- 1Florida Medical Entomology Laboratory, Institute of Food and Agricultural Sciences, University of Florida, Vero Beach, FL, United States
- 2Polk County Mosquito Control Program, Polk County Board of County Commissioners, Bartow, FL, United States
- 3Center for Global Health Infectious Disease Research, University of South Florida, Tampa, FL, United States
Vectorial capacity is an equation that integrates the major aspects of vector biology to predict the number of new mosquito-borne disease infections. Developed for studying transmission of malaria, vectorial capacity is rarely applied to zoonotic vector-borne diseases and is not often adjusted to account for seasonal changes in vector ecology. We used field data from Florida, United States, to expand the understanding of how vectorial capacity of Culiseta melanura (Coquillett), the primary enzootic vector of eastern equine encephalitis virus (EEEV), changes seasonally and its effect on EEEV risk. We determined parity via dissection and identified bloodmeals by PCR for field-collected Cs. melanura females from Central Florida. We used density of the vector, proportion of avian hosts fed upon, parity state of the vector, and mean temperature of the study area to quantify vectorial capacity as a function of season. The calculated values of vectorial capacity shifted significantly with season, with highest values observed in the summer with an additional peak in December. Linear regression revealed a strong positive correlation between vectorial capacity values and Florida EEEV equine cases in 2018, as well as cases reported during the last decade. The relationship between virus infections in equids and vectorial capacity lends support to the large effect that enzootic transmission has on epizootic outbreaks of zoonotic vector-borne pathogens.
Introduction
The ecology of zoonotic vector-borne pathogens is inherently complex, given the diversity of vectors and/or multiple hosts that are involved in their transmission (Kuno et al., 2017). Host, vector, and pathogen populations interact within the physical environment (e.g., vegetation, climate, season), such that pathogen transmission is driven by overlapping spatial-temporal distributions of hosts and vectors (Reisen, 2010). Quantifying the influence of key biological and environmental variables and mathematically integrating their relationships is useful for understanding the relative importance of each component in the spread of the pathogen (Smith et al., 2014) and illuminates opportunities for targeted interventions to disrupt transmission (Garrett-Jones, 1964b; Brady et al., 2016). Vectorial capacity (C) is an equation that accounts for the major factors of pathogen transmission by mosquitoes and is defined as the average number of new vertebrate infections per day resulting from an initial index case (Garrett-Jones, 1964b). The origins of this equation are rooted in efforts to better understand Plasmodium falciparum (human malaria parasite) transmission and assess the effectiveness of malaria control in sub-Saharan Africa (Smith et al., 2014). Nevertheless, with modifications vectorial capacity can be applied to zoonotic vector-borne disease systems and is especially relevant to pathogens vectored by mosquitoes or insects with similar life cycles (Macdonald, 1957; Garrett-Jones and Shidrawi, 1969; Smith et al., 2014). Vectorial capacity is calculated by equation 1, where ma represents the man-biting rate, a is the man-biting habit, p is the vector’s daily probability of survival, n is the extrinsic incubation period of the pathogen, and v is the vector competence (Macdonald, 1957; Garrett-Jones, 1964b):
The man-biting rate (ma) for transmission cycles in which humans are the primary host (e.g., malaria) can be estimated by human landing catch (Garrett-Jones, 1964a; Almeida et al., 2005). Because non-human animals serve as amplifying hosts of zoonotic mosquito-borne pathogens, estimating mosquito density and biting rate from human landing catches is not relevant. The parameter a also presents challenges, as it is the product of the host bloodmeal index and the feeding frequency. Polymerase chain reaction (PCR)-based methods enable measuring the former, while the latter is often estimated as the inverse of the length of the gonotrophic cycle.
Despite its potential utility in understanding zoonotic vector-borne disease, few studies of zoonotic pathogens are able to measure all of the components of vectorial capacity (Dye, 1986). As a consequence, the relationship between vectorial capacity and spillover of zoonotic mosquito-borne disease is not fully established.
Eastern equine encephalitis virus (EEEV; family Togaviridae, genus Alphavirus) is a vector-borne pathogen found in the eastern United States that causes severe disease in humans, with high case fatality rates in symptomatic persons (Bigler et al., 1976). The mosquito Culiseta melanura (Coquillett) (Diptera: Culicidae) is the primary enzootic vector of EEEV throughout eastern North America (Howard and Wallis, 1974), although a number of other species serve as bridge vectors (Armstrong and Andreadis, 2010). The distribution of Cs. melanura closely matches that of EEEV, occurring along the eastern coast of the United States and stretching west to Kansas (Darsie and Ward, 2005). Virus infection in this species has been recorded from transmission foci in many states, including Maine (Lubelczyk et al., 2013), Vermont (Molaei et al., 2015b), Connecticut (Armstrong and Andreadis, 2010), Maryland (Saugstad et al., 1972), Virginia (Molaei et al., 2015a), Alabama (Cupp et al., 2003), and Florida (Bingham et al., 2014). Culiseta melanura is an efficient biological vector of the virus (Howard and Wallis, 1974; Scott and Burrage, 1984; Vaidyanathan et al., 1997), and EEEV disseminates rapidly in this species, with biological transmission occurring in as few as 3 days at 25–30°C (Scott and Burrage, 1984). In addition, many bird species that are commonly bitten by Cs. melanura serve as amplifying hosts for the virus throughout its range (Figure 1). American robin and northern cardinal were the first or second most frequently bitten avian hosts (Figure 1) in 10 independent PCR-based studies of Cs. melanura host use, spanning seven eastern states (Molaei et al., 2006, 2013, 2015a,b, 2016; Estep et al., 2011; Bingham et al., 2014; Burkett-Cadena et al., 2015; Blosser et al., 2017; West et al., 2020). Transmission of EEEV in the United States is seasonal, and epizootic activity primarily occurs in the summer months and declines in autumn (Tenbroeck et al., 1935; Bigler et al., 1976; Scott and Weaver, 1989). In temperate regions, the first heavy frost in November or December ends virus transmission in mosquitoes (Scott and Weaver, 1989). Cases in equids can also occur during winter in the southern United States because of its warmer climate (Bigler et al., 1976; Bingham et al., 2015; Burkett-Cadena et al., 2015). In southern states, mosquitoes can be present year-round and are thought to maintain EEEV transmission in the enzootic cycle even when epizootic transmission is not observed (Burkett-Cadena et al., 2015). In Florida, reported equine cases are sporadic and occur in isolated foci (Day and Shaman, 2011). Sixty-six percent of EEEV equine cases in Florida from 2009 to 2018 were reported in summer (Florida Department of Health, 2019). Although yearly number of cases may be low in some years and regions of Florida, horse cases are the most reliable indicator of virus activity in the southeast (Bigler et al., 1976). The seroconversions of sentinel chicken flocks that are used for detection of arboviruses have a similar trend of summer (May–September) transmission in Florida (Heberlein-Larson et al., 2019). The summer peak in transmission also coincides with increased mosquito abundance and EEEV antibody seroprevalence in young birds (Tenbroeck et al., 1935; Elias et al., 2017).
