- Department of Evolutionary Animal Ecology, University of Bayreuth, Bayreuth, Germany
Chemical communication via pheromones is considered the oldest and most widespread form of communication in nature. However, the way that the enormous diversity of species-specific pheromones evolved is still of debate. One possible process driving pheromone evolution is the mate-finding and dispersal behavior, as long-distance mate-finding requires highly volatile compounds. In contrast, less volatile compounds might be sufficient attractants in species that search for mates within proximity. In the parasitoid wasp genus Leptopilina, the composition of species-specific sex pheromones ranges from highly volatile iridoid compounds through combinations of iridoids with low volatile cuticular hydrocarbons (CHCs) to only CHCs. To study the selective forces shaping the composition of sex pheromones in Leptopilina, we examined the dispersal behavior, i.e., the proportion of male and female wasps dispersing after emergence, in four species with known sex pheromone compositions. If males and females disperse immediately, long-range mate attraction might become necessary, favoring volatile iridoids over CHCs. If mating occurs directly on the host patch, short-range mate attraction by low volatile CHCs might suffice. Our analyses have revealed that the dispersal behavior of Leptopilina males and females after emergence does indeed differ between species with differently volatile sex pheromones. Specifically, males of species with iridoid sex pheromones start to disperse immediately before their females’ emergence, whereas males of species with CHC sex pheromones delay dispersal until their conspecific females emerge. While the differences in female dispersal behavior turned out to be species-specific, differences in male dispersal correlated with the volatility of female-produced sex pheromones of each species. This study significantly contributes to our understanding of the evolution of sex pheromones by differences in dispersal behavior.
Introduction
Semiochemicals that mediate a variety of intra- and interspecific communication such as the attraction and finding of mating partners, hosts, or prey, or the deterrence of enemies, are ubiquitous in nature and consequently show a remarkable diversity (Wyatt, 2014). The thousands of chemicals transmitting information between organisms belong to various compound classes (see, e.g., El-Sayed, 2018 at http://www.pherobase.com, which lists >3500 semiochemical compounds), are used alone or in combination, and range from small, light, volatile molecules to large, non-volatile compounds with higher molecular weights. Thus, these chemical messengers have a variety of different physicochemical properties (e.g., solubility in water for sex pheromones in fish, volatility in airborne signals, Mollo et al., 2017), although the prerequisites of semiochemicals should be similar for each form and function of chemical communication. Ultimately, they have to be emitted and delivered at the right concentration in order to be perceived and to elicit a response.
Despite having similar functions, the effective range of pheromones and their detection is often markedly different. If signals merely have to be detected by organisms in close vicinity to each other, non- or semi-volatile compounds such as long-chained cuticular hydrocarbons (CHCs) can suffice as contact pheromones (Blomquist and Bagnères, 2010), whereas long-range pheromones need to be volatile, to vaporize rapidly, and to spread widely in order to inform conspecifics over greater distances (alarm pheromones: e.g., Fujiwara-Tsujii et al., 2006; sex pheromones: e.g., Allison and Cardé, 2016; aggregation pheromones: e.g., Gries et al., 2015). For example, sex pheromones can be expected to be volatile, if mates need to be attracted and/or found over long distances, whereas non-volatile compounds are sufficient to elicit courtship at close range once the mating partner has been found. Because of the necessity for species-specificity in mate-finding, the compounds used for sexual communication are extremely diverse and show large variations in composition, even among closely related species (Symonds and Elgar, 2008).
The evolution of pheromone diversity is still little understood. Up to date, studies concerning differences in pheromone compositions between closely related species have focused on phylogenetic comparisons and genetic analyses with explanations such as large, saltational shifts in pheromone evolution during speciation or differential gene regulation leading to changes between species in enzymatic pathways generating the pheromone (e.g., Lassance et al., 2010, see also Symonds and Elgar, 2008). Another explanation for the chemical composition of pheromones is provided by the precursor hypothesis, which states that compounds used for communication evolve from compounds previously fulfilling non-communicative purposes in the same species (Wyatt, 2010; Bradbury and Vehrencamp, 2011; Steiger et al., 2011; Stökl and Steiger, 2017). Examples are hormones that are excreted in urine and that evolved as sex pheromones in females of the goldfish and Atlantic salmon (Stacey and Sorensen, 2009), or defensive secretions that evolved as alarm pheromones (Löfqvist, 1976), but also as sex pheromones (Boppré, 1986; Ruther et al., 2001; Geiselhardt et al., 2008) or aggregation pheromones (Wheeler and Cardé, 2013). However, research is limited with regard to the degree to which the evolution of the impressive variety of sex pheromone compositions is driven by ecological or life-history traits and behavior. One important factor that has so far been neglected in explaining this diversity is the dispersal behavior of organisms during mate-finding, a characteristic that might be closely correlated with the volatility of pheromonal compounds. As the volatility of sex pheromones will restrict communication via these chemicals to a certain distance within which the compounds can be perceived, it consequently also restricts dispersal distances. Therefore, dispersal behavior might be a life history trait that has shaped pheromone composition and its volatility and physicochemical properties.
Ideally, this hypothesis can be tested by comparing the dispersal behavior of closely-related species with known sex pheromones of different volatilities. Such an example is found in the genus Leptopilina, which are parasitic wasps attacking Drosophila larvae (Carton et al., 1986) and which comprises several closely related species with sex pheromones of different volatilities (Weiss et al., 2013, 2015; Pfeiffer et al., 2018; Böttinger et al., 2019; Böttinger et al. submitted; unpublished). Although all Leptopilina species studied until now produce iridoid compounds and CHCs, an enormous variation in their sex pheromone compositions has been found. Leptopilina heterotoma (Weiss et al., 2013) and Leptopilina japonica (Böttinger et al., 2019) use solely their species-specific volatile iridoid compounds as their female sex pheromones, whereas combinations of iridoids and non-volatile CHCs (Leptopilina boulardi, Weiss et al., 2015) or only CHCs serve as sex pheromones in several species such as Leptopilina clavipes (Pfeiffer et al., 2018), Leptopilina guineaensis (unpublished), Leptopilina pacifica (Böttinger et al. submitted), Leptopilina ryukyuensis (Böttinger et al., 2019), and Leptopilina victoriae (Weiss et al., 2015). The CHCs used as sex pheromones in the latter species consist of methyl-branched alkanes and mono- or di-unsaturated alkenes with chain lengths ranging from 27 to 37 carbons (with the exception of some trace compounds; Weiss et al., 2015; Pfeiffer et al., 2018; Böttinger et al., 2019; Böttinger et al. submitted; unpublished) and are thus of low volatility. Consequently, Leptopilina sex pheromones consisting of iridoids can be perceived over long distances, whereas those consisting of CHCs can only be perceived in close vicinity of the releasing individual. Wasps of this genus are quasi-gregarious (van den Assem et al., 1980), as each of them develops solitarily in separate Drosophila larvae, which are however mostly clumped together. Therefore, male wasps searching for mates can either stay on their natal host patch to wait for conspecific females to emerge, as males generally emerge before females (Carton et al., 1986), or can disperse to mate with females from other host patches (Fauvergue et al., 1999). Accordingly, female wasps can disperse directly after emergence or mate on their host patch before they disperse to search for hosts (Hardy and Godfray, 1990). To find their mating partners after leaving their natal host patch, wasps could either follow the long-range pheromones of their prospective partner or use host-patch-derived volatiles as attractants, because host patches are probably optimal places for mate encounters. They can subsequently use short-range pheromones for mate recognition. We propose the following two hypotheses: If males and females of Leptopilina disperse unmated immediately after emergence, long-range mate attraction might become necessary, favoring sex pheromones containing volatile iridoids. If mating occurs directly on the host patch and dispersal is delayed, short-range mate attraction by low volatile CHCs could be sufficient.
In this study, we wished to tackle these hypotheses by comparing the dispersal behaviors of male and female Leptopilina wasps of four closely related species with differently volatile sex pheromones (highly volatile iridoids: L. heterotoma and L. japonica, low volatile CHCs: L. pacifica and L. ryukyuensis). For L. heterotoma (volatile iridoid sex pheromone) and L. boulardi (iridoids with CHCs as female sex pheromone) it is known that males and females disperse actively by flight from their natal patches shortly after emergence at a similar rate over several meters (Fauvergue et al., 1999). In field releases marked female L. heterotoma wasps dispersed upwind and were caught foraging at baits up to 18 m away from their point of release (Papaj and Vet, 1990). However, nothing is known about dispersal behaviors of species with non-volatile sex pheromones, and about the way that dispersal can shape the evolution of sex pheromone diversity. We therefore tested (1) whether males and females of species that use highly volatile iridoids in the female sex-pheromone disperse earlier or at a higher rate than species that use low volatile CHCs as sex pheromones, (2) whether dispersal of males and females is delayed or occurs to a lesser extent in species that use very low volatile CHCs as sex pheromones and in which no long-range attraction is possible, (3) the odor sources to which the wasps are attracted, as they might explain the reason for the dispersal of wasps.
Materials and Methods
Species
The wasp strain of L. heterotoma is a long established lab strain that was originally collected in Leiden, Netherlands. The strain of L. japonica (subspecies L. j. japonica, Novković et al., 2011) was collected in Tokyo, Japan in 2006, whereas L. ryukyuensis and L. pacifica were collected on Iriomote-jima, Japan in 2006 and 2011, respectively. The Japanese species were kindly provided by Prof. K. T. Kimura. Wasps of the species L. heterotoma, L. japonica, and L. ryukyuensis were reared on larvae of Drosophila simulans, whereas L. pacifica wasps were reared on Drosophila virilis larvae. Wasps and flies were kept in a climate- and light-controlled room (Zettner; Bayreuth, Germany) at 25°C and ∼60% humidity and under a 16:8 h L:D cycle. About 30 flies of both sexes were placed into a jar containing fresh corn-based diet as the host patch medium (for ingredients, see Böttinger et al., 2019) and removed after 48 h. Subsequently, about 10 mated females of either L. heterotoma, L. japonica, L. pacifica, or L. ryukyuensis were placed into the jar to parasitize their host fly larvae. To obtain unmated and naïve wasps of a defined sex and age for the experiments, parasitized pupae were singly isolated prior to eclosion into 1.5 ml microcentrifuge tubes. Filter papers (5 mm diameter) with a 50:50 honey water solution were added, so that individual wasps could feed ad libitum. Mated females were obtained by placing a 2- to 6-day-old male wasp in a microcentrifuge tube containing a freshly hatched female wasp and leaving them together for approximately 12 h.
