- 1Research Center for Agricultural Information Technology, National Agriculture and Food Research Organization, Tokyo, Japan
- 2The Kyoto University Museum, Kyoto University, Kyoto, Japan
An ecological community network generally has a clustering structure formed by evolutionary and ecological processes. Because females and males of a single visitor species often differ in their evolutionary and ecological relationship with flowering plants, visitor sex should affect the clustering structure of flower–visitor networks. Two related metrics are used to evaluate clustering structures: compartmentation and modularity. Compartmentation refers to the number of clearly separate subgroups, whereas modularity describes subgroups according to their number of aggregating links. Thus, compartmentation is a measure of network fragmentation and modularity is a measure of network heterogeneity. Because male visitors tend to give priority to search for mates, we hypothesized that male visitors increased compartmentation and decreased modularity compared with female visitors. By using museum specimens of flower–visitor insects together with the plant species that they were visiting, recorded at the time of collection, we constructed 11 networks for each research site and collection year, separated each into male and female subnetworks, and then compared the two metrics between them. Results showed that compartmentation was not different between them, while male subnetworks had lower modularity than female subnetworks, and strengthened modularity of species networks together with female subnetworks. These structural characteristics of male subnetworks likely reflect less choosy and less mutualistic visits of males compared with females. This might imply that the role of male visitors in maintaining flower diversity has been overlooked.
Introduction
Ecological community networks can be considered to be assemblies of multiple species, and they are generally composed of several subgroups (Dicks et al., 2002; Olesen et al., 2007; Fortuna et al., 2010; Guimerà et al., 2010; Thébault and Fontaine, 2010). The clustering structure greatly affects the community dynamics (Grilli et al., 2016). Flower–visitor networks in particular commonly have a clustering structure (Olesen et al., 2007), and it has been shown theoretically that the clustering structure of mutualistic flower–visitor networks are more likely to cause chained extinction, because extinction of a single visitor species is likely to be followed by the second extinction of the plant species within the same subgroup that depends on the visitor species for pollination (Thébault and Fontaine, 2010).
The drivers leading to the formation of a clustering structure in flower–visitor networks include temporal and spatial variations of environmental conditions (Spiesman and Inouye, 2013; Morente-López et al., 2018) and trait-matching between visitors and flowers (Dicks et al., 2002; Dupont and Olesen, 2012; Maruyama et al., 2014). Maruyama et al. (2014) studied a network composed of hummingbirds and the flowers that they visited in Brazil and demonstrated that the critical factors leading to the formation of subgroups were flower phenology, variation in habitat environments, and trait-matching between flower corolla length and hummingbird bill length. In addition, intraspecific variations in the temporal and spatial distributions and the phenological and physiological traits of individuals of a single visitor species can also affect clustering and other network structures (Bolnick et al., 2011).
Such intraspecific variations can differ depending on the sex of the flower visitor. Male and female flower–visitors usually differ in their temporal and spatial distributions, in their flower preferences, and in the number of flowers that they visit (Michener, 1969; Paxton, 2005; Roswell et al., 2019; Smith et al., 2019). For example, male Centris pallida bees patrol near the ground to seek emerging virgin females with which to mate, and they also visit flowers there. Whereas females, after mating, visit additional flowers with more pollen and nectar rewards to provision their larvae (Alcock et al., 1977). Males of the large carpenter bee Xylocopa ogasawarensis also patrol around flowers of Scaevola sericea in their territories, while females visit the wide range of flowering plants in the Ogasawara Islands (Sugiura, 2008). In other species as well, male visitors generally make a far larger effort to mate with female partners than to visit flowers and to collect flower rewards, and then tend to visit flowers by chance (Smith et al., 2019). This male flower-visiting pattern suggests a weaker preference for a few certain flowers and more randomized and less mutualistic flower–visitor interactions compared the female flower-visiting pattern.
These sex differences in flower visiting patterns are expected to affect the clustering structure of flower–visitor networks. Two related metrics are used to describe clustering structures: modularity and compartmentation. In modular networks, there are frequent interactions within subgroups but infrequent interactions between subgroups (Newman, 2006; Boccaletti et al., 2007), whereas compartmented networks are composed of clearly separate subgroups that share no links (Lewinsohn et al., 2006; Dormann and Strauss, 2014). Thus, modularity is a measure of network heterogeneity with respect to the number of links, and compartmentation is a measure of network fragmentation. We then hypothesized that male visitors decreased modularity and increased compartmentation due to more randomized and less mutualistic visitations of males.
