- 1Laboratoire d'écologie végétale et de la faune terrestre, Département des Sciences fondamentales, Université du Québec à Chicoutimi, Chicoutimi, QC, Canada
- 2Laboratoire d'écologie historique et de dendrochronologie, Département de biologie, chimie et géographie, Centre d'études nordiques et groupe Boréas, Université du Québec à Rimouski, Rimouski, QC, Canada
Spruce budworm (SBW) outbreaks are a major disturbance in North American forests. In Quebec, Canada, the recent history of SBW outbreaks at the local scale is well-known. Studies at the Québec scale nonetheless remain rare despite the need to better understand the dynamics of SBW outbreaks at a larger scale. This study aimed to reconstruct the spatiotemporal dynamics of SBW outbreaks during the 20th century across the insect's range in southern Quebec. To this end, we sampled 83 stands throughout southern Quebec. These stands were selected according to their age and the presence of black, white, and red spruce. In fact, spruce, unlike balsam fir, survives the SBW outbreaks and can record them in these growth rings. In each stand, cores were taken from 20 spruce trees. The dendrochronological series of more than 1,600 trees were analyzed, and we identified, through the k-means grouping of stands, the spatial patterns of tree growth for the three previously documented 20th century SBW outbreaks. The outbreaks were not homogeneous across the distribution range of the insect. Two groups of stands showed early- (1905–1930) and late-century (1968–1988) outbreaks of high severity and a mid-century (1935–1965) outbreak of moderate severity. This pattern is explained mainly by the presence of the insect within the balsam fir–yellow birch and balsam fir–white birch bioclimatic domains, areas where outbreaks tend to be most severe because of the abundance of balsam fir, the main SBW host species. However, these two models differ in terms of the duration of outbreaks. A third, more northern, cluster of stands experienced lower severity outbreaks over the 20th century, a pattern explained by a lower proportion of balsam fir trees in these landscapes. Our study shows that, on the one hand, these three groups of stands are defined by outbreaks of specific duration (an outbreak period beginning when more than 20% of the trees are affected and ending when <20% of the trees are affected) and severity (in terms of percentage of affected trees), and on the other hand they are spatially distinct and subject to different climatic conditions.
Introduction
The vulnerability and susceptibility of forest stands to SBW outbreaks depend on several factors. The first factor is the abundance of host species. Balsam fir (Abies balsamea (L.) Mill.) is the main host of SBW due to the synchronism in the spring between the bud flush period of the host and the emergence of the insect larvae; this synchronous timing enhances host vulnerability and increases its mortality rate (MacLean, 1980; Blais, 1981; MacLean and Ostaff, 1989; Bergeron et al., 1995). Black (Picea mariana Mill.), white (Picea glauca (Moench) Voss), and red (Picea rubens Sarg.) spruce are secondary hosts of the insect (Morin et al., 2010) due to the highly lignified needles of white spruce and a lack of synchrony between insect emergence and the bud burst of black and red spruce (Nealis and Régnière, 2004). Black, red, and white spruce are not equally susceptible to SBW outbreaks. Hennigar et al. (2008) put forward a susceptibility hierarchy of host species and quantified that when defoliation of balsam fir was 100%, the defoliation of white, red, and black spruce was 72, 41, and 28%, respectively. Consequently, spruce trees will often survive outbreaks and record these events in their growth rings (Blais, 1957; Hennigar et al., 2008), which makes them suitable species for the dendrochronological analysis of outbreaks. A second factor of forest vulnerability is stand structure and age. SBW contributes to the dynamics of the forest mosaic (Bergeron and Dansereau, 1993; Martin et al., 2018, 2019) by eliminating mature trees (Bergeron et al., 1995). Pre-established regeneration survives insect attacks and forms the foundation of the replacement balsam fir stand (Kneeshaw and Bergeron, 1998; De Grandpré et al., 2018). It then takes several decades for the stand to recover into a mature forest that would be sensitive to the next disturbance (Blais, 1985; Martin et al., 2020b). The third factor is the climate. Indeed, temperature has a major effect on the insect's development rate, while humidity has a greater influence on its survival rate (Reichenbach and Stairs, 1984). In order for the insect to develop in all its phenological stages, the average daily air temperature must be above 10°C (Pureswaran et al., 2019). Climatic conditions have hence a major role in restricting the extent and intensity of outbreaks (Gray et al., 2000; Volney and Fleming, 2000, 2007). The capacity for SBW to cause damage depends on both insect population density and phenological timing in spring between emergence of SBW larvae and budding of host trees (Pureswaran et al., 2015, 2019); both factors are dependent on climatic conditions.
Several studies show that SBW outbreaks behave differently depending on their geographic location; for example, outbreaks are more severe and longer lasting in the balsam fir–yellow birch (Betula alleghaniensis Britton) bioclimatic domain (the transition zone between the northern temperate zone and the boreal zone; it extends between 47 and 48°N) and the balsam fir–white birch (Betula papyrifera Marsh) bioclimatic domain (an area in the southern part of the boreal zone, extending from 48 to 50°N) (Saucier et al., 2010) than within other regional domains (Gray et al., 2000; Jardon et al., 2003). The balsam fir–yellow birch bioclimatic domain represents the core of the SBW's geographic distribution and is influenced to a much greater extent by SBW outbreaks than stands within the wetter coastal areas (Bouchard and Pothier, 2010) and those found at the northern (Jardon et al., 2003; Tremblay et al., 2011; Martin et al., 2019) and southern margins of the insect's range (Fraver et al., 2007). This geographic outbreak pattern may be related to unsuitable climatic conditions for SBW (Greenbank, 1957; Régnière et al., 2012), although the lower abundance and limited continuity of host species in these marginal regions may also be contributing factors (Nealis and Régnière, 2004; Gray, 2007; Campbell, 2008).
The main objective of this study is to reconstruct the spatiotemporal dynamics of SBW outbreaks during the 20th century across the insect's range in southern Quebec. The originality of our study stems, in part, from its spatial scale. Numerous regional-scale studies have focused on the dynamics of SBW in Quebec, including studies of the Abitibi (Morin et al., 1993), Saguenay (Morin and Laprise, 1990; Morin, 1994; Krause, 1997), Outaouais (Blais, 1981), Laurentide Reserve (Blais, 1962, 1965), Gaspésie (Blais, 1961), North Shore (Bouchard and Pothier, 2010), Estrie (Filion et al., 1998), and Lower St. Lawrence (Boulanger and Arseneault, 2004) regions. Ours is a provincial-scale study. The second part of this study's originality is our aim to obtain precise information on the spatial distribution, severity, and timing of outbreaks during the 20th century. This is in contrast to the Jardon et al. (2003) study, which estimated the periodicity of outbreaks, or the Navarro et al. (2018) study, which provided a more global understanding of outbreaks as an agent of disturbance and their important role in the history of landscapes, although neither focused the specific timing of these outbreaks. In our study, we use the percentage of affected trees to determine whether a stand experienced an outbreak and, if so, assess the severity of the outbreak (Jardon, 2001; Morin et al., 2007). We hypothesized that (1) distinct SBW outbreak severity and temporality patterns can be observed during the 20th-century, and (2) different spatial locations and climatic characteristics distinguish these patterns.
Materials and Methods
Study Area
The study area (45.3–49.7°N, 68.1–79.5°W) in Quebec (Canada) covers nearly 350,000 km2 (Figure 1) and overlaps the northern temperate zone, dominated by deciduous and mixed stands, and the boreal zone, characterized by evergreen coniferous stands. According to the provincial ecological classification system, the study area encompasses six bioclimatic domains (Saucier et al., 2010) under the influence of a north–south temperature gradient and an east–west precipitation gradient (Wilson, 1971; Richard, 1987). The study area is entirely under the northern limit of commercial forests (Figure 1), beyond which harvesting is prohibited because of recurrent fires and low forest productivity (Jobidon et al., 2015). The regional climate is generally humid continental, i.e., a climate characterized by an average temperature of the four hottest months of the year above 10°C and an average temperature of the coldest month of the year below 3°C. East of the St. Lawrence River, however, the climate has a strong maritime influence characterized by higher temperatures and greater precipitation (Villeneuve, 1959).
