- 1MTA Centre for Ecological Research, Balaton Limnological Institute, Tihany, Hungary
- 2University of Veterinary Medicine, Institute for Biology, Budapest, Hungary
- 3MTA-PE Evolutionary Ecology Research Group, University of Pannonia, Veszprém, Hungary
Disentangling the mechanisms that determine community assembly in urban environments is a prerequisite for understanding the impacts of urbanization on the biota and for developing more effective rehabilitation strategies. Community structure in urban stream ecosystems is the sum of multiple processes, including local environmental and catchment level effects. However, the degree to which dispersal from the regional species pool influences urban stream communities still has not been rigorously examined. We studied the importance of the degree of urbanization, the local stream environment and the regional species pool on the assembly of stream fishes in the Pannon Biogeographic Region, Hungary. Correlation analyses between urbanization variables (human population size and a recently developed urbanization index) and local stream and riparian environmental variables did not show significant relationships, indicating that the examined 29 streams reacted to the degree of urbanization in a strongly individual manner. Variance partitioning in both linear regression and redundancy analyses showed that the downstream species pool was the most important determinant of fish species richness, community composition and abundance at urban stream sites. The effect of the local stream environment proved to be moderate, while purely urbanization variables explained only a very small proportion of variance in the data. The relative importance of shared fractions depended on the examined fish assemblage variable, but, in general, was also low or moderate. Additional principal component analyses indicated that community similarity between urban and associated non-urban “reference sites” varied widely, and that the sites did not separate to urban and reference fish community types. Overall, the results highlight that the degree of urbanization is not a strong determinant of local stream habitat and fish community characteristics in this region. Rather, historical species pool and stream characteristics shape fish communities with urbanization playing a rather individual role in some streams. Thus, rehabilitation of urban streams should not only focus on local habitat improvements, but rather consider how dispersal mechanisms from non- urban segments influence community organization at the urban sites.
Introduction
Human induced degradation of natural habitats is one of the leading factors in the decline of biodiversity worldwide (Dudgeon et al., 2006; Didham et al., 2007; Chaudhary et al., 2018). Local extinction of species and alteration of biodiversity are frequently linked to rapid human population growth and the concomitant spread of urban areas (Frissel, 1993; Czech et al., 2000; Aronson et al., 2014). Stream ecosystems are particularly vulnerable to the impacts of urbanization (Wang et al., 2000; Meyer et al., 2005; Gál et al., 2019). For example, several studies showed that urbanization could cause changes in water quality and in stream hydrology and morphology (Chadwick et al., 2006; Roy et al., 2009). In urban areas, streams are frequently confined in channels covered with impervious concrete surfaces. Combined with the alteration of the riparian zone, these modifications result channel simplification and homogenized habitat structure, which directly affect the biodiversity and ecological integrity of stream ecosystems. This process is termed the urban stream syndrome (sensu Walsh et al., 2005; Violin et al., 2011; Booth et al., 2016).
Although knowledge about the effects of urbanization on stream biota is accumulating rapidly, the complex processes of urbanization, and the relative role of interacting factors in affecting the organization of ecological assemblages are still poorly understood. In fact, recent studies reveal considerable heterogeneity in the physical and chemical characteristics of urban streams, rather than a homogenous channel type over many sites (Parr et al., 2016; Hassett et al., 2018). These studies show that the variance in assemblage structure explained by urban land cover and/or local scale instream and riparian variables can vary over a wide range (Brown et al., 2009; Engman and Ramírez, 2012; Lisi et al., 2018). Nevertheless, most studies focused only on the quantification of the effect of abiotic variables and largely neglected other potentially influencing factors in the organization of urban stream assemblages.
For example, not only catchment or local scale habitat variables likely influence the organization of assemblages in urban stream sites, but the dispersal of individual species in the stream catchment (Albanese et al., 2009) and/or the species pool of individual streams, the composition of which may be shaped by former land-use effects (Harding et al., 1998). In fact, recent studies emphasized that spatial constraints and regional species pool effects may override the importance of local habitat conditions in predicting the composition of stream assemblages (Stoll et al., 2014; Czeglédi et al., 2016). For example, Stoll et al. (2014) found that the occurrence rate of a species and species density in the regional species pool were the most important variables that explained much more proportion of the variability of species presence (34 %) and density (38 %) than local abiotic conditions (2 and 21%, respectively) in restored streams. Although dispersal has been suggested as a primary mechanism for maintaining some degree of biodiversity in urban streams (Utz et al., 2016), its relative influence, to our knowledge, still has not been determined along urbanization gradients. However, for developing more effective restoration strategies, it is important to understand not only the effects of urban development and associated physical and chemical degradation, but how dispersal mechanisms from the regional species pool interact with urban stressor variables to determine the diversity and structure of stream organisms.
The objective of this study was to quantify the importance of the degree of urbanization, the local environment and the downstream species pool on the assembly of stream fishes in the Pannon Biogeographic Region, Hungary. For stream fish, species colonization of altered reaches usually happens from downstream sites, since the species composition of fish assemblages show a strongly nested pattern along the longitudinal profile of streams (Matthews, 1998; Erős and Grossman, 2005; Grossman et al., 2010). However, abrupt changes in habitat conditions at urban sites may limit the number of fish species that can potentially colonize urban reaches. Overall, at smaller spatial scales, occurrence and abundance of species in degraded sites may be determined by the type and the extent of habitat modification and the composition of the downstream species pool (e.g., Detenbeck et al., 1992; Albanese et al., 2009). Therefore, we sampled fish assemblages and quantified instream and riparian habitat structure along an urbanization gradient, and also surveyed fish assemblages downstream from the sampled urban sites in order to characterize the potential species pool. We hypothesized that the degradation of local stream habitat will increase with the degree of urbanization, which will exert a strong effect on the structure of fish assemblages. However, we also hypothesized that species pool and associated dispersal effects would be responsible for a large proportion of variance in assemblage structure, which may be comparable with the importance of local habitat effects.
Materials and Methods
Study Sites
The study area was located in Hungary where all the streams and rivers are tributaries of the River Danube, the second largest river in Europe (catchment area 796,250 km2; length 2,847 km). The majority of the country's 93,036 km2 belong to relatively lowland areas (i.e., situated below 300 m a.s.l.), with only a very small proportion being located in submontane regions. The dominant land use type in the catchments is arable fields, with vineyards, orchards, pastures, and managed deciduous forests forming a smaller proportion.