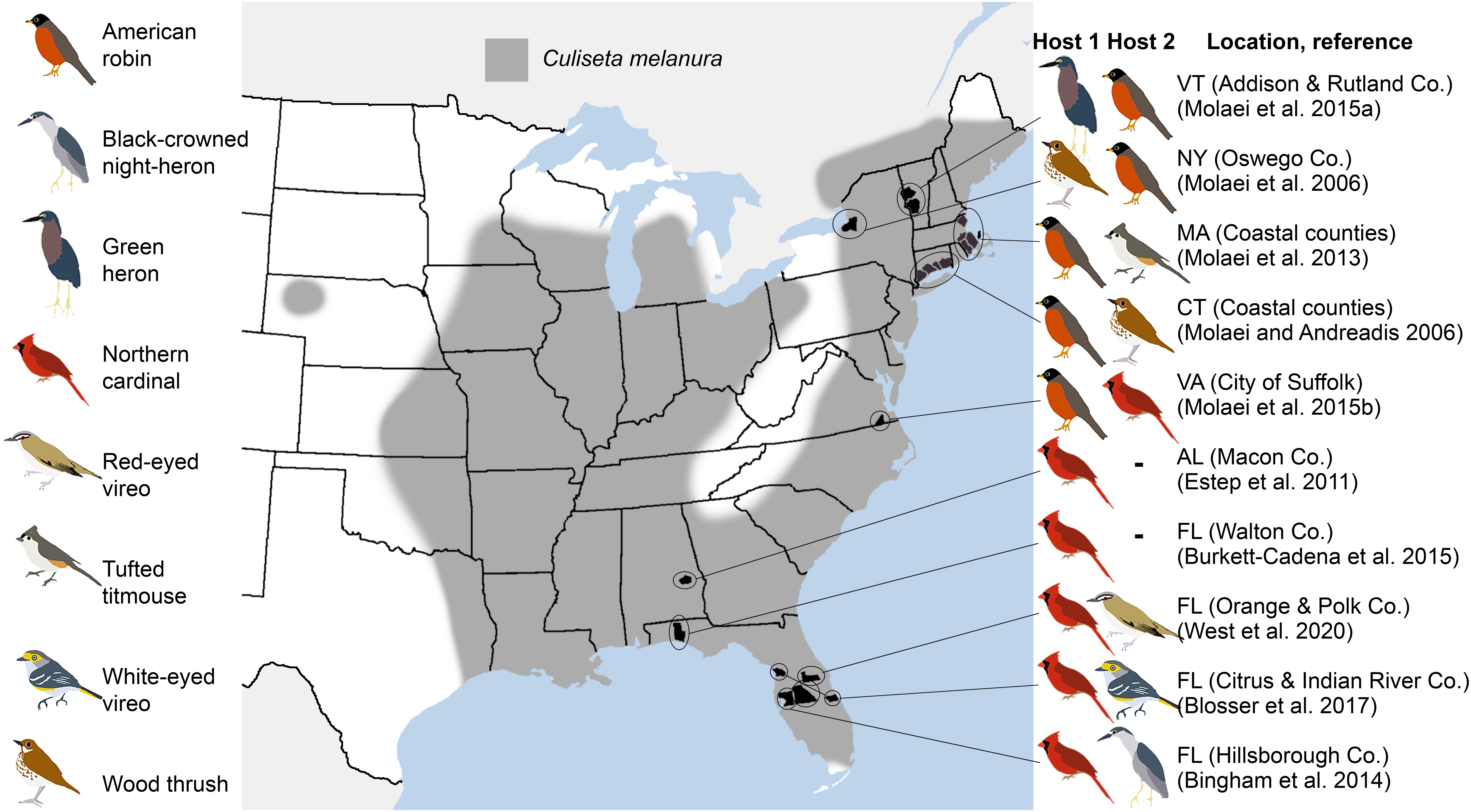
Figure 1. Geographical patterns of avian host use by Cs. melanura. The first and second most commonly bitten avian hosts are presented for PCR-based studies of bloodmeal analysis of Cs. melanura in North America. Distribution of Cs. melanura after Darsie and Ward (2005). Dash represents several equally fed upon secondary avian hosts.
The epizootic transmission of EEEV in Florida fluctuates from year to year and varies in severity between regions within the state of Florida. A large and widespread equine epidemic occurred in 2003 with 207 confirmed cases. Between 2004 and 2018, there were 6 years with fewer than 30 equine cases and 4 years with more than 90 equine cases. In the past decade (2009–2018), 41.4 mean equine cases per year were reported in Florida. Equine cases typically peak in June (mean = 11.5 cases) and are lowest in February (mean = 0.7 cases).
The goal of this study was to quantify vectorial capacity (C) of Cs. melanura for EEEV as a function of season in Central Florida, by measuring Cs. melanura abundance, avian host use, and parity throughout the year. These variables were incorporated into the vectorial capacity equation using temperature data and published data on extrinsic incubation period (n) and duration of gonotrophic cycle (gc) for this species. Importantly, although gc is not often included as a parameter of vectorial capacity, it is a biologically meaningful value that can be used to estimate a and p from field data. Quantifying vectorial capacity of Cs. melanura and comparing values with EEEV cases in equines will expand the knowledge of how Cs. melanura ecology affects EEEV transmission.
Materials and Methods
Sampling was conducted in Central Florida with six sites in Orange (3) and Polk counties (3), with evidence of prior EEEV activity (Table 1). Weekly mosquito collections were made at these six sites from January 2018 to December 2018 using six artificial resting shelters at each site for a total of 36 resting sites. Resting shelters serve as dark, protected refuges that attract blood-engorged females seeking a place to rest and digest their meals and are an effective method to collect blood-fed Cs. melanura females (Bingham et al., 2014; Blosser et al., 2017). The resting shelters used were made by placing a fitted black trash compactor bag over a cylindrical frame made with PVC pipes (Burkett-Cadena et al., 2019; Supplementary Video S1). Mosquitoes were collected by aspiration with a modified vacuum (BDH1800S Ni-Cd 18V Dustbuster, Black & Decker, MD) and collection cup (BioQuip Products, Rancho Dominguez, CA) (Blosser et al., 2017; Supplementary Video S2). Collected female mosquitoes were identified to species by examining morphological traits using a dissecting microscope and updated dichotomous keys (Darsie and Morris, 2003; Darsie and Ward, 2005; Burkett-Cadena, 2013). Samples were stored in Thermo Scientific Microcentrifuge tubes at −20°C for subsequent parity and bloodmeal analysis. Field staff conducting this research used appropriate protective clothing (long sleeves, pants) and repellent to minimize risks associated with EEEV infection.
After mosquitoes were sorted and identified, unfed or freshly blood-fed Cs. melanura females were dissected to determine parity. To make this diagnosis, ovaries were extracted into a drop of water on a slide and left to dry, making the tracheoles visible for inspection (Detinova, 1962). This method was tested on Aedes vigilax (Skuse) for accuracy and was 83.7–89.8% reliable (Hugo et al., 2014). Culiseta melanura females whose parity could not be determined were excluded from the total for the parity calculation.
The hosts fed on by Cs. melanura were previously determined by bloodmeal analysis consisting of DNA extraction, PCR, and sequencing (West et al., 2020). Extraction was performed using InstaGene Matrix (Bio-Rad, Hercules, CA, United States) followed by PCR assays using a series of five primer pairs to amplify vertebrate host bloodmeals in a series of PCRs as previously described. Details regarding primer sequences, PCR mixtures, and cycling conditions are provided in West et al. (2020).
Vectorial Capacity Calculations
The vectorial capacity values per month were calculated following Macdonald (1957) with modifications including adjustments for density and biting rate of an ornithophilic mosquito species, calculating p for a wild field population, and incorporating the monthly temperature of the study area. With the assumption that host density is equal between sites and seasons, the variable m was calculated as the mean number of female Cs. melanura per resting shelter per month. Host density is likely to be higher during spring and autumn during bird migrations but was not measured or incorporated into the vectorial capacity calculation. To calculate a, the proportion of Cs. melanura feeding on competent (avian) hosts was divided by the length of the gonotrophic cycle (gc) (Rubio-Palis, 1994). The gonotrophic cycle length of laboratory reared Cs. melanura varies from 23.2 days at 10°C to 4.5 days at 28°C (Mahmood and Crans, 1997). Estimation of gc was done using the equation gc = 1/V where and t is temperature in Celsius (Mahmood and Crans, 1997). The values of t for the study period were the mean daily temperature taken from historical weather data from the National Climatic Data Center weather stations at Gilbert Airport in Winter Garden, FL, for the samples from Polk County and at Executive Airport in Orlando, FL, for the samples from Orange County (National Climatic Data Center, 2019). The value of p was estimated with , where M is equal to the proportion parous and gc is the length of the gonotrophic cycle (Almeida et al., 2005). The variable M is the proportion of parous mosquitoes of the females with a positive parity determination. The mosquitoes with undetermined parity were not included in the C calculation and included only gravid females, females with a bloodmeal >2 days old, and females with damaged ovaries. EIP (n) was estimated for our study using data from published laboratory studies of vector competence of Cs. melanura under different temperatures. The Excel TREND function was used to define a relationship between competence (n) and temperature values along an extrapolated trend line, using ambient temperatures at each of the six field sites in Florida. The n of EEEV in Cs. melanura (50% transmission) was found to be 3 days when kept at 28°C (Scott and Burrage, 1984) and 5 days when held at 24°C (Weaver et al., 1990).
When these calculations were complete, the determined value of C for each period was compared to the mean monthly number of EEEV equine cases in Florida from the last decade and the number of cases from 2018 (Florida Department of Health, 2019). Linear regression analyses were used to determine the relationship of C values and epizootic EEEV transmission in R (R Core Team, 2014).
Results
Mosquito Abundance and Host Use
During the 2018 sampling year, 6,093 female mosquitoes, comprised of 24 different species, were collected from six sites in Orange and Polk counties, Florida (Table 2). The most abundant mosquito species were Cx. erraticus (n = 2,720) and Cs. melanura (n = 1,036). Unfed females constituted the majority (67.5–85.1%) of total females collected throughout the spring and summer (Figure 2). In autumn, however, blood-fed and gravid females (combined) accounted for more than half (53.3–59.3%) of total females (Figure 2). Abundance of Cs. melanura showed a seasonal pattern of increasing numbers in summer months, which was three times larger than winter months (Figure 3A). The density of Cs. melanura peaked in July, with a mean of 1.29 females captured per shelter (n = 170, Table 3). In August, the density remained high but a large decrease in density to 0.29 occurred in September. The smallest density occurred in February with 0.18 females per shelter. Abundance and density of Cs. melanura were significantly correlated (R2 = 0.86, df = 13, p < 0.0001).