Dispersal Experiment
In this experiment, we investigated the dispersal of wasps from their place of emergence. Dispersal is hereby defined as the leaving of the natal patch. Flies of D. simulans and D. virilis were allowed to lay eggs for 48 h on the host patch medium in small jars (70 × 40 mm height and diameter). Subsequently, 10 female wasps of either L. heterotoma, L. japonica, L. pacifica, or L. ryukyuensis were placed in the jar for 48 h to parasitize the Drosophila larvae. The jars were then transferred to transparent plastic boxes (size ca. 155 × 100 mm, 125 mm height). A micropipette tip (200 μl) was inserted into each jar lid through a hole (3 mm diameter) with the small tip end directed outwards. Previously, the small tip end had been cut and its opening diameter thus enlarged, so that the wasps once they emerged could climb out and exit the jars via these tips but could not return into the original jar. Each jar was checked three times daily (at 9 am, 3 pm, 9 pm), and the number and the sex of the wasps in the jar as well as outside the jar in the transparent box was recorded. Dispersed wasps were then removed from the transparent plastic box. The experiment was conducted 30 times per species and lasted 126 h after the emergence of the first wasp in each jar.
Attraction Olfactometer Experiment
To assess the odor sources that can mediate mate-finding, other than a long-range sex pheromone, attraction assays were performed with a Y-tube olfactometer in which wasps were allowed to decide between two presented odors. The Y-tube was made from glass (15 mm inner diameter) and consisted of a base (60 mm length) and two arms (90 mm length, divided by a 45° angle). It was positioned at a 30° slope with the arms pointing upward and illuminated from above by two LED tubes (350 lm, 5 W). Each arm was connected via Teflon tubes to separate Erlenmeyer flasks (50 ml) containing artificial host patches (5 g Drosophila rearing substrate, on which 10 D. simulans females were allowed to oviposit for 48 h). Humidified air was pumped through the flasks into the Y-tube arms at an air flow rate of 30 ml min−1. To test the attraction of males to the odor of females, 2- to 6-day-old male wasps were given the choice between the odor of a host patch and the odor of a host patch with 20 conspecific virgin female wasps (1–3 days old). In a follow-up experiment, instead of live females, 2 μl female extract [equivalent to 1/5th of a female; pooled extracts with 10 μl Dichloromethane (DCM) per female] was applied to a filter paper disk (5 mm diameter) and positioned into the end of one arm of the Y-tube. As a control, 2 μl DCM was applied to a filter paper disk and positioned in the other arm. Impregnated filter paper disks were left to evaporate for 30 s before being used for the experiment. Likewise, the attraction of females to males was tested by presenting two host patches in separate Erlenmeyer flasks, with one additionally containing 20 conspecific males (2–6 days old), to 1- to 3-day-old virgin females. To check whether males and females meet on host patches, their attraction to host patches with eggs and larvae of their host fly species was tested by presenting them with either the host patch or moist cotton pads. To differentiate whether female wasps disperse to find host patches before or after mating, the attraction of virgin and mated females to the host patch odor was compared here. For each run of the experiments, one male or female wasp was carefully placed into the entrance of the base of the Y-tube olfactometer by using an aspirator. Subsequently, the wasp was allowed to choose between the control odor (host patch, host patch with DCM solvent, or moist cotton) and the treatment odor (host patch with 20 conspecific individuals of the opposite sex, host patch with extract of conspecific females, or host patch). The experiment was stopped after the wasps had either crossed a decision line, which was marked within each Y-tube arm at a distance of 2 cm beyond the branching point, or after 5 min (attraction to odor of mating partners) or 10 min (attraction to odor of host patches), if no choice occurred, and the respective decisions were recorded. After each run, the Y-tube was turned, and the control and treatment odor were swapped. The Y-tube was cleaned with ethanol and hot water following every second run. Each individual wasp was only used once (N = 30 for each species and treatment).
Statistics
To test for differences between the dispersal curves of female and male wasps of each species, we used the log-rank test as implemented in the R function survdiff.
To analyze differences in male dispersal depending on the presence of females, the proportion of males dispersing in each observation interval within 48 h before and 48 h after the emergence of the first female was calculated. We compared the mean proportions of dispersed males in these 48-h intervals per species using Wilcoxon signed rank tests (Bonferroni corrected for multiple comparisons). Furthermore, Wilcoxon signed rank tests (Bonferroni corrected) were used to analyze male dispersal differences dependent on the volatility of female sex pheromones, in comparing pooled data for species using CHCs (L. pacifica, L. ryukyuensis) and iridoids (L. heterotoma, L. japonica) as sex pheromones.
For the analysis of the dispersal of female wasps, the proportion of females that dispersed at every time point as well as the mean proportion of dispersed females within 24-h intervals (up to 96 h) after the emergence of the first female was calculated for each jar. The differences in female dispersal between all species were examined in each 24-h interval with Kruskal–Wallis rank sum tests. As post hoc tests we used pairwise Wilcoxon signed rank tests (Bonferroni corrected). Additionally, we analyzed the differences in the dispersal quotients of female wasps of species with differently volatile sex pheromones (iridoids: L. heterotoma and L. japonica, CHCs: L. pacifica and L. ryukyuensis) by using Wilcoxon signed rank tests (Bonferroni corrected).
The decisions of wasps in the Y-tube olfactometer were analyzed using a two-sided binomial test. For each experiment, N = 30. All statistics were carried out in R Version 3.3.0.
Results
The first male wasps of L. pacifica emerged on average 34.0 ± 12.6 h (mean ± SD) before their conspecific females in the same jars, whereas males of L. japonica emerged 39.4 ± 18.1 h (mean ± SD), those of L. ryukyuensis 42.0 ± 13.9 h (mean ± SD), and L. heterotoma 46.2 ± 17.1 h (mean ± SD) on average before their females.
In total, 57.7% of the males and 57.9% of the females of L. heterotoma dispersed, whereas 76.6% of the males and 39.0% of the females of L. japonica, 46.9% of the males and 38.0% of the females of L. ryukyuensis, and 63.2% of the males and 19.7% of the females of L. pacifica dispersed (Figure 1). Dispersal differed significantly between male and female wasps in all species (Figure 1; Log-rank curve comparison tests between female and male wasps in L. heterotoma: χ2(1) = 4.3, P = 0.040; L. japonica: χ2(1) = 344, P < 0.001; L. ryukyuensis: χ2(1) = 31.6, P < 0.001; L. pacifica: χ2(1) = 411, P < 0.001). Males of all species started to disperse before the emergence of their conspecific females (Figure 1) and dispersed more quickly than their females. However, whereas males of the species L. heterotoma and L. japonica dispersed directly after emergence from their host patches and jars (Figures 1A,B), males of L. ryukyuensis and L. pacifica tended to stay longer after emergence on their host patches, before they started to disperse (Figures 1C,D). Females of all species started to disperse directly after their emergence, whereby the proportion of dispersing female wasps of L. heterotoma was about 1.5 to 3 times higher than those of the other species (Figure 1).
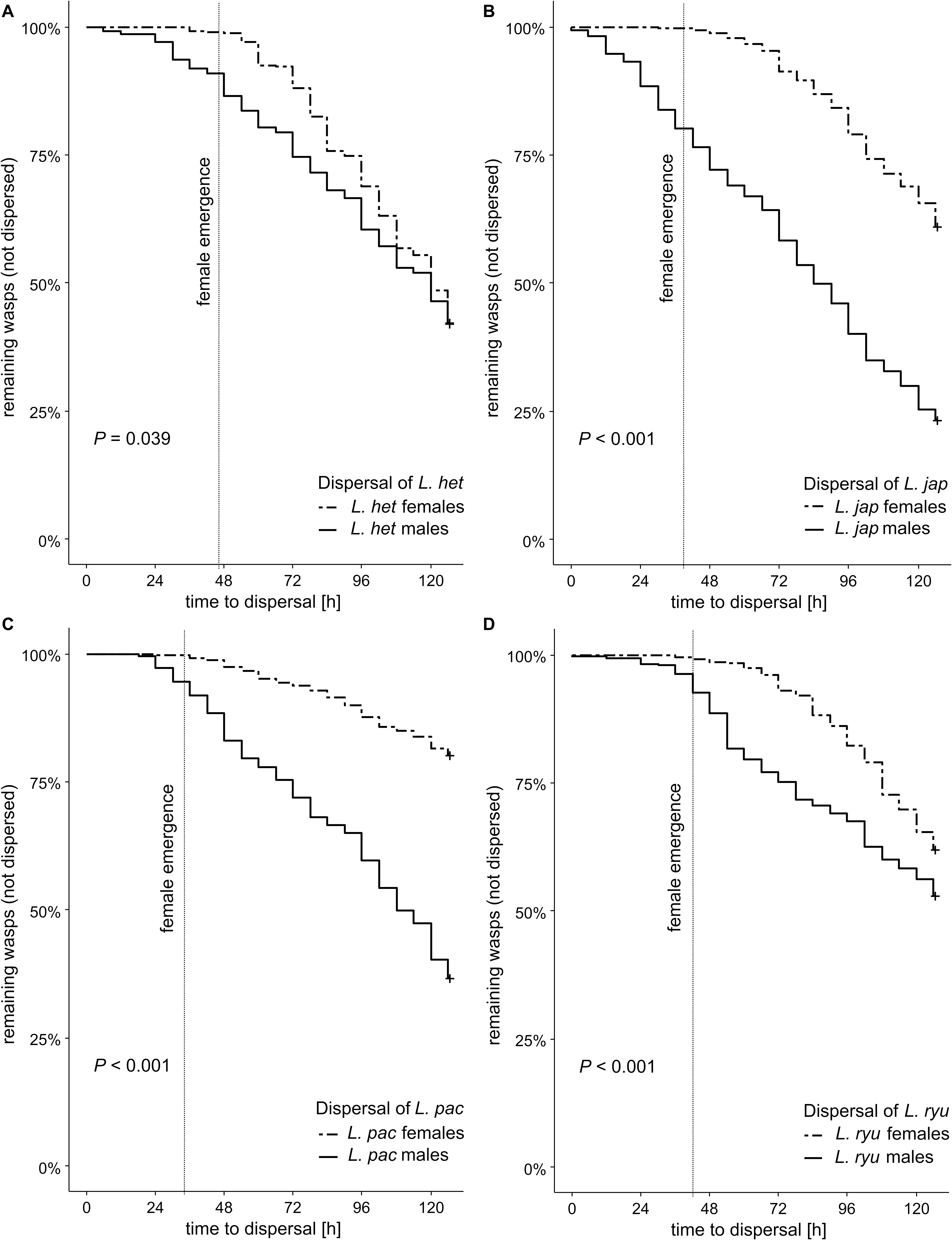
Figure 1. Kaplan Meier plots showing the probability of dispersing for female and male wasps of (A) L. heterotoma (L. het), (B) L. japonica (L. jap), (C) L. pacifica (L. pac), and (D) L. ryukyuensis (L. ryu) within the first 126 h after emergence of the first wasp per jar. Numbers of females and males were summed up over all jars. The mean time point of female emergence per species is indicated with a dotted vertical line. Statistical differences between dispersal curves of the different sexes were analyzed with log rank tests. For each experiment, N = 30.