Sampling
We developed a tree-ring data set covering the entire study area, which included two pre-existing data sets and a new data set from cores collected in the field (Figure 1). This new data set (spruce budworm project) aimed to ensure an equal distribution of sample stands across the southern portion of the province. The first existing data set (the Jardon project) was developed by Jardon et al. (2003) and the second (the overmature forest project) originates from unpublished research (UQAC Plant Ecology Laboratory) (Table 1). Geographical gaps were filled using a random stratified sampling design based on forest age, relative spruce basal area, and proximity to a road. Selected stands were ≥80 years old, had a relative spruce basal area ≥50%, and were located within 100 m of a road.
We selected spruces trees to reconstruct the history of SBW outbreaks because these species are affected by defoliation. But with a lower mortality rate than balsam fir, thereby allowing us to extend the temporal scale of the outbreak record. Although balsam fir is the preferred host of SBW, it is usually used to reconstruct the history of outbreaks only over a relatively short period because of its high mortality during outbreaks (MacLean and Ostaff, 1989; Bergeron et al., 1995; Morin et al., 2010). The three spruce species present in the study area (i.e., black, red, and white spruce) were used in this study to cover all of southern Quebec. Indeed, these three species have different spatial distributions, notably, red spruce is mostly present in the sugar maple (Acer saccharum Marsh.)- yellow birch domain while black and white spruce are more northerly. Within each stand, we randomly sampled 20 dominant or codominant spruce trees using a Pressler increment borer. We collected two cores per tree from opposing sides of the stem, 30 cm above the ground, to obtain the longest possible time series. The same sampling strategy was used for the Jardon and overmature forest projects.
Dendrochronological Methods
Tree cores were dried and sanded before being measured. Tree-ring widths were measured to the nearest 0.01 mm using the semi-automatic LINTAB® measuring bench coupled to the TSAPWin® automatic recorder. Cores were cross-dated and averaged by tree using the PAST5® program. We standardized ring-width series to eliminate low- and medium-frequency signals by applying a negative exponential function and a cubic spline smoothing function, respectively (Cook and Peters, 1981). We averaged the individual standardized series per stand. We then ran the program OUTBREAK to determine the percentage of trees affected by each outbreak for each stand chronology (Holmes and Swetnam, 1996). We defined an outbreak event as a ring-width decrease lasting at least 5 years and having a maximum growth reduction >1.28 standard deviations from the mean (Blais, 1962; Jardon, 2001; Morin et al., 2010).
Statistical Analysis
We used k-means clustering to identify the activity patterns of SBW (Hartigan and Wong, 1979), grouping the yearly percentage of trees affected by SBW outbreaks into several clusters. This approach was inspired by the studies of Moussaoui et al. (2019) and Martin et al. (2020a), which used k-means clustering to classify patterns in the diameter distribution and radial growth of the sampled trees, respectively. These studies demonstrated that k-means clustering is an effective tool for discriminating complex patterns and can be successfully applied to outbreak chronologies. The k-means clustering algorithm defines total variation within each cluster as the sum of the squared Euclidian distances between the variables and the corresponding centroid. To ensure clustering robustness, we performed 1,000 iterations of the k-means algorithm. The optimal number of clusters was determined by the highest simple structure index (SSI) (Dolnicar et al., 2000). The SSI multiplicatively combines several elements that influence the interpretability of a partitioning solution, that is, “the maximum difference between the clusters for each variable, the sizes of the most contrasting clusters and the deviation of a variable in the cluster centers compared to its overall mean” (Dimitriadou et al., 2002). The maximum value determines the optimal partition, i.e., the number of clusters (Dimitriadou et al., 2002). We also verified whether the clusters contained a homogeneous and sufficient number of sites (i.e., ≥20 sites), thereby ensuring the robustness of the subsequent analyses and the representativeness of the results at the scale of the study area. Moreover, this verification ensures representativeness of the different patterns and thus avoids overly specific clusters that would only concern a small number of stands. We performed k-means clustering with the R software, version 3.3.2 (R Development Core Team, 2019) using the vegan (Oksanen et al., 2007) package and applying a p-threshold of 0.05.
We mapped the resulting clusters. To understand the spatial variation between clusters, we tested for differences in the latitudes and longitudes of the corresponding stands between the clusters using permutational ANOVA (PERMANOVA; (Anderson, 2001) based on Euclidian distances and 10,000 iterations. When the results were significant, we then performed pairwise PERMANOVA, using Euclidian distances, 10 000 iterations, and a Bonferroni adjustment for pairwise comparisons. PERMANOVA was performed using the R software and the pairwiseAdonis (Arbizu, 2017) package, and we applied a p-threshold of 0.05.
We then assigned the mean monthly cumulative precipitation and average temperatures (1901–1995) to each stand from the corresponding grid cell of the CRU.TS.v.4.03 data set provided by the Climatic Research Unit at the University of East Anglia (Harris et al., 2014). This model is based on an updated gridded climate data set across the global land area (excluding Antarctica). The available data for our study area were provided by the Canadian Historical Temperature Database (Vincent and Gullett, 1999).
To understand how stand-scale temporal variations of outbreaks varied between and within the clusters, we carried out an analysis of the onset, end, and duration of the SBW outbreaks. For each cluster, we defined outbreaks as periods where more than 20% of trees were affected, a value used as a threshold for distinguishing minor outbreaks (Morin et al., 2010).
Finally, to determine outbreak severity, we used 20th-century outbreak periods that had been previously identified at the scale of the study area, namely O1 from 1905 to 1930, O2 from 1935 to 1965, and O3 from 1968 to 1988 (Navarro et al., 2018). We assessed outbreak severity for each stand using the average, median, and the 75th and 95th percentiles of the yearly percentage of trees affected.
For all analyses, attributes were compared between the clusters using PERMANOVA and relying on the same methodology as described above. We present our results using various techniques. Boxplots and maps highlight the climatic differences between clusters. A Gantt diagram offers a visualization of the detailed timing of the outbreaks in each cluster. Finally, two tables present the differences in the severity of outbreaks within and between clusters.
Results
The k-means clustering showed that the partition having the highest SSI included three clusters of 24, 30, and 29 stands, respectively (Figures 2A,B). The clusters presented specific patterns of outbreak severity based on the percentage of affected trees between 1900 and 1995 (Figure 2A) with three outbreak peaks at the beginning, middle, and end of the 20th century. Clusters 1 and 3 presented strong outbreak peaks at the beginning and end of the century and a relatively weaker mid-century outbreak. Although these two patterns are similar in terms of severity, they differ in terms of the duration of outbreaks. Cluster 2 on the other hand, showed outbreak peaks of intermediate severity during the 20th century and is similar in duration to Cluster 1.
