- 1Department of Forestry and Natural Resources, University of Kentucky College of Agriculture, Food and Environment, Lexington, KY, United States
- 2Weyerhaeuser Company, Columbus, MS, United States
- 3Department of Wildlife Ecology, University of Florida Institute of Food and Agricultural Sciences, Gainesville, FL, United States
Human activities are changing the environments that animal communities utilize, altering competition and co-existence dynamics as well as changing species distributions. A global consequence of human activity is increased woody cover, which changes environmental conditions on landscape, local, and fine spatial scales. These multi-scale processes are likely to change species interactions and distributions in unexpected ways. To elucidate these effects, we investigated the co-occurrence of two interacting species in a rapidly changing ecosystem. Eastern fox (Sciurus niger) and gray (Sciurus carolinensis) squirrels occur sympatrically throughout the southeastern United States. We used single-season, two-species occupancy modeling to understand what factors influence competition between the squirrels at three spatial scales. We found evidence that fox and gray squirrels compete at fine scales but not at local and landscape scales. The best model to explain fox and gray squirrel co-occurrence at fine scales was an additive fine-scale understory and tree canopy closure model. Increased fine-scale canopy closure correlated with increased fox and gray squirrel occupancy, while increased fine-scale understory cover correlated with decreased gray and fox squirrel occupancy. Fox squirrel occupancy probability declined in the presence of gray squirrels. The intensity of interaction was modified by fine-scale canopy cover and understory density. These findings support the hypothesis that species interactions can be an important factor in structuring biotic communities; however, the strength of the effect changes across spatial scales. Our results support the importance of considering species interactions at multiple scales when predicting and addressing changes in community composition in human modified and managed systems.
Introduction
Human activities are changing the environments that animal communities utilize (Lewis and Maslin, 2015). These activities can alter species interactions and co-existence dynamics and change species distributions and community composition (Lyons et al., 2016). Changes in community composition are likely to be pronounced for ecologically similar species that often co-occur through specializations on multiple dimensions which result in multi-scale “trade-offs” (Kneitel and Chase, 2004). The benefits of ecological specialization at one scale often come with fitness costs at other scales (Fry, 2003; Bonsall et al., 2004). For example, local competitive advantage may be coupled with reductions in a species’ ability to colonize new sites across a landscape (Cadotte, 2007). It is hypothesized that these multi-scale and dimensional competitive advantages allow similar species to co-occur (Kneitel and Chase, 2004). Nevertheless, the mechanism by which common, human-driven environmental changes alter how species interact at multiple scales is poorly understood (Tews et al., 2004).
Habitat loss and fragmentation, two major features of anthropogenic environmental change, are typically assessed at landscape spatial scales (Fischer and Lindenmayer, 2007). However, humans also change environments at more localized scales (Wilbanks and Kates, 1999), such as by suppressing fire or altering the number of trees within a forest patch through forest management. On a landscape, increased habitat edge from fragmentation may favor species that are poor competitors but can cross the matrix separating patches (Marvier et al., 2004). However, on the local level, fragmentation may favor species that are superior competitors, especially when resources are scarce (Ditchkoff et al., 2006). Understanding these patterns is critical to predicting how human activities will alter ecological communities.
One global environmental change that may be altering how species interact is woody plant encroachment hereinafter, woody encroachment (Eldridge et al., 2011; Stanton et al., 2018). Globally, grassy and savanna biomes are changing as woody species increase understory density and close canopies (Spector and Putz, 2006). Anthropogenic induced changes including fire suppression, increased atmospheric carbon, and unmanaged livestock grazing contribute to this environmental change (Roques et al., 2001; D’Odorico et al., 2012). Areas impacted by woody encroachment experience changes in species composition, such as open savanna specialists being replaced by generalists (Sirami and Monadjem, 2012). Woody encroachment can lead to increased homogeneity on landscape scales (Anthelme et al., 2007), while increasing structural complexity at fine scales (Sirami et al., 2009). Further, the physical characteristics of woody encroached areas may differ depending on successional conditions, with early stages exemplified by heightened understory cover and later stages defined by closed canopies with little to no understory (Van Auken and Bush, 2013).
To better understand how woody encroachment and habitat fragmentation alter species interactions on multiple scales, we investigated the co-occurrence of two sympatric species in a rapidly changing savanna ecosystem: the eastern fox (Sciurus niger) and gray (Sciurus carolinensis) squirrels. These two species have overlapping distributions throughout most of the southeastern United States. The historic pine savannas of this region are changing as hardwoods, especially oaks (Quercus spp.), have begun to dominate the understory and midstory, causing the once open canopy to close (Provencher et al., 2001; Freeman and Jose, 2009; Platt et al., 2015). Fox squirrels appear to be sensitive to competition and gray squirrels may be replacing fox squirrels in some parts of their range due to their competitive advantage in closed canopy systems (Brown and Batzli, 1985; Sexton, 1990; Sovie, 2019). Fox and gray squirrels partition resources but the scale, how they partition resources over time and space, and the intensity of interactions may change over ecological gradients (Sexton, 1990; Edwards et al., 1998; Derge and Yahner, 2000; Van Der Merwe et al., 2005; Sovie et al., 2019). Moreover, these ecological gradients and partitioning of resources are likely being altered by fragmented landscapes and woody encroachment.
Our objective was to identify if anthropogenically altered environmental conditions influence fox and gray squirrel co-occurrence across scales. Co-occurrence and conditional occupancy (i.e., the probability of a species occupying a site given the presence of another species) can be thought of as a measure of competition (Gotelli and McCabe, 2002; Ovaskainen et al., 2010; Richmond et al., 2010; Sebastian-Gonzalez et al., 2010). If a species is less likely to occur in the presence of another species, that may be a signal of competitive exclusion (Richmond et al., 2010).
We investigated squirrel co-occurrence at three biologically relevant spatial scales. In line with some ecological theories (Pearson and Dawson, 2004), we predict that species interactions are not an important driver of squirrel distributions at landscape scales. However, we predict that fox and gray squirrels interact in localized areas and their interactions may be influenced by landscape scale processes that regulate path connectivity and dispersal (Goheen et al., 2003). Finally, we predict that interactions between the squirrels may be moderated by fine-scale vegetation characteristics that increase predation risk (minimal understory and canopy cover) and decrease gray squirrel foraging advantage (Bowers et al., 1993; Van Der Merwe et al., 2005).
Materials and Methods
Study Area
To investigate fox and gray squirrel co-occurrence, we conducted field surveys throughout North and Central Florida in 2012 and 2013. The region had a humid, sub-tropical climate, with warm wet summers (May–October: average high of 35°C and 121 cm of rain) and dry, mild winters (November–April: average high of 25°C and 25 cm of rain) (Hagemeyer et al., 2010). Vegetation communities across our study sites included open grasslands, pine-dominated forests, pine hardwoods, hardwood hammocks, bottomland hardwood forests, and pine clear cuts. Canopy trees commonly included longleaf (Pinus palustris), slash (Pinus elliottii), and loblolly (Pinus taeda) pines, and turkey (Quercus laevis), live (Quercus virginiana), laurel (Quercus laurifolia), and water (Quercus nigra) oaks.
Study Design
Squirrels select habitat on multiple scales, and variables that influence selection on one scale may not have predictive power at other scales (Johnson, 1980; Greene and McCleery, 2017a). Selection on smaller scales may be dependent on processes occurring at larger scales (McGregor et al., 2014). To assess the influence of environmental features on the co-occurrence of gray and fox squirrels, we surveyed using a nested (hierarchical) design at three spatial scales: fine (0.01 ha), local (5.3 ha), and landscape (765 ha) (Figure 1; Greene and McCleery, 2017a).
