- 1Royal Botanic Garden Edinburgh, Edinburgh, United Kingdom
- 2School of Biological Sciences, Institute of Molecular Plant Science, University of Edinburgh, Edinburgh, United Kingdom
- 3Real Jardín Botánico – Consejo Superior de Investigaciones Cientificas (CSIC), Madrid, Spain
Croton is a mega-diverse genus of more than 1,200 species with great morphological diversity and highly dimorphic flowers. Staminate flowers generally possess petals and a variable number of stamens and lack of an ovary. Pistillate flowers generally lack petals or have filamentous structures instead; stamens are lacking, and the ovary is generally tricarpellate with divided styles. However, well-developed petals can be found in pistillate flowers of some African species and two New World sections, i.e., sect. Alabamenses and sect. Eluteria subsect. Eluteria. Our objectives are to compare ontogeny in dimorphic flowers of Croton which may elucidate the origin of petals, homology of the filamentous structures, nectaries, and diversity of the androecium. The development of staminate and pistillate flowers of C. alabamensis (sect. Alabamenses) and C. schiedeanus (sect. Eluteria) was studied under the scanning electron microscope (SEM) and compared with C. chilensis (sect. Adenophylli) which has filamentous structures in pistillate flowers. In staminate flowers, petals develop in alternation with sepals and later the outermost stamen whorl develops opposite to the petals. In a much later stage, nectar glands emerge in alternation with the petals. In pistillate flowers, filamentous structures and petals share the same early development in alternation with the sepals. However, growth of the filamentous structures of C. chilensis becomes arrested, while petals of C. alabamensis and C. schiedeanus develop similar to those of the staminate flowers. The main conclusions of the study are: (1) Petals and filamentous structures are homologous based on their location and shape in early development. (2) Nectaries with variable morphology develop in antesepalous position and probably represent receptacular outgrowths and are not staminodial. (3) Staminate flower development displays an unexpected diversity, including a floral cup in C. alabamensis. All investigated species have an unusual centrifugal initiation of the second stamen whorl. (4) The possible evolutionary loss and potential regain of petals among different species of Croton is discussed in relation to heterochrony. We suggest that the filamentous structures in pistillate flowers represent pedomorphic forms of petals of staminate flowers, and that the well-developed petals in C. alabamensis and C. schiedeanus are derived via a developmental reversion.
Introduction
In flowers, “petal” is a term generally applied to the showy pigmented inner perianth whorl, in contrast to the term “sepal” which represents the protective green outermost perianth whorl. The presence of pigments, delicate texture, single vascular bundle and narrow base are common features found in petals (discussions in Endress, 1994; Ronse De Craene, 2007, 2008). However, it is not always easy to classify the perianth as sepals and petals especially in cases where there is only one perianth whorl present, since this may be a result of reduction of either whorl correlated with a functional change (Ronse De Craene, 2010). Petals play a major role in pollinator attraction via visual cues but sometimes they also facilitate scent dispersal, provide nectar, and function as landing platforms for insects (Endress, 1994; Whitney et al., 2011; Ojeda et al., 2016). The presence of both sepals and petals (biseriate perianth) in flowers is the most common pattern in angiosperms, at least in core eudicots (Ronse De Craene and Brockington, 2013). Evolutionary development genetic studies in the model plants Arabidopsis and Antirrhinum found that interactions between different gene classes give rise to organ determination in each whorl of the flower (ABCDE model) (Soltis et al., 2009; Litt and Kramer, 2010; Rijpkema et al., 2010). Co-expression of A- and B-class genes is found to determine petal identity in core eudicots (Pentapetalae) (Litt and Kramer, 2010; Rijpkema et al., 2010). However, Ronse De Craene (2007) argued that homology of petals should not be deduced based on gene expression pattern alone, but should include historical, phylogenetic and ontological perspectives. There is evidence that “petals” from different groups of angiosperms may have different origins. A continuous transition in the perianth from bracts to petal-like perianth parts is observed in the ANA grade, many magnoliids (Magnoliales, Laurales) and sometimes in core eudicots (e.g., Cactaceae, Berberidopsis, Paeonia, and Theaceae), while in some magnoliids and monocots, petals may be derived by pigmenting of the inner tepals (Endress, 2008; Ronse De Craene, 2008; Remizowa et al., 2010). Petals derived from transformation of sterile stamens (staminodes) is also reported in Ranunculales (Kosuge, 1994; Erbar et al., 1998). In the core eudicots, the largest group of angiosperms, the presence of a perianth with clear distinction between sepals and petals is prominent (Ronse De Craene, 2008, 2010; Endress, 2010; Soltis et al., 2018). Two possible origins for petals in core eudicots have been proposed, i.e., andropetals (staminodial origin) or bracteopetals (bracteolar origin) (e.g., Takhtajan, 1991). Andropetals generally have a delayed development and small primordia, contrary to bracteopetals which generally have an early development and broad primordia (Ronse De Craene, 2007, 2008). However, Ronse De Craene (2007) suggested that in core-eudicots andropetals are rare, and petals are mostly derived from bracteopetals.
The family Euphorbiaceae belongs to the order Malpighiales, the largest order of the rosid clade in Pentapetalae (The Angiosperm Phylogeny Group, 2016). The family has great diversity in floral morphology and is well-known for the sophisticated inflorescence-flower pseudanthial complex called cyathium, which is found in the genus Euphorbia (Wurdack et al., 2005; Prenner and Rudall, 2007; Horn et al., 2012). However, little is known about the morphology of flowers in the whole family. The presence of petals is generally an uncommon character in the Euphorbiaceae with a scattered distribution in distantly related internal groups (Wurdack et al., 2005). The inaperturate crotonoid clade (subfamily Crotonoideae), comprising about 60 genera, is one group in Euphorbiaceae where the presence of petals – at least in staminate flowers – is a key character in defining its affinity (together with inaperturate pollen grains) (Figure 2A; Wurdack et al., 2005; Webster, 2014). The putative sister group of this clade is the articulated crotonoid clade, comprising the economically important rubber tree (Hevea) and cassava (Manihot), which does not have petals but has petaloid tepals instead (Figure 2A; Wurdack et al., 2005). So far, no developmental study has been conducted to investigate the origin of petals and their evolution in the inaperturate crotonoid clade, whether they represent the basic condition of bracteopetals or are secondarily derived andropetals.
Croton is a mega-diverse genus of more than 1,200 recognized species with a broad tropical to sub-tropical worldwide distribution in various habitats (Webster, 1993; Govaerts et al., 2000; Berry et al., 2005; van Ee et al., 2011, 2015). Its large size, high morphological variation and wide distribution create great difficulties for taxonomic studies (van Ee et al., 2011). At present, four subgenera of Croton, i.e., subg. Quadrilobi (Müll. Arg.) Pax in Engl. & Prantl, subg. Adenophylli (Griseb.) Riina, subg. Croton L. and subg. Geiseleria A. Gray., are recognized based on the molecular phylogeny (Figure 2B; van Ee et al., 2011, 2015). Croton species from African, Asian and Australian lineages belong to the subgenus Croton, while other neotropical species belong to the other three subgenera (Berry et al., 2005; van Ee et al., 2011, 2015; Haber et al., 2017). Flowers of Croton are unisexual with both genders usually borne on the same indeterminate racemose-thyrsoid inflorescence with pistillate flowers on the proximal part and staminate flowers on the distal one (Figure 1; Webster, 1993; Berry et al., 2005; van Ee et al., 2011). Staminate flowers possess a biseriate perianth with well-developed petals and variable numbers of stamens but lack a pistillode (Webster, 1993, 2014; Radcliffe-Smith, 2001; van Ee et al., 2011). In pistillate flowers petals are usually lacking as well as stamens, or filamentous structures are present in the same position as the petals. The ovary is commonly tricarpellate with a single descending ovule per carpel, and topped with three styles with various branching patterns ranging from bifid to multifid (Webster, 1993, 2014; Radcliffe-Smith, 2001; van Ee et al., 2011). Filamentous structures are not just present in Croton but also in other genera of the inaperturate crotonoid clade (Nair and Abraham, 1962; Venkata-Rao and Ramalakshmi, 1968). The origin of the filamentous structures of pistillate flowers is controversial since they were interpreted as reduced petals (Nair and Abraham, 1962; Webster, 1993; Radcliffe-Smith, 2001; Caruzo and Cordeiro, 2007; De-Paula et al., 2011) or staminodes (Gagliardi et al., 2017). However, well-developed petals instead of filamentous structures were reported in pistillate flowers of two New World sections, i.e., sect. Alabamenses (Figure 1A) and sect. Eluteria subsect. Eluteria (Figure 1B; van Ee et al., 2011), and some African species (Figure 1C; Friis and Gilbert, 2008; Berry et al., 2016). Our study aims to clarify how these petals develop compared with the filamentous structures from typical pistillate flowers and petals from staminate flowers.
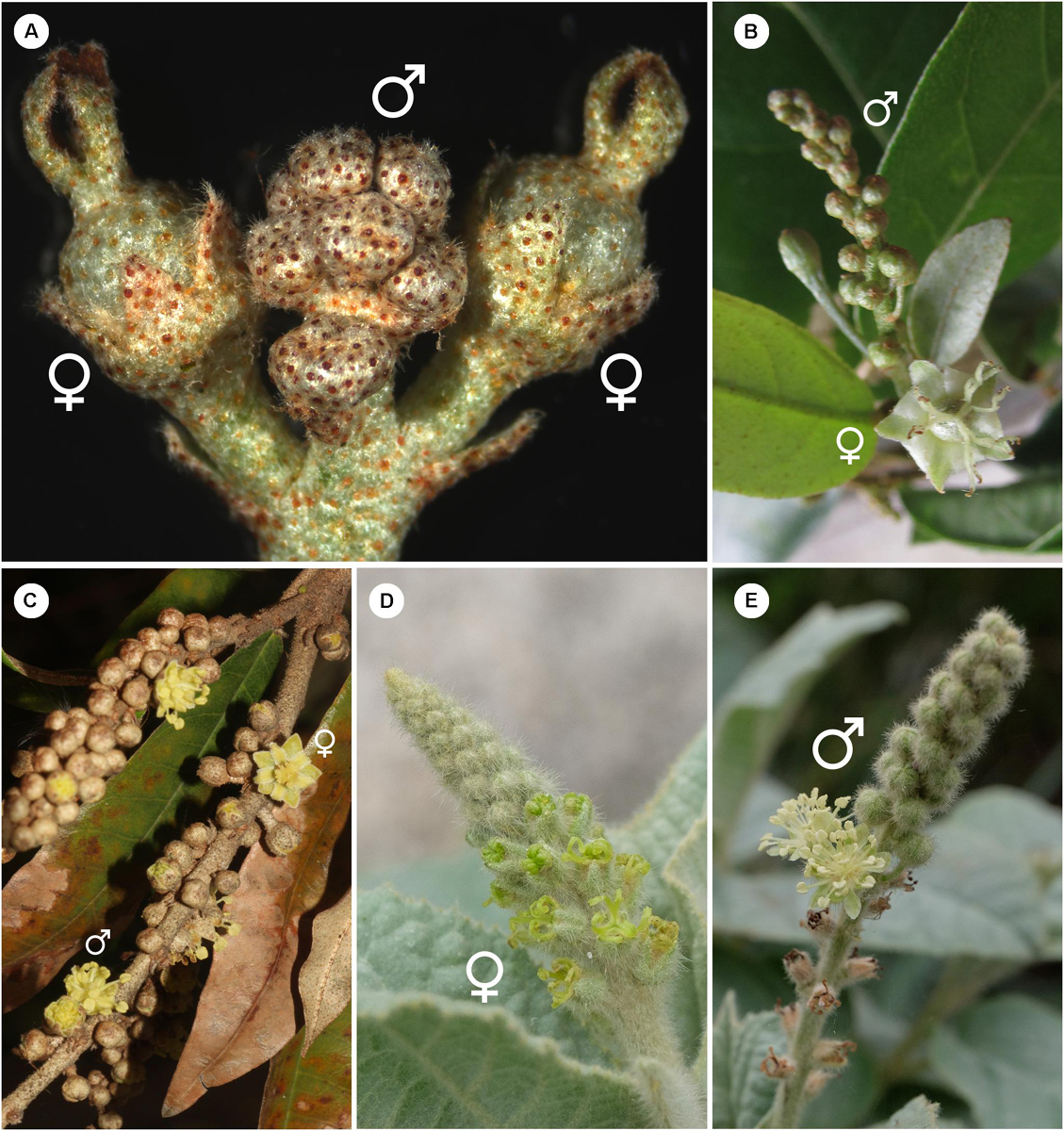
Figure 1. Inflorescence of Croton with/without petals in pistillate flower (A) Inflorescence of C. alabamensis showing blooming pistillate flowers while staminate flowers are still closed. (B) Inflorescence of C. schiedeanus with long-pedicellate pistillate flower on the proximal part and many staminate flowers on the distal part. (C) Inflorescence of C. gratissimus, an African species with petals in pistillate flowers. (D) Inflorescence of C. chilensis showing pistillate stage. (E) Inflorescence of C. chilensis in the staminate stage (two open staminate flowers).
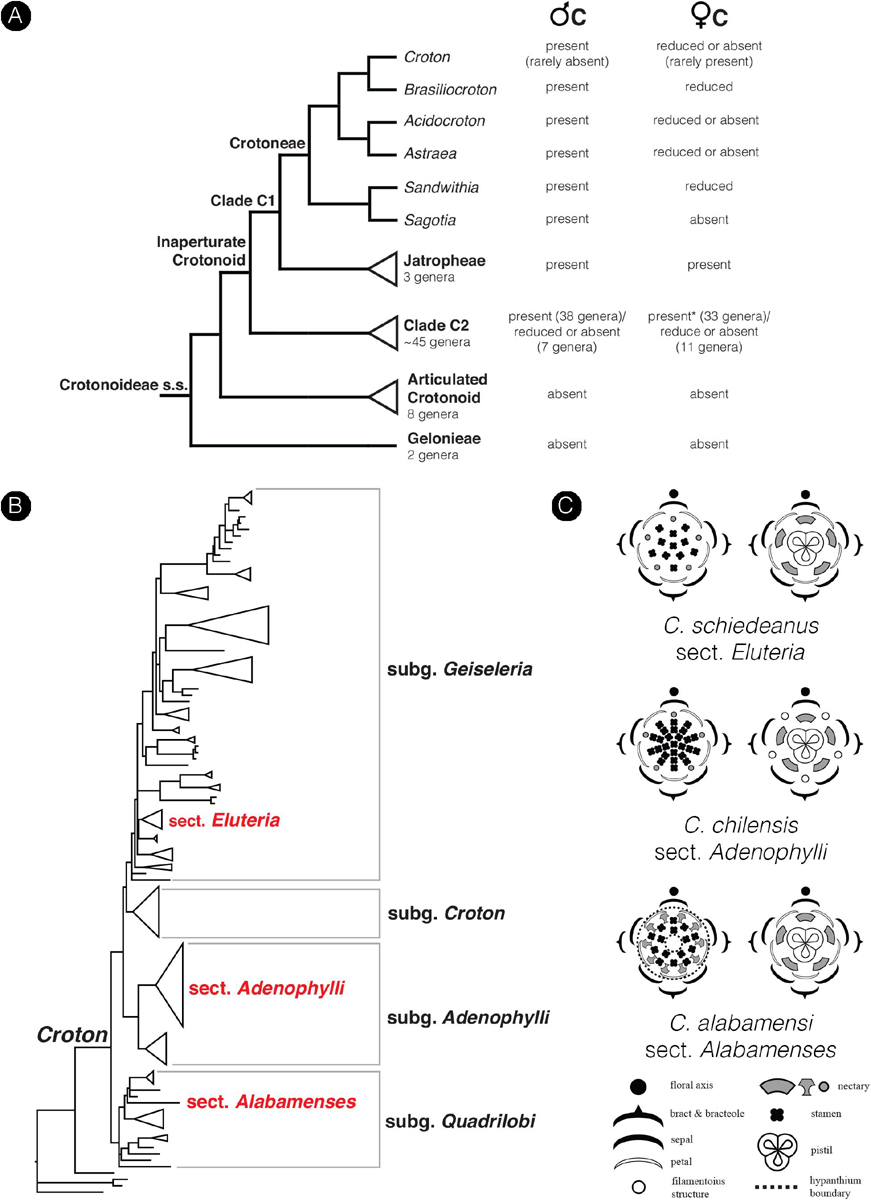
Figure 2. Diagrams show the phylogenetic relationship in the subfamily Crotonoideae and the genus Croton mapped with a number of floral characters. (A) Simplified phylogenetic cladogram of the subfamily Crotonoideae shows relationships of all subgroups with the relationship of genera in the tribe Crotoneae emphasized (modified from Wurdack et al., 2005; van Welzen et al., 2020). The occurrence of petals in staminate and pistillate flowers were mapped on the tree with data from personal observation and the literature (e.g., Radcliffe-Smith, 2001; Webster, 2014). (Astersisk) There are no data about petals in pistillate flowers of the genus Tapoïdes. (B) Simplified phylogenetic phylogram of Croton indicating four subgenera. Sections which have representative species included in the present study are labeled (modified from van Ee et al., 2011). (C) Floral diagrams of both staminate and pistillate flowers from the three species of Croton used in this study. Floral diagrams were drawn following the symbols presented in Ronse De Craene (2010).
