- Applied Ecology and Phycology, Institute of Biological Science, University of Rostock, Rostock, Germany
Global warming, as global problem, may particularly affect the vegetation of the Polar Regions. Biological soil crusts (BSCs) as pioneer communities perform a variety of important ecological functions under the harsh environmental conditions at high latitudes. The green algal genus Klebsormidium is a common member of BSCs and in the present study, the ecophysiological resilience to temperature stress of 20 strains from Arctic and Antarctica were investigated. All 20 Klebsormidium strains exhibited the capability to grow under a wide temperature range (from 6 to 28°C) and hence were characterized as psychrotolerant with optimum growth temperatures between 18°C and 26°C. Statistical analyses showed no significant differences in optimum growth temperature. However, growth rates at optimal temperatures varied between strains and indicated infraspecific physiological plasticity. Furthermore, correlation with the sampling sites as well as different BSC types were examined but no significance was confirmed. Our results revealed that Polar Klebsormidium strains are able to survive such changing conditions, and even benefit from higher environmental temperatures.
Introduction
Biological soil crusts (BSCs) are complex agglomerations formed by diverse phototrophic and heterotrophic organisms such as microalgae, cyanobacteria, lichens, bryophytes, bacteria, microfungi and microfauna (Belnap, 2006; Darby and Neher, 2016). These pioneer communities constitute the dominant vegetation cover on the temporarily snow- and ice-free soil surfaces of the Polar Regions and perform a wealth of ecological functions (Yoshitake et al., 2010; Williams et al., 2016). BSCs stabilize soil against water and wind erosion as well as cryoturbation, contribute to primary production, carbon and nitrogen fixation and benefit the growth of vascular plants (Belnap, 2002; Eldridge and Greene, 1994; Evans and Johansen, 1999; Yoshitake et al., 2010). The filamentous green alga genus Klebsormidium (Klebsormidiophyceae, Streptophyta) can be found worldwide in numerous habitats (Elster et al., 2008; Škaloud and Rindi, 2013) and is also commonly associated with BSCs in both the Arctic and Antarctica (Hayashi and Shinozaki, 2012; Pushkareva et al., 2016; Borchhardt et al., 2017a, b; Rippin et al., 2018). This genus has a pivotal role in the formation of BSCs by its filamentous morphology as well as excretion of extracellular polymeric substances (EPS), which promote the moisture retention and stabilization of the soil surface (Barberousse et al., 2006; Hu et al., 2009; Büdel et al., 2016).
The Polar Regions are characterized by harsh environmental conditions such as low temperatures, a pronounced seasonality, scarcity of liquid water and strong solar radiation, and Klebsormidium has the potential to cope with these stressors (Elster et al., 2008; Karsten and Rindi, 2010; Kaplan et al., 2012; Holzinger and Karsten, 2013; Kitzing et al., 2014). The ecophysiology of Klebsormidium has been studied on several strains from different biogeographically regions (e.g., Holzinger et al., 2014; Herburger and Holzinger, 2015; Blaas and Holzinger, 2017; Donner et al., 2017). It is known that members are poikilohydric, which describes the inability to actively regulate cellular water content. Therefore, organisms nearly completely dehydrated (Holzinger et al., 2014; Karsten and Holzinger, 2014). The electron transport chains in the thylakoid membranes are affected by cellular desiccation and inhibit photosynthesis (De Winder et al., 1990; Gray et al., 2007; Fernández-Marín et al., 2016). However, studies on Arctic and Antarctic Klebsormidium strains showed resistance against desiccation induced injuries and investigation on alpine strain of Klebsormidium crenulatum revealed molecular mechanisms such as up-regulation of photosynthetic transcripts caused by dehydration (Elster et al., 2008; Holzinger et al., 2014). Furthermore, strains accumulate osmolytes (raffinose and sucrose), free amino acids (γ-aminobutyric acid) and starch grains and oil droplets in order to retain water within the cells and protect against membrane damage and protein aggregation, which also caused by freezing (Bisson and Kirst, 1995; Manabu et al., 2008; Holzinger et al., 2014). However, the knowledge on the capabilities of Polar Klebsormidium strains to cope with increasing temperatures due to the climate change is insufficient.
Climate change is already happening. An increase of annual mean temperature of more than 1°C was determined in the Polar Regions in the last decades and the atmospheric temperature in the Artic has doubled since 1980 (Kejna et al., 2013). Mean air temperature of Antarctic King George Island was −4.3°C in 1959, while only −1.1°C was measured in 2008 (Kejna et al., 2013). It is presumed that the BSCs of the Polar Regions cannot resist the rapid climate change, and hence their composition as well as distribution will shift or BSCs will even be displaced by invasive species (Frenot et al., 2005; Pushkareva et al., 2016). Previous results of a pedological analysis of polar BSC species showed no significant influences of C, N, S, TP (total phosphorus) and pH. In addition, a statistically significant influence of precipitation on species composition was determined (Borchhardt et al., 2017b). Therefore, studies on ecophysiological performance of Polar strains not only merit a particular attention but also urgently needed. Findings, whether and how Artic and Antarctic Klebsormidium strains cope with higher temperatures, might enable to predict the development of this key vegetation in the Polar Regions under the climate change scenarios.
Materials and Methods
Study Sites
Biological soil crusts samples were collected at Svalbard (Arctic) and King George Island as well as Ardley Island (South Shetland Islands, Antarctic Peninsula, Figure 1 and Table 1). Crust material was cut out of the soil surface using plastic petri dishes (60 mm ∅) and a spatula. BSCs were air dried with open lids to avoid mold growth and stored in paper bags at a dark place.