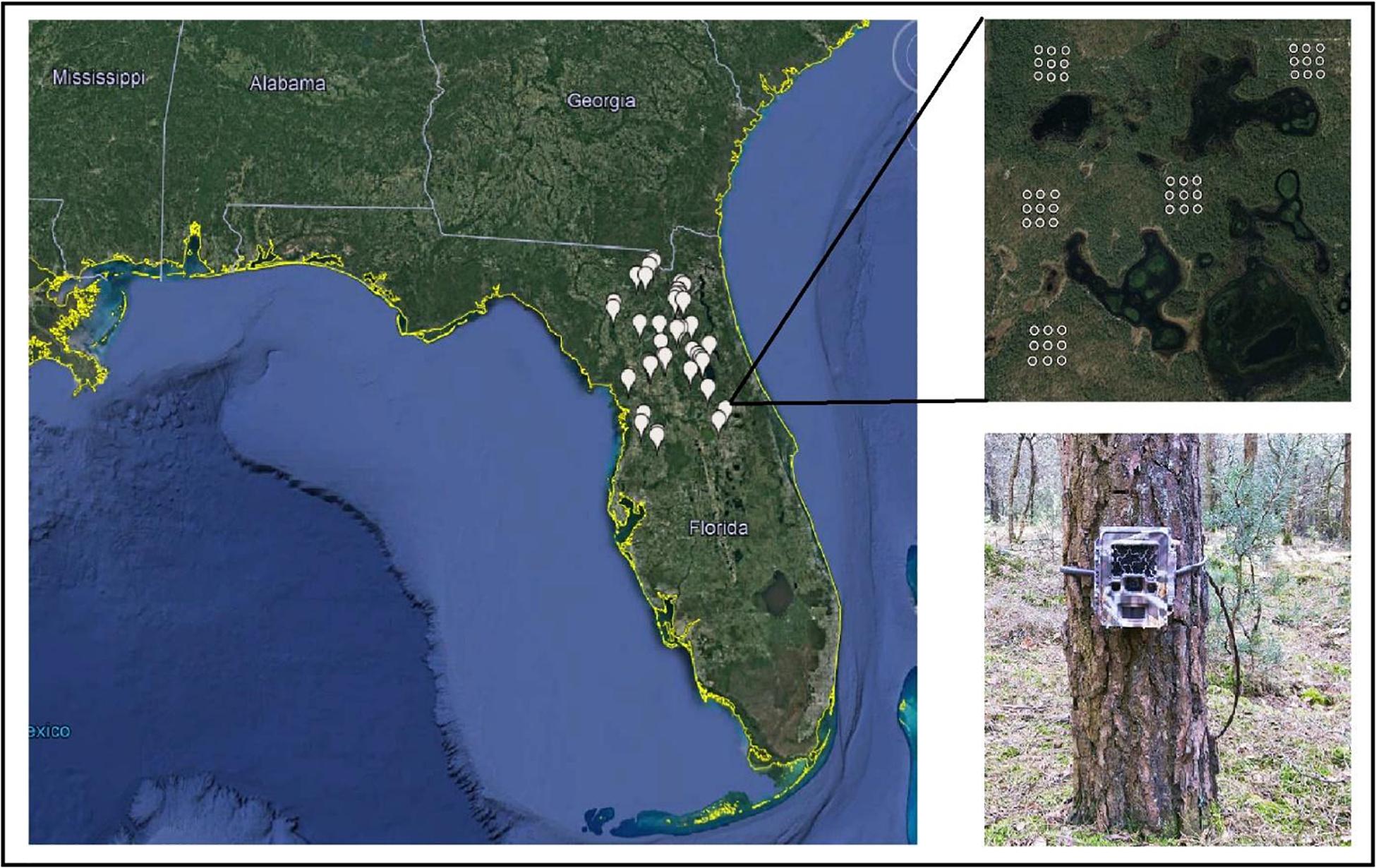
Figure 1. The distribution of the 40 landscapes, local girds, and camera points (fine scale) surveyed in a multi-scaled study of habitat use and selection of fox squirrels and gray squirrels in North and Central Florida, United States, 2013–2014.
To represent the landscape scale, we placed 40 7.65 km2 blocks throughout North and Central Florida using ArcGIS 10.1 (ESRI, Redlands, CA, United States). We selected 10 landscapes in upland pine or sandhills, 10 in mesic/shrubby flatwoods, and 20 landscapes randomly without regard to a land cover type. To represent the local scale, we randomly placed five 5.3 ha grids within each landscape. To maximize grid independence and to reduce spatial autocorrelation, we separated grids by ≥500 m; the pooled mean maximum distance moved from southeastern fox squirrel trapping and radio-telemetry studies (Greene and McCleery, 2017b). To study fine-scale occupancy, we placed nine camera traps within each grid as a 3 × 3 array with 115 m spacing between points.
We surveyed for each species using camera traps, a reliable method to survey squirrels (Greene et al., 2016; Greene and McCleery, 2017b). At each point, we placed a game camera (Bushnell Trophy Cam model 119436, Bushnell Outdoor Products, Overland Park, KS, United States) 50 cm above the ground and angled toward a bait pile of pecans and cracked corn. We set cameras to take three photos every time the camera was triggered using the camera’s “normal” sensitivity setting. We placed camera traps within a landscape and grid concurrently and allowed cameras to collect data for <10 days. We conducted camera trapping from 01 January to 01 July in 2013 and 2014 and surveyed during this time because temperatures are mild and squirrels are more active (Moore, 1957; Weigl et al., 1989). See Greene and McCleery (2017a) for more details on the camera trapping protocol.
Environmental Measurements
We measured fine, local, and landscape scale variables hypothesized to affect squirrel occupancy, detection, and co-occurrence. Non-random co-occurrence may result from species-specific habitat preference, and thus, we controlled for species-specific habitat preference by measuring variables known to influence fox and gray squirrel occupancy. These variables included pine and oak density, understory cover, canopy closure, and canopy heterogeneity (Cottam and Curtis, 1956; Conner et al., 1999; Boone et al., 2017; Greene and McCleery, 2017a). We hypothesized that structural elements such as canopy cover, canopy cover heterogeneity, understory cover, and local and landscape fragmentation and connectivity mitigate squirrel interactions due to the ability of each species to move about the matrix differently (Goheen et al., 2003). Finally, we measured variables that we expected to influence the probability of detecting fox and gray squirrels. Specifically, these included visual obstruction that may interfere with the cameras field of vision and variation in camera trapping effort across the grid and landscape.
Fine scale
Interactions between fox and gray squirrels appear to be the most pronounced at fine scales, which includes interactions on individual trees (Conner et al., 1999). Fox squirrels will change their temporal behavior if they overlap with gray squirrels at individual cameras (Sovie et al., 2019). The competitive advantage of gray squirrels may be moderated by fine-scale vegetation characteristics that increase predation risk such as minimal understory and canopy cover (Caswell, 1978). Fox squirrels may use these risky areas and avoid antagonistic interactions with gray squirrels (Brown, 1999; Steele et al., 2015). Therefore, at each fine-scale site, we measured canopy closure using a concave spherical densiometer (Lemmon, 1956; Forestry Suppliers, Inc., Model-C). To capture variation in woody encroachment, we measured understory cover using the line-intercept method (Canfield, 1941; Greene and McCleery, 2017a). Finally, we measured visual obstruction that may interfere with the cameras field of vision (<45 cm in height) in five, 1/2 m × 1/2 m quadrats (Daubenmire, 1959; Greene and McCleery, 2017a).