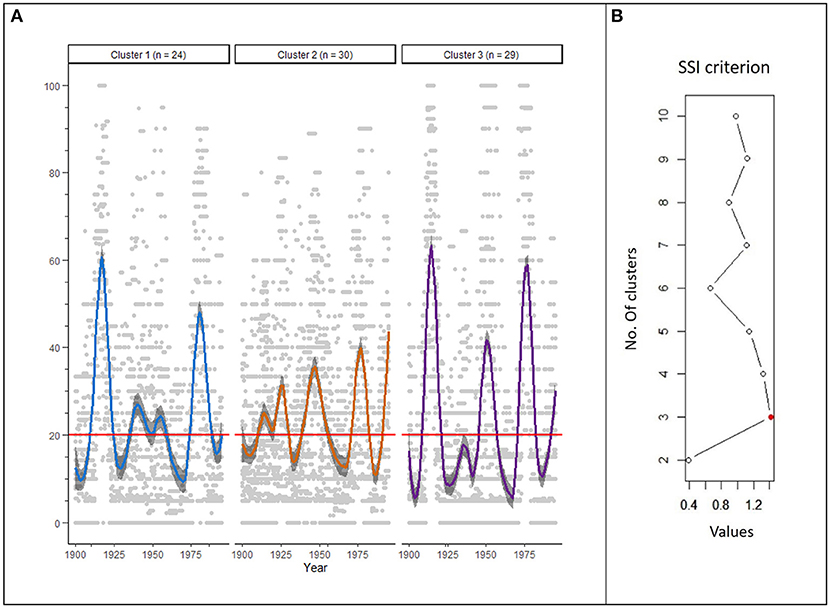
Figure 2. (A) Annual percentage of affected trees in the studied stands for the three clusters identified by k-means clustering. The curves are a LOESS (locally estimated scatterplot smoothing) of the annual percentage of trees affected per year, using a smoothing of 10%. The gray band around the curves corresponds to a 95% confidence interval. The red line corresponds to a percentage of affected trees of 20%. (B) Values of the SSI criterion according to the number of clusters. The red dot indicates the optimal SSI criterion for the partition of clusters.
The analysis of stand coordinates highlighted significant differences (Supplementary Figure 1) in latitude (F,2= 5.135; 0.008**) and longitude (F,2= 10.515; <0.001***) between clusters (Figures 3B,C), although the three clusters overlapped in the center of the study area (Figure 3A). Cluster 1 stands are located mainly in eastern and southeastern Quebec, particularly east of the St. Lawrence River, and in the northern section of the study area. Cluster 2 members are located mainly in the northwestern sector with a few stands found in the south. Cluster 3 contains stands located in southwestern Quebec and a few northern stands.
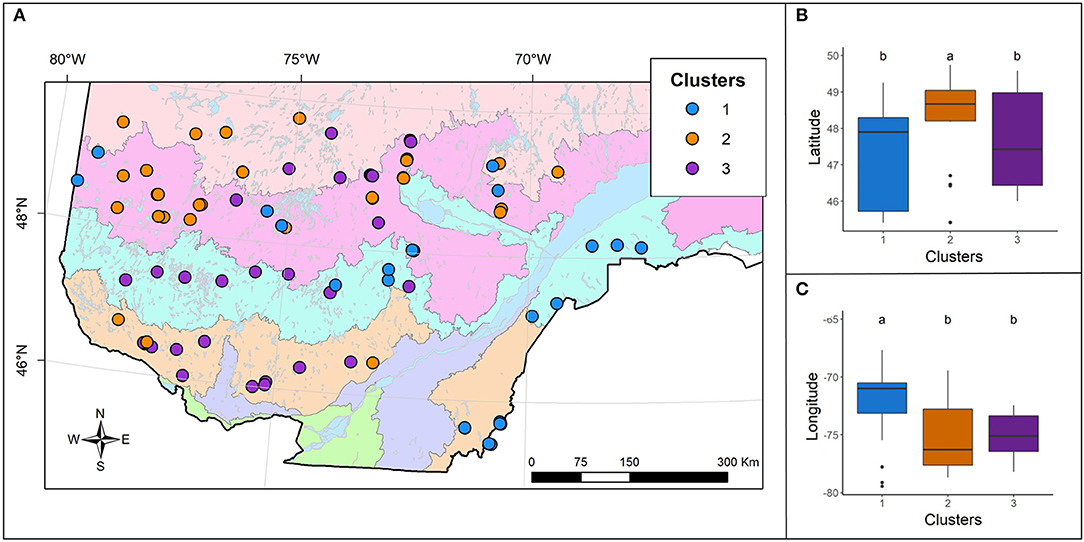
Figure 3. (A) Spatial distribution of the three observed clusters. For the background map legend see Figure 1. Boxplots representing (B) the mean latitude and (C) longitude for each cluster, presented with the post-hoc test results (a > b).
Climate variables revealed patterns of decreasing temperature from south to north and a northwest–southeast gradient of increasing precipitation (Supplementary Figure 1). Consequently, temperatures were significantly (F,2= 4.762; 0.012*) lower in cluster 2 (average annual temperature of 0.7°C) than in clusters 1 and 3 (average annual temperatures of 1.8 and 1.6 °C, respectively) (Figures 4A,B). Precipitation was significantly (F,2= 11.157; <0.001***) higher in cluster 1 (average annual precipitation 84.4 mm) than in clusters 2 and 3 (average annual precipitation 76.4 and 76.7 mm, respectively) (Figures 4C,D).
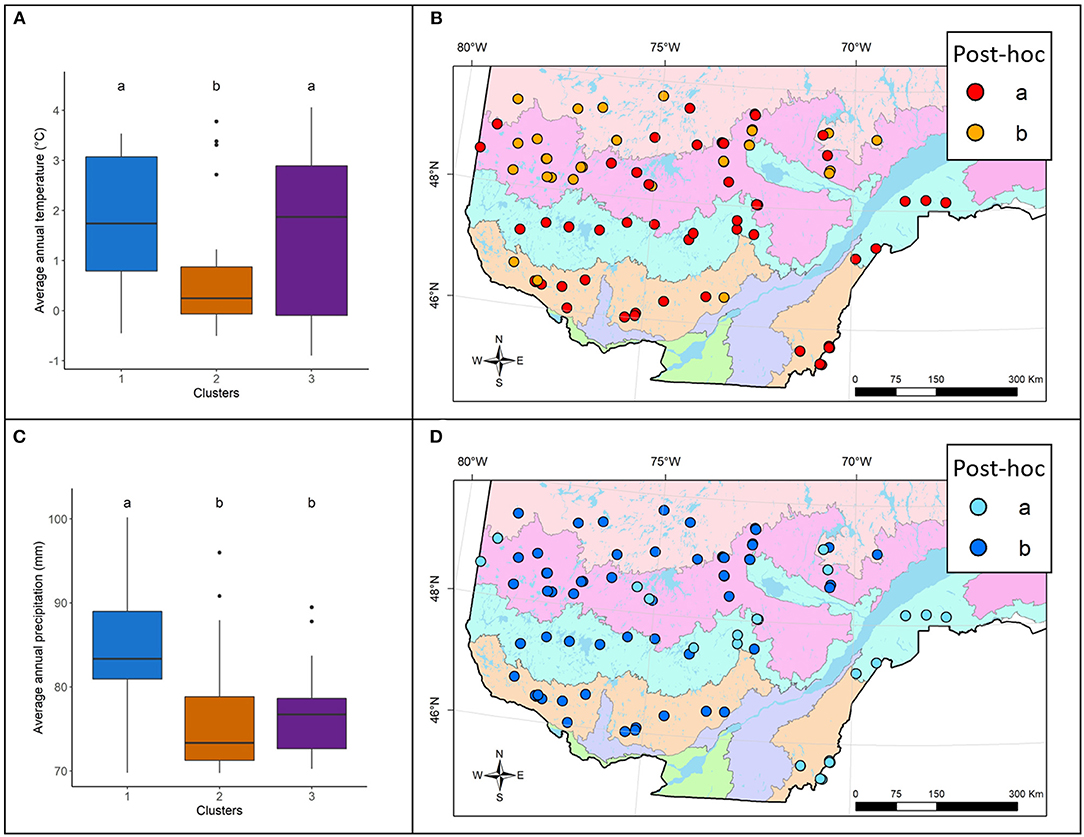
Figure 4. (A) Boxplot representing the mean annual temperature (°C) for each cluster presented with the post-hoc test results (a > b) and (B) maps showing these post-hoc results. (C) Boxplot representing the mean annual precipitation (mm) for each cluster presented with the post-hoc test results (a > b) and (D) maps showing these post hoc results. For the background map legend see Figure 1.