The dispersal of males of L. heterotoma, L. japonica, L. pacifica and L. ryukyuensis showed differences between all species and depended in two of the species on the presence of females (Figure 2). The dispersal of males of species with highly volatile sex pheromones, namely of L. heterotoma and L. japonica, did not change with the presence of females (Wilcoxon signed rank tests: V = 129, P = 0.196; V = 160, P = 1, respectively; Figure 2), as the proportions of dispersing males from their natal patch stayed the same before and after the emergence of females (Figure 2). In contrast, in L. pacifica and L. ryukyuensis, which use low volatile CHCs as sex pheromones, significantly fewer males dispersed before the emergence of the first female than afterward (Wilcoxon signed rank tests: V = 25, P < 0.001; V = 47, P = 0.007, respectively; Figure 2). Moreover, when we combined the data for species with volatile iridoids and low volatile CHCs as sex pheromones, we obtained the same result (Figure 3). In species in which the females had volatile iridoid sex pheromones, the males started to disperse directly after emergence from the natal patch, before the females emerged, and dispersed at a similar rate after the females emerged (Wilcoxon signed rank tests: V = 571, P = 0.257). However, if they had sex pheromones of low volatility, males dispersed less before female emergence and significantly more after the presence of conspecific females (Wilcoxon signed rank tests: V = 143, P < 0.001; Figure 3).
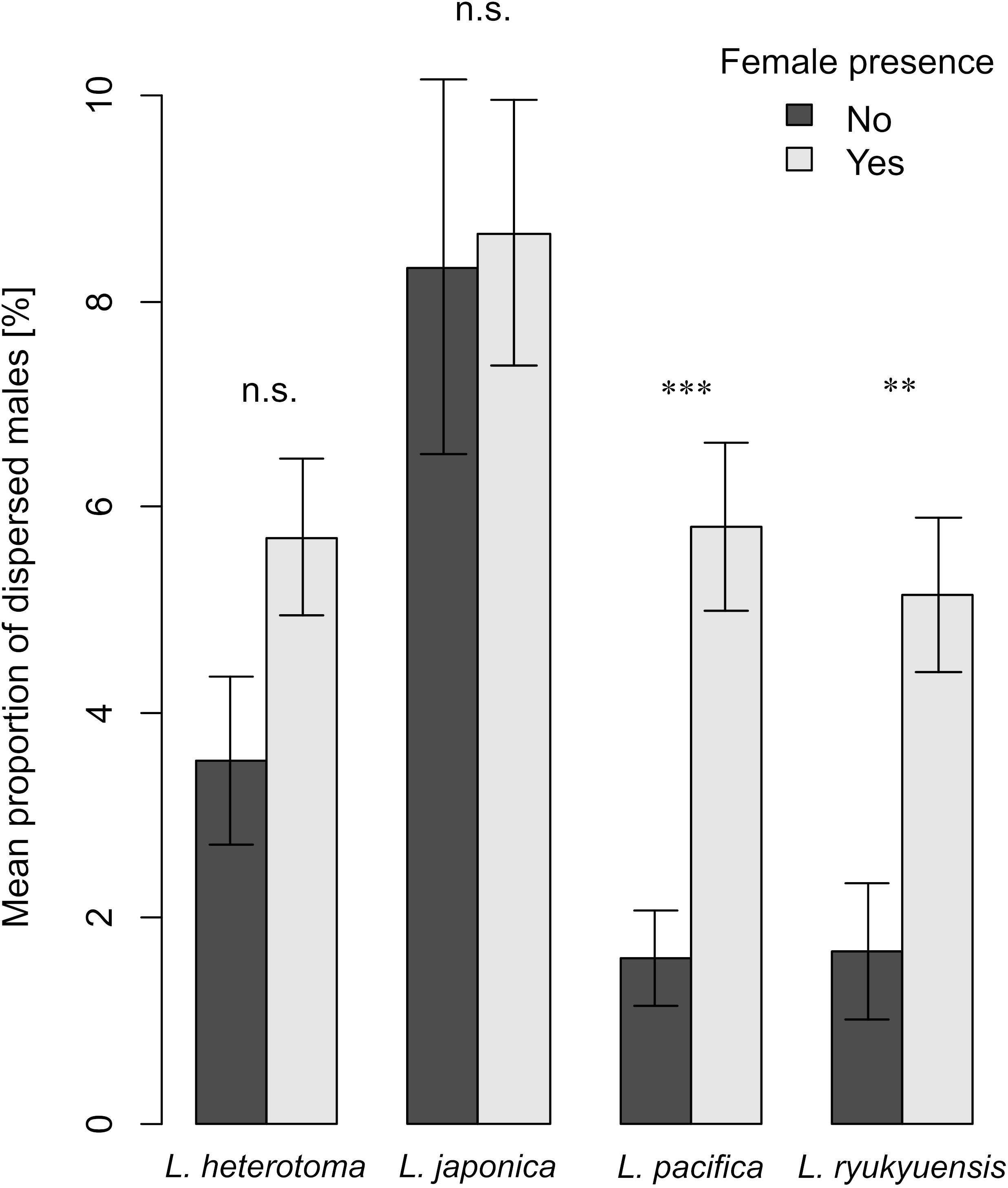
Figure 2. Barplots showing means and standard errors of the mean proportions of dispersed males within the 48 h before (dark gray bars) and within the 48 h after (light gray bars) female emergence in each jar for L. heterotoma, L. japonica, L. pacifica, and L. ryukyuensis. Dispersal before and after female emergence was compared with Wilcoxon signed rank tests (Bonferroni corrected). Asterisks indicate a significant difference ** P < 0.01, *** P < 0.001, n.s. not significant. For each experiment, N = 30.
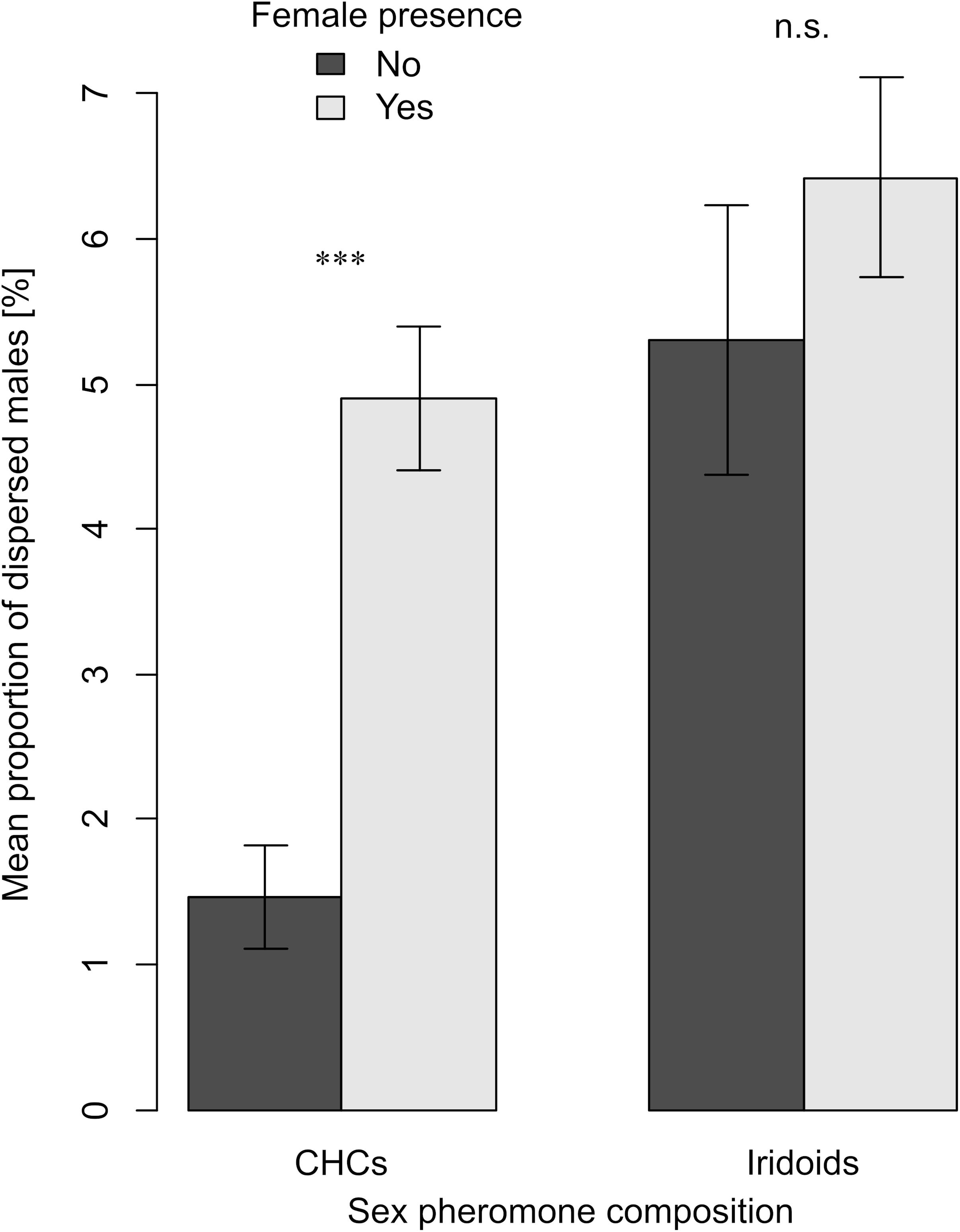
Figure 3. Barplots showing means and standard errors of the mean proportions of dispersed males within the 48 h before (dark gray bars) and within the 48 h after (light gray bars) female emergence in each jar for species using CHCs (L. pacifica and L. ryukyuensis) or iridoids (L. heterotoma, L. japonica) in the female sex pheromone. Dispersal before and after female emergence was compared with Wilcoxon signed rank tests (Bonferroni corrected). Asterisks indicate a significant difference *** P < 0.001, n.s. not significant. For each experiment, N = 60.