Local scale
Fox and gray squirrels both respond to localized (∼1–4 ha) forest structure, specifically canopy and understory cover (Brown and Batzli, 1985; Greene and McCleery, 2017a). Gray squirrels occupy areas with elevated understory cover, closed canopy cover, or dense hardwoods (Brown and Batzli, 1985). Fox squirrels appear to avoid these areas but will enter them in the absence of gray squirrels (Sovie, 2019). Increased canopy and understory cover on landscape and local scales may deter squirrel predators (Potash et al., 2019), allowing gray squirrels to proliferate and out compete fox squirrels. How fox and gray squirrels interact in localized areas may also be influenced by the degree of canopy cover fragmentation or connectivity (Zollner, 2000; Goheen et al., 2003). To measure local environmental characteristics, we used the U.S. Forest Service Tree Canopy analytical layer (Jin et al., 2013) from the 2011 National Land Cover Database. We estimated the average percentage of canopy cover at each grid from 30 × 30 m pixels. Southeastern fox squirrels generally occupy areas with non-overlapping canopies typical of older, fire maintained pine stands (Perkins et al., 2008), and thus, we defined each 30 × 30 cell as either closed (>50% canopy cover) or open (<50% canopy cover) and calculated patch metrics for closed patches. We calculated mean patch size and patch density as measures of fragmentation and used the area-weighted mean patch radius of gyration as a measure of connectivity (McGarigal, 2014). We calculated patch metrics using the calculate_lsm function in the landscapemetrics package (Hesselbarth et al., 2019) in R version 3.6.1 (R Core Team, 2019). To estimate local measures of understory cover, we used the enhanced vegetation index (EVI) from March 22, 2013, from the MODIS satellite recording (Didan, 2015). EVI is remote-sensed measure of biomass that corrects for canopy background noise and is sensitive in areas with dense vegetation (Singh et al., 2015).
Landscape scale
We examined squirrel interactions at the landscape scale even though some ecological theories suggest that environmental gradients, not biotic interactions, should drive species distribution at larger spatial scales (Pearson and Dawson, 2004). However, it is possible that species compete across landscapes (Araújo and Luoto, 2007). Specifically, fragmentation generally favors species with higher dispersal ability (Cote et al., 2017). Fragmentation and heterogeneity may increase the fox squirrels’ competitive advantage at the landscape scale, specifically when habitat characteristics mimic savanna-like conditions (Greene and McCleery, 2017a). Gray squirrels are generally found on homogeneous landscapes with large forest patches proximate to each other (Moore and Swihart, 2005). Landscape characteristics that act as barriers to gray squirrels, such as agricultural fields (Goheen et al., 2003), may diminish their competitive advantage and facilitate coexistence on large spatial scales. In contrast, processes like hardwood encroachment may increase landscape homogeneity and favor gray squirrels. Therefore, we calculated mean canopy cover, understory cover, mean patch size, patch density, and mean gyration for each 7.65 km2 landscape using the methods described for local scale metrics.
Data Analysis
We elected to investigate fox and gray squirrel interactions using multi-species occupancy models (Rota et al., 2016). Multi-species occupancy models can reveal evidence of interactions between species that are imperfectly detected and from data that cannot reliably produce density estimates (Gotelli and McCabe, 2002; Ovaskainen et al., 2010; Richmond et al., 2010; Sebastian-Gonzalez et al., 2010). We converted raw camera trap data into encounter histories (detected or not) for each species and scale. We defined sites and surveys differently between scales. Starting with the fine scale, we considered each camera trap point (N = 1,800) as a site, and each 24-h period the camera was active a survey (≤10 days). For the local scale, we considered each grid as a site (N = 200) and each camera point as a survey [9 surveys (i.e., points per grid)]. For the landscape scale, we considered each 7.65 km2 block as a site (N = 40) and the local scale grids within each landscape as a survey (N = 5).
We analyzed squirrel occupancy in R version 3.6.1 (R Core Team, 2019) using the package unmarked (Fiske et al., 2015). We used the Rota et al. (2016) single season, two-species occupancy model. In our parameterization, we estimated the probability of: (1) occupancy of fox squirrels (ΨA), (2) occupancy of gray squirrels (ΨB), (3) occupancy of fox squirrels given gray squirrels are present (ΨA:B), (4) occupancy of gray squirrels given fox squirrels are present (ΨB:A), (5) detecting fox squirrels (pA), and (6) detecting gray squirrels (pB). The Rota et al. (2016) parametrization allows for stable model behavior and the incorporation of covariates; however, it does not a priori assume one species is dominant over the other.
To identify if environmental variables influence how gray and fox squirrels interact on different scales, we were primarily interested in the conditional occupancy parameters (ΨA:B and ΨB:A). Nevertheless, we wished to control for detection and independent habitat associations [(pA), (pB), (ΨA) (ΨB)]. To determine the best models for (pA), (pB), and (ΨA) (ΨB), we undertook a sequential modeling process (Supplementary Table S1). Using the best predictors for (pA), (pB), and (ΨA) (ΨB), we then built models to test how woody encroachment, fragmentation, and habitat connectivity influence squirrel interaction (ΨA:B and ΨB:A). Depending on the stage of succession, woody encroached sites may have elevated understory cover (as measured by line-intercept or EVI), or increased canopy closure (as measured by concave densiometer or canopy cover), or both (high understory and canopy closure) (Table 1; Van Auken and Bush, 2013). We measured fragmentation as the mean patch density of closed canopy forest at grid and landscape scales and connectivity as the mean gyration at grid and landscape scales. We normalized habitat variables using a log + 1 transformation to improve model convergence. For additive models, we examined the relationships between our covariates at each scale and dropped 1 of the pair if their Pearson’s correlation coefficient was >| 0.70|. We allowed hypothesized drivers to influence squirrel occupancy across scales. For example, gray squirrels may use low canopy closure fine-scale areas and exclude fox squirrels if the broader environment has high canopy cover and supports gray squirrels (e.g., tree fall gaps) (Dill and Houtman, 1989; Van Der Merwe et al., 2005). Further, fox squirrels may use fine-scale areas with high canopy cover within highly heterogenous landscapes where gray squirrels do not usually occur (e.g., within pine savannas). Finally, we considered models that did not incorporate interaction (i.e., ΨA:B = 0) or that treated species interaction as independent from environmental conditions (i.e., constant ΨA:B = 1). We ranked models using AIC and model weight (Burnham and Anderson, 2002). We considered models within 2 AIC units of the top model competing models. We inspected the 95% CIs around the parameter estimates and β coefficients of competing models to see if the CIs crossed 0. If competing models differed from the best model by only one parameter and the CI of that parameter crossed 0, we based our inference off the simplest model (Burnham and Anderson, 2002).
Results
At the fine scale, we successfully surveyed 1,737 sites. We detected gray squirrels at 270, fox squirrels at 217, and both at 17 points. At the local (grid) scale, gray squirrels were present at 86 of 200 sites, fox squirrels at 74 sites, and both at 23 sites. At the landscape scale, we detected gray squirrels on 38 of 40 landscapes, fox squirrels on 26 sites, and both on 20 sites. Our samples captured the environmental gradient in our system (Supplementary Table S1). We found that tree canopy and understory cover were weakly positively correlated at all scales (Fine = 0.27, p < 0.01; Grid = 0.28, p < 0.01; Landscape = 0.20, p < 0.01).