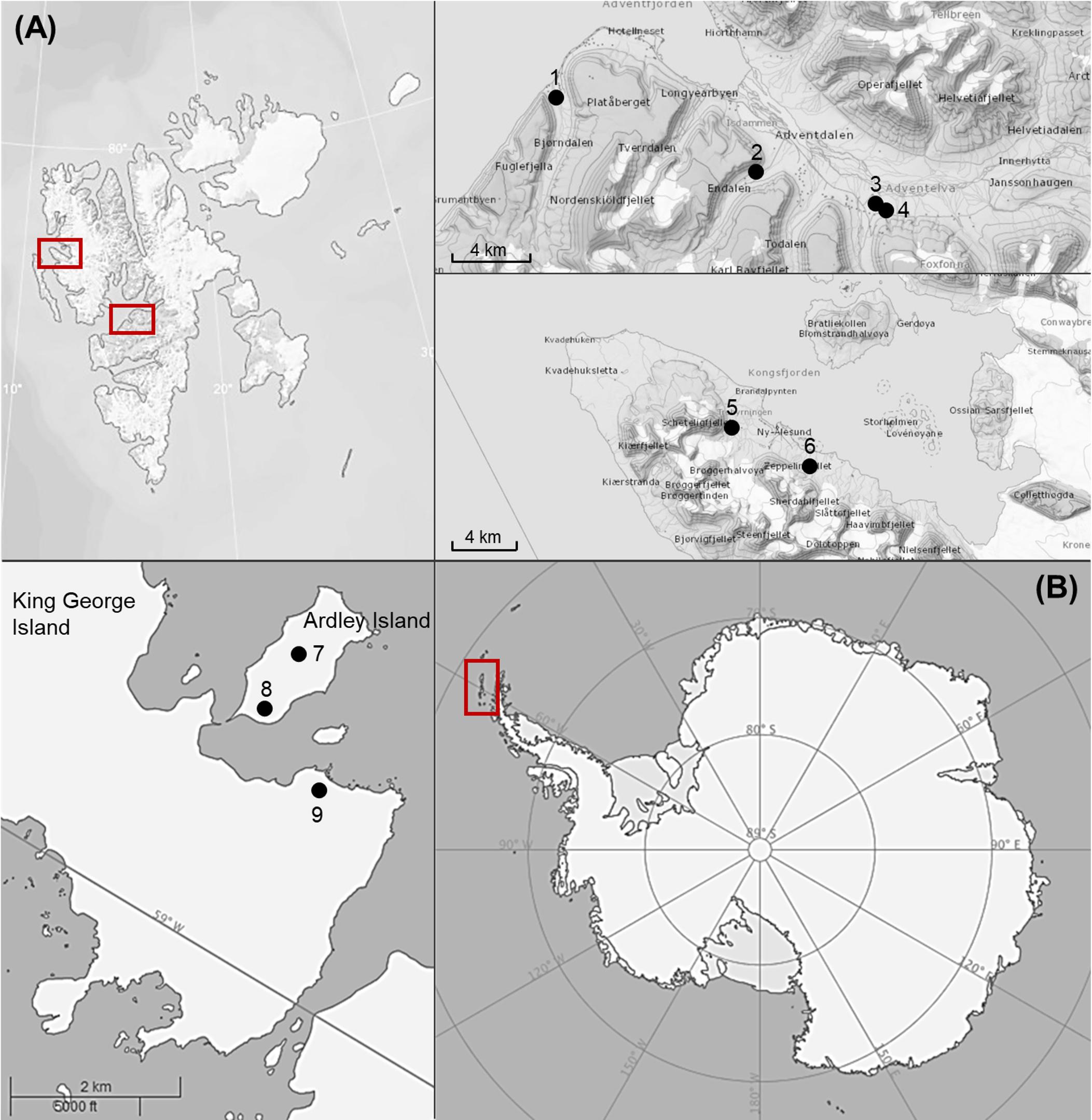
Figure 1. Map of sampling areas for collected biological soil crusts. (A) The two investigated sampling localities on Arctic Svalbard (around Ny-Alesund and Longyearbyen), (B) The two investigated sampling localities on Antarctic South Shetland Islands (Ardley and King George Island). Numbers of sampling stations are explained in Table 1.
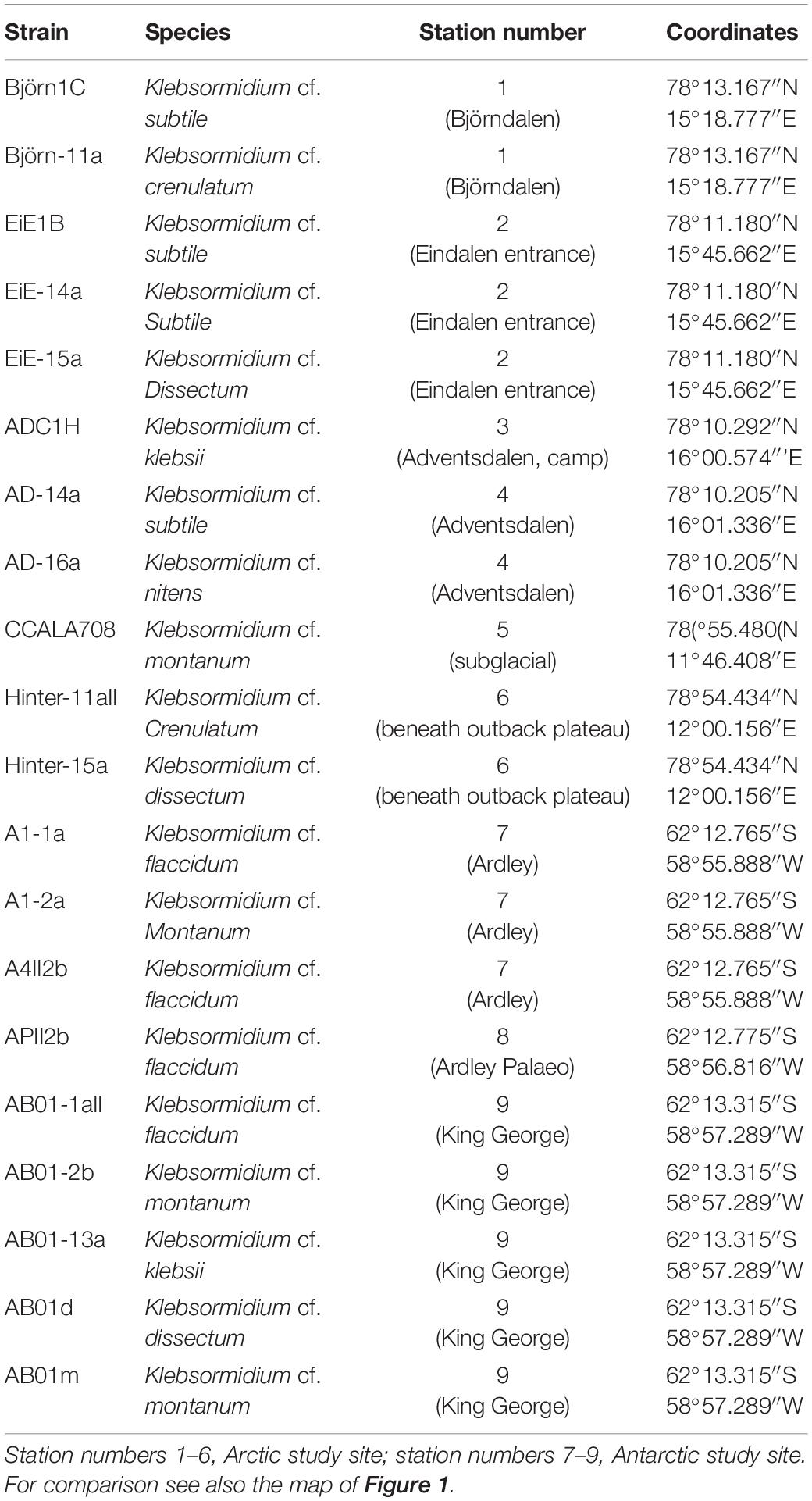
Table 1. Overview of used Klebsormidium strains including sampling stations on Arctic Svalbard and Antarctic King George and Ardley Island.
