- 1MARBEC, Université de Montpellier, CNRS, IRD, Ifremer, Montpellier, France
- 2MARBEC, Université de Montpellier, CNRS, IRD, Ifremer, Séte, France
- 3CEE-M, Université de Montpellier, CNRS, INRA, Montpellier SupAgro, Montpellier, France
By the end of the 20th century, many of the coastal lagoons along the French Mediterranean coast showed insufficient water quality and degraded ecosystem states due to anthropogenic impacts. Among these, nutrient over-enrichment, resulting in eutrophication, has been a major concern. The EU Water Framework Directive (WFD) has initiated public action to improve their water quality and ecosystem state using an approach rooted in restoration ecology. Here we analyze how this has been applied for the coastal lagoons in South France, considering eutrophication as an example of ecosystem degradation and oligotrophication as the corresponding trajectory for ecological restoration of the eutrophied coastal lagoons. Oligotrophication trajectories, initiated by the reduction of external nutrient loading, have resulted in a quick recovery (i.e., within 3 years) of integrative water column variables (Chlorophyll a, total N and P) and phytoplankton. Starting from hypertrophic systems, the oligotrophication trajectory is described by a sequence of three ecosystem states dominated respectively by (i) phytoplankton with bare non-vegetated sediments, (ii) opportunistic macroalgae, (iii) angiosperm and perennial macroalgae, punctuated by regime shifts between these ecosystem states. Nevertheless, the latter regime shift has not been observed for the most degraded ecosystems after 10-years oligotrophication. The N and P accumulated in sediments during eutrophication may also retard the ecological restoration. In shallow freshwater lakes, the phytoplankton-dominated and the angiosperm-dominated states are also characteristic for highly degraded and fully restored ecosystems states, respectively. In contrast, opportunistic macroalgae do not bloom in these systems. Hence, the alternative stable state model, used successfully for these lakes, cannot be applied straightforwardly for coastal lagoons. To be successful, ecological restoration should consider the legislative and societal questions as according the DPSIR framework it typically is a response of society. The conservation-oriented Habitats Directive systematically applies to coastal lagoons and the Birds Directive in some cases as well. The WFD approach is complementary to these more conventional nature conservation approaches. Collectively, local citizens and highly involved stakeholders strongly value the coastal lagoons and attribute very high importance to their regulating ecosystem services (ESs), while differences between stakeholder profiles are related to different perceptions and appreciations of cultural ESs. Hence, coastal lagoon ESs are very important for the different stakeholders and public policies aiming at the ecological restoration of these ecosystems may count on public support.
Introduction
The EU Water Framework Directive 2000/60/EC (WFD) presents a highly integrated approach focused on water quality, which according Voulvoulis et al. (2017) is widely accepted as the most substantial and ambitious piece of European environmental legislation to date. Interestingly, the major aim of this directive is to improve the ecological status of water bodies, which calls for a pro-active approach rooted in the theory of restoration ecology. In general, a difference is made in the literature between restoration ecology and ecological restoration; the latter is the process of assisting the recovery of an ecosystem that has been degraded, damaged, or destroyed (Society for Ecological Restoration International Science and Policy Working Group, 2004), The former, restoration ecology, is a scientific discipline, a field within ecology, focused on studying the concepts, experiences and practices of ecological restoration (Clewell and Aronson, 2013). Both restoration ecology and the WFD advocate that the approaches for ecological restoration should be based on (i) the identification of a reference state, (ii) a description of a desired state that is quite close to the reference state, and (iii) the development of a roadmap, which specifies how the ecosystem trajectory should converge toward that desired state. For restoration ecology, the reference state is a historical reference state referring to the ecosystem state before it was degraded by human impacts (Clewell and Aronson, 2013). The reference state according the WFD is the ecosystem state in conditions of minimal anthropogenic impact. Hence, for a degraded aquatic system considered for ecological restoration, this implies that such conditions of minimal anthropogenic impact occurred in the past and the reference state can thus be considered as a historical reference state sensu restoration ecology.
The Ecosystem state of many coastal lagoons worldwide has been degraded as a result of anthropogenic impacts comprising nutrient over-enrichment leading to eutrophication (Zaldívar et al., 2008), habitat destruction (De Wit, 2011; Newton et al., 2018), contaminant inputs (Covelli, 2012; Munaron et al., 2012). Environmental awareness of citizens, the practice of Integrated Coastal Zone Management (ICZM), and specific legislation derived from the EU, i.e., the WFD mentioned above together with the EU Habitats Directive 1992 (Council Directive 92/43/EEC) and the EU Birds Directive (2009) (Directive 2009/147/EC), have triggered public policies in the EU member states that value these ecosystems and aim to protect and improve their water quality, protect public health issues, conserve their biodiversity and develop their ecological potential for the delivery of ecosystem services. The WFD considers the ecological functioning of the aquatic ecosystems, including coastal lagoons, and formulates its main objectives as conserving and achieving “good ecological status” of water bodies. Therefore, the WFD is particularly important for biodiversity conservation in the EU and it has been stated that:
“the legislative framework in place to achieve the Biodiversity Strategy in aquatic ecosystems (in the EU, sic) can be linked to a complex array of interlinked policies, of which the most far-reaching ones are the Birds and Habitats Directives, Water Framework Directive, and Marine Strategic Framework Directive”
The latter has fewer implications for coastal lagoons, for which the water quality regulations are derived from the WFD. Coastal lagoons are listed as a priority habitat in the Habitats Directive and many coastal lagoons are particularly important for waterfowl and concerned by the Birds Directive (Dolbeth et al., 2016). Article 6 of the WFD requests member states to establish a register of areas which have been designated as requiring special protection under specific Community legislation for the conservation of habitats and species directly depending on water and WFD Annex IV explicitly links this requirement to the Natura 2000 sites designated according the Habitats and Birds Directives.
In this article, we use eutrophication as an example of ecosystem degradation, and oligotrophication as the corresponding trajectory for the ecological restoration of eutrophied lagoons. The many actions employed in South France to combat eutrophication of coastal lagoons and induce their oligotrophication thus represents an interesting study case for assessing ecological restoration according the theoretical framework of restoration ecology. We believe that such an analysis should particularly address the issues to which coastal lagoon managers are confronted in their daily practice. Therefore, first we propose a conceptualization placing the ecological restoration in societal context and adapt the conceptual scheme in such a way to accommodate the specificities of the WFD. Secondly, our study includes an analysis of the technical and natural science aspects of the ecological restoration practice as well as important questions concerning the societal context. Therefore, we have formulated the following three main questions (Figure 1):
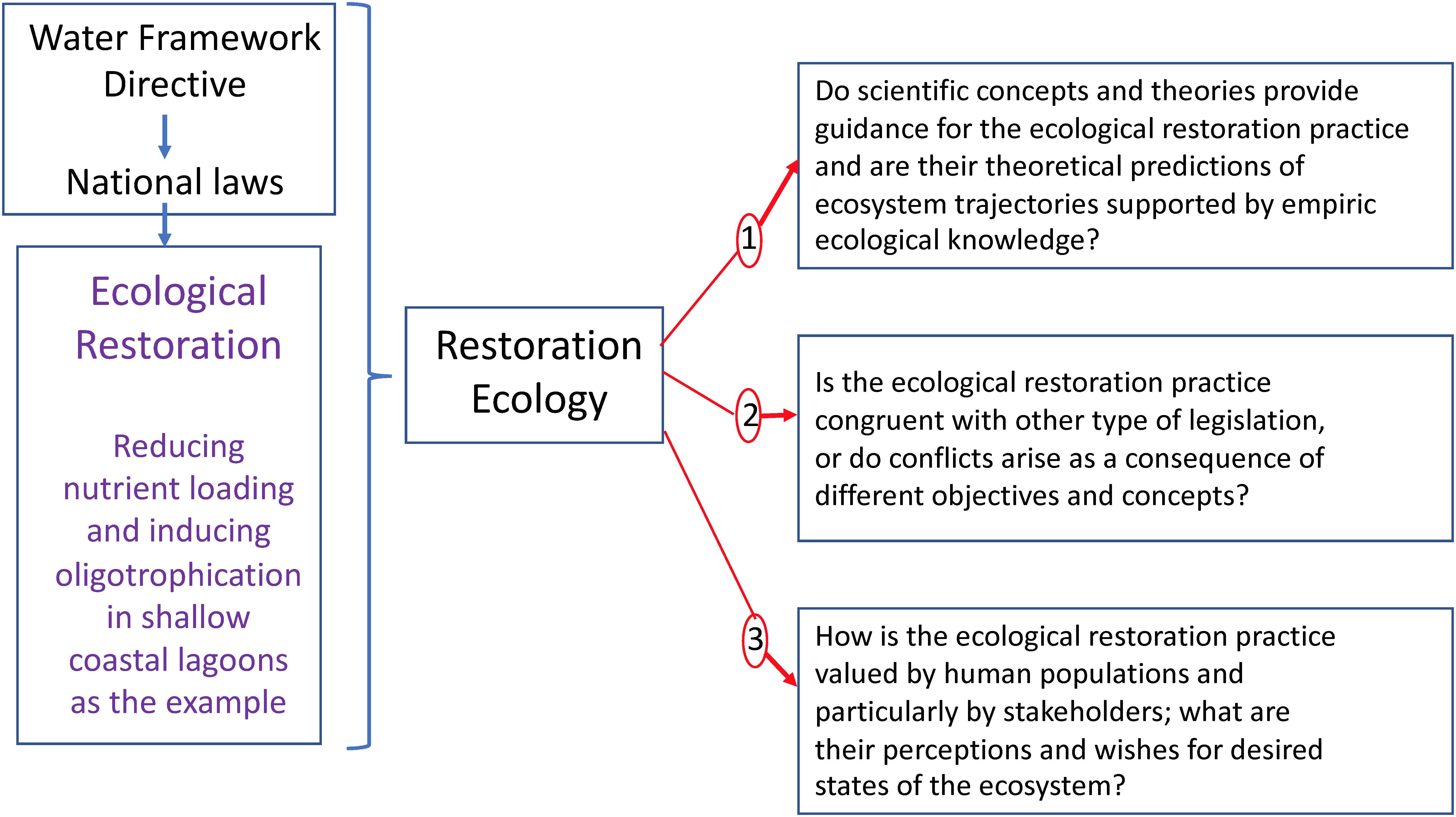
Figure 1. The Water Framework Directive (2000/60/EC) through its transposition into national laws [in France: Loi n° 2006–1772 du 30 décembre 2006 sur l’eau et les milieux aquatiques (French law)] has resulted in South France in ecological restoration practice to improve the ecological status of coastal lagoons. A particular focus has been on reducing nutrient loading to combat eutrophication and thus induce oligotrophication of coastal lagoons. In this article this practice has been analyzed according the theoretical framework of restoration ecology and three main questions have been formulated for this assessment.