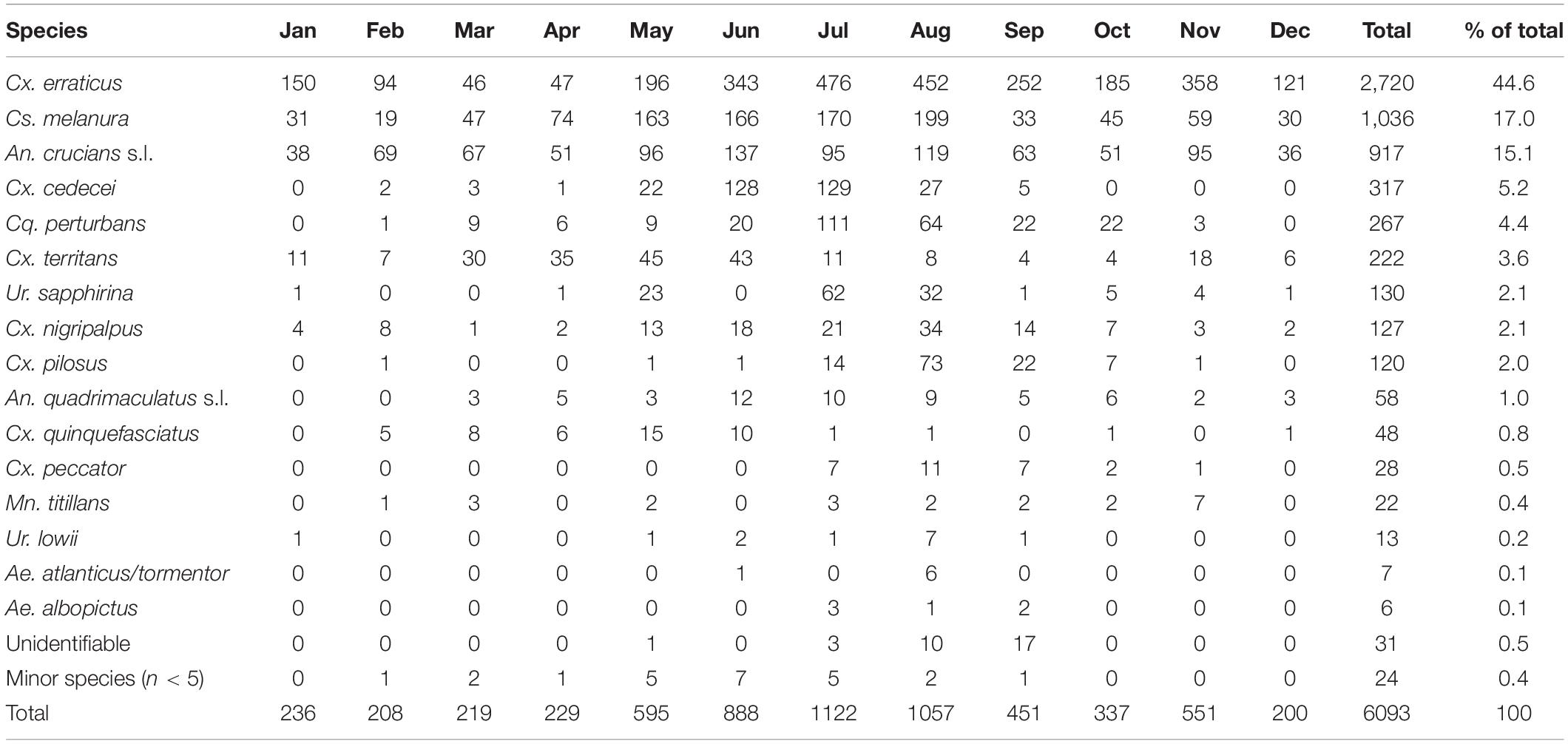
Table 2. Monthly numbers of Cs. melanura and other mosquito species. Mosquitoes were collected using resting shelters in Orange and Polk County, FL, in 2018.
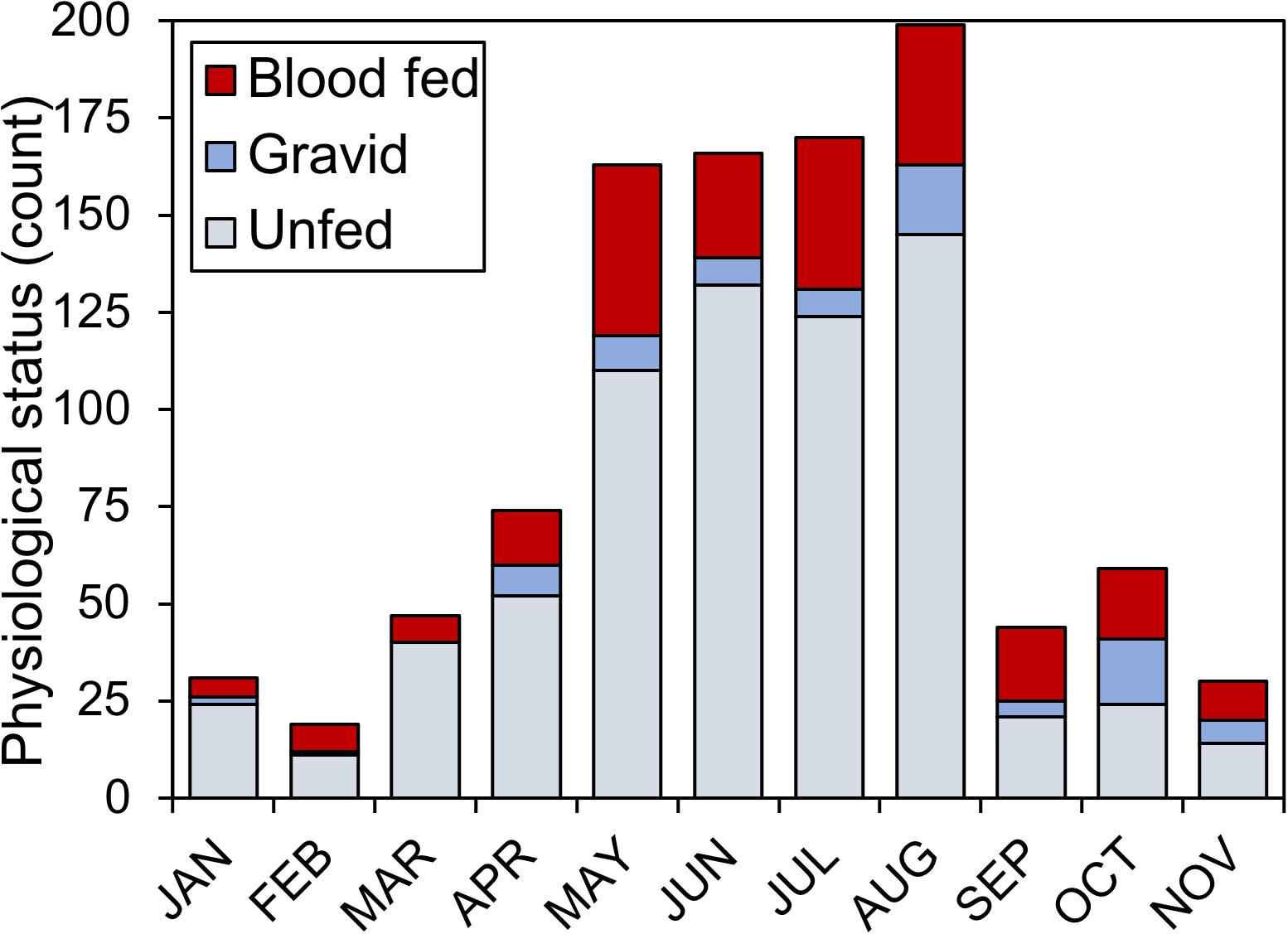
Figure 2. Physiological status of Cs. melanura females from Central Florida. Females were sampled using resting shelters at 12 total sites in Orange and Polk County, FL, United States, in 2018.