Another important aspect of flower diversity in Croton is the presence and homology of nectary tissue. Nectaries are present in both staminate and pistillate flowers of Croton in antesepalous position (alternate with petals in staminate flowers or alternate with filamentous structures in pistillate flowers) (Baillon, 1858; Michaelis, 1924; Nair and Abraham, 1962; Venkata-Rao and Ramalakshmi, 1968; De-Paula et al., 2011; Gagliardi et al., 2017). Nectaries could be vascularized or not in Croton (Nair and Abraham, 1962; Venkata-Rao and Ramalakshmi, 1968; Freitas et al., 2001; De-Paula et al., 2011) and if vascularized, the source of vascular bundles comes from sepal traces or rarely from the floral stele (De-Paula et al., 2011). A floral nectary (often described as glands or disk) is commonly present in both staminate and pistillate flowers in the subfamily Crotonoideae (Radcliffe-Smith, 2001; Webster, 2014). Nectaries have been reported to arise very late in the development of flowers of Croton and other genera in the inaperturate crotonoid clade (Liu et al., 2008, 2015; De-Paula et al., 2011; Gagliardi et al., 2016; Mao et al., 2017). In Croton and Astraea, nectaries were interpreted as staminodes because of their antesepalous position (De-Paula et al., 2011), but they were interpreted as receptacular outgrowth as well (Caruzo and Cordeiro, 2007). However, little is known of the nectary development and diversity and our study can throw more light on the origin and homology of the nectaries in Croton.
Stamens of Croton are well-known for their reflexed filaments in buds (Webster, 1993, 2014; Radcliffe-Smith, 2001; Berry et al., 2005; van Ee et al., 2011). The number of stamen in flower is also found to be highly diverse, ranging from one to more than one hundred (van Ee et al., 2008, 2011; Riina et al., 2009; Webster, 2014). The androecium of many Croton also possess the presence of outermost antepetalous stamens which is an unusual character among angiosperms (Baillon, 1858; Marchand, 1860; Michaelis, 1924; Nair and Abraham, 1962; Venkata-Rao and Ramalakshmi, 1968; Gandhi and Thomas, 1983; De-Paula et al., 2011). The origin of this character was thought to be dictated by the presence of an alternipetalous nectary (Venkata-Rao and Ramalakshmi, 1968) or by reduction of a stamen whorl (Gandhi and Thomas, 1983). However, the observation of the late development of the nectary did not support either of these hypotheses (De-Paula et al., 2011; Gagliardi et al., 2017). Investigation of floral development in several species of Croton and Astraea by De-Paula et al. (2011) found the antesepalous stamen whorl to be the first to develop followed by the antepetalous whorl. However, their studies of transverse sections revealed that the outermost whorl is the antepetalous whorl, while the antesepalous whorl is the next inner whorl, reflected on their floral diagrams (Figure 8 in De-Paula et al., 2011), which is representative for a centrifugal stamen development. More evidence from floral development is required and will be conducted in the present study.
Although our sampling of species is highly limited for such a large genus, results of a comparative floral developmental study of three highly divergent species can address a number of relevant questions linked to the floral evolution of the genus, such as (1) the origin of petals, (2) homology between petals of staminate and pistillate flowers, (3) origin of filamentous structures in the pistillate flowers, (4) androecial diversity in staminate flowers, and (5) diversification among staminate and pistillate flowers with emphasis on the nectaries. A better understanding of petal origins and their evolution in Croton will help in understanding similar phenomena in other taxa of subfamily Crotonoideae and also in the family Euphorbiaceae.
Materials and Methods
Staminate and pistillate flowers of Croton alabamensis (sect. Alabamenses) (Figure 1A) and C. schiedeanus (sect. Eluteria, subsect. Eluteria) (Figures 1B, 2B), both of which have well-developed petals in pistillate flowers, were studied and compared with flowers of C. chilensis (sect. Adenophylli) (Figures 1D,E, 2B), which has filamentous structures in pistillate flowers. Samples were collected from cultivated plants grown at the Royal Botanic Garden Edinburgh and in the field (Table 1), fixed in FAA solution (90% ethanol at 70%, 5% glacial acetic acid and 5% formalin solution at 40%), then stored in 70% ethanol.
Inflorescence and floral morphology were observed using light microscopy (Zeiss Stemi 2000-C) and photos were captured with an AxioCam MRc 5 (Zeiss).
For the developmental and detailed morphology studies, flowers from different stages were dissected under a light microscope (Zeiss Stemi SV6), dehydrated in an alcohol gradient, dehydrated in acetone, dried at CO2 critical point in a K850 critical-point dryer (Quorum Technologies), further dissected, mounted on aluminum stubs, coated with platinum (Emitech K575X) and observed with a scanning electron microscope (SEM) (Leo Supra 55-VP). All pictures were later edited in Photoshop (Adobe Inc.). Floral and inflorescence diagrams were drawn with Illustrator (Adobe Inc.).
Results
Croton Chilensis
General Morphology
The inflorescence of Croton chilensis is generally a thyrse (an indeterminate inflorescence with lateral cymes) with the presence of solitary pistillate flowers or bisexual cymules (a pistillate flower associated with several staminate flowers) on the lower part and solitary staminate flowers or staminate cymules on the upper part (Figures 1D,E). Inflorescences are produced terminally on the stem. The pistillate flowers reach maturity before the staminate flowers (Figure 1D). Both staminate and pistillate flowers are subtended by a bract and two bracteoles. Staminate flowers are borne on a slender pedicel while pistillate flowers are nearly sessile (Figure 1E). Flowers from both genders are pentamerous (Figure 2C).
The perianth of staminate flowers is differentiated into sepals and petals, both with green color (Figures 2C, 3A). Aestivation of the five sepals is quincuncial (Figure 3B). Sepals are ovate in shape with a wide base and acute apex (Figures 3C,D). The abaxial surface of sepals is covered with stellate trichomes (Figure 3C) while the adaxial surface is glabrous (Figure 3D). Five petals are arranged in cochlear or quincuncial aestivation (Figures 3E,F). Petals are obovate in shape with a narrow base (no claw) and obtuse apex (Figures 3G,H). Both surfaces of petals are generally glabrous except for the lower area of the adaxial side which is covered with many simple trichomes (Figures 3G,H). The apex of the petals is covered with papillae (Figures 3G,H). Inside the corolla whorl, there are five bilobed nectary glands alternating with the petals (Figures 3I,J). In the middle of the flowers, there are about 20 (19–24) stamens present, arranged in four whorls on a convex receptacle (could be interpreted as a short androphore by some authors – e.g., De-Paula et al., 2011) (Figure 3A). Anthers are inflexed in bud (Figure 3K) before expanding at anthesis (Figures 3A,L). The outermost stamen whorl is opposite to the petals (Figure 3A). There are several simple trichomes at the base of each filament (Figure 3L). The receptacle is pilose. There is no evidence of a gynoecial structure in the staminate flowers (Figure 3A).
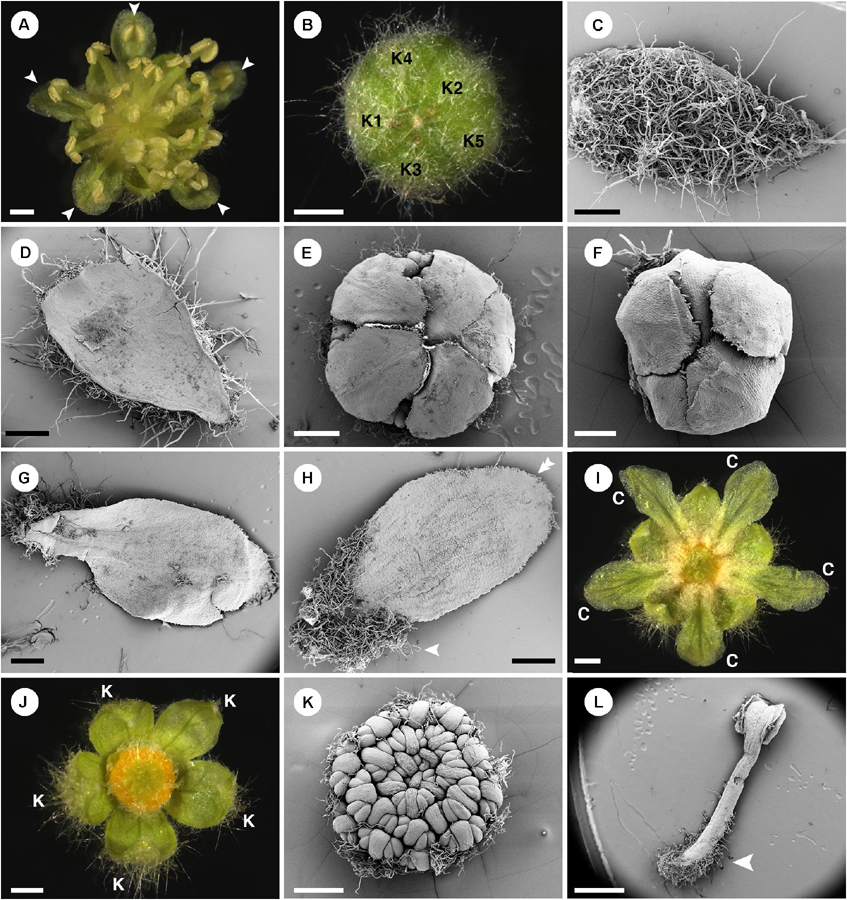
Figure 3. Morphology of staminate flowers of Croton chilensis. (A) Anthetic flower. Note, the outermost stamens are opposite petals (arrowhead). (B) A flower bud near anthesis with quincuncial aestivation. (C) Abaxial surface of a sepal covered with long stellate trichomes. (D) Adaxial surface of a sepal with glabrous surface. (E) Corolla with cochlear aestivation. (F) Corolla with quincuncial aestivation. (G) Abaxial surface of a petal with few stellate trichomes on the surface. (H) Adaxial surface of a petal with long simple trichomes covering the lower part (arrowhead). Note, there are papillae on the margin of the apex (double arrowhead). (I) A flower with stamens removed showing trichomes on the lower part of the petals covering nectary glands. (J) A flower with petals and stamens removed showing multiple yellow nectary lobes. (K) Flower bud with young androecium, perianth removed. Note, anthers are inflexed making them look like facing outward. (L) A stamen with lower part of filament covered with many simple trichomes (arrowhead). K, sepal; C, petal. Scale bars: (A,B,I,J,L) = 1,000 μm; (C–E,G,H,K) = 500 μm; (F) = 200 μm.
In pistillate flowers, the five sepals are present (Figures 2C, 4A). Aestivation of sepals is quincuncial (Figure 3B). Sepal shape is ovate with a wide base, which makes it look triangle-shaped (Figures 4B,C). The apex is acute (Figures 4B,C). Within the calyx and alternate to the sepals, there are generally filamentous structures (Figure 4D), occasionally replaced by petals (Figure 4A) or colleters (Figure 4E). Opposite to the sepals, there are five nectary glands present, alternate to the filamentous structures (Figures 4D–F). Petals, if present, have a narrow ligulate shape with acute apex (Figures 4A,F). Stamens are completely absent in the pistillate flowers. At the center of the flower, there is a gynoecium comprising three carpels (Figure 4G). The outer surface of the ovary is covered with dense stellate trichomes (Figures 4G,H). There are three styles with bifid stigmatic tip (total of six tips) (Figures 1D, 4A).
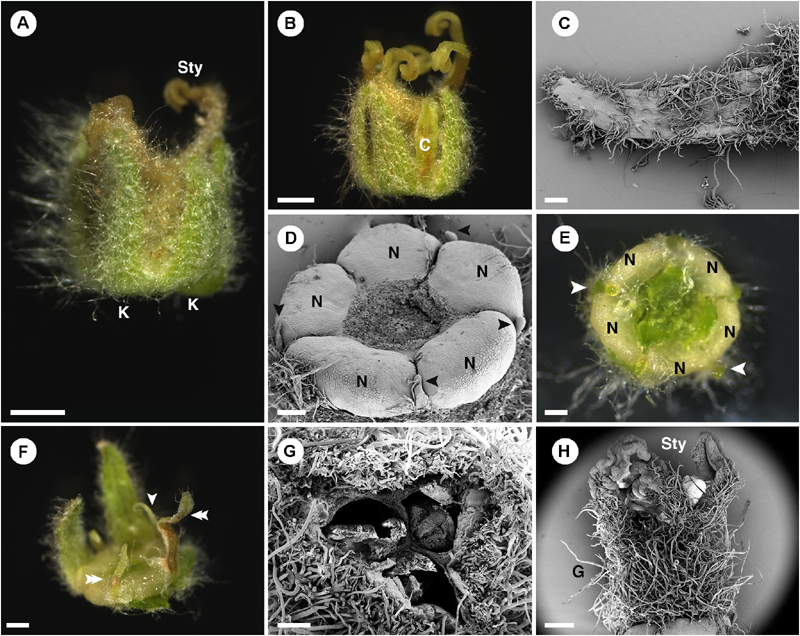
Figure 4. Morphology of pistillate flower of Croton chilensis. (A) A flower at anthesis. Note, sepals are conspicuous while petals are inconspicuous. Note, the abaxial surface of sepals is covered with long stellate trichomes. (B) An anthetic flower with a petal present. (C) Adaxial surface of a sepal covered with variably reduced stellate trichomes. (D) Five nectary glands with small filamentous structures arranged alternately on the outer part. Note, heteromorphy of filamentous structures in the same flower is present with some becoming colleters (arrowhead). (E) Filamentous structures and nectary glands at anthesis. Some filamentous structures become colleters (arrowhead). (F) Variable morphologies of organs alternating with nectaries. Some have a filamentous form (arrowhead), while some resemble petals (double arrowhead). (G) An ovary consisting of three carpels with axile placentation. (H) There are three styles on top of the ovary. The style and stigma have a papillate surface. K, sepal; Sty, style; C, petal; N, nectary; G, ovary. Scale bars: (A,B) = 1,000 μm; (C–E,G) = 200 μm; (F,H) = 500 μm.
Ontogeny of Staminate Flowers
Staminate floral primordia arise spirally on the inflorescence axis in the axil of a bract. A pair of bracteoles develops laterally of the floral primordium (Figures 5A,B). The first sepal arises abaxially (Figure 5C). The remaining sepals develop in a helical order (Figures 5C,D). The first sepal is large and almost covers the entire bud during the development (Figure 5C). Five petal primordia emerge simultaneously and alternate to the sepals (Figures 5D–I). Growth of petals tends to be more rapid on the abaxial side of the flower (Figure 5H). Following initiation of all five petals, the first whorl of stamens initiates alternating with the petals (Figure 5F). The second whorl of stamens develops next outside of the first whorl opposite the petals. However, in the early stage it is covered by the expanding petals (Figures 5G–I,K,N). The third and fourth stamen whorls later develop almost simultaneously and centripetally on the remaining floral meristem (Figures 5G–J). Arrangement and number of stamens are observed to be variable among flowers (Figures 5K,L). It is difficult to distinguish between the third and fourth whorls. A final set of three to five stamens develops at the apex of the floral meristem and sometimes the last stamen to initiate is shifted toward the center of the flower giving the false impression of a central stamen (Figures 5J,L–N). During development, stamens curve and fold inwards, giving Croton’s characteristic inflexed anther in bud (Figure 5O). Five nectary glands emerge much later in alternation with the petals.
