- 1Department of Ecology, Evolution, and Behavior, University of Minnesota, Saint Paul, MN, United States
- 2Graduate Program in Neuroscience, University of Minnesota, Minneapolis, MN, United States
Optimal mate choice based on the assessment of communication signals can be constrained by multiple sources of noise. One well-known impediment to acoustically guided mating decisions is the ambient noise created by multiple signaling individuals in large social groups, in which ambient noise can mask signals by impairing signal recognition and discrimination by receivers. Although studied far less often, another potential source of noise in communication systems stems from variability or inconsistency in how signalers produce their signals. Consistency is especially important in the context of mate choice because sexual advertisement signals are frequently produced repeatedly through time and are composed of constituent parts (e.g., notes and pulses) that are repeated within signals. Inconsistent signal production within individuals has the potential to mask between-individual differences that are often the target of receiver decision-making. In this study of Cope’s gray treefrog, Hyla chrysoscelis, we tested the hypothesis that ambient noise and inconsistent signaling, both independently and synergistically, impair discrimination of species identity. We assayed female discrimination based on pulse rate, a signal of species identity, in quiet and at three levels of ambient noise designed to simulate a breeding chorus. We used synthetic advertisement calls that were invariant or generated with one of three experimental levels of inconsistency in pulse rate, chosen based on levels of within-individual variation observed in natural calls. Pulse rate discrimination was impaired by average and above-average levels of chorus noise, but not by inconsistency in signal production. Receivers spent slightly more time making decisions at the highest level of chorus noise, but response latencies were unaffected by inconsistency. There was no evidence of synergism between ambient noise and inconsistency. Our results suggest that ambient noise, but not inconsistency in signal production, may limit sexual selection on a signal of species identity in natural settings.
Introduction
“Noise” refers to any factor that causes signal detection or discrimination errors (Shannon, 1948; Brumm and Slabbekoorn, 2005; Wiley, 2015). As such, noise is a potent source of selection on animal communication systems (Brumm, 2013), particularly on acoustic signals and auditory perception (Brumm and Slabbekoorn, 2005). The potency of noise stems from its ability to impair receiver decisions with critical impacts on evolutionary fitness, such as mate choice, that depend on recognizing and discriminating among signals. Many acoustically communicating species, for example, produce high amplitude signals and breed in dense social aggregations, where the ambient noise generated by the sounds of conspecific and heterospecific signalers is an important aspect of the acoustic environment in which mate choice occurs (Gerhardt and Huber, 2002; Brumm and Slabbekoorn, 2005). In some frog and insect communication systems, for example, the ambient noise in breeding choruses is known to impede signal recognition and signal discriminability on the basis of temporal and spectral properties (Wollerman and Wiley, 2002; Bee, 2008a; Vélez and Bee, 2011; Römer, 2013; Ward et al., 2013a; Reichert and Ronacher, 2015; Lee et al., 2017; Tanner and Bee, 2019, 2020). Consequently, ambient noise can affect the strength and direction of sexual selection imposed by receivers on signalers by limiting female preference expression.
While the impacts of biotic, abiotic, and anthropogenic sources of ambient noise are increasingly recognized (Römer, 2013; Reichert and Ronacher, 2015; Wiley, 2015; Slabbekoorn et al., 2018; Dominoni et al., 2020), other potential sources of noise in animal communication are rarely explored. One such source is inconsistency in signal production (Gerhardt and Watson, 1995; Nehring et al., 2013; Tanner and Bee, 2019, 2020). In some systems, the degree of consistency in signal production might itself function as a signal if consistent motor performance is a reliable indicator of mate quality (Ballentine, 2009; Byers et al., 2010). However, inconsistent signal production is also an important source of noise to consider. As Nehring et al. (2013) note, when “intra-individual variation does not convey any information that is useful for the receiver, it is noise, since it potentially makes it harder for the receiver to identify and interpret the information” (p. 378). Noise in signal production is particularly important in the context of mate choice because it has potential to obscure the between-individual differences that are frequently considered the targets of mate selection by receivers (Gerhardt and Watson, 1995; Tanner and Bee, 2019, 2020). Acoustic signals, for example, are typically produced repeatedly during sexual advertisement or courtship and also comprise repeated constituent elements, such as pulses or notes, that are not produced identically upon every iteration. Substantial inconsistency in signal production, even over short time intervals such as a single bout of signaling, has been widely reported in diverse taxa (e.g., orthopterans, Shaw and Herlihy, 2000; fish, Amorim and Vasconcelos, 2008; anurans, Gerhardt, 1991; reptiles, Crews, 1975; mammals, Mitani and Brandt, 1994). Despite widespread documentation of inconsistent signal production, and the comparative wealth of data demonstrating female preferences with regard to between-individual differences, few studies have investigated if and how inconsistent signaling affects signal recognition and discriminability either using simulations (Lengagne et al., 2016) or empirically (Gerhardt and Watson, 1995; Tanner and Bee, 2019, 2020). Even fewer studies have investigated the potential interaction between ambient noise and inconsistent signaling. One likely reason for the dearth of previous work on inconsistent signaling is the historical primacy of investigating the criteria that receivers use to discriminate among signalers using experimental stimuli designed explicitly to remove natural levels of within-individual variation in signal production as a potential experimental confound.
Variation in advertisement call traits within and among males, and associated female preferences, have been particularly well-studied in North American treefrogs (Hylidae) (Gerhardt, 2001; Gerhardt and Huber, 2002), such as Cope’s gray treefrog (Hyla chrysoscelis) (Gerhardt and Doherty, 1988; Gerhardt, 2001, 2008; Bush et al., 2002; Bee, 2008b; Ward et al., 2013b; Tanner et al., 2017). We recently showed that both ambient chorus noise and inconsistent signaling impair the ability of female H. chrysoscelis to discriminate among potential mates based on differences in their rate of call production (“call rate”) (Tanner and Bee, 2020), a potential non-arbitrary signal of male quality due to the high metabolic cost of signaling (Taigen and Wells, 1985). In the present study, we investigated the effects of ambient chorus noise and inconsistent signaling on the ability of female H. chrysoscelis to discriminate among potential mates based on a signal of species identity. Male H. chrysoscelis produce advertisement calls that consist of a series of repeated constituent elements, that is, a sequence of about 12 to 43 sound pulses generated via independent contractions of the body wall (McLister et al., 1995; Girgenrath and Marsh, 1997). Based on analyses of 1000 calls (20 calls/male; 50 males) the temperature-corrected (20°C) mean rate of pulse production within individual calls was 48.8 pulses per second [pps], and across males in the population, temperature-corrected mean pulse rates ranged between 41 and 64 pps (Ward et al., 2013b). Female H. chrysoscelis rely upon pulse rate to distinguish between conspecific males and males of the closely related eastern gray treefrog (Hyla versicolor), a cryptic, tetraploid sister species that breeds syntopically across their shared range (Bush et al., 2002; Schul and Bush, 2002). Males of H. versicolor produce an acoustically similar call with a slower temperature-corrected (20°C) mean pulse rate of 21.5 pps (based on analyses of 368 calls from 13 males; 14 to 58 calls/male) (Ward et al., 2013b). Across males in the population, the temperature-corrected mean pulse rate of H. versicolor calls ranged between 17 and 35 pps. Female H. chrysoscelis reject calls with pulse rates substantially lower or higher than the species typical rate (Bush et al., 2002; Gerhardt, 2008; Ward et al., 2013a). Within the natural range of variation in conspecific pulse rates, females have directional preferences for faster pulse rates (Bush et al., 2002; Tanner et al., 2017). Mismating with H. versicolor is costly because resulting offspring are sterile triploids (Gerhardt et al., 1994; Tucker and Gerhardt, 2012).
Previous studies of H. chrysoscelis have measured the extent of within-individual variation in pulse rates (Ward et al., 2013b) and established that inconsistency does not signal body condition or size (Tanner and Bee, 2019) and thus potentially acts as a source of noise in the communication channel in that it could obscure receiver estimates of meaningful call characteristics. Furthermore, while female H. chrysoscelis have preferences for more consistent (less variable) call rates, they do not discriminate among signals on the basis of inconsistency in pulse rate alone (Tanner and Bee, 2019). Here, we tested the hypothesis that ambient noise and inconsistent signaling, both independently and synergistically, impair the expression of female preferences for the pulse rates of male advertisement calls. Female preference functions were assayed across a biologically relevant range of mean pulse rates using two-alternative choice tests in which subjects were able to choose between two sequences of synthetic calls that simulated two calling males. Realistic levels of pulse rate inconsistency were introduced in both sequences by manipulating the within-individual coefficient of variation (CVw) in this call property. We performed the experiment in quiet and at three levels of ambient noise designed to reflect variation in the background noise levels of gray treefrog choruses. We predicted that high levels of both ambient noise and inconsistency would impair signal discrimination on the basis of differences in mean pulse rate, and that a synergistic effect of both noise sources would further impair pulse rate discrimination. We further predicted that response latencies would increase in noisy conditions and when subjects discriminated between highly inconsistent and more similar pulse rates because, in these difficult discrimination tasks, subjects might benefit from increased assessment times.