In this study, we examined whether visitor sex is significantly associated with modularity and compartmentation in flower–visitor networks by analyzing modified datasets of insect specimens that were collected in Kyoto, Japan, in the 1980s. A characteristic feature of these datasets is that the visitor sex is known among increasing open network datasets (e.g., Fortuna et al., 2014). We constructed 11 flower–visitor networks, one for each collection site and year, and divided each of them into two subnetworks, in which one subnetwork was composed of female visitors and plants that they visited while the other was composed of male visitors and their plants. Then modularity and compartmentation were compared between female subnetworks, male subnetworks and the original species networks (not divided by sex). Our hypothesis predicts that male subnetworks have lower modularity and higher compartmentation than female subnetworks.
Materials and Methods
Dataset
We prepared datasets for several flower–visitor networks in which the visitor sex was known by checking the sex of thousands of specimens of flower–visitor insects preserved in the Kyoto University museum (Inoue et al., 1990; Kakutani et al., 1990; Kato et al., 1990). The collection sites and years of these specimens were Ashu (35°20′34″ N, 135°45′32″ E), 1984–1987 (Kato et al., 1990), Kibune (35°08′04″ N, 135°45′51″ E), 1984–1987 (Inoue et al., 1990), and the Yoshida campus of Kyoto university (35°01′50″ N, 135°47′13″ E), 1985–1987 (Kakutani et al., 1990). Researchers and their students collected these insect specimens as part of a practical seminar on sunny days, 8–19 times at Ashu in a year, 5–10 times at Kibune, and 32–49 times at the campus of Kyoto University from April to November, by using insect nets for 10 min per location in front of flowering plants along census routes (Kato et al., 1990). The species name of the flower that each individual insect was collected from was also recorded at the time of collection. These datasets have been used previously for analyzing modularity and other network structures (e.g., Olesen et al., 2007; Bascompte et al., 2003; Dormann et al., 2009). Detailed information may be found in those papers (Inoue et al., 1990; Kakutani et al., 1990; Kato et al., 1990).
We checked the preservation status and identified the sex of each specimen as necessary and constructed a modified dataset containing a total of 5212 individual records (Supplementary Table S1). Each record included the insect species name or species level (i.e., “sp.”), sex, the plant species on which it had been collected, the collection year and site.
We constructed 11 flower–visitor networks (i.e., 4 networks for Ashu and Kibune, and 3 for the Yoshida campus), and then divided each network into a male subnetwork and a female subnetwork.
Network Analysis
To evaluate compartmentation, we calculated the number of compartments (Prado and Lewinsohn, 2004; Lewinsohn et al., 2006) by using a brute-force search in which compartments (Jordan blocks in the mathematical term) have no links each other in a bipartite matrix (Dormann et al., 2009). The number of compartments is not affected by network size, but affected by interaction density (Dormann et al., 2009). We then standardized the number of compartments by using null models that were generated by an “r2d table” method. This method randomizes flower–visitor interactions but keeps marginal totals of rows and columns in bipartite matrices (i.e., the total interaction number of every species) equivalent to the original network (Oksanen et al., 2019). Thus, in null models generated by thr “r2d table” method, the number of interactions of a given species pair (a visitor and a plant species) depends on relative abundances of the two species.
where NCstand is the standardized value of the number of compartments, NCfocal is the number of compartments of the focal network, NCnull is the mean number of compartments of 1000 null models, and sdnull is the standard deviation of those of the null models.
The modularity index, Q, ranges from 0 to 1 and indicates the number of interaction links observed within modules in excess of the number expected by chance (Dormann and Strauss, 2014). We calculated Q by a hierarchical random graph approach (Clauset et al., 2008), in which a species is randomly swapped in a dendrogram:
Aij is observed interaction frequency between species i and j, Kij is the expected interaction frequency based on the null model generated by the “r2d table” method written above, m is the total number of links; m = ΣAij, gi and gj are modules that the species i and j are assigned to, respectively, and the indicator function δ(gi, gj) = 1 if gi = gj and 0 otherwise. When Q is 0, the network has no more links within modules than would be expected by chance. Q increases when aggregated links form distinct subgroups. Because Q depends on network size (i.e., on the number of species and the number of individuals of each species in the network), we also standardized Q by using 100 null models as well as the number of compartments above (Dormann and Strauss, 2014). We compared the standardized value of compartments, NCstand, and that of modularity, Qstand, among female subnetworks, male subnetworks, and species networks.
