- 1Department of Theoretical and Applied Sciences, University of Insubria, Varese, Italy
- 2School of Natural Resources and the Environment, The University of Arizona, Tucson, AZ, United States
Intraspecific variation in home range size has important implications for the distribution of animals across landscapes and the spatial structuring of population, community, and ecosystem processes. Among species of similar trophic guild and body mass, differences in home range size can reflect extrinsic variables that exert divergent selective forces upon spacing behavior and social organization. We tested predictions about how resource availability and timing influence social system, home range size, and territoriality in two tree squirrel species of similar size and ecological niches but that differ in foraging strategy and social organization. We estimated home range size and intraspecific home range core overlap in the Mt. Graham red squirrel (Tamiasciurus fremonti grahamensis; Arizona USA; MGRS) and the Eurasian red squirrel (Sciurus vulgaris; Alps, Italy; ERS) as functions of species, sex, season, and individual’s body mass. However, body mass did not explain differences found between the two species. We found MGRS home ranges being three times smaller with higher core area exclusivity compared to ERS in all seasons. In fact, territorial MGRS evolved in a system of brief resource pulses and are larder hoarders, whereas ERS experience prolonged resource availability and are non-territorial. Only male MGRSs increased their home range during the breeding season, reflecting interspecific differences in social organization and mating behavior. Male ERS home ranges always overlap with several females to enhance mating success although male and female MGRS maintain nearly exclusive territories throughout the year. Only during spring and summer do males temporarily leave their food-based territory to increase mating opportunities with neighboring estrus females. Home range comparisons between ecologically similar species emphasize the importance of divergent extrinsic factors in shaping variability in body size–home range size scaling relationships. Timing in resource availability influenced the social structure and space use in tree squirrels of similar body size, highlighting how the coevolution of arboreal squirrels with conifer tree species has shaped their natural history.
Introduction
Individuals may travel across a discrete area, or home range, on a daily basis or over longer intervals of time in search of limited resources that include food, shelter, nesting sites, and potential mates (Burt, 1943; Moorcroft, 2012). Home range is dynamic, and its size represents a key trait for individuals in a population with important implications for the distribution of animals across landscapes and ultimately the spatial structuring of community and ecosystem processes (Stamps, 1995; Van Beest et al., 2011; Tamburello et al., 2015).
At the species level, average home range size scales positively with body mass (Jetz et al., 2004; Tamburello et al., 2015), yet tremendous variability in home range size exists among species of similar body mass and among populations of the same species (Tucker et al., 2014; Ofstad et al., 2016). There is feedback among species-, population-, and individual-level drivers such that mean home range size for a species is a product of top-down and bottom-up processes (McLoughlin and Ferguson, 2000). At the species level, home range size is a product of slow, evolutionary processes (e.g., energetics and natural history in response to climate, the physical environment). Among populations, home range size is a product of moderate-to-slow processes that occur over generations (e.g., climate on local food availability and distribution of resources); among individuals in a population, home range size is a product of rapidly changing intrinsic and extrinsic drivers (e.g., resource availability, age, sex, and body condition) (McLoughlin and Ferguson, 2000).
Species-level extrinsic drivers of home range size also influence spacing behavior and social organization and are reflected in home range exclusivity and overlap (Maher and Lott, 2000). Jetz et al. (2004) developed a mechanistic model that demonstrates how animal space use reflects both energy requirements (body size) and degree of intraspecific competition (home range overlap). Larger animals have higher energy requirements and, consequently, need larger home ranges to find sufficient (food) energy (Jetz et al., 2004; Tamburello et al., 2015). Because larger home ranges and their resources are more difficult to defend against conspecific intruders, exclusivity of home range use (hence, territoriality) decreases with increasing body size (Jetz et al., 2004) or resource distribution, availability, and predictability (McLoughlin and Ferguson, 2000; McLoughlin et al., 2001). Hence, species of similar size and occupying comparable ecological niches can have different average home range sizes and home range exclusivity due to divergent extrinsic factors, such as the co-evolutionary history within a community, distribution and predictability of limited resources, and subsequent evolution of different spacing behaviors (Maher and Lott, 2000; McLoughlin and Ferguson, 2000; McLoughlin et al., 2001).
Herein, we sought to understand the role of important species-, population-, and individual-level drivers of home range size and overlap by comparing space use of two ecologically similar tree squirrel species: an endangered population endemic to southeastern Arizona in the United States, the Mt. Graham red squirrel (Tamiasciurus fremonti grahamensis; Hope et al., 2016), and the Eurasian red squirrel (Sciurus vulgaris) in the Italian Alps (Loy et al., 2019). Tree squirrels (genus Sciurus and Tamiasciurus) have remained virtually unchanged in form and function over the last 38 million years, a testament to their successful co-evolutionary history with forest ecosystems of the world (Smith, 1970; Steele, 2008). Both species occupy similar high-elevation montane coniferous forests that produce seed crops of comparable size but that are highly variable over time, typical for pulsed resource systems. The species differ slightly in body mass with adult Mt. Graham red squirrels in our study areas weighing 160–298 g (mean 234 g) and most adult Eurasian red squirrels in our study areas weighing 270–330 g (mean 304 g; Wauters et al., 2007a). Eurasian red squirrels have overlapping home ranges with more intensively used core areas, whose size differs between the sexes and in relation to season and habitat quality (e.g., Lurz et al., 2000; Wauters et al., 2001; Thomas et al., 2018). Males have larger home ranges than females and overlap with several females to increase their probability of reproducing. Females tend to defend exclusive core areas against other females (Wauters and Dhondt, 1992; Wauters et al., 2001; Di Pierro et al., 2008; Romeo et al., 2010). The mating system is promiscuous although most females only mate with a dominant male of high body mass (Wauters et al., 1990). In North American red squirrels (T. hudsonicus and T. fremonti), territoriality is the most common social system, particularly in coniferous forests in which individuals defend a central larderhoard or midden (Kemp and Keith, 1970; Rusch and Reeder, 1978; Steele, 1998). The Mt. Graham red squirrel maintains nearly exclusive territory core areas with considerable overlap at the home range periphery, primarily driven by males overlapping female home ranges in the spring–summer breeding season (Koprowski et al., 2008). In contrast, females minimize home range overlap with other females during all seasons. Home range size is 3–10 times greater in the Mt. Graham red squirrel compared to other ecologically similar populations of North American red squirrel (T. hudsonicus), and home range size varies seasonally for both sexes with the largest home ranges documented in summer (June–August) (Koprowski et al., 2008; Munroe et al., 2009).
Because both species share similar body size and food resources, we tested for the relative importance of season, sex, resource availability, and resource predictability on individual home range size and exclusivity across multiple years. At the species level, we predicted that (1) Mt. Graham red squirrels, being highly territorial, have smaller home ranges and core areas that are defended against conspecifics than non-territorial Eurasian red squirrels, and these patterns are true for both sexes. This difference between the species should hold true when taking individual variation in body mass into account. (2) Home range core area overlap is higher in Eurasian red squirrels than Mt. Graham red squirrels because the latter can better protect a smaller territory from intruders. (3) Home range size is larger in males of both species given that, in rodents with promiscuous or polygynous mating systems, males have larger home ranges than females. At the population and individual levels, we predicted that (4) home range size varies seasonally in both species using larger ranges in spring – a time of low food availability and increased breeding activity – than in autumn when the new seed crop becomes available. Finally, we predicted that (5) seasonal differences are largest in male Mt. Graham red squirrels as males often temporarily leave their food-based territory in the spring mating season to increase mating opportunities with neighboring females; because male Eurasian red squirrels already overlap with ranges of neighboring females, we do not expect to see big seasonal differences in their range size (Wauters and Dhondt, 1992; Koprowski et al., 2008).