The archipelago Svalbard located in the Arctic Ocean ranges from 74 to 81° north latitude and from 10 to 35° east longitude. Svalbard has a mild climate compared to regions at the same latitudes because of the West Spitsbergen Current (WSC), which transports warm Atlantic water masses into the Arctic Ocean along the West coast. The mean temperature in summer is 7 and −14°C (minimum −35°C) in winter (Norwegian Meteorological Institute)1.
King George Island and the nearby Island Ardley belong to the South Shetland Islands, which are located in maritime Antarctica (61 to 63° south latitude, 54 to 63° west longitude). The archipelago is separated from the Antarctic Peninsula by the Bransfield Strait and from South America by the Drake Passage. Because of the Antarctic Circumpolar Current (ACC) the maritime Antarctica has milder climate with mean annual temperature of −2.5°C on King George (Kejna et al., 2013).
Algal Isolates and Cultivation
Biological soil crusts samples were cultured on 1.5% DifcoTM Agar (Becton Dickonson GmbH, Heidelberg, Germany) enriched with Bold’s basal medium with vitamins (Starr and Zeikus, 1993) modified by tripled nitrate concentration (3N-MBBM + V). To obtain unialgal isolates enrichment cultures were regularly screened for colonies using stereo microscope (ZS40, Olympus, Tokyo, Japan) with a magnification of 40-fold. Potential microalgal colonies were isolated from the enrichment cultures with a needle and transferred to a new agar plate. The growth of colonies was frequently monitored with the stereo microscope and several sub-isolates were generated for further purification. If no contamination with concomitant microalgae or fungi was detected the isolate was transferred to a new agar plate under sterile conditions. Strains were identified using light microscope (BX-51, Olympus, Tokyo, Japan) following the identification key of Ettl and Gärtner (2014) and descriptions by Rindi et al. (2011) as well as Mikhailyuk et al. (2014). Nine Antarctic Klebsormidium strains and eleven Arctic strains were isolated and part of the Culture Collection at the University of Rostock. One strain (CCALA 708) was delivered from the Culture Collection of Autotrophic Organisms (CCALA, Institute of Botany, Charles University, Tøeboò, Czechia).
Isolates were cultured at 15°C and 35 μmol photons m-2 s-1 (Daylight Lumilux Cool White lamps L36W/840, OSRAM Licht AG, Munich, Germany) under a 16:8 h light-dark cycle.
Growth in Response to Temperature
For in vivo measurement of the effect of 12 different temperatures on growth, as temperature gradient from 6, 8, 10, 12, 13, 14, 17, 18, 20, 23, 26 to 28°C, chlorophyll a fluorescence was used as a proxy for biomass accumulation according to Gustavs et al. (2009). Growth experiments were carried out using a modified self-constructed algal incubator (Kunststoff-Technik GmbH, Rostock, Germany) as described in Woelfel et al. (2014). with the possibility to create a temperature gradient at 35 μmol photons m-2 s-1 (LED) under a 16:8 light-dark cycle. All measurements were always done with log-phase cultures, which were pre-incubated at experimental conditions for 10 days. Pre-cultures were inoculated with 100 μl of liquid stock culture and were kept on 24 well plates. Each well was filled with 2.5 ml 3N-BBM-agar (Starr and Zeikus, 1993; BBM modified by the addition of triple nitrate concentration). After 10 days a small amount of pre-culture biomass was transferred in a new 24-well plate with four replicates for each strain. Chlorophyll a fluorescence was measured (excitation 440 nm, emission 680 nm, top read function) each day with a SpectraMax M2e multiplate reader (MPR; Molecular Devices, Biberach, Germany) using the software SoftMax Pro version 5.4 (Molecular Devices, LCC, San Jose, CA, United States). Dark-incubation of 10 min was performed in order to open all reaction centers of photosystem II before starting each measurement. Increasing fluorescence was detected as relative fluorescence units (RFUs) and the fluorescence measured directly after the inoculation serving as a starting value. Each strain was tested four times. Calculation of growth rate for each of the four replicates was performed according to the well-established protocol by Gustavs et al. (2009). Fluorescence Ft at a given time point t are calculated as Ft = F0eμt with F0 as initial fluorescence and μ (d–1) as growth rate in the respective time interval. For the calculation of μ the measured chlorophyll a fluorescence values were fitted with the equation described above. The fitting was based on the sum of the mean square error AFl which was calculated as AFl = (Ft–Ft,cal)2 with Ft (RFU) as fluorescence at a given point in time t and Ft,cal (RFU) as calculated fluorescence at a given point in time t with five subsequent fluorescence values. AFl was minimized by the Microsoft Excel add-in Solver with the model “GRG- non-linear.” Optimum growth rate was defined as growth rate values >80% according to Gustavs et al. (2010).
Multivariate Statistics
Multivariate analysis was conducted using the statistical programs PRIMER 6. Non-metric multi-dimensional scaling (MDS, Kruskal and Wish, 1978) was based on square root transformed data and Bray-Curtis similarity and the significance of similarity was tested by using ANOSIM permutation test (Clarke and Green, 1988; Clarke, 1993).