To evaluate the network position of each species in a modular network, we calculated the within-subgroup degree, z, and the between-subgroup degree, c, for each species (Guimerà et al., 2007; Olesen et al., 2007) in a network composed of male visitor species and female visitor species.
where kis is the total number of interactions (i.e., the total number of individuals collected) of species i within its own module s, is the average number of interactions in s, and SDks is the standard deviation of the numbers of interactions of all species in s, ki is the total number of interactions of species i, and kit is the number of interactions between species i and another species in module t. Thus, z is the standardized variance of the interaction number within the module that a species belongs to. Meanwhile, c increases when a species interacts with other species in many modules uniformly. When a species has no links to other modules, c is 0.
We compared z and c values between male and female visitors in each network. When species with a higher c value increase, the number of compartments should be lower. Olesen et al. (2007) analyzed 51 flower–visitor networks and proposed threshold values for z and c (z = 2.6, c = 0.62) for classifying each species as a network hub (high z, high c), module hub (low z, high c), connector (high z, low c), or peripheral node (low z, low c). Network hub species are supergeneralists and therefore key species in a modular structure (Biella et al., 2017).
To compare the standardized value of compartments and that of modularity between female subnetworks, male subnetworks, and species networks, we used a generalized linear mixed model (GLMM), in which network type was the explanatory variable, the standardized compartments or modularity was the response variable, and collection site and network ID were random effects. If significant difference was detected, multiple comparisons were carried out with Bonferroni correction (p = 0.05/3 = 0.0167). To compare z and c values between female and male visitors in each network, we used the non-parametric Wilcoxon rank sum test with Bonferroni correction (p = 0.05/11 = 0.0045). We used the free statistical software platform R version 3.5.3 (R Development Core Team, 2019) for all network analyses and statistical analyses. We used the “bipartite” package version 2.13 for calculating the number of compartments, modularity, Q, and c and z values (Dormann et al., 2009; Dormann, 2011), “exactRankTests” package for the Wilcoxon rank sum test, and the “ggplot2” package version 3.2.0 to prepare figures (Wickham, 2016).
Results
Among the original, sex-unseparated species networks, the maximum total number of visitor and plant species was 335 in Kibune in 1987 (Supplementary Table S1). In the same network, the maximum number of visitor species was 259. The maximum number of female and male visitor species was 168 and 147, respectively, in Kibune, 1987. The minimum total number of visitor and plant species was 98 in Ashu, 1985. In the same network, the minimum number of visitor species was 69. The minimum number of female visitor species was 44 in Kyoto University, 1987, and the minimum number of male visitor species was 31 in Ashu, 1985.
The average value of the standardized numbers of compartments, NCstand, of male subnetworks was 3.13 ± 1.47 (mean ± standard deviation), that of female subnetworks was 2.19 ± 0.77, and that of species networks was 2.54 ± 0.77. However, the standardized number of compartments was not significantly different among the three kinds of networks (F = 2.05, p = 0.15) (Figure 1A). The standardized modularity value, Qstand, was significantly different among the three kinds of networks (F = 28.58, p < 0.0001) (Figure 1B and Supplementary Appendix). Multiple comparisons showed that those values of species networks (30.9 ± 13.3) were higher than those of the other two subnetworks, and those of female subnetworks (21.7 ± 8.7) were higher than those of male subnetworks (14.5 ± 7.9).