Materials and Methods
Study Areas
Eurasian Red Squirrel (ERS)
We conducted the study in four sites within mature, secondary montane and subalpine mixed conifer forests in the Italian Alps with elevations ranging from 1100 to 2150 m (the upper timberline; Figure 1). All areas are in the upper Valtellina Valley in the Central Alps. Specific locations and distances between study sites are presented in Trizio et al. (2005). Study site Oga (OGA, 47 ha) is part of a mature mixed montane conifer forest dominated by Scots pine (Pinus sylvestris L.) with some Norway spruce (Picea abies Karst) and larch (Larix decidua Miller). Bormio (BOR, 93 ha) is mature secondary forest dominated by Arolla pine (Pinus cembra L.). Cedrasco (CED, 76 ha) is a mixed montane conifer forest on the northern slope of the Orobic Alps with large areas of even-age stands dominated by silver fir (Abies alba Miller) and Norway spruce with sparse Scots pine, larch, and dead trees and some beech (Fagus sylvatica L.) at lower elevations (Salmaso et al., 2009). Site Cancano (CAN, 60 ha), located in a high-elevation valley, is almost entirely composed of a dense and homogeneous (dwarf) mountain pine (Pinus mugo Turra) subalpine woodland (Romeo et al., 2010). Seeds of all conifer species mature inside cones in September–October, and cones remain closed until the first warm and dry days of the following spring. The only exception is silver fir, which sheds mature seeds in autumn of the same year.
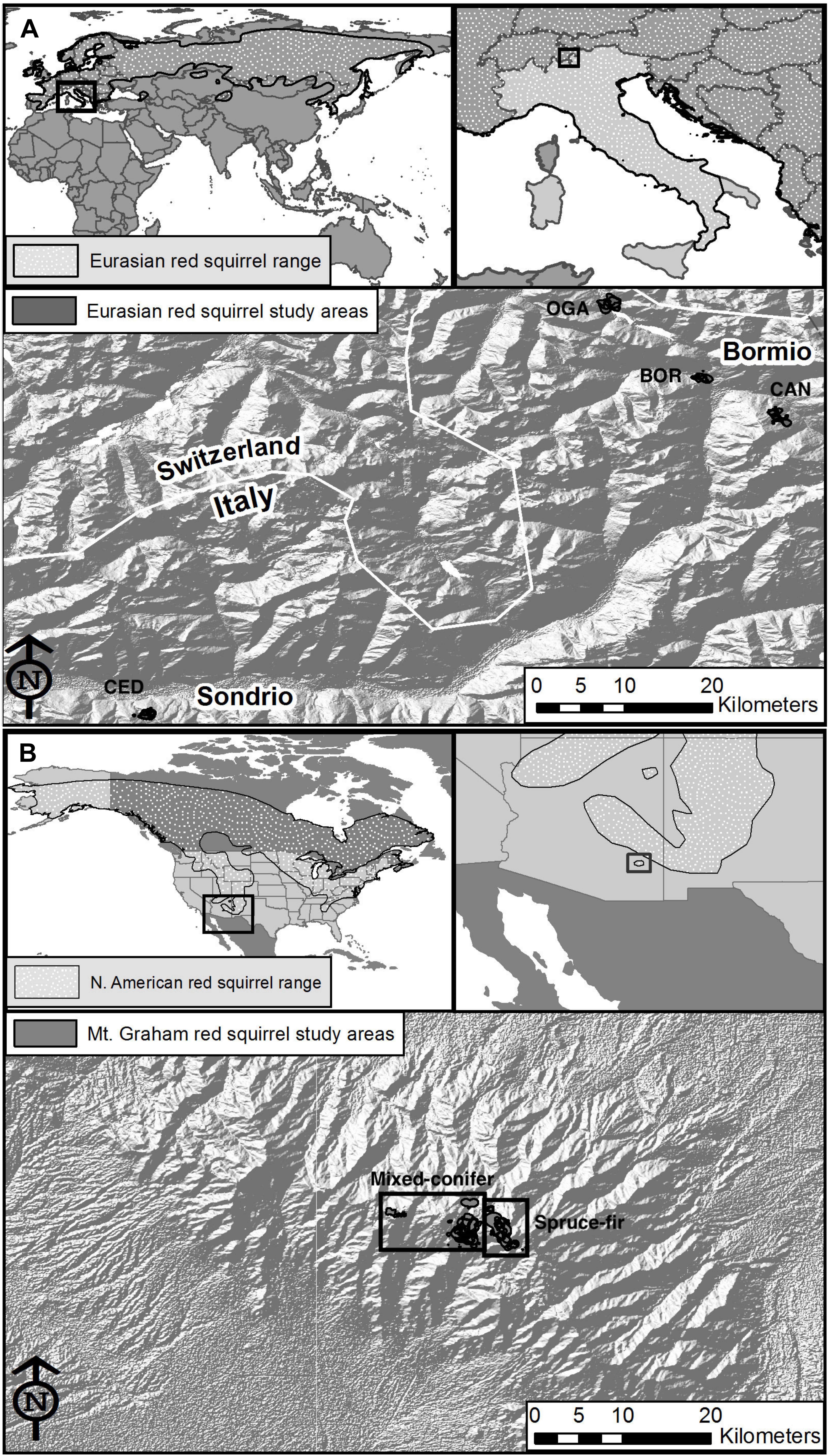
Figure 1. Overview of study areas for Eurasian red squirrels (Sciurus vulgaris) in the central Italian Alps of Lombardy, Italy (A, top) and Mt. Graham red squirrels (Tamiasciurus fremonti grahamensis) in the Pinaleño Mountains of southeastern Arizona, the United States (B, bottom). Study areas are shown in relation to the geographic range of the species or, in the case of North American red squirrels, species group.
Mt. Graham Red Squirrel (MGRS)
MGRS are restricted to mature spruce–fir and mesic mixed-conifer forest in the Pinaleño Mountains of southeastern Arizona, the United States, 32.7017°N, 109.8714°W at elevations above 2400 m (Figure 1). The Pinaleño Mountains are part of the Madrean Archipelago, a series of high-elevation peaks rising from desert grassland that are considered biodiversity hot spots (Spector, 2002). In the Pinaleño Mountains, mixed-conifer forest is dominated by Douglas fir (Pseudotsuga menzesii), southwestern white pine (Pinus strobiformis reflexis), white fir (Abies concolor), corkbark fir (Abies lasiocarpa var. arizonica), Engelmann spruce (Picea engelmannii), and aspen (Populus tremuloides). Spruce–fir forest occurs at the highest elevations and is dominated by corkbark fir and Engelmann spruce (Smith and Mannan, 1994; O’Connor et al., 2014). We examined MGRS space use and food availability in both spruce–fir and mixed-conifer forest. Our spruce–fir study areas (529 ha, 3048–3267 m) were severely damaged by infestations of western balsambark beetle (Drycoetes confusus), spruce beetle (Dendroctonus rufipennis), and introduced spruce aphid (Elatobium abietinum) followed by the stand-replacing Nuttal–Gibson fire in 2004 (O’Connor et al., 2014), and conifer seed production was reduced (Zugmeyer and Koprowski, 2009). Our mixed-conifer study areas (190 ha, 2647–2979 m) were not as heavily impacted by insect infestations and fire during the study period (2002–2016).
Three dominant conifer species in the Pinaleño Mountain study areas have winged, wind-dispersed seeds that can be collected in seedfall traps: Douglas fir, Engelmann spruce, and corkbark fir. Each of these species has cones that ripen and shed seeds in the fall of the same year. Douglas fir cones ripen in late July–early August and shed seeds mid-August–mid-September. Engelmann spruce cones ripen August–early September and shed seeds September–late October although seed dispersal continues through the winter. Corkbark fir cones open in mid-August–mid-October and shed seeds from mid-September–late October, and cones disintegrate when ripe (Burns and Honkala, 1990).
Seed and cone count methods and estimated seed (cone) energy content of the conifer species in the Alps and Mt. Graham study sites are given in Supplementary Material 1 (SM 1.1, SM 1.2). Estimated average seed energy availability over all study sites each year for the Alps and for Mt. Graham is presented in Figure 2 and SM 1.3.