Results
The results showed a wide range in the capability to growth with increasing temperatures in all investigated Klebsormidium strains (6–28°C, Figure 2). All strains grew at minimum temperature of 6°C but only four strains were able to grow at the maximum temperature of 28°C (AD-16a K. nitens, EiE-15a K. dissectum, AB01-2b K. montanum, AB01-13a K. klebsii). Two growth patterns along the temperature gradient were observed and can be characterized as a plateau-like growth optimum and a more pronounced, sharp optimum. AB01-2b K. montanum, EiE-14a K. subtile, Hinter-11aII K. crenulatum, Hinter-15a K. dissectum, EiE-15a K. dissectum, and AD-16a K. nitens exhibited a plateau-like growth optimum, whereas EiE-1B K. subtile, Björn-1C K. subtile and AB01-1D K. dissectum showed a more pronounced, sharp optimum. Conspicuous differences in optimum growth temperature of K. montanum and K. dissectum strains indicated an infraspecific physiological plasticity. Growth rates at optimal temperatures of K. montanum varied between 18 and 26°C. Both strains were isolated from Antarctic BSCs. Similar individual growth pattern was observed in K. dissectum strains, which exhibit optimum growth temperatures between 18 and 23°C. All investigated K. dissectum strains were found in Arctic biocrust communities. An overview of the optimum growth temperatures of all strains is presented in Figure 3. The MDS analysis and ANOSIM test (global R value = 0.01, p = 0.424) show neither significant grouping by Polar Regions nor by species (Figure 4).
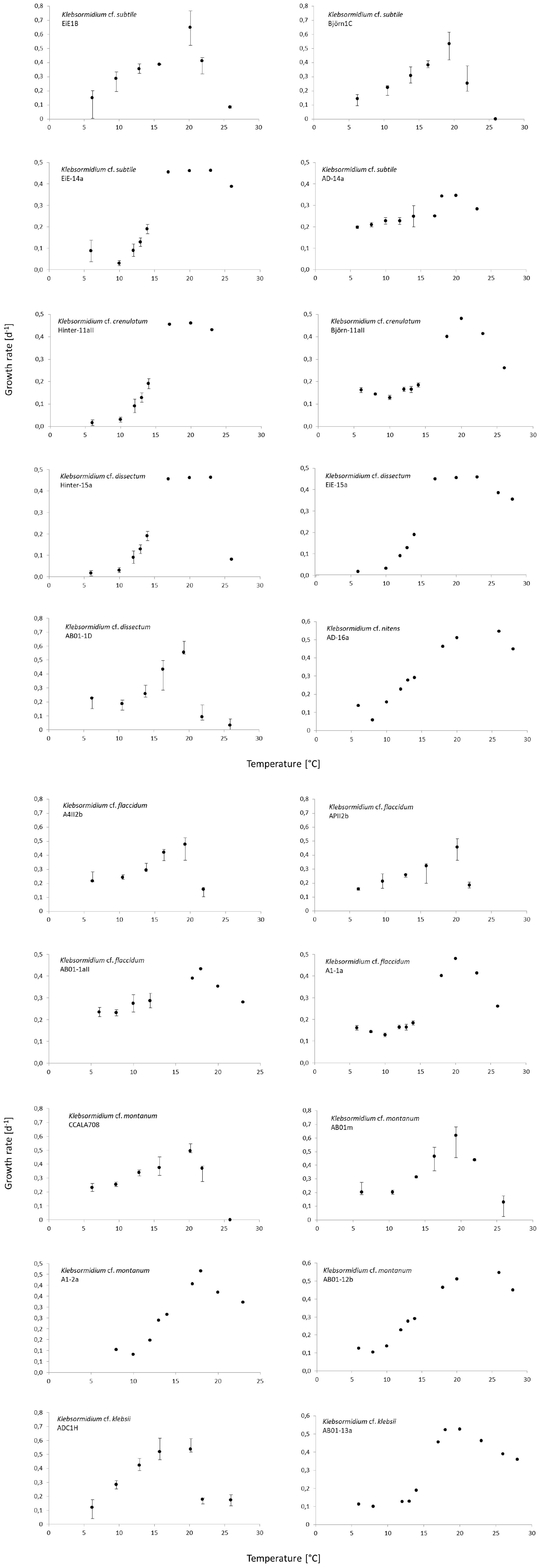
Figure 2. Median growth rate μ [d–1] as response against temperature of all 20 Klebsormidium strains. Error bars mark maximum and minimum growth rates of the four replicates for the respective temperature. The temperature range of a few strains were a little different, please keep in mind the y-axis.