When checked for species-specific effects, the proportions of dispersed female wasps differed among the four investigated species within all 24-h time intervals after female emergence (Kruskal–Wallis rank sum tests, 0–24 h: χ2 = 14.073, df = 3, P = 0.003; 24–48 h: χ2 = 25.181, df = 3, P < 0.001; 48–72 h: χ2 = 28.953, df = 3, P < 0.001; 72–96 h: χ2 = 30.331, df = 3, P < 0.001; Figure 4). The proportions of dispersed females of L. heterotoma were higher than those of the other three species, however, after Bonferroni corrections of P-values these differences were only significant within the first 48 h after emergence (pairwise Wilcoxon signed rank tests: each P < 0.05; Figure 4). The mean proportion of dispersed L. japonica females did never differ from that of L. pacifica (within 0–24 h and 24–48 h: each P = 1; 48–72 h: P = 0.108; 72–96 h: P = 0.428) or L. ryukyuensis (each P = 1; Figure 4). Proportions of dispersed female individuals did not differ between L. pacifica and L. ryukyuensis within the first 48 h after emergence (within 0–24 h and 24–48 h: each P = 1), but afterward those of L. pacifica were lower than those of L. ryukyuensis (within 48–72 h: P = 0.045; 72–96 h: P < 0.001). Albeit these species-specific differences in female dispersal, we found that when we combined species according to the volatility of their sex pheromones, the mean proportion of dispersed female wasps of species with highly volatile iridoid sex pheromones, namely of L. heterotoma and L. japonica, was significantly higher than that of females of L. pacifica and L. ryukyuensis, which have sex pheromones consisting of less volatile CHCs (Figure 5). This difference was visible in all 24-h intervals after the first female emergence in each jar (Wilcoxon signed rank tests, 0–24 h: V = 278, P = 0.006; 24–48 h: V = 417, P = 0.005; 48–72 h: V = 360, P < 0.001; 72–96 h: V = 486, P = 0.011; Figure 5).
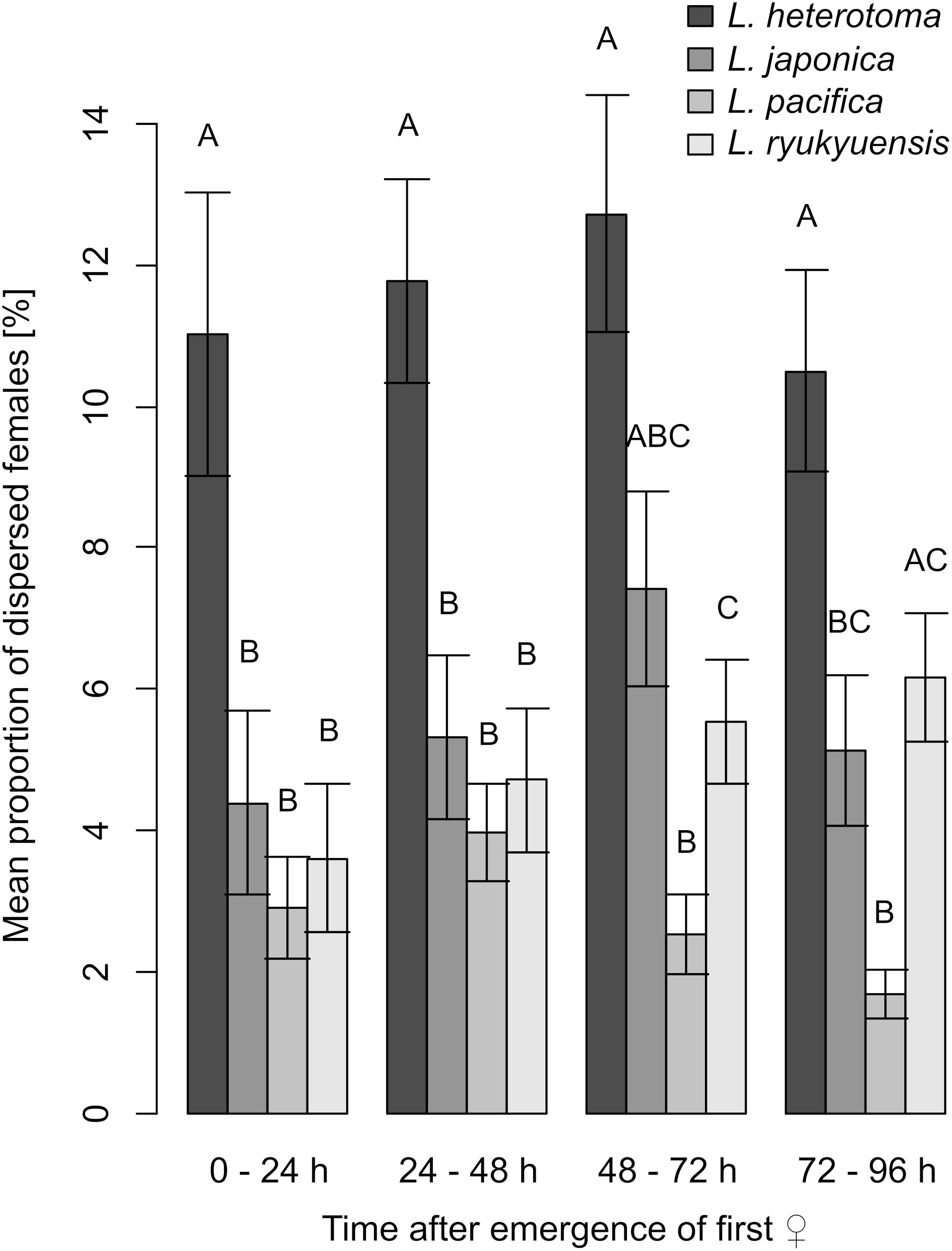
Figure 4. Barplots showing means and standard errors of the mean proportions of dispersed females within 24-h intervals after emergence of the first female in each jar for each of the species L. heterotoma, L. japonica, L. pacifica, and L. ryukyuensis. Differences between species within time intervals were analyzed using Kruskal–Wallis rank sum tests with post hoc pairwise Wilcoxon signed rank tests (Bonferroni corrected). Different letters indicate a significant difference, P < 0.05. For each experiment, N = 30.
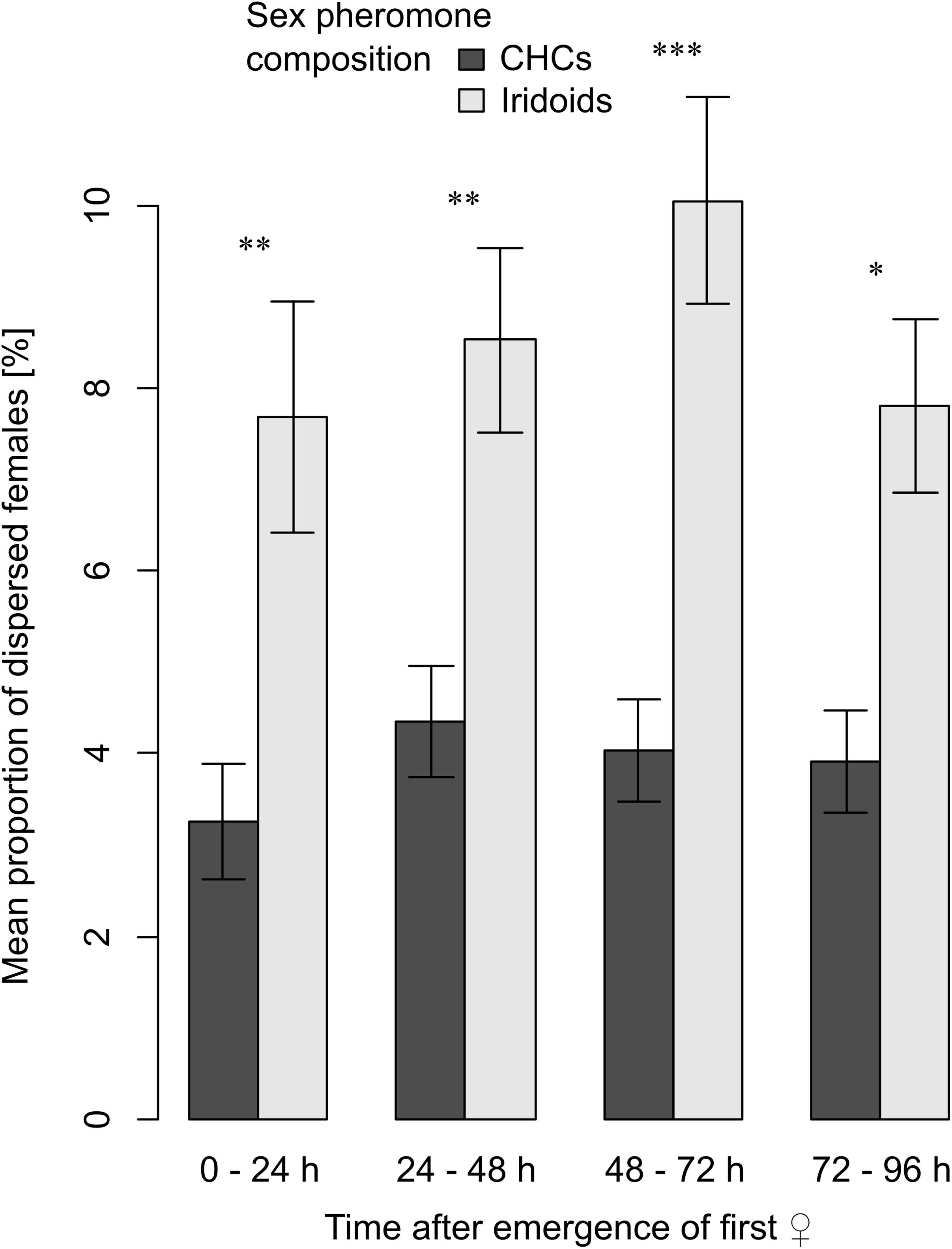
Figure 5. Barplots showing means and standard errors of the mean proportions of dispersed females within 24-h intervals after emergence of the first female in each jar for species using CHCs (L. pacifica and L. ryukyuensis, dark gray bars) or iridoids (L. heterotoma, L. japonica, light gray bars) in the female sex pheromone. Dispersal in each time interval was compared with Wilcoxon signed rank tests (Bonferroni corrected). Asterisks indicate a significant difference * P < 0.05, ** P < 0.01, *** P < 0.001. For each experiment, N = 60.