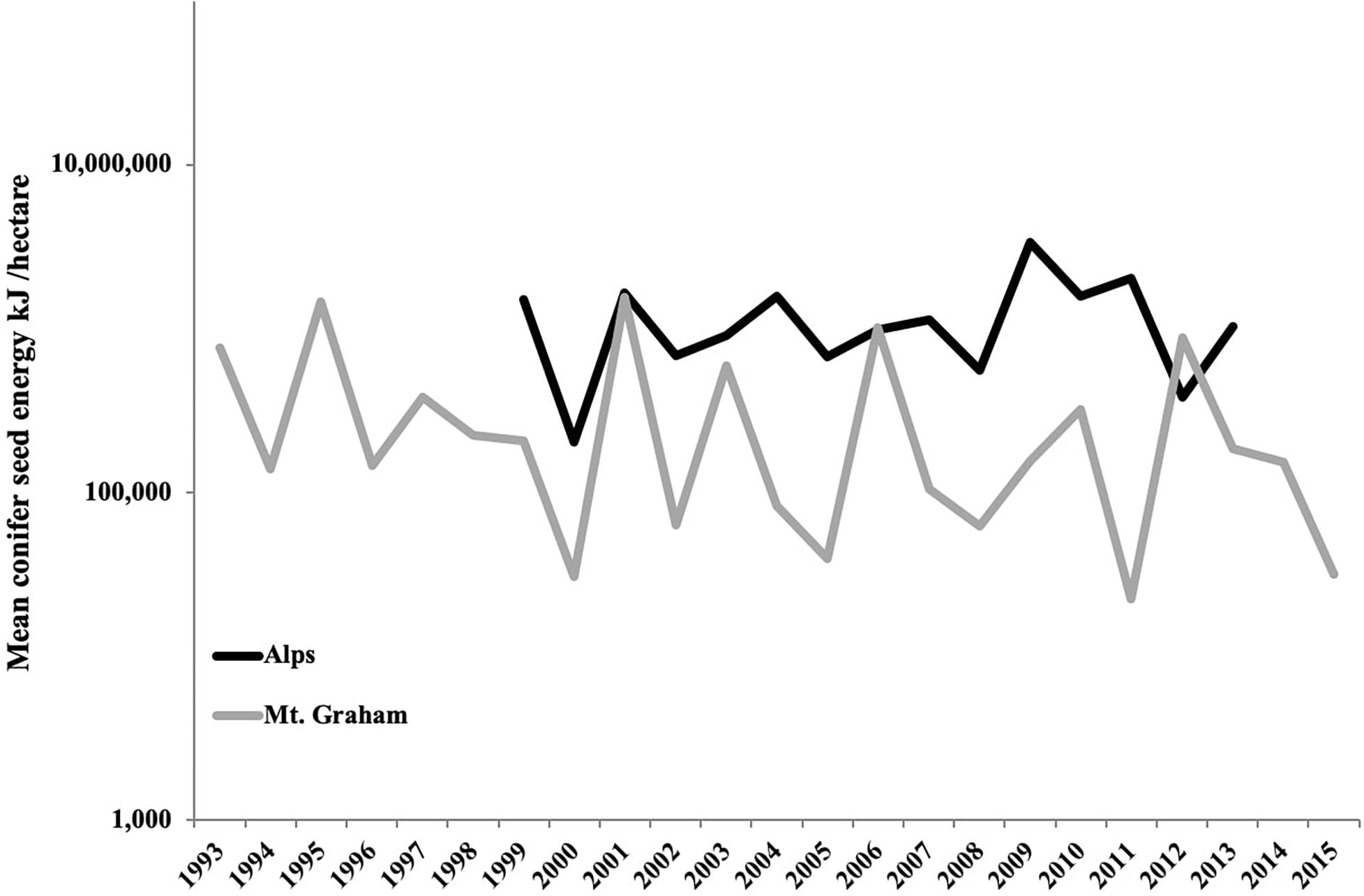
Figure 2. Mean annual conifer seed production across study areas in the Alps (Lombardy, Italy: black line) and on Mt. Graham (Pinaleño Mountains, Arizona, the United States: gray line) expressed in kJ/ha. Seed energy is a measure of food resources available to Eurasian red squirrels (Sciurus vulgaris) and Mt. Graham red squirrels (Tamiasciurus fremonti grahamensis), respectively each year. More details provided in SM 1.2–1.3.
Trapping, Handling, and Radio-Tracking
Eurasian Red Squirrel
We conducted trapping in the Italian Alps during three periods per year (April–May, June–July, September–October) between 2000 and 2016. A trapping session involved the use of 20 (CED, BOR, CAN) or 25 (OGA) ground-placed Tomahawk live traps [Tomahawk Live Trap, WI, United States: models #201 (40.64 × 12.7 × 12.7 cm) and #202 (48.26 × 15.24 × 15.24 cm)]. We placed traps on a grid with distances of 100–150 m between traps and average trap density of 0.6–0.7 traps ha–1 except for BOR, in which traps were placed along a transect due to constraints of slope and forest composition. We pre-baited traps with sunflower seeds and hazelnuts four to six times over a 30-day period and then baited and set for 5–8 days until no new, unmarked squirrels were trapped for at least 2 consecutive days (Wauters et al., 2008). We checked traps two to three times per day.
Each trapped squirrel was flushed into a light cotton handling bag with a zipper or a wire-mesh “handling cone” to minimize stress during handling and individually marked using numbered metal ear tags (type 1003 S, 10 by 2 mm, National Band and Tag, Newport, KY, United States). We weighed squirrels to the nearest 5 g using a spring balance (Pesola AG, Baar, Switzerland) and determined sex and age class on the basis of external genitalia and body mass with juveniles weighing less than 250 g (Wauters and Dhondt, 1995). We fitted adult squirrels with radio collars with adjustable necklace transmitters (PD-2C transmitters, Holohil Systems Ltd., Carp, Ontario, Canada or TW-4 transmitters, Biotrack Ltd., Wareham, Dorset, United Kingdom), and we used homing-in to relocate all radio-collared animals. Tracking periods and radio-tracking materials are described in detail elsewhere (Di Pierro et al., 2008; Romeo et al., 2010).
Mt. Graham Red Squirrel
Between June 2002 and May 2016, we trapped, radio-collared, and tracked MGRS as part of a long-term study of MGRS space use (Koprowski et al., 2008). Systematic surveys within our Pinaleño Mountain study areas over the years and seasons (March, June, September, and December) allowed us to map all occupied middens (territorial larderhoards). We used Tomahawk live traps (model #201: 40.64 × 12.7 × 12.7 cm), baited with peanuts and peanut butter and placed at an animal’s midden between 06:00 and 18:00 to capture MGRS. We checked traps every 2 h. Upon capture, we transferred each individual to a cloth handling cone (Koprowski, 2002) to measure morphological traits, apply colored ear tags, and fit radio collars (SOM 2190, Wildlife Materials International; Koprowski et al., 2008). We recaptured individuals at least every 3 months to assess body condition, reproductive status, and check radio collar fit. Methods for estimating age and reproductive condition were similar to those for ERS (Koprowski, 2005). Individuals ≥ 190 g were considered adults. We used homing and biangulation to relocate all radio-collared animals at least 12 times each month (Koprowski et al., 2008).
Statistical Analyses
All analyses were performed using R software 3.0.3 (R Development Core Team 2017). We used the 95% fixed kernel density estimator (KDE) with adjusted bandwidth (KDEadj proposed by Wauters et al., 2007b) to calculate home ranges within the R package HRTools (Preatoni and Bisi, 2013). Use of the 95% isopleth when estimating home range size excludes outlying locations and avoids an overestimation of home range size. We used the 85% KDEadj to estimate core area size because the utilization distribution curve of range size on percentage of fixes used showed a clear inflection point between the 85 and 90% isopleths (Wauters et al., 2007b). Although this estimator could potentially overestimate the size of core areas, it has the advantage that it can be used for both mono- and multinuclear core areas (Wauters et al., 2007b).