Materials and Methods
Subjects
Subjects were 289 gravid female H. chrysoscelis of the western mtDNA lineage (Ptacek et al., 1994) captured in amplexus. Amplectant pairs were collected by hand at night (2200-0200) during the breeding season (mid-May to early July) in 2015, 2016, and 2017. Gravid female treefrogs collected in amplexus are as discriminating as those captured prior to pair formation (Murphy and Gerhardt, 1996). Capture sites were located in east-central Minnesota and included Carver Park Reserve (44.52490, −93.43031; Carver County), Richardson Nature Center (44.84214, −93.37148; Hennepin County), Crow-Hassan Park Reserve (45.19471, −93.65368; Hennepin County), and Lake Maria State Park (45.32012, −93.94389; Wright County). Treefrog pairs were housed in plastic containers that were labeled with unique ID numbers and taken to the laboratory, where the frogs were placed in aged tap water and maintained at approximately 2–4°C for up to 36 h to delay the deposition of eggs. Prior to testing, we placed each pair in room-temperature aged tap water inside a temperature-controlled incubator at 20°C for at least 30 min, until they reached a body temperature of 20 ± 1°C. In empirical studies of pulse rate discrimination, temperature control is essential because male signal production and female mating preferences are temperature dependent (Gerhardt, 1978; Gerhardt and Huber, 2002). We released all treefrogs at their original capture sites within 3 days of collection. The subjects were not marked. Because females probably breed only once or at most twice during the relatively short breeding season in Minnesota (Ritke et al., 1990), and because we collected frogs from multiple, large wetlands over multiple years, the risk of recapturing the same individuals, and associated risk of pseudoreplication, is very low. We chose to tolerate this small risk of unknowingly testing the same individual twice rather than subjecting each individual to invasive marking procedures that carry some risk to the animals (e.g., toe-clipping) and typically do not last multiple years.
Acoustic Stimuli
We generated synthetic treefrog calls and ambient noise de novo using custom scripts in MATLAB® versions 2015a and 2016a (The Mathworks, Natick, MA, United States). All stimuli were generated at a sample rate of 44.1 kHz and a bit-depth of 16. Each of the two stimuli generated for a given two-alternative choice test consisted of a sequence of synthetic calls that simulated a male treefrog producing an advertisement call at a constant rate. A total of 1,820 unique stimuli were used in our phonotaxis tests, and no call sequence was assigned to more than one subject. We modeled the stimuli after natural advertisement calls produced by Cope’s gray treefrogs in east-central Minnesota, using the mean trait values published in Ward et al. (2013b) to set the values of all call traits not under consideration. Calls comprised 30 pulses with a constant, 50% pulse duty cycle. We manipulated the mean pulse rates of calls and the within-individual variation in pulse rate across the calls in a given call sequence. Within each call, pulse duration and interpulse interval were equal and determined as functions of the experimentally determined pulse rate and the fixed 50% pulse duty cycle. Call duration was always fixed in terms of the number of pulses per call (30 pulses), but the absolute duration of each call, measured in milliseconds, was allowed to vary as necessary to accommodate different pulse rates (the study-wide slowest pulse rate of 31.68 pps yielded a call duration of 931.2 ms; the study-wide fastest pulse rate of 54.19 pps yielded a call duration of 544.4 ms). Individual pulses were constructed by adding two phase-locked sinusoids with frequency components at 1,250 Hz (−11 dB) and 2,500 Hz (0 dB) and the amplitude envelope of each pulse was shaped with species-typical onsets and offsets that were fixed proportions (0.36 and 0.64, respectively) of pulse duration. The amplitude envelope of each call was given a linear onset over the first 50 ms. Stimuli were played back at 85 dB (SPL re 20 μPa, fast RMS, C-weighted), measured at a distance of 1 m, to approximate the level of a natural call (Gerhardt, 1975). Previous studies have demonstrated that females respond readily in choice tests with similarly designed synthetic stimuli and playback methodology (Ward et al., 2013b; Tanner et al., 2017; Tanner and Bee, 2019).
We generated ambient, “chorus-shaped” noise by filtering white noise to have the average long-term spectral characteristics of a gray treefrog chorus following the procedures outlined in Vélez and Bee (2011). To ensure any effects of chorus-shaped noise were not artifacts of a particular realization of a randomly generated stimulus, we replicated the noise files (N = 34). No more than ten subjects were assigned the same noise replicate. We generated the noise replicates at a sample rate of 11.025 kHz and then upsampled to 44.1 kHz; this was done to circumvent limited computing power during stimulus construction.
Experimental Design
We used a series of two-alternative choice tests to measure the shapes of female preference functions for pulse rate in the presence of ambient noise and signal inconsistency. Stimuli consisting of sequences of calls were constructed by randomly drawing a value for the pulse rate of each consecutive call within a sequence from a normal distribution having a mean and standard deviation that were specified to allow us to manipulate both mean pulse rate and inconsistency in pulse rate across treatments. The means of separate distributions were centered on pulse rates that were either −3, −2, or −1, or 0 SD from the population mean, generating nominal mean pulse rates of 35.6, 40.0, or 44.4, and 48.8 pps, respectively. We assayed female preferences between −3 and 0 standard deviations of the population mean pulse rate because, as noted previously, female H. chrysoscelis use pulse rate to discriminate between conspecific and heterospecific males (Schul and Bush, 2002). Males of the closely related H. versicolor produce acoustically similar calls with slower pulse rates and female H. chrysoscelis discriminate against slower-than-average conspecific pulse rates (Gerhardt et al., 1994; Bush et al., 2002; Ward et al., 2013a; Tanner et al., 2017). Note that a value of −2 SD (40.0 pps) corresponds to the lower end of the range of variation in conspecific (H. chrysoscelis) pulse rates (adjusted to 20°C), whereas −3 SD (35.6 pps) falls outside the range of conspecific pulse rates but approximates the upper end of the range of variation in heterospecific (H. versicolor) pulse rates (adjusted to 20°C).
To investigate the impacts of ambient noise, we replicated all two-alternative choice tests in quiet and at three levels of ambient noise (60, 70, and 80 dB SPL). In the quiet condition, no noise was broadcast. The three levels of broadcast ambient noise were chosen to approximate the mean (70 dB SPL) and ±1 SD (10 dB) of the sound pressure levels of background noise measured in east-central Minnesota gray treefrog choruses (Tanner and Bee, 2019).
We manipulated inconsistency in pulse rate by controlling in our synthetic signals the within-individual coefficient of variation in this property across calls within a sequence simulating a calling male (CVw = SD/X̄). That is, the pulse rate of each individual call within a stimulus sequence was fixed, but pulse rate was allowed to vary across calls within a sequence. In addition to calls that were invariant within a sequence (CVw = 0.000), we chose three nominal levels of within-individual (i.e., within-sequence) variation (CVw = 0.004, 0.010, 0.030; Figure 1) to match the minimum, mean, and maximum CVw previously measured in the population (Ward et al., 2013b). These population estimates of pulse rate inconsistency are based on analyses of single call bouts comprising 20 consecutively produced calls from each of 50 males (1,000 calls total); the average 20-call bout lasted 133.5 s (range: 70.4–291.3 s) (Ward et al., 2013b). To introduce realistic levels of inconsistency in pulse rate into our stimuli, we manipulated the standard deviation of the normal distributions centered on each target mean pulse rate to achieve the three desired levels of CVw for each mean pulse rate. Hence, the pulse rates of consecutive calls in each sequence were independently chosen from a distribution that allowed pulse rate to vary inconsistently according to the nominal level of CVw around the specified nominal mean pulse rate of the stimulus (see Figures 1A,B). Higher levels of inconsistency (larger CVw) correspond to broader normal distributions and thus generated more variable synthetic signals. To ensure that randomly chosen pulse rates fell within a behaviorally relevant range, we excluded pulse rate values that were higher than the fastest H. chrysoscelis pulse rate reported in Ward et al. (2013b) or lower than three standard deviations slower than the mean H. versicolor pulse rate.