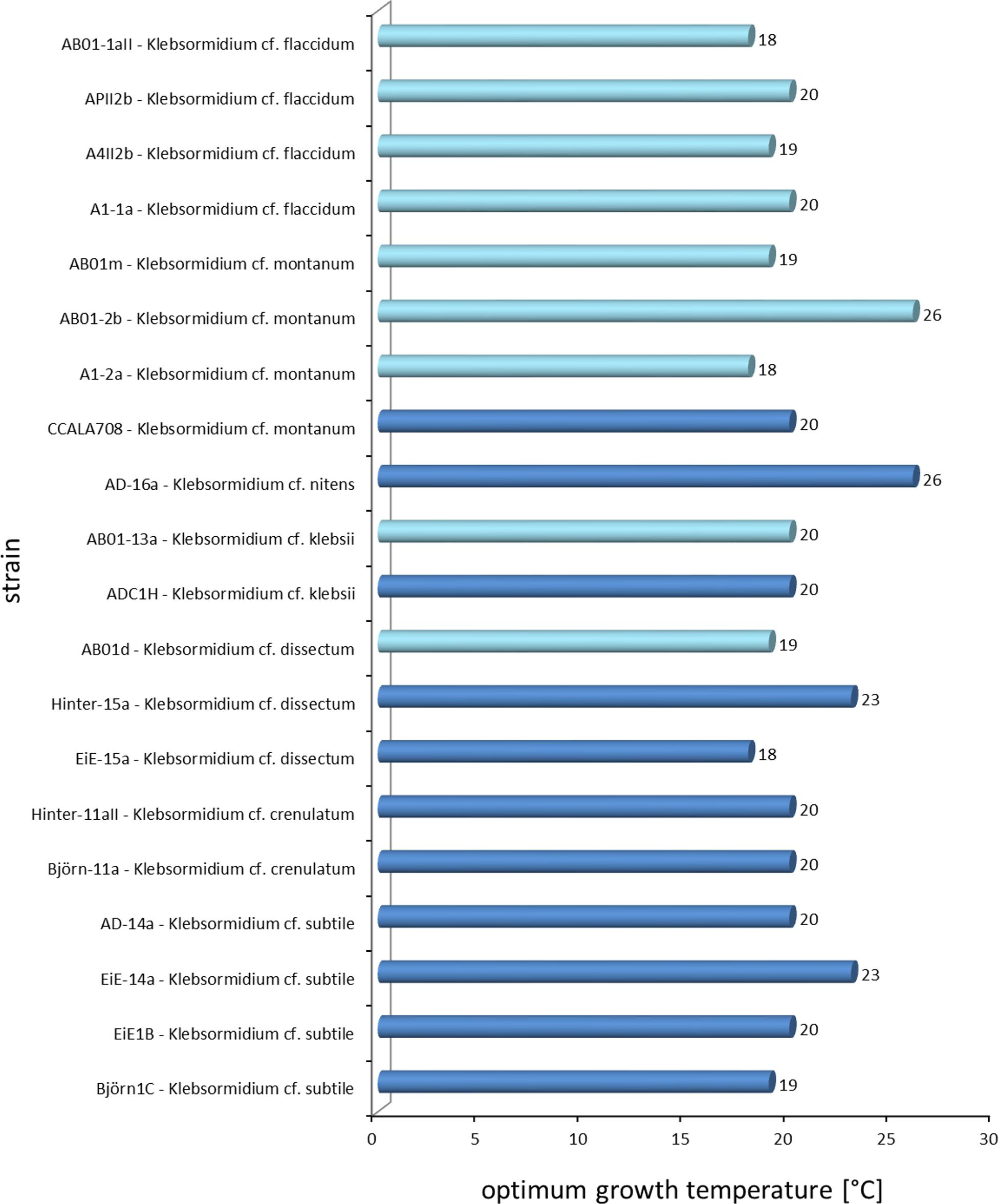
Figure 3. Overview of the optimum growth temperatures of all 20 Klebsormidium strains. Light blue = Antarctic strains, dark blue = Arctic strains.
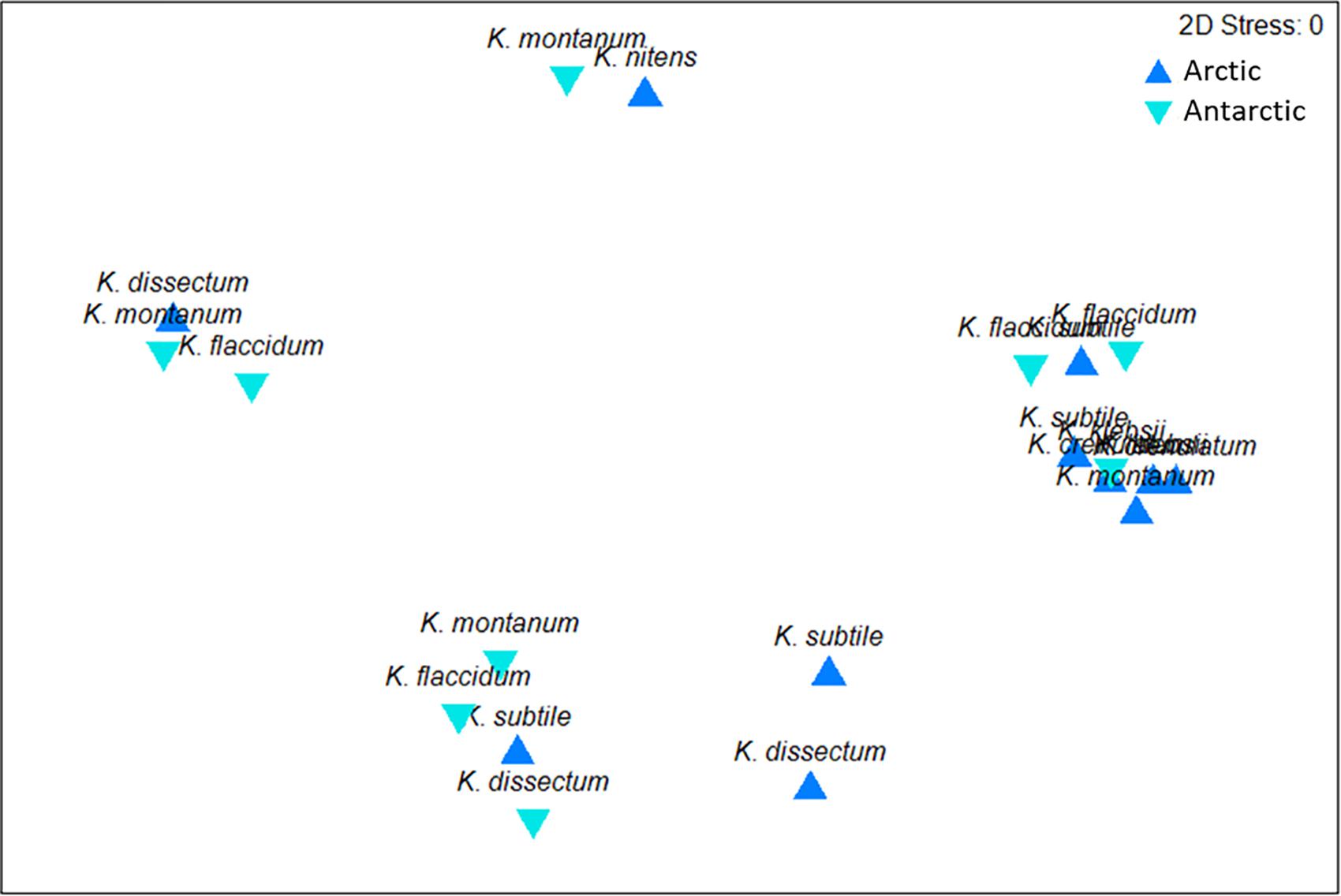
Figure 4. MDS plot based on square root transformed data and Bray-Curtis similarity. Comparison of Arctic and Antarctic Klebsormidium strains by their optimum growth temperatures. The stress value represents the quality of the graph (0 = perfect, 0.05 = good, 0.2 = poor).