1. Do scientific concepts and theories provide guidance for the ecological restoration practice and are their theoretical predictions of ecosystem trajectories supported by empiric ecological knowledge?
2. Is the ecological restoration practice congruent with other type of legislation, or do conflicts arise as a consequence of different objectives and concepts?
3. How is the ecological restoration practice valued by human populations and particularly by stakeholders; what are their perceptions and wishes for desired states of the ecosystem?
Ecological restoration of coastal lagoons has started around 2000 and is, therefore, quite novel in contrast to the ecological restoration of water quality in freshwater lakes initiated since the 1980s (Marsden, 1989; Gulati and Van Donk, 2002; Jeppesen et al., 2002). For providing scientific guidance for the technical aspects, we consider if the lessons learned from the ecological restoration of freshwater lakes can be applied to the brackish to saline coastal lagoons located at the interface between the land and the sea. A general model of alternative stable states was developed originally for shallow freshwater lakes (Scheffer et al., 1993), with a transparent water state dominated by submerged aquatic vegetation (SAV) and a turbid state dominated by phytoplankton. Hence, here we evaluate whether this model can also be applied to shallow coastal lagoons. In addition, we consider the sediment compartment as a possible internal source for nitrogen and phosphorus that could retard the oligotrophication process as has been observed in shallow freshwater lakes (Marsden, 1989; Søndergaard et al., 2003; Jeppesen et al., 2005). We report the different approaches for coastal lagoons in the WFD and the Habitats Directive and discuss the challenges faced by the managers of these ecosystems to cope simultaneously with the requirements of both directives. Concerning the social aspects, we study whether the normative approach for water quality imposed by the WFD is accepted by the local populations and stakeholders by studying their perceptions of water quality and their preferences for ecosystem services.
Study Sites and Methods
The study sites comprise the shallow coastal lagoons (average depth <2 m, surface >50 ha) along the Mediterranean coastlines of continental France and the island of Corsica, which are shown in Figure 2. Deeper coastal lagoons (average depth >2 m, in gray in Figure 2) have been excluded from this analysis as deeper water bodies show different ecological structure and functioning. In addition, deep lagoons are often complex systems comprising both shallow and deeper parts that may interact in a complex way. The terminology based on their salinities follows the Venice System (1958) and was described based on monitored salinity values in Le Fur et al. (2018). The shallow lagoons in Figure 2 cover 396 km2. Coastal lagoons occupy about 50% of the coastline along the Gulf of Lion, are numerous in the delta of the Rhône River and less common on the Côte d’Azur. In Corsica, coastal lagoons are located on the Eastern littoral facing the Tyrrhenian Sea.
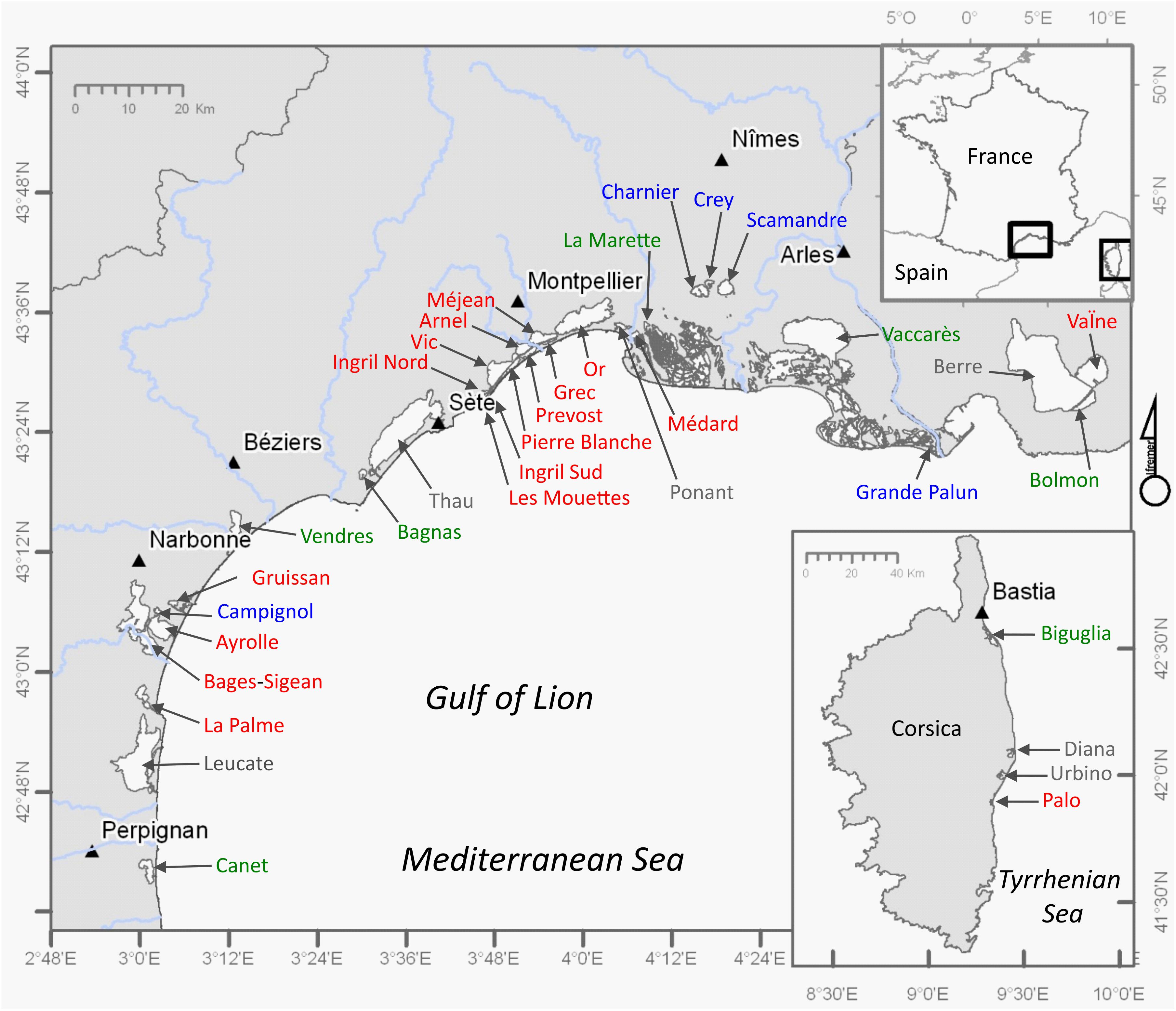
Figure 2. Distribution of coastal lagoons, recognized for the WFD implementation in France, along the Mediterranean coastlines of continental France and the island of Corsica. In gray: deep lagoons (>2 m) not considered in this study. In blue: shallow (<2 m) oligohaline lagoons; in green: shallow (<2 m) mesohaline lagoons; in red: shallow (<2 m) poly- and euhaline lagoons. Triangles represent main urban centers (>40,000 inhabitants) in the proximity of the coastal lagoons.
Coastal lagoons are characterized by permanent or temporary connections with the adjacent sea, via one or several inlets (Kjerfve, 1994). Today, very few of the shallow lagoons shown in Figure 2 have natural moving inlets (i.e., La Palme, Ayrolle, Biguglia), which only for La Palme lagoon close off completely every year (Larue and Rouquet, 2016). The natural inlet of Biguglia also tends to fill in, although it is regularly dredged to keep a permanent connection with the sea. In many cases, inlets have been modified by humans by construction of hard-substrate artificial inlet banks and are being dredged regularly. This has fixed the locations of the inlets and resulted in a permanent connection of the lagoons with the sea. In some areas artificiality is even more striking. Some of the permanent lagoons in the deltaic setting have been separated from the sea several km’s by the progression of the delta; the connections with the sea are maintained through artificial canals (Scamandre, Crey, Charnier) or through a wetland complex protected by a dike (Vaccarès). The Palavas lagoon complex (PLC) (in French: complexe lagunaire Palavasien) close to the city of Montpellier currently comprises eight lagoons (Ingril Nord, Ingril Sud, Vic, Pierre Blanche, Prévost, Arnel, Méjean, Grec) that have been created through the compartmentalization of the original “lagune de Mergueil.” The creation of the Rhône-to-Sète canal since the 18th century has separated four permanent lagoons (Ingril Nord, Vic, Arnel, Méjean) from the coastline and exchanges of these lagoons with the sea occur through this canal.
To address the questions listed in Figure 1 and described in section “Introduction” we use a combination of studies. The natural science information in this article is based on reviewing and synthesizing our previous studies (see Table 1), literature review including some gray literature that has been complemented with some additional unpublished data from three Ph.D. thesis (Leruste, 2016; Le Fur, 2018; Sy, 2019) and additional monitoring data from monitoring programs extracted from the French data bases “Banque Hydro1,” Naïade2, and “Surval,” which among others hosts the data of the Réseau Suivi Lagunaire (RSL) monitoring program (Ifremer3). In the RSL, the total Nitrogen (TN) and Total Phosphorus (TP) data of the top 5 cm of the sediment (sampled at 6-year intervals) were expressed as N and P contents in g/kg dry weight of sediments. Due to variable water contents and densities of the sediments it is not possible to convert these values to an aerial basis. These values were converted to mmol/kg for a stoichiometric analysis.
