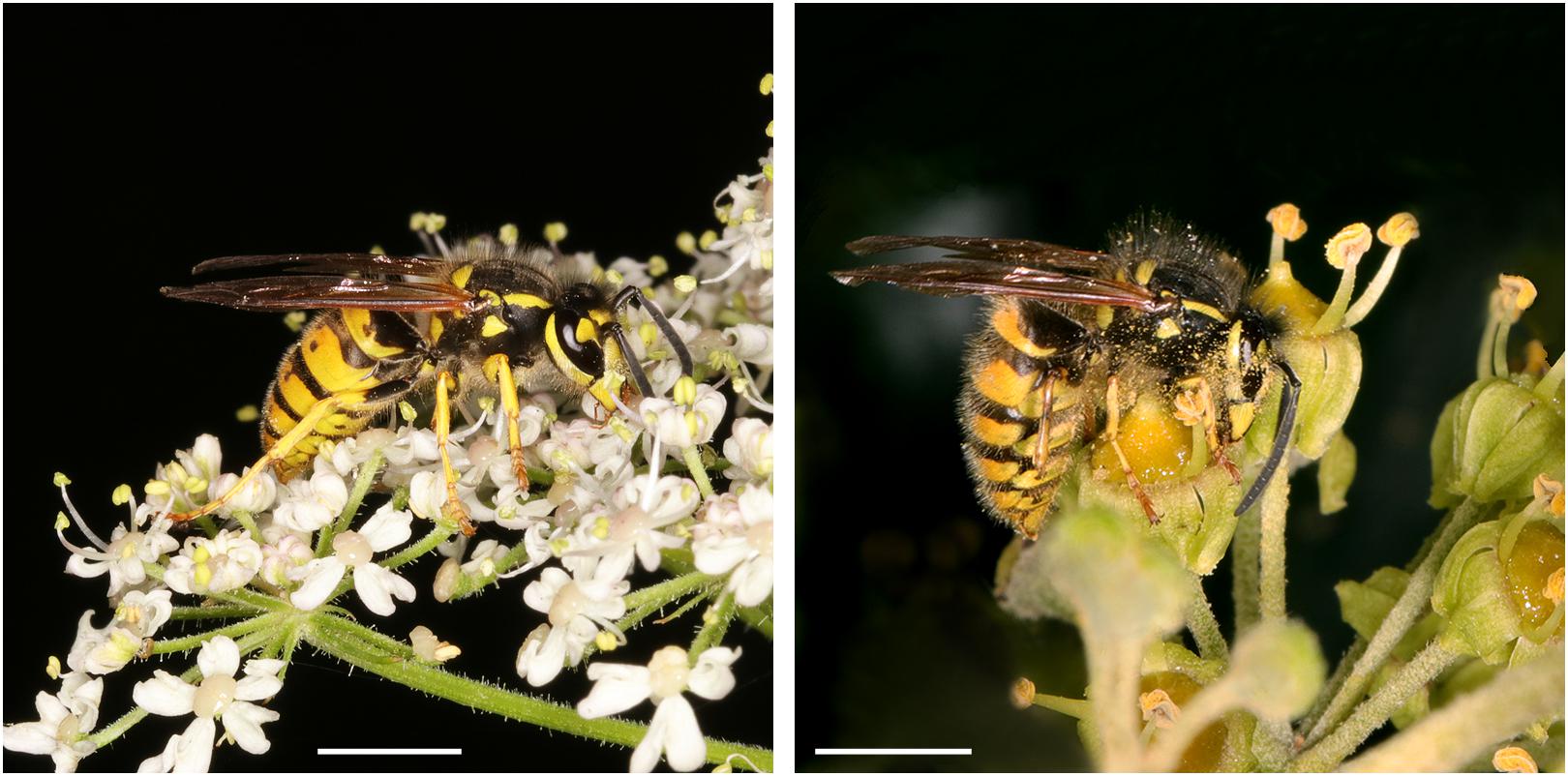
Figure 1. Vespula germanica wasp visiting inflorescences of Heracleum sphondylium (left) and Vespula vulgaris wasp visiting inflorescences of Hedera helix (right). Scale bars correspond to 0.5 cm.
Materials and Methods
Study Sites and Investigated Species
The Hedera helix L. (Araliaceae) plants used for the study were from Rosenheim (Germany; 47° 51′ 20.934″ N, 12° 7′ 38.276″ E), Salzburg (Austria; 47° 46′ 38.14″ N, 13° 3′ 58.77″ E) and Ulm (Germany; 48° 23′ 49.229″ N, 9° 59′ 54.121″ E), whereas Heracleum sphondylium L. (Apiaceae) plants were from Frasdorf (Germany; 47° 48′ 22.532″ N, 12° 15′ 48.935″ E) and Fürstätt (in Rosenheim but another site than used for H. helix; Germany; 47° 51′ 19.757″ N, 12° 5′ 46.223″ E). H. helix and H. sphondylium are perennial plants, widely distributed in Europe, with flowering periods from August to November (Metcalfe, 2005) and June to September (Sheppard, 1991), respectively. Both species have hermaphrodite flowers arranged in inflorescences. H. helix has panicles that consist of up to 20 terminal globose umbels with each having c. 10–25 actinomorphic, yellowish-green flowers (Metcalfe, 2005). The plants used in the study had hundreds/thousands of available umbels. H. sphondylium has double umbels that consist of 15–45 umbellules with each having c. 6–25 greenish-white flowers (Sheppard, 1991).
Both plants are frequently visited by vespine wasps and by many other insects including other Hymenopteran, Dipteran, Coleopteran, Hemipteran, and Lepidopteran species (Corbet, 1970; Sheppard, 1991; Metcalfe, 2005; Vezza et al., 2006; Zych, 2007; Jacobs et al., 2010), with the relative impact of visitors on pollination being largely unknown. Although Vespula wasps are the most effective pollinators for H. helix (Ollerton et al., 2007; Jacobs et al., 2010) and medium-sized flies for H. sphondylium (Zych, 2007) in some populations, both plant species have temporally and spatially variable pollinator species (Zych, 2007; Jacobs et al., 2010).
Color Analysis
The colors of H. helix and H. sphondylium flowers were investigated with a Jaz spectrophotometer (Ocean Optics Inc., Dunedin, FL, United States) equipped with a pulsed xenon light source (JAZ-PX) and attached to a fiber-optic cable (UV/VIS 400 μm; World Precision Instruments Inc., Sarasota, FL, United States). The optical fiber was fixed onto an attachment so that the light touched the investigated object under an angle of 45°. The spectral reflection from 300 to 700 nm was recorded for gynoecium, petals, and pollen. Spectralon was used as a white standard (Labsphere, North Sutton, NH, United States). The mean reflection was calculated for three to five replicates (a replicate was obtained from a mean of three measurements per plant individual) and used for the analyses. The R package pavo was used to process the obtained raw data (Maia et al., 2013).
Colors as sensed by the wasps were modeled using the color hexagon according to Chittka (1992) to assess whether the flowers stood out from the background against which they were viewed. We used, the receptor sensitivity data available for V. germanica (Peitsch et al., 1992) and the standard daylight irradiance spectrum D65 (Wyszecki and Stiles, 1982) in the model. Typically, hymenopteran species do not differ substantially in their sensory systems (Peitsch et al., 1992), and therefore, we used the spectral sensitivity functions for V. germanica as a representative approximation also for other Vespine wasp species (V. vulgaris and D. sylvestris). We used our own color measurements of H. helix leaf topsides as background in H. helix, as the inflorescences of this species are presented against their own leaves that form a dense vegetative cover. As H. sphondylium plants grow within different vegetation types, the reflectance function of a typical green leaf standard (Chittka et al., 1994) was used as background color.
Volatile Collection
Scent samples from inflorescences and green leaves were collected in situ by dynamic headspace approaches (Dötterl et al., 2005). Inflorescences or leaves were enclosed in polyethylene oven bags (c. 30 × 15 cm; Toppits®, Melitta, Germany) during scent collections. Scent samples collected from empty oven bags were used as negative controls.
To obtain samples for electrophysiological analysis and identification of EAD-active compounds, scent was trapped using large adsorbent tubes (Duran glass capillaries; outer diameter 6.0 mm; inner diameter 4.0 mm; length 80 mm; Paul Stollwerk Glasbläserei, Emmerting, Germany), filled with 10 mg each of Tenax TA (mesh 60–80) and Carbotrap B (mesh 20–40; both Supelco, Bellefonte, PA, United States) and fixed with glass wool plugs. Samples were collected for two to six hours by pulling air through the tubes using a membrane pump (G12/01 EB, Rietschle Thomas Inc., Puchheim, Germany; Flow rate: 200 mL min–1). After being bagged and before the sampling started, volatiles were sucked out of the bag for 30 s to avoid volatiles being induced by injuries during bagging. Bags were covered with aluminum foil to avoid any direct solar irradiation that would result in the condensation of large amounts of water inside the bags. All samples were collected between 10 am and 4 pm. Adsorbent tubes were finally eluted with 80 μl acetone (GC/HPLC Grade, Rotisolv, Carl Roth GmbH + Co KG, Karlsruhe; Dötterl et al., 2005). In H. helix, an average of 30 (21–43) globose umbels with 20–30 florets each, and, in H. sphondylium, an average of 36 (15–73) umbellules were bagged. We collected eight samples from a H. helix individual in Ulm and two samples from another individual in Rosenheim. Two to five samples from each individual were afterwards pooled to obtain three samples for EAD analyses. For H. sphondylium, three samples were collected from three individuals in Frasdorf and six samples from six individuals in Fürstätt. Two samples from Frasdorf were used individually, and all other samples were pooled into one large sample to finally obtain three samples for EAD analyses.
For TD-GC/MS (thermal desorption – gas chromatography/mass spectrometry) analyses, samples from various individuals were trapped in order to test for differences in the pattern of EAD-active compounds between inflorescences and leaves. Smaller adsorbent tubes (Quartz glass capillaries; outer diameter 2.5 mm; inner diameter 1.9 mm; length 25 mm; Hilgenberg GmbH, Malsfeld, Germany) filled with 1.5 mg each of Tenax TA (mesh 60–80) and Carbotrap B (mesh 20–40; both Supelco, Bellefonte, PA, United States) were used. Volatiles were sampled between 10 am and 6 pm. Inflorescence samples each from different individuals were collected from one umbel (in H. helix, on average 8 umbellules per umbel, and in H. sphondylium, on average 13 umbellules per umbel), and vegetative samples were taken from one leaf stalk (H. helix: 10 leaves per stalk, H. sphondylium: one leaf per stalk). H. helix samples were collected from inflorescences (n = 18 samples), leaves (n = 15), and air (n = 9) at various sampling sites in Ulm, Rosenheim and Salzburg for 10 min. H. sphondylium samples were collected from inflorescences (n = 27 samples), leaves (n = 10), and air (n = 6) in Frasdorf and Fürstätt. Samples were collected for 5 min or 10 min.