The onset date of the outbreaks did not differ between the clusters for all three outbreaks (Figure 5, Supplementary Figure 1); the three outbreaks of the 20th century began ca. 1909, 1939, and 1972. However, the end date for the outbreaks differed between clusters. Outbreak O1 ended significantly (F,2= 10.818; <0.001***) later for clusters 1 and 2 than for cluster 3. Outbreak O1 ended ca. 1926 for clusters 1 and 2 and ca. 1922 for cluster 3. The end date of the O2 outbreak did not differ significantly between clusters, ending ca. 1959, 1959, 1955 for clusters 1, 2, and 3, respectively. Outbreak O3 ended significantly (F,2 = 4,315; 0.019*) later for cluster 1 (ca. 1985) than for cluster 2 (ca. 1982) and ended at an intermediate date for cluster 3 (ca.1983).
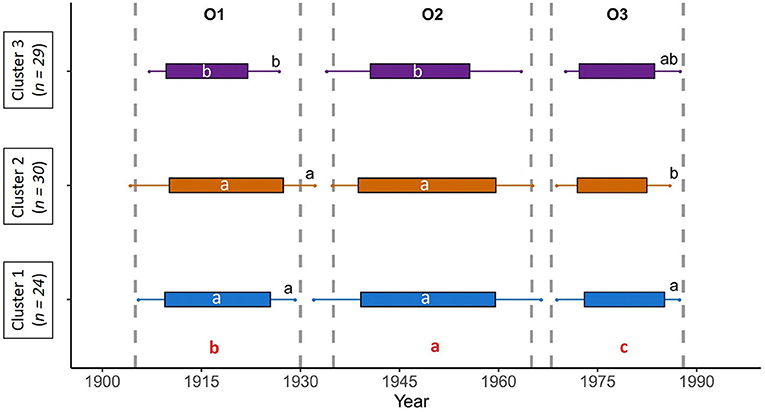
Figure 5. Gantt chart representing the timing of outbreaks for the three clusters. The boxes represent the average duration of outbreaks and the dashes represent the standard deviations of these durations for O1, O2, and O3 in each cluster. Different letters indicate significant differences at p < 0.05 between end dates (black letters) and duration (white letters) for each cluster (a > b). Red letters indicate significant differences in duration, at p < 0.05, between each outbreak (a > b > c). Dotted lines indicate the onset and end dates of each outbreak, according to Navarro et al. (2018).
The duration of the outbreaks also varied between clusters (Supplementary Figure 1). Outbreaks O1 (F,2= 5,673; 0.004**) and O2 (F,2 = 4,084; 0.021*) lasted longer for clusters 1 (16 and 20 years, respectively) and 2 (17 and 21 years, respectively) than for cluster 3 (12 and 15 years, respectively). We observed no difference in the duration of the O3 outbreak between clusters. The O3 outbreak lasted 12, 10, and 11 years for clusters 1, 2, and 3, respectively. For all clusters, the duration of the three outbreaks differed significantly (F,2 = 25.551; <0.001***). Outbreak O1 had a mean duration of 16 years, followed by O2, which lasted an average of 21 years—the longest outbreak of the century. Outbreak O3 lasted 11 years on average, the shortest outbreak of the 20th century. Considering the average duration of outbreaks and their standard deviations, the three clusters were more often in an outbreak state than in a non-outbreak state. Similarly, the time between outbreaks was very short, especially for C1 and C2.
Outbreak severity varied significantly between clusters (Table 2, Supplementary Figure 1). During outbreak O1, the percentage of affected trees was lower in cluster 2 (24.48% of trees affected on average) than in clusters 1 and 3 (36.15 and 33.15%, respectively), except when using the median as a measure (Table 2). During outbreak O2, all clusters shared a similar percentage of affected trees, with no observed significant differences, regardless of the considered parameters. Indeed, clusters 1, 2, and 3 had averages of 23.5, 26.1, and 24.2% affected trees, respectively. In contrast, the severity of outbreak O3 was greater in cluster 3 (37.5%) than cluster 2 (29.0%), with intermediate values in cluster 1 (33.6%). The within-cluster severity of outbreaks also varied (Table 3). Cluster 1 had similar yet more severe outbreaks in O1 and O3 (36.2 and 33.6%, respectively) than observed for outbreak O2 (23.5%). Outbreak severity within cluster 2 remained consistent over the century; outbreaks O1, O2, and O3 had respective percentages of affected trees of 24.5, 26.1, and 29.0%. Cluster 3, like cluster 1, had outbreak severities for O1 and O3 (33.2 and 37.5%, respectively) being significantly greater than that observed for outbreak O2 (24.2%).
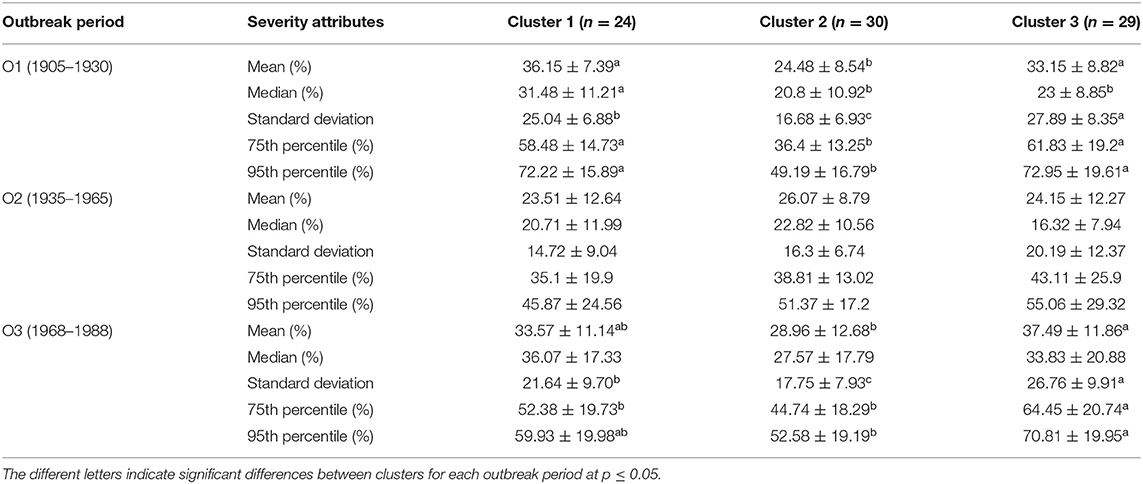
Table 2. Mean and standard deviation of severity attributes (mean, median, 75th and 95th percentile of the percentage of trees affected by SBW for each of study sites and outbreak period) between clusters for each SBW outbreak.
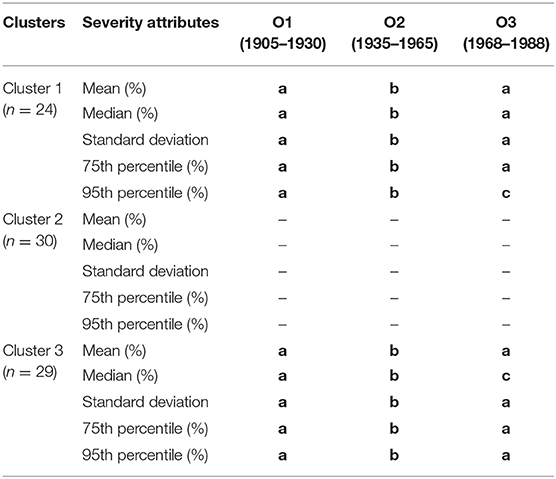
Table 3. Results of the pairwise comparisons of severity attributes (mean, median, 75th and 95th percentile of the percentage of trees affected by SBW for each of study sites and outbreak period) between the different SBW outbreaks per cluster, where a > b > c.
Discussion
In this study, we identified three spatiotemporal patterns of SBW disturbance during the 20th century in our study area. The three obtained clusters are defined by outbreaks of specific duration and severity, supporting our first hypothesis. Moreover, the three clusters are spatially distinct and subject to different climatic conditions, a finding that supports our second hypothesis.