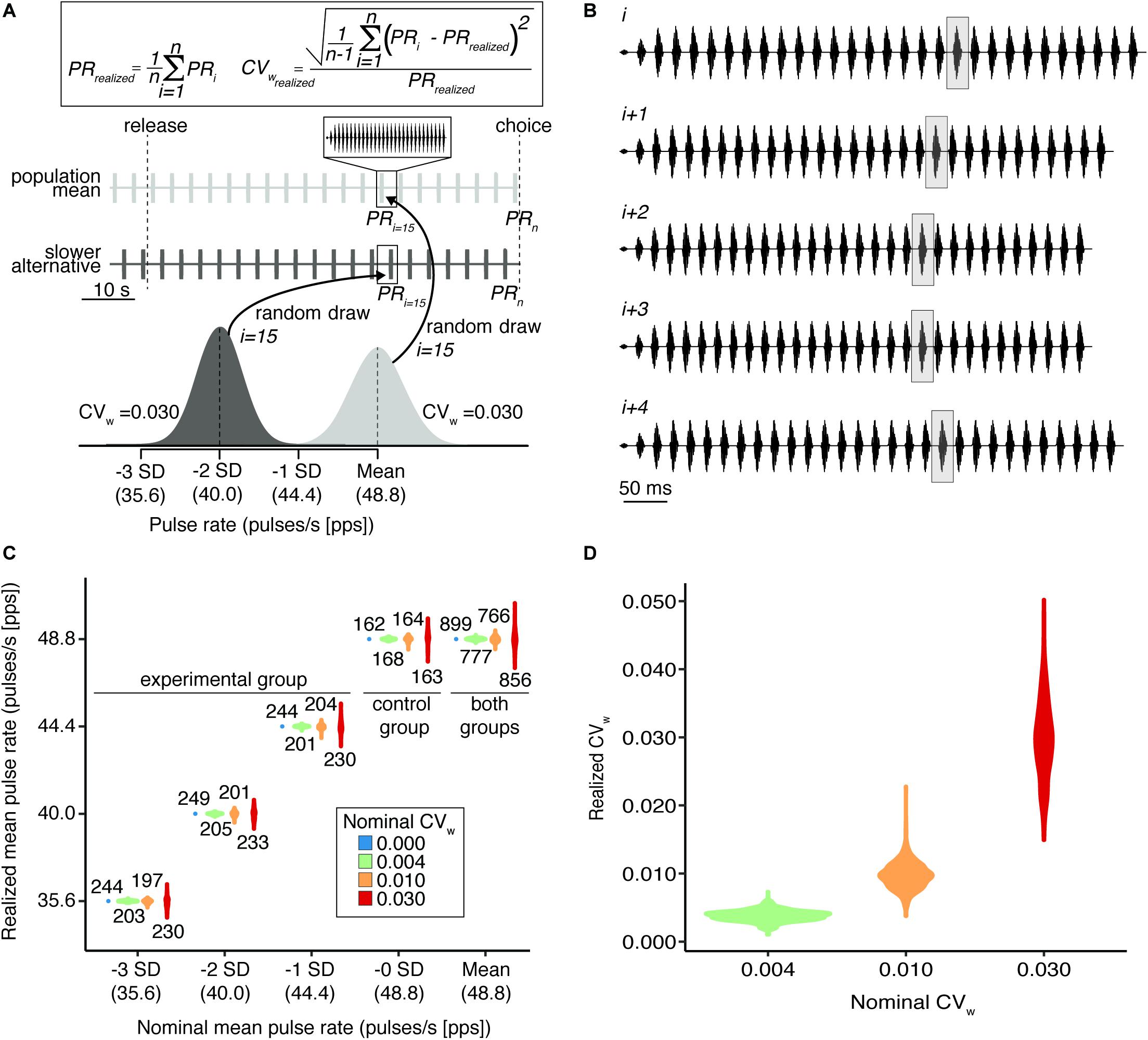
Figure 1. Relationships between realized and nominal trait values in experimental stimuli. Nominal values represent experimentally specified levels while realized values reflect averages among the stimuli that were heard by the subject in each trial. The trait values of individual calls in stimuli with higher assigned CVw were drawn from broader distributions, making these stimuli more variable. (A) Random draws from normal distributions representing the population mean (light gray) and –2 SD (dark gray) distributions used to generate two alternative signal sequences (waveforms) for a single behavioral trial. Equations (top) demonstrate the computation of realized mean pulse rate and realized CVw for a given sequence. (B) Waveforms of five representative calls with randomly drawn pulse rates (i to i + 4) from a representative stimulus sequence whose nominal mean pulse rate was 0 SD (48.8 pps) in the CVw = 0.030 inconsistency level. The 20th pulse in each call is highlighted in gray to aid in visualizing the variable pulse rate across calls. (C) Violin plots show the distributions of realized alternative pulse rates in experimental stimuli grouped by nominal mean pulse rate. Subjects assigned to the experimental group were given a choice between a stimulus whose nominal mean pulse rate matched the population mean of 48.8 pps (Mean) and an alternative stimulus with a nominally slower pulse rate (i.e., Mean versus –3, –2, or –1 SD). Subjects assigned to the control group were given a choice between two equivalent stimuli whose nominal mean pulse rates matched the population mean (i.e., Mean versus –0 SD). Text annotations show the sample size, in number of tests, at each combination of nominal mean pulse rate and coefficient of variation within stimuli (CVw). Because in each test subjects across both groups chose between a stimulus with a mean pulse rate (Mean) and one whose pulse rate was –3, –2, –1, or –0 SD from the mean, the sample sizes of the Mean alternative are necessarily sums of the other four tests performed at the same nominal level of within-individual variation. (D) Distributions of realized CVw across three nominal levels of within-individual variation. Invariant stimuli necessarily have a realized CVw of 0 and are not depicted in (D). In both panels (C,D), colors represent nominal levels of within-individual variation.
We designated separate experimental and control treatment groups. For subjects in the experimental group, we measured how female preferences for pulse rates at the population mean (48.8 pps) over slower pulse rates changed in the presence of ambient noise and inconsistency using a 3 × 4 × 4 factorial design in which we manipulated the mean pulse rate of the slower alternative stimulus (PR = 35.6 [−3 SD], 40.0 [−2 SD], 44.4 pps [−1 SD]; within subjects), ambient noise levels (quiet, 60, 70, and 80 dB SPL; within subjects), and levels of inconsistency (CVw = 0.000, 0.004, 0.010, 0.030; between subjects). Thus, each subject in the experimental group was assigned to a single level of inconsistency and tested in up to 12 behavioral trials (Nsubjects = 246; Ntrials = 2,641) in which the choice was between two equally inconsistent signals with different mean pulse rates (i.e., population mean versus −3, −2, or −1 SD) across four levels of ambient noise. Subjects in the control group (Nsubjects = 43; Ntrials = 657) chose between two signals with equal nominal mean pulse rates set at the population mean of 48.8 pps (i.e., population mean versus −0 SD) across all 16 factorial combinations of ambient noise (quiet, 60, 70, and 80 dB SPL; within subjects) and inconsistency (CVw = 0.000, 0.004, 0.010, 0.030; within subjects).
It is important to note that for both treatment groups, the two alternative stimuli in a given choice test always had the same experimentally specified, nominal level of inconsistency. Hence, all subjects in both treatment groups chose between two equally inconsistent alternatives; subjects in the experimental group were given choices of stimuli having different nominal mean pulse rates, whereas subjects in the control group chose between two stimuli with the same nominal mean pulse rate of 48.8 pps.
Testing Protocol
We conducted choice tests in a custom-built, temperature controlled, semi-anechoic chamber (2.8 m × 2.3 m × 2.1 m, length × width × height; IAC Acoustics, North Aurora, IL, United States) at 20 ± 1°C. The chamber walls and ceiling were acoustically insulated and covered in dark gray, perforated material (IAC PlanarchoicTM panel system). The floor was covered with dark gray, low-pile carpet. The testing arena was circular (2.0 m × 0.6 m, diameter × height) and constructed from hardware cloth covered with black fabric. We placed an acoustically transparent release cage (9 cm × 2 cm, diameter × height) on the floor in the center of the arena. The cage could be operated by means of a rope-and-pulley system by an observer outside the chamber. We used two Mod1 Orb speakers (Orb Audio, New York, NY, United States) for sound playback. We positioned the two speakers 90° apart on the floor outside the arena wall. Phonotaxis trials were conducted under infrared light (Tracksys, Ltd., Nottingham, United Kingdom) and scored in real time by a trained observer from outside the chamber by means of a closed-circuit television system with an infrared-sensitive video camera (Panasonic WV-BP334; Panasonic Corporation of North America, Secaucus, NJ, United States) mounted from the chamber ceiling.
Synthetic signals and ambient noise were broadcast using Adobe Audition 3.0 (Adobe Systems, Inc., San Jose, CA, United States) on a Dell Optiplex 980 PC (Dell Computer Corporation, Round Rock, TX, United States). We output audio using a MOTU model 16A 16-channel sound card (MOTU, Inc., Cambridge, MA, United States) and amplified it using Crown XLS1000 High-Density Power Amplifiers (HARMAN Professional, Northridge, CA, United States). We calibrated sounds to their target SPLs using a Bruël and Kjær Type 4950 microphone (Bruël and Kjær, Nærum, Denmark) placed 1 m from the speaker at the approximate position of a subject’s head at the beginning of a choice test. Signals and noise were broadcast from the same speaker (co-located). In a given choice test, we broadcast the same noise replicate from both speakers simultaneously and calibrated the playback level of the ambient noise with the microphone pointed directly between the speakers such that noise from both sides contributed equally to the summed SPL.
To prevent side bias and control for presentation order, we randomized for each subject which signal was played first during a choice test, the order in which tests were conducted, and which speaker played the stimulus with the nominally slower pulse rate. For subjects in the control group, we arbitrarily labeled the two nominally equivalent stimuli and then randomized the playback speaker. At the beginning of a choice test, we separated the subject from her mate and placed her in the release cage. We allowed each subject to acclimate in the quiet chamber for 60 s. At the end of the acclimation period, we began playback. When ambient noise was present, the noise began first and played for 30 s prior to the broadcast of signals. When ambient noise was absent, silence continued for a further 30 s to ensure subjects spent the same amount of time in the release cage at the beginning of each test, regardless of the ambient noise condition. The two alternative signals were played back in an alternating and non-overlapping temporal arrangement and spaced such that there was an equal period of silence before and after each call.
When the two alternating signals had each played twice, the lid of the release cage was lifted, and the subject was allowed to move freely within the arena. Tests continued until one of the following conditions was met: (1) the subject indicated a choice by entering a response zone defined as a 10 cm radius semi-circle in front of a playback speaker; (2) the subject touched the arena wall in the quadrant of the arena opposite to the quadrant separating the two playback speakers; (3) at the end of 3 min following the lifting of the lid, the subject had not left the release cage; or (4) at the end of 5 min, the subject had not entered a response zone.
We recorded the subject’s binary choice and her latency to respond, measured as the time elapsed between release from the cage and entering a response zone. Female treefrogs usually make mating decisions in 1–3 min (Schwartz et al., 2004; Tanner et al., 2017); the mean response latency across all subjects in the present study was 88.0 ± 50.8 s. This corresponds closely to the duration of the individual calling bouts over which inconsistency in pulse rate was determined by Ward et al. (2013b). Between tests, subjects were housed with their mates and returned to the incubator for a “time out” of at least 3 min. When a choice was not indicated within 5 min, the outcome of the test was scored as “no response” and following a time out, the subject was re-tested in the same test. Subjects that scored no response twice for the same test (51 of 246 subjects in the experimental group and 4 of 49 subjects in the control group) were not tested further, but we included their responses from completed tests in our statistical analyses. On average, subjects that did not complete the entire battery of tests completed 6 of 12 tests (range 1 to 11) in the experimental group and 8 of 16 tests (range 2–12) in the control group. Responses from the two groups were combined for statistical analyses.