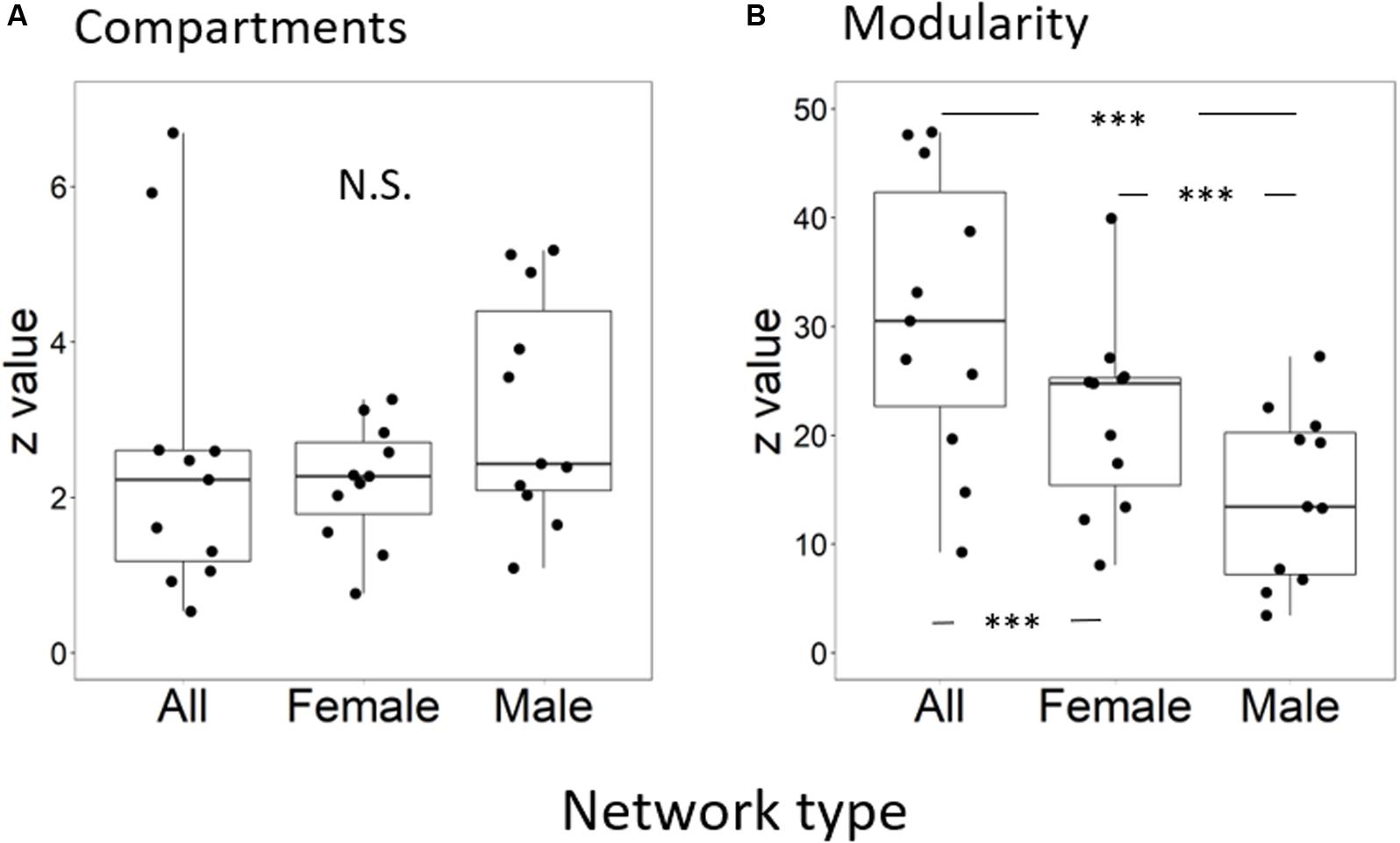
Figure 1. (A) Standardized number of compartments and (B) standardized modularity in sex-unseparated (Species) and sex-separated (Female and Male) networks. Black dots indicate observed values, the top and bottom lines of each box are the upper and lower quartiles, respectively, the horizontal line inside the box is the median, and the top and bottom ends of the whiskers indicate maximum and minimum values, respectively. Significant differences between network pairs are shown by asterisks (∗p < 0.05; ∗∗∗p < 0.001).
The within-subgroup degree, z, did not differ significantly between male visitors and female visitors in any of the 11 networks (Table 1 and Figure 2). Similarly, the between-subgroup degree, c, did not differ significantly between male and female visitors in any of the 11 networks (Table 1 and Figure 2).