We selected 29 2nd or 3rd order wadeable streams for this study, with varying degrees of urbanization, using geoinformatic maps (Figures 1A,B). In selecting the stream sites we applied the following criteria: (i) streams should be situated below 350 m a.s.l. in order to decrease the effect of natural environmental variability as much as possible among sites; (ii) all selected streams should have a segment within a settlement (urban reach) and another, more natural non-urban segment (hereafter reference site) downstream from the urban ones (species pool); (iii) no insurmountable barrier (reservoir dam, high vertical drop structure, etc.) should be between the urban and the reference site, (iv) all sites should be located within a reasonable distance from the nearest road for accessibility.
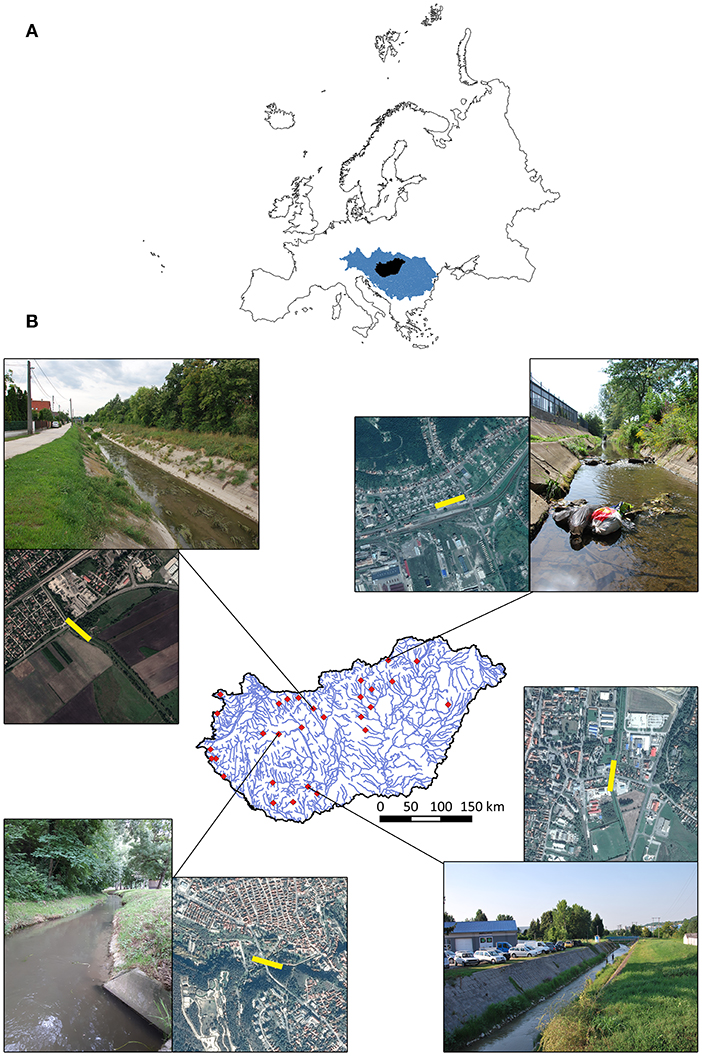
Figure 1. (A) Location of Hungary (black) in the catchment of the Danube River in Europe. (B) Distribution of the sampling sites (red squares) in Hungary and some examples of the studied streams (photos taken during fieldwork are shown) with the satellite pictures from Google Maps. The exact locations of the sampled urbanized sections are indicated by yellow rectangles.
Fish Sampling
Fish were collected during the summer months (July–August) of 2017 and 2018. Two distinct sites were sampled on each stream, one in the urban area, and the other downstream from the urban site in a more natural area. Mean distance (measured in stream kilometers) was 5.26 ± 3.41 km S.D. between the urban and the downstream sites. At each site, we surveyed a 150 m long reach by wading, single pass electrofishing using a backpack electrofishing gear (IG200/2B, PDC, 50–100 Hz, 350–650 V, max. 10 kW; Hans Grassl GmbH, Germany). This amount of sampling effort was found to yield representative samples of fish assemblages in this study area for between-site assemblage comparisons (Sály et al., 2009), and is also comparable with those routinely used elsewhere for the sampling of fish in wadeable streams (Magalhães et al., 2002; Hughes and Peck, 2008). Fish were identified to species level, counted and released back to the stream.
Environmental Variables
We followed the methodology of Erős et al. (2012, 2016) for characterizing the environmental features of urban sites, which will be reiterated here briefly. Altogether 10 transects were placed perpendicular to the main channel at each sampling site to characterize physical features of the environment. Wetted width was measured along each transect. Water depth and current velocity (at ca. 60% depth) were measured at five equally spaced points along each transect. Visual estimates of percentage substratum cover were made at every transect point as well. Percentage substratum data of the transect points were later pooled and overall percentages of substrate categories were calculated for each site. Aquatic macrovegetation (emergent, submerged, floating) and periphyton coverage were also estimated visually for each transect point and later pooled, and the overall percentage of macrophyte categories were calculated for each site. Conductivity, dissolved oxygen content, TDS (total dissolved solids), and pH were measured with an YSI EXO2 multiparameter water quality sonde (Xylem Inc. NY, USA) before fish sampling, and the content of nitrogen forms (i.e., nitrite, nitrate, ammonium) and phosphate were measured using field kits (Visocolor ECO, Macherey-Nagel GmbH & Co. KG., Germany). The habitat structure of the stream margin (i.e., along a ~10 m wide strip in both sides) was characterized by visually estimating the percentage coverage of vegetation (herbaceous and arboreal) and concrete. Altitude was measured in the field using a GPS device (Garmin Montana 650). We used these variables as they provide meaningful information on both catchment and instream level characteristics of the habitat, including possible human effects (Wang et al., 2003; Hoeinghaus et al., 2007; Erős et al., 2012).
Urbanization Variables
We used two measures for quantifying the degree of urbanization at the urban sites: (i) human population of the settlements and (ii) a recently developed urbanization index (Seress et al., 2014). Population of settlements is a widely used proxy for quantifying the degree of urbanization (e.g., Jones and Clark, 1988; Meyer et al., 2005), as it usually highly correlates with increased watershed development, area of the settlement and the amount of imperviousness per unit area (Chabaeva et al., 2009). The urbanization index scores were computed with the UrbanizationScore software1. Using Google Maps satellite images, this software calculates the degree of urbanization for a 1 km × 1 km area around a focal point (here: the center of a study site). To do this, the software relies on major land-cover feature data (proportion of buildings, vegetation, and impervious surfaces), and uses the PC1 score from a principal component analysis (PCA) of the estimated land-cover features. This process thus creates an urbanization gradient by providing an “urbanization score” for each study area, which is a continuous variable suitable for standard statistical analyses [for more details see Seress et al. (2014)].