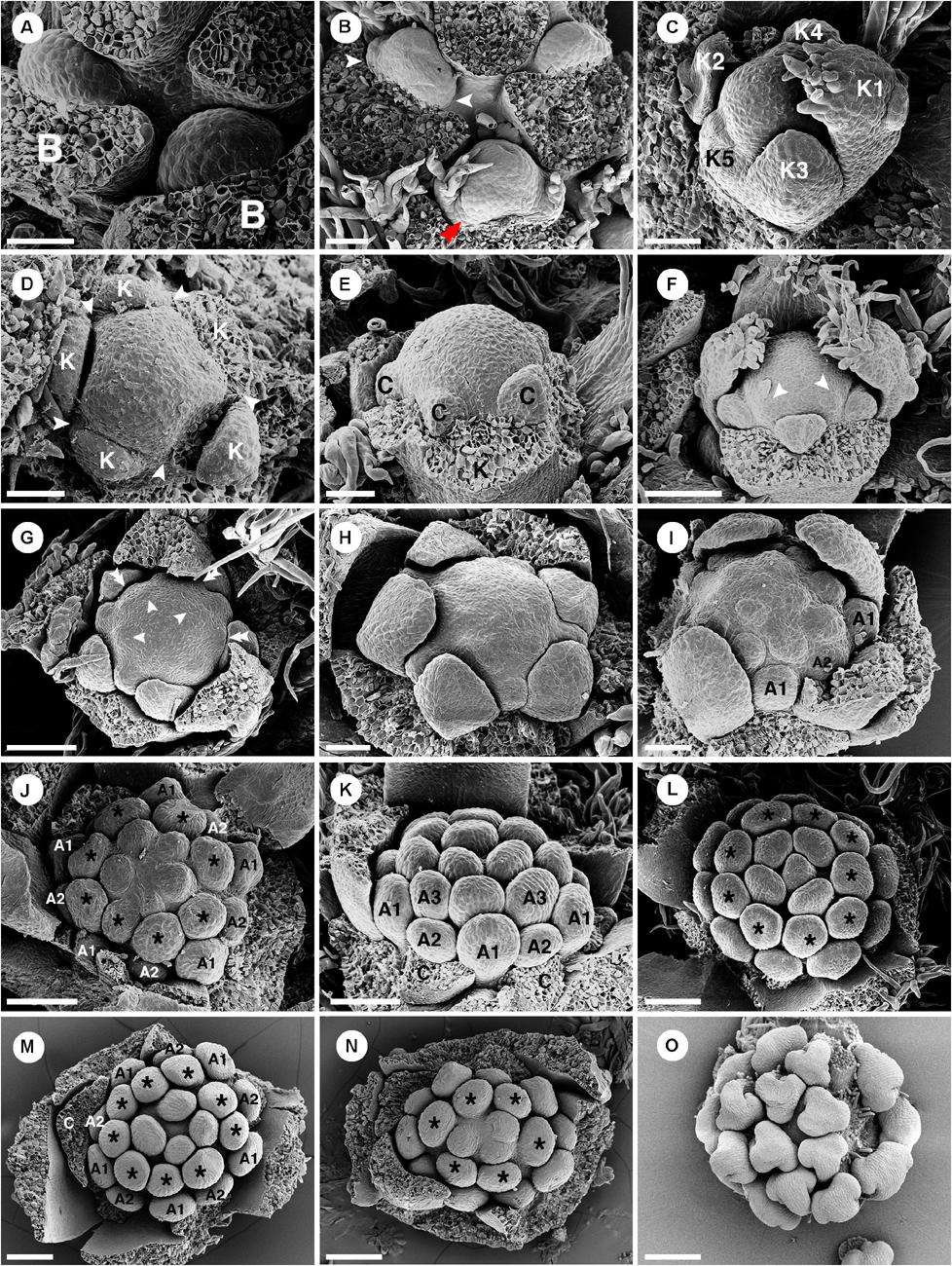
Figure 5. Ontogeny of staminate flowers of Croton chilensis. (A) Young floral primordia subtended by a bract. Note, the floral apex has a convex shape. (B) Sequence of early floral development with formation of bracteoles (arrowhead) and a first sepal (double arrowhead). (C) An older bud shows unidirectional unequal growth of sepals with the initiation of petal primordia. (D) Apical view of a very young flower bud with initiation of petal primordia (arrowhead). (E) In later stages, young petals grow and expand surrounding the central floral apex. (F) While petals are growing, the first whorl of stamens develops alternating with petals (arrowhead). (G) Next, the second whorl of stamens develops centrifugally opposite petals, while the third whorl develops centripetally alternate with the first stamen whorl. (H) Later, the fourth stamen whorl initiates. Only first, third and fourth whorls are visible. The second whorl is covered by the expanding petals which have unequal growth. (I) Next, more stamens develop but do not form a complete whorl. (J) Anomalous stamen number (eight) in the third and fourth whorls (asterisk). Five stamens are present in the central part forming a complete whorl. (K) The antepetalous second stamen whorl is pushed by the petals making the first stamen whorl become the outermost whorl. (L) Nine stamens are present in the third and fourth whorls (asterisk). Five central stamens are present but are unequally arranged. (M) The outermost stamens during a young developmental stage are stamens from the first whorl. The anomalous stamen number of nine is observed in the third and fourth whorls (asterisk). (N) A flower with an anomalous lower stamen number (total 19 stamens). Boundary between third and fourth whorls could not be observed (asterisk). (O) Later, young stamens curve toward the floral apex and become inflexed. B, bract; K, sepal; C, petal; A, stamen. Scale bars: (A–E,H,I) = 50 μm; (F,G,K,M,N) = 100 μm; (I,J) = 90 μm; (L) = 120 μm; (O) = 200 μm.
Ontogeny of Pistillate Flowers
Pistillate floral primordia emerge on the proximal to approximately middle part of the inflorescence where there is a transition to the staminate flower zone (Figures 1D,E). Each floral primordium is subtended by a bract and sided by two bracteoles. We could not observe the sepal initiation because of the presence of intertwining stellate trichomes obscuring the view. However, we could imply a 2/5 pattern as their arrangement follows a quincuncial aestivation in the mature flowers. Five petal-like primordia develop alternate to the sepals (Figures 6A–D). While initiation of primordia is initially similar to staminate flowers, growth of the organs tends to be slower. Different sizes of petal-like organs were observed, which may be the result of a non-simultaneous initiation or unequal development (Figures 6C,D). Three congenitally fused carpels arise rapidly, while petals are still in an early developmental stage (Figures 6A,B). As the gynoecium develops, petal development terminates (Figures 6A–H). Nectary tissue emerges late as a rim at the base of the gynoecium; the pressure of the sepal lobes gives the impression of a pair of nectaries developing between the petals (Figure 6G). The nectary expands as a multilobed ring around the ovary (Figures 6H,I). A bilobed style develops on top of each carpel (total of three styles/six stigmatic tips) (Figure 6H). In closed buds, some petal-like organs are elongated and develop as colleters (Figures 4D,E, 6J,K; P. Thaowetsuwan, unpublished anatomical data). Some petal-like organs develop into miniature petals with various shapes and sizes, resulting in a highly heterogeneous corolla morphology (Figures 4A,E,F, 6J–L).
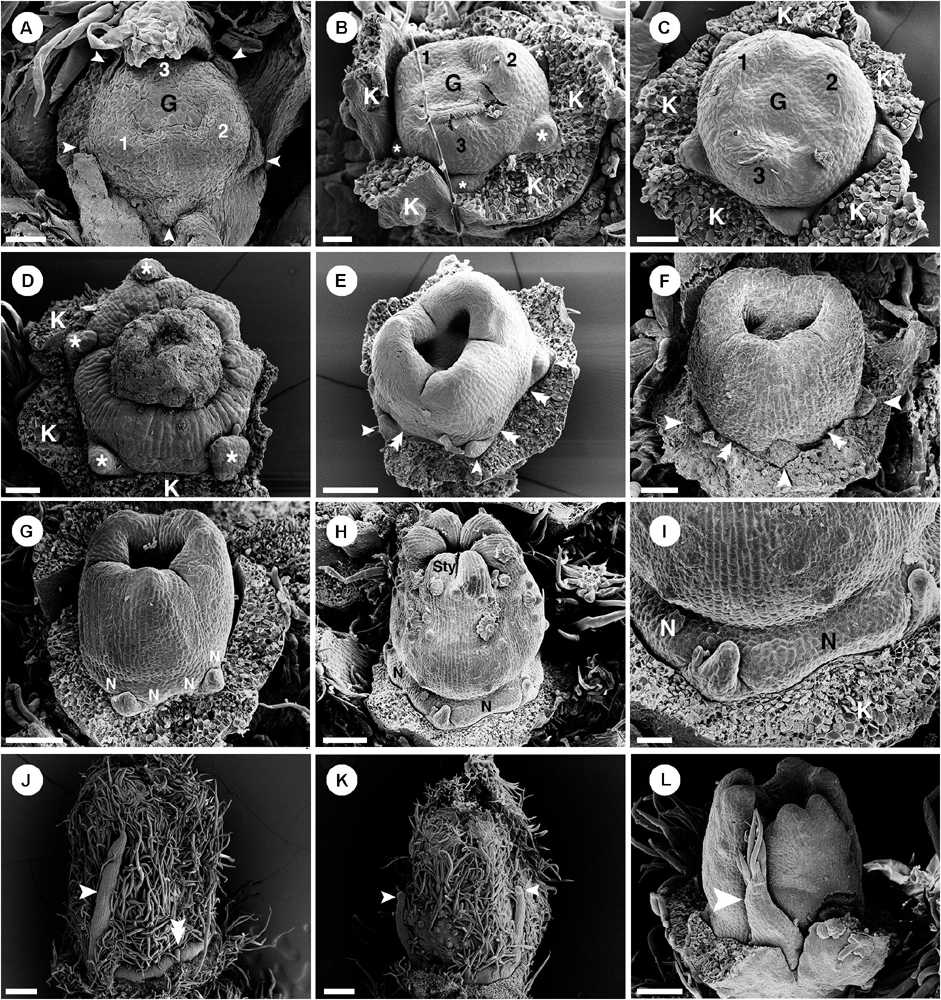
Figure 6. Ontogeny of pistillate flowers of Croton chilensis. (A) A young flower shows initiation of petal-like primordia (arrowhead) and formation of an ovary by fusion of three carpels. (B) Petal-like primordia (asterisk) are arranged alternating with sepals. (C) Petal-like organs show slight differences in growth. (D) Flower with aborted ovary. Petal-like organs clearly show unequal growth (asterisk). (E) Later, three carpels close apically while petal-like organs development is arrested (arrowhead). Nectary primordia start to initiate at the base of the ovary (double arrowhead). (F) Petal-like organs stop their development while the ovary grows and expands in size (arrowhead). Nectary tissue starts to form at the base of the ovary opposite to sepals (double arrowhead). (G) Later stage; a bilobed style is formed on top of each carpel. Nectary glands appear to be bilobed by pressure of the sepal lobes. (H) Next, a bifid style can be seen on top of each carpel. Nectariferous tissue expands to become a ring. (I) Magnified view of the previous picture shows the nectary ring expansion while petal-like organs are still minute. (J) Later, some petal-like organs grow into filamentous structures (arrowhead) while some are still arrested in their development (double arrowhead). (K) Some filamentous structures have a tendency to become a colleter (arrowhead). (L) A miniature petal is sometimes present by extension of the petal-like organs (arrowhead). G, ovary; K, sepal; N, nectary; Sty, style. Numbers on the gynoecium in (A–C) indicate carpel number. Scale bars: (A,B,I) = 40 μm; (C,D,F) = 50 μm; (E,G,L) = 100 μm; (H) = 120 μm; (J,K) = 200 μm.
Croton alabamensis
General Morphology
Flowers of Croton alabamensis are borne on racemose inflorescences with up to three pistillate flowers on the lower part and more numerous staminate flowers distally (Figure 1A). Inflorescences are produced terminally on shoots. The pistillate flowers mature much earlier than staminate flowers (Figure 1A). Both staminate and pistillate flowers are pedicellate, and subtended by a bract and two bracteoles (Figure 1A). Both staminate and pistillate flowers are pentamerous (Figure 2C).
Perianth of staminate flowers is biseriate (Figures 2C, 7A,B). Sepals have quincuncial aestivation (Figure 7A) and an ovate shape with wide base and acute apex (Figures 7B,C). The corolla also has quincuncial aestivation (Figure 7D). Petals have an ovate shape with narrow base and obtuse apex (Figures 7E,F). The abaxial side of sepals is covered with lepidote trichomes (Figures 7A,B). On the adaxial side, sepals bear stellate trichomes on their margins (Figure 7C) while petals bear simple trichomes on their margins (Figure 7F). Petals have stellate-lepidote trichomes covering their abaxial surface (Figures 7D,E). Margins and apex of petals are fimbriate comprising simple trichomes (Figures 7E,F). Inside the corolla whorl, ten nectary glands alternate with petals and outermost stamens, and are fused pairwise as five horse-shoe shaped nectaries clasping the base of the filaments (Figure 7G). There are 15–18 stamens present in the staminate flowers with the outermost whorl alternating with petals (Figure 7H). There are some stellate trichomes present on the basal part of the filaments (Figure 7I). On the apex of the floral meristem, there is an empty space without trace of carpels (Figures 2C, 7H). Stamens are incurved toward the center of the flower, but the anthers do not inflex (Figure 7H). The receptacle is pilose, covered with simple trichomes.
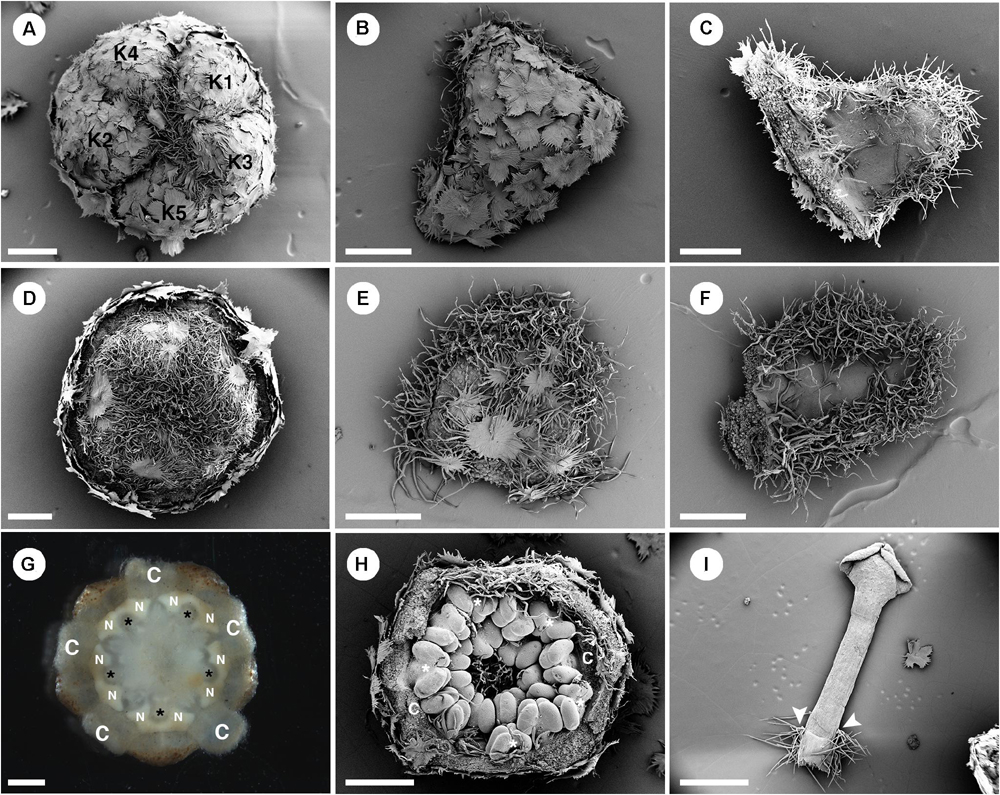
Figure 7. Morphology of staminate flowers from Croton alabamensis. (A) Calyx in the flower bud with quincuncial aestivation. Note, interlocking of trichomes on the floral apex. (B) Abaxial surface of a sepal covered with lepidote trichomes. (C) Adaxial surface of a sepal covered with some stellate trichomes on the lower part. There are many stellate trichomes on the margin. (D) Corolla in a flower bud with quincuncial aestivation. (E) Abaxial surface of a petal covered with stellate-lepidote trichomes. There are many trichomes on the margin. (F) Adaxial surface of a petal. The central area is glabrous but there are tufts of simple trichomes on the margin. (G) Horse-shoe shaped nectary glands clasping the base of outer antesepalous stamens (asterisk). (H) Stamens in the cup-shaped floral bud. The outermost stamens are arranged alternating with petals (asterisk). Note, stamens are not inflexed in bud. (H) A stamen with some stellate trichomes on the filament base (arrowhead). K, sepal; C, petal; N, nectary. Scale bars: (A–F,H) = 500 μm; (G,I) = 1,000 μm.