The best model to explain fox and gray squirrel fine-scale co-occurrence indicated that fox squirrels were less likely to occur at points occupied by gray squirrels. This interaction was moderated by fine-scale canopy cover and understory density (ΨA:B βFine canopy cover = −6.25, SE = 0.79; βFine understory density = 3.19, SE = 0.54). Fox squirrel occupancy increased with fine-scale canopy closure in the absence of gray squirrels and decreased with fine-scale canopy closure in the presence of gray squirrels (Figure 2A). Fox squirrel occupancy remained stable with increasing understory cover in the presence of gray squirrels and declined with increased fine-scale understory cover in the absence of gray squirrels (Figure 2B). We did not detect a change in gray squirrel conditional occupancy in the presence of fox squirrels (Supplementary Figure S1). Increased fine-scale canopy closure correlated with increased fox squirrel occupancy and increased fine-scale understory cover correlated with decreased fox squirrel occupancy (ΨA βFine canopy cover = 0.60, SE = 0.08; βFine understory density = −3.30, SE = 0.36). Increased fine-scale canopy closure correlated with increased gray squirrel occupancy and increased fine-scale understory cover correlated with decreased gray squirrel occupancy (ΨA βFine canopy cover = 5.91, SE = 0.76; βFine understory density = −0.12, SE = 0.36). Co-occurrence for all models at fine-scales included obstruction decreasing p for both species [pA βObstruction = −0.29, SE = 0.30; pB βObstruction = −2.18, SE = 0.31].
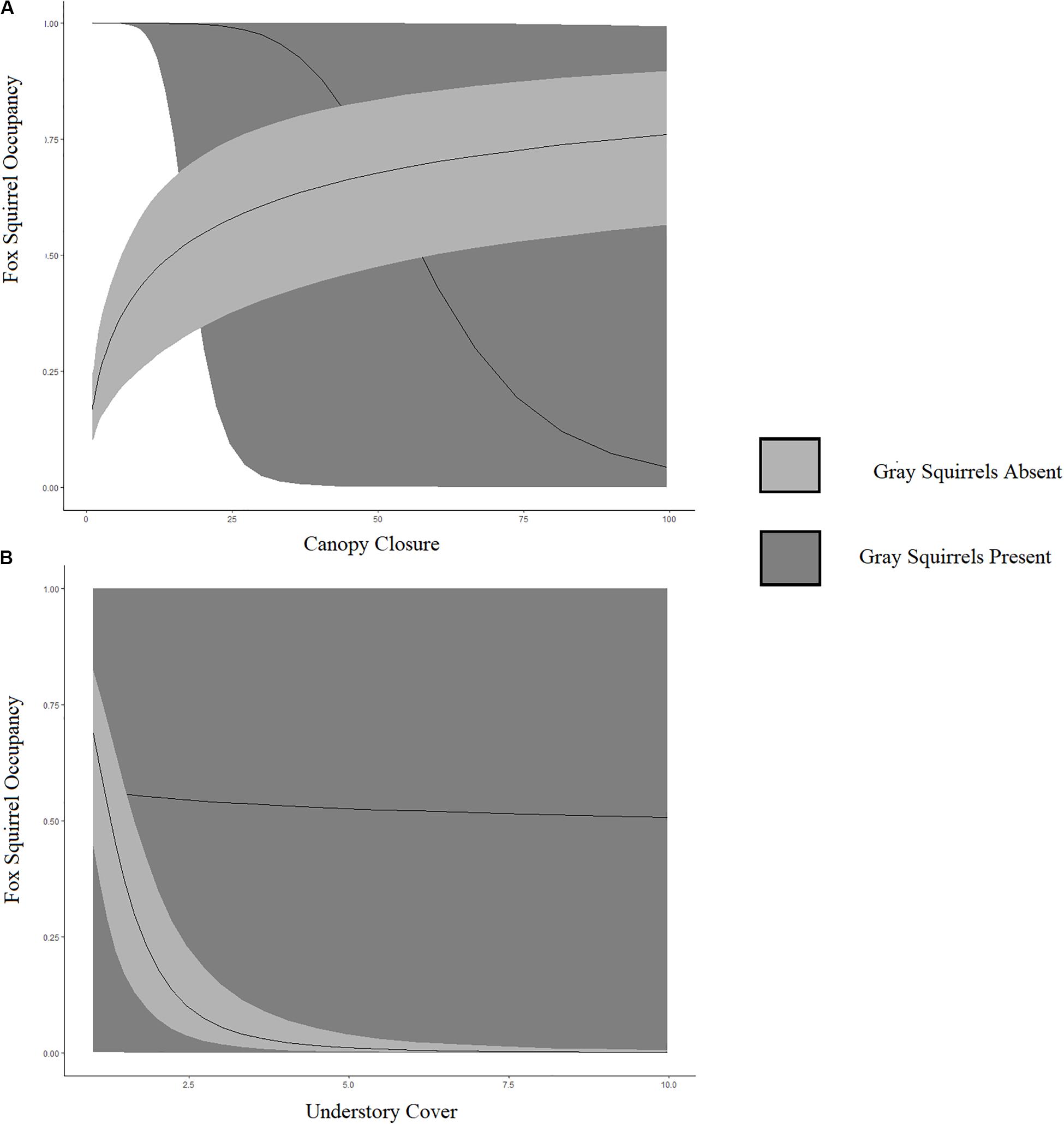
Figure 2. Relationship between fox squirrel occupancy probability and fine-scale canopy closure (A) and undercover cover (B), given gray squirrels are absent (light gray) or present (dark gray) in fine-scale sites located in North and Central Florida, United States, in 2013–2014. (A) Fox squirrel occupancy increased with fine-scale canopy closure in the absence of gray squirrels and decreased with fine-scale canopy closure in the presence of gray squirrels. (B) Fox squirrel occupancy remained stable with increasing understory cover in the presence of gray squirrels and declined with increased fine-scale understory cover in the absence of gray squirrels.
At the local scale, the best model did not include a para- meter for co-occurrence, matching the no interaction predic- tion (Table 2). Increased landscape canopy cover correlated with decreased fox squirrel occupancy (ΨA βLandscape canopy cover = −4.59, SE = 0.90), while local canopy cover correlated with increased gray squirrel occupancy (ΨB βLocal canopy = 1.16, SE = 0.28). Survey effort influenced fox squirrel detection [pA βEffort = 0.39, SE = 0.10], and gray squirrel detection was constant [pB βIntercept = −0.67, SE = 0.08]. Models incorporating landscape understory cover, mean patch area, constant interaction, and mean patch gyration had similar AICc support to the top model (Table 2), but the 95% CIs for the parameter estimate for ΨA:B crossed 0.