Discussion
The investigated Polar Klebsormidium strains exhibited relatively high optimum growth temperatures between 18 and 26°C and hence, were characterized as rather psychrotolerant taxon than as a psychrophilic or even cryophilic alga. Psychrotolerant organisms grow optimally at 20 to 25°C but are able to survive at temperatures below 0°C (Morita, 1975). Psychrophiles sensu stricto have a growth optimum temperature at <15°C and cryophilic organisms prefer temperatures below −10°C (Morita, 1975; Elster, 1999). Both Arctic and Antarctic Klebsormidium strains showed wide ranges of temperatures. Ubiquitous distribution of Klebsormidium is known and it can be concluded that members seem to be ecophysiological generalists with eurythermal growth response pattern. Most of the strains exhibit optimum temperature of 20°C, which coincides with former literature values from the Alps (20°C, Karsten et al., 2010; Karsten and Holzinger, 2012). Furthermore, results presented in this study were comparable with findings on Klebsormidium strains from other biogeographical regions, such as America (median 19°C, Donner et al., 2017) and Germany (20°C, Karsten and Rindi, 2010), and strengthen the hypothesis. Further data on the temperature requirements for growth in both closely related genera of the Klebsormidiophyceae Interfilum, Entransia as well as Hormidiella and other green algae are rare or still missing.
The Arctic Svalbard has a more fluctuating climate with higher summer and lower winter temperatures compared to the Antarctic South Shetland Islands. Furthermore, noticeable environmental differences between the Arctic and Antarctica based on their cold water and low temperature history were reported (Zacher et al., 2009). Antarctica has a much longer cold water history of about 23 Mio. years, while the geological cold water history of the Arctic is still younger (ca. 2 Mio years, Sabbe et al., 2003). However, this environmental differences could not be linked to the findings presented in this study because there are no significant differences between Antarctic and Arctic strains. In addition, BSCs microalgae such as Klebsormidium have to cope with microclimatic factors, which might explain the local as well as infraspecific differences of optimum growth temperatures.
The differences between the optimum growth temperatures and the air temperatures in the Polar Regions (average 7°C in Svalbard) initially seem to be large. However, investigations on vegetation mats in Svalbard, which described potentially BSCs, revealed an increase by 35% of the temperatures within the vegetation mats (Coulson et al., 1993). These findings constitute a rise by about 5°C during summertime and caused by heat emission due to biological activity (Coulson et al., 1993). These higher temperatures within BSCs in general might explain the ascertained optimum growth temperatures of Klebsormidium strains.
A temperature increase up to 7.7°C is predicted for the Arctic region, which will lead to a decline of snow cover and glacier retreat. Our findings indicated that Polar Klebsormidium strains are able to survive such changing conditions. Consequently, it allows to presume that Polar Klebsormidium strains might benefit from higher environmental temperatures and colonies will increase. This is already indicated by the conspicuously high abundance of Klebsormidium species in Arctic as well as Antarctic BSCs (Elster et al., 1999; Kaštovská et al., 2005; Borchhardt et al., 2017a, b, 2018).
Data Availability Statement
All datasets generated for this study are included in the article/supplementary material.
Author Contributions
SG-P performed the experiments and analyzed the data. NB collected the samples, cultivated and isolated Klebsormidium strains, identified species, performed experiments, analyzed data, created figures and table, and wrote the first draft of the manuscript. Both authors edited and revised the manuscript and approved the publication.
Funding
This study was funded by the DFG project “Polarcrust” (KA899/23-1) in the frame of the Priority Program 1158 “Antarctic Research” (DFG: Deutsche Forschungsgemeinschaft).
Conflict of Interest
The authors declare that the research was conducted in the absence of any commercial or financial relationships that could be construed as a potential conflict of interest.
Acknowledgments
We thank Jens Boy for collecting Antarctic BSCs and the Instituto Antártico Chileno for their logistic support.
Footnotes
References
Barberousse, H., Ruiz, G., Gloaguen, V., Lombardo, R. J., Djediat, C., Mascarell, G., et al. (2006). Capsular polysaccharides secreted by building façade colonisers: characterisation and adsorption to surfaces. Biofouling 22, 361–370. doi: 10.1080/08927010601035803
Belnap, J. (2002). Nitrogen fixation in biological soil crusts from southeast Utah, USA. Biol. Fertil. Soils 35, 128–135. doi: 10.1007/s00374-002-0452-x
Belnap, J. (2006). The potential roles of biological soil crusts in dryland hydrologic cycles. Hydrol. Process. 20, 3159–3178. doi: 10.1002/hyp.6325
Bisson, M. A., and Kirst, G. O. (1995). Osmotic acclimation and turgor pressure regulation in algae. Naturwissenschaften 82, 461–471. doi: 10.1007/BF01131597
Blaas, K., and Holzinger, A. (2017). F-actin reorganization upon de- and rehydration in the aeroterrestrial green alga Klebsormidium crenulatum. Micron 98, 34–38. doi: 10.1016/j.micron.2017.03.012
Borchhardt, N., Baum, C., Mikhailyuk, T., and Karsten, U. (2017a). Biological soil crusts of Arctic Svalbard - water availability as controlling factor for microalgal biodiversity. Front. Microbiol. 8:1485. doi: 10.3389/fmicb.2017.01485
Borchhardt, N., Baum, C., Thiem, D., Köpcke, T., Karsten, U., Leinweber, P., et al. (2018). Soil microbial phosphorus turnover and identity of algae and fungi in biological soil crusts along a transect in a glacier foreland. Eur. J. Soil Biol. 91, 9–17. doi: 10.1016/j.ejsobi.2018.12.006
Borchhardt, N., Schiefelbein, U., Abarca, N., Boy, J., Mikhailyuk, T., Sipman, H. J. M., et al. (2017b). Diversity of algae and lichens in biological soil crusts of Ardley and King George islands, Antarctica. Antarct. Sci. 9, 1–9. doi: 10.1017/S0954102016000638
Büdel, B., Dulić, T., Darienko, T., Rybalka, N., and Friedl, T. (2016). Cyanobacteria and Algae of Biological Soil Crusts. In Biological Soil Crusts: An Organizing Principle in Drylands. Berlin: Springer International Publishing, 55–80.