SBW Outbreak Patterns Were Spatially and Climatically Distinct
We ran k-means clustering using the percentage of affected trees within a stand based on tree-ring chronologies. We observed three spatially distinct outbreak patterns. The majority of the stands constituting cluster 1 are located in eastern Quebec, east of the St. Lawrence River. The cluster 2 stands are mainly located in the northern reach of our study area in southern Quebec, and cluster 3 stands are mainly located along a southwest–northeast transect across the central part of southern Quebec. Admittedly, the resulting clusters also include isolated stands; for example, cluster 1 contained some outlier stands situated in western Quebec, and cluster 2 included some stands in southern Quebec. Nevertheless, the presence of stands having a location distinct from those stands making up the majority of a cluster is not incongruent given the methodology and the data used, i.e., the k-means clustering algorithm and dendrochronological data. Despite these few outliers, our approach was effective in improving the understanding of SBW outbreaks in space and time. Indeed, patterns similar to those of cluster 1, characterized by a high percentage of affected trees during outbreaks O1 and O3, were observed by Boulanger and Arseneault (2004) and Boulanger et al. (2012) in regional studies conducted south of the St. Lawrence River. Cluster 2 was characterized by outbreaks having similar percentages of affected trees, in agreement with reconstructions of past outbreaks north of Lac Saint-Jean (Morin and Laprise (1990). Similarly, the cluster 3 pattern, characterized by a moderate (O2) to high (O1 and O3) severity and by stands located at the heart of the insect's distribution, has been highlighted previously, in particular by Jardon et al. (2003) and Bouchard et al. (2006). Therefore, the use of k-mean clustering to identify large-scale SBW outbreak patterns produced spatially well-defined results that are consistent with earlier local-scale studies. Finally, the results of this study help to explain the differences in outbreaks patterns between local studies conducted in different locations. The recording of outbreak periods therefore depends on the spatial scale under consideration. It is likely that at a small spatial scale, recorded outbreaks are often shorter in duration because the insect changes location on the basis of available resources (Pureswaran et al., 2016). The shorter duration of outbreaks at a local scale may explain why, at the local level, outbreaks are generally considered as punctual events or as peaks of disturbance (Martin et al., 2019). At the provincial level, on the other hand, despite the presence of peaks in severity, outbreak periods appear continuous over time. Indeed, the continuous presence throughout the 20th century of at least a number of affected trees in Quebec suggests that SBW is an continuous disturbance agent across the province.
The identified spatial patterns corresponded to specific climatic conditions. Cluster 1 included stands having a higher average annual precipitation than those in clusters 2 and 3. The mean annual temperatures of stands in cluster 2 were significantly lower than for clusters 1 and 3. The climatic conditions of cluster 3 were intermediate between clusters 1 and 2. These results are generally consistent with large-scale patterns of Quebec climate. Villeneuve (1959) pointed out that although there is a humid temperate climate throughout southern Quebec, a more maritime climate can be observed in the St. Lawrence estuary, and a drier continental climate is present across the remainder of the province. This supports our results that show greater precipitation in cluster 1 (stands generally located in eastern Quebec) relative to the drier climate in the more continental clusters 2 and 3. However, Villeneuve (1959) also highlighted the existence of several climatic regions within the continental climate category, which occur because of the influence of inland water masses, latitude, and altitude. These climatic patterns match the spatial distribution of our two continental clusters; cluster 2 has colder temperatures than cluster 3. SBW is also a climate-dependent species (Greenbank, 1957; Williams and Liebhold, 1995, 1997, 2002; Pureswaran et al., 2015, 2019), and climatic conditions can both directly affect SBW, such as influencing survival, reproduction, and the displacement of populations, and indirectly affect SBW, including through changes in the upper (predators and parasites) and lower (host trees) trophic levels. As a result, the various outbreak patterns identified in our study can be clearly associated with various known climatic regions. Hence, our approach could discriminate SBW outbreak patterns on the basis of consistent climatic gradients.
Variability in Outbreak Severity and Timing Within the SBW Outbreak Patterns
The outbreaks of each cluster were defined by varying severities and timings. Nonetheless, we observed, for each cluster, three outbreak peaks—at the beginning, middle, and end of the century—and these three outbreak peaks correspond well to the three known outbreak periods of the 20th century (Blais, 1965; Jardon et al., 2003; Boulanger and Arseneault, 2004; Morin et al., 2007; Navarro et al., 2018). Yet, the severity of these outbreaks differed between clusters. In clusters 1 and 3, the severity of outbreaks O1 and O3 was significantly greater than that of outbreak O2. It is therefore likely that the severity of outbreak O1 led to a landscape characterized by a scarcity of mature host trees, thereby leading to a less severe O2 outbreak. As time passed, the abundance of mature trees and balsam fir progressively increased (MacLean, 1984; MacLean and Ostaff, 1989); as a result, the O3 outbreak was as severe as the O1 outbreak. Morin et al. (2007) highlighted this succession of alternating high-low-high severities for 20th-century outbreaks. Our results underscore that this alternating pattern of outbreak severity is common to large and continuous areas in Quebec for forest stands that have remained unburned for an extended period.
The severity of each outbreak also varied between clusters. The severity of the O1 outbreak was higher in clusters 1 and 3 than within cluster 2. For the less severe O2 outbreak, however, the severity was similar for each cluster. Finally, during the O3 outbreak, cluster 2 experienced a lower severity outbreak than clusters 1 and 3, which had outbreaks of intermediate severity. The pronounced severity of outbreaks O1 and O3 within clusters 1 and 3 likely stemmed from the greater abundance of mature host species, in contrast to cluster 2 stands, which are located further north in a zone characterized by a lower proportion of balsam fir (Bergeron et al., 1995; MacLean and MacKinnon, 1997). During outbreak O2, clusters 1 and 3 experienced lower severity outbreaks owing to the high-low-high alternating pattern of severity highlighted above. Cluster 2, having stands located further north, lies in an area having a higher proportion of black spruce. Black spruce is less responsive to SBW outbreaks than red and white spruce (Hennigar et al., 2008), and this difference in sensitivity could explain the lower severity of the O1 and O3 outbreaks observed within cluster 2. Nevertheless, we did not observe any significant differences in the severity of the O2 outbreak between clusters, which suggests that the proportion of black spruce in the landscape plays a smaller role in determining outbreak severity than balsam fir availability and climate.
The duration of the three outbreaks also varied. For all clusters, the O3 outbreak was significantly shorter than outbreaks O1 and O2. This reduced duration relates to the O3 outbreak, which occurred in the 1980s, representing the most severe outbreak ever recorded. This outbreak destroyed between 139 and 238 million m3 of timber on public lands in Quebec (Boulet et al., 1996). Its severity led to more a rapid defoliation and death of host individuals. The degree of severity of this outbreak can be linked to the insect's population dynamics. Explosive population growth of SBW over the entire territory causes extensive damage to host trees (Morris, 1963; Royama, 1984); however, this massive population increase leads, in turn, to the explosive growth of predator and parasite populations, leading to the crash of SBW populations.
In addition, the O2 outbreak was significantly longer than the O1 and O3 outbreaks. Noting that the O2 outbreak was also the least severe of the 20th century, this pattern of a long, less severe outbreak supports the hypothesis that a low outbreak severity is related to the population dynamics of the insect. A slow SBW population growth and a gradual dispersal across the territory causes predator and parasite populations to increase slowly as well, lengthening the duration of the outbreak. These duration-intensity relationships suggest least severe outbreaks are longer lasting and are in contrast to the short, intense outbreaks, such as outbreak O3. Our temporal analysis of outbreaks shows that, despite variations in severity, the three 20th-century outbreaks began synchronously in all clusters. Several authors have suggested that the 20th-century epidemics developed initially in the southern areas of mixed forest and then spread to the coniferous forests further north (Hardy et al., 1983, 1986; Gray et al., 1997). Others hypothesized that these outbreaks spread from southern regions, characterized by a high proportion of balsam fir, to northern regions, which have a lower proportion of balsam fir (Royama, 1984; Blais, 1985; Morin and Laprise, 1990; Morin et al., 1993). Our results refute this northward expansion hypothesis. Our findings indicate that the onset of all three outbreaks was synchronous over the entire study area. The synchronicity between the more northern stands in cluster 2 and the more southern stands in clusters 1 and 3 suggests that these more northerly SBW populations were already present at the turn of the century, as suggested previously (Morin, 1994; Morin and Laprise, 1997). These remote populations may result from the overflow of more southern populations toward the end of the 19th century (Royama, 1984; Blais, 1985; Morin et al., 1993).