Statistical Analysis
Experimental stimuli had trait values drawn from normal distributions and were therefore intrinsically variable and dependent on the response latency of the subjects (Figure 1). In trials in which subjects listened longer before indicating a choice, realized mean pulse rates and realized levels of inconsistency more closely matched the nominal levels specified by our experimental design. It was therefore necessary to account for the trait values of experimental stimuli that were actually experienced by a given subject during a given trial. Otherwise, stochastic deviations from the nominal pulse rate and CVw specified by our treatment levels would introduce error into analyses. To account for the trait values subjects actually experienced, we performed a post hoc calculation of the realized mean pulse rate and CVw in the sequence of signals experienced by the subject during the trial (Figure 1). We used the response latency to exclude calls from each sequence that the subject did not hear (i.e., that did not occur) between the beginning of playback and indicating a choice. We then calculated the average CVw as the arithmetic mean of the realized CVw values computed for the two alternative stimuli in each test. We did this to reduce the dimensionality of predictor variables. Because both stimuli in a given test always had the same nominal level of inconsistency, across the experiment we observed that the mean difference between the realized CVw values for the two alternative stimuli in a given choice test was very small (9.07 × 10–5; 95% of differences fell in the interval [−6.04 × 10–5, 2.42 × 10–4]; range [−2.74 × 10–2, 2.58 × 10–2]).
We fit two generalized estimating equation (GEE) models to examine female preference functions based on two response variables: binary choices (the probability that a subject chose the stimulus with the faster pulse rate) and response latencies (time elapsed prior to making a choice). GEE is an extension of generalized linear models (GLM) compatible with either binary or continuous response variables and designed for repeated measures of the same individual (Hardin and Hilbe, 2012). We specified exchangeable correlation structures, which assume that correlations between observations of the same subject are homogenous. Wald statistics with a significance criterion of α = 0.05 were used for hypothesis testing. In each model, we included the following independent variables: main effects of the realized mean pulse rate of the stimulus with the slower pulse rate, average realized CVw, and ambient noise level, and all two-way interactions.
Results
Within the range of pulse rates tested, subjects showed pronounced preferences for calls with realized mean pulse rates (hereafter, “pulse rate”) near the population mean over calls with slower pulse rates, but were less likely to choose the faster pulse rate when the two alternatives had similar rates (β = −0.288, W = 85.90, p < 0.001; Figure 2 and Table 1). The probability of choosing the population mean pulse rate over a slower alternative was 0.770 in two-choice tests overall (2,540 of 3,298 tests) and 0.819 in tests performed in quiet with invariant stimuli (186 of 227 tests). Subjects were most likely to choose the population mean pulse rate when the pulse rate of the alternative was much slower (Figure 2); in tests with a nominal pulse rate of 35.6 pps (−3 SD), for example, subjects chose the faster, population-mean pulse rate with probability 0.946 (827 of 874 tests; Figure 2). Results from the control group confirmed that playback speaker assignment (and thus side of the testing arena) did not affect the outcome of trials (GEE: β = −0.077, W = 0.04, p = 0.840), suggesting no side bias was present in our experimental set-up.
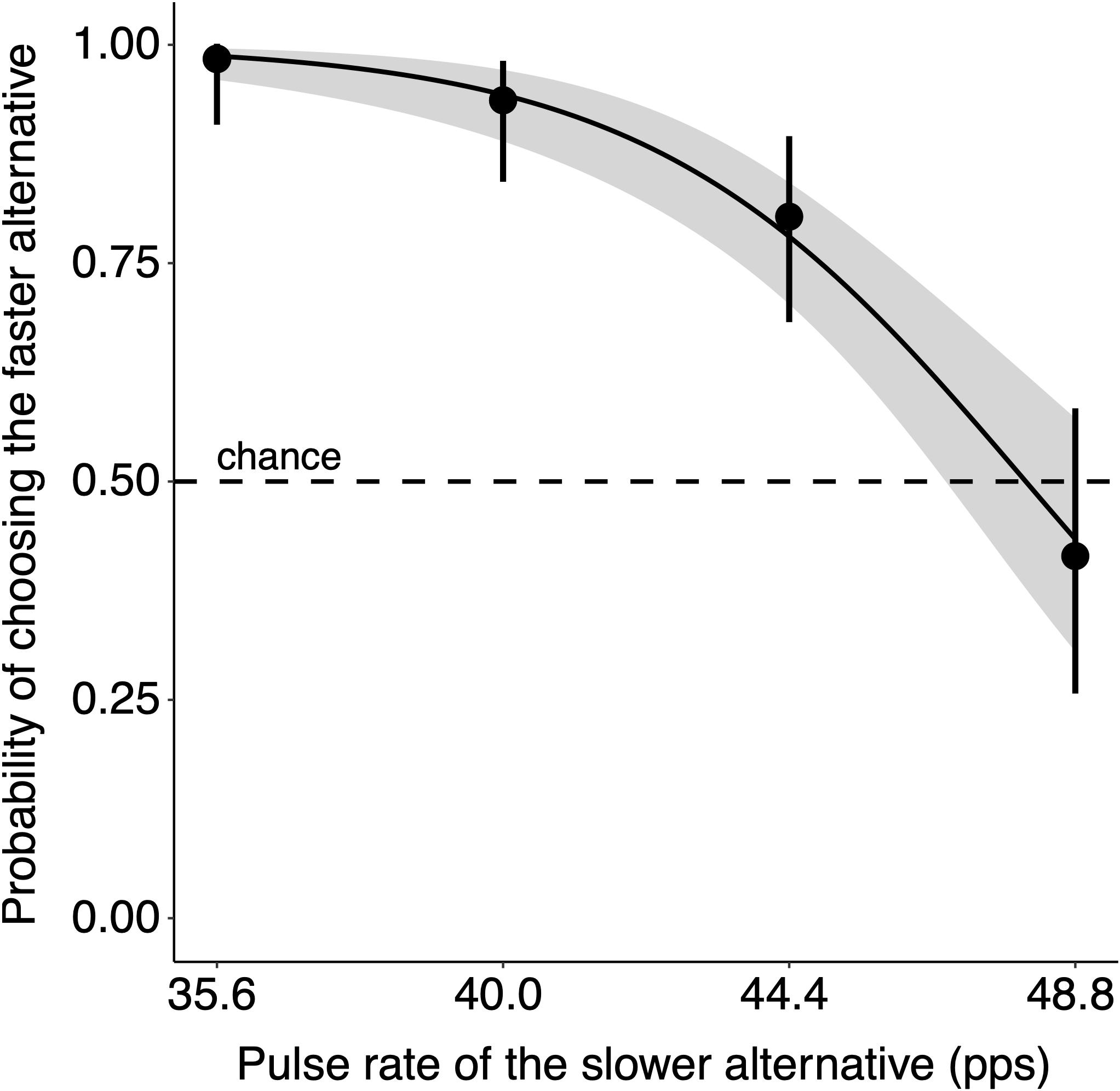
Figure 2. Pulse rate preference function in quiet conditions and the absence of inconsistency (CVw = 0). Subjects chose between a stimulus whose nominal mean pulse rate was equal to the population mean (0 SD = 48.8 pps) and a stimulus whose nominal mean pulse rate was 0, 1, 2, or 3 SD slower (48.8, 44.4, 40.0, or 35.6 pps, respectively). The proportion choosing the faster realized mean pulse rate declined as the two alternative pulse rates became more similar. Individual points show the proportion of tests in which subjects chose the faster stimulus calculated across all tests with the same nominal mean pulse rate. The solid black line shows the logistic regression line of best fit with gray shading illustrating standard error. The dashed line shows 0.5, or the probability of choosing the stimulus with the faster pulse rate by chance. The error bars show 95% Clopper–Pearson (“exact binomial”) confidence intervals.
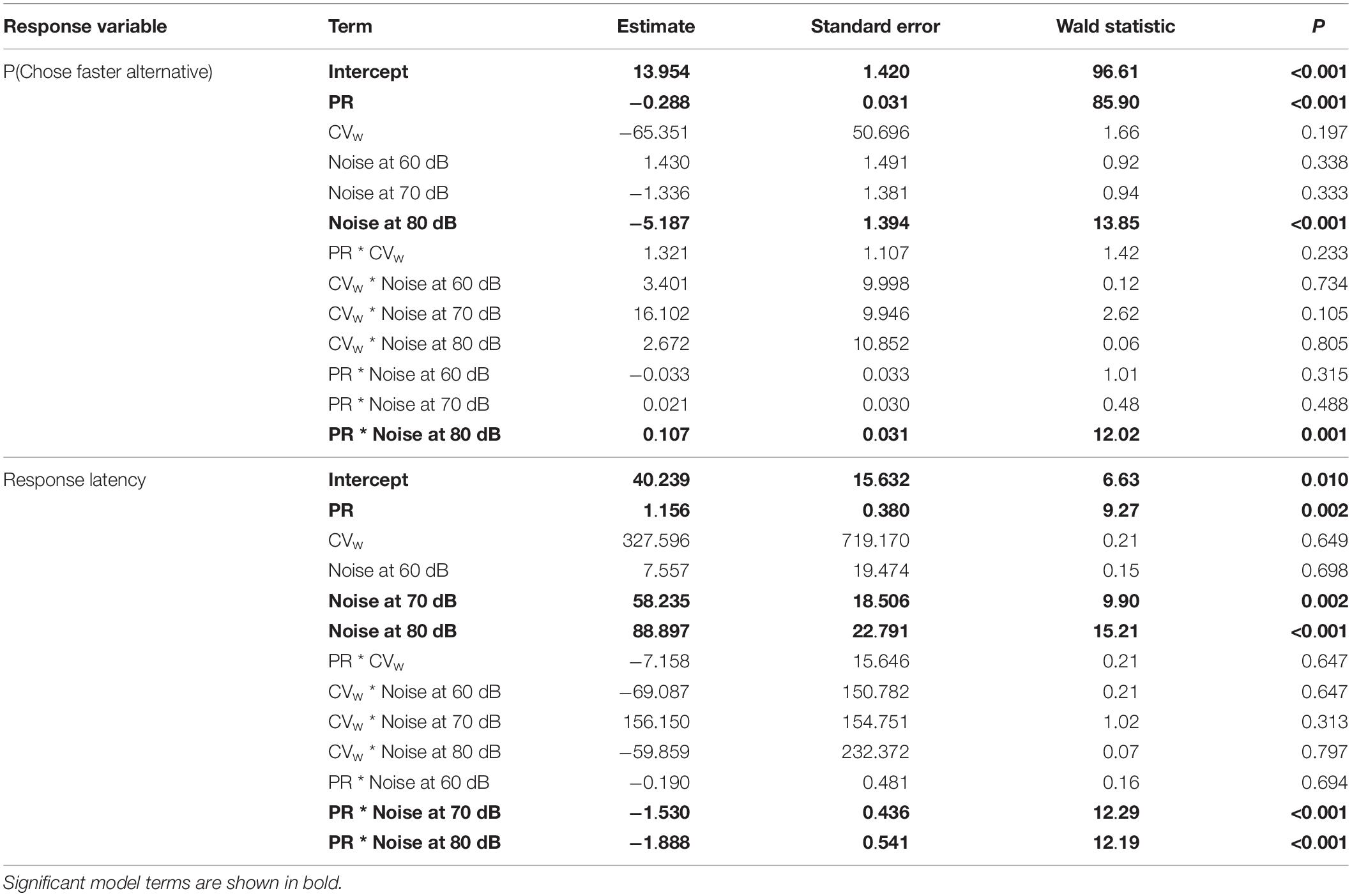
Table 1. Output from two GEE models – one for the proportion of subjects choosing the population mean pulse rate over a slower pulse rate and one for response latency – examining the effects of the realized mean pulse rate (PR) of the slower pulse rate stimulus, arithmetic mean of the realized coefficients of variation in the two alternative stimuli (CVw), and ambient noise level (Noise), including all two-way interactions (Nsubjects = 289, Ntrials = 3,298).