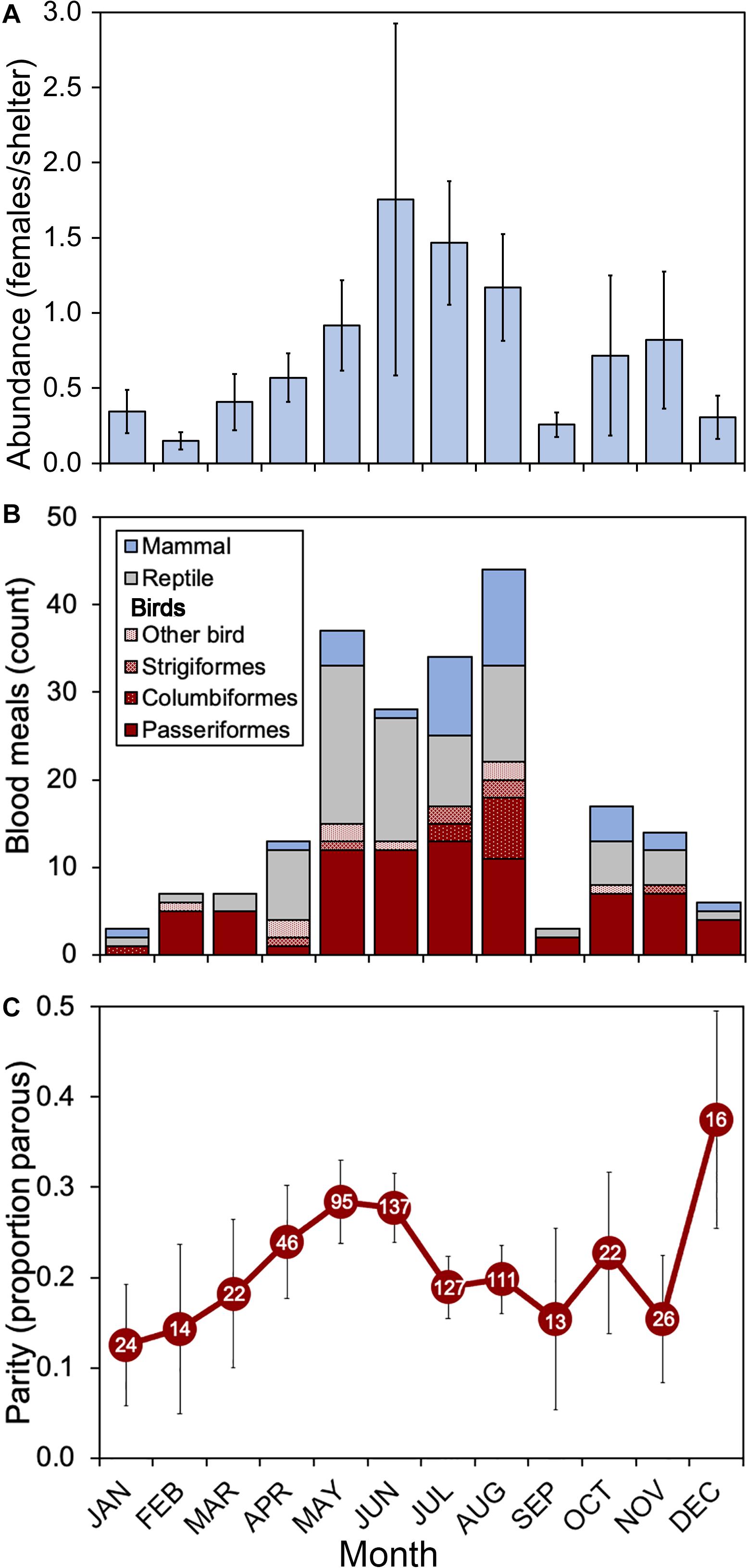
Figure 3. Seasonal patterns of abundance, host use and parity of Cs. melanura in Central Florida. (A) Abundance represents the mean number of female mosquitoes per artificial resting shelter. Bars indicate standard errors of means. (B) Vertebrate hosts (class level) determined by PCR and Sanger sequencing of blood-engorged females. “Other birds” includes Galliformes (n = 4) and one each of Charadriiformes, Coraciiformes, Cuculiformes, Gruiformes, and Pelecaniformes. (C) Parity represents the proportion of females with tracheal skeins. Sample sizes appear within markers. Bars represent SE. Culiseta melanura collected from Orange and Polk County, FL, United States, in 2018.
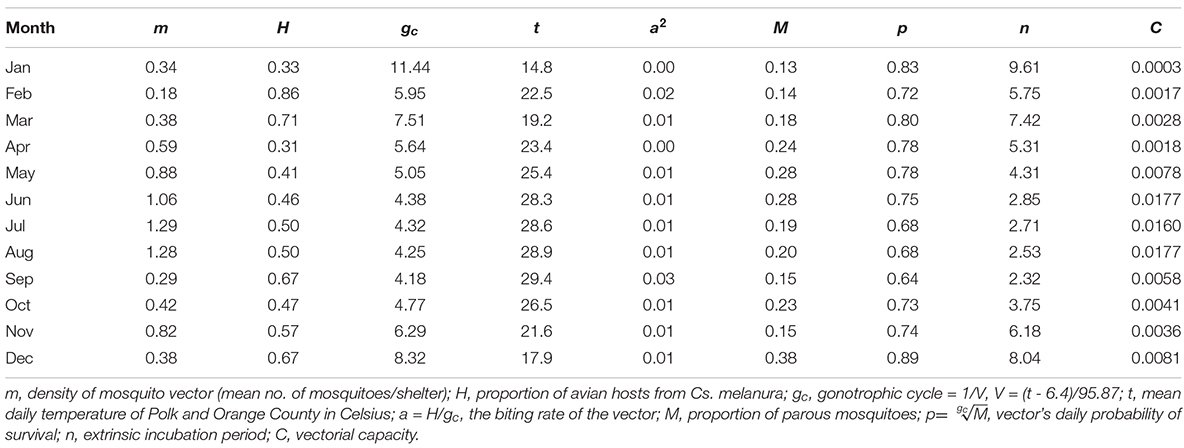
Table 3. Vectorial capacity components of Cs. melanura for EEEV. Mosquitoes were collected in Orange and Polk County, FL, in 2018.
Host use by Cs. melanura in 2018 was previously determined by bloodmeal analysis and resulted in 105 avian (49.3%), 74 reptilian (34.7%), and 34 mammalian (16.0%) bloodmeals. Avian host use varied by month (Figure 3B), from a low of 31% of total bloodmeals to a high of 86% of total bloodmeals. Bird bloodmeals were most common every month except April, May, and June, when reptile bloodmeals were frequent (Figure 3B). In September, only three bloodmeals were identified, which corresponded to a period of very low Cs. melanura abundance (Figure 3A). Avian species identified from bloodmeals consisted of 9 orders (Figure 3B) and 22 families of birds. The avian hosts most fed upon were northern cardinal, red-eyed vireo, mourning dove, Carolina wren, barred owl, pine warbler, and house wren. The largest number of bloodmeals came from songbirds (Order Passeriformes) with 79 positive identifications (75% of avian hosts), with other avian meals derived from Columbiformes (n = 10), Strigiformes (n = 7), Galliformes (n = 4) and single meals (n = 1) from four other avian orders (Charadriiformes, Coraciiformes, Cuculiformes, Gruiformes and Pelecaniformes).
Parity, Gonotrophic Cycle, Extrinsic Incubation, and Survival
Parity was determined for 653 Cs. melanura females of 1,036 collected (63.0%). The proportion of parous females ranged from 0.13 in January to 0.38 in December of 2018 (Figure 3C). From January to May, the proportion of parous females increased gradually to reach relatively high levels (0.28) in May and June, followed by a substantial decrease in July and August (0.19–0.20). From September through December, low numbers of females (n = 13–26) were available for parity determination, resulting in fluctuating parity for the remainder of the year. Of 257 blood engorged Cs. melanura females from Orange and Polk County, only 65 (25.3%) were successfully determined for parity, due to maturation of egg follicles. Of these, 7 (10.8%) were found to be parous.
Incorporating the mean monthly temperatures from the study sites enabled the calculation of n and gc, the latter of which is also used to estimate a and p. The lowest mean temperature of Orange and Polk counties was recorded at 14.8°C in January, and the highest mean temperature of 29.4°C was recorded in September. The relationships between temperature and each of the parameter estimates for n, gc, and p are shown in Figure 4. The monthly temperatures in Orange and Polk County were similar, with a mean difference of 0.3°C. At these temperatures, the gc calculated for Cs. melanura ranged from 4.2 to 11.4 days in length (Table 3). The estimated n for Cs. melanura ranged from 2.3 days in September to 9.6 days in January (mean = 5.1 days). Daily probability of survival varied modestly over the study period, compared to other variables (Table 3), ranging from a low of 0.63 in September to a high of 0.89 in December.