The end of outbreaks and therefore their duration, however, vary from one cluster to another. O1 and O2 outbreaks were indeed longer in clusters 1 and 2 than in cluster 3. This longer duration can be explained by the relatively moderate climatic conditions (Greenbank, 1957) and the continuous presence of host species (Nealis and Régnière, 2004; Gray, 2007; Campbell, 2008) within the various bioclimatic domains represented by cluster 3. All these factors favor the insect and its wide dispersal. This pattern suggests that cluster 3 is spatially the most appropriate area for SBW outbreaks and that in cluster 3, SBW consumes resources faster and attains an outbreak peak earlier, thereby facilitating the rapid growth of predators and parasites, as well as depleting host trees; when combined, these factors precipitate the collapse of the insect population (Royama, 1984, 1992). Our results also indicate that for all clusters, the 1980s outbreak was shorter in duration than the two previous 20th-century outbreaks. Various authors have hypothesized that a tri-trophic relationship between host trees, insects and their natural enemies, and late 20th-century climate change explains this change in duration (Dale et al., 2001; Pureswaran et al., 2015). In addition, Dale et al. (2001) illustrate that climate change can lead to increases in insect populations and alter the intensity and frequency of outbreaks. This shift also produces several indirect effects, including the loss of host species and increased populations of insect predators. It is therefore possible that an increase in SBW populations owing to climate change contributed to the more rapid decline of insect host species during the O3 outbreak.
Overall, we highlighted in this study that the characteristics of SBW outbreaks are dependent on three factors: spatial location, climate, and forest composition (Gray, 2007). However, we used indirect data (i.e., reconstruction of outbreak patterns using dendrochronological data), which means that we are unable to reconstruct in detail the specific local climate, vegetation, and stand structure at the time of the various outbreaks. Hence, the direct influence of these factors requires further assessment; in particular, it demands a better understanding of the influence of stand characteristics on outbreaks.The identification of those factors most strongly related to the obtained clusters would (i) help identify the local environmental, ecological, and climatic conditions able to explain the obtained patterns of duration, severity, and timing, and (ii) test hypotheses related to the dynamics of insect populations at several space and time scales. Moreover, studying these local factors would bolster predictions of the effects of changes in insect distribution on forest stands and the associated ecological resources.
Data Availability Statement
The datasets presented in this study can be found in online repositories. The names of the repository/repositories and accession number(s) can be found in the article/Supplementary Material.
Author Contributions
CB and HM conceptualized and investigated the study. CB carried out the dendrochronological data collection and analysis. HM provided the necessary material resources and contributed to the funding. CB and MM carried out the statistical analyses, wrote the initial draft of the manuscript, and edited the manuscript. HM and DA supervised the study. CB, MM, HM, and DA reviewed the manuscript. All authors contributed to the article and approved the submitted version.
Funding
Funding for the study was provided by the Industrial Research Chair–CRSNG for black spruce growth and the influence of the spruce budworm on landscape variability in the boreal zone.
Conflict of Interest
The authors declare that the research was conducted in the absence of any commercial or financial relationships that could be construed as a potential conflict of interest.
Acknowledgments
We thank E. Dussaut Chouinard, J.-P. Labrecque Foy, C. Pageau, and J.-G. Girard for their assistance in tree-core sampling. We also thank J.-P. Labrecque Foy, J. Lavoie, L. Savard, and B. Maurin for their assistance with both the dendrochronological preparation and measurements of growth rings. We would like to thank F. Guibal for taking part in the proofreading of this manuscript and for his enlightened comments. We thank M. Hay for verifying the English in the text.
Supplementary Material
The Supplementary Material for this article can be found online at: https://www.frontiersin.org/articles/10.3389/fevo.2020.544088/full#supplementary-material
References
Anderson, M. J. (2001). A new method for non-parametric multivariate analysis of variance. Austral. Ecol. 26, 32–46. doi: 10.1111/j.1442-9993.2001.01070.pp.x
Arbizu, M. (2017). Pairwiseadonis: Pairwise Multilevel Comparison Using Adonis. R Package, version 0.0.
Bergeron, Y., and Dansereau, P. R. (1993). Predicting the composition of canadian southern boreal forest in different fire cycles. J. Vegetation Sci. 4, 827–832. doi: 10.2307/3235621
Bergeron, Y., Leduc, A., Joyal, C., and Morin, H. (1995). Balsam fir mortality following the last spruce budworm outbreak in northwestern quebec. Can. J. For. Res. 25, 1375–1384. doi: 10.1139/x95-150
Blais, J. (1957). Some relationships of the spruce budworm, Choristoneura fumiferana (Clem.) to black spruce, Picea mariana (Moench) voss. For. Chron. 33, 364–372. doi: 10.5558/tfc33364-4
Blais, J. (1961). Spruce budworm outbreaks in the lower St. Lawrence and Gaspé regions. For. Chron. 37, 192–202. doi: 10.5558/tfc37192-3
Blais, J. (1962). Collection and analysis of radial-growth data from trees for evidence of past spruce budworm outbreaks. For. Chron. 38, 474–484. doi: 10.5558/tfc38474-4
Blais, J. (1965). Spruce budworm outbreaks in the past three centuries in the Laurentide Park, Quebec. For. Sci. 11, 130–138.
Blais, J. (1981). Mortality of balsam fir and white spruce following a spruce budworm outbreak in the ottawa river watershed in Quebec. Can. J. For. Res. 11, 620–629. doi: 10.1139/x81-085
Blais, J. (1985). The ecology of the eastern spruce budworm: a review and discussion. For. Chron. 61, 494–498. doi: 10.5558/tfc61494-6
Bouchard, M., Kneeshaw, D., and Bergeron, Y. (2006). Forest dynamics after successive spruce budworm outbreaks in mixedwood forests. Ecology 87, 2319–2329. doi: 10.1890/0012-9658(2006)87[2319:FDASSB]2.0.CO;2
Bouchard, M., and Pothier, D. (2010). Spatiotemporal variability in tree and stand mortality caused by spruce budworm outbreaks in eastern Quebec. Can. J. For. Res. 40, 86–94. doi: 10.1139/X09-178
Boulanger, Y., and Arseneault, D. (2004). Spruce budworm outbreaks in eastern Quebec over the last 450 years. Can. J. For. Res. 34, 1035–1043. doi: 10.1139/x03-269
Boulanger, Y., Arseneault, D., Morin, H., Jardon, Y., Bertrand, P., and Dagneau, C. (2012). Dendrochronological reconstruction of spruce budworm (Choristoneura fumiferana) outbreaks in southern Quebec for the last 400 years. Can. J. For. Res. 42, 1264–1276. doi: 10.1139/x2012-069
Boulet, B., Chabot, M., Dorais, L., Dupont, A., Gagnon, R., and Morneau, L. (1996). Entomologie Forestière. Manuel de Foresterie. Sainte-Foy, Québec: Les Presses de l'Université Laval. 1008–1043.
Campbell, E. M. (2008). Patrons temporels et spatiaux de la sévérité des épidémies de la tordeuse des bourgeons de l'épinette en relation avec les conditions bioclimatiques dans l'est du Canada. (Ph.D. dissertation), Montreal, QC: Université du Québec à Montréal.