Per year, we estimated total averaged and seasonal home ranges: spring–early summer (March–July), which included mating and the main breeding season for both species (gestation, birth, and lactation for spring litters), and late summer–autumn (August–November), the period when animals start feeding on maturing (August) and fully mature (September–November) seeds of the current year’s cone crop.
Because the two species slightly differed in average body mass (ERS: 319 g; MGRS: 233 g), to follow the mammalian body mass–space use scaling rules, we applied the equation calculated by Tucker et al. (2014) for omnivores [Log (home range size) = −0.94 + 1.12 Log (body mass)] to our two study species so that we could predict their home range size based on their body mass and diet. We ln transformed home range size (ha) and core area size (ha) to meet the assumption of normality (Shapiro–Wilk’s test W = 0.99). To discern the species–home range size relationship from the body mass–home range size relationship, we ran two competing LMM with ln home range size as the dependent variable. The first had species (ERS vs. MGRS), sex (male, female). and season (breeding season, seed season) as fixed effects and included the species by sex, species by season and sex by season interactions. The second had body mass, sex, and season as fixed effects and included the body mass by sex, body mass by season, and sex by season interactions. We ln transformed body mass following Ofstad et al. (2016). In both linear mixed effect models (LMMs), individual was added as a random factor to account for repeated measures of the range of a given animal. Because it was not our aim to explore variation in home range size among years and not all years had data for both species, year was added as a random factor and not as a fixed effect. Model fit was based on the difference in log likelihood between the two models. The same approach was used for the ln of the core area size.
We ran the models with the best fit for the home range size and core area size and used the differences of least square means (hereafter DLSM) with Tukey p-value adjustment for multiple comparisons to analyze pairwise comparisons of two-factor interactions and obtain effect-size estimates.
We estimated overlap of core areas (85% KDEadj) as the percentage of overlap of a squirrel’s core area with the core area of all other radio-collared squirrels in the population (Wauters and Dhondt, 1992). We used the square root transformation of total proportion of core-area overlap of each individual squirrel within a given season and year as dependent variables to meet the assumption of normality (Shapiro–Wilk’s test W = 0.98). We tested our hypothesis of differences in core-area overlap between the species with an LMM with species (ERS vs. MGRS), sex of the individual, sex of the overlapper [four categories: male by males (MM), male by females (MF), female by males (FM), and female by females (FF)], and season (breeding season, seed season) as fixed effects and all the two-factor and the three-factor interactions. Individual was added as a random factor to account for repeated measures of the overlap of a given animal. Because it was not our aim to explore variation in core-area overlap among years and not all years had data for both species, year was added as a random factor. We used the DLSM with Tukey p-value adjustment for multiple comparisons to analyze pairwise comparisons of two-factor interactions.
Results
Home Range and Core Area Size
We obtained 139 (87 squirrels: 51 males, 36 females) home range size estimates for ERS and 720 (260 squirrels: 128 males, 132 females) for MGRS (see Supplementary Material 2 for detailed sample size per area, year, and season). On average, ERS home range size was three times larger than MGRS (mean ± SD: ERS = 20.92 ± 20.77 ha; MGRS 6.45 ± 11.20 ha). The formula by Tucker et al. (2014) predicted home range size of 3.18 ha for ERS and 2.25 ha for MGRS. Hence, based on their average body mass, ERS should have a 41% larger home range than MGRS. However, the model with the species as independent variable showed a better fit than the model with body mass for the home range size (difference in LogLikelihood = 32.26; df = 1; p < 0.0001).
Home ranges were larger during the breeding season than in the season of tree-seed maturation and consumption (Tables 1, 2). Several pairwise comparisons were significant. Both male and female ERS had larger home ranges than male and female MGRS, respectively (DLSM: males ERS – MGRS = 1.19 ± 0.12, t393 = 9.70, p < 0.0001; females ERS – MGRS = 0.91 ± 0.14, t426 = 6.69, p < 0.0001; Table 1). The difference in home range size between the two species was consistent over both seasons (DLSM: ERS – MGRS breeding season = 0.98 ± 0.11, t586 = 8.68, p < 0.0001; ERS – MGRS seed season = 1.12 ± 0.11, t607 = 10.3, p < 0.0001; Table 1). Moreover, the difference in home range size between the sexes was significant only for ERS with male ERS occupying larger ranges than females and no difference between male and female home ranges in MGRS (DLSM: ERS males –females = 0.35 ± 0.15, t417 = 2.30, one-tailed p = 0.049; MGRS males – females = 0.07 ± 0.08, t299 = 0.83, p = 0.84).
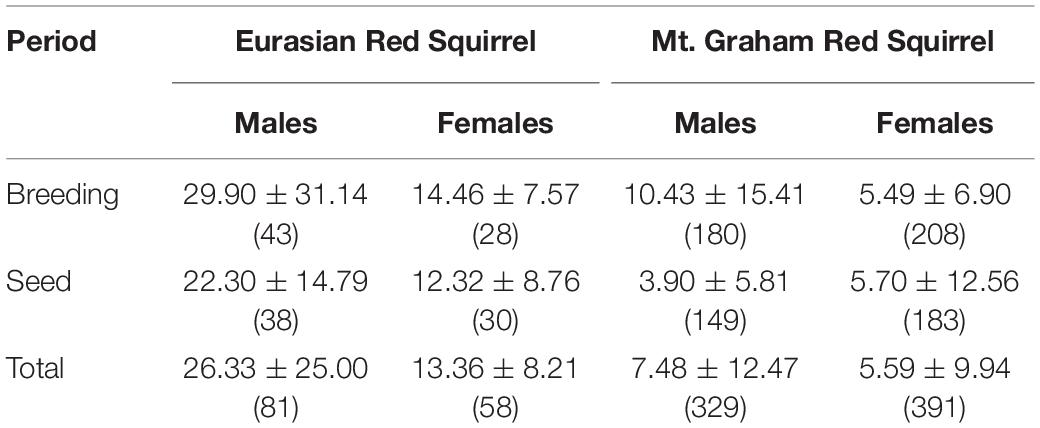
Table 1. Average home range size (ha, mean ± SD, sample size between brackets) of male and female Eurasian red squirrels and Mt. Graham red squirrels per season (breeding and seed) and independent of season (Total).
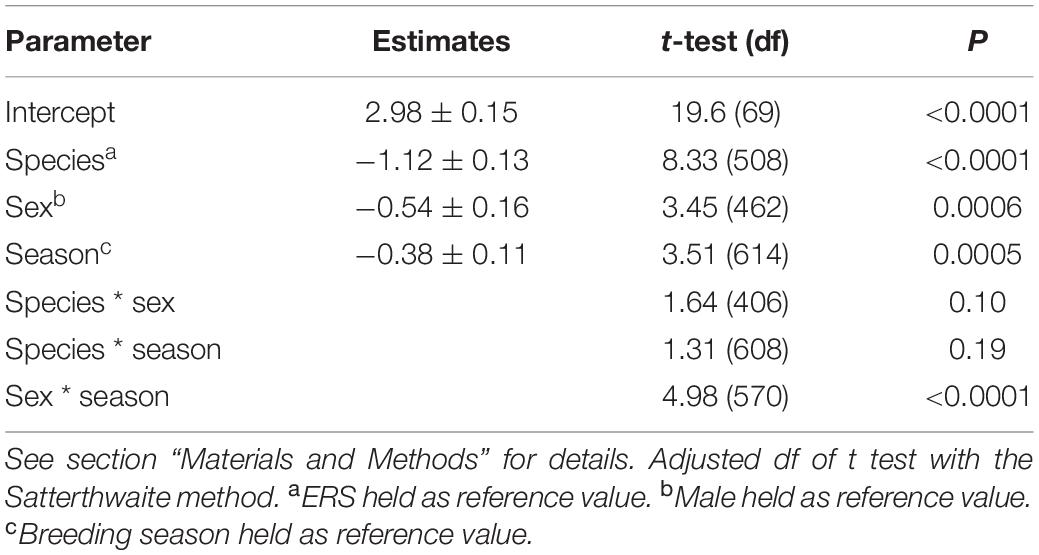
Table 2. Linear mixed model parameter estimates for important drivers of squirrel home range size (ln transformed).