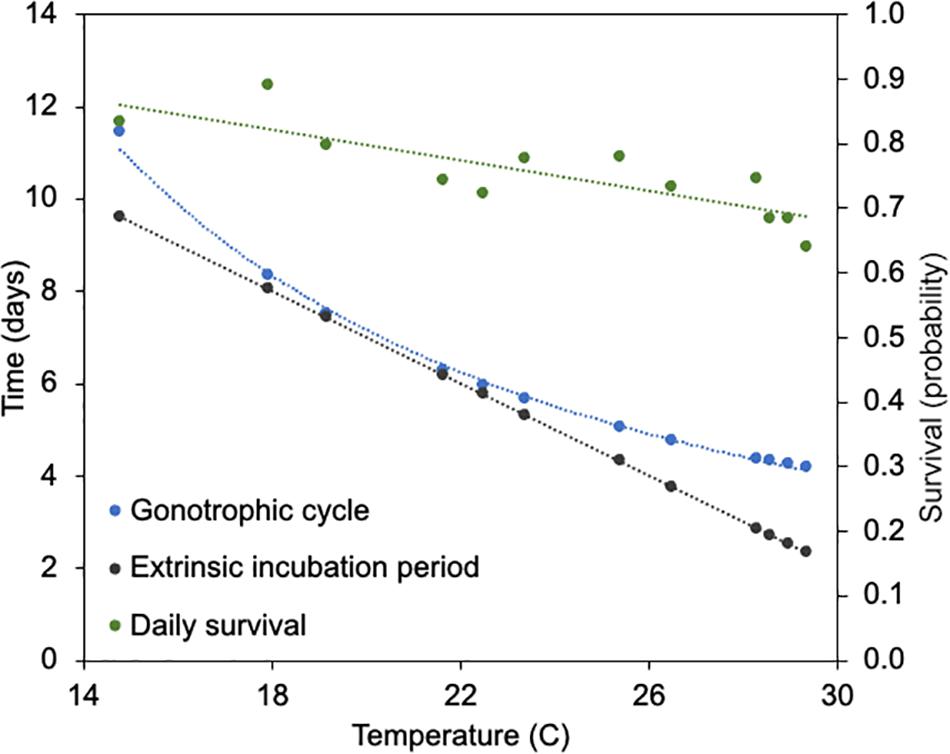
Figure 4. Relationship between temperature, gonotrophic cycle length, extrinsic incubation period for eastern equine encephalitis virus and daily survival of Cs. melanura from Central Florida. Temperature represents average monthly temperature in Orange and Polk County, FL, United States, in 2018.
EEEV Transmission in Florida
A total of 55 equine cases (Figure 5; FDOH data) was recorded in Florida in 2018, which was somewhat higher than the 10-year average of 41.4 cases per year. Epizootic transmission of EEEV was particularly high in winter and spring of 2018 (January to May) with more than twice as many cases as the annual mean for the same period over the past ten years. The numbers of equine cases declined sharply after June of 2018, with just four cases in August, one case each in September and October, and no cases reported in November or December. The 10-year average shows low numbers of cases (0.7–1.9) from September through April (8 consecutive months), with the bulk of cases (10.8–11.5/month) occurring in June and July. In Orange and Polk counties in 2018, 2 horse cases and 13 sentinel chicken seroconversions to EEEV were reported. In the last decade, Orange and Polk counties have reported 2 and 12 total EEEV horse cases, respectively.
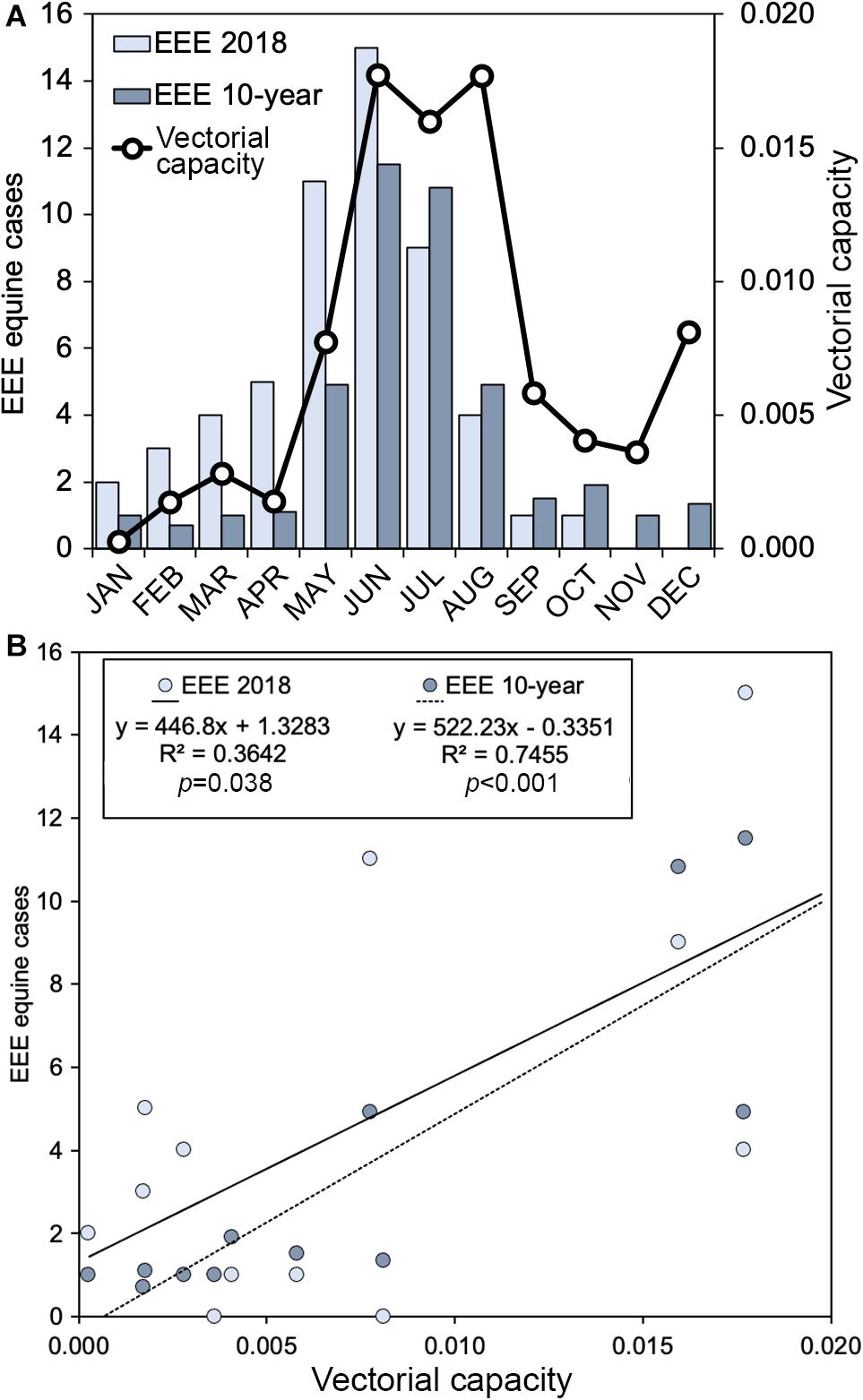
Figure 5. Relationship of vectorial capacity of Cs. melanura for EEEV and equine cases from Florida. (A) Monthly values of vectorial capacity (black line) and EEEV cases in equines (bars); (B) Scatterplot of vectorial capacity and EEEEV cases in equines with best fit linear regression lines. Values of the vectorial capacity (C) were calculated using Cs. melanura collected from two Central Florida counties. EEEV cases are monthly aggregates for the year of Cs. melanura sampling (2018) or monthly averages over the prior decade.
Vectorial Capacity
Using data from mosquito abundance, parity, bloodmeal source, and temperature, vectorial capacity (C) values of Cs. melanura for EEEV were calculated for the months of 2018 (Table 3). The m variable is traditionally calculated as the number of vectors per host. Because the density variable used in this study is the number of mosquitoes per shelter, the m is likely underestimated and C values are relatively smaller than previous studies (Garrett-Jones and Shidrawi, 1969). Estimated values of C were lowest (0.0003) in January and remained low throughout winter and early spring (Figure 5A). C increased in May, reaching highest values (0.0160–0.0177) in June, July and August (Figure 5A). In September, C decreased substantially and continued to lower until December, when a small resurgence was observed (Figure 5A).
Equine cases of EEEV generally reflected seasonal changes in estimated values of C for Cs. melanura, with higher numbers of EEEV cases observed during months with greater C (Figure 5A). Linear regression revealed a significant positive relationship between C and Equine EEEV cases for the year 2018 (R2 = 0.36, df = 10, p = 0.038) and the mean monthly number of cases from the prior decade (R2 = 0.75, df = 10, p < 0.001) (Figure 5B). During 2018, 55 positive EEEV cases were reported from equids as well as five emu flocks. Epizootic transmission from January to May was more than twice as high in 2018 than the mean of the last decade.