Ambient noise presented at the highest experimental level impaired female expression of pulse rate preferences (Figures 3A,B and Table 1). Subjects were significantly less likely to choose the faster, population-mean pulse rate in the 80-dB condition (probability 0.723; 581 of 803 tests; β = −5.187, W = 13.85, p < 0.001) than in quiet (probability 0.795; 662 of 833 tests). There was a significant two-way interaction between the pulse rate of the slower stimulus and the highest level of ambient noise (β = 0.107, W = 12.02, P < 0.001), such that female preference functions for pulse rate were less steep when measured in the presence of ambient noise at 80 dB. In contrast, the level of inconsistency in the two alternative stimuli, measured as the average realized CVw, had no effect on the probability that subjects chose the stimulus with the population-mean pulse rate (β = −65.351, W = 1.66, p = 0.197; Figure 3B). Subjects chose the population-mean pulse rate with probability 0.793 in tests with perfectly consistent stimuli, compared to 0.744 (CVw = 0.004), 0.768 (CVw = 0.010), and 0.771 (CVw = 0.030) in tests using calls with inconsistent pulse rates (Supplementary Figure S1). Inconsistency and ambient noise did not interact synergistically to affect the probability of choosing the faster pulse rate (Table 1 and Supplementary Figure S2).
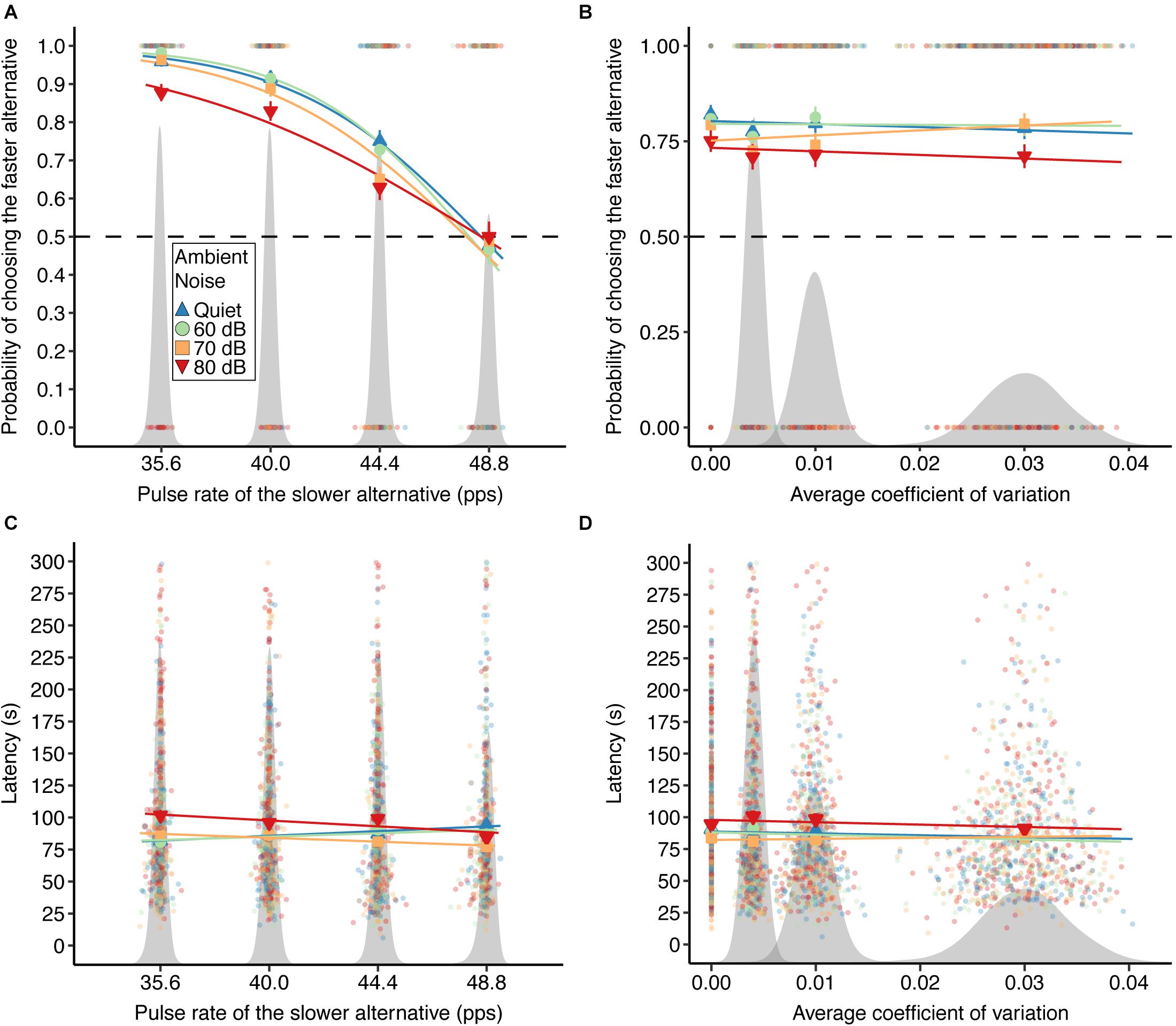
Figure 3. The effects of pulse rate, ambient noise, and inconsistency on receiver responses. (A) The probability of choosing the preferred, faster pulse rate declined as a function of the realized pulse rate of the slower alternative. (B) The probability of choosing the faster stimulus was not affected by the average CVw of the two alternative stimuli. In panels (A,B), the dashed black line shows 0.5, the probability of choosing the stimulus with the faster pulse rate at random. Response latency as a function of (C) the realized pulse rate of the slower alternative and (D) the average coefficient of variation within males. In all panels, the results of individual tests are shown with pale, circular symbols. Colors and symbols shown in the legend depict mean response variables within combinations of predictors, while lines show linear model fits and error bars show standard errors. Different colors and symbols represent levels of ambient noise. Gray shading shows the distributions of experimental stimuli for each plot: realized mean alternative pulse rates are shown in panels (A,C), and average realized coefficients of variation are shown in panels (B,D).
The mean (±SD) response latency across all trials was 88.0 ± 50.8 s (range 6–300 s). Response latency modestly increased as a function of the pulse rate of the slower stimulus (β = 1.156, W = 9.27, p = 0.002; Figure 3C), such that on average, subjects spent longer making decisions when two alternatives had more similar pulse rates. When tests were performed in quiet with perfectly consistent signals, mean response latencies were 81.9 ± 40.6, 87.0 ± 47.9, 87.3 ± 48.3, and 94.0 ± 54.3 s when the nominal pulse rate of the designated slower alternative was 35.6 (−3 SD), 40.0 (−2 SD), 44.4 (−1 SD), and 48.8 (0 SD) pps, respectively. Accounting for the pulse rate of the slower alternative and inconsistency, ambient noise at both 70 dB (β = 58.235, W = 9.90, p = 0.002) and 80 dB (β = 88.897, W = 15.21, p < 0.001) significantly increased response latencies, such that subjects spent more time making decisions when listening in population-mean and above-average noise levels. The pulse rate of the slower stimulus interacted significantly with ambient noise at both 70 dB (β = −1.530, W = 12.29, p < 0.001) and 80 dB (β = −1.888, W = 12.19, p < 0.001) such that the effect of the slower mean pulse rate was reversed at these levels of ambient noise relative to the quiet and 60 dB conditions (Figure 3C). There was no significant effect of inconsistency on response latency (β = 327.596, W = 0.21, p = 0.649; Figure 3D). Mean response latencies were 89.2 ± 46.3 s (CVw = 0.000), 89.6 ± 55.2 s (CVw = 0.004), 87.7 ± 52.3 s (CVw = 0.010), and 85.6 ± 49.9 s (CVw = 0.030) within nominal levels of inconsistency. Inconsistency and ambient noise did not interact synergistically to affect the latency to respond (Table 1).