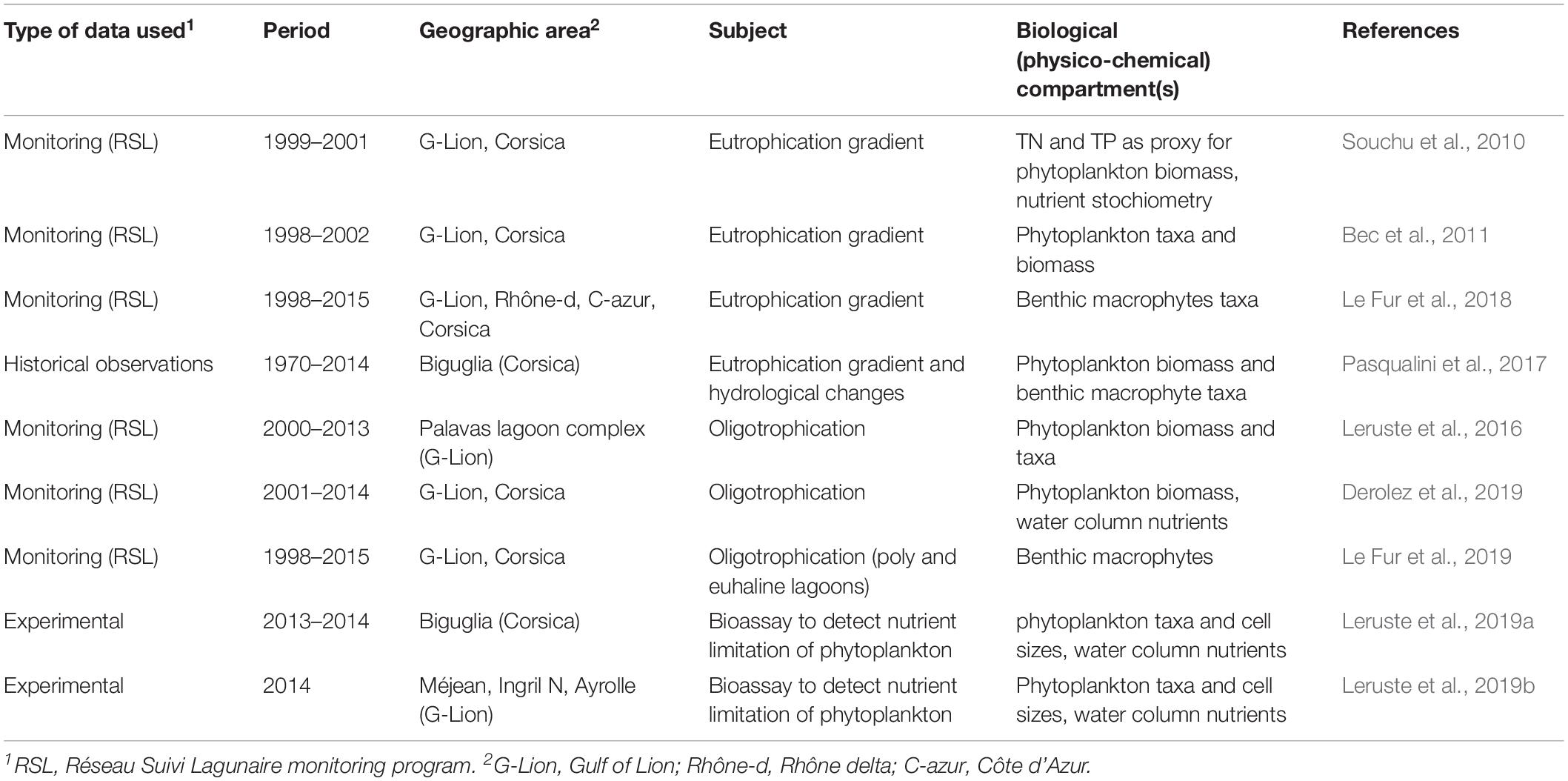
Table 1. Scientific papers used to synthesize and review the information, which served to create the knowledge base about eutrophication gradients and oligotrophication trajectories in shallow Mediterranean coastal lagoons in South France.
The question of the congruencies between WFD and Habitats directives has been based on analysis of legal texts and technical documents of the European Commission. In addition to the previous studies on citizens and stakeholder perceptions and opinions reviewed in this article, it also includes new results from citizen workshops organized in 2017 and 2018 at two different places in the eastern and western vicinity of the PLC. Forty-three randomly selected citizens working or recreating in both parts of the lagoon complex participated in the workshops (see Supplementary Tables S1, S2 for details of the survey). The methodology was based on Sy et al. (2018) using Q-method to analyze consensus and diverging preferences of local citizens for ecosystem services provided by these lagoons. The results obtained for the citizens were compared with those obtained for the highly involved stakeholders (Sy et al., 2018).
Results and Discussion
Concept of Ecological Restoration in the Frame of the WFD
Ecological Restoration is a human action focused on degraded ecosystems that can thus be taken into account by the DPSIR framework, as this framework aims at analyzing the interactions between humans and ecosystems. Accordingly, ecological restoration should be considered as a Response (see Figure 3). In general, responses in DPSIR can potentially target D (drivers), P (pressures), S (state), and I (impact), although for ecological restoration it is clear that drivers and impacts are not really pertinent targets. Acting on major drivers is beyond the scope of action for ecological restoration sensu stricto; i.e., increasing urbanization and intensification of agriculture are drivers that could potentially be changed by spatial planning and agricultural policies, respectively, but not directly by ecological restoration. As ecological restoration has the ambition to repair degraded ecosystems it does not make sense to target the impacts; this would correspond to combating the symptoms and thus completely neglects the major objectives set for ecological restoration. Hence, ecological restoration could target the P (pressures) and the S (state).
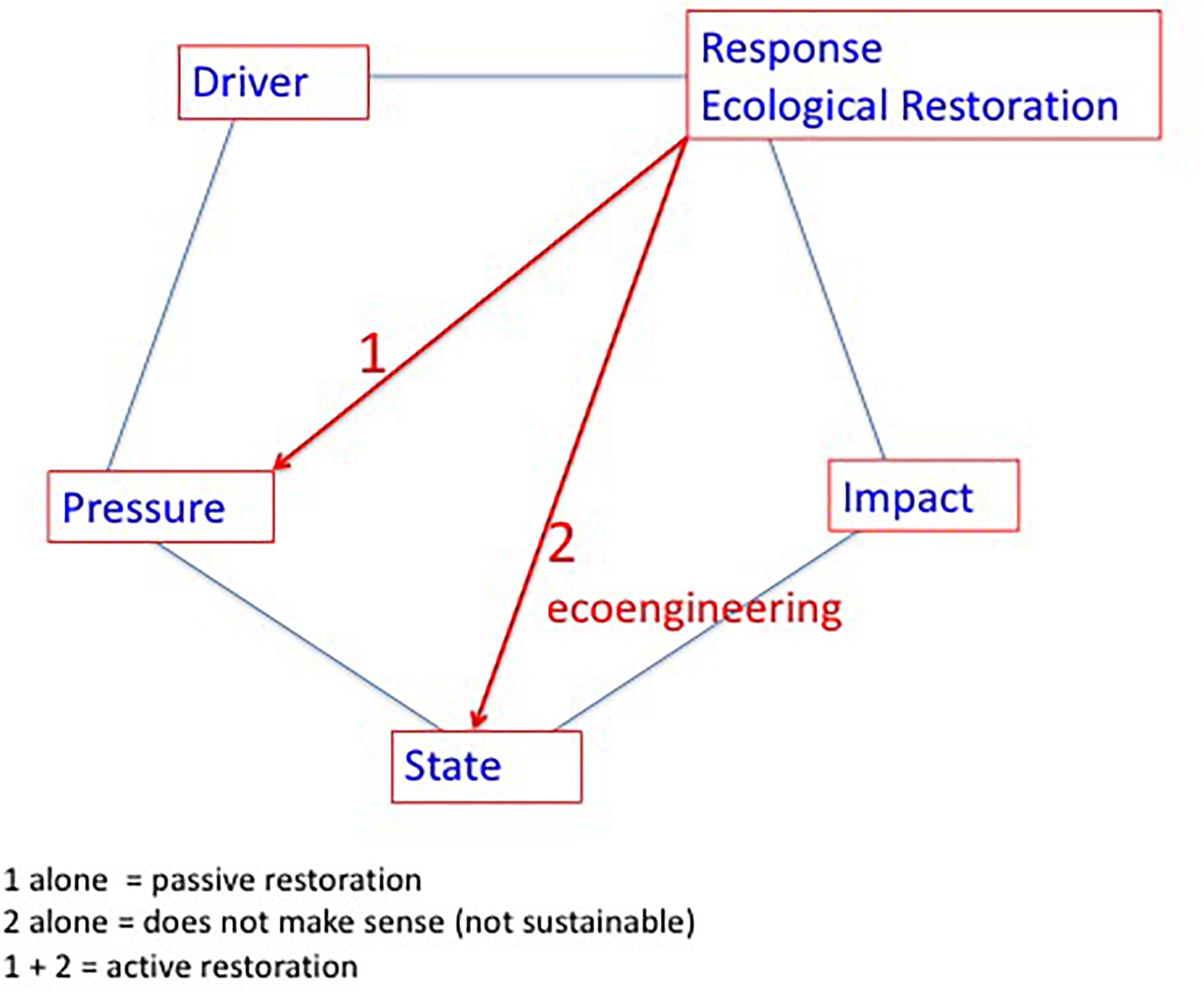
Figure 3. Placing the Ecological Restoration practice in the D (drivers), P (pressures), S (state), I (impact), and R (response) framework. Accordingly, Ecological Restoration clearly is a societal response that targets 1) reducing the pressure on the ecosystem and 2) interfering directly with the ecosystem state through eco-engineering.
For the problem of eutrophication of coastal lagoons, the pressure corresponds most often to nutrient over-enrichment mainly caused by nutrient loadings from their watershed. Hence, targeting the pressure thus corresponds to reducing the nutrient loading into the coastal lagoons. This action induces the reverse of eutrophication, i.e., oligotrophication. However, there is some debate about terminology, e.g., the term re-oligotrophication has been coined to describe the trajectories for lakes (Jeppesen et al., 2002) and coastal lagoons (Le Fur et al., 2019). This latter term seems to imply that the eutrophied systems were originally oligotrophic and that the trajectory should lead to full oligotrophic conditions. As not all coastal lagoons were originally oligotrophic (Nixon, 2009), it is preferable to use the more neutral term oligotrophication, which designates a process of moving toward more oligotrophic conditions. Moreover, in some cases the term de-eutrophication has been used.
An example of targeting the state (Figure 3) is seeding or planting marine angiosperms (Orth et al., 2012, MEPS thematic section, Van Katwijk et al., 2016), which are indicator species for good ecological conditions in coastal lagoons. Nevertheless, it is obvious that such eco-engineering activities alone without tackling the pressures on the system is not sustainable and would most likely result in failure in the short or mid-term (Van Katwijk et al., 2009; Cunha et al., 2012), a conclusion that has been confirmed in a recent study (Van Katwijk et al., 2016). We differentiated between passive and active ecological restoration (De Wit et al., 2017). Accordingly, passive ecological restoration is defined by action focused on reducing and combating the pressure (1 alone in Figure 3) and relies on the spontaneous ecological processes in the ecosystem for a trajectory toward improved conditions. Active ecological restoration implies a combination of reducing the pressure on the ecosystem in conjunction with eco-engineering in situ to improve the state of the ecosystem directly. A pertinent example for active ecological restoration in coastal lagoons is to combine a reduction of nutrient loading with seeding or planting marine angiosperms.
Figure 4 is the conceptual presentation derived from restoration ecology (Clewell and Aronson, 2013) that can be used as a roadmap and has been adapted to accommodate the specific requirements of the WFD. In the WFD, ecological status is measured using indicators and calculated as an ecological quality ratio (EQR; Zaldívar et al., 2008), with EQR being equal to one for the reference state. Hence, the EQR measures the deviation from reference conditions. The reference state is then taken as the ideal target for the ecological restoration, which is considered according WFD as high ecological quality status. Nevertheless, the WFD is flexible and for achieving the target allows “low levels of distortion resulting from human activity,” which “deviate only slightly from those normally associated with the surface water body type under undisturbed conditions” (WFD, Annex V) and ecological quality status are characterized as good for these conditions. Hence good or high ecological status correspond to the “Desired state” of the system that should be achieved in the future before a given deadline. After reduction of the pressure it is presumed that the system will move gradually toward this desired state, probably passing through one or multiple transient states. The recovery can be speed up by combining the reduction of the pressure with additional eco-engineering measures. The trajectories in the context of this study correspond to the different oligotrophication trajectories for the different scenarios. Hence, scientific knowledge of oligotrophication processes in coastal lagoons is of paramount importance for managers.