Electrophysiological Analysis
Physiologically active volatiles in antennae of vespine wasps (V. germanica, V. vulgaris) were detected using GC/EAD. Wasps were collected in Rosenheim (47° 51′ 11.426″ N; 12° 5′ 46.525″ E), Ulm (48° 23′ 50.19″ N; 9° 59′ 56.67″ E), and Salzburg (47° 46′ 38.309″ N; 13° 3′ 58.662″ E) during foraging flights on H. helix flowers and were stored in the dark at 4°C (max. 24 h). The antennae were cut off at the base and tip and positioned between two capillaries filled with Ringer solution (8.0 g L–1 NaCl, 0.4 g L–1 KCl, 0.4 g L–1 CaCl2). The base of an antenna was connected to the reference capillary, the tip was connected to the recording capillary. The capillaries were connected to silver wires and placed in front of the GC outlet (Lukas et al., 2019).
The setup consisted of a GC (Agilent 7890 A, Santa Clara, CA, United States) equipped with a flame ionization detector (FID) and an electroantennographic detection system (EAD). The EAD system was equipped with a transfer line, heated to 220°C, and a 2-Channel USB acquisition controller (IDAC-2; Syntech, Kirchzarten, Germany). The GC was equipped with a ZB-5 fused silica column (5% phenyl polysiloxane; 30 m long, inner diameter 0.32 mm, film thickness 0.25 μm, Phenomenex). Hydrogen was used as the carrier gas (flow: 3 mL min–1) into which solvent samples (see volatile collection) were injected (1 μl; 250°C) in the splitless mode at an oven temperature of 40°C. The split vent opened (1:100) 0.5 min after injection and the oven temperature increased by 10°C min–1 to 220°C (held for 2 min). The column was split into two deactivated capillaries by a 4-way-microflow splitter (Gerstel, Mühlheim, Germany) of which one led to the FID (2 m × 0.15 μm inner diameter) and the other to the EAD (1 m × 0.2 μm inner diameter). Nitrogen gas (N2; flow: 25 mL min–1) was used as the make-up gas and was introduced through the fourth arm of the splitter. The outlet of the EAD was placed in a cleaned and humidified airflow (tube inner diameter: 7.5 mm) directed over the wasp antenna (Heiduk et al., 2015). Responses from 22 (12 V. germanica, 10 V. vulgaris) and 15 (8 V. germanica, 7 V. vulgaris) wasp individuals (all workers) to H. helix and H. sphondylium floral scent samples, respectively, were recorded. After the measurements, individuals were frozen for species identification. As both species responded to the same set of compounds, we pooled the data for further analyses. Volatiles to which five or more individuals responded were considered as EAD-active. Active compounds were assigned to GC/MS runs by comparing the elution sequence and Kovats retention indices.
Chemical Analysis
Solvent scent samples were analyzed using a GC/MS system (QP2010 Ultra, Shimadzu) to identify the EAD-active compounds. The GC was equipped with a ZB-5 fused silica column (5% phenyl polysiloxane; 30 m long, inner diameter 0.32 mm, film thickness 0.25 μm, Phenomenex, Aschaffenburg, Germany) and helium was used as the carrier gas (flow: 3 mL min–1). Samples (1 μl; split ratio 1:1) were injected by means of an AOC-20i autoinjector (Shimadzu, Tokyo, Japan) at 200°C. At the end of the column, the effluent was split (AFT splitter package, Shimadzu) into two deactivated capillaries (inner diameter 0.2 mm). One thereof (length: 70 cm) transferred two thirds of the effluent to a connected EAD system (not used in the study), whereas the other (length: 2 m) transferred one third of the effluent to the MS. The temperature of the GC oven was started at 40°C (held for 1 min) and was then increased by 10°C per min to 220°C (held for 2 min). The MS interface worked at 220°C and the ion source at 200°C. Mass spectra were taken at 70 eV (El mode) from m/z 30 to 350, and data were further processed as described above (Heiduk et al., 2015; Lukas et al., 2019).
TD samples were analyzed with an automatic thermal desorption system (model TD-20, Shimadzu, Japan), connected to a GC/MS (model QP2010 Ultra El, Shimadzu, Japan), which was equipped with a ZB-5 fused silica column (5% phenyl polysiloxane; 60 m long, inner diameter 0.25 mm, film thickness 0.25 μm, Phenomenex, Aschaffenburg, Germany). Samples were run with a split ratio of 1:1, and helium was used as the carrier gas (flow: 1.5 mL min–1). The temperature in the GC oven was started at 40°C, was increased by 6°C per min to 250°C, and was held constant for 1 min. The MS interface worked at 250°C. Mass spectra were taken at 70 eV (El mode) from m/z 34 to 350 (Heiduk et al., 2015; Oliveira et al., 2017).
Data were processed by using the GCMSsolution package, Version 4.41 (Shimadzu Corporation). Compounds were tentatively identified by using both the mass spectral libraries Adams (2007), W9N11, NIST 11, FFNSC 2 and ESSENTIAL OILS (available in MassFinder 3) and literature data on Kovats retention indices (KRI, based on n-alkane series) of the compounds. Only compounds with a calculated Kovats index ±10 compared with various data bases were considered (NIST 11; Adams, 2007). The identity of the compounds was confirmed by a comparison of mass spectra and retention times on all used systems with synthetic standard compounds, if they were available in the reference collection of the Plant Ecology Laboratory of the University of Salzburg. For quantitative analyses, 100 ng each of c. 150 compounds, among them monoterpenes, aliphatic, and aromatic compounds, were injected into the GC/MS system. The mean of the peak areas (total ion current) of these components was used to estimate the total amount of the EAD-active compounds (see Etl et al., 2016).
Only compounds identified as EAD-active based on solvent scent samples were considered for the analysis of TD samples. EAD-active compounds found in ambient air samples were excluded from the analyses. Volatiles were categorized as floral compounds when they were only found in inflorescence samples or when they occurred only in traces in leaf samples, but at higher amounts in flower samples. Identified volatile compounds were grouped in classes according to Knudsen et al. (2006).
Differences in the floral scent bouquet of EAD-active compounds of the two plant species were analyzed using Primer 6.1.15 (Clarke and Gorley, 2005; Anderson et al., 2008). Thereby, semi-quantitative (relative amount of single scent components with respect to the total amount in a sample; Bray-Curtis index) similarities were calculated. Data for semi-quantitative analysis were square-root-transformed in order to reduce the influence of compounds available in large relative amounts. Similarities and dissimilarities in the samples were visualized using non-metric multidimensional scaling. Based on the Bray-Curtis matrix, an ANOSIM (one-way; 9999 permutations) was performed using the same software package to test for a plant species effect. Based on the transformed data (square root), a SIMPER (one-way; cut-off percentage: 90) was performed using Primer in order to evaluate the contribution of single substances to the observed dissimilarity between the species.
Behavioral Experiments
Heracleum sphondylium
Bioassays with H. sphondylium (hogweed) plants were conducted with foraging-experienced Dolichovespula sylvestris wasps, as mainly individuals of this wasp species were active during the main flowering period of this species, whereas Vespula wasp individuals were still low in number and their nests small and thus could not be used for these experiments. Two nests were transferred to wooden boxes (30 cm × 50 cm × 50 cm) with two entrance holes (diameter 5 cm) and were placed on the ground of a flight cage (2 m × 3 m × 3 m) located close to the campus of the University of Ulm. The wasps were allowed to forage inside and outside of the flight cage once they had been introduced to the cage in order to habituate them to the new environment. The wasps were offered sugar water, dead mealworms, apples, and H. sphondylium flowers inside the flight cage. After approximately one week, the door of the flight cage was closed, and the experiments started two days later. Food sources were removed one hour before an experiment started.
To determine the relative importance of olfactory and visual inflorescence signals of H. sphondylium in attracting wasps, we performed two-choice cylinder (29 cm height, 10 cm diameter) assays following Burger et al. (2010), in which we offered coupled and decoupled olfactory and visual plant signals to the wasps. Visual signals were investigated using transparent cylinders made of acrylic UV-transmitting glass without holes. Olfactory signals were investigated using black cylinders with small holes in order to block visual signals, but to allow the diffusion of scents. The combined olfactory and visual signals were investigated using transparent cylinders with holes. Release of volatiles from cylinders that included olfactory signals was supported by a membrane pump (flow: 1 L min–1; G12/01 EB, Rietschle Thomas Inc., Puchheim, Germany). Five inflorescences together were placed in the cylinders for each test, and empty cylinders were used as negative controls.
In detail, we tested decoupled and combined olfactory and visual inflorescence signals of H. sphondylium against empty controls. Since olfactory signals were more attractive than visual signals, we also tested combined olfactory and visual against olfactory inflorescence signals in order to investigate whether visual traits enhanced the attractiveness of olfactory signals. The two choices were offered 60 cm apart from each other on a table in the middle of the flight cage. An experiment lasted for 20 min during which the position of the choices was changed after 10 min. A maximum of three experiments was performed per day. Only wasps that directly approached the choices were recorded. A specific choice assay was conducted twice each or until at least a minimum number of 12 wasps participated. To ensure that an individual wasp was counted only once in a specific choice assay, we caught the responding wasps with an insect net and marked and released them back into the cage after the experiment. One individual wasp might, however, have participated in different choice tests. For statistical analysis, data were calculated by the exact binomial test.
Hedera helix
For experiments with H. helix, which flowers later in the year than H. sphondylium, we used V. germanica wasps for behavioral experiments. This species was still frequently foraging, when nests of D. sylvestris were empty. A transportable flight cage (90 cm x 60 cm x 60 cm) was open at one of the sides (90 cm × 60 cm) and placed with this side on the soil above a ground nest of V. germanica, available in the Botanical Garden of the Paris-Lodron-University of Salzburg. One side of the cage consisted of a transparent plastic layer, which transmitted wavelengths above 300 nm, as determined by a Jaz Spectrometer (Ocean Optics Inc., Dunedin, FL, United States). The other sides consisted of white gauze (n = 3) and blue plastic (n = 1; positioned opposite to the direction of the sun), respectively. Wasps leaving the nest and entering the flight cage were used for experiments.
We tested whether wasps discriminated between leaf and inflorescence visual appearances and scents based on decoupled olfactory and visual signals. We also tested the attractiveness of combined olfactory and visual signals against decoupled olfactory and visual signals, and whether synthetic scents consisting of EAD-active volatiles explained the attractiveness of natural olfactory signals.
The inflorescences and vegetative branches of H. helix used for the experiments were collected in Salzburg (47° 48′ 00.7″N; 13° 03′ 27.3″E). Only inflorescences in full bloom and visually undamaged leaved branches were collected. Five inflorescences (c. 50 umbels) or leafy branches (c. 50 leaves) were bound together for each experiment, with the cut ends being wrapped with parafilm (Bemis, United States) to reduce the emission of green leaf volatiles that could influence the behavior of wasps (Brodmann et al., 2008).