Discussion
Mosquito Abundance and Host Use
Culiseta melanura density in Central Florida was highest in the late spring and summer (June–August) which contrasts with results from Edman et al. (1972) and Blosser et al. (2017) where decreases of abundance in late spring were reported. These contrasting patterns suggest that phenology of this important vector species is plastic and likely varies from year to year. Studies from the northeastern United States have shown that hydrological conditions drive Cs. melanura abundance (Skaff et al., 2017), but that temperature shapes the rate of larval development (Mahmood and Crans, 1997). Interestingly, high ambient temperature during larval development caused a dramatic decrease in adult emergence success, from 59.6% emergence at 28°C, to 1.0% emergence at 32°C, in laboratory studies (Mahmood and Crans, 1997). September was the warmest month in 2018 with a mean daily temperature of 29.4°C and a mean daily high temperature of 33.9°C. The high temperatures observed in September corresponded with very low Cs. melanura abundance (Figure 3A) during a period of otherwise relatively high mosquito density. Importantly, other commonly collected mosquitoes, including EEEV bridge vectors Cx. erraticus, Cx. nigripalpus, and Cq. perturbans, did not undergo similar decreases in abundance (Table 2), suggesting that high temperatures adversely affect Cs. melanura uniquely and may have cascading effects on EEEV, as high Cs. melanura abundance is significantly correlated with EEEV transmission (Skaff et al., 2017). Our finding that the increase in Cs. melanura abundance observed in the spring (Figure 3A) closely parallels the increase in EEEV cases in horses that occurred in May 2018 supports a relationship between Cs. melanura abundance and EEEV transmission.
Our finding that the biting rate by Cs. melanura varies throughout the year (Table 3) has important implications for understanding how C changes seasonally, and perhaps how vector abundance influences host use. Morris et al. (1980) found that non-avian feedings by Cs. melanura occurred simultaneously with high populations, irrespective of distance from the breeding swamp. Similarly, we observed that during the period of peak Cs. melanura abundance (May–August), feedings upon reptiles and mammals were at their greatest (Figures 3A,B). It is unclear how abundance and host use are related; however, increasing numbers of attacking mosquitoes (Cx. nigripalpus) have been shown to result in increasing levels of defensive behaviors exhibited by birds and a corresponding reduction in the proportions of successfully feeding female mosquitoes (Edman et al., 1974). Large numbers of questing Cs. melanura females could be thwarted from successfully obtaining a bloodmeal from preferred avian hosts and shift to biting available mammals or reptiles in the environment. Under this scenario, Cs. melanura could serve as an epizootic vector of EEEV.
Parity, Gonotrophic Cycle, Extrinsic Incubation, and Survival
Our finding that parity rates varied throughout the year is expected, given the natural cycles of emergence, blood-feeding and oviposition. The relatively high parity rates observed in spring (Figure 3C), followed by a decrease in summer is reflective of patterns observed in New York (Morris et al., 1980). However, much higher parity rates (>75% in some samples) were observed in New York, compared to Florida (generally <30%). Parity does not directly factor into the vectorial capacity equation, but it contributes to estimates of the age of the vector population, an important determinant of the potential infectiousness of the population. Increasing parity indicates an older vector population with prior host contact, which is thought to correspond with higher infection rates in the vector and host (Lines et al., 1991).
Gonotrophic cycle length and the extrinsic incubation period of Cs. melanura were not measured directly but were estimated using ambient mean temperature at area weather stations, and data from published studies. In general, both gc and n have negative relationships with temperature, with longer gonotrophic cycles and extrinsic incubation periods at lower temperatures. Interestingly, because of differences in the shape of the relationships of these two variables and temperature (linear versus non-linear) gc and n are virtually identical at moderate temperatures (18–22°C) but differ by nearly 2 days at both upper and lower temperature extremes (Figure 4). The effect of this discordance between gc and n on transmission of EEEV is not known, yet could have dampening effect on transmission at temperature extremes. Females that have completed a gonotrophic cycle, but with incomplete extrinsic incubation periods would not be “ready” to transmit virus upon the next bite. A better understanding of the time between oviposition and blood-feeding by Cs. melanura would help to determine whether this temperature-variation discordance is important in nature.
Daily survival probability (p), as estimated using parity and length of the gonotrophic cycle (Almeida et al., 2005) also has a negative relationship with temperature, which is not surprising given its derivation. The lowest estimated daily survival was observed in September (0.64) when very high temperatures were observed, a relationship which was supported by very low abundances during that month (Figure 5A). Estimated values of p in this study align with findings of Chamberlain and Sudia (1955) that mosquitoes which were incubated at lower temperatures survived longer.
EEEV Transmission in Florida
The epizootic transmission of EEEV in Florida can fluctuate greatly from year to year and the number of equine cases was greater than average in 2018, with a total of 55 equine cases (Figure 5A). The phenology of epizootic transmission in 2018 was fairly representative of the 10-year average with one notable exception. The peak in equine cases in 2018 occurred earlier in the year, with greater than normal cases in May, and fewer than normal cases in July (Figure 5A). The reason behind the early peak in EEEV is unclear. However, our data from Central Florida show that during May Cs. melanura had relatively high parity (c.f. Figure 3C) and the species took a relatively large fraction of bloodmeals from non-avian hosts during this period (Figure 3B). Surprisingly, Cs. melanura abundance was not particularly high in May 2018, although the population had been increasing steadily since February.
Vectorial Capacity
Our study represents an important step in demonstrating links between seasonal variation in vectorial capacity and epizootic spillover of a zoonotic vector-borne pathogen and indicates that concepts developed to describe relationship between entomologic factors and anthroponotic vector-borne disease also apply to vector-borne zoonoses. Interestingly, vectorial capacity of Cs. melanura explained a larger fraction of the variation in historical (10-year average) EEEV epizootic cases (75%) than that of the concurrent year (36%). Notable departures from the relationship between epizootic EEEV cases and C occurred in August and December, when C was estimated to be relatively high, but the numbers of equine cases were relatively low (Figure 5A). The disparity in December is likely due to low sample sizes of mosquitoes (<20) used to calculate relative avian host use (66.6%) and proportion of parous females (38%) in that month (Figure 3A), possibly artificially inflating the estimates of C for December. Conversely, C values in August were based upon robust numbers of females used to determine host use (n = 44) and parity (n = 111). The low numbers of equine cases in August is therefore perplexing. Bridge vector species, such as Cx. erraticus and Cq. perturbans, were also common during August, and would have presumably contributed to EEEV transmission, further confounding the low numbers of equine cases. Vaccination rates in horses could increase in response to the outbreak, lowering the numbers of cases reported. Additional work should be done to understand how C of a zoonotic pathogen vector might fundamentally differ from that of vectors of anthroponotic pathogens.
This work has several limitations, many of which are the result of difficulties studying field populations of mosquitoes that feed principally on wild vertebrate hosts. Using the number of females per resting shelter is an indirect method of estimating density, in contrast to measuring incidence of bites per host per day. Previously, measuring density indirectly resulted in three to four times lower density of Anopheles than using direct measurements (Garrett-Jones, 1964a). If density of Cs. melanura was measured directly using landing rates on birds, density values would likely be higher and better reflect the actual contact rates between vectors and amplifying hosts. One assumption of vectorial capacity is that the pathogen is transmitted by only one species of vector to one type of host (Dye, 1986). The a for our calculation assumes that all avian hosts are equally competent. But avian EEEV competence has not been measured in all avian hosts found in this study. The host competence of an avian species may differ depending on family, size, recent exposure to an exotic species, or could differ between individuals (Komar et al., 1999). The parity rates of winter and autumn were based upon low sample sizes (<30 per month) which may have affected the accuracy of parity estimates, and variables that were calculated from it. In addition to environmental factors such as predation, infection with EEEV may decrease the survival rate of mosquitoes (Scott and Lorenz, 1998; Moncayo et al., 2000; Kramer and Ciota, 2015). Importantly, few data are available to accurately estimate the relationship between temperature and EIP for Cs. melanura. These relationships are likely population/strain dependent and correlated with not just mean, but also min/max temperatures. Future experimental studies with the population used here over a range of temperatures regimes would be invaluable to parameterize models.
Conclusion
In conclusion, a positive association between monthly EEEV infections in equids and vectorial capacity of the vector was observed, supporting the hypothesis that enzootic transmission by Cs. melanura has a large effect on epizootic outbreaks. This study reveals how aspects of the biology of an important enzootic arbovirus vector, such as abundance, host use and longevity, change throughout the year, and how these changes possibly drive to spillover of a human pathogen. This information may aid in the prevention, monitoring, and control of arboviruses, including EEEV, by providing detailed information on the relationships between key biological variables of the vector and seasonal patterns of pathogen transmission.