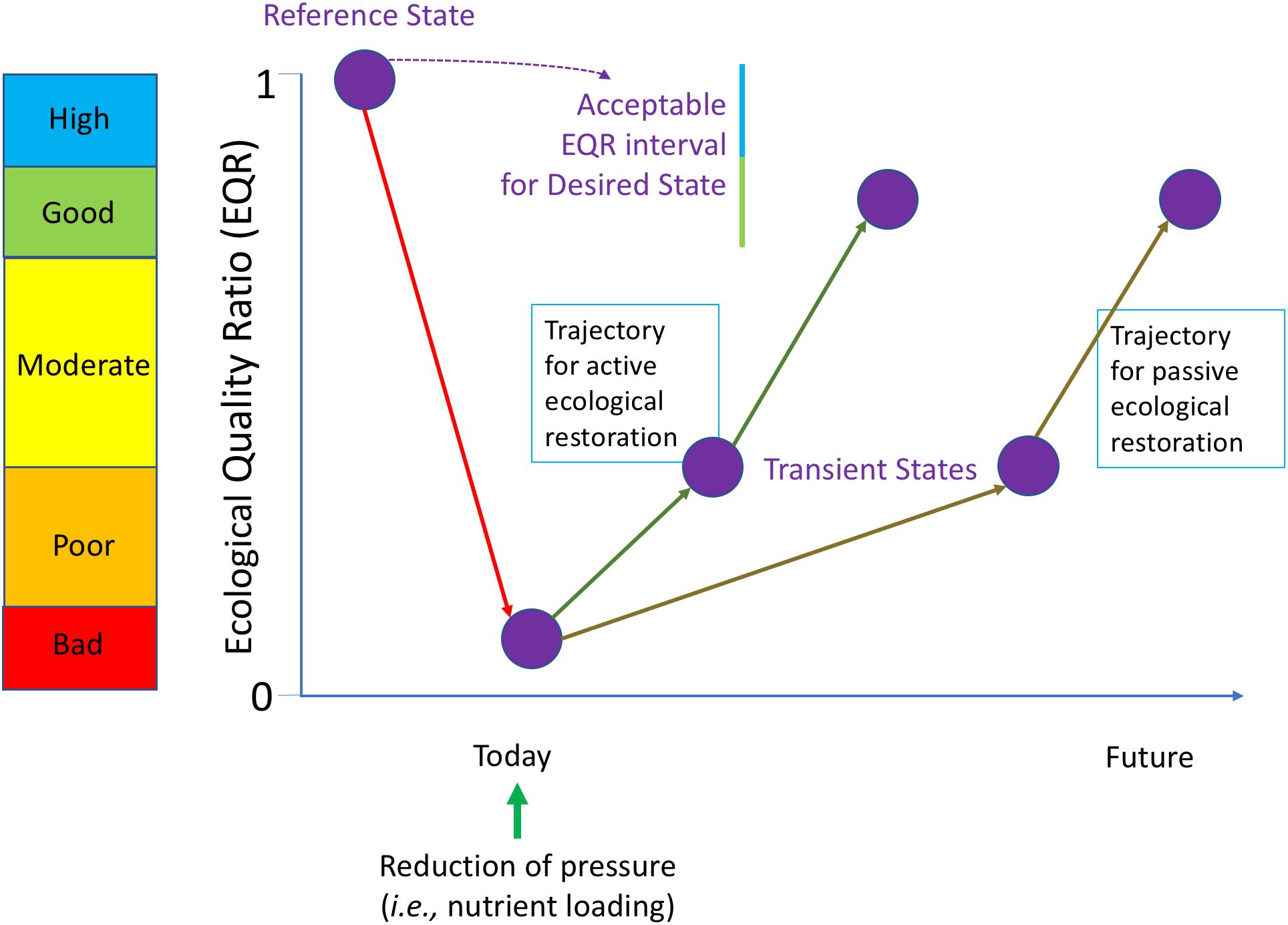
Figure 4. Roadmap for ecological restoration applied in the frame of the WFD. The ecosystem states are characterized by a set of biological quality elements (BQE, e.g., phytoplankton, macrophytes) used as indicators and expressed as an Ecological Quality Ratio (EQR) represented on the Y-axis, with their qualifications for the corresponding ecological status (color coding). A historical ecosystem state not impacted by human pressures is taken as the reference condition for the ecological restoration. The WFD allows a small deviation from these reference conditions by targeting good or high ecological status for the Desired State of the coastal lagoons. After reduction of the pressure it is presumed that the degraded ecosystem (in the example currently in bad ecological status) will move gradually toward the Desired State, probably passing through one or multiple transient states (passive restoration). The recovery can be speed up by combining the reduction of the pressure with additional eco-engineering measures (active restoration, cf. Figure 3).
Can Scientific Knowledge of Eutrophication and Oligotrophication Trajectories in Mediterranean Coastal Lagoons Be Used to Provide Guidance for Ecological Restoration Actions?
Nutrient Loading Into Coastal Lagoons
In general, the demographic developments in the coastal zone since the 1950s and the resulting increased urbanization with insufficient sanitation and sewage treatments were the main drivers for the nutrient over-enrichment in the shallow coastal lagoons, particularly for those close to the cities of Montpellier, Narbonne, and Perpignan (cf. Figure 2). The urbanization to the south of Bastia accelerated later and particularly increased during the first decade of the 21st century (Pasqualini et al., 2017). Subsequently, action has been undertaken in these areas to reduce the nutrient loadings by improved sanitation (Leruste et al., 2016; De Wit et al., 2017; Pasqualini et al., 2017; Derolez et al., 2019; Le Fur et al., 2019). The case of the PLC is described in more detail. In the coastal lagoons of this complex, the bulk of the nutrient inputs originated from the urban waste-water treatment plant (WWTP) of the Montpellier agglomeration that discharged its effluents in the Lez River. As a result, most nutrients entered these lagoons via the Lez River and the Rhône-to-Sète canal. In 2005, a major investment was realized of 150 Million€ to upgrade the waste-water treatment facility in Montpellier, and create an off-shore outfall (Leruste et al., 2016; De Wit et al., 2017). All eight lagoons of the PLC benefited from this investment. In 2009, the WWTP of the city of Palavas (a smaller facility) was connected to the main WWTP in Montpellier, which resulted in an additional major reduction of nutrient loading into the Grec lagoon (Le Fur et al., 2019).
The annual fluxes of N and P carried by the Lez River are presented in Figure 5. This complete charge does not enter the lagoons as part flows through the river mouth directly into the Mediterranean Sea. Nevertheless, the Lez River represent the major tributary to the coastal lagoons of the PLC and this Figure 5 gives therefore a good indication of the nutrient enrichment pressure on the system. From the Lez River, some water directly leaks into the Méjean and Arnel lagoons, but most enters the lagoons through the Rhône-to-Sète canal. As a result, the lagoon complex showed in the early 2000s an interesting gradient ranging from hypertrophic conditions in the lagoons close to the intersection of the Lez River and the canal to mesotrophic conditions in the two Ingril lagoons located farthest away (Bec et al., 2011; Souchu et al., 2010; Leruste et al., 2016; De Wit et al., 2017). Comparing the periods before (2003–2005) and after (2007–2012) showed decreases of 75 and 87% of total N and P, respectively (see Figure 5). Considering all other sources together with the major contribution from the Lez River it has been estimated that the intervention resulted in a reduction of the nutrient loadings from the watershed into the PLC of 83 and 73%, for total N and total P, respectively (Meinesz et al., 2013). Moreover, in the Lez River there was a strong shift in the relative proportions of NH4+ and NO3–, with NH4+ representing 79 and 20% of dissolved inorganic nitrogen (DIN), before and after, respectively. Before, organic nitrogen, i.e., the sum of dissolved and particulate organic nitrogen (DON and PON, respectively), represented 23% of total N and dropped to virtually 0 after the intervention (Figure 5).
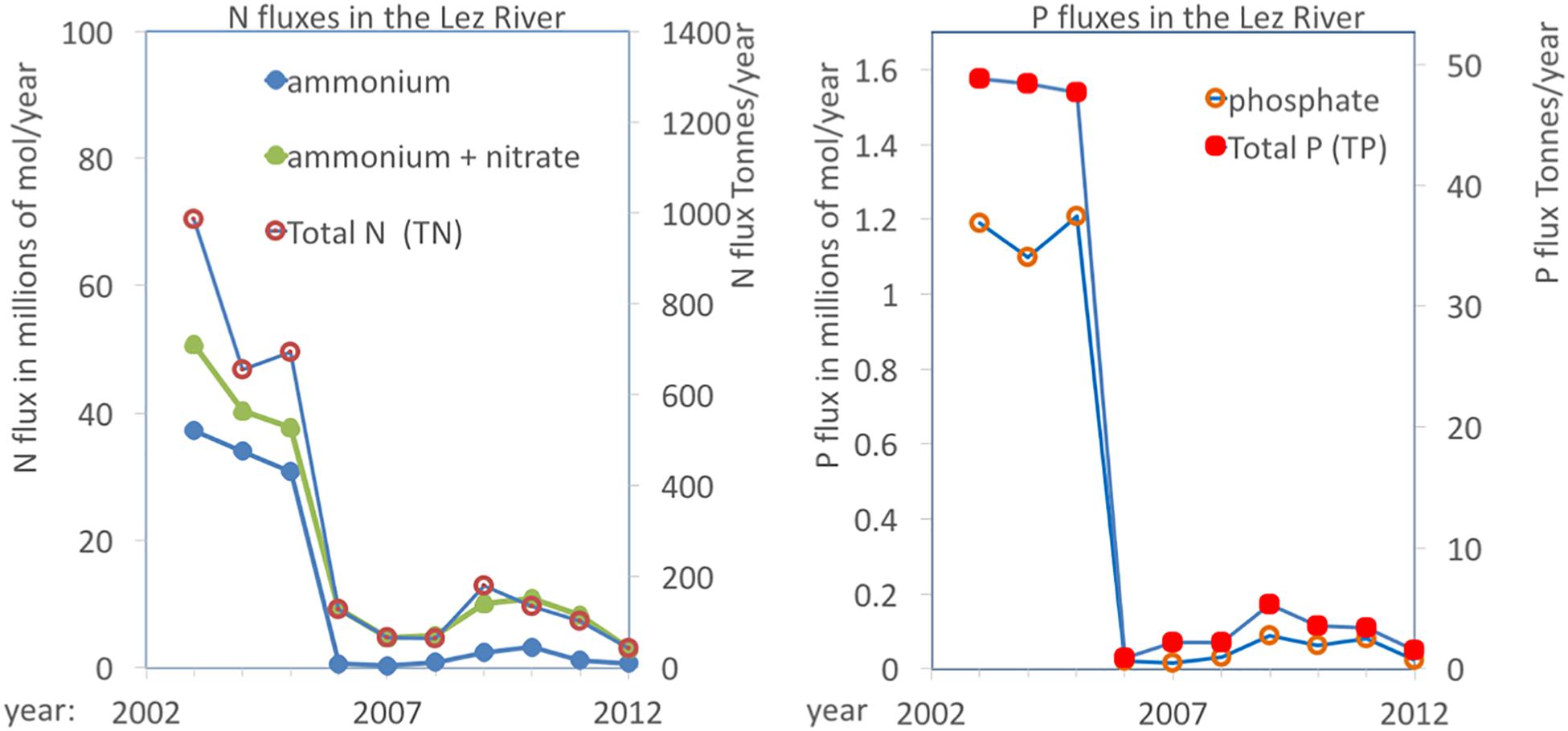
Figure 5. Annual fluxes of N and P compounds in the Lez River. Values can be read on both scales (left in millions of mols/year; right in metric tonnes/year for N and P, respectively). Source: “Banque Hydro” and “Naïade” (réseau de données eaufrance, DCE).