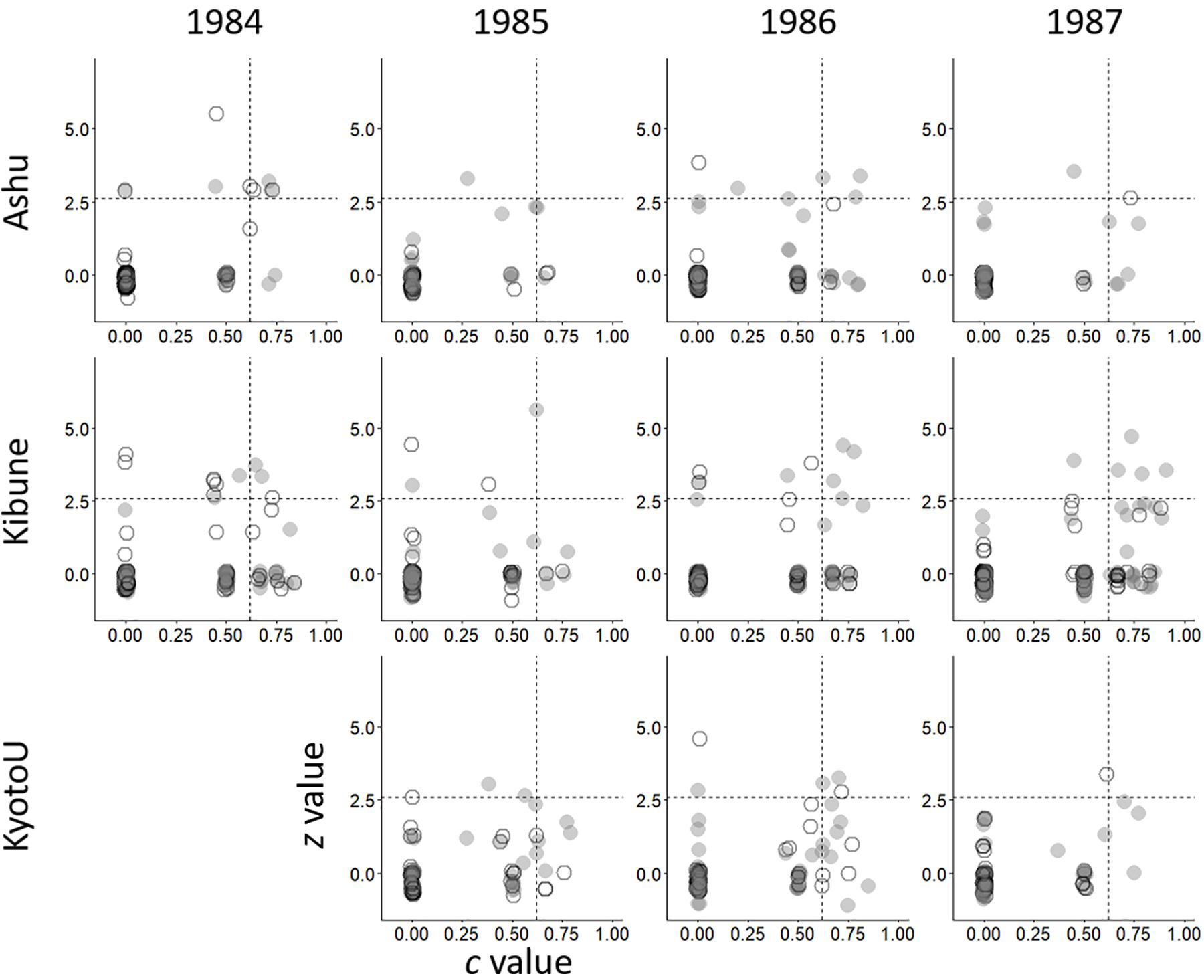
Figure 2. Network positions of males (gray circles) and females (white circles) of each species according to their within-group degree (z) and between-group degree (c) in each network composed of visitor females, visitor males, and plant species. Horizontal and vertical dashed lines indicate the threshold values proposed by Olesen et al. (2007) for separating network hub (top right), module hub (bottom right), connector (top left), and peripheral nodes (bottom right).
The network hub species often differed between the sex-unseparated species networks and the sex-separated networks (Supplementary Table S2). For example, in Ashu, 1984, the syrphids (hoverflies) Melanostoma scalare and Eristalis cerealis were network hub species in the species network, but in the female visitor subnetwork, Hylaeus globula (a small bee) and Bombus diversus diversus (a bumblebee) were network hub species, and in the male visitor subnetwork, E. cerealis, Eurystylus coelestialium (Hemiptera), and H. globula were network hub species. No network hub species was detected in 5 of the 11 species networks, or in 3 of the 11 sex-separated networks.
Discussion
Our analyses showed that visitor sex was significantly associated with the modularity, but not with the compartmentation of flower–visitor networks. Male subnetworks had a lower standardized modularity than female subnetworks. Thus, interaction links of male visitors were more evenly and widely scattered in flower–visitor networks. These characteristic structures of male subnetworks may be attributed to more haphazard and less mutualistic nature of flower visits by males, which tend to make a large effort to search for mates. Conversely, we suggest that higher standardized modularity of female subnetworks can be attributed to the reward-expecting and mutualistic visits. Meanwhile, compartmentation was not different between the two subnetworks. Compartmentation, in other words, fragmentation of a network, might not be affected by difference of flower–visiting pattern between female and male visitors, though our results based on the limited datasets should be discussed together with further studies in future.