Since the experiments with H. helix were conducted in a smaller cage than the experiments with H. sphondylium, those with H. helix could not be performed with the cylinders described above, and the test equipment had to be adjusted to the smaller area available. Visual signals were investigated with transparent polyethylene oven bags (30 × 20 cm; Toppits®, Melitta, Germany), which were sealed by a soldering iron once the plant material had been introduced. These bags are scent-impermeable but transmitted light in the range relevant for insect vision (300–700 nm; Chittka, 1992) as determined by the Jaz Spectrometer described above. Olfactory signals were investigated with perforated bags (30 × 20 cm; Toppits®, Melitta, Germany; c. 300 holes; diameter c. 1 mm, punched with a laboratory needle) covered with a non-transparent white net (gauze), which blocked the visual signals of the plant material, but was scent permeable. Combined olfactory and visual signals were investigated by using perforated bags only. Release of volatiles from the bags was supported by a membrane pump (flow: 0.9 L min–1; G12/01 EB, Rietschle Thomas Inc., Puchheim, Germany).
Synthetic flower scent was applied to filter paper (diameter 7 cm) and offered as olfactory signals in perforated polyethylene bags covered with gauze. The sample used for the experiments contained all EAD-active compounds classified as “floral compound”, except for dihydrooxoisophorone, which was not available (Tables 1, 2). The compounds were added as a pure substance or diluted in mineral oil (Sigma-Aldrich, BioUltra, Darmstadt, Germany) to the stock solution of the synthetic mixture according to Table 2. All compounds except for β-ocimene, linalool oxide pyranoid (BOC Science), and acetophenone (Fluka) were obtained from Sigma-Aldrich, and all compounds except for phenylacetaldehyde (90%) had a purity of at least 95%. The solution had a total volume of 296 μl, which corresponded to 10,000 umbels; 29.6 μl of this solution (corresponding to 1,000 umbels) was taken and mixed with in 970.4 μl mineral oil. In a next step, the solution was diluted 1:10 in mineral oil. An amount of 50 μl of the final solution was added to a filter paper, which released the compounds in almost identical semi-quantitative amounts as the headspace samples from umbels, as determined by GC/MS of the dynamic headspace samples collected from filter papers. The amount used in the bioassay corresponded to the amount of floral scent emitted by five umbels of H. helix.
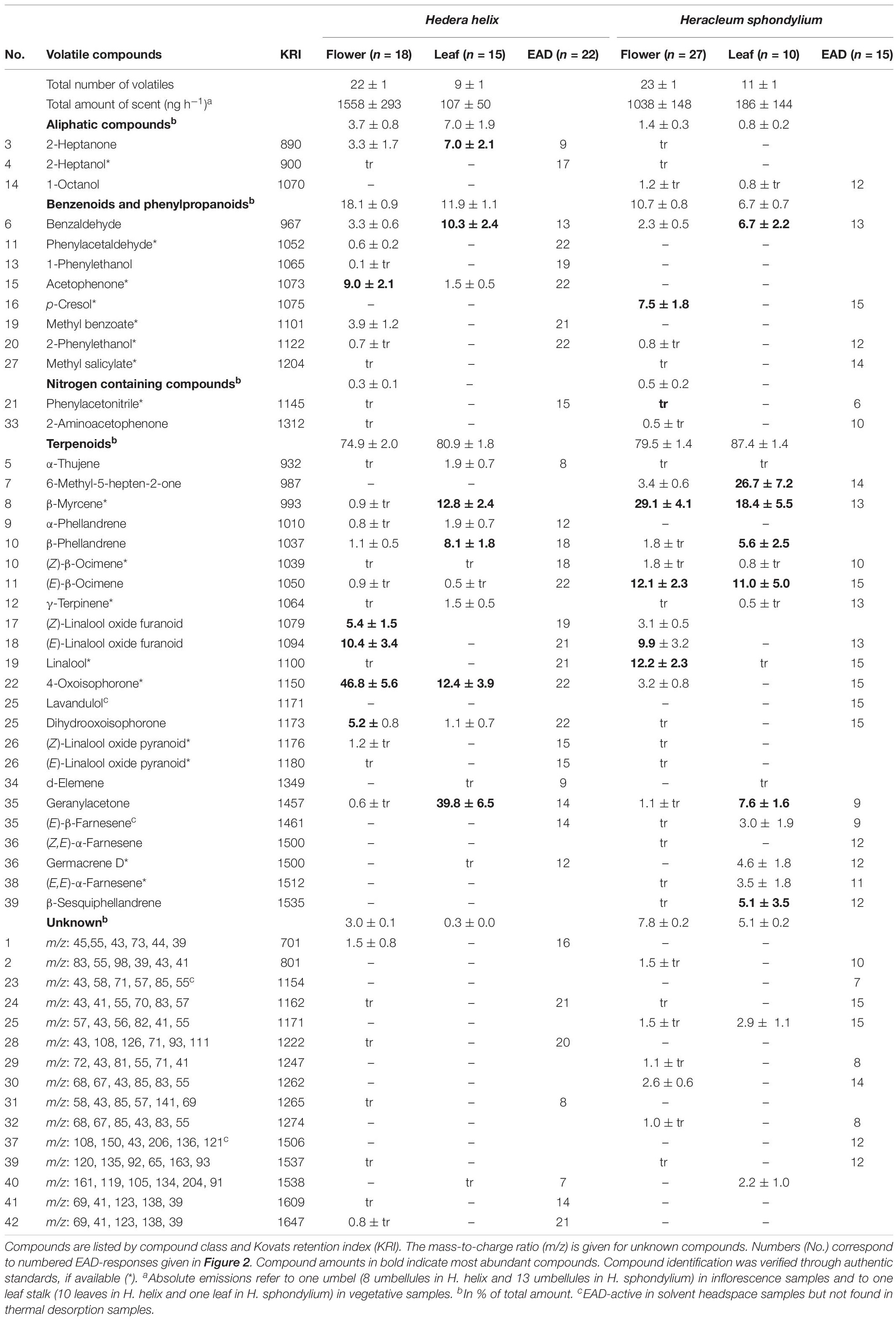
Table 1. Total and relative amounts (mean ± standard error, tr, trace amounts <0.5%) of electrophysiologically active volatiles in antennae of Vespula germanica/Vespula vulgaris wasps (EAD: number of responding antennae) detected in floral scent samples of Hedera helix and Heracleum sphondylium.
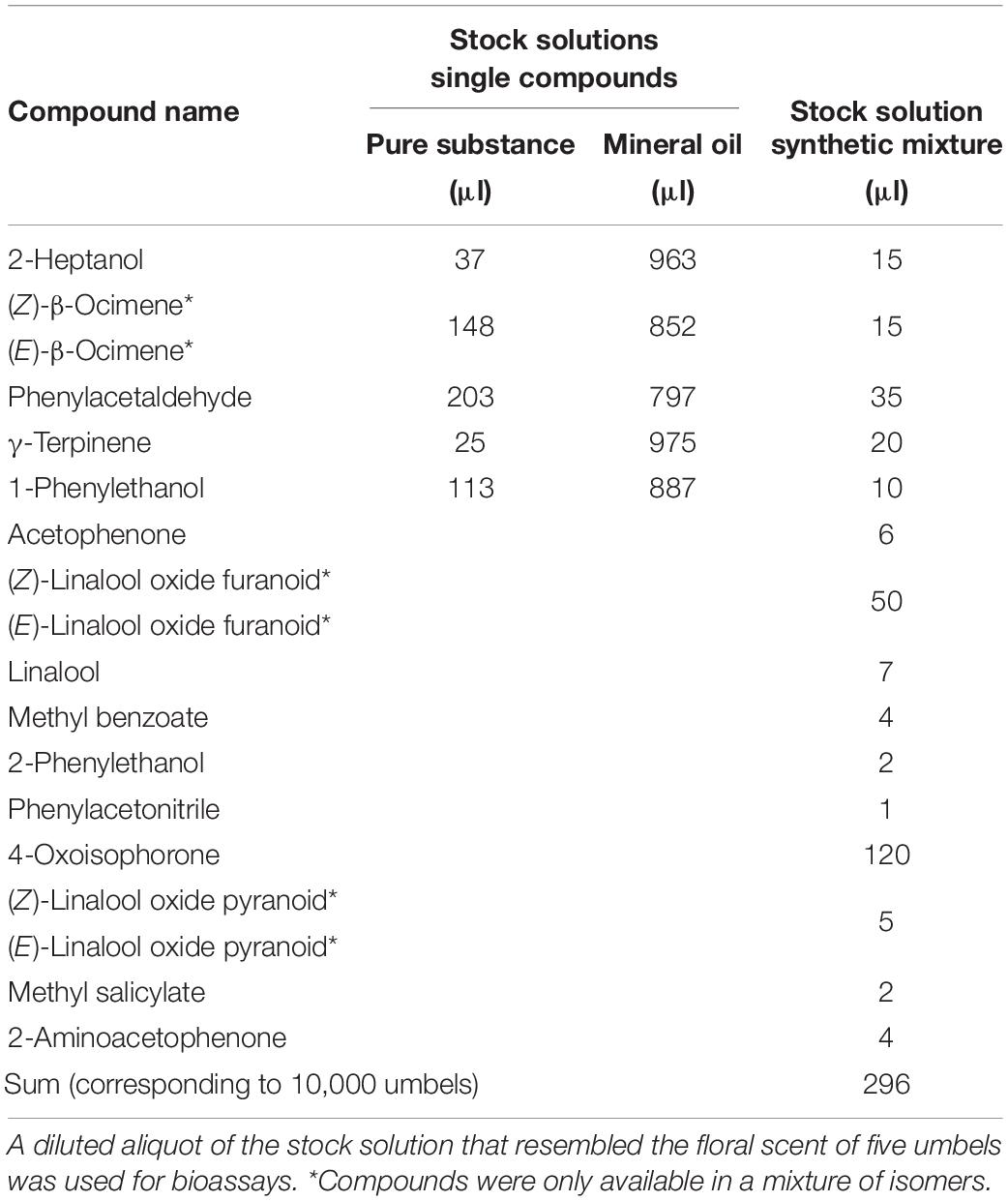
Table 2. Composition of synthetic solution from Hedera helix based on semi-quantitative amounts identified in headspace samples according to Table 1.