In pistillate flowers, there are two perianth whorls (Figures 2C, 8A,B). Sepal aestivation is quincuncial. Sepals have an ovate shape with a wide base and acute apex (Figures 8A–D). In sepals, the abaxial surface is covered by lepidote trichomes (Figures 8A–C), while the adaxial surface is glabrous on the lower part but covered with some stellate and simple trichomes on the upper part (Figure 8D). Petals of pistillate flowers are similar to those of staminate flowers but there is a mix of lepidote and stellate-lepidote trichomes present along the midrib on the abaxial side (Figure 8E). Petals have an oblong shape with narrow base and obtuse apex (Figures 8E,F). The petals are arranged in alternation with free but confluent nectary glands (Figure 8G). Inside the nectary, there is a tricarpellate ovary covered with lepidote trichomes (Figure 8A). On top of the ovary, three styles are present with simple style/stigma, but sometimes a slightly divided stigma is present (Figure 8H).
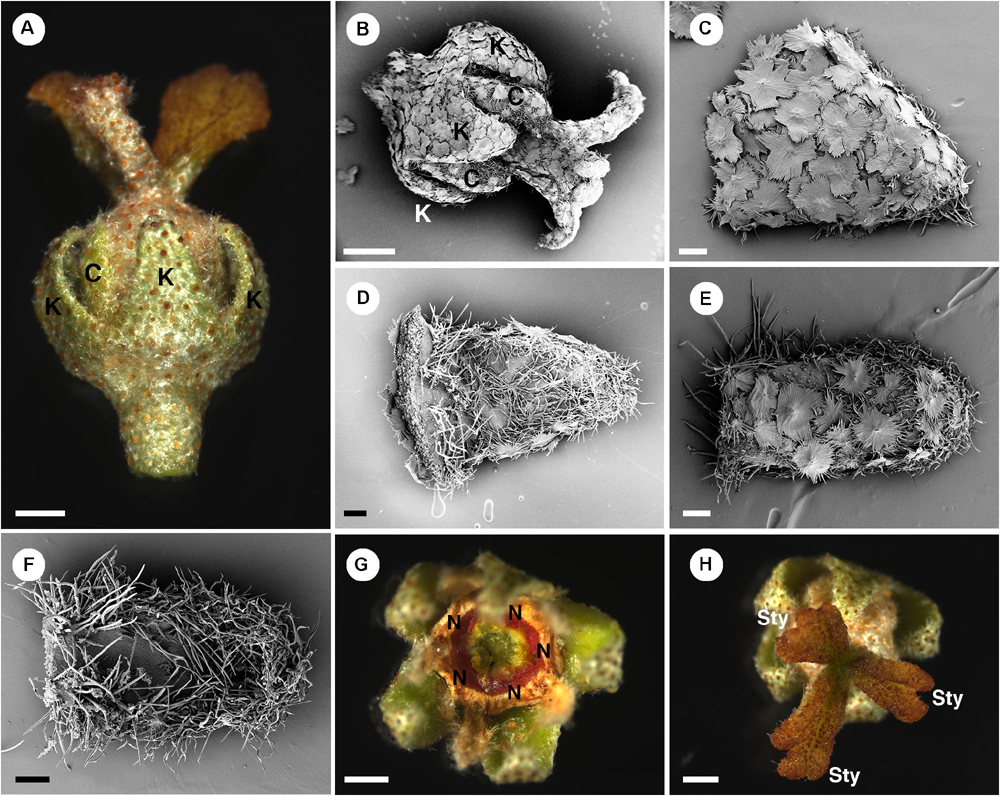
Figure 8. Morphology of pistillate flowers of Croton alabamensis. (A) A flower near anthesis. Fully developed petals are present. Styles are bilobed but do not fork. (B) A flower near anthesis with visible sepals and petals. Styles are slightly bifid in this flower. Note outer surface of all organs is covered with lepidote trichomes. (C) Abaxial side of a sepal covered with lepidote trichomes. (D) Adaxial side of a sepal. There are many stellate trichomes covering the surface. (E) Abaxial side of a petal covered with lepidote and stellate-lepidote trichomes. (F) Adaxial side of a petal. The margin is densely covered with simple trichomes. (G) A nectary ring inside the corolla initially with yellow color that later changes to red. (H) Three styles and stigmatic surfaces. Note, stigmatic lobe number vary from two to four. K, sepal; C, petal; N, nectary; Sty, style. Scale bars: (A,B,G,H) = 1,000 μm; (C–F) = 200 μm.
Ontogeny of Staminate Flowers
Only staminate flowers from C. alabamensis var. alabamensis were used in the developmental study. Staminate flowers develop spirally on the upper part of the racemose inflorescence (Figure 1A). Each floral primordium is subtended by a bract and two bracteoles (Figure 9A). The first sepal emerges on the abaxial side of the flower (Figures 9A,B), followed by additional sepals in a 2/5 pattern (Figure 9B). Floral primordia are initially convex shaped (Figures 9A,B) but later shift vertically to a concave shape due to hypanthium formation during petal initiation (Figure 9C). Five petal primordia emerge alternating with sepals (Figure 9C). Petals develop unidirectionally toward the abaxial side of the flower (Figures 9C–E). Following initiation of petals, two whorls of five stamens emerge almost simultaneously, with the antepetalous stamen primordia developing more rapidly in early stages (Figure 9D). A third whorl of five stamens is initiated centripetally (Figure 9E), resulting in fifteen stamens arranged in three whorls of five on the slope of the hypanthium (Figures 9F,G). Stamen growth tends to be unequal with one side developing faster (Figure 9E). No stamen, nor any other floral organ develops at the center of the flower (Figures 9F–I). During maturation, stamens curve toward the center but are never inflexed (Figures 9G–I). Much later after stamen initiation, five nectary glands develop alternating with petals and opposite the outermost antesepalous stamen whorl. Nectary glands expand to surround the base of the filaments of antesepalous stamens, clearly visible at anthesis (Figure 7G).
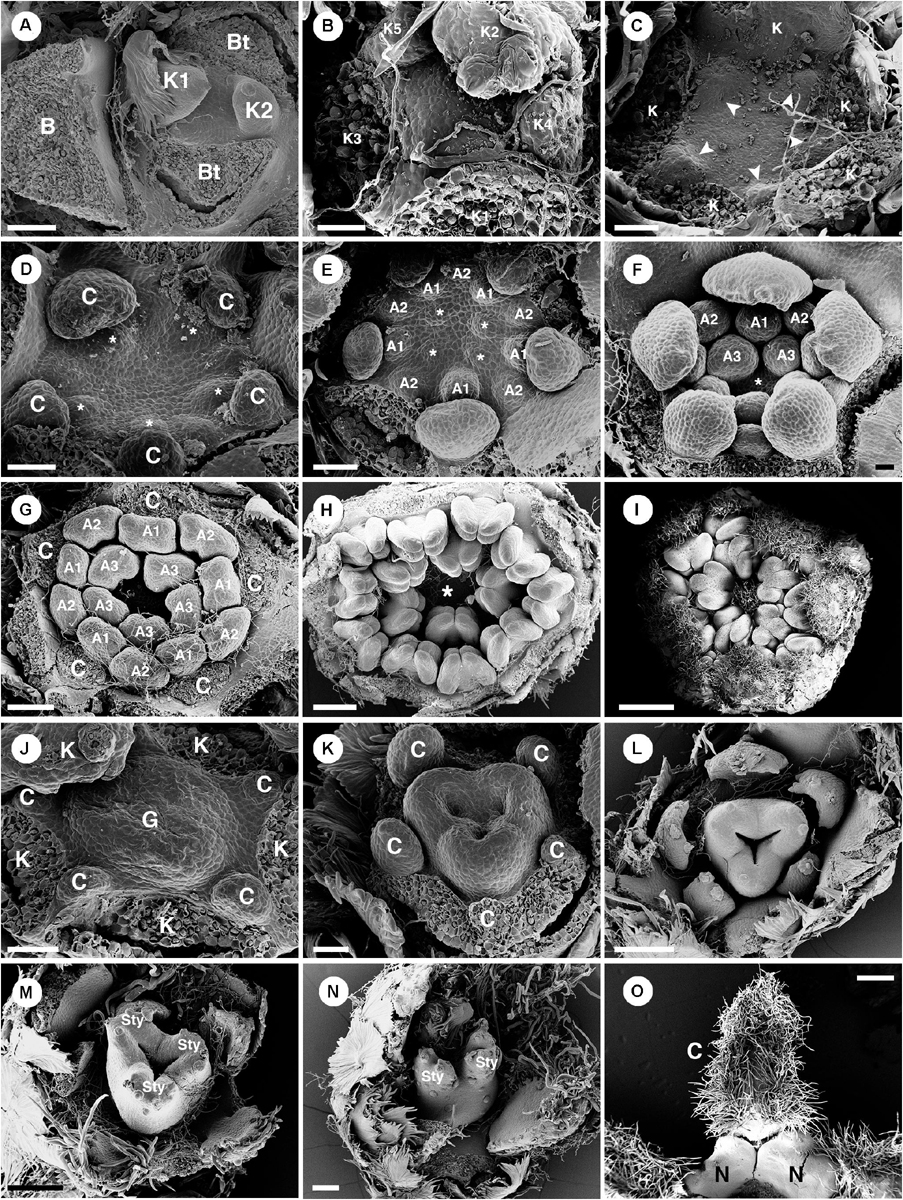
Figure 9. Ontogeny of staminate (A–I) and pistillate (J–O) flowers of Croton alabamensis. (A) A young convex floral primordium subtended by a bract and two bracteoles. The first and second sepals are visible. (B) Soon, more sepal primordia initiate and grow unequally in a quincuncial (2/5) pattern. Note, the floral primordium is still convex in shape. (C) After that, a hypanthium starts to form while five petal primordia initiate alternating with sepals (arrowhead). (D) Later, petals continue to grow and clearly show unequal growth. Two whorls of stamens are visible. Stamens from the antepetalous whorl (asterisk) is bigger than stamens from the alternipetalous whorl. Note, a hypanthium is well developed making the flower to become cup-shaped. (E) Next, the third whorl of stamens develops centripetally (asterisk). (F) The hypanthium starts to grow while petals and three whorls of stamens are visible. There is an empty space in the middle of the flower (asterisk). (G) Flower in later stage with all perianth parts removed shows three whorls of stamens. The outermost whorl alternates with petals. (H) Apical view of older flower bud shows central empty space (asterisk). (I) Later, stamens grow and bend toward the center but never become inflexed. (J) Pistillate flower in an early stage shows five petal primordia and a tricarpellate ovary formation. (K) In a later stage, petal primordia continue to grow unequally. Three fused carpels are clearly seen. (L) Next, petals continue to expand and the ovary start to close. (M) A style starts to form on top of each carpel. (N) Three bilobed styles are visible on the ovary. (O) At a much older stage, five bilobed nectary glands are formed alternating with petals. K, sepal; C, petal; A, stamen; G, ovary; Sty, style; N, nectary. Scale bars: (A,G,N) = 100 μm; (B,F) = 30 μm; (C–E,J,K) = 50 μm; (H) = 200 μm; (I) = 1,000 μm; (L,M) = 150 μm; (O) = 400 μm.
Ontogeny of Pistillate Flowers
Ontogeny of the pistillate flower was observed in C. alabamensis var. alabamensis. Pistillate flowers are located on the lower part of the inflorescence (Figure 1A). A bract and two bracteoles subtend the flower. Five sepals are present with quincuncial aestivation implying a helical initiation pattern. Five petals emerge simultaneously alternate to sepals (Figure 9J). A congenitally fused tri-carpellate ovary develops rapidly, even when petal primordia are still young (Figure 9J). Petal growth is unequal with the abaxial petals developing faster (Figures 9J–L). Contrary to C. chilensis, all petals in pistillate flowers continue to expand until they resemble petals in staminate flowers at anthesis (Figures 9J–O). A bifid style develops on top of each carpel (Figure 9N). However, some samples have a simple style at the mature stage, while some flowers retain a bifid style (Figure 9N). Much later, nectary glands develop at the base of the ovary in an antesepalous position (Figure 9O).
Croton schiedeanus
General Morphology
In Croton schiedeanus, flowers are produced spirally on racemose inflorescences (Figure 1B). Inflorescences are axillary borne on the main axis. One to two pistillate flowers are present on the proximal part and bloom earlier than staminate flowers on the distal part (Figure 1B). Both staminate and pistillate flowers are pedicellate and subtended by a bract and two bracteoles (Figures 1B, 2C). The pedicels of staminate flowers are slender while pedicels of pistillate flowers are bulky and longer (Figure 1B). Flowers from both genders are pentamerous.
There are two whorls of perianth parts in staminate flowers (Figures 10A,D, 2C). Sepal shape is ovate with a wide base and acute apex (Figures 10B,C). The arrangement of sepals follows a quincuncial pattern (Figure 10A). The abaxial side of sepals is covered by lepidote trichomes (Figure 10B), while on the adaxial side there are many simple trichomes present near the margin and the apex (Figure 10C). Petal shape is ovate with a narrow base and obtuse apex (Figures 10E,F) arranged in cochlear aestivation (Figure 10D). The abaxial surface of petals is mostly glabrous with one or two lepidote trichomes present (Figure 10E). The adaxial surface of petals is also mostly glabrous and sparsely covered with simple trichomes (Figure 10F). Margin and apex of petals are fimbriate, lined with simple trichomes (Figures 10E,F). Outside the androecium, there are five bilobed nectary glands alternate with the petals (Figure 10F). Centrally, about 11 stamens are arranged on a convex receptacle with the outermost whorl opposite to petals (Figures 2C, 10H). Anthers are inflexed in bud (Figures 10F–H). Filaments are generally glabrous but sometimes a stellate trichome may be present (Figure 10H). There is no indication of an ovary.
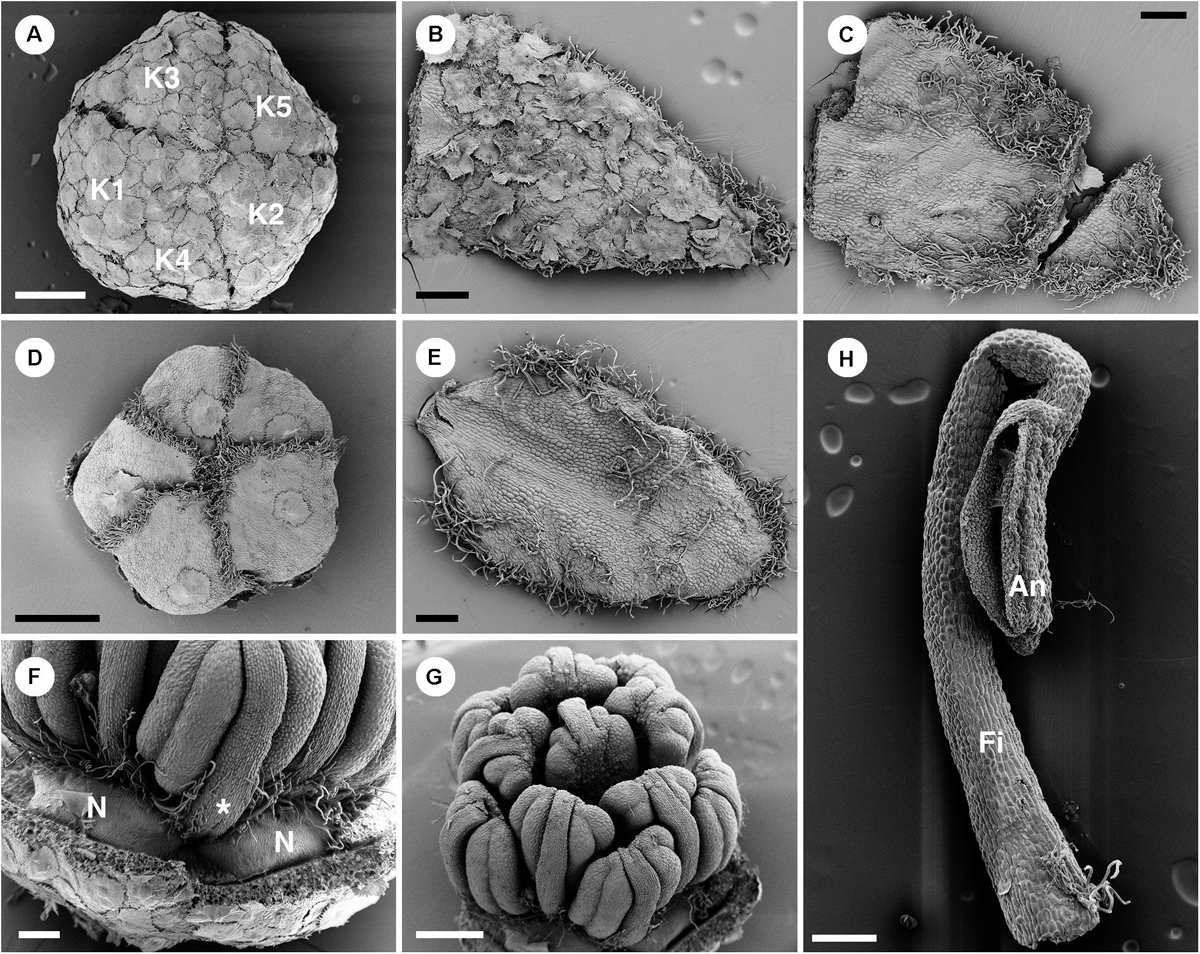
Figure 10. Morphology of staminate flowers of Croton schiedeanus. (A) Calyx in flower bud with quincuncial aestivation. The surface is covered with lepidote trichomes. (B) Abaxial surface of a sepal with lepidote trichomes. (C) Adaxial surface of a sepal with many simple trichomes on the margin and apex. (D) Corolla in a flower bud with cochlear aestivation. There is a lepidote trichome visible on each petal. Note the frimbriate margin of petals. (E) Adaxail side of a sepal with few simple trichomes on the surface. There are many simple trichomes lining the margin and apex. (F) Nectaries located in alternation with petals and outermost stamens (asterisk). (G) There are 11 stamens arranged in two whorls surrounding a central stamen. The outermost stamens are arranged opposite petals. Note, stamens are inflexed in a flower bud. (H) A stamen with a stellate trichome on the base of the filament. K, sepal; N, nectary; Fi, filament; An, anther. Scale bars: (A,D,G) = 500 μm; (B,C,E,F,H) = 200 μm.