Home range size was influenced by season in MGRS but not in ERS. Within-species comparisons of seasonal variation in home range size showed that ERS did not use larger breeding ranges than autumn (seed season) ranges (DLSM = 0.18 ± 0.10, t612 = 1.80, p = 0.27), but in MGRS, breeding ranges were much larger than autumn ranges (DLSM = 0.33 ± 0.11, t562 = 7.71, p < 0.0001) (Table 1). MGRS males and females respond differently to the breeding period as indicated by a significant sex by season interaction. Male MGRS enlarge their home ranges in the breeding season compared to seed season (DLSM = 0.45 ± 0.07, t602 = 6.67, p < 0.0001), and females do not (DLSM = 0.06 ± 0.07, t581 = 0.94, p = 0.78). Consequently, males use larger home ranges than females in the breeding season, but not in the (autumn) seed season (DLSM males vs. females breeding season = 0.40 ± 0.09, t481 = 4.25, p = 0.0002; seed season = 0.02 ± 0.10, t503 = 0.19, p = 0.99). Note that this effect is mainly due to MGRS whose males leave their territories for mating opportunities in the breeding season (Table 1).
The model with the species as independent variable showed a better fit than the model with body mass for the core are size (difference in LogLikelihood = 37.65, df = 1, p < 0.0001). Differences between the species, sexes, and seasons, and the above-described interactions persist when using 85% KDEadj core area size (see Supplementary Material 3). Overall, core areas of male ERS were about four times larger than of male MGRS; those of female ERS were about two to three times larger than of female MGRS.
Core-Area Overlap
We used data from 1938 home range core overlaps from 408 different squirrels over a 16-year period. The type III ANOVA of the LMM model revealed that ERS had higher core-area overlap than MGRS [Species effect F(1,443) = 39.2, p < 0.0001; Table 3]. The sex of the individual by sex of overlapper effect was significant [male by males (MM), male by females (MF), female by males (FM), and female by females (FF)] [F(3,1286) = 22.5, p < 0.0001] although the season effect was not [F(1,1796) = 0.42, p = 0.52]. However, the interactions of species by sex of the individual by sex of overlapper [F(3,1326) = 31.6, p < 0.0001] and of season by sex of the individual by sex of overlapper [F(3,1619) = 3.03, p = 0.029] were statistically significant although the three-factor interaction was not [F(3,1619) = 2.40, p = 0.066]. Because of the species effect and significant interactions with species, we further analyzed the core-area overlap patterns for each species.
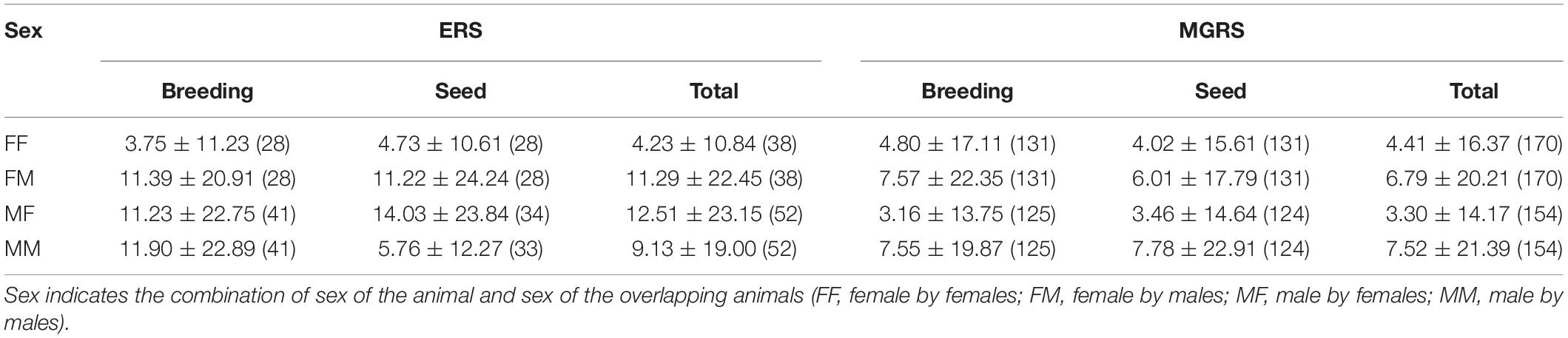
Table 3. Average core-area overlap (%, mean ± SD, sample size between parentheses) of male and female Eurasian red squirrels (ERS) and Mt. Graham red squirrels (MGRS) by season (breeding and seed) and independent of season (total).
In ERS, patterns of core-area overlap differed by sex and with season (Table 3 and SM 4.1). In the breeding season, female–female (FF) overlap was smaller than all other categories (DLSM, all ps < 0.001). In the seed season, FF overlap was smaller than female–male (FM) overlap (p < 0.0001), confirming intrasexual territoriality among adult female ERS. During the breeding season, ERS females were more strongly overlapped by males (FM) than males by males (MM) or males by females (MF) (DLSM, all ps < 0.001). During the seed season, there were no significant differences in proportion core-area overlap among the different combinations of the sexes (all ps > 0.05) except the abovementioned FF with FM.
In MGRS, percentage overlap of 85% KDE core areas was small and core-area overlap patterns between the sexes did not change with season (Table 3 and SM 4.2). Males had a higher core-area overlap with other males than with females (DLSM both seasons, all ps < 0.0001). Male–female (MF) overlap was also slightly smaller than female–female overlap (DLSM both seasons, all ps < 0.05; SM 4.2).
Discussion
In this study, we compared the space use of the Mt. Graham red squirrel and the Eurasian red squirrel, two species with similar body size and food resources but different foraging strategies and social organization. Because of the slight difference in body mass between the two species, we first tested the importance of body mass in explaining the variation in home range and core area size. The study by Tucker et al. (2014) predicted a much smaller difference than the threefold larger home ranges of ERS measured in this study. Note also that the home ranges used by both squirrel species were much larger than predicted values: on average, 21 ha or six times larger for ERS and 6.45 ha or three times larger for MGRS. The big discrepancy between the predicted and observed size of home ranges suggests how other factors, other than body mass and diet, could affect and define the space use of these species. Moreover, because of analysis of the models’ fit, body mass did not seem to specifically influence the variation in home range size, confirming a low importance of this trait in our study system and the comparable sizes of the two squirrels. We found, instead, that, although living in similar mixed-conifer ecosystems, the timing in food resource availability is very different between the western United States and the Italian Alps, and it has been a powerful selective force upon the social evolution and space use of the two squirrels. Masting, or the synchronous, episodic flood of resources, is a reproductive strategy in trees thought to have evolved as a co-evolutionary response to seed predation (Boutin et al., 2006; Steele, 2008). A slow process such as the physical environment and climate along with millennia in an active arms race with seed predator communities (e.g., Benkman, 2010) have driven divergent tree morphologies and life history strategies. In mixed-conifer forests of the western United States, such as on Mt. Graham, many conifers produce cones that ripen and release their seeds in autumn of the same year (Burns and Honkala, 1990). To capitalize on this highly ephemeral and pulsed resource (Boutin et al., 2006), squirrels in the genus Tamiasciurus clip the immature cones and cache them in middens before the cones open and release seeds. The moist microclimate of the midden preserves the closed cones, allowing access to a rich food supply throughout the winter and following spring (Smith and Mannan, 1994; Hurly and Lourie, 1997). In the Alps, cones from pine and spruce species do not open until mid or late April the following spring, permitting the ERS to feed on cones in the tree canopy during autumn and winter and feed on scatter-hoard cones (of pines) in late spring when no new cones are available in the trees (Wauters and Dhondt, 1987; Molinari et al., 2006). Hence, for ERS, food resources are more strongly spaced and cannot be defended against conspecifics, and this difference in food distribution and defensibility has ultimately led to the divergent space use patterns and social organization for the two squirrel species. The differences in resource timing, availability, and defensibility between the two forest ecosystems are reflected in species- and population-level patterns of home range size and home range overlap for the two squirrels, demonstrated by the fact that across sexes, MGRS had smaller, exclusive home ranges and core areas compared to ERS, supporting our first two predictions.