In detail, we tested (1) decoupled olfactory and visual inflorescence signals against respective vegetative controls and (2) combined olfactory and visual signals against decoupled olfactory and visual signals. The synthetic sample (3) was tested against a solvent control (mineral oil: Sigma-Aldrich, BioUltra, Darmstadt, Germany) and against natural olfactory signals of H. helix inflorescences. The two choices were offered at a 30 cm distance from the nest entrance and 60 cm from each other. An experiment lasted for one hour (from the installation to the removal of the cage above the nest entrance), during which an average of c. 175 wasps was simultaneously flying in the cage. Only during two of the choice experiments was a smaller number of wasps (visual versus leaf control: 30 wasps and synthetic versus solvent control: 50 wasps) active in the cage. A maximum of two experiments was performed per day, between 11 am and 14 pm. Each specific choice assay was conducted 2–3 times. Given the difficulty of discriminating approaching wasps from wasps randomly passing by a choice in this smaller flight cage, only wasps that landed on the bags were included in the statistical analysis (exact binomial test). We cannot exclude that a single wasp landed more than once during a specific assay: however, as we did not reward the wasps, and as a high number of wasps was active in the cage (see above), this issue (pseudo replication) is unlikely to have strongly biased the results.
Results
EAD-Active Compounds
Heracleum sphondylium flowers emitted 41 compounds EAD-active in the antennae of V. germanica and V. vulgaris (Table 1 and Figure 2), all of which, with the exception of three compounds, were also available in the TD samples. Thereof, 20 volatiles were classified as inflorescence-specific, 15 were emitted from inflorescence and vegetative plant material (including six compounds found only in traces in vegetative material, which is why they were also described as inflorescence compounds), and three were only found in vegetative plant material. Overall, we detected 19 terpenoids, four aromatics, three aliphatic compounds, three nitrogen-containing compounds, and six unknown compounds in floral samples (Table 1), where only 18 of these physiologically active compounds were found in leaf samples. These included 14 terpenoids and one aromatic, one aliphatic, and two unknown compounds. The most abundant EAD-active floral components were linalool (12 ± 2% (mean ± SE)), (E)-linalool oxide furanoid (10 ± 3%), and p-cresol (8 ± 2%), whereas the most abundant components in vegetative samples were 6-methyl-5-hepten-2-one (27 ± 7%), β-myrcene (18 ± 6%), (E)-β-ocimene (11 ± 5%), benzaldehyde (7 ± 2%), and geranylacetone (8 ± 2%). None of the other compounds exceeded 5% in the floral and vegetative samples, respectively. The total amounts of EAD-active compounds were 1038 ± 148 ng umbel–1 h–1 (mean ± SE) and 186 ± 144 ng leaf–1 h–1 in the floral and vegetative samples, respectively.
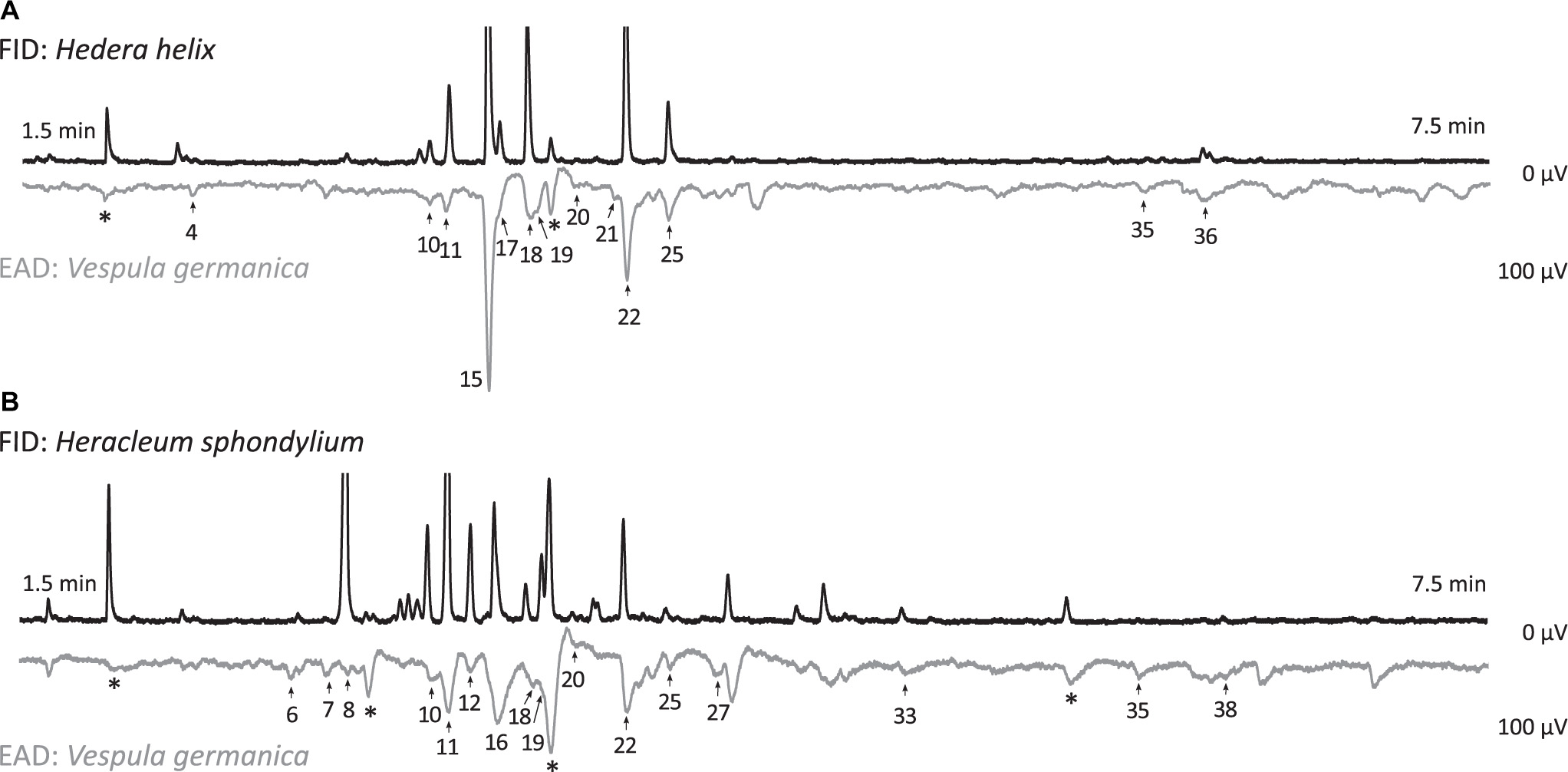
Figure 2. Electroantennographic responses of Vespula vulgaris antennae (EAD: electroantennographic detection) to floral scent samples (FID: flame ionization detection) of (A) Hedera helix and (B) Heracleum sphondylium. Numbers of EAD responses correspond to numbers given in Table 1 (*, responses to contamination). Axes show retention time and amplitude of responses.
Hedera helix inflorescences emitted 37 compounds that stimulated responses in the antennae of V. germanica and V. vulgaris wasps (Table 1 and Figure 2), all of which, with the exception of one compound, were also available in the TD samples. Thereof, 19 volatiles were inflorescence-specific, 14 were emitted from inflorescences and vegetative plant material (including seven compounds found only in traces in vegetative material, which is why they were also described as inflorescence compounds), and three were only found in vegetative plant material. Overall, we detected 15 terpenoids, seven aromatics, and two aliphatic, three nitrogen-containing, and six unknown compounds (Table 1) in floral samples of H. helix, whereas only 17 of these physiologically active compounds were found in leaf samples. These included twelve terpenoids and three aromatics, one aliphatic, and one unknown compound. The most abundant EAD-active components, classified as inflorescence-specific, were 4-oxoisophorone [47 ± 6% (mean ± SE)], (E)-linalool oxide furanoid (10 ± 3%), acetophenone (9 ± 2%), (Z)-linalool oxide furanoid (5 ± 2%), and dihydrooxoisophorone (5 ± 1%). None of the other compounds exceeded a mean relative amount of 5%. The most abundant compounds in vegetative samples (EAD-activity based on floral compounds) were geranylacetone [39 ± 7% (mean ± SE)], β-myrcene (13 ± 2%), benzaldehyde (10 ± 2%), β-phellandrene (8 ± 2%), and 2-heptanone (7 ± 2%). The total amounts of EAD-active scents were 1558 ± 293 ng umbel–1 h–1 (mean ± SE) and 107 ± 50 ng leaf–1 h–1 in floral and vegetative samples, respectively.
Significant semi-quantitative (ANOSIM: R = 0.996; p < 0.01; Figure 3) differences were found in the EAD-active floral scent bouquets of H. helix and H. sphondylium. The average Bray-Curtis dissimilarity in scent between the two species was 71%, and the compounds most responsible for this dissimilarity were 4-oxoisophorone (contributing 11% to this dissimilarity according to a SIMPER analysis), β-myrcene (9%), linalool (5%), and acetophenone (5%). Overall, 12 EAD-active compounds were common to the flower scents of the two plant species (+ one unknown; Table 1 and Figure 2), whereof seven [(Z)-β-ocimene, (E)-linalool oxide furanoid, linalool, 2-phenylethanol, phenylacetonitrile, 4-oxoisophorone, dihydrooxoisophorone] and an unknown component were flower-specific, and five [benzaldehyde, (E)-β-ocimene, geranylacetone, (E)-β-farnesene, germacrene D] were also found in vegetative samples.