Data Availability Statement
All datasets presented in this study are included in the article/Supplementary Material.
Author Contributions
RW, CB, and NB-C carried out the methodology. RW and NB-C analyzed the data. All authors contributed to the study design and writing of the manuscript.
Funding
This research was supported by the Cooperative Agreement Number U01CK000510 to TU, funded by the Centers for Disease Control and Prevention, and NIFA 005446. The content is solely the responsibility of the authors and does not represent the official view of the Centers for Disease Control and Prevention.
Conflict of Interest
The authors declare that the research was conducted in the absence of any commercial or financial relationships that could be construed as a potential conflict of interest.
Acknowledgments
We thank the following for their assistance with mosquito sampling: Jackson Mosley, Hugo Ortiz Saavedra, and Roger Johnson at Polk County Mosquito Control Program; Kelly Deutsch, Rafael Melendez, and others at Orange County Mosquito Control District. This study could not have been done without their cooperation and hard work. We also thank Carolina Acevedo for help with bloodmeal analysis, Erik Blosser for help with mosquito identifications, and Diana Rojas and Annsley West for help with field collections.
Supplementary Material
The Supplementary Material for this article can be found online at: https://www.frontiersin.org/articles/10.3389/fevo.2020.00270/full#supplementary-material
References
Almeida, A. P. G., Baptista, G. S. S. S., Sousa, C. C. A. G., Novo, M. M. T. L., Ramos, H. C., Panella, N. A., et al. (2005). (Diptera: Culicidae) in Macao, China, in relation to dengue virus transmission. J. Med. Entomol. 42, 419–428. doi: 10.1093/jmedent/42.3.419
Armstrong, P. M., and Andreadis, T. G. (2010). Eastern equine encephalitis virus in mosquitoes and their role as bridge vectors. Emerg. Infect. Dis. 16, 1869–1874. doi: 10.3201/eid1612.100640
Bigler, W., Lassing, E., Buff, E., Prather, E., Beck, E., and Hoff, G. (1976). Endemic eastern equine encephalomyelitis in Florida: a twenty-year analysis, 1955–1974. Am. J. Trop. Med. Hyg. 25, 884–890. doi: 10.4269/ajtmh.1976.25.884
Bingham, A. M., Burkett-Cadena, N. D., Hassan, H. K., and Unnasch, T. R. (2015). Vector competence and capacity of Culex erraticus (Diptera: Culicidae) for eastern equine encephalitis virus in the southeastern United States. J. Med. Entomol. 53, 473–476. doi: 10.1093/jme/tjv195
Bingham, A., Burkett-Cadena, N., Hassan, H., McClure, C., and Unnasch, T. (2014). Field investigations of winter transmission of eastern equine encephalitis virus in Florida. Am. J. Trop. Med. Hyg. 91, 685–693. doi: 10.4269/ajtmh.14-0081
Blosser, E. M., Lord, C. C., Stenn, T., Acevedo, C., Hassan, H. K., Reeves, L. E., et al. (2017). Environmental drivers of seasonal patterns of host utilization by Culiseta melanura (Diptera: Culicidae) in Florida. J. Med. Entomol. 54, 1365–1374. doi: 10.1093/jme/tjx140
Brady, O. J., Godfray, H. C. J., Tatem, A. J., Gething, P. W., Cohen, J. M., McKenzie, F. E., et al. (2016). Vectorial capacity and vector control: reconsidering sensitivity to parameters for malaria elimination. Trans. R. Soc. Trop. Med. Hyg. 110, 107–117. doi: 10.1093/trstmh/trv113
Burkett-Cadena, N. D., Bingham, A., Hunt, B., Morse, G., and Unnasch, T. (2015). Ecology of Culiseta melanura and other mosquitoes (Diptera: Culicidae) from Walton County, FL, during winter period 2013-2014. J. Med. Entomol. 52, 1074–1082. doi: 10.1093/jme/tjv087
Burkett-Cadena, N. D. (2013). Mosquitoes of the Southeastern United States. Tuscaloosa, AL: University of Alabama Press.
Burkett-Cadena, N. D., Hoyer, I., Blosser, E., and Reeves, L. (2019). Human-powered pop-up resting shelter for sampling cavity-resting mosquitoes. Acta Trop. 190, 288–292.
Chamberlain, R., and Sudia, W. D. (1955). The effects of temperature upon the extrinsic incubation of eastern equine encephalitis in mosquitoes. Am. J. Hyg. 62, 295–305. doi: 10.1093/oxfordjournals.aje.a119780
Cupp, E. W., Klingler, K., Hassan, H. K., Viguers, L. M., and Unnasch, T. R. (2003). Transmission of eastern equine encephalomyelitis virus in central alabama. Am. J. Trop. Med. Hyg. 68, 495–500. doi: 10.4269/ajtmh.2003.68.495
Darsie, R. F., and Morris, C. D. (2003). Keys to the Adult Females and Fourth Instar Larvae of the Mosquitoes of Florida (Diptera, Culicidae). Buckingham: Florida Mosquito Control Association.
Darsie, R. F., and Ward, R. A. (2005). Identification and Geographical Distribution of the Mosquitoes of North America, North of Mexico. Gainesville, FL: University Press of Florida.
Day, J. F., and Shaman, J. (2011). Mosquito-Borne Arboviral Surveillance and the Prediction of Disease Outbreaks, Flavivirus Encephalitis. London: InTech Open.
Detinova, T. (1962). Age-grouping Methods in Diptera of Medical Importance. Geneva: World Health Organization.
Dye, C. (1986). Vectorial capacity: must we measure all its components? Parasitol. Today 2, 203–209. doi: 10.1016/0169-4758(86)90082-7
Edman, J. D., Webber, L. A., and Schmid, A. A. (1974). Effect of host defenses on the feeding pattern of Culex nigripalpus when offered a choice of blood sources. J. Parasitol. 60, 874–883.
Edman, J., Webber, L., and Kale, H. (1972). Host-feeding patterns of Florida mosquitoes II. Culiseta. J. Med. Entomol. 9, 429–434. doi: 10.1093/jmedent/9.5.429
Elias, S. P., Keenan, P., Kenney, J. L., Morris, S. R., Covino, K. M., Robinson, S., et al. (2017). Seasonal patterns in eastern equine encephalitis virus antibody in songbirds in Southern Maine. Vector Borne Zoonotic Dis. 17, 325–330. doi: 10.1089/vbz.2016.2029
Estep, L. K., McClure, C. J., Burkett-Cadena, N. D., Hassan, H. K., Hicks, T. L., Unnasch, T. R., et al. (2011). A multi-year study of mosquito feeding patterns on avian hosts in a Southeastern focus of eastern equine encephalitis virus. Am. J. Trop. Med. Hyg. 84, 718–726. doi: 10.4269/ajtmh.2011.10-0586
Florida Department of Health (2019). Mosquito-Borne Disease Surveillance. Available online at: http://www.floridahealth.gov/diseases-and-conditions/mosquito-borne-diseases/surveillance.html (accessed January 7, 2019).
Garrett-Jones, C. (1964a). A Method for Estimating the Man-Biting Rate. Geneva: World Health Organization.
Garrett-Jones, C. (1964b). Prognosis for interruption of malaria transmission through assessment of the mosquito’s vectorial capacity. Nature 204, 1173–1175. doi: 10.1038/2041173a0
Garrett-Jones, C., and Shidrawi, G. R. (1969). Malaria vectorial capacity of a population of Anopheles gambiae: an exercise in epidemiological entomology. Bull. World Health Org. 40, 531–545.
Heberlein-Larson, L. A., Tan, Y., Stark, L. M., Cannons, A. C., Shilts, M. H., Unnasch, T. R., et al. (2019). Complex epidemiological dynamics of eastern equine encephalitis virus in Florida. Am. J. Trop. Med. Hyg. 100, 1266–1274 doi: 10.4269/ajtmh.18-0783
Howard, J. J., and Wallis, R. C. (1974). Infection and transmission of eastern equine encephalomyelitis virus with colonized Culiseta melanura (Coquillett). Am. J. Trop. Med. Hyg. 23, 522–525. doi: 10.4269/ajtmh.1974.23.522
Hugo, L. E., Quick-Miles, S., Kay, B., and Ryan, P. (2014). Evaluations of mosquito age grading techniques based on morphological changes. J. Med. Entomol. 45, 353–369. doi: 10.1093/jmedent/45.3.353
Komar, N., Dohm, D. J., Turell, M. J., and Spielman, A. (1999). Eastern equine encephalitis virus in birds: relative competence of European starlings (Sturnus vulgaris). Am. J. Trop. Med. Hyg. 60, 387–391. doi: 10.4269/ajtmh.1999.60.387
Kramer, L. D., and Ciota, A. T. (2015). Dissecting vectorial capacity for mosquito-borne viruses. Curr. Opin. Virol. 15, 112–118. doi: 10.1016/j.coviro.2015.10.003
Kuno, G., Mackenzie, J. S., Junglen, S., Hubálek, Z., Plyusnin, A., and Gubler, D. J. (2017). Vertebrate reservoirs of arboviruses: myth, synonym of amplifier, or reality? Viruses 9:185. doi: 10.3390/v9070185
Lines, J. D., Wilkes, T. J., and Lyimo, E. O. (1991) Human malaria infectiousness measured by age-specific sporozoite rates in Anopheles gambiae in Tanzania. Parasitology 102, 167–177. doi: 10.1017/s0031182000062454
Lubelczyk, C., Mutebi, J.- P., Robinson, S., Elias, S. P., Smith, L. B., Juris, S. A., et al. (2013). An epizootic of eastern equine encephalitis virus, Maine, USA in 2009: outbreak description and entomological studies. Am. J. Trop. Med. Hyg. 88, 95–102. doi: 10.4269/ajtmh.2012.11-0358
Macdonald, G. (1957). The Epidemiology and Control of Malaria. New York, NY: Oxford University Press.