Pistillate flowers are borne on longer and thicker pedicels than staminate flowers (Figure 11A). In pistillate flowers, both sepals and petals are present (Figures 1B, 2C, 11B). Sepals have an ovate shape with wide base and acute apex (Figures 11C,D), arranged in quincuncial aestivation. On the abaxial side of the sepals, there are many lepidote trichomes covering the whole surface (Figure 11C). On the adaxial side, there are stellate trichomes on the upper part, but the lower part is glabrous (Figure 11D). Petals have an obovate shape with narrow base and obtuse apex and margins of petals overlap in a quincuncial aestivation only in the upper part (Figures 11C–H). Petal lobes alternate with nectary glands (Figures 2C, 11H,I). The abaxial surface of petals is mostly glabrous with a few lepidote trichomes on the midrib (Figures 11E,F). The adaxial surface is also mostly glabrous with some simple trichomes near the margin (Figure 11G). The margin of petals is fimbriate, densely covered with crushed stellate and simple trichomes (Figures 11E–H). Five nectary lobes are present opposite the sepals and are basally confluent at the base of the petals (Figures 11H,I). Within the nectary, there is a tricarpellate ovary covered with lepidote trichomes (Figure 11J). Three styles are present with twice bifid (Figure 1B) or multifid stigmatic tips (Figure 11K).
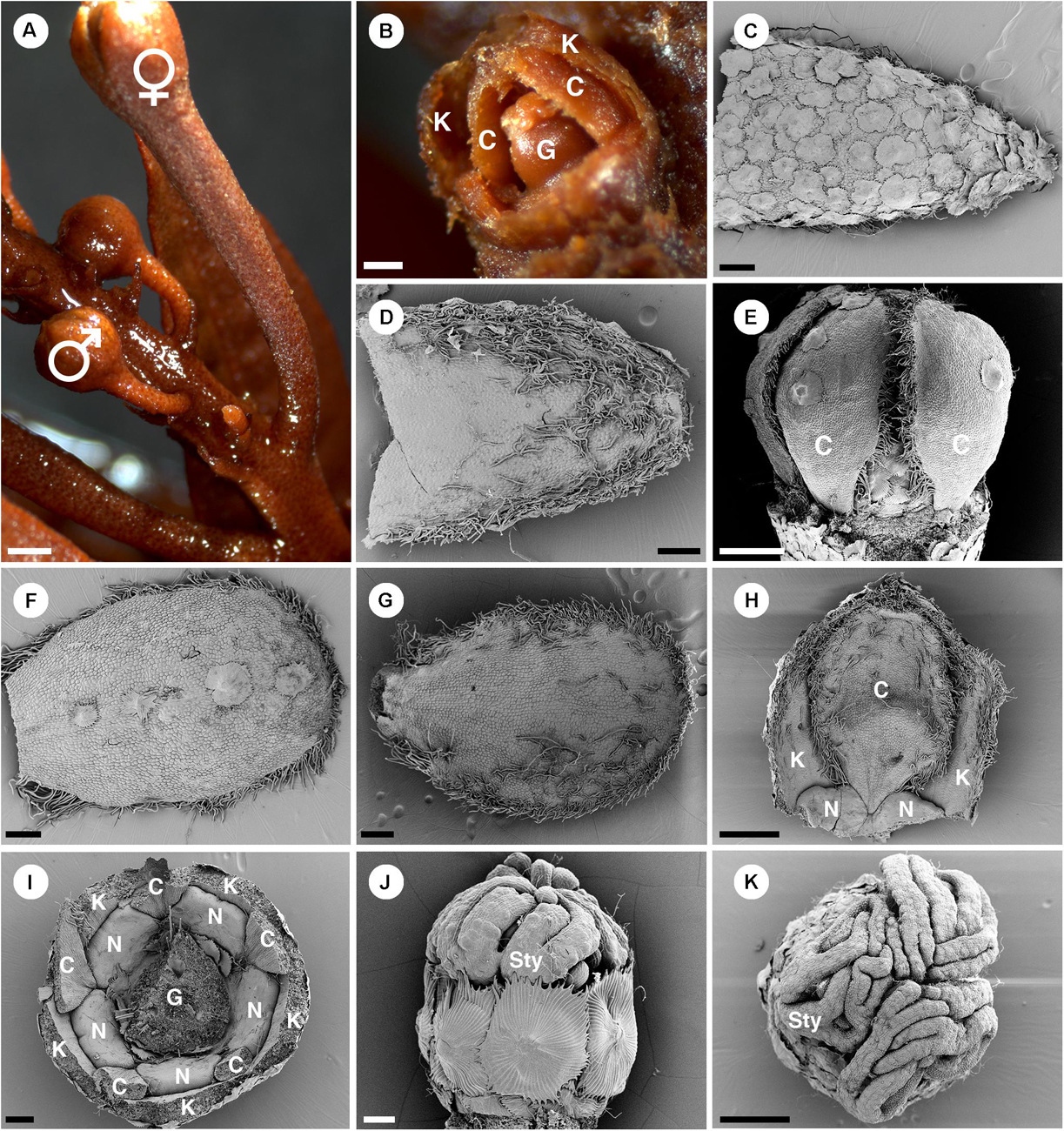
Figure 11. Morphology of pistillate flowers of Croton schiedeanus. (A) An inflorescence with a pistillate flower on the lower part and many proximal staminate flower buds. The pistillate flower has a longer and thicker pedicel than staminate flower buds. (B) A pistillate flower bud with some sepals removed. There are petals visible within. (C) Abaxial side of a sepal covered completely with lepidote trichomes. (D) Adaxial side of a sepal with simple and stellate trichomes present. (E) A pistillate flower bud which calyx and nectary glands removed. Petals show a narrow base. (F) Abaxial side of a petal. There are few lepidote trichomes along the midrib. The margin is fimbriate. (G) Adaxial side of a petal. The fimbriate margin consists of crushed stellate trichomes and densely packed simple trichomes. (H) A petal is arranged alternating with sepals and nectaries. Note, the base looks like a claw due to the influence of the nectary expansion during development. (I) A flower bud in which the ovary and part of the perianth were removed. There are five bilobed nectary glands located around the ovary. (J) Ovary with surface covered with lepidote trichomes. There are styles on top of it. (K) Styles on top of an ovary with multifid stigmatic tips. K, sepal; C, petal; G, ovary; N, nectary; Sty, style. Scale bars: (A) = 1,000 μm; (B–D,F,G,I) = 200 μm; (E,H,K) = 500 μm; (J) = 100 μm.
Ontogeny of Staminate Flowers
Staminate flowers develop on the distal part of the inflorescence (Figure 1B). Flowers are subtended by a bract and two bracteoles. Five sepals are present (Figure 2C). The shape of floral primordia is convex, similar to C. chilensis (Figures 12A–I). Five petals emerge simultaneously alternating to the sepals (Figures 12B,C). Later growth is unequal with the abaxial petals developing faster (Figures 12D–F). The first whorl of five stamens emerges alternating with the petals (Figures 12E,F). Next, the second stamen whorl emerges outside the first whorl (centrifugally) opposite the petals (Figures 12F,G). The last stamen to develop is a single stamen occupying the central area of the flower (Figures 12H,I). During maturation, stamens curve and fold resulting in an inflexed anther appearance (Figure 10H). Nectary glands initiate much later after the stamen development in alternation with petals.
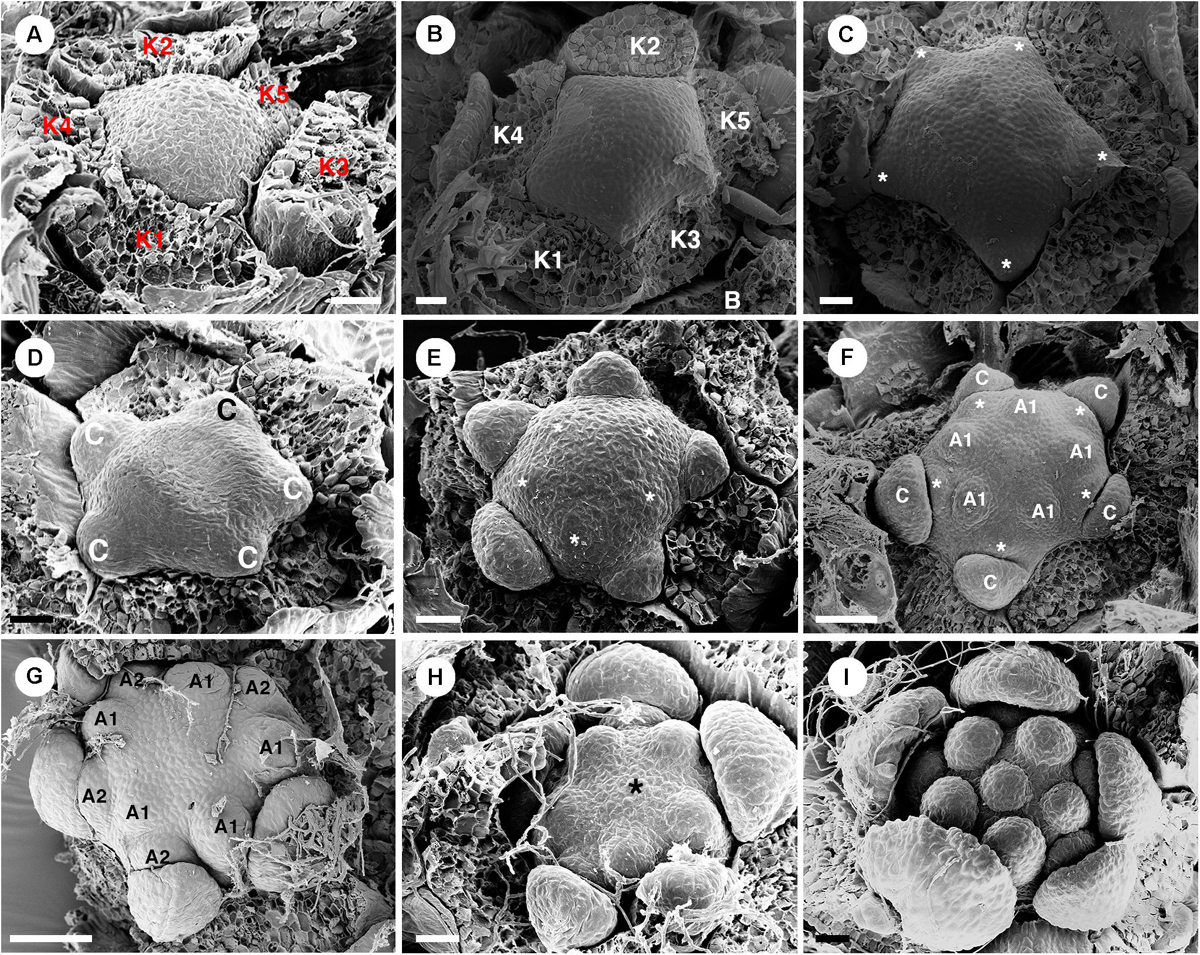
Figure 12. Ontogeny of staminate flowers from Croton schiedeanus. (A) A very young flower bud with five sepals initiating first. The rest of the floral primordium has a convex shape. (B) Apical view of a similar stage; petal primordia start to initiate alternating with sepals. (C) Next, petals grow and expand (asterisk). (D) Petals show a unidirectional growth pattern. (E) Next, the first stamen whorl starts to develop on the convex receptacle alternating with petals (asterisk). (F) Soon, the second whorl of stamens develops centrifugally outside of the first whorl opposite petals. (G) Apical view of slightly older stage, two stamen whorls are visible with the outermost whorl opposite petals. (H) After that, a central stamen starts to initiate on the central empty space (asterisk). (I) Apical view of a young flower shows the completion of stamen development. K, sepal; C, petal; A, stamen. Scale bars: (A–E,I) = 20 μm; (F,G) = 50 μm.
Ontogeny of Pistillate Flowers
There are few pistillate flowers of C. schiedeanus located on the proximal part of the inflorescence (Figures 1B, 2C). A pistillate flower is borne in the axil of a bract and sided by two bracteoles. Sepals initiate spirally in a 2/5 pattern (Figure 13A). Five petal primordia develop alternate to them (Figures 13B,C) and show unequal growth similar to other species (Figures 13D,E). Similar to C. alabamensis, petals continue to grow and expand throughout the floral development (Figures 13C–F,I). Three congenitally fused carpels initiate at the center of flower (Figures 13E,F). The ovary continues to develop and enlarge in size (Figures 13F–I). Later three styles arise on top of the ovary. Many lobes appear on each style (Figure 13H) which later becomes multifid (Figure 11K) derived from a bifid pattern (Figure 13I). Antesepalous nectaries (Figure 11I) develop in the late ontogeny.
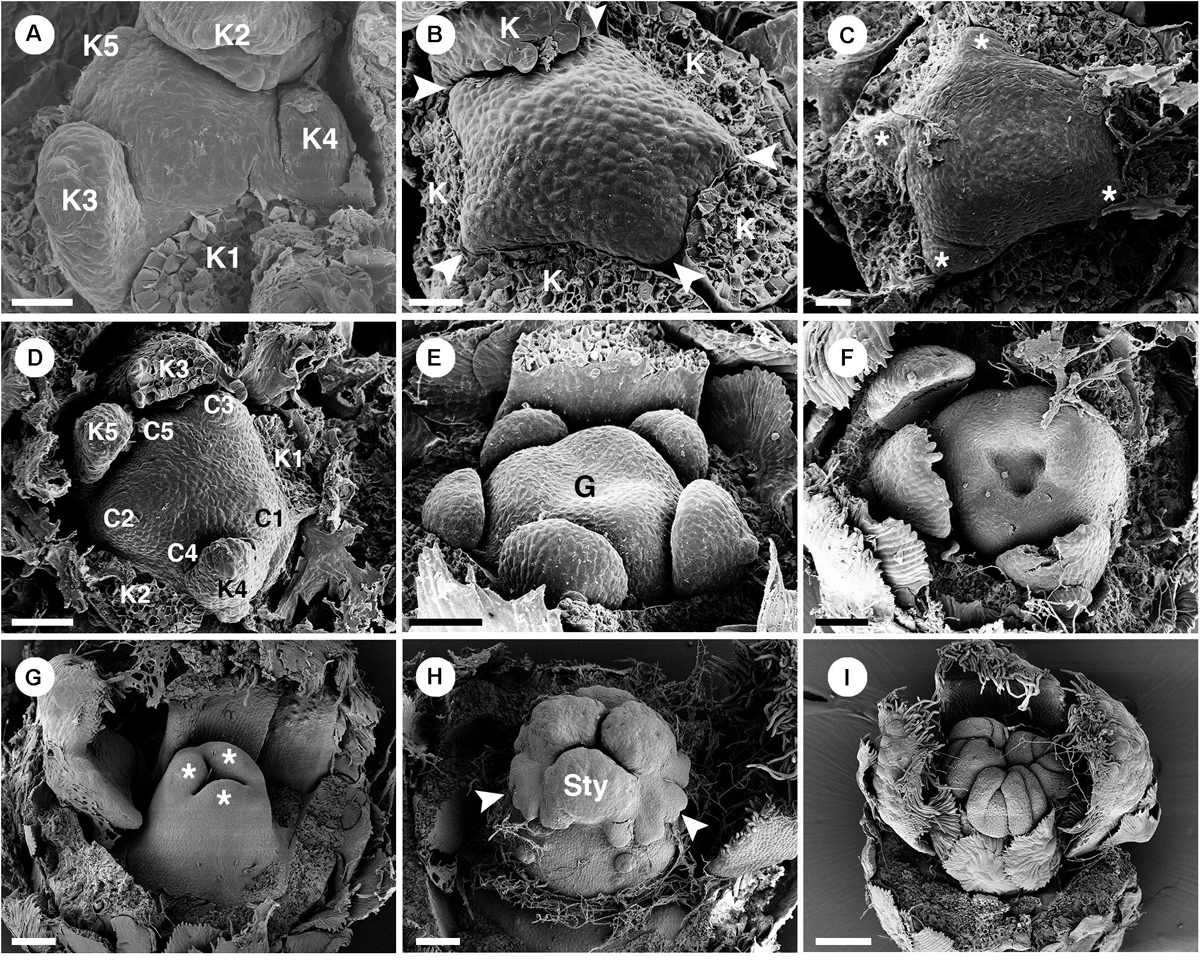
Figure 13. Ontogeny of pistillate flowers of Croton schiedeanus. (A) An early stage of a flower shows unequal sepal lobes on the convex floral primordium. (B) Next, petal primordia start to form alternating with sepals (arrowhead). (C) Apical view of older stage; petals start to expand surrounding the convex receptacle (asterisk). (D) View of a similar stage shows unequal growth of petals. (E) Next, an ovary initiates with three fused carpels. Note, petals continue to grow in a unidirectional pattern. (F) An old flower bud shows expansion of petals and ovary. (G) Stage of ovary closure. The lobe on top of each carpel starts to form a style (asterisk). (H) Three styles are formed on top of the ovary. Note, there are many lobes visible (arrowhead). (I) Later, styles dissected into many parts. K, sepal; C, petal; G, ovary; Sty, style. Scale bars: (A–C) = 20 μm; (D–F) = 5 0μm; (G) = 90 μm; (H) = 100 μm; (I) = 150 μm.