At the population and individual levels, resource availability, timing of breeding, and the sex of individuals can exert seasonal influences on home range size and exclusivity. Social interactions have an important role in shaping animal movements, especially during the breeding season, when one or both sexes might need to adapt their space use to encounter potential mates (Madison, 1980; Lazenby-Cohen and Cockburn, 1991; Van Beest et al., 2011). Thus, we hypothesized that home ranges would be smaller for both species in autumn when the new seed crop becomes available and larger for both species during the spring breeding season (prediction 4). During spring, we predicted that MGRS would range farther in search of mates, and ERS would range farther primarily in search of scatter-hoarded food. However, we found that only male MGRS increased their home range during the breeding season. In contrast, we did not find any seasonal variation in the home range sizes of either male or female ERS. The difference between the two species can be explained by their different social organization and mating behavior. Male ERS home ranges always overlap with several females to increase their chances of reproducing during the breeding season when three to five neighboring males engage in mating chases (Wauters et al., 1990). The dominant male tends to defend the estrus female from lower ranked competitors and obtains the majority of matings, but in some cases, females accept or even solicit copulations by subordinate males (promiscuous mating system with male-defense polygyny; Schwagmeyer, 1990; Wauters et al., 1990; Waterman, 2008). In contrast, male and female MGRS maintain nearly exclusive territories throughout the year with the exception of spring and summer breeding forays by males, wherein they temporarily leave their food-based territory to increase mating opportunities with neighboring estrus females (Koprowski et al., 2008; Lane et al., 2009). During the mating chase, many males can fight to obtain copulations with the estrous female: a scramble-competition mating system (Lane et al., 2009). This relationship between space use and mating system is demonstrated by male MGRS having larger home ranges than females during the breeding season (partially supporting predictions 3 and 5) although this pattern was not observed for ERSs.
Seasonality and sex of individuals in the population also influenced home range exclusivity as reflected in patterns of core-area overlap between sexes of both species. Analysis of core-area exclusivity also allowed us to test the degree to which species-specific social systems (territorial vs. non-territorial species) are upheld for the populations studied here. In general, core-area overlap among ERS was much higher than observed in MGRS except for ERS F-F overlap. Low intrasexual overlap among female ERS (F-F) supports marked female intra-sexual territoriality documented in previous studies (Wauters and Dhondt, 1992; Di Pierro et al., 2008; Romeo et al., 2010). Female ERS defend exclusive core areas against members of the same sex because their home range is strictly related to food resources and habitat quality. Defense of an exclusive core area is important for successful reproduction as only dominant females are capable of rearing offspring (Wauters and Dhondt, 1989, 1992). In MGRS, core-area overlap was consistently low across seasons. Although we found an increase of MGRS male home range size during the breeding period, the core-area size remained constant throughout the year for both sexes with little F-M overlap. The stable size of the core area can be explained by tree squirrel reproductive physiology and the scramble-competition mating system of MGRS. Female tree squirrels are in estrus for about 1 single day (often even less than 24 h) during a breeding season of 2–4 months. As a result, females are relatively asynchronous, and males appear to monitor reproductive status by olfaction (Gurnell, 1987; Arbetan, 1992; Steele and Koprowski, 2001). Dozens of males can be attracted to a female about 5 days before estrus, e.g., Thompson (1977), so the fast excursions of males into female territories may not affect core-area size and relative overlap because males keep their center of activity around their middens to defend the food resource from conspecifics (Gerhardt, 2005; Donald and Boutin, 2011). Thus, population-level patterns of territoriality in MGRS and non-territoriality in Italian ERS are similar to species-level patterns (prediction 2). Although seasonality and sex of individuals do contribute to variation in home range size and exclusivity at the population level, a long co-evolutionary history in ecosystems with different timing in and defensibility of resources appears to be the dominant driver in the divergent foraging, social, and mating systems of the two species. The differences in foraging, social, and mating systems, in turn, drive species-level differences in home range size and exclusivity.
We capitalized on long-term data sets (Arregoitia et al., 2018) available for two ecologically similar species allowing us to test the importance of individual-, population-, and species-level ecological drivers (McLoughlin and Ferguson, 2000) of home range size and exclusivity. Our models took into account the heterogeneity of our data set with larger sample sizes over a longer time period for the MGRS to avoid any bias in our results. Moreover, because of the important role of climate and food resources in shaping animal movements over short and long periods of time (e.g., Lurz et al., 2000; Van Beest et al., 2011; Morellet et al., 2013), our models considered possible annual fluctuations. Although tree species are different, the two forest ecosystems produce similar food items in terms of range in cone and seed size and range in energy content, and yearly fluctuations in seed production are of the same order of magnitude in both the Alps and Mt. Graham (Figure 2; see also Supplementary Material 1). In future studies, we will test how annual fluctuations in conifer seed production affect home range size of both species over time and relate annual variation in home range sizes to temporal trends in climate, habitat disturbance, and habitat loss.
Conclusion
Tree-life history strategies dictate the availability and predictability of resources – strong selective forces that have contributed to divergent foraging and social systems among tree squirrels (e.g., territorial vs. non-territorial; larder hoarding vs. scatter-hoarding) (Smith, 1970; Steele, 2008). Such species-level constraints on spacing and foraging are ultimately reflected in species-specific patterns of home range size and exclusivity (McLoughlin and Ferguson, 2000) and contribute to observed deviations from the mean home range–body mass scaling relationship for ecologically similar species of slightly different body size.
Although faster processes, such as seasonality and annual fluctuations in the availability and quality of resources at multiple scales, are important drivers of individual- and population-level home range dynamics (Maher and Lott, 2000; McLoughlin et al., 2001; Van Beest et al., 2011), it is important to consider the co-evolutionary history of species within their respective ecosystems to understand higher-level constraints on home range size, foraging strategy, and social systems (Ofstad et al., 2016). Processes such as the co-evolution between animals and their resource base and competition for resources (Connell, 1980; Benkman, 2010) lead to niche differentiation and speciation and are likely the ultimate factors driving the variation in home range size for ecologically similar species of comparable mass.
Data Availability Statement
The datasets generated for this study are available on request to the corresponding author.
Ethics Statement
The animal study was reviewed and approved by the all field work on Mt. Graham red squirrels was conducted under the University of Arizona Institutional Animal Care and Use Committee protocol # 08-024, Arizona Game and Fish Department scientific collecting permit # SP654189, U.S. Fish and Wildlife Service permit # TE041875-0 and adhered to the American Society of Mammalogists guidelines for the use of wild mammals in research (Sikes et al., 2016). All field work on Eurasian red squirrels in Italy was conducted by the University of Insubria staff under the permits: decree 1488 of 14/07/2000, decree 7489 of 29/04/2002, decree 5663 of 05/04/2004, decree 2456 of 07/03/2006, and decree 1702 of 23/02/2009 of Direzione Generale Agricoltura of the Lombardy Region, Italy.
Author Contributions
LW, AM, and JK collaborated to develop the framework and ideas in this manuscript, managed, and obtained funding for long-term ecological studies, and gave final approval for publication. FB, LW, MJM, and MVM compiled the long-term data sets. FB and MVM analyzed the data. MVM, MJM, and LW wrote the manuscript. All authors contributed to the article and approved the submitted version.
Funding
This research was supported by grants to JK from the University of Arizona, USDA Forest Service Rocky Mountain Research Station #10-JV-188, Coronado National Forest, Arizona Game & Fish Department (#I10010, # I13003, and #I16002), U.S. Fish & Wildlife Service, the Arizona Agricultural Experiment Station, and grants to LW and AM from Province of Sondrio, Stelvio National Park and Project COFIN 2003 (# 2003053710-006) of the Italian Ministry of Education, University and Research (MIUR).