Statistical Analysis
Urbanization and Habitat Features
Spearman rank correlation values were computed between the urbanization and local habitat variables to ascertain whether the degree of urbanization can be related to the degradation of the studied streams.
We used PCA on the correlation matrix of the recorded habitat data to characterize the water chemistry and the physical structure of the urban sampling sites. TDS was omitted before the analyses because it showed strong correlation with conductivity (Spearman's rho = 0.99; p < 0.001). Spearman correlation test was used to compute the correlation values between the environmental variables and the component scores of the sites along the first three PC axes.
Fish Assemblages and the Importance of Predictor Variables
We conducted PCAs both on species composition and abundance data to quantify and visually examine the similarity of fish assemblages between the urban and their associated reference sites. Prior to abundance-based analysis, data were Hellinger transformed (Legendre and Gallagher, 2001). In the ordination space, the shorter the distance between the urban and the corresponding reference sites, the greater the similarity between their fish assemblages. These analyses thus help to visualize the importance of the species pool in determining fish assemblage structure of the urban sites relative to other urban or reference sites.
We applied variance partitioning procedures (Borcard et al., 2018) for quantifying the importance of the degree of urbanization, the local environment and the species pool in determining the structure of fish assemblages of the urban sites. Note that prior to running the variance partitioning models, we checked for the potential effects of spatial factors on fish assemblage structure. For this, we conducted Spearman rank correlation analysis to explore the relationship between fish assemblage similarity (Euclidean distance based on abundance data) and spatial distance (km) between urban and their associated reference sites and this correlation proved to be insignificant (Spearman's rho = −0.06; p = 0.745). Moreover, former variance partitioning analyses indicated a statistically non-significant effect of spatial distance among sampling sites across Hungary on fish assemblages (adj. R2 = 0.061; p = 0.131) (Tóth et al., 2019). Based on these results, we chose not to include these spatial variables in our variance partitioning models. We ran three separate analyses: (i) for the number of species, (ii) for the fish composition data and (iii) for the abundance data. We used partial multiple linear regression for partitioning the explained variation in the number of species (Borcard et al., 1992; Erős et al., 2009). Specifically, the following three sets of explanatory variables were used: (i) degree of urbanization: population of the settlements and the urban index scores; (ii) local environment: the coordinates of the urban sites along the first three principal components in the PCA applied on the local environment data (see above); (iii) species pool: number of species of the reference sites. The advantage of running a PCA on the original environmental data prior to a further analysis is that it reduces the number of variables to a small number of explanatory variables (here environmental gradients, see e.g., Heino et al., 2005; Czeglédi et al., 2016). Further, we used redundancy analysis (RDA) (Peres-Neto et al., 2006; Borcard et al., 2018) to quantify the relative contribution of the three sets of explanatory variables on the composition and abundance structure of urban fish assemblages. Hellinger transformed abundance data of the species were used as response variables for the abundance-based analysis. Here, we included the same explanatory variables into the models for the degree of urbanization, and for the local environment as we did in the multiple linear regression. However, for characterizing the species pool, we conducted PCAs on the fish composition and Hellinger transformed abundance data of the reference sites and used the first, second and third components as explanatory variables. These variables explained 22.5, 14.8, 9.4, and 30.6, 18.4, 16.4% of the variance for compositional and abundance data, respectively. Variation in the number of species, fish composition, and abundance was partitioned into pure urbanization level, pure local environment and pure species pool factors, and their shared and unexplained proportions using adjusted R2 values (Borcard et al., 2018). All statistical analyses were performed in R (R Development Core Team, 2018) using packages “vegan” (Oksanen et al., 2019), “factoextra” (Kassambara and Mundt, 2017), “FactoMineR” (Le et al., 2008), and “Hmisc” (Harrel and Dupont, 2012).
Results
Urbanization and Habitat Features
The population size of the settlements varied between 128 and 204,156 (33,794 mean ± 46,775 S.D.). The urbanization index characterized an urbanization gradient, which was indicated by the increasing dominance of buildings and roads along the first principal component (Figure 2, positive correlation scores). The other end of the gradient (negative correlation scores) was represented by more natural surroundings with a higher proportion of forest and other vegetation types.
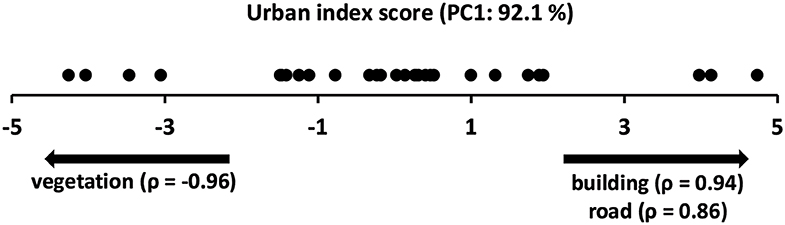
Figure 2. Distribution of the urban sites along the first axis of the PCA based on the urbanization index scores. Spearman rank correlation values between the urbanization index variables and site scores are shown below the axis.
The population of the settlements and the urban index scores showed a significant positive correlation (Table 1). However, correlation values revealed only a weak relationship between the urbanization and the stream habitat variables. Only submerged aquatic vegetation showed a significantly negative correlation with the urban index scores.
PCA on environmental variables indicated high variability among the urban sites. The first principal component revealed a natural environmental gradient from stream sites with silty substrate, dense emergent aquatic macrovegetation and mainly herbaceous bank vegetation (negative correlation scores) to well-oxygenated streams with higher altitude, higher current velocity and higher proportion of coarse substrate (e.g., gravel, stone) (positive correlation scores) (Table 2). On the contrary, the second principal component was more related to a habitat alteration gradient from more urbanized sites with higher proportion of concrete substrate and shoreline and alkaline pH (positive correlation scores) to sites which showed more natural characteristics (e.g., vegetation dominated shoreline, natural substrate, wider, and deeper streambed) (negative correlation scores) (Table 2). Sandy substrate, submerged and floating leaved vegetation and some chemical parameters correlated significantly negatively with the third PCA axis (Table 2).