To assess the odor sources that enable mate-finding after the dispersal of males and females, attraction assays were performed in which wasps could decide between two presented odors. Males of all species were attracted to the odor of their living conspecific females (L. heterotoma: P < 0.001; L. japonica: P < 0.001; L. ryukyuensis: P < 0.001; L. pacifica: P = 0.016; Figure 6A) and to the extract of their females (L. heterotoma: P < 0.001; L. japonica: P < 0.001; L. ryukyuensis: P = 0.016; L. pacifica: P < 0.001; Figure 6B), irrespective of the composition of the female sex pheromone, but not to the odor of host patches (L. heterotoma: P = 0.362; L. japonica: P = 0.361; L. ryukyuensis: P = 0.585; L. pacifica: P = 0.856; Figure 6C). Females, however, were not attracted to the odor of males (L. heterotoma: P = 0.585; L. japonica: P = 0.855; L. ryukyuensis: P = 1; L. pacifica: P = 0.099; Figure 7A), but to the odor of host patches (virgin L. heterotoma: P = 0.016; mated L. heterotoma: P = 0.016; virgin L. japonica: P = 0.005; mated L. japonica: P < 0.001; virgin L. ryukyuensis: P < 0.001; mated L. ryukyuensis: P < 0.001; virgin L. pacifica: P < 0.001; mated L. pacifica: P < 0.001; Figures 7B,C). No difference was noted in this decision according to the mating status of the female wasps (Figures 7B,C).
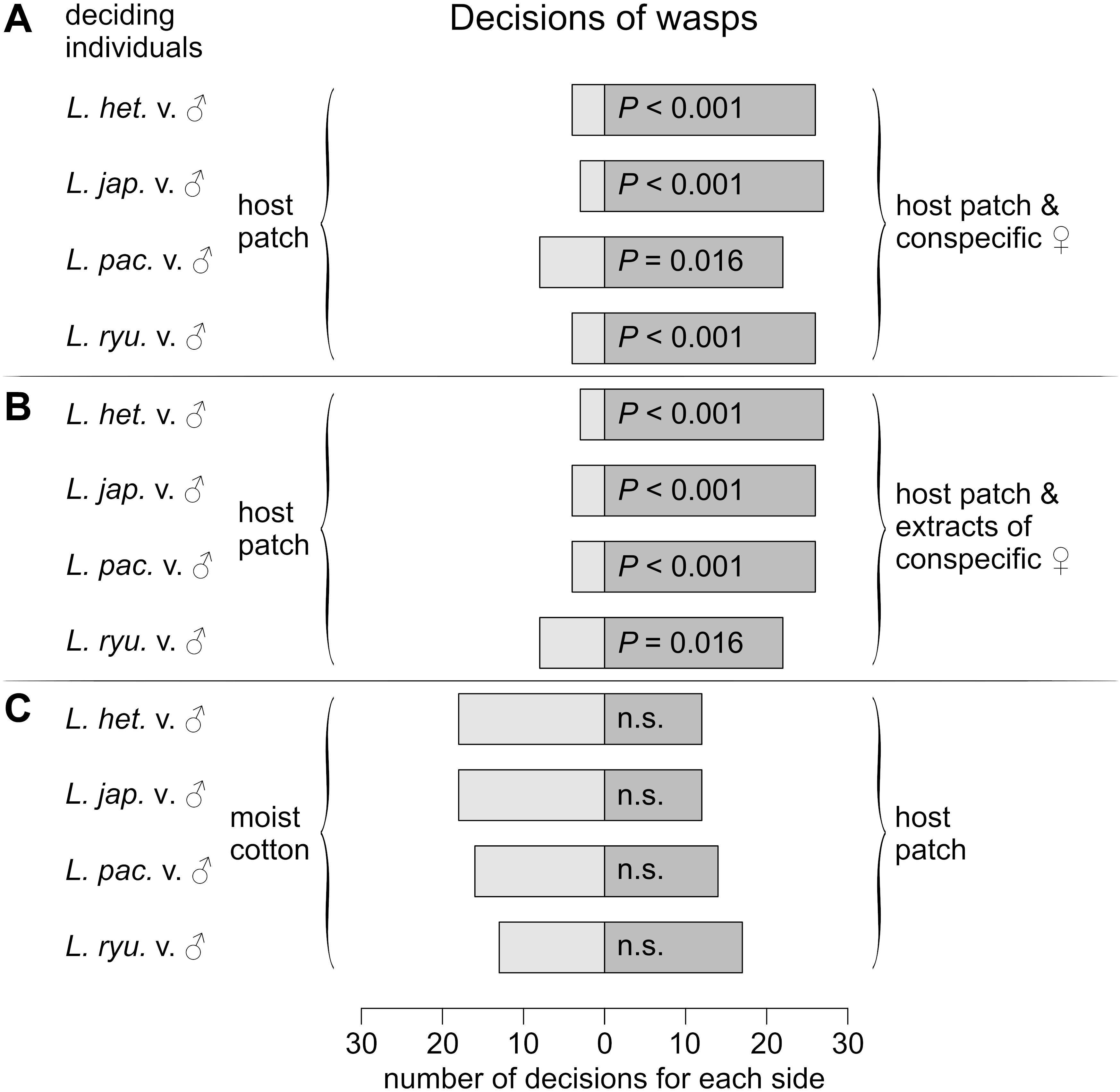
Figure 6. Frequency of decision for control (light gray bars) or sample (dark gray bars) of males of L. heterotoma, L. japonica, L. pacifica, or L. ryukyuensis in a Y-tube experiment with a choice between the odor of unexploited host patches or host patches with either (A) 20 conspecific females or (B) extracts of their females; (C) Decisions of males of L. heterotoma, L. japonica, L. pacifica, or L. ryukyuensis for host patches or clean humidified air. P-values are given for two-sided binomial tests. For each experiment, N = 30. L. het. = Leptopilina heterotoma, L. jap. = Leptopilina japonica, L. pac. = Leptopilina pacifica, L. ryu. = Leptopilina ryukyuensis, v. = virgin, mc = moist cotton, n.s. = not significant.
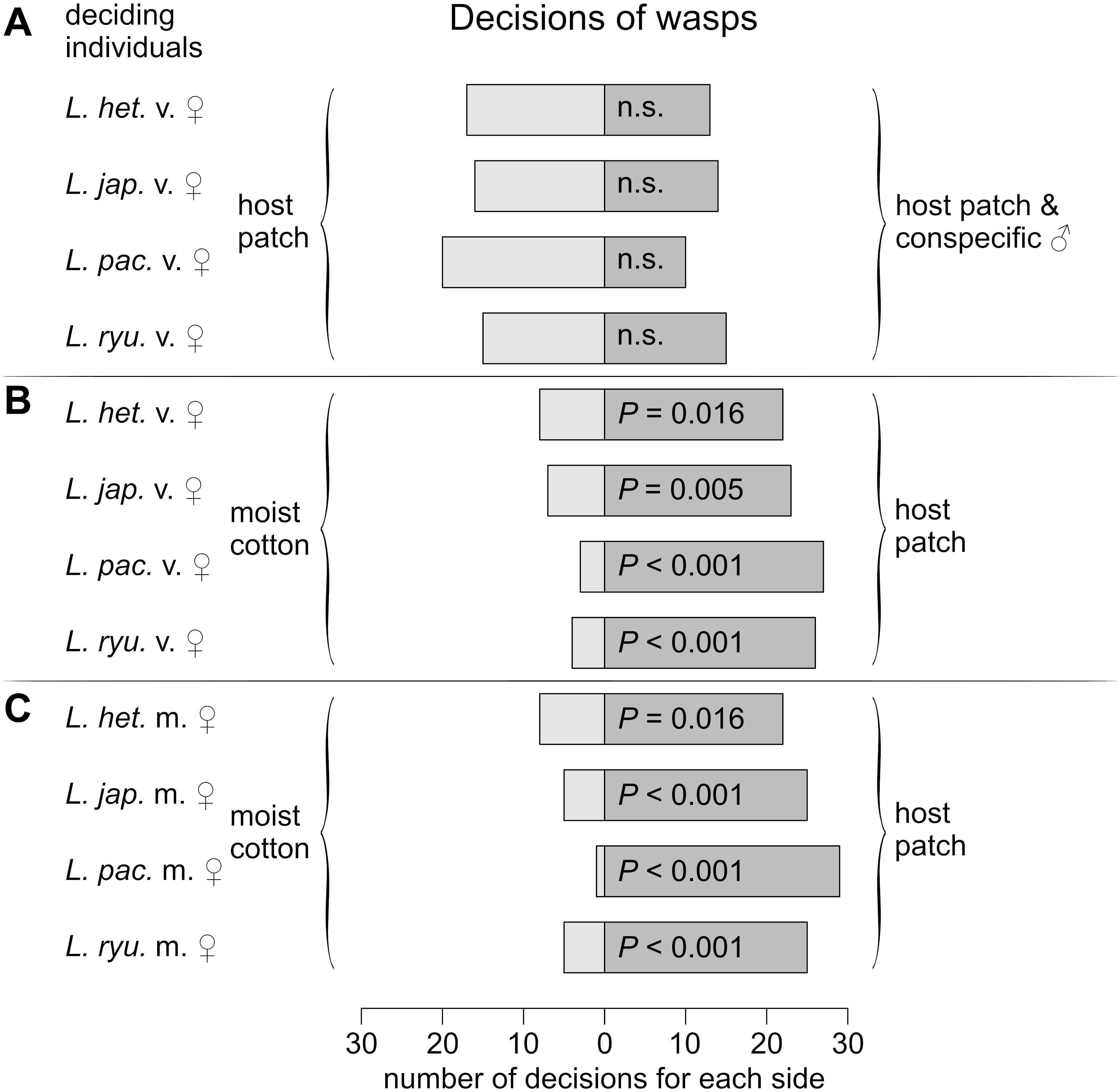
Figure 7. Frequency of decision for control (light gray bars) or sample (dark gray bars) of virgin females of L. heterotoma, L. japonica, L. pacifica, or L. ryukyuensis in a Y-tube experiment with a choice between the odor of unexploited host patches or (A) host patches with 20 conspecific males or (B) clear humidified air provided by moist cotton pads, (C) Decisions of mated females of each of the species when choosing between the odor of unexploited host patches or clean humidified air. P-values are given for two-sided binomial tests. For each experiment, N = 30. L. het. = Leptopilina heterotoma, L. jap. = Leptopilina japonica, L. pac. = Leptopilina pacifica, L. ryu. = Leptopilina ryukyuensis, v. = virgin, m. = mated, n.s. = not significant.