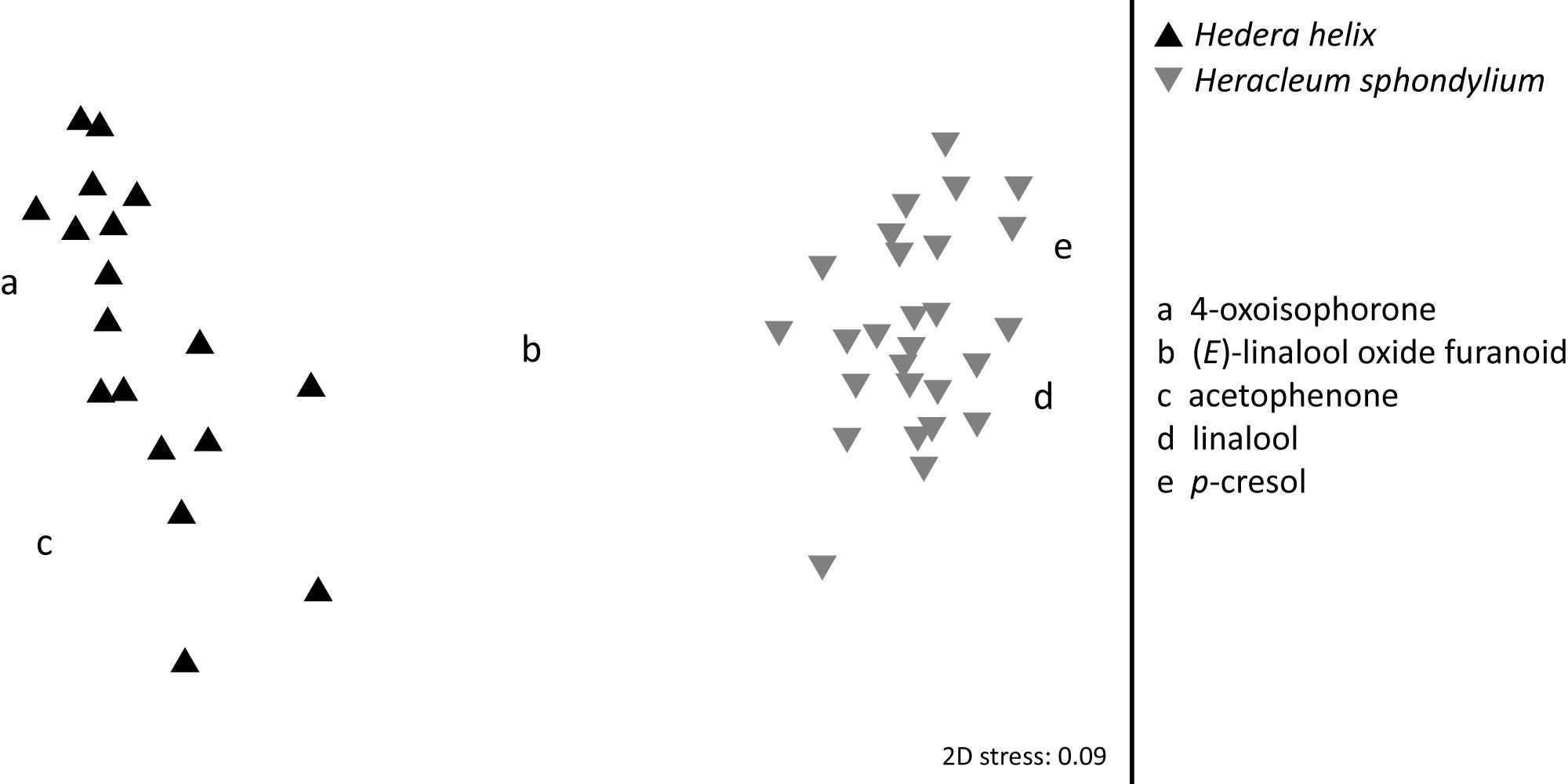
Figure 3. Semi-quantitative differences between EAD-active compounds emitted by flowers of Hedera helix and Heracleum sphondylium. Letters describe the main floral compounds responsible for the differences between plants.
Color Analysis
The flower organ colors of H. helix and H. sphondylium were separated from each other in the color hexagon (Figure 4). H. helix plant organs built a close group (all pairwise comparisons <0.1 hexagon units) at the intersections of the categories UV-blue and UV, whereas the plant organs of H. sphondylium were located in the blue-green intersection of the hexagon. H. helix petals and pollen lay close to the center of the hexagon (0.08, 0.10 units), whereas the gynoecium occurred at a slightly larger distance (0.16 units) from the center. H. sphondylium gynoecium and petals were also found to lie close to each other (<0.1 units), with both organs having the longest distance (0.39 units) to the center, whereas H. sphondylium pollen was closer to the center (0.25 units).
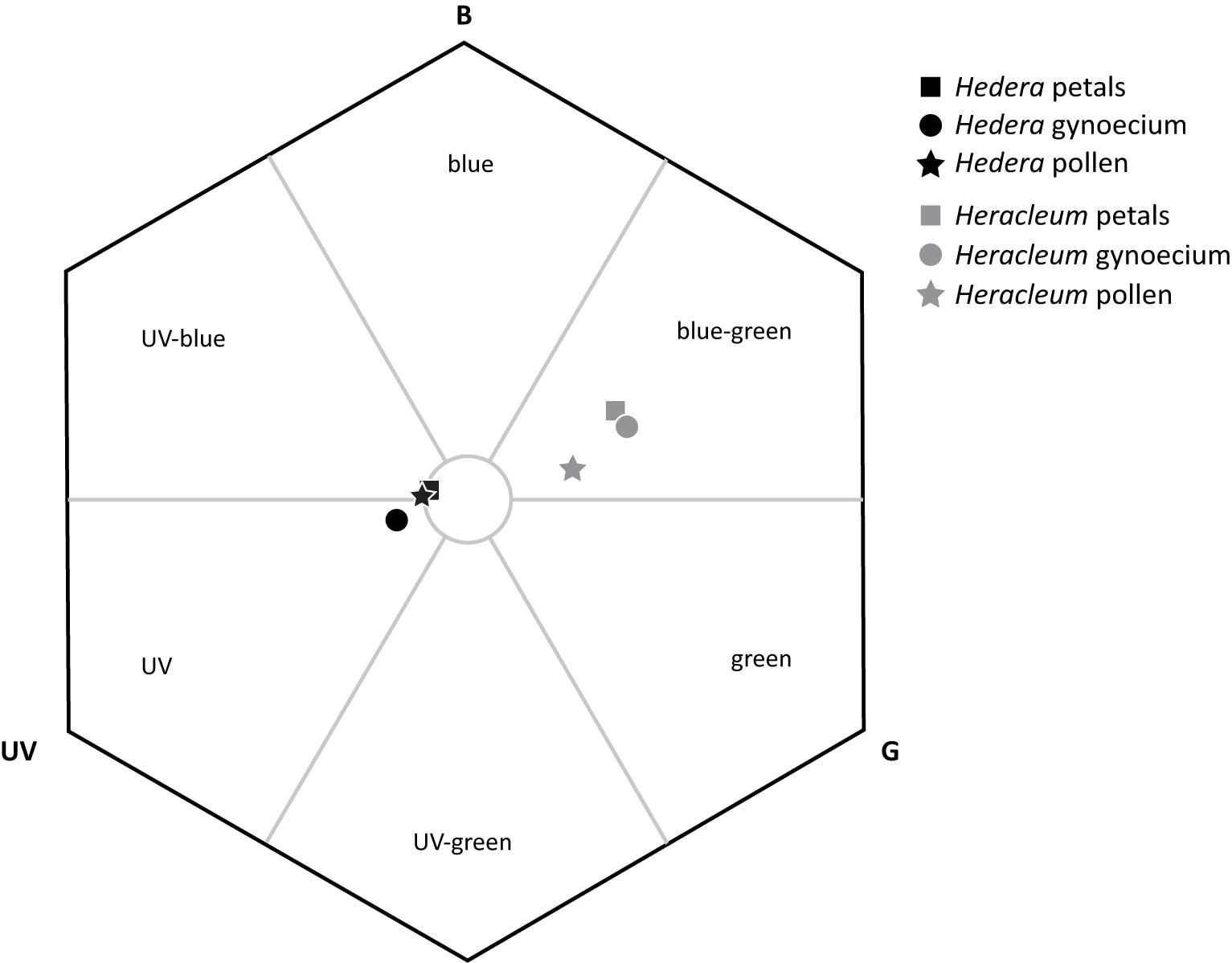
Figure 4. Spectral color analysis of Hedera helix and Heracleum sphondylium floral organs, provided in a color hexagon based on the visual spectrum of Vespula germanica (B, blue; G, green; UV, UV receptor). A circle with 0.1 units in radius is given to demonstrate the region of background colors.
Behavioral Experiments
Decoupled visual signals of H. sphondylium inflorescences were equally attractive as the empty control (Figure 5A,a), whereas decoupled olfactory signals of inflorescences attracted significantly more Dolichovespula wasps than the empty control (Figure 5A,b). The combined olfactory and visual signals were significantly more attractive for wasps than both the empty control (Figure 5A,c) and the decoupled olfactory signals of H. sphondylium inflorescences (Figure 5A,d).
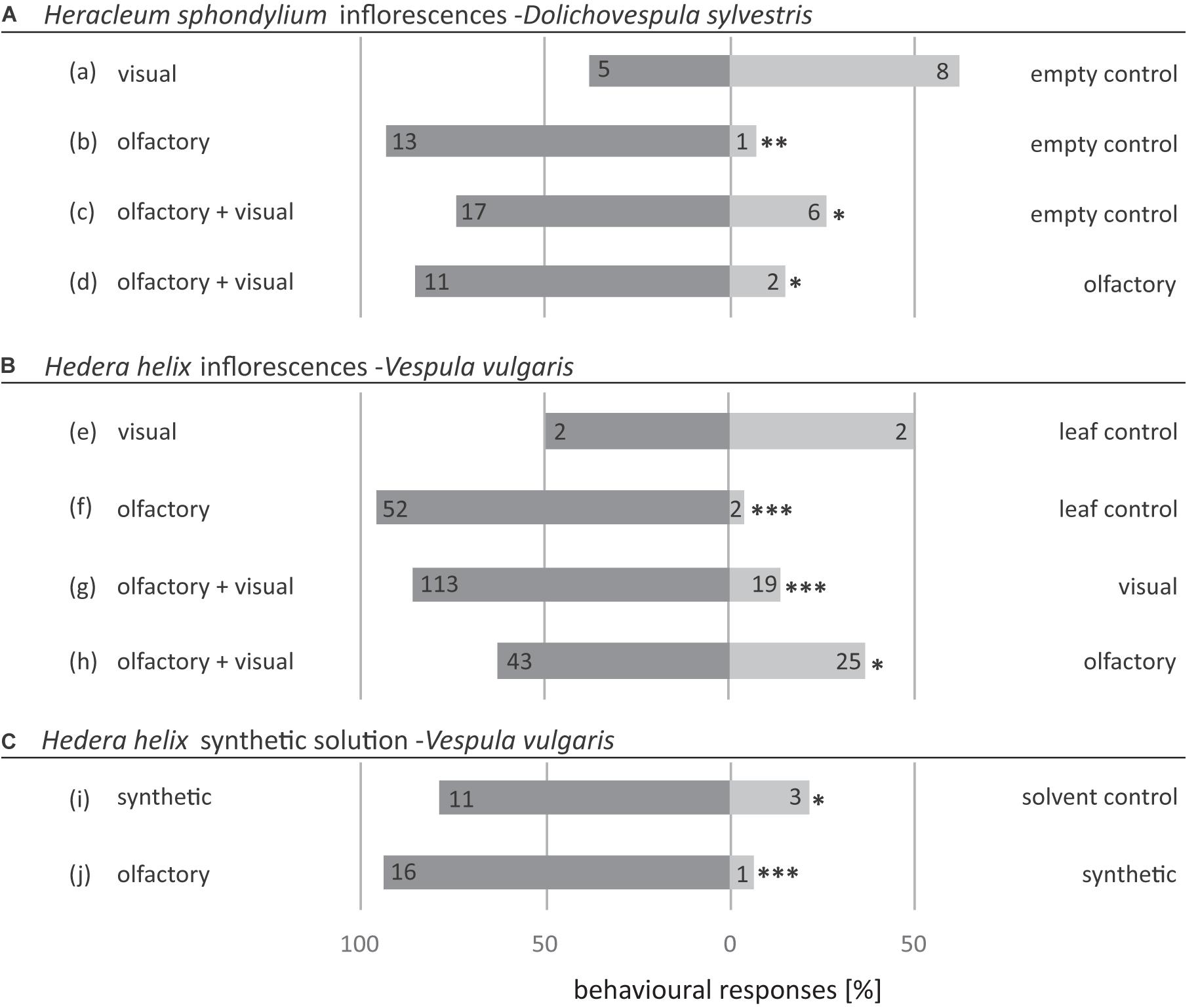
Figure 5. Attractiveness of (A) olfactory and visual cues of inflorescences of Hedera helix to Vespula germanica wasps, (B) synthetic Hedera helix scent samples to Vespula germanica wasps, and (C) olfactory and visual cues of inflorescences of Heracleum sphondylium to Dolichovespula sylvestris wasps. The synthetic solution consisted of EAD-active compounds of H. helix (Table 2). Non-flowering Hedera helix twigs were used as a leaf control (a,b). Mineral oil was used as a solvent control (e). Numbers inside the bars indicate absolute numbers of responding wasps (exact binomial test; *p < 0.05, **p < 0.01, ***p < 0.001).