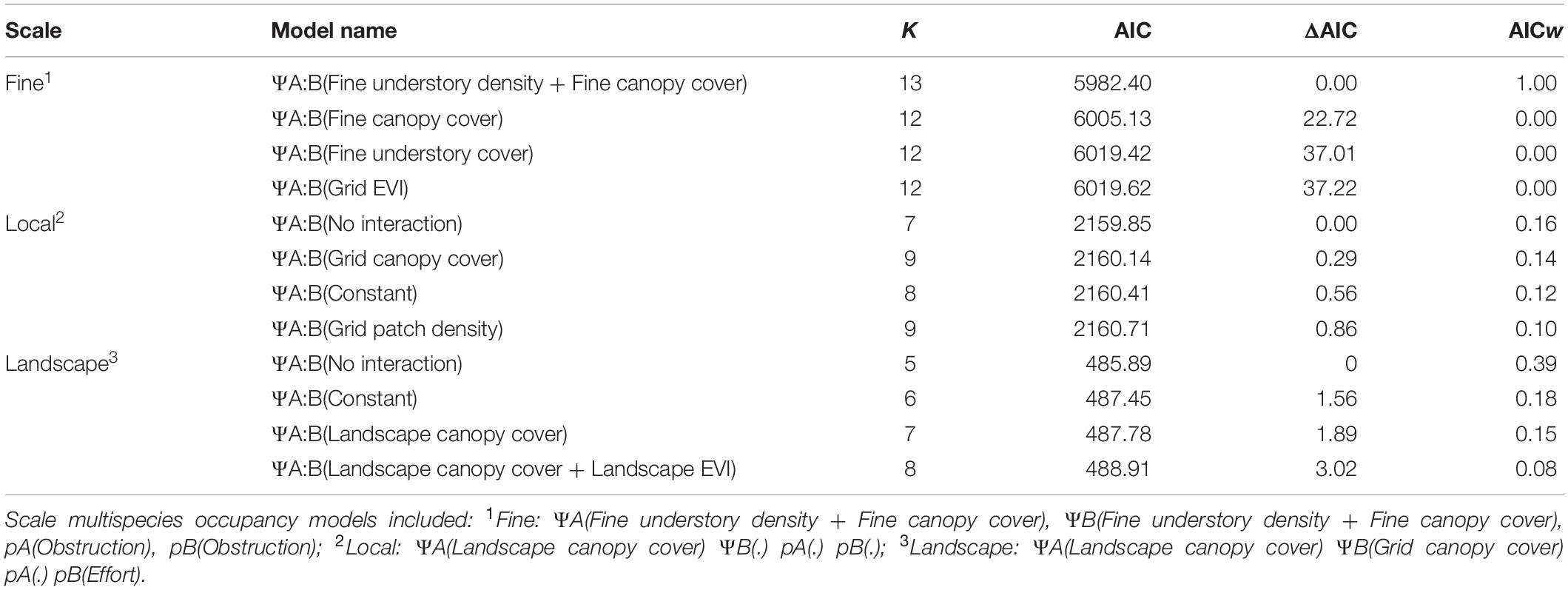
Table 2. Top four multispecies occupancy models for fox and gray squirrels in North and Central Florida, United States, for fine, local, and landscape scales based on AIC ranking.
At the landscape scale, the best model also did not include a parameter for co-occurrence, matching the no interaction prediction (Table 2). Fox squirrel occupancy correlated with decreased landscape canopy cover (ΨA βLandscape canopy cover = −5.21, SE = 2.12), while gray squirrel occupancy was constant (ΨB = βIntercept = 1.52, SE = 0.44). The model included constant detection probability for both fox and gray squirrels [pA βIntercept 0.25, SE = 0.18; pB βIntercept 0.10, SE = 0.17]. The model representing constant species interaction had a similar AICc support (Table 2); however, the 95% CIs for the parameter estimate for ΨA:B crossed 0.
Discussion
We found patterns of fox and gray squirrels co-occurrence that are indicative of potential competition at fine scales (0.01 ha) but not at local (5.3 ha) or landscape scales (765 ha). At fine scales, fox squirrels were less likely to occur in areas occupied by gray squirrels, while gray squirrel occupancy was not influenced by the presence of fox squirrels. Further, physical site characteristics helped to explain the co-occurrence patterns of fox and gray squirrels. In the absence of gray squirrels, fox squirrels readily utilized closed canopy areas but did not utilize them in the presence of gray squirrels. These findings support the hypothesis that the strength of species interactions changes over spatial scales and vegetation gradients (Kelt et al., 1995; Pearson and Dawson, 2004).
In the few fine-scale environments where they co-occurred fox squirrels were limited by gray squirrel in areas of closed canopy. These fine-scale interactions may help us explain the broad habitat partitioning patterns we observed. Generalist gray squirrels were ubiquitous in human modified landscapes, while specialist fox squirrels were not. In the more open landscapes where fox squirrel still occur, they appear limited at the fine scale by gray squirrels in areas of closed vegetation which are spreading across the region. These fine-scale patterns suggest a competitive advantage for gray squirrels in conditions that are increasingly common and also suggest a potential mechanism for broader scale patterns.
Fox and gray squirrels both responded positively to increases in canopy closure and negatively to increases in understory cover; however, they differed in the intensity of their response to each factor. Fox squirrels responded strongly (>25× more than gray squirrels) to understory cover and gray squirrels responded strongly to canopy closure (∼10× more than fox squirrels). Our results suggest that gray squirrels are better at utilizing closed canopy areas and outcompete fox squirrels in these areas. In the absence of gray squirrels, fox squirrels appear to readily utilize sites with closed canopies. Our results support the idea that interaction between these species may also be mediated by predation (Caswell, 1978; Van Der Merwe et al., 2005). Increases in understory density limit sightlines to detect predators for fox squirrels, thus reducing foraging efficiency (Potash et al., 2019). Further, increases in canopy closure reduce the foraging efficiency of gray and fox squirrel avian predators (Bowers et al., 1993; Van Der Merwe et al., 2005). Increases in canopy and understory cover are often due to human-induced factors including increased atmospheric CO2 and fire suppression (Roques et al., 2001; D’Odorico et al., 2012). Historically, fire shaped southeastern forests by maintaining broken canopies and low understory growth (Glitzenstein et al., 1995). Fire suppression on a local scale results in woody succession, first by increasing understory density (discouraging fox squirrels) and eventually leading to closed canopies (favoring the invasion of gray squirrels) (Keane et al., 2002; Swihart et al., 2007). Gray squirrels are efficient foragers and can persist in highly modified systems (Williamson, 1983; Kenward and Holm, 1989). Their ability to utilize resources in a small area may compensate for their poor dispersal ability across fragmented landscapes (Williamson, 1983; Goheen et al., 2003). Fine-scale interaction with fox and gray squirrels and increased canopy cover at the landscape scale may result in the loss of fox squirrels across woody encroached systems (Conner et al., 1999; Van Der Merwe et al., 2005; Greene and McCleery, 2017a).
As woody encroachment alters the interactions between fox and gray squirrels, there may be cascading effects throughout the ecosystem. Gray squirrels tolerate higher densities and overlap than fox squirrels (Koprowski, 1994), increasing both inter and intraspecific competition in invaded ecosystems. Fox and gray squirrels are important seed predators and scatter hoarders. Competition alters the probability that a seed is cached and where animals place those seeds (Muñoz and Bonal, 2011). Changes in scatter hoarding behavior alter the dispersal and reproductive success of many tree species (Whittaker and Jones, 1994; Terborgh et al., 2001; Silman et al., 2003). In the presence of competitors, squirrels are more likely to cache seeds in high-risk areas, such as broken canopy savannas (Heinrich and Pepper, 1998; Murray et al., 2006). Placing hard mast in risky, open areas may accelerate hardwood encroachment in these areas and act as a positive feedback mechanism. Thus, the invasion of pine savannas by gray squirrels due to woody encroachment may have long-term consequences for forest structure. Future investigations of the interactions between fox and gray squirrels may benefit from considering density dependent interactions and temporal changes in occupancy rates. Competition is a density dependent process and the relationship between fox squirrels, gray squirrels, and their environment may be highly dependent on their relative densities (Rosenzweig, 1991; van Beest et al., 2014). Further, we investigated a specific point in time for our analysis and compared different habitat types as a “space-for-time” substitution (Pickett, 1989). Longer term monitoring of fox and gray squirrel co-occupancy may reveal more nuanced relationships or expose competition at larger scales.