Discussion
Dispersal Can Explain Sex Pheromone Composition
Within the genus Leptopilina, all species studied to date have different combinations of low volatile CHCs and/or volatile iridoids as female-produced sex pheromones (Weiss et al., 2013, 2015; Pfeiffer et al., 2018; Böttinger et al., 2019; Böttinger et al. submitted). The evolution of this female sex pheromone diversity might have been shaped by the dispersal behavior of male and female wasps, as we have found a correlation between the post-emergence dispersal of males (Figures 2, 3) and females (Figure 5) and the volatility of the sex pheromone of the females. We show here that males of the species L. pacifica and L. ryukyuensis, in which the long-chained CHCs of the females elicit courtship (Böttinger et al., 2019; Böttinger et al. submitted), delay their dispersal until females emerge at their host patch. In contrast, in the species L. heterotoma and L. japonica, whose female sex pheromones consist of volatile iridoid compounds (Weiss et al., 2013; Böttinger et al., 2019), a high proportion of males disperse before the first females emerge. No change occurs in the rate of male dispersal after the emergence of the females. Furthermore, the dispersal of female wasps with highly volatile iridoid sex pheromones is continuously more pronounced than that of females with low volatile CHC-based sex pheromones when species are pooled according to the sex pheromone volatility (Figure 5). However, this difference is due to the high proportions of dispersing L. heterotoma females compared to those of the other species (Figure 4) and not due to the sex pheromone differences of females. Therefore, the differences in the sex pheromone compositions of the genus Leptopilina can be explained by the different dispersal patterns of male wasps. Consequently, post-emergence dispersal behavior might have shaped the evolution of the female sex pheromones in this genus. To our knowledge, this is the first time that the volatility of female sex pheromones has been associated with the dispersal behavior of males.
Emergence of Females Is Important for Male Dispersal
We have found that the time point of female emergence (about 2 days after male emergence in all species) demonstrates a changing point in the dispersal of males in the species with low volatile CHCs as sex pheromones. In these species (L. pacifica and L. ryukyuensis), the small proportion of males dispersing before the emergence of conspecific females indicates that males of these species stay at the host patches to wait for the females to emerge in order to ensure successful mate-finding and mating. This can be explained by the fact that mate location over long distances is not possible through the use of long-chained CHCs as sex pheromones. The risk of dispersing and not being able to find mating partners when CHCs are used as sex pheromones is probably high, and it is thus beneficial for males of these species to wait for the emergence of potential mates. In many other species of parasitoid wasps, males wait for conspecific females to emerge to mate with them. In Cotesia waterstoni, for example, male wasps wait at their place of emergence, recognize cocoons of conspecific females because of the leakage of CHCs of the females through the wall of the cocoon (Howard, 1992), and chew these open to mate with the females (Evans, 1964; Gordh and Móczár, 1990). Furthermore, in the parasitoid wasp Lariophagus distinguendus, males emerge before females and recognize the pheromones of the females while the latter are still pupated (Steiner et al., 2005). Also, approximately 50–60% of individuals in the gregarious parasitoid wasp, Cotesia glomerata, mate on the natal patch (Kitano, 1976; Tagawa and Hidaka, 1982; Gu and Dorn, 2003). The dispersal of the remaining newly emerged wasps of C. glomerata before mating is assumed to be induced by pheromones to reduce fierce competition, as males as well as mated females use the compound heptanal to repel siblings (Xu et al., 2019). Mating on the host patch of emergence leads to local mate competition (LMC; Hamilton, 1967) among male wasps for access to females. This LMC can lead to physical fights among male wasps (Godfray, 1994; Boulton et al., 2015), resulting in some cases, as in fig wasps or most wasps of the genus Melittobia, in the killing of competitors (Herre et al., 1997; Matthews et al., 2009). However, nothing is not known about male-male combat for mating opportunities or competition-mediated dispersal in male Leptopilina wasps. LMC can also result in female-biased sex ratios, as in, for example, the jewel wasp Nasonia vitripennis (Ruther et al., 2009). Here, offspring sex ratios can be adjusted, depending on the foundress number, in order to minimize competition between brothers. If only one foundress female lays eggs, the minimal necessary number of males to inseminate all female offspring of the brood will be produced (Ruther et al., 2009 and references therein). However, although LMC has been described in some Leptopilina species, consequences such as strong female-biased sex ratios have not been observed to date (Fauvergue et al., 1999).
Iridoid Sex Pheromones Are Used for Long-Range Attraction
In contrast to the species with CHCs as sex pheromones, males of Leptopilina species with iridoids as sex pheromones, namely L. heterotoma and L. japonica, dispersed directly after emergence and showed no difference in their rate of dispersal after the emergence of their females (Figures 2, 3). This result was expected, as the dispersal of male wasps (before female emergence) is attributed to mate-searching; long-range mate-finding for males of these species is possible because of the volatile iridoid sex pheromone emitted by the females. Males of L. boulardi and L. heterotoma have previously been shown to be attracted to volatiles of virgin females in the field (Fauvergue et al., 1999). Male Leptopilina wasps benefit from (off-patch) mate-searching as they mate several times, while females mate only once (van den Assem, 1968). Therefore, males with iridoid female sex pheromones might benefit from direct dispersal, as they could have increased mating opportunities with unrelated conspecifics compared to males that stay at the natal patch. The mating strategy of Leptopilina species with iridoids as sex pheromones could be the avoidance of inbreeding through direct dispersal, as discussed below.
Long-Range Attraction in Species With CHC-Based Sex Pheromones
Males of species with CHC-based sex pheromones also disperse from the host patch, although later than males of species with iridoid-based sex pheromones. As mate searching is the most likely reason for males to disperse, a long range mate-finding mechanism must also exist in species that use CHCs as sex pheromones. Indeed, we have shown in the olfactometer experiments, that the males of all the studied Leptopilina species are attracted to the compounds of their conspecific females (Figure 6). This is not surprising for species with iridoids as long-range sex pheromones. However, even species that use the long-chained CHCs of their females as sex pheromones are attracted over longer distances (in the Y-tube olfactometer) to their females. We can exclude that the attraction is mediated by low-volatile CHCs, as long-chained CHCs are solely perceivable in the close vicinity of the emitting individual (e.g., Schiestl and Ayasse, 2000; Blomquist and Bagnères, 2010; Ruther et al., 2011; Kühbandner et al., 2012; Ruther, 2013). For example, in wasps of the genus Lariophagus, the activity of the female pheromone consisting of CHCs is restricted to a distance of 0–5 mm (Ruther et al., 2000; Steiner et al., 2005, 2007a). This suggests that, even in the Leptopilina species with CHCs as sex pheromones, other compounds lead the males to conspecific females. These compounds are either the iridoid compounds of the females or the odor of the host patch. In several insect species, host-plant volatiles or host-associated volatile cues are used for the location of mating partners from a distance. Several parasitic wasps with CHC-based female sex pheromones, such as C. glomerata, use volatiles emitted by plants damaged by their host insects as long-range attraction cues to find mates (Xu et al., 2017; Xu and Turlings, 2018). Moreover, males of L. distinguendus orient themselves to the same volatiles emitted by host larval feces to which females are also attracted (Steidle and Ruther, 2000; Steiner et al., 2007b). This is not, however, the case in males of the genus Leptopilina, for which we have found no attraction of males to odors of host patches (Figure 6C). Our results therefore suggest that, even in species with CHC-based sex pheromones, the iridoid compounds emitted by females are used for long-range attraction of males.
Dispersal of Females
Female dispersal of the four Leptopilina species is species-specific and not dependent on the volatility of the sex pheromone composition of females (Figure 4). Females of L. heterotoma (volatile iridoid sex pheromones) showed consistently the highest proportion of dispersed individuals compared to the other species (Figure 4). As in males, when the data for species with pheromones of similar volatility were pooled, we have found higher percentages of dispersing females in L. heterotoma and L. japonica, the species using iridoids as sex pheromones, than in the species that use CHCs as sex pheromones (Figure 5). Female dispersal is important and essential for the location and establishment of new hosts or food sources and for the enlargement of distribution areas of that species (Lidicker and Stenseth, 1992; Vinson, 1998). The dispersal of females is necessary as usually no hosts suitable for oviposition are present at the site of emergence (Hardy and Godfray, 1990). We can conclude that female wasps of the four investigated species, and probably also other species of the genus Leptopilina, leave their host patch to search for oviposition sites, as females of all species were not attracted to conspecific males in the olfactometer experiments (hence, they are not interested in mate-searching; Figure 7A), but are attracted to host patch odors, irrespective of their mating status (Figures 7B,C). This result is consistent with the findings of earlier studies showing that female Leptopilina wasps are attracted to volatile cues of their host habitats (Vet and van der Hoeven, 1983; Dicke et al., 1984; Vet and van Opzeeland, 1984). We assume that the female wasps that dispersed out of the jars were mated, as the encounter rate of males and females on the host patches was high, and in a study of Hardy and Godfray (1990), almost all female L. heterotoma wasps found at host patches were mated. However, even if females of Leptopilina disperse before mating, the attraction of males via the iridoid compounds is possible.