Although these results might lead us to expect more network hub species among female visitors, within-subgroup degrees—z values—and between-subgroup degrees—c values—did not differ between female and male visitors. Some male visitors, like some female visitors, were network hubs in the sex-separated networks (Supplementary Table S2). Therefore, contributions to a modular structure should not differ significantly between visitors of the two sexes. Our finding of sex differences in compartmentation and modularity thus appears to conflict with the absence of any sex differences in between- and within-module degrees. This conflict can be resolved by considering different arrangements of female and male visitors on two-dimensional bipartite matrices of flower–visitor networks. When a flower–visitor network is expressed as a bipartite matrix, central core nodes are generalist visitors (flowers) interacting with generalist flowers (visitors), and peripheral nodes are specialist visitors (flowers) interacting with specialist flowers (visitors) (Dupont and Olesen, 2012). If peripheral visitors tend to visit peripheral flowers that are not visited by generalists, then the network should have more compartments and lower standardized modularity, as observed among the male subnetworks, despite their having similar between- and within-module values.
Because species networks, as a combination of female and male subnetworks, had the highest standardized modularity, the modular structure of flower–visitor networks was synergistically enhanced by female and male visitors. Thus, male subnetworks boosted the modular structure of female subnetworks.
We offer another hypothesis that observed modular flower–visitor networks may be more stable than otherwise owing to the presence of male visitors. Flower–visitor networks have been assumed to be mutualistic (Vázquez et al., 2009), and theoretical studies have indicated that higher modularity destabilizes mutualistic networks (Thébault and Fontaine, 2010; Stouffer and Bascompte, 2011; Grilli et al., 2016). Although these studies suggest that flower–visitor networks with higher modularity should be unstable, many modular flower–visitor networks have been observed in the field (Olesen et al., 2007). This gap between theoretical predictions of instability and the frequent observations of modular flower–visitor networks (Kaiser-Bunbury et al., 2010) may be partly accounted for by considering visitor sex. Thus, male subnetworks may intensify modularity of the species networks but not weaken stability because of less mutualistic visits by males. Fontaine et al. (2011) showed that relative modularity values of networks with more non-mutualistic interactions are far lower than those of networks with more mutualistic interactions. Furthermore, when mutualistic and less mutualistic interactions are mixed, the effect of modularity on network stability is greatly reduced (Sauve et al., 2014). Thus, in our hypothesis, owing to the presence of male visitors, flower–visitor networks may not be as mutualistic, and hence not as unstable as previously thought. Complex structures observed in flower–visitor networks may be better understood by taking visitor sex into account.
Data Availability Statement
The full data sets are available on Dryad at http://dx.doi.org/10.5061/dryad.hdr7sqvd1.
Author Contributions
SK designed the study, conducted data collection and statistical analyses, and wrote the manuscript. TK helped collect the data and advised logical presentations and statistical analyses.
Funding
This study was funded by Japan Society for the Promotion of Science KAKENHI Grant Number JP25291102 and the Ministry of Agriculture, Forestry, and Fisheries, Japan, through a research project entitled “Monitoring and enhancement of pollinators for crop production”.
Conflict of Interest
The authors declare that the research was conducted in the absence of any commercial or financial relationships that could be construed as a potential conflict of interest.
Acknowledgments
We thank Masayoshi Hiraiwa and Takayuki Ohgushi for many valuable discussions.
Supplementary Material
The Supplementary Material for this article can be found online at: https://www.frontiersin.org/articles/10.3389/fevo.2020.00124/full#supplementary-material
References
Alcock, J., Jones, C. E., and Buchmann, S. L. (1977). Male mating strategies in the bee Centris pallida Fox (Anthophoridae: Hymenoptera). Am. Nat. 111, 145–155.
Bascompte, J., Jordano, P., Melián, C. J., and Olesen, J. M. (2003). The nested assembly of plant–animal mutualistic networks. Proc. Nat. Acad. Sci. U.S.A. 100, 9383–9387. doi: 10.1073/pnas.1633576100
Biella, P., Ollerton, J., Barcella, M., and Assini, S. (2017). Network analysis of phenological units to detect important species in plant–pollinator assemblages: can it inform conservation strategies? Commun. Ecol. 18, 1–10. doi: 10.1556/168.2017.18.1.1
Boccaletti, S., Ivanchenko, M., Latora, V., Pluchino, A., and Rapisarda, A. (2007). Detecting complex network modularity by dynamical clustering. Phys. Rev. E 75:45102. doi: 10.1103/PhysRevE.75.045102
Bolnick, D. I., Amarasekare, P., Araújo, M. S., Bürger, R., Levine, J. M., Novak, M., et al. (2011). Why intraspecific trait variation matters in community ecology. Trends Ecol. Evol. 26, 183–192. doi: 10.1016/j.tree.2011.01.009
Clauset, A., Moore, C., and Newman, M. E. (2008). Hierarchical structure and the prediction of missing links in networks. Nature 453, 98–101. doi: 10.1038/nature06830
Dicks, L. V., Corbet, S. A., and Pywell, R. F. (2002). Compartmentalization in plant–insect flower visitor webs. J. Anim. Ecol. 71, 32–43. doi: 10.1046/j.0021-8790.2001.00572.x
Dormann, C. F. (2011). How to be a specialist? Quantifying specialisation in pollination networks. Netw. Biol. 1, 1–20.