What Can Be Learned From Shallow Lakes for Succession Patterns of Primary Producers in Mediterranean Coastal Lagoons?
Decades before tackling the eutrophication problems in coastal lagoons, the ecological restoration of aquatic systems started with the oligotrophication of shallow freshwater lakes. Therefore, it is inspiring to review freshwater lake oligotrophication and tempting to use it as a guideline for the ecological restoration of coastal lagoons. Scheffer (2001) stated “Ponds and shallow lakes can be very clear with abundant submerged plants, or very turbid due to a high concentration of phytoplankton and suspended sediment particles.” This statement has been related to the alternative stable state theory (Scheffer et al., 1993). Accordingly, two attractors exist for these type of ecosystems, i.e., the SAV stable state attractor and the turbid stable state attractor, which dominate at very low and very high nutrient loadings, respectively. At intermediate nutrient loadings both attractors coexist and mathematically the ecosystem shows these two alternative stable states, which are each stabilized by a mixture of positive and negative feedback loops (Scheffer et al., 1993). Hence, a window of environmental conditions exists with alternative stable states in these aquatic ecosystems, where the actually occurring ecosystem state depends on the history of the system. Following the Scheffer model for shallow lakes, for environmental conditions within the window, the system can remain in the SAV state during increasing eutrophication until it reaches a critical turbidity, which is imposed by the minimum light requirements for growth and survival of the SAV. Above this threshold, the aquatic ecosystem shows a forward regime shift toward the turbid state. On the other hand, when nutrient loading is reduced in a turbid eutrophic lake, the system remains in the turbid state until the phytoplankton densities have decreased to such low values with a corresponding turbidity below the threshold. Hence, the model provides one possible explanation for hysteresis during eutrophication/oligotrophication trajectories. In addition, it can predict vulnerability of ecosystems states to perturbation, as for conditions within the window of two alternative stable states a perturbation may act to induce a regime shift (Beisner et al., 2003), e.g., a perturbation that causes a sudden die-back of SAV could result in a regime shift into the turbid state.
We may now ask the question, whether these two attractors and corresponding ecosystem states also exist for coastal lagoons? In general, the above-mentioned statement of Scheffer (2001) is pertinent for coastal lagoons as well. The clear water SAV dominated state in eu- and polyhaline lagoons is found under oligotrophic conditions and corresponds to the marine angiosperms, Zostera noltei Hornemann, 1832, Ruppia cirrhosa (Petagna) Grande, 1918, Ruppia maritima Linnaeus, 1753, Cymodocea nodosa (Ucria) Ascherson, 1870 and some slow-growing perennial macroalgae as e.g., Acetabularia acetabulum (Linnaeus) P. C. Silva, 1952, and Valonia aegagropila C. Agardh, 1823 (Le Fur et al., 2018, 2019). On the other hand, the highly turbid state is characterized by dense phytoplankton blooms often dominated by small phytoplankton (Bec et al., 2011; Leruste et al., 2016), belonging to picophytoplankton (<3 μm size; Bec et al., 2011) and ultraphytoplankton (>3 μm and <5 μm size; Li, 1995), with Chlorophyll a (Chl a) concentrations that may achieve several hundreds of mg/m3. Clear examples of the latter are Or lagoon and before 2005 Méjean lagoon as well. Both ecosystem states have similar positive and negative feedback loops as those observed for their freshwater counterparts that stabilize these ecosystem states (Maxwell et al., 2017; Le Fur et al., 2019).
The succession patterns of primary producers with increasing eutrophication in Mediterranean coastal lagoons (Le Fur et al., 2018) are different from that of freshwater lakes. Accordingly, Le Fur et al. (2018) observed that poly- and euhaline lagoons follow the same general pattern as described by Schramm (1999) for the shallow coastal zone; i.e., with increasing eutrophication four stages have been observed, comprising successively (i) healthy marine angiosperms and perennial macroalgae, (ii) declining angiosperms with increasing loads of epiphytes, (iii) opportunistic macroalgae, and (iv) phytoplankton and bare non-vegetated sediment. In contrast, oligohaline coastal lagoons are dissimilar, because no blooms of macroalgae do develop. Hence, shallow oligohaline coastal lagoons are more similar to freshwater lakes. The dominant SAV in the Mediterranean oligohaline lagoons are charophytes and the angiosperm Stuckenia pectinata (Linnaeus) Börner, 1912 (formerly Potamogeton pectinatus). The latter species form long stems allowing an important proportion of its leaves to float at the surface of the water. This way, this species escapes from competition with planktonic algae for light. Mesohaline coastal lagoons are intermediate between the oligohaline on one side and the poly- and euhaline lagoons on the other side. As in poly- and euhaline lagoons, opportunistic macroalgae develop at intermediate eutrophication levels. On the other side, Ruppia species are characteristic angiosperms in mesohaline lagoons, which like S. pectinata form long stems with floating leaves (Le Fur et al., 2018).
In conclusion, in meso-, poly-, and euhaline lagoons, the Scheffer model for shallow freshwater lakes is not directly applicable as a third group, i.e., opportunistic macroalgae, can dominate primary producer communities and as a rather persistent community represent a third stable state in addition to the angiosperms and planktonic algae stable states. By comparison, the stage of declining angiosperms with increasing loads of epiphytes appears as a transient state, that is not stable because the developing epiphytes weaken their own support. It thus appears that the opportunistic macroalgae occupy a niche in the window where bistability of the two end members could occur. However, so far no clear mathematical analysis allows to determine whether the opportunistic macroalgal states completely overrules the theoretical window of bistability, or whether multiple stability domains exist in coastal lagoons. Increasing eutrophication in these coastal lagoons is correctly characterized by regime shifts according to the Schramm scheme (Le Fur et al., 2018). Nevertheless, threshold effects and regime shifts do not necessarily imply multiple stable states, as very steep but continuous shifts in equilibrium states are also possible (Petraitis and Hoffman, 2010). Viaroli et al. (2008) suggested two possible mechanisms for the regime shift from angiosperms to floating macroalgae, i.e., either as the conventional mechanism based on continuous shift or on a so-called dynamic shift implying a domain of bi-stability. One conclusion from their work, i.e., “The alternative states which occur through the transition from pristine to modified primary producer communities can also be viewed as a sequence of stable states…….” (Viaroli et al., 2008) is a bit ambiguous in this respect. We think that modified primary producer communities along eutrophication gradients in coastal lagoons should indeed be viewed as a sequence of stable states, but that it remains so far, uncertain whether these represent continuous shifts in equilibrium states or whether it hides multiple stable state domains.
Oligotrophication Trajectories
Eight-year to seventeen-year time series from monitoring programs have been used to study the oligotrophication trajectories in the French Mediterranean coastal lagoons, which allowed to make comparisons before and after nutrient reduction measures (Leruste et al., 2016; Derolez et al., 2019; Le Fur et al., 2019). In addition, a longer time series of more heterogeneous observations was available for Biguglia lagoon (Pasqualini et al., 2017). The monitoring effort was concentrated during summer and based on three samplings in June, July, and August, successively. Water column variables, including nutrients (NO3–, NO2–, NH4+, PO43–, silicates), TN, TP, Chl a, and the pico- and nanophytoplankton cell abundances in 16 lagoons, including shallow and deeper lagoons, have been analyzed by a between-station principal component analysis (PCA). The trajectories of 14-year time series for some selected stations in shallow lagoons (Ayrolle, Bages, Méjean) have been plotted in the PCA plane (Derolez et al., 2019). The first axis of this PCA, which explained 81% of the variation, correlated mainly with Chl a, TN, TP, and dissolved inorganic phosphorus (DIP, i.e., PO43–), and was clearly interpreted as the main eutrophication axis. The second axis (11% of variance explained) was correlated to DIN. The station in Méjean lagoon, which was hypertrophic before 2005, moved toward oligotrophy, although it was still eutrophic in 2014. The station in Bages, which was eutrophic before 2004 also moved toward oligotrophy, albeit less abruptly than the station in Méjean. It was concluded that integrative water column variables (Chl a, TN, and TP) recover quickly, i.e., within 1–3 years after nutrient reduction measures (Derolez et al., 2019).
Changes in phytoplankton communities following nutrient reduction measures were studied for the coastal lagoons of the PLC (Leruste et al., 2016). The Chl a concentrations in the hypertrophic Méjean were 185 ± 131 μgL–1 before and dropped on average by one order of magnitude after the nutrient input reduction (cf. Figure 5), with a concomitant drop of autotrophic picoeukaryotes cell numbers. There was a shift from small diatoms that still dominated in 2006 to green algae. The other lagoons of the PLC also showed significant decreases in Chl a after nutrient input reduction and increases in the proportions of peridin-containing dinophytes (Leruste et al., 2016), probably related to their mixotrophic capacities (phagocytosis and osmotrophy). Oligotrophication in shallow freshwater lakes also results in a drop of Chl a concentrations and phytoplankton abundance with the species composition shifting toward diatoms, cryptophytes and chrysophytes (Jeppesen et al., 2005). It has been observed that summer phytoplankton communities in the Mediterranean coastal lagoons in South France are clearly limited by P under oligotrophic conditions and with increasing eutrophication level change through P/N co-limitation to N-limitation (Souchu et al., 2010). It appears that this tendency is maintained during the oligotrophication trajectories and perhaps even strengthened. Hence, during the summer of 2014, bioassay experiments showed that the phytoplankton community in Méjean lagoon was clearly N-limited. The ambient concentrations of DIN and DIP were around 1 and 2.5 μM, respectively, showing excess of DIP. In contrast, a co-limitation by N and P was observed in the eutrophic Biguglia, the mesotrophic Ingril and the oligotrophic Ayrolle lagoons (Figure 2); the latter was studied for comparisons. In the PLC, the phytoplankton communities of the Ingril and Méjean lagoons strongly responded to the experimental nutrient pulses, suggesting that despite their oligotrophication trajectories, these lagoons were still vulnerable to occasional eutrophication events (Leruste et al., 2019a, b).