Our results suggest that human modification of environmental conditions favor generalist species that can utilize fine-scale resources. In our system, canopies are closing on fine, local, and landscape scales due to human activities. Generalists, like gray squirrels, have greater tolerances to the broad landscape changes occurring in our system (Sirami and Monadjem, 2012). Fox squirrels, like many longleaf pine savanna specialists, did not occur in areas with extensive canopy cover indicative of homogenizing human landscapes. In our system, generalists like gray squirrels, Southern flying squirrels (Glaucomys volans), and Virginia opossums (Didelphis virginiana) with good fine-scale competitive advantages are likely to replace specialists like fox squirrels, red cockaded woodpeckers (Leuconotopicus borealis), and gopher tortoises (Gopherus polyphemus), ultimately simplifying species diversity in this imperiled ecosystem. Our results support the importance of considering species interactions at multiple scales in predicting and addressing changes in community composition in human modified systems (Araújo and Luoto, 2007).
Data Availability Statement
The raw data supporting the conclusions of this article will be made available by the authors, without undue reservation.
Ethics Statement
The animal study was reviewed and approved by the University of Florida Institutional Animal Care and Use Committee.
Author Contributions
All authors contributed to the writing and review of the manuscript. AS conducted the data analysis. DG and RM conceived the study design. DG conducted the data collection.
Funding
This project was funded by the USDA National Institute of Food and Agriculture and Florida’s State Wildlife Grant through Florida’s Wildlife Legacy Initiative (Agreement 1142) and U.S. National Institute of Food and Agriculture, Hatch project FLA-WEC-005125.
Conflict of Interest
DG is employed by the Weyerhaeuser Company.
The remaining authors declare that the research was conducted in the absence of any commercial or financial relationships that could be construed as a potential conflict of interest.
Acknowledgments
Special thanks to the Florida Fish and Wildlife Commission, National Forest Service, Florida Forest Service, Florida Park Service, The Florida National Guard’s Camp Blanding Joint Training Center, the University of Florida’s Ordway-Swisher Biological Station, Plant Science Research and Education Unit, Austin Cary Forest, and two private landowners who granted us permission to conduct research on their lands. We also thank J. Mulvey, C. Godwin, F. Hayes, B. Piper, W. W. Boone IV, and the many volunteers whose countless hours conducting surveys made this project successful and M. S. Sovie for copy editing the manuscript.
Supplementary Material
The Supplementary Material for this article can be found online at: https://www.frontiersin.org/articles/10.3389/fevo.2020.00239/full#supplementary-material
References
Anthelme, F., Villaret, J. C., and Brun, J. J. (2007). Shrub encroachment in the Alps gives rise to the convergence of sub-alpine communities on a regional scale. J. Veg. Sci. 18, 355–362. doi: 10.1111/j.1654-1103.2007.tb02547.x
Araújo, M. B., and Luoto, M. (2007). The importance of biotic interactions for modelling species distributions under climate change. Glob. Ecol. Biogeogr. 16, 743–753.
Bonsall, M. B., Jansen, V. A., and Hassell, M. P. (2004). Life history trade-offs assemble ecological guilds. Science 306, 111–114.
Boone, W. W., McCleery, R. A., and Reichert, B. E. (2017). Fox squirrel response to forest restoration treatments in longleaf pine. J. Mammal. 98, 1594–1603. doi: 10.1093/jmammal/gyx110
Bowers, M. A., Jefferson, J. L., and Kuebler, M. G. (1993). Variation in giving-up densities of foraging chipmunks (Tamias striatus) and squirrels (Sciurus carolinensis). Oikos 66, 229–236.
Brown, B. W., and Batzli, G. O. (1985). Field manipulations of fox and gray squirrel populations: how important is interspecific competition? Can. J. Zool. 63, 2134–2140. doi: 10.1139/z85-314
Brown, J. S. (1999). Vigilance, patch use and habitat selection: foraging under predation risk. Evol. Ecol. Res. 1, 49–71.
Burnham, K., and Anderson, D. (2002). Model Selection and Multi-Model Inference, 2nd Edn. New York, NY: Springer.
Cadotte, M. W. (2007). Competition–colonization trade-offs and disturbance effects at multiple scales. Ecology 88, 823–829. doi: 10.1890/06-1117
Canfield, R. H. (1941). Application of the line interception method in sampling range vegetation. J. For. 39, 388–394.
Caswell, H. (1978). Predator-mediated coexistence: a nonequilibrium model. Am. Nat. 112, 127–154. doi: 10.1086/283257
Conner, L. M., Landers, J. L., and Michener, W. K. (1999). Fox squirrel and gray squirrel associations within minimally disturbed longleaf pine forests. Proc. Annu. Conf. Southeast. Assoc. Fish Wildl. Agencies 53, 364–374.
Cote, J., Bestion, E., Jacob, S., Travis, J., Legrand, D., and Baguette, M. (2017). Evolution of dispersal strategies and dispersal syndromes in fragmented landscapes. Ecography 40, 56–73. doi: 10.1111/ecog.02538
Cottam, G., and Curtis, J. T. (1956). The use of distance measures in phytosociological sampling. Ecology 37, 451–460. doi: 10.2307/1930167
Derge, K. L., and Yahner, R. H. (2000). Ecology of sympatric fox squirrels (Sciurus niger) and gray squirrels (S. carolinensis) at forest-farmland interfaces of Pennsylvania. Am. Midl. Nat. 143, 355–370.
Didan, K. (2015). MOD13Q1 MODIS/Terra Vegetation Indices 16-Day L3 Global 250m SIN Grid V006. NASA EOSDIS Land Processes DAAC. doi: 10.5067/MODIS/MOD13Q1.006
Dill, L. M., and Houtman, R. (1989). The influence of distance to refuge on flight initiation distance in the gray squirrel (Sciurus carolinensis). Can. J. Zool. 67, 233–235. doi: 10.1139/z89-033
Ditchkoff, S. S., Saalfeld, S. T., and Gibson, C. J. (2006). Animal behavior in urban ecosystems: modifications due to human-induced stress. Urban Ecosyst. 9, 5–12. doi: 10.1007/s11252-006-3262-3
D’Odorico, P., Okin, G. S., and Bestelmeyer, B. T. (2012). A synthetic review of feedbacks and drivers of shrub encroachment in arid grasslands. Ecohydrology 5, 520–530. doi: 10.1002/eco.259
Edwards, J. W., Heckel, D. G., and Guynn, D. C. Jr. (1998). Niche overlap in sympatric populations of fox and gray squirrels. J. Wildl. Manag. 62, 354–363.
Eldridge, D. J., Bowker, M. A., Maestre, F. T., Roger, E., Reynolds, J. F., and Whitford, W. G. (2011). Impacts of shrub encroachment on ecosystem structure and functioning: towards a global synthesis. Ecol. Lett. 14, 709–722. doi: 10.1111/j.1461-0248.2011.01630.x
Fischer, J., and Lindenmayer, D. B. (2007). Landscape modification and habitat fragmentation: a synthesis. Glob. Ecol. Biogeogr. 16, 265–280. doi: 10.1111/j.1466-8238.2007.00287.x
Fiske, I., Chandler, R., Miller, D., Royle, A., Kery, M., Hostetler, J., et al. (2015). Package ‘unmarked’. R Project for Statistical Computing.