Mahmood, F., and Crans, W. J. (1997). A thermal heat summation model to predict the duration of the gonotrophic cycle of Culiseta melanura in nature. J. Am. Mosq. Control Assoc. 13, 92–94.
Molaei, G., and Andreadis, T. G. (2006). Identification of avian-and mammalian-derived bloodmeals in Aedes vexans and Culiseta melanura (Diptera: Culicidae) and its implication for West Nile virus transmission in connecticut, USA. J. Med. Entomol. 43, 1088–1093. doi: 10.1603/0022-2585(2006)43[1088:ioaamb]2.0.co;2
Molaei, G., Andreadis, T. G., Armstrong, P. M., Thomas, M. C., Deschamps, T., Cuebas-Incle, E., et al. (2013). Vector-host interactions and epizootiology of eastern equine encephalitis virus in Massachusetts. Vector Borne Zoonotic Dis. 13, 312–323. doi: 10.1089/vbz.2012.1099
Molaei, G., Armstrong, P. M., Abadam, C. F., Akaratovic, K. I., Kiser, J. P., and Andreadis, T. G. (2015a). Vector-host interactions of Culiseta melanura in a focus of eastern equine encephalitis virus activity in southeastern Virginia. PLoS One 10:e0136743. doi: 10.1371/journal.pone.0136743
Molaei, G., Armstrong, P. M., Graham, A. C., Kramer, L. D., and Andreadis, T. G. (2015b). Insights into the recent emergence and expansion of eastern equine encephalitis virus in a new focus in the Northern New England USA. Parasit. Vectors 8:516.
Molaei, G., Thomas, M. C., Muller, T., Medlock, J., Shepard, J. J., Armstrong, P. M., et al. (2016). Dynamics of vector-host interactions in avian communities in four eastern equine encephalitis virus foci in the northeastern US. PLoS Negl. Trop. Dis. 10:e0004347. doi: 10.1371/journal.pntd.0004347
Molaei, G., Oliver, J., Andreadis, T. G., Armstrong, P. M., and Howard, J. J. (2006). Molecular identification of blood-meal sources in Culiseta melanura and Culiseta morsitans from an endemic focus of eastern equine encephalitis virus in New York. Am. J. Trop. Med. Hyg. 75, 1140–1147. doi: 10.4269/ajtmh.2006.75.1140
Moncayo, A. C., Edman, J. D., and Turell, M. J. (2000). Effect of eastern equine encephalomyelitis virus on the survival of Aedes albopictus, Anopheles quadrimaculatus, and Coquillettidia perturbans (Diptera: Culicidae). J. Med. Entomol. 37, 701–706. doi: 10.1603/0022-2585-37.5.701
Morris, C. D., Zimmerman, R. H., and Edman, J. D. (1980). Epizootiology of eastern equine encephalomyelitis virus in upstate New York, USA: II. Population dynamics and vector potential of adult Culiseta melanura (Diptera: Culicidae) in relation to distance from breeding site. J. Med. Entomol. 17, 453–465.
National Climatic Data Center (2019). Climate Data Online. Available online at: https://www.ncdc.noaa.gov/cdo-web/ (accessed March 8, 2019).
R Core Team (2014). R: A Language and Environment for Statistical Computing. Vienna: R Foundation for Statistical Computing.
Reisen, W. K. (2010). Landscape epidemiology of vector-borne diseases. Annu. Rev. Entomol. 55, 461–483. doi: 10.1146/annurev-ento-112408-085419
Rubio-Palis, Y. (1994). Variation of the vectorial capacity of some anophelines in western Venezuela. Am. J. Trop. Med. Hyg. 50, 420–424. doi: 10.4269/ajtmh.1994.50.420
Saugstad, E., Dalrymple, J., and Eldridge, B. (1972). Ecology of arboviruses in a Maryland freshwater swamp: i. population dynamics and habitat distribution of potential mosquito vectors. Am. J. Epidemiol. 96, 114–122. doi: 10.1093/oxfordjournals.aje.a121437
Scott, T. W., and Weaver, S. C. (1989). Eastern equine encephalomyelitis virus: epidemiology and evolution of mosquito transmission. Adv. Virus Res. 37, 277–328. doi: 10.1016/s0065-3527(08)60838-6
Scott, T. W., and Burrage, T. G. (1984). Rapid infection of salivary glands in Culiseta melanura with eastern equine encephalitis virus: an electron microscopic study. Am. J. Trop. Med. Hyg. 33, 961–964. doi: 10.4269/ajtmh.1984.33.961
Scott, T. W., and Lorenz, L. H. (1998). Reduction of Culiseta melanura fitness by eastern equine encephalomyelitis virus. Am. J. Trop. Med. Hyg. 59, 341–346. doi: 10.4269/ajtmh.1998.59.341
Skaff, N. K., Armstrong, P. M., Andreadis, T. G., and Cheruvelil, K. S. (2017). Wetland characteristics linked to broad-scale patterns in Culiseta melanura abundance and eastern equine encephalitis virus infection. Parasit. Vectors 10:501. doi: 10.1093/jmedent/18.6.501
Smith, D. L., Perkins, T.A., Reiner, R. C. Jr., Barker, C. M., Niu, T., Chaves, L. F., et al. (2014). Recasting the theory of mosquito-borne pathogen transmission dynamics and control. Trans. R. Soc. Trop. Med. Hyg. 108, 185–197.
Tenbroeck, C., Hurst, E. W., and Traub, E. (1935). Epidemiology of equine encephalomyelitis in the eastern United States. J. Exp. Med. 62, 677–685. doi: 10.1084/jem.62.5.677
Vaidyanathan, R., Edman, J., Cooper, L., and Scott, T. (1997). Vector competence of mosquitoes (Diptera: Culicidae) from Massachusetts for a sympatric isolate of eastern equine encephalomyelitis virus. J. Med. Entomol. 34, 346–352. doi: 10.1093/jmedent/34.3.346
Weaver, S. C., Scott, T. W., and Lorenz, L. H. (1990). Patterns of eastern equine encephalomyelitis virus infection in Culiseta melanura (Diptera: Culicidae). J. Med. Entomol. 27, 878—891. doi: 10.1093/jmedent/27.5.878
Keywords: vectorial capacity, seasonal, arbovirus, zoonosis, epizootic
Citation: West RG, Mathias DR, Day JF, Boohene CK, Unnasch TR and Burkett-Cadena ND (2020) Vectorial Capacity of Culiseta melanura (Diptera: Culicidae) Changes Seasonally and Is Related to Epizootic Transmission of Eastern Equine Encephalitis Virus in Central Florida. Front. Ecol. Evol. 8:270. doi: 10.3389/fevo.2020.00270
Received: 02 June 2020; Accepted: 29 July 2020;
Published: 21 August 2020.
Edited by:
Laura Gangoso, Consejo Superior de Investigaciones Científicas (CSIC), SpainReviewed by:
Sasha Rafi Azar, The University of Texas Medical Branch at Galveston, United StatesAlexander Ciota, Wadsworth Center, United States
Renke Lühken, Kajaani University of Applied Sciences, Finland
Copyright © 2020 West, Mathias, Day, Boohene, Unnasch and Burkett-Cadena. This is an open-access article distributed under the terms of the Creative Commons Attribution License (CC BY). The use, distribution or reproduction in other forums is permitted, provided the original author(s) and the copyright owner(s) are credited and that the original publication in this journal is cited, in accordance with accepted academic practice. No use, distribution or reproduction is permitted which does not comply with these terms.
*Correspondence: Nathan D. Burkett-Cadena, bmJ1cmtldHRjYWRlbmFAdWZsLmVkdQ==