Evolution
The way in which the various sex pheromones, i.e., volatile iridoids and low-volatile CHCs, evolved in the genus Leptopilina is not clear (Weiss et al., 2013, 2015; Pfeiffer et al., 2018; Böttinger et al., 2019; Böttinger et al. submitted). The sex pheromone composition does not show a phylogenetic pattern, i.e., closely related sister-species do not have a markedly more similar or more different sex pheromone composition than more distantly related species (unpublished and Böttinger et al., 2019). The high diversity in sex pheromone composition between species would not have been necessary for species divergence, as species specificity could have easily been achieved within one class of compounds. The data presented here show that the dispersal of male wasps of the genus Leptopilina correlates with the volatility of the species-specific sex pheromones of the females. Immediate dispersal seems to be more beneficial for species with long-range iridoids as female sex pheromones, as in species with short-range CHC as female sex pheromones. Staying on the host patch after emergence might result in sibling matings and inbreeding, if the number of foundress females on this host patch has been low, and the offspring consequently is highly related. Inbreeding is often considered as major ultimate cause and important driver of dispersal (e.g., Bengtsson, 1978; Greenwood, 1980; Pusey, 1987). For example, in the parasitoid wasp C. glomerata, inbreeding avoidance is the main trigger of natal dispersal (Ruf et al., 2011). Depending on the strength of inbreeding depression, individual wasps benefit from mating off-patch and, hence, disperse further away from the natal patch, or, alternatively, require mate-recognition compounds enabling the distinction of inbred kin or ideal mating partners. Such compounds might be the sex- and species-specific CHCs, as they can inform a prospective partner not only about the individual’s condition and genetic make-up, but also about the relatedness of the two individuals (reviewed in Steiger and Stökl, 2014). Although offspring of inbred Leptopilina wasps do not show inbreeding depression or a strong female-biased sex ratio (Biémont and Bouletreau, 1980; Hey and Gargiulo, 1985; Fauvergue et al., 1999), we propose that Leptopilina species with iridoids as sex pheromones avoid inbreeding by their stronger urge to disperse and to out-breed, whereas the species with low volatile sex pheromones make use of the information presented in CHCs to circumvent mismating with too closely related conspecifics. These species therefore do not need to disperse directly after emergence, reducing the costs of mate search for males. Analyses of inbreeding consequences in species with CHCs as sex pheromones should yield further relevant insights.
Data Availability Statement
All datasets presented in this study are included in the article/Supplementary Material.
Author Contributions
Both authors conceived the study, analyzed the data, and revised the manuscript. LB designed, performed the experiments, and wrote the first draft.
Funding
This work was supported by grants from the German Research Foundation DFG (STO 966/1-2 and STO 966/2-1). This publication was funded by the German Research Foundation DFG and the University of Bayreuth in the funding program Open Access Publishing.
Conflict of Interest
The authors declare that the research was conducted in the absence of any commercial or financial relationships that could be construed as a potential conflict of interest.
Acknowledgments
We wish to thank Maximilian Körner and Madlen Prang for helpful discussions on statistical analyses. We also wish to thank Prof. M. T. Kimura (Hokkaido University, Japan) for providing us with wasp strains of L. ryukyuensis, L. japonica, and L. pacifica.
Supplementary Material
The Supplementary Material for this article can be found online at: https://www.frontiersin.org/articles/10.3389/fevo.2020.557527/full#supplementary-material
Supplementary Table 1 contains the dataset of the dispersal experiment, corresponding to Figures 1–5. Supplementary Tables 2–7 contain the results of the attraction assays in the olfactometer, corresponding to Figures 6, 7.
References
Allison, J. D., and Cardé, R. T. (2016). Pheromone Communication in Moths: Evolution, Behavior, and Application. Oakland, CA: University of California Press.
Bengtsson, B. O. (1978). Avoiding inbreeding: at what cost? J. Theor. Biol. 73, 439–444. doi: 10.1016/0022-5193(78)90151-0
Biémont, C., and Bouletreau, M. (1980). Hybridization and inbreeding effects on genome coadaptation in a haplo-diploid hymenoptera: Cothonaspis boulardi (Eucoilidae). Experientia 36, 45–47. doi: 10.1007/bf02003961
Blomquist, G. J., and Bagnères, A.-G. (2010). Insect Hydrocarbons: Biology, Biochemistry and Chemical Ecology. Cambridge: Cambridge University Press.
Boppré, M. (1986). Insects pharmacophagously utilizing defensive plant chemicals (Pyrrolizidine alkaloids). Naturwissenschaften 73, 17–26. doi: 10.1007/BF01168801
Böttinger, L. C., Hofferberth, J., Ruther, J., and Stökl, J. (2019). Semiochemicals mediating defense, intraspecific competition, and mate finding in Leptopilina ryukyuensis and L. japonica (Hymenoptera: Figitidae), Parasitoids of Drosophila. J. Chem. Ecol. 45, 241–252. doi: 10.1007/s10886-019-01052-w
Boulton, R. A., Collins, L. A., and Shuker, D. M. (2015). Beyond sex allocation: the role of mating systems in sexual selection in parasitoid wasps. Biol. Rev. 90, 599–627. doi: 10.1111/brv.12126
Bradbury, J. W., and Vehrencamp, S. L. (2011). Principles of Animal Communication. Sunderland, MA: Sinauer Associates.
Carton, Y., Boulétreau, M., van Alphen, J. J. M., and van Lenteren, J. C. (1986). “The Drosophila parasitic wasps,” in The Genetics and Biology of Drosophila, eds M. Ashburner, H. L. Carson, and J. N. Thompson (Orlando, FL: Academic Press), 347–394.
Dicke, M., Lenteren, J. C., Boskamp, G. J. F., and Dongen-van Leeuwen, E. (1984). Chemical stimuli in host-habitat location by Leptopilina heterotoma (Thomson) (Hymenoptera: Eucoilidae), a parasite of Drosophila. J. Chem. Ecol. 10, 695–712. doi: 10.1007/BF00988537
El-Sayed, A. M. (2018). The Pherobase: Database of Pheromones and Semiochemicals. Available online at: www.pherobase.com
Evans, H. E. (1964). A synopsis of the American Bethylidae (Hymenoptera, Bethylidae). Bull. Mus. Comp. Zool. 132, 1–222.
Fauvergue, X., Fleury, F., Lemaitre, C., and Allemand, R. (1999). Parasitoid mating structures when hosts are patchily distributed: field and laboratory experiments with Leptopilina boulardi and L. heterotoma. Oikos 86, 344–356. doi: 10.2307/3546451
Fujiwara-Tsujii, N., Yamagata, N., Takeda, T., Mizunami, M., and Yamaoka, R. (2006). Behavioral responses to the alarm pheromone of the ant Camponotus obscuripes (Hymenoptera: Formicidae). Zool. Sci. 23, 353–358. doi: 10.2108/zsj.23.353
Geiselhardt, S., Jakobschy, D., Ockenfels, P., and Peschke, K. (2008). A sex pheromone in the desert tenebrionid beetle Parastizopus armaticeps. J. Chem. Ecol. 34, 1065–1071. doi: 10.1007/s10886-008-9488-1
Godfray, H. C. J. (1994). Parasitoids. Behavioral and Evolutionary Ecology. Chichester: Princeton University Press.
Gordh, G., and Móczár, L. (1990). A catalog of the world Bethylidae (Hymenoptera: Aculeata). Mem. Amer. Ent. Inst. 46, 1–364.
Gu, H., and Dorn, S. (2003). Mating system and sex allocation in the gregarious parasitoid Cotesia glomerata. Anim. Behav. 66, 259–264. doi: 10.1006/anbe.2003.2185
Greenwood, P. J. (1980). Mating systems, philopatry and dispersal in birds and mammals. Anim. Behav. 28, 1140–1162. doi: 10.1016/s0003-3472(80)80103-5
Gries, R., Britton, R., Holmes, M., Zhai, H., Draper, J., and Gries, G. (2015). Bed bug aggregation pheromone finally identified. Angew. Chem. Int. Ed Engl. 54, 1135–1138. doi: 10.1002/anie.201409890
Hamilton, W. D. (1967). Extraordinary sex ratios. Science 156, 477–488. doi: 10.1126/science.156.3774.477
Hardy, I. C. W., and Godfray, H. C. J. (1990). Estimating the frequency of constrained sex allocation in field populations of hymenoptera. Behaviour 114, 137–147. doi: 10.1163/156853990X00086
Herre, E. A., West, S. A., Cook, J. M., Compton, S. G., and Kjellberg, F. (1997). “Fig-associated wasps: pollinators and parasites, sex ratio adjustment and male polymorphism, population structure and its consequences,” in The Evolution of Mating Systems in Insects and Arachnids, eds J. C. Choe and B. J. Crespi (Cambridge: Cambridge University Press), 226–239. doi: 10.1017/cbo9780511721946.014
Hey, J., and Gargiulo, M. K. (1985). Sex-ratio changes in Leptopilina heterotoma in response to inbreeding. J. Hered. 76, 209–211. doi: 10.1093/oxfordjournals.jhered.a110069
Howard, R. W. (1992). Comparative analysis of cuticular hydrocarbons from the ectoparasitoids Cephalonomia waterstoni and Laelius utilis (Hymenoptera: Bethylidae) and their respective hosts, Cryptolestes ferrugineus (Coleoptera: Cucujidae) and Trogoderma variabile (Coleoptera: Dermestidae). Ann. Entomol. Soc. Am. 85, 317–325. doi: 10.1093/aesa/85.3.317
Kitano, H. (1976). Studies on the courtship behavior of Apanteles glomeratus L. 3. On the behavior of males and females after their emergence from cocoons. Physiol. Ecol. Japan 17, 383–393.