The impact of the oligotrophication process on the macrophyte assemblages was studied in 21 poly- and euhaline lagoons, by comparing the taxonomic composition of the macrophytes sampled at different stations in the lagoons with variables characterizing the water column using 17 years of observations (Le Fur et al., 2019). The observations were again restricted to the summer period. The data comprised a series of paired data tables, i.e., for species and the other for the water column environmental conditions. Two axes were considered for the multivariate analysis (STATICO factor map); the first axis (88.5% of total variability explained) correlated with Chl a concentrations, turbidity, TN and TP, and was again interpreted as the main eutrophication axes. The second axis (9.5% of total variability explained) correlated with DIN and DIP concentrations. A general scheme was inferred for the changes in macrophyte assemblages during the oligotrophication process. Hence, when placing hypertrophic and oligotrophic conditions end to end, the oligotrophication trajectories were described by a sequence of three ecosystem states dominated by (i) phytoplankton with bare non-vegetated sediments, (ii) opportunistic macroalgae, (iii) seagrass and perennial macroalgae, punctuated by regime shifts between these ecosystem states. The regime shift from the phytoplankton-dominated state to opportunistic macroalgae was observed in Méjean lagoon, where Ulva rigida C. Agardh, 1823, dominated in 2009 after a strong decrease in Chl a (see above), followed by more diverse communities since 2012 with among others different Gracilaria spp. and Chaetomorpha aerea (Dillwyn) Kützing, 1849. However, during the 10-year period following the reduction of the nutrient loading, Méjean lagoon did not achieve the oligotrophic state dominated by seagrass and perennial macroalgae (Le Fur et al., 2019).
Interestingly, for primary producers, the oligotrophication trajectories in poly- and euhaline lagoons appear as the inverse of the eutrophication trajectories (cf. above, Schramm, 1999), with the exception of the transitional state occurring during eutrophication of angiosperms perishing under a high coverage of epiphytes. Again, during oligotrophication, opportunistic macroalgae, including Ulva intestinalis Linnaeus, 1753, U. rigida, C. aerea, and Gracilaria spp., occupy an intermediate domain between the two end members, i.e., ecosystem states dominated by (i) phytoplankton and (ii) seagrass and perennial macroalgae, at the hypertrophic and oligotrophic extremes, respectively. We have assumed that these three ecosystem states are the result of attraction basins reinforced by positive and negative feedback loops. Hence, these three attraction basins and the oligotrophication trajectories have been plotted graphically (Figure 6) and compared with the STATICO factor map (Le Fur et al., 2019). Despite representing the hypertrophic state, the attraction basin for phytoplankton-dominated systems is projected at low DIN and DIP levels, related to the high affinity uptake capacities of phytoplankton.
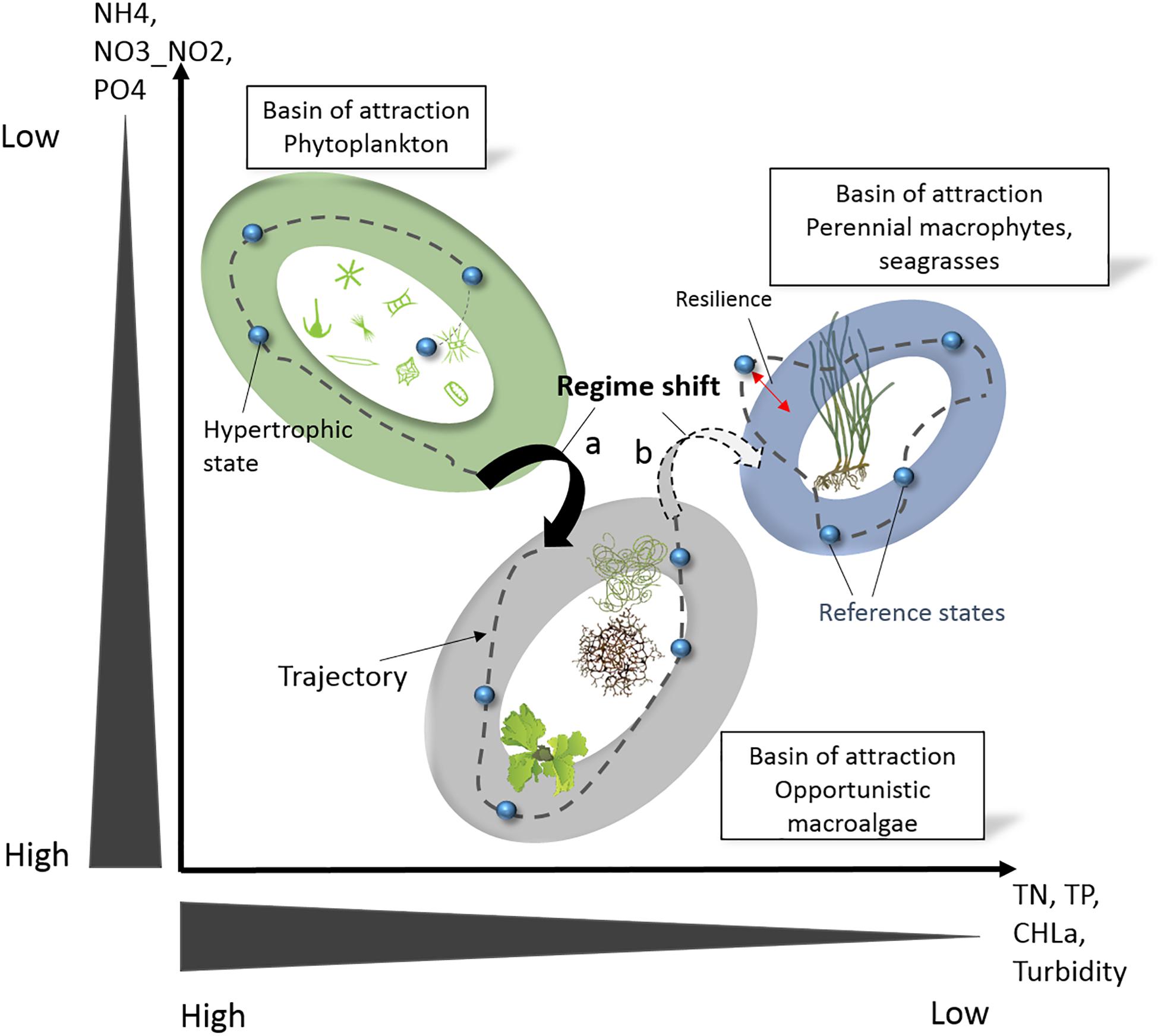
Figure 6. Illustration of ecosystem states and regime shifts along oligotrophication trajectories in Mediterranean coastal lagoons. The ellipses represent the three basins of attraction that were identified, i.e., three ecosystem states dominated by (i) phytoplankton with bare non-vegetated sediments, (ii) opportunistic macroalgae, and (iii) seagrass and perennial macroalgae. The small blue spheres represent the different ecosystem states and the stippled lines their trajectory according their chronology. Small pictograms courtesy of the Integration and Application Network, University of Maryland Center for Environmental Science (ian.umces.edu/symbols/). Creative Commons license, reproduced from Le Fur et al. (2019).
Sediment N and P
Figure 7 shows that the sediments of the oligotrophic lagoons La Palme and Ayrolle are characterized by low TP and low TN contents of approximately 7 and 50–80 mmol/kg, respectively. These values are roughly four times higher in the hypertrophic Méjean lagoon. The geometric mean regression shows for the poly- and euhaline lagoons a N/P molar ratio of 19.3, which is close to the Redfield ratio of 16. This suggests that during eutrophication, the sediments become enriched in N and P, probably largely due to the accumulation of organic matter. The regression line shows that on average 5.8 mmol/kg TP is predicted for TN = 0. Hence, even in the absence of organic matter, phosphorus is expected to be present, which can be explained by the presence of inorganic mineral forms like phosphoapatites and P sorbed on iron compounds. Søndergaard et al. (2003) stated that “Internal P loading originates from a pool accumulated in the sediment at high external loading, and significant amounts of phosphorus in lake sediments may be bound to redox-sensitive iron compounds or fixed in more or less labile organic forms.” In addition, the mineralization of the organic matter in the sediment provide inorganic N and P that could sustain a flux of DIN and DIP across the sediment-water interface, which represents thus another internal source for the water column.