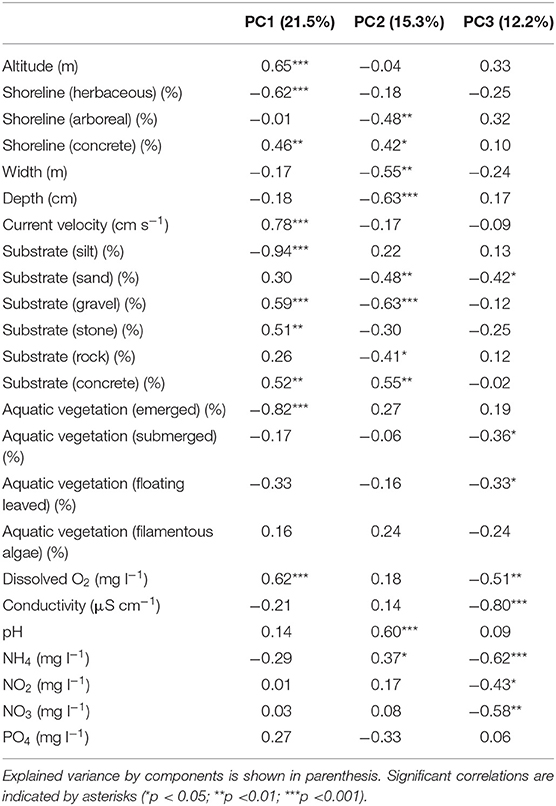
Table 2. Results of the PCA conducted on the local habitat variables of the urban sites with the Spearman rank correlation values between the local habitat variables and PCA component scores of the sites.
Fish Assemblages and the Importance of Predictor Variables
Average species richness were 6.8 ± 3.8 S.D. (min:1, max:18) and 6.2 ± 4.0 (min:1, max:15) in the reference and urban sites, respectively. The average difference in pairwise species richness between the reference and urban sites was 2.5 ± 1.8 S.D. Fish assemblage composition and abundance structure of the two types of sites did not show clear separation along the combination of the first two principal components (Figures 3A,B). Rather, the similarity between the urban and their associated reference sites varied widely.
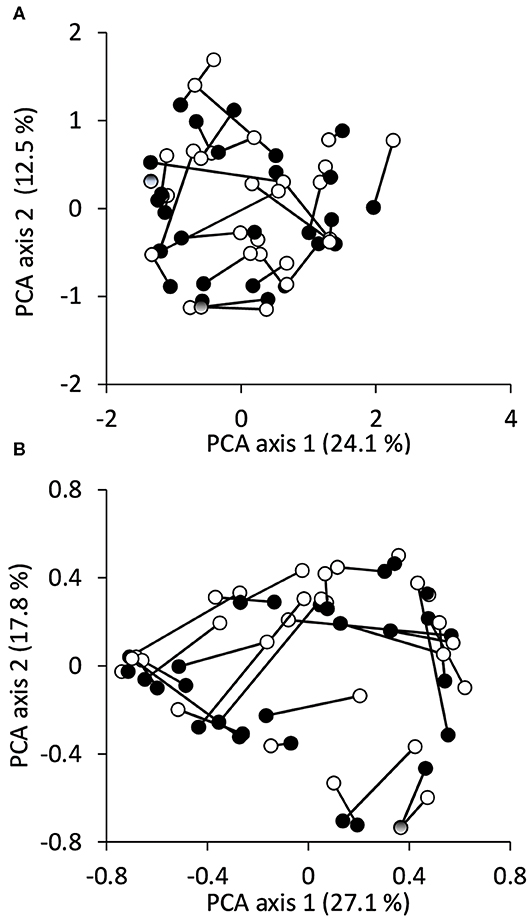
Figure 3. Ordination plot of the PCA of the studied urban and their associated reference sites (linked to each other) based on (A) fish species composition and (B) abundance data. Black symbols: urban sites; white symbols: reference sites.
The three explanatory variable groups in the variance partitioning procedures explained altogether 67.9, 32.6, and 27.1% in the number of species, fish composition and abundance data of the urban sites, respectively (Table 3). For the species number, the largest amount of variation was explained purely by the number of species of the reference sites (p < 0.001), although the unique contribution of the local environment was also significant (p = 0.014). For fish composition, most of the variation was explained by the pure effect variable groups. Of these, the downstream species pool was the most determining variable group, although the effects of the local stream environment and the degree of urbanization also proved to be significant (species pool: p < 0.001, local environment: p = 0.030, degree of urbanization: p = 0.027). For the abundance data, most of the variation was explained jointly by the local environment and species pool effects, indicating intercorrelation between these two sets of variables. Among pure variable groups, only the abundance of fish species in the reference sites explained significantly the abundance structure (p = 0.003).
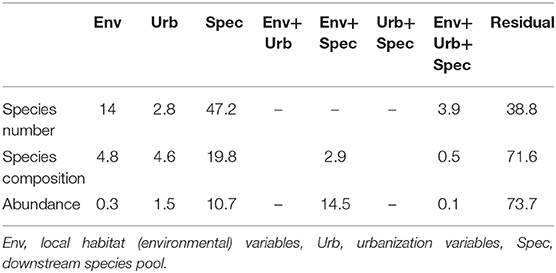
Table 3. Results of the variance partitioning analyses (% explained and residual variance) for species number, species composition, and abundance.
Discussion
Disentangling the mechanisms that determine community assembly in urban environments is a prerequisite for understanding the impacts of urbanization on the biota and for developing more effective rehabilitation strategies. In this study, we found that the local stream environment and the downstream species pool were more important in the assembly of urban stream fish than pure urbanization variables (urbanization index, size of the human population) which had a small, but significant effect on species composition only.