Freeman, J. E., and Jose, S. (2009). The role of herbicide in savanna restoration: effects of shrub reduction treatments on the understory and overstory of a longleaf pine Flatwoods. For. Ecol. Manag. 257, 978–986. doi: 10.1016/j.foreco.2008.10.041
Fry, J. D. (2003). Detecting ecological trade-offs using selection experiments. Ecology 84, 1672–1678. doi: 10.1890/0012-9658(2003)084[1672:detuse]2.0.co;2
Glitzenstein, J. S., Platt, W. J., and Streng, D. R. (1995). Effects of fire regime and habitat on tree dynamics in north Florida longleaf pine savannas. Ecol. Monogr. 65, 441–476. doi: 10.2307/2963498
Goheen, J. R., Swihart, R. K., Gehring, T. M., and Miller, M. S. (2003). Forces structuring tree squirrel communities in landscapes fragmented by agriculture: species differences in perceptions of forest connectivity and carrying capacity. Oikos 102, 95–103. doi: 10.1034/j.1600-0706.2003.12336.x
Gotelli, N. J., and McCabe, D. J. (2002). Species co-occurrence: a meta-analysis of JM Diamond’s assembly rules model. Ecology 83, 2091–2096. doi: 10.1890/0012-9658(2002)083[2091:scoama]2.0.co;2
Greene, D. U., and McCleery, R. A. (2017a). Multi-scale responses of fox squirrels to land-use changes in Florida: utilization mimics historic pine savannas. For. Ecol. Manag. 391, 42–51. doi: 10.1016/j.foreco.2017.02.001
Greene, D. U., and McCleery, R. A. (2017b). Reevaluating fox squirrel (Sciurus niger) population declines in the southeastern United States. J. Mammal. 98, 502–512.
Greene, D. U., McCleery, R. A., Wagner, L. M., and Garrison, E. P. (2016). A comparison of four survey methods for detecting fox squirrels in the southeastern United States. J. Fish Wildl. Manag. 7, 99–106. doi: 10.3996/082015-jfwm-080
Hagemeyer, B. C., Jordan, L. A., Moses, A. L., Spratt, S. M., and Van Dyke, D. F. (2010). “Climatological, meteorological, and societal implications for the large number of fatalities from central Florida Dry Season tornadoes during El Niño,” in Proceedings of the 22nd Conference on Climate Variability and Change. American Meteorology Society, Atlanta.
Heinrich, B., and Pepper, J. W. (1998). Influence of competitors on caching behaviour in the common raven, Corvus corax. Anim. Behav. 56, 1083–1090. doi: 10.1006/anbe.1998.0906
Hesselbarth, H. K., Sciaini, S., With, K. A., Wiegand, K., and Nowosad, J. (2019). landscapemetrics: an open-source R tool to calculate landscape metrics. Ecography 42, 1648–1657. doi: 10.1111/ecog.04617
Jin, S., Yang, L., Danielson, P., Homer, C., Fry, J., and Xian, G. (2013). A comprehensive change detection method for updating the National Land Cover Database to circa 2011. Rem. Sens. Environ. 132, 159–175. doi: 10.1016/j.rse.2013.01.012
Johnson, D. H. (1980). The comparison of usage and availability measurements for evaluating resource preference. Ecology 61, 65–71. doi: 10.2307/1937156
Keane, R. E., Ryan, K. C., Veblen, T. T., Allen, C. D., Logan, J. A., Hawkes, B., et al. (2002). “The cascading effects of fire exclusion in Rocky Mountain ecosystems,” in Rocky Mountain Futures: an Ecological Perspective, eds J. Baron and P. R. Ehrlich, (Washington, DC: Island Press), 133–152.
Kelt, D. A., Taper, M. L., and Meserve, P. L. (1995). Assessing the impact of competition on community assembly: a case study using small mammals. Ecology 76, 1283–1296. doi: 10.2307/1940935
Kenward, R. E., and Holm, J. L. (1989). What future for British red squirrels? Biol. J. Linn. Soc. 38, 83–89. doi: 10.1111/j.1095-8312.1989.tb01565.x
Kneitel, J. M., and Chase, J. M. (2004). Trade-offs in community ecology: linking spatial scales and species coexistence. Ecol. Lett. 7, 69–80. doi: 10.1046/j.1461-0248.2003.00551.x
Lemmon, P. E. (1956). A spherical densiometer for estimating forest overstory density. For. Sci. 2, 314–320.
Lewis, S. L., and Maslin, M. A. (2015). Defining the anthropocene. Nature 519, 171–180. doi: 10.1038/nature14258
Lyons, S. K., Amatangelo, K. L., Behrensmeyer, A. K., Bercovici, A., Blois, J. L., Davis, M., et al. (2016). Holocene shifts in the assembly of plant and animal communities implicate human impacts. Nature 529, 80–83. doi: 10.1038/nature16447
Marvier, M., Kareiva, P., and Neubert, M. G. (2004). Habitat destruction, fragmentation, and disturbance promote invasion by habitat generalists in a multispecies metapopulation. Risk Anal. 24, 869–878. doi: 10.1111/j.0272-4332.2004.00485.x
McGarigal, K. (2014). “Landscape pattern metrics,” in Wiley StatsRef: Statistics Reference Online, eds N. Balakrishnan, T. Colton, B. Everitt, W. Piegorsch, F. Ruggeri, and J. L. Teugels, (Hoboken, NJ: John Wiley & Sons).
McGregor, H. W., Legge, S., Jones, M. E., and Johnson, C. N. (2014). Landscape management of fire and grazing regimes alters the fine-scale habitat utilisation by feral cats. PLoS One 9:e109097. doi: 10.1371/journal.pone.0109097
Moore, J. C. (1957). The natural history of the fox squirrel, Sciurus niger shermani. Bull. AMNH 113:1. doi: 10.1007/978-3-319-23534-9_1
Moore, J. E., and Swihart, R. K. (2005). Modeling patch occupancy by forest rodents: incorporating detectability and spatial autocorrelation with hierarchically structured data. J. Wildl. Manag. 69, 933–949. doi: 10.2193/0022-541x(2005)069[0933:mpobfr]2.0.co;2
Muñoz, A., and Bonal, R. (2011). Linking seed dispersal to cache protection strategies. J. Ecol. 99, 1016–1025. doi: 10.1111/j.1365-2745.2011.01818.x
Murray, A. L., Barber, A. M., Jenkins, S. H., and Longland, W. S. (2006). Competitive environment affects food-hoarding behavior of Merriam’s kangaroo rats (Dipodomys merriami). J. Mammal. 87, 571–578. doi: 10.1644/05-mamm-a-172r1.1
Ovaskainen, O., Hottola, J., and Siitonen, J. (2010). Modeling species co-occurrence by multivariate logistic regression generates new hypotheses on fungal interactions. Ecology 91, 2514–2521. doi: 10.1890/10-0173.1
Pearson, R. G., and Dawson, T. P. (2004). Bioclimate envelope models: what they detect and what they hide—response to Hampe. Glob. Ecol. Biogeogr. 13, 471–473. doi: 10.1111/j.1466-822x.2004.00112.x
Perkins, M. W., Conner, L. M., and Howze, M. B. (2008). The importance of hardwood trees in the longleaf pine forest ecosystem for Sherman’s fox squirrels. For. Ecol. Manag. 255, 1618–1625. doi: 10.1016/j.foreco.2007.11.020
Pickett, S. T. (1989). “Space-for-time substitution as an alternative to long-term studies,” in Long-Term Studies in Ecology, ed. G. E. Likens, (New York, NY: Springer), 110–135. doi: 10.1007/978-1-4615-7358-6_5
Platt, W. J., Entrup, A. K., Babl, E. K., Coryell-Turpin, C., Dao, V., Hebert, J. A., et al. (2015). Short-term effects of herbicides and a prescribed fire on restoration of a shrub-encroached pine savanna. Restor. Ecol. 23, 909–917.
Potash, A. D., Conner, L. M., and McCleery, R. A. (2019). Vertical and horizontal vegetation cover synergistically shape prey behaviour. Anim. Behav. 152, 39–44.