Decoupled visual signals of the inflorescences and vegetative material of H. helix were only weakly attractive. Both attracted only two Vespula wasps when tested against each other (Figure 5B,e). This is in strong contrast to the decoupled olfactory inflorescence signals of H. helix, which attracted significantly more Vespula wasps when tested against decoupled olfactory signals of a vegetative control (Figure 5B,f). The combined olfactory and visual inflorescence signals were significantly more attractive than both decoupled visual (Figure 5B,g) and olfactory inflorescence (Figure 5B,h) signals.
The synthetic scent sample resembling the flower-specific compounds of H. helix was significantly more attractive than the solvent control (Figure 5C,i), but less attractive when compared with the scent emitted from the natural inflorescences of H. helix (Figure 5C,j).
Discussion
Our study shows that olfactory signals are key in attracting vespine wasps to the inflorescences of Hedera helix and Heracleum sphondylium. Both plant species release various compounds that elicit electrophysiological responses in vespine wasps. For H. helix, we additionally show that electrophysiologically active compounds are also behaviorally active, but to a lower extent than the natural scent. In both species, visual traits increase the attractiveness of olfactory signals, although they are not attractive on their own.
Our finding that olfactory floral signals are more important than visual ones for vespine wasps during their search for food plants is in agreement with several studies on the host location of different floral visitors (Hodgkison et al., 2007; Raguso, 2008b; Primante and Dötterl, 2010; Dötterl et al., 2011; Johnson et al., 2011; De Vega et al., 2014) and of wasps in specialized associations (e.g., Ayasse et al., 2003; Brodmann et al., 2008; Shuttleworth and Johnson, 2009a; Burger et al., 2017). In some other diurnal systems, however, visual signals are similar to (Milet-Pinheiro et al., 2012; Rachersberger et al., 2019) or more attractive than (Barragán-Fonseca et al., 2020) olfactory floral signals for attracting pollinators from a distance. Wasps are only rarely attracted by visual traits alone to wasp-favored flowers (but see Brodmann et al., 2012), which often appear in cryptic colors similar to the background vegetation (Shuttleworth and Johnson, 2012). The finding that wasps integrate olfactory and visual floral signals of the studied plants shows that they behave similarly when looking for nectar (present study) and proteins. To locate prey, olfactory cues often play a key role (Brodmann et al., 2008, 2009, 2012) and are assumed to elicit landing behaviors (Raveret Richter and Jeanne, 1985), whereas visual cues are used for area location (Collett, 1996; Raveret Richter, 2000). However, not all measured colors of the floral organs can be detected against the background vegetation based on our color modeling. In H. helix, only the color loci of the gynoecium but not the petals and pollen had a distance greater than 0.1 hexagon units from the center, which suggests that only this floral organ can be discriminated from green leaves (Dyer and Chittka, 2004). In contrast, all measured color loci of H. sphondylium flowers were clearly distinct from background colors.
Data on the floral scents of H. helix have recently been published (10 compounds; Vanderplanck et al., 2017), but not a single compound thereof is among those identified in the present study. Instead, Vanderplanck et al. (2017) list compounds such as cyclopentane and 1H-cyclopropa(a)naphthalene, which are typical contaminants of floral scent analyses. Furthermore, we are not aware of a previous study involving the analysis of the scent of H. sphondylium. Other species of Heracleum release several compounds, mainly terpenoids, that are also described in the present study (Borg-Karlson et al., 1993; Tollsten and Øvstedal, 1994; Tollsten et al., 1994). For example, Heracleum sibiricum, a species that also has vespine wasps among its generalist pollinators (Zych, 2002), emits linalool and (E)-linalool oxide furanoid, which are the main compounds detected in the present and previous studies (Borg-Karlson et al., 1993).
Most of the identified volatiles in the studied plants are widespread floral compounds known from plants with various pollination systems (Knudsen et al., 2006). Many of the compounds are also physiologically (Blight et al., 1997; Burger et al., 2013; Mas et al., 2018; Lukas et al., 2019) or behaviorally (Henning et al., 1992) active in honeybees but only few were known to be active in wasps before our study (but see Brodmann et al., 2008, 2012; Brown et al., 2015). As demonstrated by behavioral assays the compounds elicited also behavioral responses in wasps. Some EAD-active compounds of H. sphondylium are less common among the floral scents, especially the nitrogen-bearing 2-aminoacetophenone and its likely derivate m/z 120, 135, 92, 65, 163, 93 (Johnson et al., 2020). 2-Aminoacetophenone has been shown to attract various flies to bacteria (Kapsetaki et al., 2014), and its likely derivative is EAD-active in the scent of fly-pollinated Wurmbea flowers, a lily that exhibits a fecal odor (Johnson et al., 2020). Moreover, the scent of H. sphondylium is reminiscent of the smell of dung, possibly because of the presence of p-cresol. This compound occurs, as a major compound in various plants, especially in species with a sapromyiophilous pollination system that mimics dung (Kite et al., 1998; Jürgens et al., 2006, 2013; Knudsen et al., 2006).
We have not found compounds among those identified in H. helix or H. sphondylium that might be selective for wasps or that are known as typical vespine wasp attractants, such as heptyl butyrate, isobutanol, and acetic acid (Davis et al., 1969; Landolt, 1998; Day and Jeanne, 2001; Landolt et al., 2007; El-Sayed et al., 2009; Landolt and Zhang, 2016; Buteler et al., 2018), which possibly resemble the odors from rotten fruits. For example, the floral scent of Gomphocarpus physocarpus, an asclepiad pollinated by vespid wasps, is characterized by acetic acid as a main compound (Burger et al., 2017). In contrast, a broad range of widespread floral scent compounds (e.g., terpenes, aromatics) that show some overlaps with the scent bouquets of H. helix and H. sphondylium is described for pollination systems with pompilid wasps as the principle pollinators (Shuttleworth and Johnson, 2012). In these systems, nectar properties additionally play a key role to avoid non-wasp visitors (Shuttleworth and Johnson, 2009b); this is probably not the case in the above studied systems as H. helix and H. sphondylium are frequently visited by many different insects (Corbet, 1970; Sheppard, 1991; Metcalfe, 2005; Vezza et al., 2006; Zych, 2007; Jacobs et al., 2010). The floral scent bouquets of both plants include mainly typical floral scent components and are not dominated by green leaf volatiles as it is described for the wasp flowers Epipactis helleborine (Brodmann et al., 2009) and Scrophularia umbrosa (Brodmann et al., 2012) that mimic herbivore feeding to attract wasps as pollinators.
Instead, we conclude, that wasps seem to be attracted to the studied plants by floral scent compounds that are also physiologically and/or behaviorally active for other flower visitors, such as bees, moths, butterflies, and flies (Andersson, 2003; Dötterl and Vereecken, 2010; Johnson et al., 2020; The Pherobase, 2020). The release of such compounds might be the reason that H. helix and H. sphondylium are attractive to insects of various orders and families. We show here, for the first time, that those compounds are also involved in chemical communication between nectar-foraging vespine wasps and host plants with a generalist-type pollination system.
Data Availability Statement
The raw data supporting the conclusions of this article will be made available by the authors, without undue reservation.
Author Contributions
SD, MA, and HB designed the study. KL and HB performed the experiments. KL, SD, and HB analyzed the data. SD gave advise with regard to scent analyses. KL wrote the manuscript with contributions from SD, MA, and HB. All authors contributed to the article and approved the submitted version.
Funding
This work was supported by the Deutsche Forschungsgemeinschaft (DFG), Research Project BU 3051/1-2.
Conflict of Interest
The authors declare that the research was conducted in the absence of any commercial or financial relationships that could be construed as a potential conflict of interest.
Acknowledgments
We gratefully acknowledge C. Giovianni Galizia for valuable discussions, Roman Fuchs for his help with the electrophysiological experiments, Elisabeth Egger for finding a wasp nest in the Botanical Garden of Salzburg, and the gardeners of the Botanical Garden of Ulm for growing plants for the behavioral experiments. We also thank Theresa Jones for linguistic advice and two reviewers for valuable comments. All experiments comply with the current laws of the country in which they were performed: in this case, Germany and Austria.
References
Adams, R. P. (2007). Identification of Essential oil Components by Gas Chromatography/Mass Spectrometry, Vol. 456. Carol Stream, IL: Allured Publishing Corporation.
Akre, R. D., Greene, A., MacDonald, J. F., Landolt, P. J., and Davis, H. G. (1981). The Yellowjackets of America North of Mexico. US Department of Agriculture: Science and Education Administration. Agricultural Handbook Number 552. Washington DC: U.S. Government Printing Office.
Anderson, M., Gorley, R. N., and Clarke, R. K. (2008). Permanova+ for Primer: Guide to Software and Statistical Methods. Plymouth: PRIMER-E Limited.
Andersson, S. (2003). Antennal responses to floral scents in the butterflies Inachis io, Aglais urticae (Nymphalidae), and Gonepteryx rhamni (Pieridae). Chemoecology 13, 13–20. doi: 10.1007/s000490300001
Ayasse, M. (2006). “Floral scent and pollinator attraction in sexually deceptive orchids,” in Biology of Floral Scent, eds N. Dudareva and E. Pichersky (Boca Raton, FL: CRC Press), 233–256.