Interestingly, urbanization variables did not show relationship with local stream environmental variables, indicating that the degree of urbanization did not influence significantly the riparian and instream environmental characteristics in this region. In other words, we found relatively natural stream conditions in relatively densely populated areas and vice versa, relatively degraded stream conditions in less built-up areas, similarly for example to the study of Wang et al. (2001) who also found that stream habitat did not correlate well with increasing urbanization in Wisconsin, USA. In fact, PCA results of stream environmental variables suggest that natural environmental gradients were more important determinants of between stream differences than clearly anthropogenic modifications (i.e., concrete bank and substrate). However, the non-interactive effects of natural and anthropogenic factors on general stream characteristics are hard to disentangle based on multivariate field data (Erős et al., 2012). Overall, the results suggest that within stream environmental degradation was very site/stream specific. A likely explanation for this result is that, even though our study sites were distributed along a well-defined urbanization gradient, the majority of the sites were only moderately urbanized. Thus, we could characterize only a relatively short urbanization gradient, due to the lack of large metropolitan areas in Hungary, not like those found in other countries (e.g., USA). Nevertheless, documenting patterns and understanding processes in the early phase of urbanization is important to provide evidence for possible future effects, especially since urbanization processes are ongoing intensively in this region (Tóth et al., 2019).
Several studies justify the importance of habitat structure and diversity to the structure of stream fish assemblages (Gorman and Karr, 1978; Matthews, 1998). Channelization with concrete material makes both within stream habitat and the bank extremely simplified, hereby decreasing hydrologic and geomorphic diversity (Walsh et al., 2005). However, this statement is valid only if the concrete channel is regularly maintained by water management. Spading of the concrete channel by hydrologic erosion can create diverse habitat conditions for stream fish, especially if finer sediment (sand and gravel) from upstream non-urban areas fill the channel, even if at least partly. In fact, the different combinations of concrete, bank stabilizing rock, stone and finer sediment was characteristical for many urban stream sites in Hungary, which can even increase micro- and mesohabitat level hydrologic and geomorphic diversity in contrast to the sand and/or gravel substrate which is the characteristics of more natural streams. In addition, we also observed that the stream margin was differently modified and maintained by urban management practices, ranging from clearcut vegetation to totally abandoned riparian zone. These diverse, but stream specific modification effects could strongly determine why we could not find overall and clear responses to urbanization variables in the studied region neither for environmental nor for fish assemblage variables.
Fish assemblages of urban and reference sites did not clearly separate and both types showed high variability in their fish assemblage structure. Even the corresponding urban and reference site fish assemblages showed large variation, with some urban sites showing more similarity to the reference sites of other streams or reference sites to other urban sites. These results suggest the relatively low predictability of stream fish assemblages in this human-modified landscape (see also Erős et al., 2012), or at least that urbanization in itself was an insufficient predictor of fish assemblage characteristics. Our findings thus confirm other studies, which found highly variable and sometimes even weak responses of fish to land use gradients (e.g., Utz et al., 2010; Tóth et al., 2019). In contrast, most studies showed clear negative influence of increasing urbanization on fish assemblages (Helms et al., 2005; Morgan and Cushman, 2005; Slawski et al., 2008). These contradictions among the studies probably exist because of the differences in the urbanization gradient as well as the sensitivity of fish assemblages to urbanization, which can be largely different among biogeographic regions.
Local and catchment level variables, land use history and dispersal related factors can influence fish assemblage organization to a different degree in human-modified landscapes, and consequently, their interactive effects are hard to disentangle based on regional scale field observations (Wang et al., 1997; Bourassa et al., 2017). Although similarity between fish assemblages of urban and their associated reference sites varied widely, species pool of downstream sites proved to be the most important pure explanatory variable of urban fish assemblages in our variance partitioning models. These results complement recent findings, which also emphasized the role of regional species pool in shaping assemblage structure in degraded stream systems (e.g., Sundermann et al., 2011; Stoll et al., 2014). Species pool effects can prevail via dispersal processes between the urban and their associated reference sites (Utz et al., 2016) or simply by the overarching effect of historical factors over recent local habitat conditions (Harding et al., 1998; Filipe et al., 2009). In degraded stream habitats such as urbanized ones, where extinction risk is generally higher, colonization from downstream sites could be particularly important for fish populations to survive. This theory has a long history in the general ecological literature. For example, Brown and Kodric-Brown (1977) suggested that local extinction of species may be overcome by colonization from the regional species pool. However, under consecutive harsh conditions, urban streams could be population sinks (Utz et al., 2016) and the long term persistence of fish might depend on the characteristics of the downstream assemblages (e.g., species and trait composition, age structure, etc.). For example, Albanese et al. (2009) showed that the abundance and mobility of downstream fish species were key factors in colonization and population recovery processes. In this context, ensuring dispersion within and between stream segments is critically important for the long term survival of fish populations in degraded stream habitats (Bond and Lake, 2003; Brown et al., 2009).
Species richness of downstream sites was an especially good determinant of species richness of upstream urban sites and highly exceeded the predictive power of the habitat variables of urban sites. This result further confirms the role of stream history in shaping fish assemblage characteristics in this region. On the contrary, most of the variance was unexplained in the species composition and abundance-based models. This result suggests the role of other unmeasured variables (e.g., biotic interactions) in shaping the composition and abundance of species in urban sites. Environmental stochasticity (i.e., temporal variations in environmental conditions) and neutral effects could also largely contribute to the differences in the composition and species abundance between urban and their corresponding reference sites. Interestingly, local habitat variables and species pool effects intercorrelated and jointly influenced the abundance of fishes in urban sites, not like in the case of species richness, where the effect of these factors was well-separated. However, the driving of these mechanisms is difficult to interpret using a snapshot regional-scale survey, especially since the importance of individual factors in shaping population abundance can be largely different among species (Wenger et al., 2008).
There is an intense debate in the stream ecological literature whether the degree of urbanization has to attain a certain threshold until assemblage level effects can be observed (Utz et al., 2010). Studies using land cover variables suggest that impervious surface cover (ISC) has to attain at least 10–15% until significant degradation in fish assemblages occurs (Wang et al., 2000, 2001; King et al., 2005; Morgan and Cushman, 2005). Other studies, however, highlight that changes may occur even at the lowest level of land conversion (Meador et al., 2005; Utz et al., 2010). Although we did not quantify ISC directly at the catchment level, it was inherently used to calculate the urbanization index in this study (see the calculation of urbanization scores in the methods), and we found that its value varied between 22 and 98% in the vicinity of the streams. Our results thus, while support the former conclusion, also highlight that it is not enough to measure only land cover variables to understand assemblage responses to urbanization. It is at least equally important that we have detailed field data on local environmental conditions and on the regional (here stream level) species pool, because only these have the potential to reveal stream specific effects and thereby they significantly contribute to better understand the organization of ecological assemblages in human-modified landscapes.