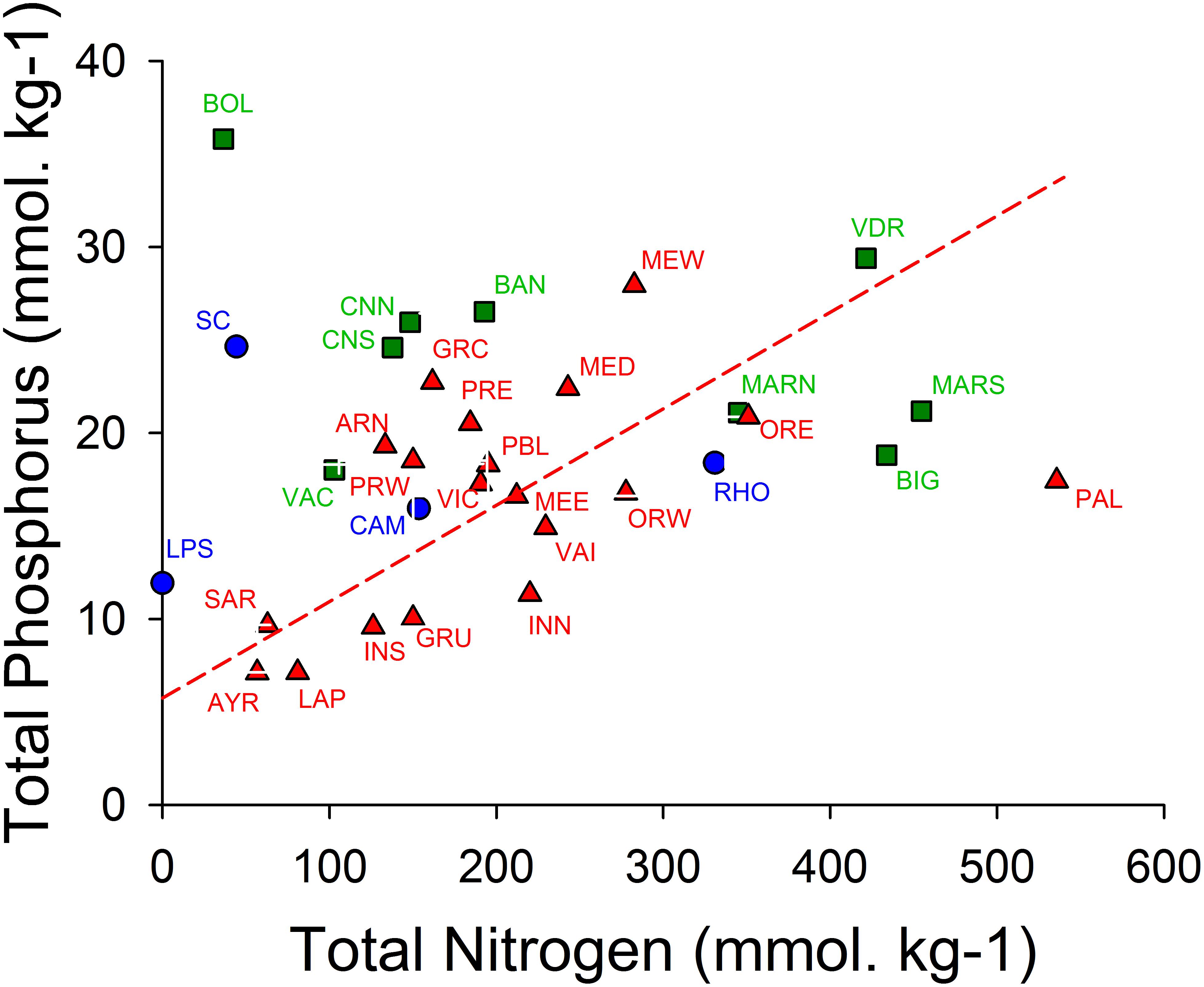
Figure 7. Graph of total nitrogen (TN) and total phosphorus (TP) contents of the top 5 cm of the sediments expressed per dry weight (DW) of sediments. Oligohaline lagoons in blue circles, mesohaline lagoons in green squares and poly- and euhaline lagoons in red triangles (cf. Figure 2). A geometric mean regression was calculated for the poly- and euhaline lagoons and depicted as a broken red line (TP = 0.0519 × TN + 5.752 (mol ⋅ kg–1), r = 0.494), corresponding to a N/P molar ratio of 19.27. Codes for sampling stations: AYR, Ayrolle; ARN, Arnel; BAN, Bagnas; PBL, Pierre Blanche; BIG, Biguglia; BOL, Bolmon; CAM, Campignol; CNN, CNS, Canet North and South stations, respectively; GRC, Grec; GRU, Gruissan; INN, Ingril Nord; INS, Ingril Sud; LAP, La Palme; LPS, La Palissade; MARN, La Marette; MED, Médard; MEE, MEW, Méjean, East and West stations, respectively; ORE, ORW, Or East and West stations, respectively; PAL, Palo; PRE, PRW, Prévost, East and West stations, respectively; RHO, Rhône de Saint Romans; SC, Scamandre; VAI, Vaïne; VDR, Vendres.
Coastal Lagoons in the Habitats Directive and Links With WFD Approaches
The scientific literature about coastal lagoons in Europe comprises numerous studies on the implications of the WFD, but to our knowledge very few studies have assessed the consequences of its co-implementation with the Habitats and Birds Directives for these ecosystems. While the WFD explicitly links the WFD with the Habitats and Birds Directives (WFD, Annex VI) in common day practice, coastal lagoon managers in the EU are systematically confronted with co-implementation of WFD and Habitats Directive, in some cases even together with the Birds Directive.
Coastal lagoons are considered in the WFD as transitional water bodies (TWB) together with river mouth ecosystems (water bodies in estuaries and deltas). For the WFD application in the French Mediterranean water basin, the coastal lagoons are considered as a subcategory within TWB, i.e., Mediterranean lagoons, which are non-tidal or nano-tidal. Moreover, the “Comité de Bassin Rhône Méditerranée” decided in 2009 to exclude ephemeral coastal lagoons as well as permanent coastal lagoons smaller than 50 ha. The Habitats Directive recognizes coastal lagoon as a priority habitat 1150, which has been defined (European Commission DG Environment, 2013) as “expanses of shallow coastal salt water, of varying salinity and water volume, wholly or partially separated from the sea by sand banks or shingle, or, less frequently, by rocks. Salinity may vary from brackish water to hypersalinity depending on rainfall, evaporation and through the addition of fresh seawater from storms, temporary flooding of the sea in winter or tidal exchange. With or without vegetation from Ruppietea maritimae, Potametea, Zosteretea, or Charetea (CORINE 91: 23.21 or 23.22).” The latter are vegetation units defined by phytosociology. Hence, the coastal lagoons listed in Figure 2 all belong to the priority habitat 1150 of the Habitats directive and have been included in Natura 2000 sites. In France, many temporal saline ponds along the Mediterranean coastline have also been included in the priority habitat 1150. In some cases, the temporal ponds are coastal lagoons according the definition of Kjerfve (1994), i.e., with a temporal inlet connecting with the sea. In such cases, during summer the inlet closes and the lagoon dries out completely. However, many of the temporal ponds that function as endorheic systems without direct connection to the sea, have also been included in habitat 1150. Nonetheless, such an approach is fully acceptable according the Interpretation Manual of European Union Habitats – EUR28 (European Commission DG Environment, 2013) as “the salt basins and salt ponds may also be considered as lagoons, providing they had their origin on a transformed natural old lagoon or on a saltmarsh, and are characterized by a minor impact from exploitation.” Many of the former salt-ponds in abandoned Salinas typically represent such a case (De Wit et al., 2019). In the PLC two Salinas have been abandoned, i.e., the Salins de Frontignan and Villeneuve, which have been included as peripheral wetlands in the Natura 2000 site of the “etangs Palavasiens” (FR9101410). In the Mediterranean climate, these temporal ponds are typically filled with water from rain and run-off after heavy rainfall in autumn and tend to dry out during late spring or the summer period. The salinity of the ephemeral water column, which originates in the endorheic systems from dissolving the salt in the soils, varies strongly during the year. The aquatic plants in these ephemeral ponds comprise species of the association of the angiosperm Althenia filiformis Petit, 1928 and the charophyte Lamprothamnium papulosum (K. Wallroth) J. Groves, 1916.
The Natura 2000 network is a network of protected areas designated according the Habitats directive [Site of Community Interest (SCI)] and the Birds Directive [Special Protection Areas (SPA)]. The member states have an obligation to report on the conservation status of habitats within Natura 2000 sites. It is a major challenge to combine the management and surveillance monitoring for the “ecological status” according the WFD with that for the “conservation status” of habitats and species according the Habitats Directive and extent these to the temporal lagoons.
Communication With Coastal Lagoon Stakeholders, Their Values, Perceptions, and Opinions
The normative approach of the WFD is based on the use of EQRs calculated for different biological quality elements (BQE, e.g., phytoplankton, macrophytes). Based on the EQR values classes are attributed using the qualifications Bad, Poor, Moderate, Good, and High, based on the deviation from the reference state corresponding to conditions of minimal anthropogenic impact. However, an aggregated qualification is attributed based on the principle “one out all out,” which means that the BQE with the lowest EQR determines the overall EQR and quality class, thus overruling all the others.
These aggregated qualifications are often restituted to the managers, policy makers and general public by using a color code. Accordingly, Figure 8 presents the color coding for the ecological status of the water column in the PLC restituted every year by the Lagoon monitoring network “RSL” (IFREMER, 2014).
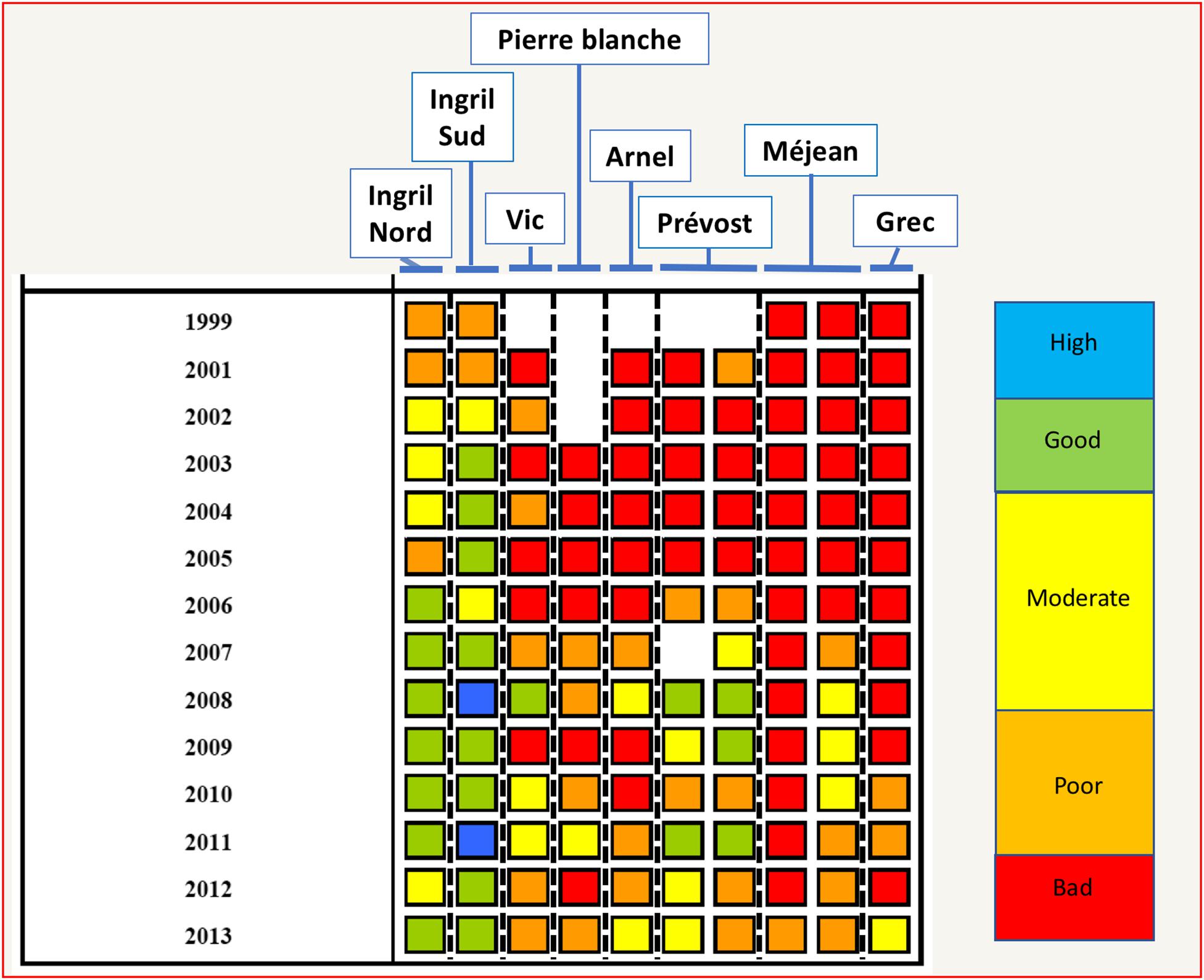
Figure 8. Color coding restitution of water quality by the Lagoon Monitoring Network for the lagoons of the PLC from 1999–2013 (Figure designed using Tableau 11.2, page 175 of IFREMER, 2014, Courtesy Ifremer).