Ayasse, M., Schiestl, F. P., Paulus, H. F., Ibarra, F., and Francke, W. (2003). Pollinator attraction in a sexually deceptive orchid by means of unconventional chemicals. Proc. R. Soc. Lond. B Biol. Sci. 270, 517–522. doi: 10.1098/rspb.2002.2271
Balkenius, A., Rosèn, W., and Kelber, A. (2006). The relative importance of olfaction and vision in a diurnal and a nocturnal hawkmoth. J. Comp. Physiol. A 192, 431–437. doi: 10.1007/s00359-005-0081-6
Barragán-Fonseca, K. Y., Van Loon, J. J., Dicke, M., and Lucas-Barbosa, D. (2020). Use of visual and olfactory cues of flowers of two brassicaceous species by insect pollinators. Ecol. Entomol. 45, 45–55. doi: 10.1111/een.12775
Blight, M. M., Le Métayer, M., Delègue, M. H. P., Pickett, J. A., Marion-Poll, F., and Wadhams, L. J. (1997). Identification of floral volatiles involved in recognition of oilseed rape flowers, Brassica napus by honeybees, Apis mellifera. J. Chem. Ecol. 23, 1715–1727. doi: 10.1023/b:joec.0000006446.21160.c1
Borg-Karlson, A. K., Valterová, I., and Nilsson, L. A. (1993). Volatile compounds from flowers of six species in the family Apiaceae: bouquets for different pollinators? Phytochemistry 35, 111–119. doi: 10.1016/s0031-9422(00)90518-1
Brodmann, J., Emer, D., and Ayasse, M. (2012). Pollinator attraction of the wasp-flower Scrophularia umbrosa (Scrophulariaceae). Plant Biol. 14, 500–505. doi: 10.1111/j.1438-8677.2011.00525.x
Brodmann, J., Twele, R., Francke, W., Hölzler, G., Zhang, Q. H., and Ayasse, M. (2008). Orchids mimic green-leaf volatiles to attract prey-hunting wasps for pollination. Curr. Biol. 18, 740–744. doi: 10.1016/j.cub.2008.04.040
Brodmann, J., Twele, R., Francke, W., Yi-Bo, L., Xi-qiang, S., and Ayasse, M. (2009). Orchid mimics honey bee alarm pheromone in order to attract hornets for pollination. Curr. Biol. 19, 1368–1372. doi: 10.1016/j.cub.2009.06.067
Brown, R. L., El-Sayed, A. M., Unelius, C. R., Beggs, J. R., and Suckling, D. M. (2015). Invasive Vespula wasps utilize kairomones to exploit honeydew produced by sooty scale insects, Ultracoelostoma. J. Chem. Ecol. 41, 1018–1027. doi: 10.1007/s10886-015-0635-1
Burger, H., Ayasse, M., Dötterl, S., Kreissl, S., and Galizia, C. G. (2013). Perception of floral volatiles involved in host-plant finding behaviour: comparison of a bee specialist and generalist. J. Comp. Physiol. A 199, 751–761. doi: 10.1007/s00359-013-0835-5
Burger, H., Dötterl, S., and Ayasse, M. (2010). Host-plant finding and recognition by visual and olfactory floral cues in an oligolectic bee. Funct. Ecol. 24, 1234–1240. doi: 10.1111/j.1365-2435.2010.01744.x
Burger, H., Dötterl, S., Häberlein, C. M., Schulz, S., and Ayasse, M. (2011). An arthropod deterrent attracts specialised bees to their host plants. Oecologia 168, 727–736. doi: 10.1007/s00442-011-2136-4
Burger, H., Jürgens, A., Ayasse, M., and Johnson, S. D. (2017). Floral signals and filters in a wasp- and a bee-pollinated Gomphocarpus species (Apocynaceae: Asclepiadeae). Flora 232, 83–91. doi: 10.1016/j.flora.2017.05.009
Buteler, M., Fernandez, P., Stadler, T., Weaver, D. K., Yossen, B., and Lozada, M. (2018). Heptyl butyrate, a putative pheromone involved in social communication of Vespula germanica wasps. Insectes Soc. 65, 95–101. doi: 10.1007/s00040-017-0591-6
Chittka, L. (1992). The colour hexagon: a chromaticity diagram based on photoreceptor excitations as a generalized representation of colour opponency. J. Comp. Physiol. A 170, 533–543.
Chittka, L., Shmida, A., Troje, N., and Menzel, R. (1994). Ultraviolet as a component of flower reflections, and the colour perception of hymenoptera. Vision Res. 34, 1489–1508. doi: 10.1016/0042-6989(94)90151-1
Collett, T. S. (1996). Insect navigation en route to the goal: multiple strategies for the use of landmarks. J. Exp. Biol. 199, 227–235.
Corbet, S. A. (1970). Insects on hog weed flowers: a suggestion for a student project. J. Biol. Educ. 4, 133–143. doi: 10.1080/00219266.1970.9653628
Davis, H. G., Eddy, G. W., McGovern, T. P., and Beroza, M. (1969). Heptyl butyrate, a new synthetic attractant for yellow jackets. J. Econ. Entomol. 62:1245.
Day, S. E., and Jeanne, R. L. (2001). Food volatiles as attractants for yellowjackets (Hymenoptera: Vespidae). Environ. Entomol. 30, 157–165. doi: 10.1603/0046-225x-30.2.157
De Vega, C., Herrerdobsoa, C. M., and Dötterl, S. (2014). Floral volatiles play a key role in specialized ant pollination. Perspect. Plant Ecol. Evol. Syst. 16, 32–42. doi: 10.1016/j.ppees.2013.11.002
Dobson, H. E. M. (2006). “Relationship between floral fragrance composition and type of pollinator,” in Biology of Floral Scent, eds N. A. Dudareva and E. Pichersky (Boca Raton, FL: CRC Press), 146–198.
Dötterl, S., Füssel, U., Jürgens, A., and Aas, G. (2005). 1, 4-Dimethoxybenzene, a floral scent compound in willows that attracts an oligolectic bee. J. Chem. Ecol. 31, 2993–2998. doi: 10.1007/s10886-005-9152-y
Dötterl, S., Milchreit, K., and Schäffler, I. (2011). Behavioural plasticity and sex differences in host finding of a specialized bee species. J. Comp. Physiol. A 197, 1119–1126. doi: 10.1007/s00359-011-0673-2
Dötterl, S., and Vereecken, N. J. (2010). The chemical ecology and evolution of bee–flower interactions: a review and perspectives. Can. J. Zool. 88, 668–697. doi: 10.1139/z10-031
Dyer, A. G., and Chittka, L. (2004). Biological significance of distinguishing between similar colours in spectrally variable illumination: bumblebees (Bombus terrestris) as a case study. J. Comp. Physiol. A Neuroethol. Sens. Neural Behav. Physiol. 190, 105–114. doi: 10.1007/s00359-003-0475-2
El-Sayed, A. M., Manning, L. A., Unelius, C. R., Park, K. C., Stringer, L. D., White, N., et al. (2009). Attraction and antennal response of the common wasp, Vespula vulgaris (L.), to selected synthetic chemicals in New Zealand beech forests. Pest Manag. Sci. 65, 975–981. doi: 10.1002/ps.1782
Etl, F., Berger, A., Weber, A., Schönenberger, J., and Dötterl, S. (2016). Nocturnal plant bugs use cis-jasmone to locate inflorescences of an Araceae as feeding and mating site (Heteroptera: Miridae). J. Chem. Ecol. 42, 300–304. doi: 10.1007/s10886-016-0688-9
Fandino, R. A., Haverkamp, A., Bisch-Knaden, S., Zhang, J., Bucks, S., Nguyen, T. A. T., et al. (2019). Mutagenesis of odorant coreceptor Orco fully disrupts foraging but not oviposition behaviors in the hawkmoth Manduca sexta. Proc. Natl. Acad. Sci. U.S.A. 116, 15677–15685. doi: 10.1073/pnas.1902089116
Fateryga, A. V. (2010). Trophic relations between vespid wasps (Hymenoptera, Vespidae) and flowering plants in the Crimea. Entomol. Rev. 90, 698–705. doi: 10.1134/s0013873810060047
Grison-Pigé, L., Bessière, J. M., and Hossaert-McKey, M. (2002). Specific attraction of fig-pollinating wasps: role of volatile compounds released by tropical figs. J. Chem. Ecol. 28, 283–295.
Heiduk, A., Kong, H., Brake, I., von Tschirnhaus, M., Tolasch, T., Tröger, A., et al. (2015). Deceptive Ceropegia dolichophylla fools its kleptoparasitic fly pollinators with exceptional floral scent. Front. Ecol. Evol. 3:66. doi: 10.3389/fevo.2015.00066
Henning, J. A., Peng, Y. S., Montague, M. A., and Teuber, L. R. (1992). Honey bee (Hymenoptera: Apidae) behavioral response to primary alfalfa (Rosales: Fabaceae) floral volatiles. J. Econ. Entomol. 85, 233–239. doi: 10.1093/jee/85.1.233
Hodgkison, R., Ayasse, M., Kalko, E. K. V., Häberlein, C., Schulz, S., Mustapha, W. A. W., et al. (2007). Chemical ecology of fruit bat foraging behavior in relation to the fruit odors of two species of paleotropical bat-dispersed figs (Ficus hispida and Ficus scortechinii). J. Chem. Ecol. 33, 2097–2110. doi: 10.1007/s10886-007-9367-1
Jacobs, J. H., Clark, S. J., Denholm, I., Goulson, D., Stoate, C., and Osborne, J. L. (2010). Pollinator effectiveness and fruit set in common ivy, Hedera helix (Araliaceae). Arthropod Plant Interact. 4, 19–28. doi: 10.1007/s11829-009-9080-9
Johnson, S. D. (2005). Specialized pollination by spider-hunting wasps in the African orchid Disa sankeyi. Plant Syst. Evol. 251, 153–160. doi: 10.1007/s00606-004-0234-z
Johnson, S. D., Burgoyne, P. M., Harder, L. D., and Dötterl, S. (2011). Mammal pollinators lured by the scent of a parasitic plant. Proc. R. Soc. B Biol. Sci. 278, 2303–2310. doi: 10.1098/rspb.2010.2175
Johnson, S. D., Sivechurran, J., Doarsamy, S., and Shuttleworth, A. (2020). Dung mimicry: the function of volatile emissions and corolla patterning in fly-pollinated Wurmbea flowers. New Phytol. doi: 10.1111/nph.16791
Jürgens, A., Dötterl, S., and Meve, U. (2006). The chemical nature of fetid floral odours in stapeliads (Apocynaceae-Asclepiadoideae-Ceropegieae). New Phytol. 172, 452–468. doi: 10.1111/j.1469-8137.2006.01845.x
Jürgens, A., Wee, S. L., Shuttleworth, A., and Johnson, S. D. (2013). Chemical mimicry of insect oviposition sites: a global analysis of convergence in angiosperms. Ecol. Lett. 16, 1157–1167. doi: 10.1111/ele.12152
Kapsetaki, S. E., Tzelepis, I., Avgousti, K., Livadaras, I., Garantonakis, N., Varikou, K., et al. (2014). The bacterial metabolite 2-aminoacetophenone promotes association of pathogenic bacteria with flies. Nat. Commun. 5:4401.
Kite, G. C., Hetterscheid, W. L., Lewis, M. J., Boyce, P. C., Ollerton, J., Cocklin, E., et al. (1998). “Inflorescence odours and pollinators of Arum and Amorphophallus (Araceae),” in Reproductive Biology in Systematics, Conservation and Economic Botany, eds S. J. Owens and P. J. Rudall (Kew: Royal Botanic Gardens), 295–315.