Cook, E. R., and Peters, K. (1981). The smoothing spline: a new approach to standardizing forest interior tree-ring width series for dendroclimatic studies. Tree Ring Bull. 41, 45–53.
Dale, V. H., Joyce, L. A., McNulty, S., Neilson, R. P., Ayres, M. P., Flannigan, M. D., et al. (2001). Climate change and forest disturbances: climate change can affect forests by altering the frequency, intensity, duration, and timing of fire, drought, introduced species, insect and pathogen outbreaks, hurricanes, windstorms, ice storms, or landslides. BioScience 51, 723–734. doi: 10.1641/0006-3568(2001)051[0723:CCAFD]2.0.CO;2
De Grandpré, L., Waldron, K., Bouchard, M., Gauthier, S., Beaudet, M., Ruel, J.-C., et al. (2018). Incorporating insect and wind disturbances in a natural disturbance-based management framework for the boreal forest. Forests 9:471. doi: 10.3390/f9080471
Dimitriadou, E., Dolničar, S., and Weingessel, A. (2002). An examination of indexes for determining the number of clusters in binary data sets. Psychometrika 67, 137–159. doi: 10.1007/BF02294713
Dolnicar, S., Grabler, K., and Mazanec, J. A. (2000). Analyzing destination images: a perceptual charting approach. J. Travel Tourism Market. 8, 43–57. doi: 10.1300/J073v08n04_03
Filion, L., Payette, S., Delwaide, A., and Bhiry, N. (1998). Insect defoliators as major disturbance factors in the high-altitude balsam fir forest of mount mégantic, southern Quebec. Can. J. For. Res. 28, 1832–1842. doi: 10.1139/x98-161
Fraver, S., Seymour, R. S., Speer, J. H., and White, A. S. (2007). Dendrochronological reconstruction of spruce budworm outbreaks in northern Maine, USA. Can. J. For. Res. 37, 523–529. doi: 10.1139/X06-251
Gray, D. R. (2007). The relationship between climate and outbreak characteristics of the spruce budworm in eastern Canada. Clim. Change 87, 361–383. doi: 10.1007/s10584-007-9317-5
Gray, D. R., Régnière, J., and Boulet, B. (1997). ). “A spatial analysis of the ESBW historical data of Quebec”, in Proceedings of the 17th Eastern Spruce Budworm Research Work Conference (Québec: Rep. LAU-X-113B).
Gray, D. R., Régnière, J., and Boulet, B. (2000). Analysis and use of historical patterns of spruce budworm defoliation to forecast outbreak patterns in Quebec. For. Ecol. Manage. 127, 217–231. doi: 10.1016/S0378-1127(99)00134-6
Greenbank, D. (1957). The role of climate and dispersal in the initiation of outbreaks of the spruce budworm in New Brunswick: II. the role of dispersal. Can. J. Zool. 35, 385–403. doi: 10.1139/z57-029
Hardy, Y., Lafond, A., and Hamel, L. (1983). The epidemiology of the current spruce budworm outbreak in Quebec. For. Sci. 29, 715–725.
Hardy, Y., Mainville, M., and Schmitt, D. (1986). An Atlas of Spruce Budworm Defoliation in Eastern North America, 1938–1980 (USDA; Miscellaneous Publication).
Harris, I., Jones, P. D., Osborn, T. J., and Lister, D. H. (2014). Updated high-resolution grids of monthly climatic observations–the CRU TS3. 10 dataset. Int. J. Climatol. 34, 623–642. doi: 10.1002/joc.3711
Hartigan, J. A., and Wong, M. A. (1979). Algorithm AS 136: A k-means clustering algorithm. J. R. Stat. Soc. C 28, 100–108. doi: 10.2307/2346830
Hennigar, C. R., MacLean, D. A., Quiring, D. T., and Kershaw, J. A. Jr. (2008). Differences in spruce budworm defoliation among balsam fir and white, red, and black spruce. For. Sci. 54, 158–166. doi: 10.1093/forestscience/54.2.158
Holmes, R., and Swetnam, T. (1996). Program OUTBREAK Users Manual. Laboratory of Tree-Ring Research. University of Arizona: Tucson.
Jardon, Y. (2001). Analyses temporelles et spatiales des épidémies de la tordeuse des bourgeons de l'épinette au Québec. (Ph.D. dissertation), Montreal, QC: Université du Québec à Montréal.
Jardon, Y., Morin, H., and Dutilleul, P. (2003). Périodicité et synchronisme des épidémies de la tordeuse des bourgeons de l'épinette au Québec. Can. J. For. Res. 33, 1947–1961. doi: 10.1139/x03-108
Jobidon, R., Bergeron, Y., Robitaille, A., Raulier, F., Gauthier, S., Imbeau, L., et al. (2015). A biophysical approach to delineate a northern limit to commercial forestry: the case of Quebec's boreal forest. Can. J. For. Res. 45, 515–528. doi: 10.1139/cjfr-2014-0260
Kneeshaw, D. D., and Bergeron, Y. (1998). Canopy gap characteristics and tree replacement in the southeastern boreal forest. Ecology 79, 783–794. doi: 10.1890/0012-9658(1998)079[0783:CGCATR]2.0.CO;2
Krause, C. (1997). The use of dendrochronological material from buildings to get information about past spruce budworm outbreaks. Can. J. For. Res. 27, 69–75. doi: 10.1139/x96-168
MacLean, D. A. (1980). Vulnerability of fir-spruce stands during uncontrolled spruce budworm outbreaks: a review and discussion. For. Chron. 56, 213–221. doi: 10.5558/tfc56213-5
MacLean, D. A. (1984). Effects of spruce budworm outbreaks on the productivity and stability of balsam fir forests. For. Chron. 60, 273–279. doi: 10.5558/tfc60273-5
MacLean, D. A., and MacKinnon, W. E. (1997). Effects of stand and site characteristics on susceptibility and vulnerability of balsam fir and spruce to spruce budworm in New Brunswick. Can. J. For. Res. 27, 1859–1871. doi: 10.1139/x97-146
MacLean, D. A., and Ostaff, D. P. (1989). Patterns of balsam fir mortality caused by an uncontrolled spruce budworm outbreak. Can. J. For. Res. 19, 1087–1095. doi: 10.1139/x89-165
Martin, M., Fenton, N., and Morin, H. (2018). Structural diversity and dynamics of boreal old-growth forests case study in Eastern Canada. For. Ecol. Manage. 422, 125–136. doi: 10.1016/j.foreco.2018.04.007
Martin, M., Krause, C., Fenton, N. J., and Morin, H. (2020a). Unveiling the diversity of tree growth patterns in boreal old-growth forests reveals the richness of their dynamics. Forests 11:252. doi: 10.3390/f11030252
Martin, M., Krause, C., and Morin, H. (2020b). Linking radial growth patterns and moderate-severity disturbance dynamics in boreal old-growth forests driven by recurrent insect outbreaks: a tale of opportunities, successes, and failures. Ecol. Evol. 1–21. doi: 10.1002/ece3.7080
Martin, M., Morin, H., and Fenton, N. J. (2019). Secondary disturbances of low and moderate severity drive the dynamics of eastern Canadian boreal old-growth forests. Ann. For. Sci. 76:108. doi: 10.1007/s13595-019-0891-2
Morin, H. (1994). Dynamics of balsam fir forests in relation to spruce budworm outbreaks in the boreal zone of Quebec. Can. J. For. Res. 24, 730–741. doi: 10.1139/x94-097
Morin, H., Jardon, Y., and Gagnon, R. (2007). “Relationship between spruce budworm outbreaks and forest dynamics in eastern North America,” in Plant Disturbance Ecology the Process and the Response, eds E. A. Johnson and K. Miyanishi (Amsterdam: Academic Press), 555–564. doi: 10.1016/B978-012088778-1/50019-4
Morin, H., Jardon, Y., Simard, S., Payette, S., and Filion, L. (2010). “Détection et reconstitution des épidémies de la tordeuse des bourgeons de l'épinete (Choristoneura fumiferana) à l'aide de la dendrochronologie,” in La dendroécologie. Principes, Méthodes et Applications, eds S. Payette and L. Filion (Québec: Presses de l'université Laval), 415–436.