Clarke, K. R. (1993). Non-parametric multivariate analyses of changes in community structure. Aust. J. Ecol. 18, 117–143. doi: 10.1111/j.1442-9993.1993.tb00438.x
Clarke, K. R., and Green, R. H. (1988). Statistical design and analysis for a ‘Biological Effect’ study. Mar. Ecol. Prog. Ser. 46, 213–226.
Coulson, S., Hodkinson, I. D., Strathdee, A., Bale, J. S., Block, W., Worland, M. R., et al. (1993). Simulated climate change - the interaction between vegetation type and microhabitat temperatures At Ny-Alesund, Svalbard. Polar Biol. 13, 67–70.
Darby, B. J., and Neher, D. A. (2016). Microfauna within biological soil crusts. Ecol. Stud. 226, 139–157. doi: 10.1007/978-3-319-30214-0_8
De Winder, B., Matthius, H. C., and Mur, L. R. (1990). The effect of dehydration and ion stress on carbon dioxide fixation in drought-tolerant phototrophic micro-organisms. FEMS Microbiol. Lett. 74, 33–38. doi: 10.1111/j.1574-6941.1990.tb01669.x
Donner, A., Glaser, K., Borchhardt, N., and Karsten, U. (2017). Ecophysiological response on dehydration and temperature in terrestrial Klebsormidium (Streptophyta) isolated from biological soil crusts in central european grasslands and forests. Microb. Ecol. 73, 850–864. doi: 10.1007/s00248-016-0917-913
Eldridge, D., and Greene, R. (1994). Microbiotic soil crusts - a review of their roles in soil and ecological processes in the rangelands of Australia. Aust. J. Soil Res. 32:389. doi: 10.1071/SR9940389
Elster, J., Degma, P., Kováčik, L., Valentová, L., Šramková, K., and Batista Pereira, A. (2008). Freezing and desiccation injury resistance in the filamentous green alga Klebsormidium from the Antarctic, Arctic and Slovakia. Biologia 63, 843–851. doi: 10.2478/s11756-008-0111-112
Elster, J., Lukesová, A., Svoboda, J., Kopecky, J., and Kanda, H. (1999). Diversity and abundance of soil algae in the polar desert, Sverdrup Pass, central Ellesmere Island. Polar Rec. 35:231. doi: 10.1017/S0032247400015515
Evans, R. D., and Johansen, J. R. (1999). Microbiotic crusts and ecosystem processes. Crit. Rev. Plant. Sci. 18, 183–225. doi: 10.1080/07352689991309199
Fernández-Marín, B., Holzinger, A., and García-Plazaola, J. (2016). “Photosynthetic strategies of desiccation-tolerant organisms,” in Handbook of Photosynthesis, ed. M. Pessarakli (Boca Raton, FL: CRC Press), 663–681. doi: 10.1201/9781315372136-36
Frenot, Y., Chown, S. L., Whinam, J., Selkirk, P. M., Convey, P., Skotnicki, M., et al. (2005). Biological invasions in the Antarctic: extent, impacts and implications. Biol. Rev. 80, 45–72. doi: 10.1017/S1464793104006542
Gray, D. W., Lewis, L. A., and Cardon, Z. G. (2007). Photosynthetic recovery following desiccation of desert green algae (Chlorophyta) and their aquatic relatives. Plant Cell Environ. 30, 1240–1255. doi: 10.1111/j.1365-3040.2007.01704.x
Gustavs, L., Schumann, R., Eggert, A., and Karsten, U. (2009). In vivo growth fluorometry: accuracy and limits of microalgal growth rate measurements in ecophysiological investigations. Aquat. Microb. Ecol. 55, 95–104. doi: 10.3354/ame01291
Gustavs, L., Eggert, A., Michalik, D., and Karsten, U. (2010). Physiological and biochemical responses of green microalgae from different habitats to osmotic and matric stress. Protoplasma 243, 3–14. doi: 10.1007/s00709-009-0060-9
Hayashi, Y., and Shinozaki, A. (2012). Visualization of microbodies in Chlamydomonas reinhardtii. J. Plant Res. 125, 579–586. doi: 10.1007/s10265-011-0469-z
Herburger, K., and Holzinger, A. (2015). Localization and quantification of callose in the streptophyte green algae zygnema and Klebsormidium: correlation with desiccation tolerance. Plant Cell Physiol. 56, 2259–2270. doi: 10.1093/pcp/pcv139
Holzinger, A., and Karsten, U. (2013). Desiccation stress and tolerance in green algae: consequences for ultrastructure, physiological and molecular mechanisms. Front. Plant Sci. 4:327. doi: 10.3389/fpls.2013.00327
Holzinger, A., Kaplan, F., Blaas, K., Zechmann, B., Komsic-Buchmann, K., and Becker, B. (2014). Transcriptomics of desiccation tolerance in the streptophyte green alga Klebsormidium reveal a land plant-like defense reaction. PLoS One 9:e110630. doi: 10.1371/journal.pone.0110630
Hu, S.-P., Zhou, Y., Zhang, L., Zhu, X.-D., Li, L., Luo, L.-J., et al. (2009). Correlation and quantitative trait loci analyses of total chlorophyll content and photosynthetic rate of rice (Oryza sativa) under water stress and well-watered conditions. J. Integr. Plant Biol. 51, 879–888. doi: 10.1111/j.1744-7909.2009.00846.x
Kaplan, F., Lewis, L., Wastian, J., and Holzinger, A. (2012). Plasmolysis effects and osmotic potential of two phylogenetically distinct alpine strains of Klebsormidium (Streptophyta). Protoplasma 249, 789–804. doi: 10.1007/s00709-011-0324-z
Karsten, U., and Holzinger, A. (2012). Light, temperature, and desiccation effects on photosynthetic activity, and drought-induced ultrastructural changes in the green alga Klebsormidium dissectum (Streptophyta) from a High Alpine Soil Crust. Microb. Ecol. 63, 51–63. doi: 10.1007/s00248-011-9924-6
Karsten, U., and Holzinger, A. (2014). Green algae in alpine biological soil crust communities: acclimation strategies against ultraviolet radiation and dehydration. Biodivers. Conserv. 23, 1845–1858. doi: 10.1007/s10531-014-0653-652