Discussion
We examined the impact of ambient noise and inconsistent signal production on species recognition by female Cope’s gray treefrogs. Our main results can be summarized as follows. Consistent with earlier studies, we found pronounced directional preferences for the population-mean pulse rate over slower pulse rates (Gerhardt, 2008; Ward et al., 2013a; Tanner et al., 2017). In general, subjects discriminated reliably between signals on the basis of pulse rate, reflecting the robust nature of species recognition mechanisms in gray treefrogs. Consistent with our hypothesis, however, natural levels of ambient noise simulating a gray treefrog chorus had significant impacts on mate choice. Specifically, discrimination against slower-than-average pulse rates was reduced, and subjects spent longer making mating decisions, in high levels of ambient noise. In stark contrast to our hypothesis, inconsistency in signal production had no measurable effects on pulse rate discrimination, and it did not interact synergistically with ambient noise.
Ambient Noise
While mate choice was unaffected by noise presented at the lowest level, ambient noise presented at a high amplitude caused females to choose the slower, non-preferred signal more often than they did in quiet. In nature, the sound levels of gray treefrog choruses can be highly variable from one night to the next (Tanner and Bee, 2019) and the extent of the masking effect of ambient noise experienced by receivers is also variable according to the spatial relationship between the target signal and the noise source (Bee, 2007, 2008a; Nityananda and Bee, 2012; Ward et al., 2013a; Caldwell et al., 2016). Thus, both night-to-night and spatial variation in the intensity of selection imposed on males and signals by treefrog receivers are to be expected. As a population-level consequence, selection on pulse rate may be less intense than estimates made in quiet listening conditions (e.g., Tanner et al., 2017) would otherwise suggest. Individual H. chrysoscelis receivers should be at greater risk of making pulse rate discrimination errors on nights when the chorus is better attended and, thus, noisier. Errors in pulse rate discrimination can potentially lead to errors in species recognition in this system (e.g., Bee, 2008a), which would have potentially devastating consequences, because heterospecific matings produce sterile, triploid offspring (Gerhardt et al., 1994; Servedio and Noor, 2003; Tucker and Gerhardt, 2012). Such call trait discrimination errors are also expected to result in reduced receiver fitness if specific features of the call signal mate quality (Bee, 2008b; Ward et al., 2013b; Tanner and Bee, 2020).
Response latencies were also significantly higher in the presence of high levels of ambient noise; however, the estimated increase in response latencies reported here was modest in the context of a single breeding episode. At present, it is difficult to assess the extent to which such small noise-induced increases in response latency might materially affect individuals under natural mate searching conditions. This difficulty arises, in part, because we currently lack sufficient data on female sampling strategies prior to mate choice in frogs (Murphy and Gerhardt, 2002; Schwartz et al., 2004; Murphy, 2012) to definitively conclude that increases in response latency in the presence of ambient noise negatively impact females or their mating decisions under natural listening conditions. However, one viable and potentially relevant consequence of increased response latencies in the presence of ambient noise could be an increased cost of mate searching. For example, time spent searching for a mate may incur missed opportunity costs (i.e., time not spent foraging), or expose females to pond-dwelling predators (e.g., larger frogs, giant water bugs) and parasites (e.g., leeches) to which they might otherwise be less vulnerable (Crowley et al., 1991; Magnhagen, 1991; Grafe, 1997; Zuk and Kolluru, 1998; Martin and Wagner, 2010; Bonachea and Ryan, 2011; Beckers and Wagner, 2018). In addition, mate searching in natural environments may be further complicated, relative to controlled laboratory settings, by the potentially larger distances females must traverse in ponds to select a mate, the increased complexity of natural habitats, the vastly more numerous potential choices in a breeding chorus, and the fact that multicomponent advertisement signals vary along multiple behaviorally relevant dimensions at the same time (Tanner et al., 2017). Thus, the effect of chorus noise on the time spent making decisions may be more important in natural populations. It follows from Signal Detection Theory that increased listening time may serve to prevent errors in trait discrimination because receivers are, in effect, sampling from a distribution of male calls to estimate the central trait value of the signaler and listening for longer is equivalent to drawing more values from the distribution (Wiley, 2006). Consistent with this idea, female túngara frogs may increase their response latencies as the trait values of two alternative signals become increasingly similar (Bosch et al., 2000) and errors in signal discrimination become more likely. We hypothesized that longer listening times might be especially important in the presence of ambient noise when calls were inconsistent, and thus, increased sampling could result in more accurate estimates of mean pulse rates. However, we ultimately found no evidence of synergistic effects between ambient noise and inconsistency.
Overall, our findings on the effects of ambient noise add to a growing body of evidence that suggests receivers of diverse taxa sometimes fail to express well-documented mating preferences in natural soundscapes (Wollerman and Wiley, 2002; Bee and Schwartz, 2009; Bee et al., 2012; Römer, 2013; Reichert and Ronacher, 2015; Lee et al., 2017; Tanner and Bee, 2020). Ambient noise thus provides at least a partial explanation for why between-individual variation in sexually selected traits is maintained in spite of apparently persistent sexual selection (“the lek paradox”; Kirkpatrick and Ryan, 1991; Møller and Pomiankowski, 1993; Pomiankowski and Møller, 1995). The impacts of ambient noise on female preference expression probably also explain, at least partially, why field experiments sometimes fail to show the same sexual selection measured in laboratory studies (Sullivan and Hinshaw, 1992; Friedl, 2006; Dawson and Ryan, 2009). Such findings suggest that experiments performed under ideal listening conditions, that is, in the absence of the ambient noise that is a feature of many natural signaling contexts, may tend to overestimate the intensity of sexual selection on signals because they artificially inflate signal discriminability.
Inconsistent Signaling
Pulse rate preferences were reliably expressed in spite of natural levels of inconsistency in signal production that effectively widened the signal distributions. This key finding is not consistent with our hypothesis and stands in stark contrast with results from an earlier study showing that female preferences for faster rates of call production during a bout of signaling eroded as a function of inconsistency in signaling (Tanner and Bee, 2020). In that study, inconsistency in call rate more profoundly limited expression of female mating preferences than the better-known effects of ambient noise. The striking difference between the impacts of inconsistency on call rate discrimination (Tanner and Bee, 2020) and those on pulse rate discrimination presented here might be explained by the different biological functions of discrimination based on differences in pulse rate versus call rate in H. chrysoscelis. Female H. chrysoscelis use pulse rate (and not call rate) to distinguish between conspecific males and males of the cryptic, tetraploid sister-species, H. versicolor (Schul and Bush, 2002), which produce an acoustically similar call. In contrast, call rate is considered a potential non-arbitrary signal of male quality due to the high energetic costs of calling (Taigen and Wells, 1985; Wells and Taigen, 1986). Compared with mate quality assessment (i.e., call rate discrimination), species recognition (i.e., pulse rate discrimination) may generally be more robust against the impacts of inconsistent signaling because the costs of mating with the wrong species are expected to far outweigh those of mating with a low-quality conspecific (but see Pfennig, 2007).
On the other hand, the difference between the results of the present study and those of Tanner and Bee (2020) may be attributable to the differing magnitudes of inconsistency in pulse rate versus call rate in natural populations, which informed our methodology. While both experiments introduced natural levels of inconsistency, pulse rate is far less variable than call rate within individuals (Gerhardt, 1991; Ward et al., 2013b). Consequently, the distributions of signal traits with different means did not overlap in the experiment described here, which limited the opportunity for mistakes in mean trait value estimation by receivers and thus might account for the absence of effects of inconsistent pulse rates on pulse rate discrimination. A potential linkage between the biological and methodological explanations for the difference in experimental outcomes is that, given the importance of pulse rate in species recognition, past selection may have acted to minimize the level of inconsistency in this call trait. The present study was not designed to test this hypothesis; future studies could do so by incorporating experimental stimuli with levels of inconsistency that exceed the current range of natural variation. An additional factor that may have contributed to these different results is that receiver estimation of pulse rate and call rate take place over different timescales, with pulse rate potentially being estimated within the space of a single call, while call rate must be estimated by listening to multiple calls.
Within-individual variation in pulse rate may play some role in communication in this species, but if so, that role remains unknown. Tanner and Bee (2019) showed that inconsistency in pulse rate did not signal male body size or condition. In the congener Hyla avivoca, males dynamically adjust their interpulse silent intervals (and consequently, the pulse rate) to avoid overlapping pulses with calling neighbors, and females prefer calls with interdigitated pulses to calls whose pulses overlap with those of another call (Martínez-Rivera and Gerhardt, 2008). However, no similar pulse rate adjustment occurs in the gray treefrogs, H. chrysoscelis and H. versicolor (Gerhardt, 1991; Ward et al., 2013b). In recent years, there has been increasing interest in understanding the causes and consequences of various aspects of within-individual variation, including individual plasticity (Nussey et al., 2007; Rodríguez et al., 2013), persistent individual differences in behavior (“animal personality”; Dingemanse and Wolf, 2010; Dingemanse et al., 2010, 2012), and between-individual differences in intraindividual variability (Stamps et al., 2012). However, the interplay of within- and between-individual variation in the context of signal discrimination and mate choice remains poorly understood. Further study is needed to understand how the ubiquitous within-individual variation in communication behaviors – particularly the inconsistency in signal production that occurs within signalers – impacts receiver decision-making.
Conclusion
We conclude that ambient noise alters the landscape of receiver-mediated selection on signals in Cope’s gray treefrog, and likely in other species that communicate in noisy social environments. We suggest that when receiver behavior is measured in quiet, simplified laboratory conditions, we are likely to overestimate the action of sexual selection in natural environments due to artificially high signal discriminability. Our data did not support the hypothesis that inconsistent production of a species recognition signal (pulse rate) acts, like ambient noise, to limit the expression of female preferences. This finding is in contrast to a similar assay performed in the same species manipulating call rate, in which female preferences were even more profoundly impacted by inconsistent signaling than by ambient noise. Thus, we note that the sources of noise that prevent expression of female preferences are variable across contexts and systems, and even between individual components of multicomponent signals (Tanner and Bee, 2020). A complete understanding of how communication systems evolve will require close examination of noise sources and their effects on receiver behavior in realistically complex environments.