In conclusion, we found that the local stream environment and the historical species pool of the streams were more important determinants of urban stream fish assemblages than clearly urbanization related variables. It seems that most urban streams still have the potential to recover from the degradation effects of urbanization in this region. However, rehabilitation of urban streams should not only focus on local habitat improvements, but rather consider how dispersal mechanisms from non-urban segments influence community organization at urban stream segments. Only this can ensure the long term persistence of stream fish assemblages in an increasingly urbanized world.
Data Availability Statement
The datasets generated for this study are available on request to the corresponding author.
Ethics Statement
All procedures described in this paper were in accordance with Hungarian laws, and were licensed by the Ministry of Agriculture of Hungary (IC permission number: HHgF/252-4/2018; TE permission number: HHgF/252-1/2018).
Author Contributions
TE and IC conceived the study and analyzed the data. IC, BK, RT, and TE conducted the fieldwork. IC, TE, and GS led the writing and editing of the manuscript.
Funding
This study was supported by the GINOP 2.3.3-15-2016-00019 (Sustainable use of ecosystem services—research for mitigating the negative effect of climate change, land use change and biological invasion), the NKFI K128496 (Effects of anthropogenic disturbances on freshwater biodiversity) and the Ecology for Society (MTA KEP) projects.
Conflict of Interest
The authors declare that the research was conducted in the absence of any commercial or financial relationships that could be construed as a potential conflict of interest.
Acknowledgments
The authors acknowledge the financial support provided by the GINOP 2.3.3-15-2016-00019, the NKFI K128496 and the Ecology for Society (MTA KEP) projects.
Footnotes
1. ^freely available online at: https://keplab.mik.uni-pannon.hu/en/urbanization-index
References
Albanese, B., Angermeier, P. L., and Peterson, J. T. (2009). Does mobility explain variation in colonisation and population recovery among stream fishes? Freshw. Biol. 54, 1444–1460. doi: 10.1111/j.1365-2427.2009.02194.x
Aronson, M. F. J., La Sorte, F. A., Nilon, C. H., Katti, M., Goddard, M. A., Lepczyk, C. A., et al. (2014). A global analysis of the impacts of urbanization on bird and plant diversity reveals key anthropogenic drivers. Proc. R. Soc. 281:20133330. doi: 10.1098/rspb.2013.3330
Bond, N. R., and Lake, P. S. (2003). Local habitat restoration in streams : constraints on the effectiveness of restoration for stream biota. Ecol. Manag. Restor. 4, 193–198. doi: 10.1046/j.1442-8903.2003.00156.x
Booth, D. B., Roy, A. H., Smith, B., and Capps, K. A. (2016). Global perspectives on the urban stream syndrome. Freshw. Sci. 35, 412–420. doi: 10.1086/684940
Borcard, D., Gillet, F., and Legendre, P. (2018). Numerical Ecology With R. New York, NY: Springer Verlag.
Borcard, D., Legendre, P., and Drapeau, P. (1992). Partialling out the spatial component of ecological variation. Ecology 73, 1045–1055. doi: 10.2307/1940179
Bourassa, A. L., Fraser, L., and Beisner, B. E. (2017). Benthic macroinvertebrate and fish metacommunity structure in temperate urban streams. J. Urban Ecol. 3, 1–14. doi: 10.1093/jue/jux012
Brown, J. H., and Kodric-Brown, A. (1977). Turnover rates in insular biogeography: effect of immigration on extinction. Ecology 58, 445–449. doi: 10.2307/1935620
Brown, L. R., Gregory, M. B., and May, J. T. (2009). Relation of urbanization to stream fish assemblages and species traits in nine metropolitan areas of the United States. Urban Ecosyst. 12, 391–416. doi: 10.1007/s11252-009-0082-2
Chabaeva, A., Civco, D. L., and Hurd, J. D. (2009). Assessment of impervious surface estimation techniques. J. Hydrol. Eng. 14, 377–387. doi: 10.1061/(ASCE)1084-0699(2009)14:4(377)
Chadwick, M. A., Dobberfuhl, D. R., Benke, A. C., Huryn, A. D., Suberkropp, K., and Thiele, J. E. (2006). Urbanization affects stream ecosystem function by altering hydrology, chemistry, and biotic richness. Ecol. Appl. 16, 1796–1807. doi: 10.1890/1051-0761(2006)016[1796:UASEFB]2.0.CO;2
Chaudhary, A., Pourfaraj, V., and Mooers, A. O. (2018). Projecting global land use-driven evolutionary history loss. Divers. Distrib. 24, 158–167. doi: 10.1111/ddi.12677
Czech, B., Krausman, P. R., and Devers, P. K. (2000). Economic associations among causes of species endangerment in the United States. Bioscience 50:593. doi: 10.1641/0006-3568(2000)050[0593:EAACOS]2.0.CO;2
Czeglédi, I., Sály, P., Takács, P., Dolezsai, A., Nagy, S. A., and Erős, T. (2016). The scales of variability of stream fish assemblages at tributary confluences. Aquat. Sci. 78, 641–654. doi: 10.1007/s00027-015-0454-z
Detenbeck, N. E., DeVore, P. W., Niemi, G. J., and Lima, A. (1992). Recovery of temperate-stream fish communities from disturbance: a review of case studies and synthesis of theory. Environ. Manage. 16, 33–53. doi: 10.1007/BF02393907
Didham, R. K., Tylianakis, J. M., Gemmell, N. J., Rand, T. A., and Ewers, R. M. (2007). Interactive effects of habitat modification and species invasion on native species decline. Trends Ecol. Evol. 22, 489–496. doi: 10.1016/j.tree.2007.07.001
Dudgeon, D., Arthington, A. H., Gessner, M. O., Kawabata, Z.-I., Knowler, D. J., Lévêque, C., et al. (2006). Freshwater biodiversity: importance, threats, status and conservation challenges. Biol. Rev. 81, 163–182. doi: 10.1017/S1464793105006950
Engman, A. C., and Ramírez, A. (2012). Fish assemblage structure in urban streams of Puerto Rico: the importance of reach- and catchment-scale abiotic factors. Hydrobiologia 693, 141–155. doi: 10.1007/s10750-012-1100-6
Erős, T., and Grossman, G. D. (2005). Fish biodiversity in two Hungarian streams : a landscape-based approach. Arch. Hydrobiol. 162, 53–71. doi: 10.1127/0003-9136/2005/0162-0053