Dormann, C. F., Fründ, J., Blüthgen, N., and Gruber, B. (2009). Indices, graphs and null models: analyzing bipartite ecological networks. Open Ecol. J. 2, 7–24. doi: 10.2174/1874213000902010007
Dormann, C. F., and Strauss, R. (2014). A method for detecting modules in quantitative bipartite networks. Methods Ecol. Evol. 5, 90–98. doi: 10.1111/2041-210X.12139
Dupont, Y. L., and Olesen, J. M. (2012). Stability of modular structure in temporal cumulative plant-flower-visitor networks. Ecol. Complex. 11, 84–90. doi: 10.1016/j.ecocom.2012.03.004
Fontaine, C., Guimarães, P. R., Kéfi, S., Loeuille, N., and Memmot, J. (2011). The ecological and evolutionary implications of merging different types of networks. Ecol. Lett. 14, 1170–1181. doi: 10.1111/j.1461-0248.2011.01688.x
Fortuna, M. A., Ortega, R., and Bascompte, J. (2014). The web of Life. arXiv [Preprint], Available online at: https://arxiv.org/abs/1403.2575v1 (accessed April 15, 2020).
Fortuna, M. A., Stouffer, D. B., Olesen, J. M., Jordano, P., Mouillot, D., Krasnov, B. R., et al. (2010). Nestedness versus modularity in ecological networks: two sides of the same coin? J. Anim. Ecol. 79, 811–817. doi: 10.1111/j.1365-2656.2010.01688.x
Grilli, J., Rogers, T., and Allesina, S. (2016). Modularity and stability in ecological communities. Nat. Commun. 7:12031. doi: 10.1038/ncomms12031
Guimerà, R., Sales-Pardo, M., and Amaral, L. A. N. (2007). Module identification in bipartite and directed networks. Phys. Rev. E 76:36102. doi: 10.1103/PhysRevE.76.036102
Guimerà, R., Stouffer, D. B., Sales-Pardo, M., Leicht, E. A., Newman, M. E., and Amaral, L. A. N. (2010). Origin of compartmentalization in food webs. Ecology 91, 2941–2951. doi: 10.1890/09-1175.1
Inoue, T., Kato, M., Kakutani, T., Suka, T., and Itino, T. (1990). Insect-flower relationship in the temperate deciduous forest of Kibune, Kyoto: an overview of the flowering phenology and the seasonal pattern of insect visits. Contr. Biol. Lab. Kyoto Univ. 27, 377–463.
Kaiser-Bunbury, C. N., Muff, S., Memmott, J., Müller, C. B., and Caflisch, A. (2010). The robustness of pollination networks to the loss of species and interactions: a quantitative approach incorporating pollinator behaviour. Ecol. Lett. 13, 442–452. doi: 10.1111/j.1461-0248.2009.01437.x
Kakutani, T., Inoue, T., Kato, M., and Ichihashi, H. (1990). Insect-flower relationship in the campus of Kyoto University, Kyoto: an overview of the flowering phenology and the seasonal pattern of insect visits. Contr. Biol. Lab. Kyoto Univ. 27, 465–521.
Kato, M., Kakutani, T., Inoue, T., and Itino, T. (1990). Insect-flower relationship in the primary beech forest of Ashu, Kyoto: an overview of the flowering phenology and the seasonal pattern of insect visits. Contr. Biol. Lab. Kyoto Univ. 27, 309–375.