Data Availability Statement
The original contributions presented in the study are publicly available. The data can be found here: 10.13020/9bem-wj63.
Ethics Statement
All procedures described herein were carried out in accordance with the principles of the Basel Declaration and the Animal Behavior Society/Association for the Study of Animal Behaviour guidelines for the ethical treatment of animals. Our procedures met all legal requirements of the United States of America and were approved by the University of Minnesota Institutional Animal Care and Use Committee under protocol 1701-34456A.
Author Contributions
JT collected, curated, and analyzed the data and wrote the first draft of the manuscript. Both authors designed the experiment, secured funding, contributed to the analysis and interpretation of the data, and edited the manuscript.
Funding
JT was supported by a Ford Foundation Pre-Doctoral Fellowship, the National Science Foundation Graduate Research Fellowship under Grant No. 00039202, and an National Science Foundation Postdoctoral Research Fellowship in Biology (1811930). This work was funded by an Animal Behavior Society Student Research Grant and a Joyce Davenport Fellowship in Natural History through the Bell Museum of Natural History to JT, and National Science Foundation grant IOS-1452831 to MB.
Conflict of Interest
The authors declare that the research was conducted in the absence of any commercial or financial relationships that could be construed as a potential conflict of interest.
Acknowledgments
We thank J. Dewey, M. Elson, A. Hartman, and other members of the Bee lab 2015–2017 for help collecting treefrogs and conducting behavioral trials. We thank H. Brumm, H. C. Gerhardt, G. Klump, and J. Schul for helpful discussions about this work. The Three Rivers Park District, Minnesota State Parks, Ramsey County, and the Minnesota Department of Natural Resources granted access to field sites.
Supplementary Material
The Supplementary Material for this article can be found online at: https://www.frontiersin.org/articles/10.3389/fevo.2020.00256/full#supplementary-material
References
Amorim, M. C. P., and Vasconcelos, R. O. (2008). Variability in the mating calls of the Lusitanian toadfish Halobatrachus didactylus: cues for potential individual recognition. J. Fish Biol. 73, 1267–1283. doi: 10.1111/j.1095-8649.2008.01974.x
Ballentine, B. (2009). The ability to perform physically challenging songs predicts age and size in male swamp sparrows, Melospiza georgiana. Anim. Behav. 77, 973–978. doi: 10.1016/j.anbehav.2008.12.027
Beckers, O. M., and Wagner, W. E. (2018). Males and females evolve riskier traits in populations with eavesdropping parasitoids. Behav. Ecol. Sociobiol. 72, 174.
Bee, M. A. (2007). Sound source segregation in grey treefrogs: spatial release from masking by the sound of a chorus. Anim. Behav. 74, 549–558. doi: 10.1016/j.anbehav.2006.12.012
Bee, M. A. (2008a). Finding a mate at a cocktail party: spatial release from masking improves acoustic mate recognition in grey treefrogs. Anim. Behav. 75, 1781–1791. doi: 10.1016/j.anbehav.2007.10.032
Bee, M. A. (2008b). Parallel female preferences for call duration in a diploid ancestor of an allotetraploid treefrog. Anim. Behav. 76, 845–853. doi: 10.1016/j.anbehav.2008.01.029
Bee, M. A., and Schwartz, J. J. (2009). Behavioral measures of signal recognition thresholds in frogs in the presence and absence of chorus-shaped noise. J. Acoust. Soc. Am. 126, 2788–2801. doi: 10.1121/1.3224707
Bee, M. A., Vélez, A., and Forester, J. D. (2012). Sound level discrimination by gray treefrogs in the presence and absence of chorus-shaped noise. J. Acoust. Soc. Am. 131:4188. doi: 10.1121/1.3699271
Bonachea, L. A., and Ryan, M. J. (2011). Localization error and search costs during mate choice in Túngara Frogs, Physalaemus pustulosus. Ethology 117, 56–62. doi: 10.1111/j.1439-0310.2010.01843.x
Bosch, J., Rand, A. S., and Ryan, M. J. (2000). Signal variation and call preferences for whine frequency in the túngara frog, Physalaemus pustulosus. Behav. Ecol. Sociobiol. 49, 62–66. doi: 10.1007/s002650000280
Brumm, H., and Slabbekoorn, H. (2005). Acoustic communication in noise. Adv. Study Behav. 35, 151–209.
Bush, S. L., Gerhardt, H. C., and Schul, J. (2002). Pattern recognition and call preferences in treefrogs (Anura: Hylidae): a quantitative analysis using a no-choice paradigm. Anim. Behav. 63, 7–14. doi: 10.1006/anbe.2001.1880
Byers, J., Hebets, E., and Podos, J. (2010). Female mate choice based upon male motor performance. Anim. Behav. 79, 771–778. doi: 10.1016/j.anbehav.2010.01.009
Caldwell, M. S., Lee, N., and Bee, M. A. (2016). Inherent directionality determines spatial release from masking at the tympanum in a vertebrate with internally coupled ears. J. Assoc. Res. Otolaryngol. 17, 259–270. doi: 10.1007/s10162-016-0568-6
Crews, D. (1975). Inter- and intraindividual variation in display patterns in the lizard, Anolis carolinensis. Herpetologica 31, 37–47.
Crowley, P. H., Travers, S. E., Linton, M. C., Cohn, S. L., Sih, A., and Sargent, R. C. (1991). Mate density, predation risk, and the seasonal sequence of mate choices: a dynamic game. Am. Nat. 137, 567–596. doi: 10.1086/285184
Dawson, B., and Ryan, M. J. (2009). Early experience leads to changes in the advertisement calls of male Physalaemus pustulosus. Copeia 2009, 221–226. doi: 10.1643/ce-07-254
Dingemanse, N. J., Bouwman, K. M., van de Pol, M., van Overveld, T., Patrick, S. C., Matthysen, E., et al. (2012). Variation in personality and behavioural plasticity across four populations of the great tit Parus major. J. Anim. Ecol. 81, 116–126. doi: 10.1111/j.1365-2656.2011.01877.x
Dingemanse, N. J., Kazem, A. J. N., Réale, D., and Wright, J. (2010). Behavioural reaction norms: animal personality meets individual plasticity. Trends Ecol. Evol. 25, 81–89. doi: 10.1016/j.tree.2009.07.013
Dingemanse, N. J., and Wolf, M. (2010). Recent models for adaptive personality differences: a review. Philos. Trans. R. Soc. 365, 3947–3958. doi: 10.1098/rstb.2010.0221
Dominoni, D. M., Halfwerk, W., Baird, E., Buxton, R. T., Fernández-Juricic, E., Fristrup, K. M., et al. (2020). Why conservation biology can benefit from sensory ecology. Nat. Ecol. Evol. 4, 502–511. doi: 10.1038/s41559-020-1135-4
Friedl, T. W. (2006). Individual male calling pattern and male mating success in the european treefrog (Hyla arborea): is there evidence for directional or stabilizing selection on male calling behaviour? Ethology 112, 116–126. doi: 10.1111/j.1439-0310.2005.01132.x
Gerhardt, H. C. (1975). Sound pressure levels and radiation patterns of the vocalizations of some North American frogs and toads. J. Comp. Physiol. 102, 1–12. doi: 10.1007/bf00657481
Gerhardt, H. C. (1978). Temperature coupling in the vocal communication system of the gray tree frog, Hyla versicolor. Science 199, 992–994. doi: 10.1126/science.199.4332.992
Gerhardt, H. C. (1991). Female mate choice in treefrogs: static and dynamic acoustic criteria. Anim. Behav. 42, 615–635. doi: 10.1016/s0003-3472(05)80245-3
Gerhardt, H. C. (2001). Acoustic communication in two groups of closely related treefrogs. Adv. Study Behav. 30, 99–166.
Gerhardt, H. C. (2008). Phonotactic selectivity in two cryptic species of gray treefrogs: effects of differences in pulse rate, carrier frequency and playback level. J. Exp. Biol. 211, 2609–2616. doi: 10.1242/jeb.019612
Gerhardt, H. C., and Doherty, J. A. (1988). Acoustic communication in the gray treefrog, Hyla versicolor: evolutionary and neurobiological implications. J. Comp. Physiol. A 162, 261–278. doi: 10.1007/bf00606090
Gerhardt, H. C., and Huber, F. (2002). Acoustic Communication in Insects and Anurans: Common Problems and Diverse Solutions. Chicago: The University of Chicago Press.
Gerhardt, H. C., Ptacek, M. B., Barnett, L., and Torke, K. G. (1994). Hybridization in the diploid-tetraploid treefrogs Hyla chrysoscelis and Hyla versicolor. Copeia 1994, 51–59.
Gerhardt, H. C., and Watson, G. F. (1995). Within-male variability in call properties and female preference in the grey treefrog. Anim. Behav. 50, 1187–1191. doi: 10.1016/0003-3472(95)80035-2
Girgenrath, M., and Marsh, R. L. (1997). In vivo performance of trunk muscles in tree frogs during calling. J. Exp. Biol. 200, 3101–3108.
Grafe, T. U. (1997). Costs and benefits of mate choice in the lek-breeding reed frog, Hyperolius marmoratus. Anim. Behav. 53, 1103–1117. doi: 10.1006/anbe.1996.0427
Hardin, J., and Hilbe, J. (2012). Generalized Estimating Equations, 2nd Edn. New York, NY: Chapman & Hall.