Discussion
Petal Evolution in Croton
Petals are generally found in staminate flowers of Croton (Webster, 1993; Berry et al., 2005; van Ee et al., 2011). Filamentous structures are reported to occur in pistillate flowers in the same position as petals in staminate flowers (De-Paula et al., 2011; Gagliardi et al., 2017). In the past the filamentous structures have been interpreted either as reduced petals or as staminodes (Michaelis, 1924; Nair and Abraham, 1962; Webster, 1993; Radcliffe-Smith, 2001; Caruzo and Cordeiro, 2007; De-Paula et al., 2011; Gagliardi et al., 2017). However, fully developed petals are found in several species from two New World sections, i.e., section Alabamenses and section Eluteria subsection Eluteria (Figure 2B; van Ee et al., 2011), and some African species (Friis and Gilbert, 2008; Berry et al., 2016). The section Alabamenses is a monotypic group in subgenus Quadrilobi without any close relative with well-developed petals in pistillate flowers (van Ee et al., 2011). The section Eluteria (subgenus Geiseleria) consists of three subsections comprising 22 species (van Ee et al., 2011). The presence of well-developed petals in pistillate flowers is reported in the 15 species of subsection Eluteria, whereas the other two subsections lack well-developed petals in pistillate flowers (van Ee and Berry, 2009; van Ee et al., 2011). In the subgenus Croton, pistillate flowers with petals are found in some African species, e.g., C. gratissimus and C. megalocarpus, which are nested among other species without petals in pistillate flowers (van Ee et al., 2015; Haber et al., 2017). Therefore, it is clear that petals in pistillate flowers have evolved independently among distantly related groups within Croton (Figure 2B). Our study demonstrates that in the early developmental stages, petals of staminate flowers are similar to petals and filamentous structures of pistillate flowers. Petals develop as broadly based structures in alternation with sepals before the androecium or gynoecium (Figure 5A vs. Figure 6C: C. chilensis; Figure 9C vs. Figure 9J: C. alabamensis; Figure 12C vs. Figure 13C: C. schiedeanus). So, it is interesting to know if petals in Croton (and other inaperturate crotonoid taxa) have a tepalar origin (bracteopetals) or staminodial origin (andropetals). Andropetals have a delayed initiation; they develop as part of the androecium (as part of fascicles or an androecial ring primordium), and primordia are characteristically narrow and morphologically comparable to stamens (Ronse De Craene, 2008). However, petals of Croton in both staminate and pistillate flowers (including filamentous structures) initiate as broad primordia immediately after sepals (Figures 5A, 6C, 9C,J, 12C, 13C) which are characteristics of bracteopetals (Ronse De Craene, 2008). At maturity, petals of pistillate and staminate flowers are identical. The morphology of petals and sepals also appears to be highly similar, with a broad range of trichomes developing on both organs, implying that perianth parts represent homologous structures.
We consider that the strongly different morphologies of petals and filamentous structures at maturity could be explained by heterochrony. Heterochrony implies a shift in the timing of development of different organs, leading to changes in the shape of size of organs, resulting in evolutionary change. This was recognized as one of the major drivers of morphological evolution (Li and Johnston, 2000; Box and Glover, 2010; Ronse De Craene, 2018; Buendía-Monreal and Gillmor, 2018). After initiation, petals in staminate flowers continue to grow and expand throughout the whole development until maturity (Figures 5, 9, 12), while growth of pistillate petals is soon arrested or strongly delayed (Figures 6, 9, 13) suggesting an early developmental blockage of petal development (pedomorphosis). The presence of fully developed petals in pistillate flowers of C. alabamensis and C. schiedeanus suggests that in those species there is a reversal of the process. One could speculate that the presence of fully developed petals in pistillate flowers resembles the ancestral condition. However, C. alabamensis, C. schiedeanus (sect. Eluteria) and the above-mentioned African species are nested in clades containing other Croton species having no petals in pistillate flowers (Figure 2B; Berry et al., 2005; van Ee et al., 2011, 2015), suggesting that the absence of fully developed petals is plesiomorphic in these clades. Most of the genera in tribe Crotoneae also lack fully developed petals in pistillate flowers (Figure 2B; Webster, 2014; Silva et al., 2020). However, among them only pistillate flowers of Sandwithia are reported to have miniature petals (Lanjouw, 1932; Secco, 1987; P. Thaowetsuwan, unpublished data). Outside Crotoneae, petals in pistillate flowers are present in several groups. In the tribe Jatropheae, a sister tribe of the Crotoneae, all three genera, i.e., Jatropha, Joannesia, and Vaupesia, have petals in both staminate and pistillate flowers (Velloso, 1798; Ducke, 1922; Schultes, 1955; Dehgan and Webster, 1979; Radcliffe-Smith, 2001; Webster, 2014). Moreover, Grossera, Cavacoa, Leeuwenbergia, and Dodecastigma, which are in lower grades of another inaperturate crotonoid clade, are reported to have petals in pistillate flowers as well (Radcliffe-Smith, 2001; Webster, 2014; van Welzen et al., 2020). Therefore, the presence of fully developed petals may be an ancestral character of the inaperturate crotonoids (clade C1 and C2 of the subfamily Crotonoideae following Wurdack et al., 2005). However, the articulated crotonoid clade and Gelonieae clade, at the basal nodes of the subfamily Crotonoideae, do not have petals (Figure 2B; Radcliffe-Smith, 2001; Wurdack et al., 2005; Webster, 2014).
Since fully developed petals occur in groups distantly related with Croton (Figure 2B), one could consider the occurrence of fully developed petals in pistillate flowers of some Croton species as a case of “homoplasy.” However, combining both phylogenetic and ontogenetic perspectives, we conclude that this phenomenon is preferably explained as an “apomorphic tendency” (or cryptic apomorphy). This represents a morphological character (fully developed petals in pistillate flowers) that appears in some members but not in all species (Croton) and can be genetically switched on or off because of deeply shared genetic attributes. As a result this character is not recognized as a synapomorphy on the phylogenetic tree (Figure 2B; Endress, 2003; Endress and Matthews, 2006; Ronse De Craene, 2010, 2018). Our idea is supported by the mixture of petal-like and filamentous structures in some pistillate flowers from various species of Croton (Figure 4F; P. Thaowetsuwan, unpublished data). The reduction of petals in pistillate flowers may also be explained by a shift in B gene expression. In Arabidopsis, the mutation of AP3 and PI genes (B-class genes) results in the transformation of petals into sepals and stamens into carpels (Bowman et al., 1991; Jack et al., 1992; Goto and Meyerowitz, 1994; Litt and Kramer, 2010; Rijpkema et al., 2010). In strongly heteromorphic species, pistillate flowers often have reduced petals or lack petals altogether, as in Croton (e.g., Gunnera in Gunneraceae: Ronse De Craene and Wanntorp, 2006; Grevea in Montiniaceae: Ronse De Craene, 2016; Myriophyllum in Haloragaceae: Kubitzki, 2007). This suggests that petal reduction is linked to a shifting balance of B-genes responsible for petal identity in these plants. A reduced B-gene expression may be responsible for the reduction of petals to small appendages, and in the case of Croton schiedeanus and C. alabamensis a reversed shift might be the cause of a strong petal morphology.
Mechanical pressure is another important factor that shapes the morphology of floral organs (Ronse De Craene, 2018). We found that the growth and mature morphology of petals in Croton is affected by the pressure exercised on the flower bud during development. As filamentous structures develop more slowly than well-developed petals, they are more prone to be squeezed between the enclosing calyx and the massive ovary. As a result, the filamentous structures become long and narrowly shaped (Figures 4F, 6J,K) differing greatly from fully developed petals. The morphology of fully developed petals in pistillate flowers is also shaped by the alternating nectary glands in both staminate and pistillate flowers (Figures 4A,F, 6L). However, nectary glands in pistillate flowers are much larger and sometimes confluent in a ring. Pressure from adjacent nectary glands induce the petals in pistillate flowers to have a very narrow base resembling clawed petals or staminodial structures (Figures 11H,I). However, with ontogenetic data, we could confirm that the petals of pistillate flowers represent bracteopetals and the claw-like morphology is a result of spatial pressure from nectary glands in the later stages.
We could not find any concrete evidence of ecological factors that lead to the development of fully developed petals in pistillate flowers. Evolutionary pressure from flower-insect interaction may be an important factor. However, very little attention has been paid to pollination studies in Croton. Three pollination syndromes were reported in Croton, e.g., wind pollination (Domínguez et al., 1989), insect pollination (Freitas et al., 2001; Pires et al., 2004; Narbona and Dirzo, 2010), or mixed pollination (Novo et al., 2010). However, those studies were conducted in few species of Croton. C. alabamensis was initially reported to have wind pollination but later observation suggested insect pollination, supported by the presence of nectaries in both genders (van Ee et al., 2006). It is known that all pistillate flowers of Croton have nectar producing glands surrounding the ovary to attract insects. The presence of petals in pistillate flowers may help increase flower visibility. However, petals in Croton are generally green or white in color similar to sepals and may not contribute to insect attraction (Figures 1C, 3A,I). We also observed in the present study that in two Croton species with fully developed petals, there are fewer pistillate flowers in the inflorescence, suggesting that the presence of petals may increase the chance of pollination; however, many other Croton species possess a smaller number of pistillate flowers in inflorescences without having fully developed petals (P. Thaowetsuwan, unpublished data). All neotropical Croton and African species with petals in pistillate flowers also have lepidote trichomes. However, we think that this association is just a coincidence because other Croton with lepidote trichomes do not have fully developed petals in pistillate flowers (P. Thaowetsuwan, unpublished data; van Ee et al., 2011). Further interdisciplinary studies, including genetics, pollination ecology, and morphology, are needed to clarify the presence of fully developed petals in pistillate flowers of some Croton.
The Interpretation of Nectaries in Croton
Nectaries are either found as five bilobed antesepalous nectaries or as an interrupted nectary ring in both staminate and pistillate flowers of all three species (Figures 3J, 4D, 7G, 8G, 10F, 11I). Nectary lobes appear bilobed in pistillate flowers, apparently developing as two primordia adjacent to each petal (total of ten) which later expand and fuse together (see De-Paula et al., 2011). However, closer detail reveals that the nectary develops from a rim of tissue below the ovary and becomes increasingly dissected by the intervening filamentous structures (Figures 6E–I). We could not observe the initiation stage of the nectary primordia in staminate flowers. However, results from a previous study suggest the emergence of a single primordium (De-Paula et al., 2011). In staminate flowers of C. alabamensis, however, there are ten nectary lobes alternating with petals and stamens, arranged into five horse-shoe shaped nectaries clasping the base of the outermost stamens (Figure 7G). We hypothesized that during development, nectary glands initiate alternating with the petals similar to other species of Croton (Figure 2C). However, this space is already occupied by stamens in C. alabamensis. Therefore, the nectary glands develop preferentially on the available space (adjacent to stamens/petals) rather than opposite the sepals (Figure 7G).
Throughout the genus, nectaries of pistillate flowers are variously fused, often in a ring at the base of the gynoecium (Freitas et al., 2001; Caruzo and Cordeiro, 2007; De-Paula et al., 2011) while nectaries of staminate flowers are generally free (P. Thaowetsuwan, unpublished data; De-Paula et al., 2011). Different authors interpreted the origin of nectaries either as receptacular outgrowths (Caruzo and Cordeiro, 2007) or as transformed staminodes (De-Paula et al., 2011; Gagliardi et al., 2017). De-Paula et al. (2011) interpreted nectaries as secretory staminodes based on their antesepalous position in the flower, despite their late initiation. Ronse De Craene and Smets (2001) suggested that staminodes can be transformed into nectaries with a retention of the original stamen vasculature diverging from the stele (e.g., Harungana, Hypericaceae; Averrhoa, Oxalidaceae). However, in Croton, the nectaries are generally not supplied by a clear whorl of bundles as would be the case with stamen-derived nectaries (as in Harungana of Hypericaceae: Ronse de Craene and Smets, 1991a). Instead, nectaries from most species are supplied by traces derived from sepal bundles (De-Paula et al., 2011). Moreover, nectaries in some species of Jatropha were found to be supplied by vascular bundles from both sepals and petals, or from petals alone (Venkata-Rao and Ramalakshmi, 1968). Venkata-Rao and Ramalakshmi (1968) interpreted the nectary of Jatropha as of receptacular origin because they are not supplied by bundles from the floral stele. In the Crotoneae genus Acidocroton, non-vascularized multi-lobed nectary glands are present forming a ring around the androecium in staminate flowers or the gynoecium in pistillate flowers (P. Thaowetsuwan, unpublished data; Fawcett and Rendle, 1920). Further expansion of the nectary into the androecial zone forming a honeycomb-like nectary is observed in some Acidocroton species, emphasizing the variability of the nectary development in the tribe (P. Thaowetsuwan, unpublished data). Vascular connections in Croton appear to be opportunistic, since non-vascularized nectaries have also been reported in C. bonplandianus (Venkata-Rao and Ramalakshmi, 1968), C. sarcopetalus (Freitas et al., 2001), and in the related genus Astraea (De-Paula et al., 2011). Non-vascularized floral nectaries were also found in the genus Brasiliocroton, the sister genus of Croton (P. Thaowetsuwan, unpublished data). The late initiation, variation in number and shape, as well as the variability in its vasculature have been used as criteria to justify the interpretation of the floral nectary in the family Brassicaceae as of receptacular origin (Arber, 1931; Bowman and Smyth, 1998). A similar variability in shape, number, development and opportunistic vascular connections was found in the genus Croton, supporting a receptacular origin of the nectaries in the genus, while the only argument supporting the staminodial origin is their antesepalous (alternipetalous) position. Considering the nectary as of receptacular origin, links the floral morphology of Euphorbiaceae with other families in the order Malpighiales. A systematic survey by Bernardello (2007) suggests that most nectaries of Malpighiales are of receptacular (and hypanthial) origin, with the occasional presence of staminodial nectaries, e.g., Bonnetiaceae, some Clusiaceae and Linaceae. Interpreting the nectaries as staminodial in Croton because of their position in alternation with the other stamens is a static approach that does not consider the spatial constraints during development, where space is mostly available between the petals. The outer stamen whorl in most Croton is antepetalous and the greatest space for the late development of nectaries is in antesepalous position. The presence of horse-shoe shaped nectaries clasping the stamen bases in staminate flowers of C. alabamensis (Figure 7G) also show plasticity of nectary position supporting the interpretation of a receptacular origin.
Floral Diversity in Staminate Flowers
Our observations suggest that diversity of structures and their development in staminate flowers of Croton is unexpectedly high. At maturity, the receptacle of staminate flowers of C. chilensis and C. schiedeanus is convex, as reported in many species from previous studies (Michaelis, 1924; De-Paula et al., 2011; Gagliardi et al., 2017) while C. alabamensis has a concave receptacle in the form of a hypanthium (Figures 2C, 7G,H, 9). Cup-shaped flowers of C. alabamensis were reported before (Ginzbarg, 1992) but it has never been considered to be unique among the genus Croton before. A similar condition has been found in staminate flowers from other Crotoneae genera, viz., Sagotia racemosa (slightly concave) and Brasiliocroton muricatus (flat receptacle), but in those species, stamens fully occupy the floral apex area, contrary to C. alabamensis with a central empty space (P. Thaowetsuwan, unpublished data).