Morin, H., and Laprise, D. (1990). Histoire récente des épidémies de la tordeuse des bourgeons de l'épinette au nord du lac Saint-Jean (Québec): une analyse dendrochronologique. Can. J. For. Res. 20, 1–8. doi: 10.1139/x90-001
Morin, H., and Laprise, D. (1997). Seedling bank dynamics in boreal balsam fir forests. Can. J. For. Res. 27, 1442–1451. doi: 10.1139/x97-113
Morin, H., Laprise, D., and Bergeron, Y. (1993). Chronology of spruce budworm outbreaks near lake duparquet, abitibi region, Quebec. Can. J. For. Res. 23, 1497–1506. doi: 10.1139/x93-189
Morris, R. F. (1963). The dynamics of epidemic spruce budworm populations. Memoirs Entomol. Soc. Canada 95, 1–12. doi: 10.4039/entm9531fv
Moussaoui, L., Leduc, A., Fenton, N. J., Lafleur, B., and Bergeron, Y. (2019). Changes in forest structure along a chronosequence in the black spruce boreal forest: identifying structures to be reproduced through silvicultural practices. Ecol. Indic. 97, 89–99. doi: 10.1016/j.ecolind.2018.09.059
Navarro, L., Morin, H., Bergeron, Y., and Girona, M. M. (2018). Changes in spatiotemporal patterns of 20th century spruce budworm outbreaks in eastern Canadian boreal forests. Front. Plant Sci. 9:1905. doi: 10.3389/fpls.2018.01905
Nealis, V. G., and Régnière, J. (2004). Insect host relationships influencing disturbance by the spruce budworm in a boreal mixedwood forest. Can. J. For. Res. 34, 1870–1882. doi: 10.1139/x04-061
Oksanen, J., Kindt, R., Legendre, P., O'Hara, B., Stevens, M. H. H., Oksanen, M. J., et al. (2007). The vegan package. Commun. Ecol. Package 10, 631–637.
Pureswaran, D. S., De Grandpré, L., Paré, D., Taylor, A., Barrette, M., Morin, H., et al. (2015). Climate-induced changes in host tree-insect phenology may drive ecological state-shift in boreal forests. Ecology 96, 1480–1491. doi: 10.1890/13-2366.1
Pureswaran, D. S., Johns, R., Heard, S. B., and Quiring, D. (2016). Paradigms in eastern spruce budworm (Lepidoptera: Tortricidae) population ecology: a century of debate. Environ. Entomol. 45, 1333–1342. doi: 10.1093/ee/nvw103
Pureswaran, D. S., Neau, M., Marchand, M., De Grandpré, L., and Kneeshaw, D. (2019). Phenological synchrony between eastern spruce budworm and its host trees increases with warmer temperatures in the boreal forest. Ecol. Evol. 9, 576–586. doi: 10.1002/ece3.4779
R Development Core Team (2019). A Language and Environment for Statistical Computing. Vienna, Austria: R Foundation for Statistical Computing 2012. Available online at: https://www.R-project.org (accessed August 12, 2020).
Régnière, J., St-Amant, R., and Duval, P. (2012). Predicting insect distributions under climate change from physiological responses: spruce budworm as an example. Biol. Invasions 14, 1571–1586. doi: 10.1007/s10530-010-9918-1
Reichenbach, N. G., and Stairs, G. R. (1984). Response of the western spruce budworm (Lepidoptera: Tortricidae) to temperature and humidity: developmental rates and survivorship. Environ. Entomol. 13, 611–618. doi: 10.1093/ee/13.2.611
Richard, P. (1987). “Le couvert végétal au Québec-Labrador et son histoire postglaciaire,” in Le Naturaliste Canadien (Montréal: Département de géographie, Université de Montréal).
Royama, T. (1992). Analytical Population Dynamics. New York, NY: Chapman Hall, 371. doi: 10.1007/978-94-011-2916-9
Royama, T.-O. (1984). Population dynamics of the spruce budworm Choristoneura fumiferana. Ecol. Monogr. 54, 429–462. doi: 10.2307/1942595
Saucier, J.-P., Gosselin, J., Morneau, C., and Grondin, P. (2010). Utilisation de la classification de la végétation dans l'aménagement forestier au Québec. Revue For. Française LXII−3–4, 428–438. doi: 10.4267/2042/38956
Tremblay, M.-J., Rossi, S., and Morin, H. (2011). Growth dynamics of black spruce in stands located between the 51st and 52nd parallels in the boreal forest of Quebec, Canada. Can. J. For. Res. 41, 1769–1778. doi: 10.1139/x11-094
Villeneuve, G.-O. (1959). Bref aperçu climatique du Québec méridional. Cahiers Géogr. Québec 3, 153–162. doi: 10.7202/020175ar
Vincent, L. A., and Gullett, D. (1999). Canadian historical and homogeneous temperature datasets for climate change analyses. Int. J. Climatol. 19, 1375–1388. doi: 10.1002/(SICI)1097-0088(199910)19:12<1375::AID-JOC427>3.0.CO;2-0
Volney, W. J. A., and Fleming, R. A. (2000). Climate change and impacts of boreal forest insects. Agric. Ecosyst. Environ. 82, 283–294. doi: 10.1016/S0167-8809(00)00232-2
Volney, W. J. A., and Fleming, R. A. (2007). Spruce budworm (Choristoneura spp.) biotype reactions to forest and climate characteristics. Glob. Chang. Biol. 13, 1630–1643. doi: 10.1111/j.1365-2486.2007.01402.x
Williams, D., and Liebhold, A. (1997). Latitudinal shifts in spruce budworm (Lepidoptera: Tortricidae) outbreaks and spruce-fir forest distrbutions with climate change. Acta Phytopathol. Entomol. Hung 32, 205–215.
Williams, D. W., and Liebhold, A. M. (1995). Forest defoliators and climatic change: potential changes in spatial distribution of outbreaks of western spruce budworm (Lepidoptera: Tortricidae) and gypsy moth (Lepidoptera: Lymantriidae). Environ. Entomol. 24, 1–9. doi: 10.1093/ee/24.1.1
Williams, D. W., and Liebhold, A. M. (2002). Climate change and the outbreak ranges of two North American bark beetles. Agric. For. Entomol. 4, 87–99. doi: 10.1046/j.1461-9563.2002.00124.x
Keywords: insect outbreaks, spatiotemporal dynamics, disturbance severity, dendrochronology, multiscale interactions, climatic conditions, Choristoneura fumiferana (Clem.)
Citation: Berguet C, Martin M, Arseneault D and Morin H (2021) Spatiotemporal Dynamics of 20th-Century Spruce Budworm Outbreaks in Eastern Canada: Three Distinct Patterns of Outbreak Severity. Front. Ecol. Evol. 8:544088. doi: 10.3389/fevo.2020.544088
Received: 19 March 2020; Accepted: 09 December 2020;
Published: 07 January 2021.
Edited by:
Scott Andrew Mensing, University of Nevada, Reno, United StatesReviewed by:
Bo Zhang, University of New Brunswick Fredericton, CanadaAaron Weiskittel, University of Maine, United States
Copyright © 2021 Berguet, Martin, Arseneault and Morin. This is an open-access article distributed under the terms of the Creative Commons Attribution License (CC BY). The use, distribution or reproduction in other forums is permitted, provided the original author(s) and the copyright owner(s) are credited and that the original publication in this journal is cited, in accordance with accepted academic practice. No use, distribution or reproduction is permitted which does not comply with these terms.
*Correspondence: Cassy Berguet, Y2Fzc3kuYmVyZ3VldDEmI3gwMDA0MDt1cWFjLmNh