Kirkpatrick, M., and Ryan, M. J. (1991). The evolution of mating preferences and the paradox of the lek. Nature 350, 33–38. doi: 10.1038/350033a0
Lee, N., Ward, J. L., Vélez, A., Micheyl, C., and Bee, M. A. (2017). Frogs exploit statistical regularities in noisy acoustic scenes to solve cocktail-party-like problems. Curr. Biol. 27, 743–750. doi: 10.1016/j.cub.2017.01.031
Lengagne, T., Voituron, Y., and Gomez, D. (2016). Male within-individual variability in a sexual signal component and its impact on female choice. Behav. Ecol. 28, 108–116. doi: 10.1093/beheco/arw120
Magnhagen, C. (1991). Predation risk as a cost of reproduction. Trends Ecol. Evol. 6, 183–186. doi: 10.1016/0169-5347(91)90210-o
Martin, C. M., and Wagner, W. E. (2010). Female field crickets incur increased parasitism risk when near preferred song. PLoS One 5:e9592. doi: 10.1371/journal.pone.0009592
Martínez-Rivera, C. C., and Gerhardt, H. C. (2008). Advertisement-call modification, male competition, and female preference in the bird-voiced treefrog Hyla avivoca. Behav. Ecol. Sociobiol. 63, 195–208. doi: 10.1007/s00265-008-0650-0
McLister, D., Stevens, E. D., and Bogart, J. P. (1995). Comparative contractile dynamics of calling and locomotor muscles in three hylid frogs. J. Exp. Biol. 198, 1527–1538.
Mitani, J. C., and Brandt, K. L. (1994). Social factors influence the acoustic variability in the long-distance calls of male chimpanzees. Ethology 96, 233–252. doi: 10.1111/j.1439-0310.1994.tb01012.x
Møller, A. P., and Pomiankowski, A. (1993). Why have birds got multiple sexual ornaments? Behav. Ecol. Sociobiol. 32, 167–176.
Murphy, C. G. (2012). Simultaneous mate-sampling by female barking treefrogs (Hyla gratiosa). Behav. Ecol. 23, 1162–1169. doi: 10.1093/beheco/ars093
Murphy, C. G., and Gerhardt, C. H. (1996). Evaluating the design of mate-choice experiments: the effect of amplexus on mate choice by female barking treefrogs, Hyla gratiosa. Anim. Behav. 51, 881–890. doi: 10.1006/anbe.1996.0092
Murphy, C. G., and Gerhardt, H. C. (2002). Mate sampling by female barking treefrogs (Hyla gratiosa). Behav. Ecol. 13, 472–480. doi: 10.1093/beheco/13.4.472
Nehring, V., Wyatt, T. D., and d’Ettorre, P. (2013). “Noise in chemical communication,” in Animal Communication and Noise, ed. H. Brumm (Berlin: Springer), 373–405. doi: 10.1007/978-3-642-41494-7_13
Nityananda, V., and Bee, M. A. (2012). Spatial release from masking in a free-field source identification task by gray treefrogs. Hear. Res. 285, 86–97. doi: 10.1016/j.heares.2012.01.003
Nussey, D. H., Wilson, A. J., and Brommer, J. E. (2007). The evolutionary ecology of individual phenotypic plasticity in wild populations. J. Evol. Biol. 20, 831–844. doi: 10.1111/j.1420-9101.2007.01300.x
Pfennig, K. S. (2007). Facultative mate choice drives adaptive hybridization. Science 318, 965–967. doi: 10.1126/science.1146035
Pomiankowski, A., and Møller, A. P. (1995). A resolution of the lek paradox. Proc. R. Soc. Lond. B 260, 21–29. doi: 10.1098/rspb.1995.0054
Ptacek, M. B., Gerhardt, H. C., and Sage, R. D. (1994). Speciation by polyploidy in treefrogs: multiple origins of the Tetraploid, Hyla versicolor. Evolution 48, 898–908. doi: 10.1111/j.1558-5646.1994.tb01370.x
Reichert, M. S., and Ronacher, B. (2015). Noise affects the shape of female preference functions for acoustic signals. Evolution 69, 381–394. doi: 10.1111/evo.12592
Ritke, M. E., Babb, J. G., and Ritke, M. K. (1990). Life history of the gray treefrog (Hyla chrysoscelis) in Western Tennessee. J. Herpetol. 24, 135–141.
Rodríguez, R. L., Rebar, D., and Fowler-Finn, K. D. (2013). The evolution and evolutionary consequences of social plasticity in mate preferences. Anim. Behav. 85, 1041–1047. doi: 10.1016/j.anbehav.2013.01.006
Römer, H. (2013). “Masking by noise in acoustic insects: problems and solutions,” in Animal Communication and Noise, ed. H. Brumm (Berlin: Springer-Verlag), 33–63. doi: 10.1007/978-3-642-41494-7_3
Schul, J., and Bush, S. L. (2002). Non-parallel coevolution of sender and receiver in the acoustic communication system of treefrogs. Proc. R. Soc. B 269, 1847–1852. doi: 10.1098/rspb.2002.2092
Schwartz, J. J., Huth, K., and Hutchin, T. (2004). How long do females really listen? Assessment time for female mate choice in the grey treefrog, Hyla versicolor. Anim. Behav. 68, 533–540. doi: 10.1016/j.anbehav.2003.09.016
Servedio, M. R., and Noor, M. A. F. (2003). The role of reinforcement in speciation: theory and data. Annu. Rev. Ecol. Evol. Syst. 34, 339–364. doi: 10.1146/annurev.ecolsys.34.011802.132412
Shaw, K. L., and Herlihy, D. P. (2000). Acoustic preference functions and song variability in the hawaiian cricket Laupala cerasina. Proc. R. Soc. Lond. 267, 577–584. doi: 10.1098/rspb.2000.1040
Slabbekoorn, H., Dooling, R. J., Popper, A. N., and Fay, R. R. (eds) (2018). Effects of Anthropogenic Noise on Animals. New York, NY: Springer Science+Business Media.
Stamps, J. A., Briffa, M., and Biro, P. A. (2012). Unpredictable animals: individual differences in intraindividual variability (IIV). Anim. Behav. 83, 1325–1334. doi: 10.1016/j.anbehav.2012.02.017
Sullivan, B. K., and Hinshaw, S. H. (1992). Female choice and selection on male calling behaviour in the grey treefrog Hyla versicolor. Anim. Behav. 44, 733–744. doi: 10.1016/s0003-3472(05)80299-4
Taigen, T. L., and Wells, K. D. (1985). Energetics of vocalization by an anuran amphibian (Hyla versicolor). J. Comp. Physiol. B 155, 163–170. doi: 10.1007/bf00685209
Tanner, J. C., and Bee, M. A. (2019). Within-individual variation in sexual displays: signal or noise? Behav. Ecol. 30, 80–91. doi: 10.1093/beheco/ary165
Tanner, J. C., and Bee, M. A. (2020). Inconsistent sexual signaling degrades optimal mating decisions in animals. Sci. Adv. 6:eaax3957. doi: 10.1126/sciadv.aax3957
Tanner, J. C., Ward, J. L., Shaw, R. G., and Bee, M. A. (2017). Multivariate phenotypic selection on a complex sexual signal. Evolution 71, 1742–1754. doi: 10.1111/evo.13264
Tucker, M. A., and Gerhardt, H. C. (2012). Parallel changes in mate-attracting calls and female preferences in autotriploid tree frogs. Proc. R. Soc. B 279, 1583–1587. doi: 10.1098/rspb.2011.1968
Vélez, A., and Bee, M. A. (2011). Dip listening and the cocktail party problem in grey treefrogs: signal recognition in temporally fluctuating noise. Anim. Behav. 82, 1319–1327. doi: 10.1016/j.anbehav.2011.09.015
Ward, J. L., Buerkle, N. P., and Bee, M. A. (2013a). Spatial release from masking improves sound pattern discrimination along a biologically relevant pulse-rate continuum in gray treefrogs. Hear. Res. 306, 63–75. doi: 10.1016/j.heares.2013.09.006
Ward, J. L., Love, E. K., Vélez, A., Buerkle, N. P., O’Bryan, L. R., and Bee, M. A. (2013b). Multitasking males and multiplicative females: dynamic signalling and receiver preferences in Cope’s grey treefrog. Anim. Behav. 86, 231–243. doi: 10.1016/j.anbehav.2013.05.016
Wells, K., and Taigen, T. (1986). The effect of social interactions on calling energetics in the gray treefrog (Hyla versicolor). Behav. Ecol. Sociobiol. 19, 9–18. doi: 10.1007/bf00303837
Wiley, R. H. (2006). Signal detection and animal communication. Adv. Study Behav. 36, 217–247. doi: 10.1016/s0065-3454(06)36005-6
Wiley, R. H. (2015). Noise Matters: The Evolution of Communication. Cambridge, MA: Harvard University Press.
Wollerman, L., and Wiley, R. H. (2002). Background noise from a natural chorus alters female discrimination of male calls in a Neotropical frog. Anim. Behav. 63, 15–22. doi: 10.1006/anbe.2001.1885
Keywords: acoustic communication, anuran, mate choice, noise, sexual selection, signaling, species recognition, within-individual variation
Citation: Tanner JC and Bee MA (2020) Species Recognition Is Constrained by Chorus Noise, but Not Inconsistency in Signal Production, in Cope’s Gray Treefrog (Hyla chrysoscelis). Front. Ecol. Evol. 8:256. doi: 10.3389/fevo.2020.00256
Received: 29 March 2020; Accepted: 14 July 2020;
Published: 31 July 2020.
Edited by:
Hope Klug, The University of Tennessee at Chattanooga, United StatesReviewed by:
Ximena E. Bernal, Purdue University, United StatesHamilton Farris, Louisiana State University, United States
Copyright © 2020 Tanner and Bee. This is an open-access article distributed under the terms of the Creative Commons Attribution License (CC BY). The use, distribution or reproduction in other forums is permitted, provided the original author(s) and the copyright owner(s) are credited and that the original publication in this journal is cited, in accordance with accepted academic practice. No use, distribution or reproduction is permitted which does not comply with these terms.
*Correspondence: Jessie C. Tanner, amVzc2llLmMudGFubmVyQGdtYWlsLmNvbQ==
†Present address: Jessie C. Tanner, Centre for Evolutionary Biology, School of Biological Sciences, The University of Western Australia, Crawley, WA, Australia