Moreover, our observation found that the second whorl of stamens from all three species develops centrifugally opposite to petals (Figures 5, 9, 12). In C. schiedeanus, this whorl appears to be the outermost whorl throughout the whole ontogeny (Figures 2C, 10G, 12) as was previously reported for other Croton species (De-Paula et al., 2011). In early stages of development of C. chilensis, the alternipetalous whorl is the outermost whorl (Figures 5G–N) while at anthesis, it is the antepetalous whorl (Figures 2C, 3A). This shift in stamen position in C. chilensis could be explained by the fact that in the early stages, growth of the outermost antepetalous stamens is restricted by the expanding petals. Therefore, the alternipetalous whorl appears to be outermost and the first to arise. In later stages, nectary glands which develop on the empty space opposite the sepals emphasize the more central position of the alternipetalous stamen whorl. Finally, at anthesis petals start to spread out, allowing the antepetalous stamens to appear as the outermost whorl. Croton alabamensis also has a centrifugal stamen development but with the alternipetalous stamens as the initially smaller outermost whorl, while antepetalous stamens are initially larger and arranged as an inner whorl (Figures 7H, 9D–I). One possible explanation is that the vertical expansion of the hypanthium makes petals appear in a higher position than the stamen developing zone (Figure 9D), eliminating pressure from petals on the stamen primordia; therefore, antepetalous stamens have the possibility to emerge first or at least grow faster.
The centrifugal stamen development is unusual among angiosperms. This phenomenon is normally linked with a high stamen number (polyandry) (Corner, 1946; Ronse De Craene and Smets, 1992; Rudall, 2010, 2011). However, staminate flowers of Croton generally have about 11–16 stamens (P. Thaowetsuwan, unpublished data) which fall in the range of oligandry (flowers with the number of stamen two times the number of petals) to lower scale polyandry (flowers with the number of stamens much higher than petals). The development of the androecium in Croton is also different from other patterns of centrifugal stamen development, which is caused by a subdivision of primordia and represent a secondary stamen increase (cf. Ronse De Craene and Smets, 1992). Some cases of centrifugal stamen development in oligandric and lower-polyandric flower were reported before (Table 2). The centrifugal stamen development has often been linked with a reduction from ancestral flowers with polyandric centrifugal stamen development (Corner, 1946; Leins and Erbar, 1994). Alternatively, a centrifugal stamen development in oligandric flowers is interpreted as the result of a shift linked with obdiplostemony (Ronse De Craene and Bull-Hereñu, 2016). However, as discussed in Ronse De Craene and Bull-Hereñu (2016), a shift in position is linked with differential growth processes associated with pressures in the flower. The different shifts in position of outer alternipetalous stamens in the investigated Croton are caused by differential pressures during the development of the flower. For Croton, it is possible that polyandry is a derived character since it is mostly found in subgenus Adenophylli (van Ee et al., 2011). Moreover, previously described cases of oligandric centrifugal stamen development occur in bisexual flowers, while centrifugal stamen development in unisexual (staminate) flowers is rare. There are few reports of this phenomenon in staminate flowers of Phytelephantoid palms (Uhl and Moore, 1977), Populus in Salicaceae (Kaul, 1995), Medusagyne oppositifolia in Ochnaceae (Ronse De Craene, 2017) and Monococcus echinophorus in Petiveriaceae (Vanvinckenroye et al., 1997), but those developmental patterns are different from the one found in Croton (Table 2). Therefore, centrifugal stamen development in unisexual oligandric to lower-polyandric flowers such as Croton and Astraea, may be a unique feature. So far there is no floral ontogenetic study in other genera in the tribe Crotoneae apart of Astraea and Croton. Since the presence of centrifugal stamen development appears in all previous studied Croton, and also Astraea (De-Paula et al., 2011), we hypothesize that this developmental pattern may be present in the ancestor of Croton and perhaps in the common ancestor of Croton and Astraea too. Further studies in other genera in the tribe Crotoneae are needed to confirm our hypothesis.
The presence of the outermost stamen whorl opposite to the petals is found in all previously investigated Croton (and also Astraea) (Baillon, 1858; Marchand, 1860; Michaelis, 1924; Nair and Abraham, 1962; Venkata-Rao and Ramalakshmi, 1968; Gandhi and Thomas, 1983; De-Paula et al., 2011; Gagliardi et al., 2017) and is likely an ancestral character. In the present study, the arrangement of stamens with the alternipetalous stamens as an outermost whorl is almost unique for C. alabamensis and has never been reported in Croton before. The only other case of the alternipetalous stamen whorl as an outermost whorl is found in some species of Croton section Moacroton [Figure 1F in van Ee et al., 2008 (C. ekmanii); P. Thaowetsuwan, unpublished data] which is a specialized group adapted to serpentine soils found in the Caribbean islands (Borhidi, 1991). Despite C. alabamensis and Croton section Moacroton belonging to the same subgenus Quadrilobi, they are not closely related (van Ee et al., 2008, 2011). Therefore, the presence of the alternipetalous outermost whorl should be considered as the result of parallel evolution (cf. Vasconcelos et al., 2017). Croton alabamensis and Croton section Moacroton (except C. poecilanthus and C. maestrensis) also share the presence of non-inflexed stamens which is unique among all Croton species (Berry et al., 2005; van Ee et al., 2008, 2011). Nevertheless, stamen morphology from these two groups is different.
Inner stamen whorls of Croton chilensis show great variation in number and arrangement among different flowers. The variation may be influenced by spatial constraints between adjacent organs, which result in a different floral architecture in each flower. Developmental irregularity could occasionally happen as shown in a staminate flower of C. fuscescens (section Julocroton) from De-Paula et al. (2011). However, our observations found that irregular stamen development occurs more often in Croton subgenus Adenophylli in which many species have high stamen numbers (more than 20 stamens) leading to more than 100 stamens in some species from the medusae group in section Cyclostigma (P. Thaowetsuwan, unpublished data). Further studies on Croton with very high stamen numbers (section Adenophylli, section Cyclostigma), and very low numbers (section Moacroton, section Crotonopsis, section Eremocarpus, C. monanthogynus, etc.) may reveal novel stamen development patterns.
Conclusion
Our study reveals that floral development in both staminate and pistillate flowers of three species of Croton is highly diverse. Staminate flowers show a high variation of stamen number and development of the androecium that can be linked to spatial constraints during development. Sepals and petals in Croton are recognized as homologous structures, with petals equivalent to bracteopetals. In pistillate flowers, a heterochronic shift is responsible for the development of filamentous structures as under-developed petals. The presence of fully developed petals in C. alabamensis and C. schiedeanus suggests the independent reversal of this process and represents apomorphic tendencies rather than homoplasies. Nectaries are demonstrated to have a receptacular origin rather than staminodial, as supported by developmental and anatomical evidence.
Being a mega-diverse genus of the family Euphorbiaceae, Croton represents a great model system for the study of floral evolution. However, there have been few detailed floral morphological investigations until now, possibly hampered by the small size of flowers. We hope that this study will trigger further investigations on the floral ontogeny of Croton and other Euphorbiaceous genera to explore the evolutionary dynamics of their floral morphology.
Data Availability Statement
The raw data supporting the conclusions of this article will be made available by the authors, without undue reservation, to any qualified researcher.
Author Contributions
PT: manuscript preparation, experiment design, and conducting the experiment. SR: manuscript preparation, and conducting the experiment. RR: manuscript preparation and sample provision. LR: manuscript preparation and experiment design. All authors contributed to the article and approved the submitted version.
Funding
PT was funded by the Development and Promotion of Science and Technology talents project (DPST) Scholarship, Royal Thai Government. SR was supported by the Scottish International Education Trust. The Royal Botanic Garden Edinburgh was supported by the Scottish Government’s Rural and Environment Science and Analytical Services Division.
Conflict of Interest
The authors declare that the research was conducted in the absence of any commercial or financial relationships that could be construed as a potential conflict of interest.
Acknowledgments
We thank Lady Bird Johnson Wild Flower Center/Texas, USA for seeds of C. alabamensis var. taxensis. We also thank Gunnar Ovstebo and the horticultural team at RBGE for growing and maintaining living collections of Croton. Technical assistance of Frieda Christie with light microscopy and scanning electron microscopy is acknowledged. This paper is part of the Ph.D. thesis of the PT funded by the Development and Promotion of Science and Technology talents project (DPST) Scholarship, Royal Thai Government and the MSc dissertation of the second author supported by The Scottish International Education Trust.
References
Arber, A. (1931). Studies in floral morphology. I. On some structural features of the cruciferous flower 1. New Phytol. 30, 11–41. doi: 10.1111/j.1469-8137.1931.tb07402.x
Bachelier, J. B., Endress, P. K., and Ronse De Craene, L. P. (2011). “Comparative floral structure and development of Nitrariaceae (Sapindales) and systematic implications,” in Flowers on the Tree of Life, eds L. Wanntorp and L. Ronse De Craene (Cambridge: Cambridge University Press), 181–217. doi: 10.1017/CBO9781139013321.008
Bello, M. A., Hawkins, J. A., Rudall, P. J., Bello, M. A., Hawkins, J. A., and Rudall, P. J. (2008). Floral morphology and development in Quillajaceae and Surianaceae (Fabales), the species-poor relatives of Leguminosae and Polygalaceae. Ann. Bot. 101, 1433–1434. doi: 10.1093/aob/mcn073
Bernardello, G. (2007). “A systematic survey of floral nectaries,” in Nectaries and Nectar, eds S. W. Nicolson, M. Nepi, and E. Pacini (Dordrecht: Springer), 19–128. doi: 10.1007/978-1-4020-5937-7_2
Berry, P. E., Hipp, A. L., Wurdack, K. J., Van Ee, B., and Riina, R. (2005). Molecular phylogenetics of the giant genus Croton and tribe Crotoneae (Euphorbiaceae sensu stricto) using ITS and TrnL-TrnF DNA sequence data. Am. J. Bot. 92, 1520–1534. doi: 10.3732/ajb.92.9.1520
Berry, P. E., Van Ee, B. W., Kainulainen, K., and Achtemeier, L. (2016). Croton cupreolepis (Euphorbiaceae), a new coppery-lepidote tree species from eastern Madagascar. Syst. Bot. 41, 977–982. doi: 10.1600/036364416X694099
Bowman, J. L., and Smyth, D. R. (1998). Patterns of petal and stamen reduction in Australian species of Lepidium L. (Brassicaceae). Intern. J. Plant Sci. 159, 65–74. doi: 10.1086/297522
Bowman, J. L., Smyth, D. R., and Meyerowitz, E. M. (1991). Genetic interactions among floral homeotic genes of Arabidopsis. Development 112, 1–20.
Box, M. S., and Glover, B. J. (2010). A plant developmentalist’s guide to paedomorphosis: reintroducing a classic concept to a new generation. Trends Plant Sci. 15, 241–246. doi: 10.1016/j.tplants.2010.02.004
Buendía-Monreal, M., and Gillmor, C. S. (2018). The times they are A-changin’: heterochrony in plant development and evolution. Front. Plant Sci. 9:1349. doi: 10.3389/fpls.2018.01349
Caruzo, M. B. R., and Cordeiro, I. (2007). Sinopse da tribo Crotoneae dumort (Euphorbiaceae s.s.) no Estado de São Paulo, Brasil. Hoehnea 34, 571–585. doi: 10.1590/S2236-89062007000400011
Dehgan, B., and Webster, G. L. (1979). Morphology and infrageneric relationships of the genus Jatropha Euphorbiaceae. Univer. Calif. Public. Bot. 74, 1–73.
De-Paula, O. C., das Graças Sajo, M., Prenner, G., Cordeiro, I., and Rudall, P. J. (2011). Morphology, development and homologies of the perianth and floral nectaries in Croton and Astraea (Euphorbiaceae-Malpighiales). Plant Syst. Evol. 292, 1–14. doi: 10.1007/s00606-010-0388-389
Domínguez, C. A., Dirzo, R., Bullock, S. H., and Dominguez, C. A. (1989). On the function of floral nectar in Croton suberosus (Euphorbiaceae). Oikos 56:109. doi: 10.2307/3566093
Ducke, A. (1922). Plantes ouvelles ou peu connues de la région amazonienne. Archiv. Jardim Botânico do Rio de Janeiro 3, 269.
Endress, P., and Matthews, M. (2006). Elaborate petals and staminodes in eudicots: diversity, function, and evolution. Org. Div. Evol. 6, 257–293. doi: 10.1016/j.ode.2005.09.005
Endress, P. K. (1994). Diversity and Evolutionary Biology Of Tropical Flowers. Cambridge: Cambridge University Press.
Endress, P. K. (2003). “What should a ‘complete’ morphological phylogenetic anaylsis entail?,” in Deep Morphology: Towards A Renaissance Of Morphology In Plant Systematics, eds T. F. Stuessy, V. Mayer, and E. Hörandl (Liechtenstein: Ganter Verlag), 131–164.
Endress, P. K. (2008). Perianth biology in the basal grade of extant angiosperms. Intern. J. Plant Sci. 169, 844–862. doi: 10.1086/589691
Endress, P. K. (2010). Flower structure and trends of evolution in eudicots and their major subclades. Ann. Missouri Bot. Garden 97, 541–583. doi: 10.3417/2009139
Erbar, C., Kusma, S., and Leins, P. (1998). Developmental and interpretation of nectary organs in Ranunculaceae. Flora 194, 317–332. doi: 10.1016/s0367-2530(17)30920-9
Fawcett, W., and Rendle, A. B. (1920). Flora of Jamaica, Containing Descriptions Of The Flowering Plants Known From The Island Vol. 4 Dicotyledons Family Leguminosae to Callitrichae. London: The trustees of the british museum.
Freitas, L., Bernardello, G., Galetto, L., and Paoli, A. A. S. (2001). Nectaries and reproductive biology of Croton sarcopetalus (Euphorbiaceae). Bot. J. Linnean Soc. 136, 267–277. doi: 10.1111/j.1095-8339.2001.tb00572.x
Friis, I., and Gilbert, M. G. (2008). Croton megalocarpoides sp. nov. an arborescent Euphorbiaceae from semi-evergreen vegetation in S Somalia and E Kenya. Nordic J. Bot. 4, 327–331. doi: 10.1111/j.1756-1051.1984.tb01503.x
Gagliardi, K. B., Cordeiro, I., and Demarco, D. (2016). Protection and attraction: bracts and secretory structures in reduced inflorescences of Malpighiales. Flora 220, 52–62. doi: 10.1016/j.flora.2016.02.003
Gagliardi, K. B., Cordeiro, I., and Demarco, D. (2017). Flower development in species of Croton (Euphorbiaceae) and its implications for floral morphological diversity in the genus. Austr. J. Bot. 65:538. doi: 10.1071/BT17045
Gandhi, K. N., and Thomas, R. D. (1983). A note on the androecium of the genus Croton and flowers in general of the family Euphobiaceae. Phytologia 54, 6–8.
Ginzbarg, S. (1992). A new disjunct variety of Croton alabamensis (Euphorbiaceae) from Texas. SIDA Contribut. Bot. 15, 41–52.
Goto, K., and Meyerowitz, E. M. (1994). Function and regulation of the Arabidopsis floral homeotic gene PISTILLATA. Genes Dev. 8, 1548–1560. doi: 10.1101/gad.8.13.1548
Govaerts, R., Frodin, D. G., and Radcliffe-Smith, A. (2000). World Checklist And Bibliography Of Euphorbiaceae (And Pandaceae) 2. Kew: Royal Botanic Garden Kew.