Kühbandner, S., Sperling, S., Mori, K., and Ruther, J. (2012). Deciphering the signature of cuticular lipids with contact sex pheromone function in a parasitic wasp. J. Exp. Biol. 215, 2471–2478. doi: 10.1242/jeb.071217
Lassance, J.-M., Groot, A. T., Liénard, M. A., Antony, B., Borgwardt, C., Andersson, F., et al. (2010). Allelic variation in a fatty-acyl reductase gene causes divergence in moth sex pheromones. Nature 466, 486–489. doi: 10.1038/nature09058
Lidicker, W. Z., and Stenseth, N. C. (1992). “To disperse or not to disperse: who does it and why?,” in Animal Dispersal, eds N. C. Stenseth and W. Z. Lidicker (London: Chapman & Hall), 21–36. doi: 10.1007/978-94-011-2338-9_2
Löfqvist, J. (1976). Formic acid and saturated hydrocarbons as alarm pheromones for the ant Formica rufa. J. Insect. Physiol. 22, 1331–1346. doi: 10.1016/0022-1910(76)90155-4
Matthews, R. W., González, J. M., Matthews, J. R., and Deyrup, L. D. (2009). Biology of the Parasitoid Melittobia (Hymenoptera: Eulophidae). Annu. Rev. Entomol. 54, 251–266. doi: 10.1146/annurev.ento.54.110807.090440
Mollo, E., Garson, M. J., Polese, G., Amodeo, P., and Ghiselin, M. T. (2017). Taste and smell in aquatic and terrestrial environments. Nat. Prod. Rep. 34, 496–513. doi: 10.1039/c7np00008a
Novković, B., Mitsui, H., Suwito, A., and Kimura, M. T. (2011). Taxonomy and phylogeny of Leptopilina species (Hymenoptera: Cynipoidea: Figitidae) attacking frugivorous drosophilid flies in Japan, with description of three new species. Entomol. Sci. 14, 333–346. doi: 10.1111/j.1479-8298.2011.00459.x
Papaj, D. R., and Vet, L. E. M. (1990). Odor learning and foraging success in the parasitoid Leptopilina heterotoma. J. Chem. Ecol. 16, 3137–3150. doi: 10.1007/BF00979616
Pfeiffer, L., Ruther, J., Hofferberth, J., and Stökl, J. (2018). Interference of chemical defence and sexual communication can shape the evolution of chemical signals. Sci. Rep. 8:970. doi: 10.1038/s41598-017-18376-w
Pusey, A. E. (1987). Sex-biased dispersal and inbreeding avoidance in birds and mammals. Trends Ecol. Evol. 2, 295–299. doi: 10.1016/0169-5347(87)90081-4
Ruf, D., Dorn, S., and Mazzi, D. (2011). Females leave home for sex: natal dispersal in a parasitoid with complementary sex determination. Anim. Behav. 81, 1083–1089. doi: 10.1016/j.anbehav.2011.02.028
Ruther, J. (2013). “Novel insights into pheromone-mediated communication in parasitic hymenopterans,” in Chemical Ecology of Insect Parasitoids, eds E. Wajnberg and S. Colazza (Hoboken, NJ: Wiley-Blackwell), 112–144. doi: 10.1002/9781118409589.ch6
Ruther, J., Döring, M., and Steiner, S. (2011). Cuticular hydrocarbons as contact sex pheromone in the parasitoid Dibrachys cavus. Entomol. Exp. Appl. 140, 59–68. doi: 10.1111/j.1570-7458.2011.01129.x
Ruther, J., Homann, M., and Steidle, J. L. M. (2000). Female-derived sex pheromone mediates courtship behaviour in the parasitoid Lariophagus distinguendus. Entomol. Exp. Appl. 96, 265–274. doi: 10.1023/A:1004000918376
Ruther, J., Matschke, M., Grabe, L.-A., and Steiner, S. (2009). Quantity matters: male sex pheromone signals mate quality in the parasitic wasp Nasonia vitripennis. Proc. R. Soc. Lond. B 276, 3303–3310. doi: 10.1098/rspb.2009.0738
Ruther, J., Reinecke, A., Tolasch, T., and Hilker, M. (2001). Make love not war: a common arthropod defence compound as sex pheromone in the forest cockchafer Melolontha hippocastani. Oecologia 128, 44–47. doi: 10.1007/s004420100634
Schiestl, F. P., and Ayasse, M. (2000). Post mating odor in females of the solitary bee. Andrena nigroaenea (Apoidea, Andrenidae), inhibits male mating behavior. Behav. Ecol. Sociobiol. 48, 303–307. doi: 10.1007/s002650000241
Stacey, N., and Sorensen, P. (2009). “Hormonal pheromones in fish,” in Hormones, Brain and Behavior, ed. D. W. Pfaff (Amsterdam: Elsevier), 639–682. doi: 10.1016/b978-008088783-8.00018-8
Steidle, J. L. M., and Ruther, J. (2000). Chemicals used for host recognition by the granary weevil parasitoid Lariophagus distinguendus. J. Chem. Ecol. 26, 2665–2675.
Steiger, S., Schmitt, T., and Schaefer, H. M. (2011). The origin and dynamic evolution of chemical information transfer. Proc. R. Soc. Lond. B 278, 970–979. doi: 10.1098/rspb.2010.2285
Steiger, S., and Stökl, J. (2014). The role of sexual selection in the evolution of chemical signals in insects. Insects 5, 423–438. doi: 10.3390/insects5020423
Steiner, S., Mumm, R., and Ruther, J. (2007a). Courtship pheromones in parasitic wasps: comparison of bioactive and inactive hydrocarbon profiles by multivariate statistical methods. J. Chem. Ecol. 33, 825–838. doi: 10.1007/s10886-007-9265-6
Steiner, S., Steidle, J. L. M., and Ruther, J. (2007b). Host-associated kairomones used for habitat orientation in the parasitoid Lariophagus distinguendus (Hymenoptera: Pteromalidae). J. Stored Prod. Res. 43, 587–593. doi: 10.1016/j.jspr.2007.03.009
Steiner, S., Steidle, J. L. M., and Ruther, J. (2005). Female sex pheromone in immature insect males - a case of pre-emergence chemical mimicry? Behav. Ecol. Sociobiol. 58, 111–120. doi: 10.1007/s00265-005-0930-x
Stökl, J., and Steiger, S. (2017). Evolutionary origin of insect pheromones. Curr. Opin. Insect. Sci. 24, 36–42. doi: 10.1016/j.cois.2017.09.004
Symonds, M. R. E., and Elgar, M. A. (2008). The evolution of pheromone diversity. Trends Ecol. Evol. 23, 220–228. doi: 10.1016/j.tree.2007.11.009
Tagawa, J., and Hidaka, T. (1982). Mating behaviour of the braconid wasp, Apanteles glomeratus L. (Hymenoptera: Braconidae): mating sequence and the factor for correct orientation of male to female. Appl. Entomol. Zool. 17, 32–39. doi: 10.1303/aez.17.32
van den Assem, J. (1968). Reproductive behaviour of Pseudeucoila bochei (Hymenoptera: Cynipidae). Neth. J. Zool. 19, 641–649. doi: 10.1163/002829669X00080
van den Assem, J., Gijswijt, M. J., and Nübel, B. K. (1980). Observations on courtship - and mating strategies in a few species of parasitic wasps (Chalcidoidea). Neth. J. Zool. 30, 208–227. doi: 10.1163/002829679X00386
Vet, L. E. M., and van der Hoeven, R. (1983). Comparison of the behavioural response of two Leptopilina species (Hymenoptera: Eucoilidae), living in different microhabitats, to kairomone of their host (Drosophilidae). Neth. J. Zool. 34, 220–227. doi: 10.1163/002829684X00173
Vet, L. E. M., and van Opzeeland, K. (1984). Olfactory microhabitat selection in Leptopilina heterotoma (Thomson) (Hym.: Eucoilidae), a parasitoid of Drosophilidae. Neth. J. Zool. 35, 497–504. doi: 10.1163/002829685X00352
Vinson, S. B. (1998). The general host selection behavior of parasitoid Hymenoptera and a comparison of initial strategies utilized by larvaphagous and oophagous species. Biol. Control 11, 79–96. doi: 10.1006/bcon.1997.0601
Weiss, I., Hofferberth, J., Ruther, J., and Stökl, J. (2015). Varying importance of cuticular hydrocarbons and iridoids in the species-specific mate recognition pheromones of three closely related Leptopilina species. Front. Ecol. Evol. 3:19. doi: 10.3389/fevo.2015.00019
Weiss, I., Rössler, T., Hofferberth, J., Brummer, M., Ruther, J., and Stökl, J. (2013). A nonspecific defensive compound evolves into a competition-avoidance cue and a female sex-pheromone. Nat. Commun. 4:2767. doi: 10.1038/ncomms3767
Wheeler, C. A., and Cardé, R. T. (2013). Defensive allomones function as aggregation pheromones in diapausing Ladybird Beetles, Hippodamia convergens. J. Chem. Ecol. 39, 723–732. doi: 10.1007/s10886-013-0293-0
Wyatt, T. D. (2010). Pheromones and signature mixtures: defining species-wide signals and variable cues for identity in both invertebrates and vertebrates. J. Comp. Physiol. A 196, 685–700. doi: 10.1007/s00359-010-0564-y
Xu, H., Desurmont, G., Degen, T., Zhou, G., Laplanche, D., Henryk, L., et al. (2017). Combined use of herbivore-induced plant volatiles and sex pheromones for mate location in braconid parasitoids. Plant Cell Environ. 40, 330–339. doi: 10.1111/pce.12818
Xu, H., and Turlings, T. C. J. (2018). Plant volatiles as mate-finding cues for insects. Trends Plant Sci. 23, 100–111. doi: 10.1016/j.tplants.2017.11.004
Keywords: sex pheromone, mating system, evolution, chemical communication, insects, Leptopilina, Drosophila
Citation: Böttinger LC and Stökl J (2020) Dispersal From Natal Patch Correlates With the Volatility of Female Sex Pheromones in Parasitoid Wasps. Front. Ecol. Evol. 8:557527. doi: 10.3389/fevo.2020.557527
Received: 30 April 2020; Accepted: 19 August 2020;
Published: 08 October 2020.
Edited by:
Sébastien Lebreton, Aix-Marseille Université, FranceReviewed by:
Hao Xu, Nanjing Agricultural University, ChinaJustin Gaudon, University of Waterloo, Canada
Bishwo Mainali, Macquarie University, Australia
Copyright © 2020 Böttinger and Stökl. This is an open-access article distributed under the terms of the Creative Commons Attribution License (CC BY). The use, distribution or reproduction in other forums is permitted, provided the original author(s) and the copyright owner(s) are credited and that the original publication in this journal is cited, in accordance with accepted academic practice. No use, distribution or reproduction is permitted which does not comply with these terms.
*Correspondence: Lea C. Böttinger, Lea.Boettinger@uni-bayreuth.de