Erős, T., Heino, J., Schmera, D., and Rask, M. (2009). Characterising functional trait diversity and trait-environment relationships in fish assemblages of boreal lakes. Freshw. Biol. 54, 1788–1803. doi: 10.1111/j.1365-2427.2009.02220.x
Erős, T., Sály, P., Takács, P., Specziár, A., and Bíró, P. (2012). Temporal variability in the spatial and environmental determinants of functional metacommunity organization - stream fish in a human-modified landscape. Freshw. Biol. 57, 1914–1928. doi: 10.1111/j.1365-2427.2012.02842.x
Erős, T., Takács, P., Specziár, A., Schmera, D., and Sály, P. (2016). Effect of landscape context on fish metacommunity structuring in stream networks. Freshw. Biol. 62, 215–228. doi: 10.1111/fwb.12857
Filipe, A. F., Araújo, M. B., Doadrio, I., Angermeier, P. L., and Collares-Pereira, M. J. (2009). Biogeography of Iberian freshwater fishes revisited : the roles of historical versus contemporary constraints. J. Biogeogr. 36, 2096–2110. doi: 10.1111/j.1365-2699.2009.02154.x
Frissel, C. A. (1993). Topology of extinction and endangerment of native fishes in the Pacific Northwest and California (U.S.A.). Conserv. Biol. 7, 342–354. doi: 10.1046/j.1523-1739.1993.07020342.x
Gál, B., Szivák, I., Heino, J., and Schmera, D. (2019). The effect of urbanization on freshwater macroinvertebrates – knowledge gaps and future research directions. Ecol. Indic. 104, 357–364. doi: 10.1016/j.ecolind.2019.05.012
Gorman, O. T., and Karr, J. R. (1978). Habitat structure and stream fish communities. Ecology 59, 507–515. doi: 10.2307/1936581
Grossman, G. D., Ratajczak, R. E., Farr, M. D., Wagner, C. M., and Petty, J. T. (2010). Why there are fewer fish upstream?. in Community Ecology of Stream Fishes: Concepts, Approaches, and Techniques (Bethesda: American Fisheries Society), 73, 63–81.
Harding, J. S., Benfield, E. F., Bolstad, P. V., Helfman, G. S., and Jones, E. B. D. (1998). Stream biodiversity: the ghost of land use past. Proc. Natl. Acad. Sci.U.S.A. 95, 14843–14847. doi: 10.1073/pnas.95.25.14843
Harrel, F. E., and Dupont, M. C. (2012). Package ‘Hmisc'. R: A Language and Environment for Statistical Computing. Vienna: R Foundation for Statistical Computing.
Hassett, B. A., Sudduth, E. B., Somers, K. A., Urban, D. L., Violin, C. R., Wang, S.-Y., et al. (2018). Pulling apart the urbanization axis : patterns of physiochemical degradation and biological response across stream ecosystems. Freshw. Sci. 37, 653–672. doi: 10.1086/699387
Heino, J., Paavola, R., Virtanen, R., and Muotka, T. (2005). Searching for biodiversity indicators in running waters: do bryophytes, macroinvertebrates, and fish show congruent diversity patterns? Biodivers. Conserv. 14, 415–428. doi: 10.1007/s10531-004-6064-z
Helms, B. S., Feminella, J. W., and Pan, S. (2005). Detection of biotic responses to urbanization using fish assemblages from small streams of western Georgia, USA. Urban Ecosyst. 8, 39–57. doi: 10.1007/s11252-005-1418-1
Hoeinghaus, D. J., Winemiller, K. O., and Birnbaum, J. S. (2007). Local and regional determinants of stream fish assemblage structure: inferences based on taxonomic vs. functional groups. J. Biogeogr. 34, 324–338. doi: 10.1111/j.1365-2699.2006.01587.x
Hughes, R. M., and Peck, D. V. (2008). Acquiring data for large aquatic resource surveys: the art of compromise among science, logistics, and reality. J. North Am. Benthol. Soc. 27, 837–859. doi: 10.1899/08-028.1
Jones, R. C., and Clark, C. C. (1988). Impact of watershed urbanization on stream insect communities. J. Am. Water Resour. Assoc. 23, 1047–1055. doi: 10.1111/j.1752-1688.1987.tb00854.x
Kassambara, A., and Mundt, F. (2017). Factoextra: Extract and Visualize the Results of Multivariate Data Analyses. R package version 1.0.5. Available online at: https://CRAN.R-project.org/package=factoextra (accessed September 27, 2020).
King, R. S., Baker, M. E., Whigham, D. F., Weller, D. E., Jordan, T. E., Kazyak, P. F., et al. (2005). Spatial considerations for linking watershed land cover to ecological indicators in streams. Ecol. Appl. 15, 137–153. doi: 10.1890/04-0481
Le, S., Josse, J., and Husson, F. (2008). FactoMineR : an R package for multivariate analysis. J. Stat. Softw. 25, 1–18. doi: 10.18637/jss.v025.i01
Legendre, P., and Gallagher, E. D. (2001). Ecologically meaningful transformations for ordination of species data. Oecologia 129, 271–280. doi: 10.1007/s004420100716
Lisi, P. J., Childress, E. S., Gagne, R. B., Hain, E. F., Lamphere, B. A., Walter, R. P., et al. (2018). Overcoming urban stream syndrome: trophic flexibility confers resilience in a Hawaiian stream fish. Freshw. Biol. 63, 492–502. doi: 10.1111/fwb.13091
Magalhães, M. F., Batalha, D. C., and Collares-Pereira, M. J. (2002). Gradients in stream fish assemblages across a Mediterranean landscape: contributions of environmental factors and spatial structure. Freshw. Biol. 47, 1015–1031. doi: 10.1046/j.1365-2427.2002.00830.x
Meador, M. R., Coles, J. F., and Zappia, H. (2005). Fish assemblage responses to urban intensity gradients in contrasting metropolitan areas: Birmingham, Alabama and Boston, Massachusetts. Am. Fish. Soc. Symp. 47, 409–423. doi: 10.1049/iet-cvi.2013.0217
Meyer, J. L., Paul, M. J., and Taulbee, W. K. (2005). Stream ecosystem function in urbanizing landscapes. J. North Am. Benthol. Soc. 24, 602–612. doi: 10.1899/04-021.1
Morgan, R. P., and Cushman, S. F. (2005). Urbanization effects on stream fish assemblages in Maryland, USA. J. North Am. Benthol. Soc. 24, 643–655. doi: 10.1899/04-019.1
Oksanen, J., Guillaume Blanchet, F., Friendly, M., Kindt, R., Legendre, P., McGlinn, D., et al. (2019). Vegan: Community Ecology Package. R package version 2.5-6. Available online at: https://CRAN.R-project.org/package=vegan (accessed September 27, 2020).