Haber, E. A., Kainulainen, K., Van Ee, B. W., Oyserman, B. O., and Berry, P. E. (2017). Phylogenetic relationships of a major diversification of Croton (Euphorbiaceae) in the western Indian Ocean region. Bot. J. Linn. Soc. 183, 532–544. doi: 10.1093/botlinnean/box004
Hardy, C. R., and Stevenson, D. W. (2000). Floral Organogenesis in some species of Tradescantia and Callisia (Commelinaceae). Int. J. Plant Sci. 161, 551–562. doi: 10.1086/314279
Horn, J. W., van Ee, B. W., Morawetz, J. J., Riina, R., Steinmann, V. W., Berry, P. E., et al. (2012). Phylogenetics and the evolution of major structural characters in the giant genus Euphorbia L.(Euphorbiaceae). Mol. Phyl. Evol. 63, 305–326. doi: 10.1016/j.ympev.2011.12.022
Jack, T., Brockman, L. L., and Meyerowitz, E. M. (1992). The homeotic gene APETALA3 of Arabidopsis thaliana encodes a MADS box and is expressed in petals and stamens. Cell 68, 683–697. doi: 10.1016/0092-8674(92)90144-2
Juncosa, A. M., and Tomlinson, P. B. (1987). Floral development in mangrove Rhizophoraceae. Am. J. Bot. 74, 1263–1279. doi: 10.1002/j.1537-2197.1987.tb08740.x
Kaul, R. B. (1995). Reproductive structure and organogenesis in a cottonwood, Populus deltoides (Salicaceae). Int. J. Plant Sci. 156, 172–180. doi: 10.1086/297238
Kosuge, K. (1994). Petal evolution in Ranunculaceae. Plant Syst. Evol. Suppl. 8, 185–191. doi: 10.1007/978-3-7091-6910-0_11
Kubitzki, K. (2007). “Haloragaceae,” in The Families And Genera Of Vascular Plants Volume IX Flowering Plants. Eudicots Berberidopsidales, Buxales, Crossosomatales, Fabales p.p., Geraniales, Gunnerales, Myrtales p.p., Proteales, Saxifragales, Vitales, Zygophyllales, Clusiaceae Alliance, Passifloraceae Alliance, Dilleniaceae, Huaceae, Picramniaceae, Sabiaceae, ed. K. Kubitzki (London: Springer), 184–190.
Lanjouw, J. (1932). Contributions to the flora of tropical America: XI. Bull. Miscellan. Inform. 1932:183. doi: 10.2307/4118526
Leins, P., and Erbar, C. (1985). Ein beitrag zur blütenentwicklung der aristolochiaceen, einer vermittlergruppe zu den monokotylen. Bot. Jahrb. Syst. 107, 343–368.
Leins, P., and Erbar, C. (1994). Flowers in Magnoliidae and the origin of flowers in other subclasses of the angiosperms II. The relationships between flowers of Magnoliidae, Dilleniidae, and Caryophyllidae. Plant Syst. Evol. Suppl. 8, 209–218. doi: 10.1007/978-3-7091-6910-0_13
Li, P., and Johnston, M. O. (2000). Heterochrony in plant evolutionary studies through the twentieth century. Bot. Rev. 66, 57–88. doi: 10.1007/BF02857782
Litt, A., and Kramer, E. M. (2010). The ABC model and the diversification of floral organ identity. Semin. Cell Dev. Biol. 21, 129–137. doi: 10.1016/j.semcdb.2009.11.019
Liu, H., Lin, S., and Liao, J. (2015). Floral ontogeny of two Jatropha species (Euphorbiaceae s.s.) and its systematic implications. Pakist. J. Bot. 47, 959–965.
Liu, H.-F., Deng, Y.-F., and Liao, J.-P. (2008). Floral organogenesis of three species of Jatropha (Euphorbiaceae). J. Syst. Evol. 46, 53–61.
Mao, Y., Liu, W., Chen, X., Xu, Y., Lu, W., Hou, J., et al. (2017). Flower development and sex determination between male and female flowers in Vernicia fordii. Front. Plant Sci. 8:1291. doi: 10.3389/fpls.2017.01291
Michaelis, P. (1924). Blütenmorphologische Untersuchungen an den euphorbiaceen, unter besonderer berücksichtigung der phylogenie der angiospermenblüte. Bot. Abhandlungen 3:150.
Nair, N. C., and Abraham, V. (1962). Floral morphology of a few species of Euphorbiaceae. Proc. Indian Acad. Sci. Sect. B 56, 1–12.
Narbona, E., and Dirzo, R. (2010). A reassessment of the function of floral nectar in Croton suberosus (Euphorbiaceae): a reward for plant defenders and pollinators. Am. J. Bot. 97, 672–679. doi: 10.3732/ajb.0900259
Novo, R. R., Souza, J. T., and Cardoso de Castro, C. (2010). First report of predation on floral visitors by crab spiders on Croton selowii Baill (Euphorbiaceae). Acta Bot. Brasil. 24, 592–594. doi: 10.1590/S0102-33062010000200029
Ojeda, D. I., Valido, A., Fernández de Castro, A. G., Ortega-Olivencia, A., Fuertes-Aguilar, J., Carvalho, J. A., et al. (2016). Pollinator shifts drive petal epidermal evolution on the Macaronesian Islands bird-flowered species. Biol. Lett. 12:20160022. doi: 10.1098/rsbl.2016.0022
Pires, M. M. Y., de Souza, L. A., and Terada, Y. (2004). Biologia floral de Croton urucurana Baill (Euphorbiaceae) ocorrente em vegetação ripária da ilha Porto Rico, Porto Rico, Estado do Paraná, Brasil. Acta Scientiar. Biol. Sci. 26, 209–215. doi: 10.4025/actascibiolsci.v26i2.1638
Prenner, G., and Rudall, P. J. (2007). Comparative ontogeny of the cyathium in Euphorbia (Euphorbiaceae) and its allies: exploring the organ, flower, inflorescence boundary. Am. J. Bot. 94, 1612–1629. doi: 10.3732/ajb.94.10.1612
Remizowa, M. V., Sokoloff, D. D., and Rudall, P. J. (2010). Evolutionary history of the monocot flower. Ann. Missouri Bot. Garden 97, 617–645. doi: 10.3417/2009142
Riina, R., Berry, P. E., and van Ee, B. W. (2009). Molecular phylogenetics of the dragon’s blood Croton section Cyclostigma (Euphorbiaceae): a polyphyletic assemblage unraveled. Syst. Bot. 34, 360–374. doi: 10.1600/036364409788606415
Rijpkema, A. S., Vandenbussche, M., Koes, R., Heijmans, K., and Gerats, T. (2010). Variations on a theme: changes in the floral ABCs in angiosperms. Semin. Cell Dev. Biol. 21, 100–107. doi: 10.1016/j.semcdb.2009.11.002
Ronse De Craene, L. (2018). Understanding the role of floral development in the evolution of angiosperm flowers: clarifications from a historical and physico-dynamic perspective. J. Plant Res. 131, 367–393. doi: 10.1007/s10265-018-1021-1
Ronse De Craene, L., and Bull-Hereñu, K. (2016). Obdiplostemony: the occurrence of a transitional stage linking robust flower configurations. Ann. Bot. 117, 709–724. doi: 10.1093/aob/mcw017
Ronse De Craene, L. P. (2007). Are petals sterile stamens or bracts? The origin and evolution of petals in the core eudicots. Ann. Bot. 100, 621–630. doi: 10.1093/aob/mcm076
Ronse De Craene, L. P. (2008). Homology and evolution of petals in the core eudicots. Syst. Bot. 33, 301–325. doi: 10.1600/036364408784571680
Ronse De Craene, L. P. (2010). Floral Diagrams: An Aid To Understanding Flower Morphology And Evolution. Cambridge: Cambridge University Press.
Ronse De Craene, L. P. (2016). “Montiniaceae,” in The Families and Genera of Vascular Plants, Volume 14 Flowering Plants: Eudicots - Aquifoliales, Boraginales, Bruniales, Dipsacales, Escalloniales, Garryales, Paracryphiales, Solanales (except Convolvulaceae), Icacinaceae, Metteniusaceae, Vahliaceae, eds J. W. Kadereit, V. Bittrich, and K. Kubitzki (London: Springer), 269–274. doi: 10.1007/978-3-319-28534-4_24
Ronse De Craene, L. P. (2017). Floral development of the endangered genus Medusagyne (Medusagynaceae-Malpighiales): Spatial constraints of stamen and carpel increase. Int. J. Plant Sci. 178, 639–649. doi: 10.1086/692989
Ronse De Craene, L. P., and Brockington, S. F. (2013). Origin and evolution of petals in angiosperms. Pl. Ecol. Evol. 146, 5–25. doi: 10.5091/plecevo.2013.738
Ronse De Craene, L. P., Laet, J. D., and Smets, E. E. (1996). Morphological Studies in Zygophyllaceae. II. The floral development and vascular anatomy of Peganum harmala. Am. J. Bot. 83, 201–215. doi: 10.1002/j.1537-2197.1996.tb12698.x
Ronse de Craene, L. P., and Smets, E. (1991a). Androecium and floral nectaries of Harungana madagascariensis (Clusiaceae). Plant Syst. Evol. 178, 179–194.
Ronse de Craene, L. P., and Smets, E. (1991b). Morphological studies in Zygophyllaceae I. The floral development and vascular anatomy of Nitraria retusa. Am. J. Bot. 78, 1438–1448. doi: 10.1002/j.1537-2197.1991.tb12610.x
Ronse De Craene, L. P., and Smets, E. (1992). Complex polyandry in the Magnoliatae: definition, distribution and systematic value. Nordic J. Bot. 12, 621–649. doi: 10.1111/j.1756-1051.1992.tb01839.x
Ronse De Craene, L. P., and Smets, E. F. (2001). Staminodes: their morphological and evolutionary significance. Bot. Rev. 67, 351–402. doi: 10.1007/BF02858099
Ronse De Craene, L. P., and Wanntorp, L. (2006). Evolution of floral characters in Gunnera (Gunneraceae). Syst. Bot. 31, 671–688. doi: 10.1600/036364406779695951
Rudall, P. J. (2010). All in a spin: centrifugal organ formation and floral patterning. Curr. Opin. Plant Biol. 13, 108–114. doi: 10.1016/j.pbi.2009.09.019
Rudall, P. J. (2011). “Centrifugal stamens in a modern phylogenetic context: was Corner right?,” in Flowers on The Tree of Life, eds L. Wanntorp and L. P. Ronse De Craene (Cambridge: Cambridge University Press), 142–155. doi: 10.1017/CBO9781139013321.006
Schultes, R. E. (1955). A new generic concept in the Euphorbiaceae. Bot. Museum Leaf. Harv. Univer. 17, 27–36.
Secco, R. D. S. (1987). Aspectos sistemáticos e evolutivos do gênero Sandwithia Lanj (Euphorbiaceae) em relação às suas afinidades. Boletim do Museu Paraense Emilio Goeldi Ser. Bot. 3, 157–181.
Silva, O. L. M., Riina, R., and Cordeiro, I. (2020). Phylogeny and biogeography of Astraea with new insights into the evolutionary history of Crotoneae (Euphorbiaceae). Mol. Phylogenet. Evol. 145:106738. doi: 10.1016/j.ympev.2020.106738
Smyth, D. R., Bowman, J. L., and Meyerowitz, E. M. (1990). Early flower development in Arabidopsis. Plant Cell 2:755. doi: 10.2307/3869174
Soltis, D. E., Soltis, P. S., Endress, P. K., Chase, M. W., Manchester, S. R., Judd, W. S., et al. (2018). Phylogeny and Evolution Of The Angiosperms. Revised And Updated Edition. London: The university of chicago press.
Soltis, P. S., Brockington, S. F., Yoo, M.-J., Piedrahita, A., Latvis, M., Moore, M. J., et al. (2009). Floral variation and floral genetics in basal angiosperms. Am. J. Bot. 96, 110–128. doi: 10.3732/ajb.0800182
Takhtajan, A. (1991). Evolutionary Trends In Flowering Plants. New York, NY: Columbia University Press.
The Angiosperm Phylogeny Group (2016). An update of the angiosperm phylogeny group classification for the orders and families of flowering plants: APG IV. Bot. J. Linnean Soc. 181, 1–20. doi: 10.1111/boj.12385
Tucker, S. C., and Bernhardt, P. (2000). Floral ontogeny, pattern formation, and evolution in Hibbertia and Adrastaea (Dilleniaceae). Am. J. Bot. 87, 1915–1936. doi: 10.2307/2656843
Uhl, N. W., and Moore, H. E. (1977). Centrifugal stamen initiation in Phytelephantoid palms. Am. J. Bot. 64, 1152–1161. doi: 10.1002/j.1537-2197.1977.tb10805.x
van Ee, B. W., and Berry, P. E. (2009). A phylogenetic and taxonomic review of Croton (Euphorbiaceae S.S.) on Jamaica including the description of Croton jamaicensis, a new species of section Eluteria. Syst. Bot. 34, 129–140. doi: 10.1600/036364409787602203
van Ee, B. W., Berry, P. E., Riina, R., and Amaro, J. E. G. (2008). Molecular phylogenetics and biogeography of the caribbean-centered Croton subgenus Moacroton (Euphorbiaceae s.s.). Bot. Rev. 74, 132–165. doi: 10.1007/s12229-008-9003-y
van Ee, B. W., Forster, P. I., and Berry, P. E. (2015). Phylogenetic relationships and a new sectional classification of Croton (Euphorbiaceae) in Australia. Austr. Syst. Bot. 28:219. doi: 10.1071/SB15016
van Ee, B. W., Jelinski, N., Berry, P. E., and Hipp, A. L. (2006). Phylogeny and biogeography of Croton alabamensis (Euphorbiaceae), a rare shrub from Texas and Alabama, using DNA sequence and AFLP data. Mol. Ecol. 15, 2735–2751. doi: 10.1111/j.1365-294X.2006.02970.x
van Ee, B. W., Riina, R., and Berry, P. E. (2011). A revised infrageneric classification and molecular phylogeny of New World Croton (Euphorbiaceae). Taxon 60, 791–823. doi: 10.1002/tax.603013
van Welzen, P. C., Guerrero, S. A., Arifiani, D., Bangun, T. J. F., Bouman, R. W., Eurlings, M. C. M., et al. (2020). Weda, a new genus with two new species of Euphorbiaceae-Crotonoideae from Halmahera (North Maluku, Indonesia) and phylogenetic relationships of the Australasian tribe Ricinocarpeae. J. Syst. Evol. (in press). doi: 10.1111/jse.12581
Vanvinckenroye, P. F., Ronse De Craene, L. P., and Smets, E. F. (1997). The floral development of Monococcus echinophorus (Phytolaccaceae). Can. J. Bot. 75, 1941–1950. doi: 10.1139/b97-906
Vasconcelos, T. N. C., Prenner, G., Santos, M. F., Wingler, A., and Lucas, E. J. (2017). Links between parallel evolution and systematic complexity in angiosperms—A case study of floral development in Myrcia s.l. (Myrtaceae). Perspect. Plant Ecol. Evol. Syst. 24, 11–24. doi: 10.1016/j.ppees.2016.11.001
Velloso, J. M. D. C. (1798). “Joannesia,” in Alographia Dos Alkalis Fixos, ed. S. T. Ferreira (Lisbon: Na offc. de simão thaddeo ferreira), 199–201.
Venkata-Rao, C., and Ramalakshmi, T. (1968). Floral anatomy of the Euphorbiaceae-I. Some non-Cyathium taxa. J. Indian Bot. Soc. 47, 278–300.
Webster, G. L. (1993). A provisional synopsis of the sections of the genus Croton (Euphorbiaceae). Taxon 42, 793–823. doi: 10.2307/1223265
Webster, G. L. (2014). “Euphorbiaceae,” in The Families and Genera Of Vascular Plants Volume XI. Flowering plants. Eudicots: Malpighiales, ed. K. Kubitzki (London: Springer), 51–216.
Whitney, H. M., Bennett, K. M. V., Dorling, M., Sandbach, L., Prince, D., Chittka, L., et al. (2011). Why do so many petals have conical epidermal cells? Ann. Bot. 108, 609–616. doi: 10.1093/aob/mcr065
Keywords: bracteopetal, dimorphism, filamentous structure, heterochrony, nectary, petal reduction, spatial constraints, stamen development
Citation: Thaowetsuwan P, Ritchie S, Riina R and Ronse De Craene L (2020) Divergent Developmental Pathways Among Staminate and Pistillate Flowers of Some Unusual Croton (Euphorbiaceae). Front. Ecol. Evol. 8:253. doi: 10.3389/fevo.2020.00253
Received: 06 January 2020; Accepted: 13 July 2020;
Published: 04 August 2020.
Edited by:
Alessandro Minelli, University of Padua, ItalyReviewed by:
Paula Rudall, Royal Botanic Gardens, Kew, United KingdomAna Maria Rocha De Almeida, California State University, East Bay, United States
Vidal De Freitas Mansano, Rio de Janeiro Botanical Garden, Brazil
Copyright © 2020 Thaowetsuwan, Ritchie, Riina and Ronse De Craene. This is an open-access article distributed under the terms of the Creative Commons Attribution License (CC BY). The use, distribution or reproduction in other forums is permitted, provided the original author(s) and the copyright owner(s) are credited and that the original publication in this journal is cited, in accordance with accepted academic practice. No use, distribution or reproduction is permitted which does not comply with these terms.
*Correspondence: Louis Ronse De Craene, bHJvbnNlZGVjcmFlbmVAcmJnZS5vcmcudWs=