Conflict of Interest
The authors declare that the research was conducted in the absence of any commercial or financial relationships that could be construed as a potential conflict of interest.
Acknowledgments
We would like to thank the Mt. Graham Red Squirrel Research Program graduate and undergraduate research assistants and V. L. Greer for invaluable help in the field, for data collection, and data management. We also thank Ambrogio Molinari and the ASPER project graduate and undergraduate students for their help with fieldwork. We thank two reviewers that with their time and critical reading have improved the initial version of this manuscript.
Supplementary Material
The Supplementary Material for this article can be found online at: https://www.frontiersin.org/articles/10.3389/fevo.2020.00174/full#supplementary-material
References
Arbetan, P. (1992). The Mating System of the Red Squirrel, Tamiasciurus Hudsonicus. Doctoral dissertation, University of Kansas, Kansas.
Arregoitia, L. D. V., Cooper, N., and D’Elía, G. (2018). Good practices for sharing analysis-ready data in mammalogy and biodiversity research. Hystrix. Ital. J. Mammal. 29, 155–161. doi: 10.4404/hystrix-00133-2018
Benkman, C. W. (2010). Diversifying coevolution between crossbills and conifers. Evol. Educ. Outreach 3, 47–53. doi: 10.1007/s12052-009-0190-8
Boutin, S., Wauters, L. A., McAdam, A. G., Humphries, M. M., Tosi, G., and Dhondt, A. A. (2006). Anticipatory reproduction and population growth in seed predators. Science 314, 1928–1930. doi: 10.1126/science.1135520
Burns, R. M., and Honkala, B. H. (1990). Silvics of North America Volume 1. Conifers. Washington, D.C: United States Department of Agriculture Forest Service Agriculture Handbook, 654.
Burt, W. H. (1943). Territoriality and home range concepts as applied to mammals. J. Mammal. 24, 346–352.
Connell, J. H. (1980). Diversity and the coevolution of competitors, or the ghost of competition past. Oikos 35:131. doi: 10.2307/3544421
Di Pierro, E., Molinari, A., Tosi, G., and Wauters, L. A. (2008). Exclusive core areas and intrasexual territoriality in Eurasian red squirrels (Sciurus vulgaris) revealed by incremental cluster polygon analysis. Ecol. Res. 23, 529–542. doi: 10.1007/s11284-007-0401-0
Donald, J. L., and Boutin, S. (2011). Intraspecific cache pilferage by larder-hoarding red squirrels (Tamiasciurus hudsonicus). J. Mammal. 92, 1013–1020. doi: 10.1644/10-mamm-a-340.1
Gerhardt, F. (2005). Food pilfering in larder-hoarding red squirrels (Tamiasciurus hudsonicus). J. Mammal. 86, 108–114. doi: 10.1644/1545-15422005086<0108:FPILRS<2.0.CO;2
Hope, A. G., Malaney, J. L., Bell, K. C., Salazar-Miralles, F., Chavez, A. S., Barber, B. R., et al. (2016). Revision of widespread red squirrels (genus: Tamiasciurus) highlights the complexity of speciation within North American forests. Mol. Phylogenet. Evol. 100, 170–182. doi: 10.1016/j.ympev.2016.04.014
Hurly, T. A., and Lourie, S. A. (1997). Scatterhoarding and larderhoarding by red squirrels: size, dispersion, and allocation of hoards. J. Mammal. 78, 529–537. doi: 10.2307/1382904
Jetz, W., Carbone, C., Fulford, J., and Brown, J. H. (2004). The scale of animal space use. Science 356, 266–268. doi: 10.1126/science.1102138
Kemp, G. A., and Keith, L. B. (1970). Dynamics and regulation of red squirrel (Tamiasciurus hudsonicus) populations. Ecology 51, 763–779. doi: 10.2307/1933969
Koprowski, J. L. (2002). Handling tree squirrels with a safe and efficient restraint. Wildl. Soc. Bull. 30, 101–103.
Koprowski, J. L. (2005). Annual cycles in body mass and reproduction of endangered Mt. Graham red squirrels. J. Mammal. 86, 309–313. doi: 10.1644/bwg-232.1
Koprowski, J. L., King, S. R. B., and Merrick, M. J. (2008). Expanded home ranges in a peripheral population: space use by endangered Mt. Graham red squirrels. Endanger. Species Res. 4, 227–232. doi: 10.3354/esr00026
Lane, J. E., Boutin, S., Gunn, M. R., and Coltman, D. W. (2009). Sexually selected behaviour: red squirrel males search for reproductive success. J. Anim. Ecol. 78, 296–304. doi: 10.1111/j.1365-2656.2008.01502.x
Lazenby-Cohen, K. A., and Cockburn, A. (1991). Social and foraging components of the home range in Antechinus stuartii (Dasyuridae: Marsupialia). Aust. J. Ecol. 16, 301–307. doi: 10.1111/j.1442-9993.1991.tb01058.x
Loy, A., Aloise, G., Ancillotto, L., Angelici, F., Bertolino, S., Capizzi, D., et al. (2019). Mammals of Italy: an annotated checklist. Hystrix Ital. J. Mammal. 30, 87–106. doi: 10.4404/hystrix-00196-2019
Lurz, P. W. W., Garson, P. J., and Wauters, L. A. (2000). Effects of temporal and spatial variations in food supply on the space and habitat use of red squirrels (Sciurus vulgaris). J. Zool. 251, 167–178. doi: 10.1017/S0952836900006038
Madison, D. M. (1980). Space use and social structure in meadow voles. Microtus pennsylvanicus. Behav. Ecol. Sociobiol. 7, 65–71. doi: 10.1007/BF00302520
Maher, C. R., and Lott, D. F. (2000). A review of ecological determinants of territoriality within vertebrate species. Am. Midl. Nat. 143, 1–29. doi: 10.1674/0003-0031
McLoughlin, P. D., and Ferguson, S. H. (2000). A hierarchical pattern of limiting factors helps explain variation in home range size. Écoscience 7, 123–130. doi: 10.1080/11956860.2000.11682580
McLoughlin, P. D., Ferguson, S. H., and Messier, F. (2001). Intraspecific variation in home range overlap with habitat quality: a comparison among brown bear populations. Evol. Ecol. 14, 39–60. doi: 10.1023/a:1011019031766
Molinari, A., Wauters, L. A., Airoldi, G., Cerinotti, F., Martinoli, A., and Tosi, G. (2006). Cone selection by Eurasian red squirrels in mixed conifer forests in the Italian Alps. Acta Oecol. 30, 1–10. doi: 10.1016/j.actao.2005.11.004
Moorcroft, P. R. (2012). Mechanistic approaches to understanding and predicting mammalian space use: recent advances, future directions. J. Mammal. 93, 903–916. doi: 10.1644/11-MAMM-S-254.1
Morellet, N., Bonenfant, C., Börger, L., Ossi, F., Cagnacci, F., Heurich, M., et al. (2013). Seasonality, weather and climate affect home range size in roe deer across a wide latitudinal gradient within Europe. J. Anim. Ecol. 82, 1326–1339. doi: 10.1111/1365-2656.12105
Munroe, K. E., Koprowski, J. L., and Greer, V. L. (2009). “Reproductive ecology and home range size of red squirrels: do Mt. Graham red squirrels fit the pattern?,” in The Last Refuge of the Mt. Graham Red Squirrel, eds H. R. Sanderson and J. L. Koprowski (Tucson: The University of Arizona Press), 287–298.
O’Connor, C. D., Falk, D. A., Lynch, A. M., and Swetnam, T. W. (2014). Fire severity, size, and climate associations diverge from historical precedent along an ecological gradient in the Pinaleño Mountains. Arizona, USA. For. Ecol. Manag. 329, 264–278. doi: 10.1016/j.foreco.2014.06.032
Ofstad, E. G., Herfindal, I., Solberg, E. J., and Sæther, B. E. (2016). Home ranges, habitat and body mass: simple correlates of home range size in ungulates. Proc. Biol. Sci. 283:20161234. doi: 10.1098/rspb.2016.1234
Preatoni, D. G., and Bisi, F. (2013). HRTools: Commodity Functions for Home Range Calculation. Available online at: https://r-forge.r-project.org/R/?group_id=1531 (accessed April 10, 2020).