Knudsen, J. T., Eriksson, R., Gershenzon, J., and Ståhl, B. (2006). Diversity and distribution of floral scent. Bot. Rev. 72:1. doi: 10.1663/0006-8101(2006)72%5B1:dadofs%5D2.0.co;2
Landolt, P., and Zhang, Q. H. (2016). Discovery and development of chemical attractants used to trap pestiferous social wasps (Hymenoptera: Vespidae). J. Chem. Ecol. 42, 655–665. doi: 10.1007/s10886-016-0721-z
Landolt, P. J. (1998). Chemical attractants for trapping yellowjackets Vespula germanica and Vespula pensylvanica (Hymenoptera: Vespidae). Environ. Entomol. 27, 1229–1234. doi: 10.1093/ee/27.5.1229
Landolt, P. J., Toth, M., and Josvai, J. (2007). First European report of social wasps trapped in response to acetic acid, isobutanol, 2-methyl-2-propanol and heptyl butyrate in tests conducted in Hungary. Bull. Insectol. 60, 7–11.
Lukas, K., Harig, T., Schulz, S., Hadersdorfer, J., and Dötterl, S. (2019). Flowers of European pear release common and uncommon volatiles that can be detected by honey bee pollinators. Chemoecology 29, 211–223. doi: 10.1007/s00049-019-00289-x
Maia, R., Eliason, C. M., Bitton, P. P., Doucet, S. M., and Shawkey, M. D. (2013). pavo: an R package for the analysis, visualization and organization of spectral data. Methods Ecol. Evol. 4, 906–913.
Mas, F., Harper, A., Horner, R., Welsh, T., Jaksons, P., and Suckling, D. M. (2018). The importance of key floral bioactive compounds to honey bees for the detection and attraction of hybrid vegetable crops and increased seed yield. J. Sci. Food Agric. 98, 4445–4453. doi: 10.1002/jsfa.8967
Milet-Pinheiro, P., Ayasse, M., Schlindwein, C., Dobson, H. E. M., and Dötterl, S. (2012). Host location by visual and olfactory floral cues in an oligolectic bee: innate and learned behaviour. Behav. Ecol. 23, 531–538. doi: 10.1093/beheco/arr219
Narbona, E., and Dirzo, R. (2010). A reassessment of the function of floral nectar in Croton suberosus (Euphorbiaceae): a reward for plant defenders and pollinators. Am. J. Bot. 97, 672–679. doi: 10.3732/ajb.0900259
Nazarov, V. V. (1995). Pollination of Steveniella satyrioides (Orchidaceae) by wasps (Hymenoptera, Vespoidea) in the Crimea. Lindleyana 10, 109–114.
Oliveira, P. E., Tomé, C. E. R., Torezan-Silingardi, H. M., Dötterl, S., Silberbauer-Gottsberger, I., and Gottsberger, G. (2017). Differential pollination modes between distant populations of Unonopsis guatterioides (Annonaceae) in Minas Gerais and Amazonas, Brazil. Flora 232, 39–46. doi: 10.1016/j.flora.2016.07.014
Ollerton, J. (2017). Pollinator diversity: distribution, ecological function, and conservation. Annu. Rev. Ecol. Evol. Syst. 48, 353–376. doi: 10.1146/annurev-ecolsys-110316-022919
Ollerton, J., Johnson, S. D., Cranmer, L., and Kellie, S. A. M. (2003). The pollination ecology of an assemblage of grassland asclepiads in South Africa. Ann. Bot. 92, 807–834. doi: 10.1093/aob/mcg206
Ollerton, J., Killick, A., Lamborn, E., Watts, S., and Whiston, M. (2007). Multiple meanings and modes: on the many ways to be a generalist flower. Taxon 56, 717–728. doi: 10.2307/25065855
Ollerton, J., Winfree, R., and Tarrant, S. (2011). How many flowering plants are pollinated by animals? Oikos 120, 321–326. doi: 10.1111/j.1600-0706.2010.18644.x
Ômura, H., and Honda, K. (2005). Priority of color over scent during flower visitation by adult Vanessa indica butterflies. Oecologia 142, 588–596. doi: 10.1007/s00442-004-1761-6
Peitsch, D., Fietz, A., Hertel, H., de Souza, J., Ventura, D. F., and Menzel, R. (1992). The spectral input systems of hymenopteran insects and their receptor-based colour vision. J. Comp. Physiol. A 170, 23–40. doi: 10.1007/bf00190398
Primante, C., and Dötterl, S. (2010). A syrphid fly uses olfactory cues to find a non-yellow flower. J. Chem. Ecol. 36, 1207–1210. doi: 10.1007/s10886-010-9871-6
Rachersberger, M., Cordeiro, G. D., Schäffler, I., and Dötterl, S. (2019). Honeybee pollinators use visual and floral scent cues to find apple (Malus domestica) flowers. J. Agric. Food Chem. 67, 13221–13227. doi: 10.1021/acs.jafc.9b06446
Raguso, R. A. (2008a). Start making scents: the challenge of integrating chemistry into pollination ecology. Entomol. Exp. Appl. 128, 196–207. doi: 10.1111/j.1570-7458.2008.00683.x
Raguso, R. A. (2008b). Wake up and smell the roses: the ecology and evolution of floral scent. Annu. Rev. Ecol. Evol. Syst. 39, 549–569. doi: 10.1146/annurev.ecolsys.38.091206.095601
Raveret Richter, M. A. (2000). Social wasp (Hymenoptera: Vespidae) foraging behavior. Annu. Rev. Entomol. 45, 121–150. doi: 10.1146/annurev.ento.45.1.121
Raveret Richter, M. A., and Jeanne, R. L. (1985). Predatory behavior of Polybia sericea (Olivier), a tropical social wasp (Hymenoptera: Vespidae). Behav. Ecol. Sociobiol. 16, 165–170. doi: 10.1007/bf00295151
Schäffler, I., Steiner, K. E., Haid, M., Van Berkel, S. S., Gerlach, G., Johnson, S. D., et al. (2015). Diacetin, a reliable cue and private communication channel in a specialized pollination system. Sci. Rep. 5:12779.
Schiestl, F. P. (2005). On the success of a swindle: pollination by deception in orchids. Naturwissenschaften 92, 255–264. doi: 10.1007/s00114-005-0636-y
Schiestl, F. P., Peakall, R., Mant, J. G., Ibarra, F., Schulz, C., Franke, S., et al. (2003). The chemistry of sexual deception in an orchid-wasp pollination system. Science 302, 437–438. doi: 10.1126/science.1087835
Shuttleworth, A., and Johnson, S. D. (2009a). A key role for floral scent in a wasp-pollination system in Eucomis (Hyacinthaceae). Ann. Bot. 103, 715–725. doi: 10.1093/aob/mcn261
Shuttleworth, A., and Johnson, S. D. (2009b). The importance of scent and nectar filters in a specialized wasp-pollination system. Funct. Ecol. 23, 931–940. doi: 10.1111/j.1365-2435.2009.01573.x
Shuttleworth, A., and Johnson, S. D. (2012). The Hemipepsis wasp-pollination system in South Africa: a comparative analysis of trait convergence in a highly specialized plant guild. Bot. J. Linn. Soc. 168, 278–299. doi: 10.1111/j.1095-8339.2012.01216.x
Song, Q., Yang, D., Zhang, G., and Yang, C. (2001). Volatiles from Ficus hispida and their attractiveness to fig wasps. J. Chem. Ecol. 27, 1929–1942.
The Pherobase (2020). Database of Pheromones and Semiochemicals. Available online at: http://www.pherobase.com (accessed April 29, 2020).
Tollsten, L., Knudsen, J. T., and Bergström, L. G. (1994). Floral scent in generalistic Angelica (Apiaceae)—an adaptive character? Biochem. Syst. Ecol. 22, 161–169. doi: 10.1016/0305-1978(94)90006-x
Tollsten, L., and Øvstedal, D. O. (1994). Differentiation in floral scent chemistry among populations of Conopodium majus (Apiaceae). Nord. J. Bot. 14, 361–368. doi: 10.1111/j.1756-1051.1994.tb00619.x
Vanderplanck, M., Vereecken, N. J., Grumiau, L., Esposito, F., Lognay, G., Wattiez, R., et al. (2017). The importance of pollen chemistry in evolutionary host shifts of bees. Sci. Rep. 7:43058.
Vezza, M., Nepi, M., Guarnieri, M., Artese, D., Rascio, N., and Pacini, E. (2006). Ivy (Hedera helix L.) flower nectar and nectary ecophysiology. Int. J. Plant Sci. 167, 519–527. doi: 10.1086/501140
Wiemer, A. P., Sérsic, A. N., Marino, S., Simões, A. O., and Cocucci, A. A. (2012). Functional morphology and wasp pollination of two South American asclepiads (Asclepiadoideae–Apocynaceae). Ann. Bot. 109, 77–93. doi: 10.1093/aob/mcr268
Wyszecki, G., and Stiles, W. S. (1982). Color Science: Concepts and Methods, Quantitative Data and Formulae, 2nd Edn. New York, NY: Wiley, 130–175.
Zych, M. (2002). Pollination biology of Heracleum sphondylium L. (Apiaceae): the advantages of being white and compact. Acta Soc. Bot. Pol. 71, 163–170. doi: 10.5586/asbp.2002.019
Zych, M. (2007). On flower visitors and true pollinators: the case of protandrous Heracleum sphondylium L. (Apiaceae). Plant Syst. Evol. 263, 159–179. doi: 10.1007/s00606-006-0493-y
Zych, M., Junker, R. R., Nepi, M., Stpiczyńska, M., Stolarska, B., and Roguz, K. (2019). Spatiotemporal variation in the pollination systems of a supergeneralist plant: is Angelica sylvestris (Apiaceae) locally adapted to its most effective pollinators? Ann. Bot. 123, 415–428. doi: 10.1093/aob/mcy140
Keywords: vespine wasps, nectar-seeking behavior, generalist flowers, floral color, floral scent
Citation: Lukas K, Dötterl S, Ayasse M and Burger H (2020) Olfactory and Visual Floral Signals of Hedera helix and Heracleum sphondylium Involved in Host Finding by Nectar-Foraging Social Wasps. Front. Ecol. Evol. 8:571454. doi: 10.3389/fevo.2020.571454
Received: 10 June 2020; Accepted: 02 October 2020;
Published: 30 October 2020.
Edited by:
Florian Paul Schiestl, University of Zurich, SwitzerlandReviewed by:
Adam Shuttleworth, University of KwaZulu-Natal, South AfricaAlexander Haverkamp, Wageningen University & Research, Netherlands
Copyright © 2020 Lukas, Dötterl, Ayasse and Burger. This is an open-access article distributed under the terms of the Creative Commons Attribution License (CC BY). The use, distribution or reproduction in other forums is permitted, provided the original author(s) and the copyright owner(s) are credited and that the original publication in this journal is cited, in accordance with accepted academic practice. No use, distribution or reproduction is permitted which does not comply with these terms.
*Correspondence: Hannah Burger, info@hannah-burger.de