Karsten, U., Lütz, C., and Holzinger, A. (2010). Ecophysiological performance of the aeroterrestrial green alga Klebsormidium crenulatum (charophyceae, streptophyta) isolated from an alpine soil crust with an emphasis on desiccation stress. J. Phycol. 46, 1187–1197. doi: 10.1111/j.1529-8817.2010.00921.x
Karsten, U., and Rindi, F. (2010). Ecophysiological performance of an urban strain of the aeroterrestrial green alga Klebsormidium sp. (Klebsormidiales, Klebsormidiophyceae). Eur. J. Phycol. 45, 426–435. doi: 10.1080/09670262.2010.498587
Kaštovská, K., Elster, J., Stibal, M., and Santrůcková, H. (2005). Microbial assemblages in soil microbial succession after glacial retreat in Svalbard (high arctic). Microb. Ecol. 50, 396–407. doi: 10.1007/s00248-005-0246-244
Kejna, M., Arazny, A., and Sobota, I. (2013). Climatic change on King George Island in the years 1948–2011. Polish Polar Res. 34, 213–235. doi: 10.2478/popore
Kitzing, C., Pröeschold, T., and Karsten, U. (2014). UV-induced effects on growth, photosynthetic performance and sunscreen contents in different populations of the green alga Klebsormidium fluitans (Streptophyta) from alpine soil crusts. Microb. Ecol. 67, 327–340. doi: 10.1007/s00248-013-0317-x
Manabu, N., Kenji, M., Matsuo, U., Nagao, M., Matsui, K., and Uemura, M. (2008). Klebsormidium flaccidum, a charophycean green alga exhibits cold acclimation that is closely associated with compatible solute accumumation and ultrastructural changes. Plant Cell Environ. 31, 872–885. doi: 10.1111/j.1365-3040.2008.01804.x
Mikhailyuk, T., Holzinger, A., Massalski, A., and Karsten, U. (2014). Morphology and ultrastructure of Interfilum and Klebsormidium (Klebsormidiales, Streptophyta) with special reference to cell division and thallus formation. Eur. J. Phycol. 49, 395–412. doi: 10.1080/09670262.2014.949308
Pushkareva, E., Johansen, J. R., and Elster, J. (2016). A review of the ecology, ecophysiology and biodiversity of microalgae in Arctic soil crusts. Polar Biol. 39, 1–14. doi: 10.1007/s00300-016-1902-1905
Rindi, F., Mikhailyuk, T. I., Sluiman, H. J., Friedl, T., and López-Bautista, J. M. (2011). Phylogenetic relationships in interfilum and Klebsormidium (Klebsormidiophyceae, Streptophyta). Mol. Phylogenet. Evol. 58, 218–231. doi: 10.1016/j.ympev.2010.11.030
Rippin, M., Borchhardt, N., Williams, L., Colesie, C., Jung, P., Büdel, B., et al. (2018). Genus richness of microalgae and Cyanobacteria in biological soil crusts from Svalbard and Livingston Island: morphological versus molecular approaches. Polar Biol. 41, 909–923. doi: 10.1007/s00300-018-2252-2
Sabbe, K., Verleyen, E., Hodgson, D. A., Vanhoutte, K., and Vyverman, W. (2003). Benthic diatom flora of freshwater and saline lakes in the Larsemann Hills and Rauer Islands, East Antarctica. Antarct. Sci. 15, 227–248. doi: 10.1017/S095410200300124X
Škaloud, P., and Rindi, F. (2013). Ecological differentiation of cryptic species within an asexual protist morphospecies: a case study of filamentous green alga Klebsormidium (Streptophyta). J. Eukaryot. Microbiol. 60, 350–362. doi: 10.1111/jeu.12040
Starr, R. C., and Zeikus, J. A. (1993). UTEX - the culture collection of algae at the University of Texas at Austin 1993 list of cultures. J. Phycol. 29, 1–106. doi: 10.1111/j.0022-3646.1993.00001.x
Williams, L., Borchhardt, N., Colesie, C., Baum, C., Komsic-Buchmann, K., Rippin, M., et al. (2016). Biological soil crusts of Arctic Svalbard and of Livingston Island, Antarctica. Polar Biol. 40, 399–411. doi: 10.1007/s00300-016-1967-1961
Woelfel, J., Schoknecht, A., Schaub, I., Schaub, I., Enke, N., and Schumann, R. (2014). Growth and photosynthesis characteristics of three benthic diatoms from the brackish southern Baltic Sea in relation to varying environmental conditions. Phycologia. 53, 639–651. doi: 10.2216/14-019.1
Yoshitake, S., Uchida, M., Koizumi, H., Kanda, H., and Nakatsubo, T. (2010). Production of biological soil crusts in the early stage of primary succession on a High Arctic glacier foreland. New Phytol. 186, 451–460. doi: 10.1111/j.1469-8137.2010.03180.x
Keywords: Aeroterrestrial algae, growth, ecophysiology, temperature stress, Polar Regions
Citation: Borchhardt N and Gründling-Pfaff S (2020) Ecophysiological Response Against Temperature in Klebsormidium (Streptophyta) Strains Isolated From Biological Soil Crusts of Arctic and Antarctica Indicate Survival During Global Warming. Front. Ecol. Evol. 8:153. doi: 10.3389/fevo.2020.00153
Received: 20 August 2019; Accepted: 05 May 2020;
Published: 05 June 2020.
Edited by:
Maik Veste, Brandenburg University of Technology Cottbus-Senftenberg, GermanyReviewed by:
Felizitas Bajerski, German Collection of Microorganisms and Cell Cultures GmbH (DSMZ), GermanyShi-Bao Zhang, Chinese Academy of Sciences, China
Copyright © 2020 Borchhardt and Gründling-Pfaff. This is an open-access article distributed under the terms of the Creative Commons Attribution License (CC BY). The use, distribution or reproduction in other forums is permitted, provided the original author(s) and the copyright owner(s) are credited and that the original publication in this journal is cited, in accordance with accepted academic practice. No use, distribution or reproduction is permitted which does not comply with these terms.
*Correspondence: Nadine Borchhardt, n.borchhardt@googlemail.com