Romeo, C., Wauters, L. A., Preatoni, D., Tosi, G., and Martinoli, A. (2010). Living on the edge: space use of Eurasian red squirrels in marginal high-elevation habitat. Acta Oecol. 36, 604–610. doi: 10.1016/j.actao.2010.09.005
Rusch, D. A., and Reeder, W. G. (1978). Population ecology of Alberta red squirrels. Ecology 59, 400–420. doi: 10.2307/1936382
Salmaso, F., Molinari, A., Di Pierro, E., Ghisla, A., Martinoli, A., Preatoni, D., et al. (2009). Estimating and comparing food availability for tree-seed predators in typical pulsed-resource systems: Alpine conifer forests. Plant Biosyst. 143, 258–267. doi: 10.1080/11263500902722451
Schwagmeyer, P. L. (1990). “Ground squirrel reproductive behavior and mating competition: a comparative perspecitve,” in Contemporary Issues in Comparative Psychology, ed. D. A. Dewsbury (Sunderland, MA: Sinauer Associates, Inc), 175–196. doi: 10.1037/11525-008
Sikes, R. S., and Gannon, W. L., Animal Care and Use Committee of the American Society of Mammalogists. (2016). Guidelines of the American Society of Mammalogists for the use of wild mammals in research. J. Mammal. 92, 235–253. doi: 10.1644/10-MAMM-F-355.1
Smith, A. A., and Mannan, R. W. (1994). Distinguishing characteristics of Mount Graham red squirrel midden sites. J. Wildl. Manage. 58, 437–445.
Smith, C. C. (1970). The coevolution of pine squirrels (Tamiasciurus) and conifers. Ecol. Monogr. 40, 349–371. doi: 10.2307/1942287
Spector, S. (2002). Biogeographic crossroads as priority areas for biodiversity conservation. Conserv. Biol. 16, 1480–1487. doi: 10.1046/j.1523-1739.2002.00573.x
Stamps, J. (1995). Motor learning and the value of familiar space. Am. Nat. 146, 41–58. doi: 10.1086/285786
Steele, M. A. (2008). Evolutionary interactions between tree squirrels and trees: a review and synthesis. Curr. Sci. 95, 871–877.
Steele, M. A., and Koprowski, J. L. (2001). North American Tree Squirrels. Washington, DC: Smithsonian Institution Press.
Tamburello, N., Côté, I. M., and Dulvy, N. K. (2015). Energy and the scaling of animal space use. Am. Nat. 186, 196–211. doi: 10.1086/682070
Thomas, L. S., Teich, E., Dausmann, K. H., Reher, S., and Turner, J. M. (2018). Degree of urbanisation affects Eurasian red squirrel activity patterns. Hystrix, Ital. J. Mammal. 29, 175–180. doi: 10.4404/hystrix-00065-2018
Thompson, D. C. (1977). Reproductive behavior of the grey squirrel. Can. J. Zool. 55, 1176–1184. doi: 10.1139/z77-152
Trizio, I., Crestanello, B., Galbusera, P., Wauters, L. A., Tosi, G., Matthysen, E., et al. (2005). Geographical distance and physical barriers shape the genetic structure of Eurasian red squirrels (Sciurus vulgaris) in the Italian Alps. Mol. Ecol. 14, 469–481. doi: 10.1111/j.1365-294X.2005.02428.x
Tucker, M. A., Ord, T. J., and Rogers, T. L. (2014). Evolutionary predictors of mammalian home range size: body mass, diet and the environment. Glob. Ecol. Biogeogr. 23, 1105–1114. doi: 10.1111/geb.12194
Van Beest, F. M., Rivrud, I. M., Loe, L. E., Milner, J. M., and Mysterud, A. (2011). What determines variation in home range size across spatiotemporal scales in a large browsing herbivore? J. Anim. Ecol. 80, 771–785. doi: 10.1111/j.1365-2656.2011.01829.x
Waterman, J. M. (2008). “Male mating strategies in rodents,” in Rodent Societies: An Ecological & Evolutionary Perspective, eds J. O. Wolff and P. W. Sherman (Chicago, IL: University of Chicago Press), 27–41.
Wauters, L., and Dhondt, A. (1995). Lifetime reproductive success and its correlates in female Eurasian red squirrels. Oikos 72:402. doi: 10.2307/3546126
Wauters, L., and Dhondt, A. A. (1989). Body weight, longevity and reproductive success in red squirrels (Sciurus vulgaris). J. Anim. Ecol. 58, 637–651.
Wauters, L., and Dhondt, A. A. (1992). Spacing behaviour of red squirrels. Sciurus vulgaris: variation between habitats and the sexes. Anim. Behav. 43, 297–311. doi: 10.1016/S0003-3472(05)80225-8
Wauters, L., Dhondt, A. A., and De Vos, R. (1990). Factors affecting male mating success in red squirrels (Sciurus vulgaris). Ethol. Ecol. Evol. 2, 195–204. doi: 10.1080/08927014.1990.9525486
Wauters, L., Githiru, M., Bertolino, S., Molinari, A., Tosi, G., and Lens, L. (2008). Demography of alpine red squirrel populations in relation to fluctuations in seed crop size. Ecography 31, 104–114. doi: 10.1111/j.2007.0906-7590.05251.x
Wauters, L. A., and Dhondt, A. A. (1987). Activity budget and foraging behaviour of the red squirrel (Sciurus vulgaris Linnaeus, 1758) in a coniferous habitat. Zeitschrift Säugetierkd 52, 341–353.
Wauters, L. A., Gurnell, J., Preatoni, D., and Tosi, G. (2001). Effects of spatial variation in food availability on spacing behaviour and demography of Eurasian red squirrels. Ecography 24, 525–538. doi: 10.1111/j.1600-0587.2001.tb00487.x
Wauters, L. A., Preatoni, D. G., Molinari, A., and Tosi, G. (2007a). Radio-tracking squirrels: performance of home range density and linkage estimators with small range and sample size. Ecol. Modell. 202, 333–344. doi: 10.1016/j.ecolmodel.2006.11.001
Wauters, L. A., Vermeulen, M., Van Dongen, S., Bertolino, S., Molinari, A., Tosi, G., et al. (2007b). Effects of spatio-temporal variation in food supply on red squirrel Sciurus vulgaris body size and body mass and its consequences for some fitness components. Ecography 30, 51–65. doi: 10.1111/j.2006.0906-7590.04646.x
Keywords: conifer-squirrel coevolution, home range size variation, Eurasian red squirrel, Mt. Graham red squirrel, foraging strategy, producer–consumer dynamics
Citation: Mazzamuto MV, Merrick MJ, Bisi F, Koprowski JL, Wauters L and Martinoli A (2020) Timing of Resource Availability Drives Divergent Social Systems and Home Range Dynamics in Ecologically Similar Tree Squirrels. Front. Ecol. Evol. 8:174. doi: 10.3389/fevo.2020.00174
Received: 23 January 2020; Accepted: 15 May 2020;
Published: 25 June 2020.
Edited by:
Jane Waterman, University of Manitoba, CanadaReviewed by:
Jeff Bowman, Ontario Ministry of Natural Resources, CanadaAtilla Ferreguetti, Rio de Janeiro State University, Brazil
Copyright © 2020 Mazzamuto, Merrick, Bisi, Koprowski, Wauters and Martinoli. This is an open-access article distributed under the terms of the Creative Commons Attribution License (CC BY). The use, distribution or reproduction in other forums is permitted, provided the original author(s) and the copyright owner(s) are credited and that the original publication in this journal is cited, in accordance with accepted academic practice. No use, distribution or reproduction is permitted which does not comply with these terms.
*Correspondence: Maria Vittoria Mazzamuto, bXZtYXp6YW11dG9AYXJpem9uYS5lZHU=