Provencher, L., Herring, B. J., Gordon, D. R., Rodgers, H. L., Tanner, G. W., Hardesty, J. L., et al. (2001). Longleaf pine and oak responses to hardwood reduction techniques in fire-suppressed Sandhills in northwest Florida. For. Ecol. Manag. 148, 63–77. doi: 10.1016/s0378-1127(00)00525-9
R Core Team (2019). R: A Language and Environment for Statistical Computing. Vienna, Austria: R Foundation for Statistical Computing. https://www.R-project.org/
Richmond, O. M., Hines, J. E., and Beissinger, S. R. (2010). Two-species occupancy models: a new parameterization applied to co-occurrence of secretive rails. Ecol. Appl. 20, 2036–2046. doi: 10.1890/09-0470.1
Roques, K. G., O’connor, T. G., and Watkinson, A. R. (2001). Dynamics of shrub encroachment in an African savanna: relative influences of fire, herbivory, rainfall and density dependence. J. Appl. Ecol. 38, 268–280. doi: 10.1046/j.1365-2664.2001.00567.x
Rosenzweig, M. L. (1991). Habitat selection and population interactions: the search for mechanism. Am. Nat. 137, 5–28.
Rota, C. T., Ferreira, M. A., Kays, R. W., Forrester, T. D., Kalies, E. L., McShea, W. J., et al. (2016). A multispecies occupancy model for two or more interacting species. Methods Ecol. Evol. 7, 1164–1173. doi: 10.1111/2041-210x.12587
Sebastian-Gonzalez, E., Sánchez-Zapata, J. A., Botella, F., and Ovaskainen, O. (2010). Testing the heterospecific attraction hypothesis with time-series data on species co-occurrence. Proc. R. Soc. B Biol. Sci. 277, 2983–2990. doi: 10.1098/rspb.2010.0244
Sexton, O. J. (1990). Replacement of fox squirrels by gray squirrels in a suburban habitat. Am. Midl. Nat. 124, 198–205.
Silman, M. R., Terborgh, J. W., and Kiltie, R. A. (2003). Population regulation of a dominant rain forest tree by a major seed predator. Ecology 84, 431–438. doi: 10.1890/0012-9658(2003)084[0431:proadr]2.0.co;2
Singh, K. K., Davis, A. J., and Meentemeyer, R. K. (2015). Detecting understory plant invasion in urban forests using LiDAR. Int. J. Appl. Earth Obs. Geoinf. 38, 267–279. doi: 10.1016/j.jag.2015.01.012
Sirami, C., and Monadjem, A. (2012). Changes in bird communities in Swaziland savannas between 1998 and 2008 owing to shrub encroachment. Divers. Distrib. 18, 390–400. doi: 10.1111/j.1472-4642.2011.00810.x
Sirami, C., Seymour, C., Midgley, G., and Barnard, P. (2009). The impact of shrub encroachment on savanna bird diversity from local to regional scale. Divers. Distrib. 15, 948–957. doi: 10.1111/j.1472-4642.2009.00612.x
Sovie, A. R. (2019). Response of Vertebrates to Hardwood Encroachment in a Pine Savanna. Ph.D. dissertation, University of Florida, Gainesville, FL.
Sovie, A. R., Greene, D. U., Frock, C. F., Potash, A. D., and McCleery, R. A. (2019). Ephemeral temporal partitioning may facilitate coexistence in competing species. Anim. Behav. 150, 87–96. doi: 10.1016/j.anbehav.2019.01.020
Spector, T., and Putz, F. E. (2006). Crown retreat of open-grown Southern live oaks (Quercus virginiana) due to canopy encroachment in Florida, USA. For. Ecol. Manag. 228, 168–176. doi: 10.1016/j.foreco.2006.03.001
Stanton, R. A. Jr., Boone, W. W. IV, Soto-Shoender, J., Fletcher, R. J. Jr., Blaum, N., and McCleery, R. A. (2018). Shrub encroachment and vertebrate diversity: a global meta-analysis. Glob. Ecol. Biogeogr. 27, 368–379. doi: 10.1111/geb.12675
Steele, M. A., Rompre, G., Stratford, J. A., Zhang, H., Suchocki, M., and Marino, S. (2015). Scatterhoarding rodents favor higher predation risks for cache sites: the potential for predators to influence the seed dispersal process. Integr. Zool. 10, 257–266. doi: 10.1111/1749-4877.12134
Swihart, R. K., Goheen, J. R., Schnelker, S. A., and Rizkalla, C. E. (2007). Testing the generality of patch and landscape-level predictors of tree squirrel occurrence at a regional scale. J. Mammal. 88, 564–572. doi: 10.1644/06-mamm-a-275r.1
Terborgh, J., Lopez, L., Nuñez, P., Rao, M., Shahabuddin, G., Orihuela, G., et al. (2001). Ecological meltdown in predator-free forest fragments. Science 294, 1923–1926. doi: 10.1126/science.1064397
Tews, J., Brose, U., Grimm, V., Tielbörger, K., Wichmann, M. C., Schwager, M., et al. (2004). Animal species diversity driven by habitat heterogeneity/diversity: the importance of keystone structures. J. Biogeogr. 31, 79–92. doi: 10.1046/j.0305-0270.2003.00994.x
Van Auken, O. W., and Bush, J. K. (eds) (2013). “Encroachment and secondary succession,” in Invasion of Woody Legumes, (New York, NY: Springer), 11–14. doi: 10.1007/978-1-4614-7199-8_3
van Beest, F. M., McLoughlin, P. D., Vander Wal, E., and Brook, R. K. (2014). Density-dependent habitat selection and partitioning between two sympatric ungulates. Oecologia 175, 1155–1165. doi: 10.1007/s00442-014-2978-7
Van Der Merwe, M., Brown, J. S., and Jackson, W. M. (2005). The coexistence of fox (Sciurus niger) and gray (S. caroliniensis) squirrels in the Chicago metropolitan area. Urban Ecosyst. 8:335. doi: 10.1007/s11252-005-4865-9
Weigl, P. D., Steele, M. A., Sherman, L. J., Ha, J. C., and Sharpe, T. L. (1989). The Ecology of the Fox Squirrel (Sciurus niger) in North Carolina: Implications for Survival in the Southeast. Tallahassee, FL: Bulletin-Tall Timbers Research Station.
Whittaker, R. J., and Jones, S. H. (1994). The role of frugivorous bats and birds in the rebuilding of a tropical forest ecosystem, Krakatau, Indonesia. J. Biogeogr. 21, 245–258.
Wilbanks, T. J., and Kates, R. W. (1999). Global change in local places: how scale matters. Clim. Change 43, 601–628.
Williamson, R. D. (1983). Identification of urban habitat components which affect eastern gray squirrel abundance. Urban Ecol. 7, 345–356. doi: 10.1016/0304-4009(83)90020-7
Keywords: gray squirrel, fox squirrel, competition, multispecies occupancy, woody encroachment
Citation: Sovie AR, Greene DU and McCleery RA (2020) Woody Cover Mediates Fox and Gray Squirrel Interactions. Front. Ecol. Evol. 8:239. doi: 10.3389/fevo.2020.00239
Received: 10 April 2020; Accepted: 01 July 2020;
Published: 30 July 2020.
Edited by:
Toni Gossmann, Bielefeld University, GermanyReviewed by:
Michael Alan Steele, Wilkes University, United StatesBurt P. Kotler, Ben-Gurion University of the Negev, Israel
Copyright © 2020 Sovie, Greene and McCleery. This is an open-access article distributed under the terms of the Creative Commons Attribution License (CC BY). The use, distribution or reproduction in other forums is permitted, provided the original author(s) and the copyright owner(s) are credited and that the original publication in this journal is cited, in accordance with accepted academic practice. No use, distribution or reproduction is permitted which does not comply with these terms.
*Correspondence: Adia R. Sovie, YWRpYS5zb3ZpZUB1a3kuZWR1