Lewinsohn, T. M., Inácio Prado, P., Jordano, P., Bascompte, J., and Olesen, J. M. (2006). Structure in plant–animal interaction assemblages. Oikos 113, 174–184. doi: 10.1111/j.0030-1299.2006.14583.x
Maruyama, P. K., Vizentin-Bugoni, J., Oliveira, G. M., Oliveira, P. E., and Dalsgaard, B. (2014). Morphological and spatio-temporal mismatches shape a neotropical savanna plant-hummingbird network. Biotropica 46, 740–747. doi: 10.1111/btp.12170
Morente-López, J., Lara-Romero, C., Ornosa, C., and Iriondo, J. M. (2018). Phenology drives species interactions and modularity in a plant-flower visitor network. Sci. Rep. 8:9386. doi: 10.1038/s41598-018-27725-2
Newman, M. E. (2006). Modularity and community structure in networks. Proc. Nat. Acad. Sci. U.S.A. 103, 8577–8582. doi: 10.1073/pnas.0601602103
Oksanen, J. F., Guillaume, B., Friendly, M., Kindt, R., Legendre, P., McGlinn, D., et al. (2019). Vegan: Community Ecology Package. R Package Version 2.5-4. Available online at: https://CRAN.R-project.org/package=vegan (accessed May 19, 2020).
Olesen, J. M., Bascompte, J., Dupont, Y. L., and Jordano, P. (2007). The modularity of pollination networks. Proc. Nat. Acad. Sci. U.S.A. 104, 19891–19896. doi: 10.1073/pnas.0706375104
Paxton, R. J. (2005). Male mating behaviour and mating systems of bees: an overview. Apidologie 36, 145–156. doi: 10.1051/apido:2005007
Prado, P. I., and Lewinsohn, T. M. (2004). Compartments in insect–plant associations and their consequences for community structure. J. Anim. Ecol. 73, 1168–1178. doi: 10.1111/j.0021-8790.2004.00891.x
R Development Core Team (2019). R: A Language and Environment for Statistical Computing. Vienna: R Foundation for Statistical Computing.
Roswell, M., Dushoff, J., and Winfree, R. (2019). Male and female bees show large differences in floral preference. PLoS One 14:e0214909. doi: 10.1371/journal.pone.0214909
Sauve, A. M., Fontaine, C., and Thébault, E. (2014). Structure–stability relationships in networks combining mutualistic and antagonistic interactions. Oikos 123, 378–384. doi: 10.1111/j.1600-0706.2013.00743.x
Smith, G. P., Bronstein, J. L., and Papaj, D. R. (2019). Sex differences in pollinator behavior: patterns across species and consequences for the mutualism. J. Anim. Ecol. 88, 971–985. doi: 10.1111/1365-2656.12988
Spiesman, B. J., and Inouye, B. D. (2013). Habitat loss alters the architecture of plant-pollinator interaction networks. Ecology 94, 2688–2696. doi: 10.1890/13-0977.1
Stouffer, D. B., and Bascompte, J. (2011). Compartmentalization increases food-web persistence. Proc. Nat. Acad. Sci. U.S.A. 108, 3648–3652. doi: 10.1073/pnas.1014353108
Sugiura, S. (2008). Male territorial behavior of the endemic large carpenter bee, Xylocopa (Koptortosoma ogasawarensis (Hymenoptera: Apidae), on the oceanic Ogasawara Islands. Eur. J. Entomol. 105, 153–157. doi: 10.14411/eje.2008.021
Thébault, E., and Fontaine, C. (2010). Stability of ecological communities and the architecture of mutualistic and trophic networks. Science 329, 853–856. doi: 10.1126/science.1188321
Vázquez, D. P., Blüthgen, N., Cagnolo, L., and Chacoff, N. P. (2009). Uniting pattern and process in plant–animal mutualistic networks: a review. Ann. Bot. 103, 1445–1457. doi: 10.1093/aob/mcp057
Keywords: compartment, mutualism, network, pollinator, sex
Citation: Kishi S and Kakutani T (2020) Male Visitors May Decrease Modularity in Flower–Visitor Networks. Front. Ecol. Evol. 8:124. doi: 10.3389/fevo.2020.00124
Received: 04 December 2019; Accepted: 16 April 2020;
Published: 28 May 2020.
Edited by:
Hang Sun, Kunming Institute of Botany (CAS), ChinaReviewed by:
Kazuhiro Takemoto, Kyushu Institute of Technology, JapanQiang Fang, Henan University of Science and Technology, China
Copyright © 2020 Kishi and Kakutani. This is an open-access article distributed under the terms of the Creative Commons Attribution License (CC BY). The use, distribution or reproduction in other forums is permitted, provided the original author(s) and the copyright owner(s) are credited and that the original publication in this journal is cited, in accordance with accepted academic practice. No use, distribution or reproduction is permitted which does not comply with these terms.
*Correspondence: Shigeki Kishi, a2lzaXNpZ2VraUBnbWFpbC5jb20=