Parr, T. B., Smucker, N. J., Bentsen, C. N., and Neale, M. W. (2016). Potential roles of past, present, and future urbanization characteristics in producing varied stream responses. Freshw. Sci. 35, 436–443. doi: 10.1086/685030
Peres-Neto, P. R., Legendre, P., Dray, S., and Borcard, D. (2006). Variation partitioning of species data matrices: estimation and comparison of fractions. Ecology 87, 2614–2625. doi: 10.1890/0012-9658(2006)87[2614:VPOSDM]2.0.CO;2
R Development Core Team (2018). A Language and Environment for Statistical Computing. Vienna: R Foundation for Statistical Computing. Available online at: https://www.R-project.org
Roy, A. H., Dybas, A. L., Fritz, K. M., and Lubbers, H. R. (2009). Urbanization affects the extent and hydrologic permanence of headwater streams in a midwestern US metropolitan area. J. North Am. Benthol. Soc. 28, 911–928. doi: 10.1899/08-178.1
Sály, P., Erős, T., Takács, P., Specziár, A., Kiss, I., and Bíró, P. (2009). Assemblage level monitoring of stream fishes: the relative efficiency of single-pass vs. double-pass electrofishing. Fish. Res. 99, 226–233. doi: 10.1016/j.fishres.2009.06.010
Seress, G., Lipovits, Á., Bókony, V., and Czúni, L. (2014). Quantifying the urban gradient: a practical method for broad measurements. Landsc. Urban Plan. 131, 42–50. doi: 10.1016/j.landurbplan.2014.07.010
Slawski, T. M., Veraldi, F. M., Pescitelli, S. M., and Pauers, M. J. (2008). Effects of tributary spatial position, urbanization, and multiple low-head dams on warmwater fish community structure in a midwestern stream. North Am. J. Fish. Manag. 28, 1020–1035. doi: 10.1577/M06-186.1
Stoll, S., Kail, J., Lorenz, A. W., Sundermann, A., and Haase, P. (2014). The importance of the regional species pool, ecological species traits and local habitat conditions for the colonization of restored river reaches by fish. PLoS ONE 9:e84741. doi: 10.1371/journal.pone.0084741
Sundermann, A., Stoll, S., and Haase, P. (2011). River restoration success depends on the species pool of the immediate surroundings. Ecol. Appl. 21, 1962–1971. doi: 10.1890/10-0607.1
Tóth, R., Czeglédi, I., Kern, B., and Erős, T. (2019). Land use effects in riverscapes: diversity and environmental drivers of stream fish communities in protected, agricultural and urban landscapes. Ecol. Indic. 101, 742–748. doi: 10.1016/j.ecolind.2019.01.063
Utz, R. M., Hilderbrand, R. H., and Raesly, R. L. (2010). Regional differences in patterns of fish species loss with changing land use. Biol. Conserv. 143, 688–699. doi: 10.1016/j.biocon.2009.12.006
Utz, R. M., Hopkins, K. G., Beesley, L., Booth, D. B., Hawley, R. J., Baker, M. E., et al. (2016). Ecological resistance in urban streams : the role of natural and legacy attributes. Freshw. Sci. 35, 380–397. doi: 10.1086/684839
Violin, C. R., Cada, P., Sudduth, E. B., Hassett, B. A., Penrose, D. L., and Bernhardt, E. S. (2011). Effects of urbanization and urban stream restoration on the physical and biological structure of stream ecosystems. Ecol. Appl. 21, 1932–1949. doi: 10.1890/10-1551.1
Walsh, C. J., Roy, A. H., Feminella, J. W., Cottingham, P. D., Groffman, P. M., Morgan, I. I., et al. (2005). The urban stream syndrome: current knowledge and the search for a cure. J. North Am. Benthol. Soc. 24, 706–723. doi: 10.1899/04-028.1
Wang, L., Lyons, J., and Kanehl, P. (2001). Impacts of urbanization on stream habitat and fish across multiple spatial scales. Environ. Manage. 28, 255–266. doi: 10.1007/s002670010222
Wang, L., Lyons, J., Kanehl, P., Bannerman, R., and Emmons, E. (2000). Watershed urbanization and changes in fish communities in southeastern Wisconsin streams. J. Am. Water Resour. Assoc. 36, 1173–1189. doi: 10.1111/j.1752-1688.2000.tb05719.x
Wang, L., Lyons, J., Kanehl, P., and Gatti, R. (1997). Influences of watershed land use on habitat quality and biotic integrity in Wisconsin streams. Fisheries 22, 6–12.
Wang, L., Lyons, J., Rasmussen, P., Seelbach, P., Simon, T., Wiley, M., et al. (2003). Watershed, reach, and riparian influences on stream fish assemblages in the Northern Lakes and Forest Ecoregion, U.S.A. Can. J. Fish. Aquat. Sci. 60, 491–505. doi: 10.1139/F03-043
Keywords: urban streams, fish assemblage, species pool, historical effects, habitat structure, migration, variance partitioning
Citation: Czeglédi I, Kern B, Tóth R, Seress G and Erős T (2020) Impacts of Urbanization on Stream Fish Assemblages: The Role of the Species Pool and the Local Environment. Front. Ecol. Evol. 8:137. doi: 10.3389/fevo.2020.00137
Received: 04 February 2020; Accepted: 23 April 2020;
Published: 15 May 2020.
Edited by:
Pedro Giovâni Da Silva, Federal University of Minas Gerais, BrazilReviewed by:
Mauricio Cetra, Federal University of São Carlos, BrazilAna Filipa Filipe, Universidade Do Porto, Portugal
Copyright © 2020 Czeglédi, Kern, Tóth, Seress and Erős. This is an open-access article distributed under the terms of the Creative Commons Attribution License (CC BY). The use, distribution or reproduction in other forums is permitted, provided the original author(s) and the copyright owner(s) are credited and that the original publication in this journal is cited, in accordance with accepted academic practice. No use, distribution or reproduction is permitted which does not comply with these terms.
*Correspondence: István Czeglédi, czegledi.istvan@okologia.mta.hu; mullercega@gmail.com