Figure 8 shows that in general the water quality has improved from 2000 to 2013 for the lagoons of the PLC, although in 2013 only two (Ingril Nord and Ingril Sud) of eight lagoons had achieved “good” ecological status for the water column, while the rest was reported as poor or moderate. Social representation of water quality by the local populations was identified and compared to the water quality assessment carried out according to the French surveillance monitoring system (Audouit et al., 2019). More than half of the interviewees in the PLC considered water quality as moderate (40%) or good (26%). In 2013, only Ingril Nord and Ingril Sud lagoons were in a good status. Hence, social representation gave a higher score than the surveillance monitoring. Partly this difference could be attributed to the conservative scoring used in the WFD based on the principle “one out all out” (Audouit et al., 2019). Nevertheless, both the surveillance monitoring and the social perception of water quality explain a perception in society of the failure of achieving WFD goals for a large number of coastal lagoons. Interestingly, the social representation of the biodiversity of the coastal lagoons was much more positive than that of water quality, with 40% valuing as high and 40% valuing as good for the lagoons of the PLC. In general, interviewees judged that the current situation was better than in the past (Audouit et al., 2019), meaning that some positive impact of public policy and management has been perceived by the general public.
The lack of full restoration of water quality and of other aspects for qualifying as good ecosystem state (e.g., benthic macrophytes) raised the question whether the reduction of the nutrient loading should not be combined with additional eco-engineering measures (cf. Figures 3, 4, active restoration). Therefore, the hypertrophic Méjean and the mesotrophic Ingril Sud lagoons were compared. While it was considered that Ingril Sud lagoon was already on a trajectory for spontaneous recovery after the nutrient reduction (passive restoration), additional measures for active restoration were considered for Méjean lagoon. These measures comprise a first phase based on collection of macroalgae and their exportation from the lagoon followed by a second phase based on planting marine angiosperms. Based on these suggestions, four scenarios were defined for Méjean lagoon, ranging from passive to a high level of active ecological restoration and questionnaires were designed for residents and tourists and face-to-face interviews were performed. More than 85% of both residents and tourists expressed that they have a positive perception of the ecological and societal importance of coastal lagoons and of their ecological restoration. A willingness to pay for active restoration (i.e., additional costs for the eco-engineering measures on top of the 150 Million€ engaged for the improvement of the water quality, see above) was expressed by 70 and 60% of the resident and tourists’ respondents, respectively. The residents were willing to pay each about 25€ a year for the highest level of ecological restoration and willing to allocate a much smaller amount of about 5€ for the improvement of the infrastructure for visiting the site (De Wit et al., 2017).
The study of how stakeholders perceive ecosystem services of coastal lagoons and how they value them it is important to understand the benefits people obtain from these ecosystems and get insights on their expectations for the future. Hence, such an assessment of ecosystem services is very instrumental for ecological restoration projects to assess whether the project corresponds to the expectations and perceptions of the stakeholders. Monetary valuations of ecosystem services may present an obvious advantage for ecological restoration projects as it allows a straightforward cost-benefit analysis (De Groot et al., 2013; De Wit et al., 2017). Nevertheless, monetary approaches can be problematic when working with stakeholders as most of the ecosystem services provided by the coastal lagoons areas are non-market services and, stakeholders in general, tend to reject the monetary approaches. Therefore, we turned to non-monetary approaches and used the Q-method (Sy et al., 2018), which is based on a serious card game that allows to capture levels of consensus and divergence among participants. Thirty-one ecosystem services provided by the lagoons were selected by a focus group (see Table 2) and highly involved stakeholders were asked to rank these ecosystem services. The highly involved stakeholders were characterized by their strong involvement in following the management process and many of them were members of the Natura 2000 committee. They were grouped in seven categories, i.e., local government, private sector, NGOs, scientists, public and para-public sectors, managers, and local residents. Statistical analysis was improved by bootstrapping in order to obtain additional and more detailed measures of variability and thus help to better understand the data and the outcomes. Accordingly, three groups of these stakeholders, each sharing similar point of views the group, were extracted (Sy et al., 2018). Here, we complement this study with new data obtained through citizen workshops for local citizens (see Supplementary Table S2 for their sociodemographic composition). These local citizens can thus be considered as less-involved stakeholders (e.g., García-Nieto et al., 2015).
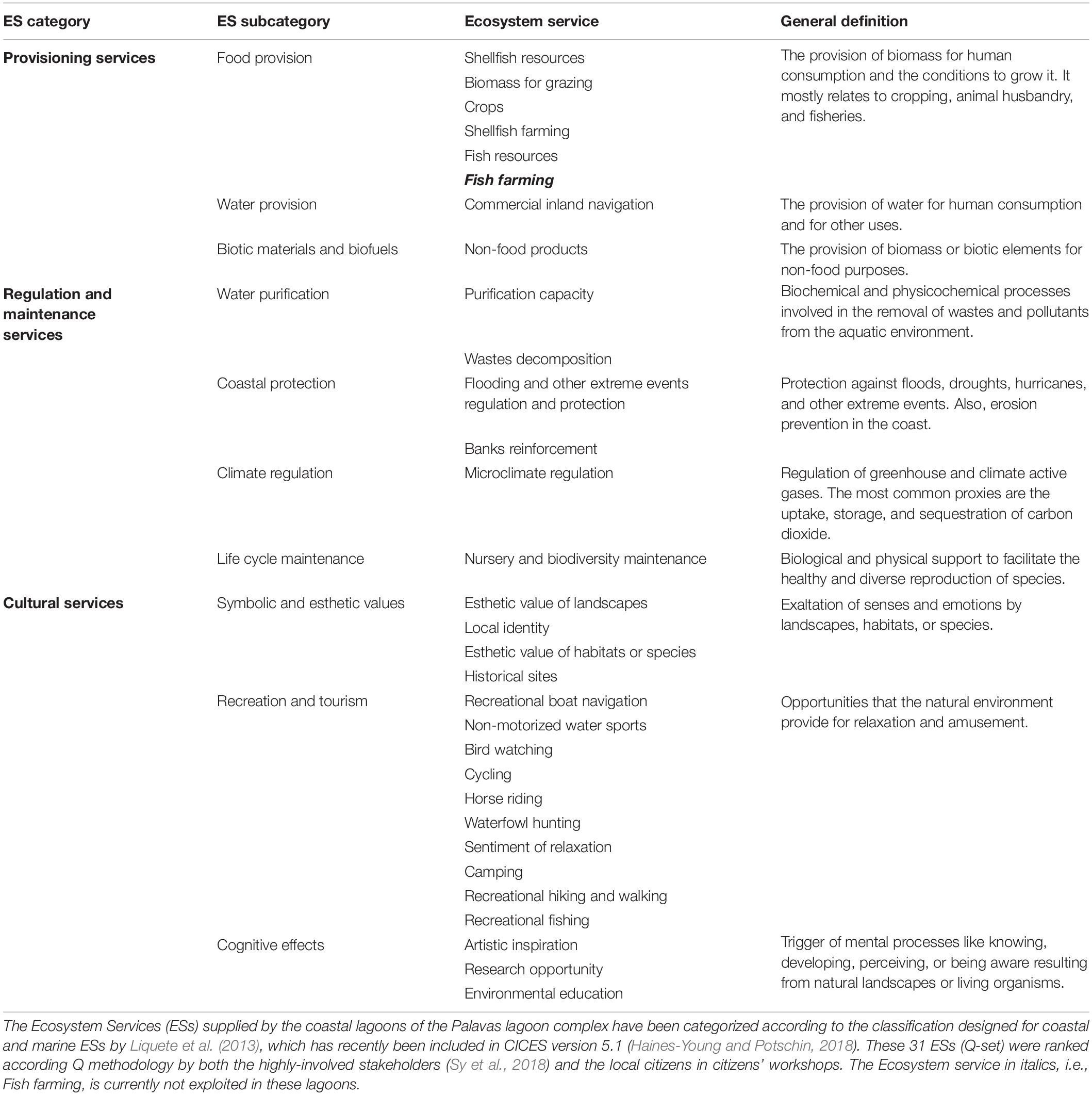
Table 2. Modified from Sy et al. (2018).
Forty-three citizens actively participated in these citizen workshops and completed the Q-method ranking of the same 31 ecosystem services (Table 2), ranked earlier by the highly involved stakeholders (Sy et al., 2018). The analyses again extracted three different groups which are listed in Table 3 with their most salient results (more detail in Supplementary Figures S1, S2). All three groups attributed high level of priority to regulation and maintenance services. Consensus among these citizens was particularly striking for providing protection against flooding and other extreme natural events. Biodiversity and nursery, water purification capacity and microclimate regulation were among the top of priorities for two or one of the groups, but the overlapping variability of the bootstrapped estimates indicated still a certain degree of consensus of their importance. There was also consensus on the most unimportant side, which was most likely based on mere rejection, i.e., for camping. Two of the three groups also seemed to reject waterfowl hunting. Group #1, which gave an absolute priority to regulation and maintenance services, was quite similar to the preferences of group #2 of the highly involved stakeholders of the PLC, characterized as “environmental and territorial approach” (Sy et al., 2018). Group #2 had a clearly naturalist profile, giving among the regulating services the highest priority to biodiversity and nursery services with strong preferences for cultural services as bird watching and esthetic value of species and habitats. This result was rather similar to group #1’s preferences (“environmental and hedonic vision” identified for the highly involved stakeholders (Sy et al., 2018). The hedonist aspect is also reflected by the importance group #2 attached to the sentiment of relaxation. A very small group #3 comprising only four persons, among which three from the eastern part of the PLC, was atypical, because it valued two provisioning services very high, i.e., (i) shellfish farming, developed in the Prévost lagoon and (ii) commercial inland navigation, related to traffic on the Rhône-to-Sète canal running through the lagoon complex. This group was hardly interested in naturalistic aspects, although it valued the esthetic value of the landscape very high. Perhaps this is related to a sensitivity for heritage aspects.
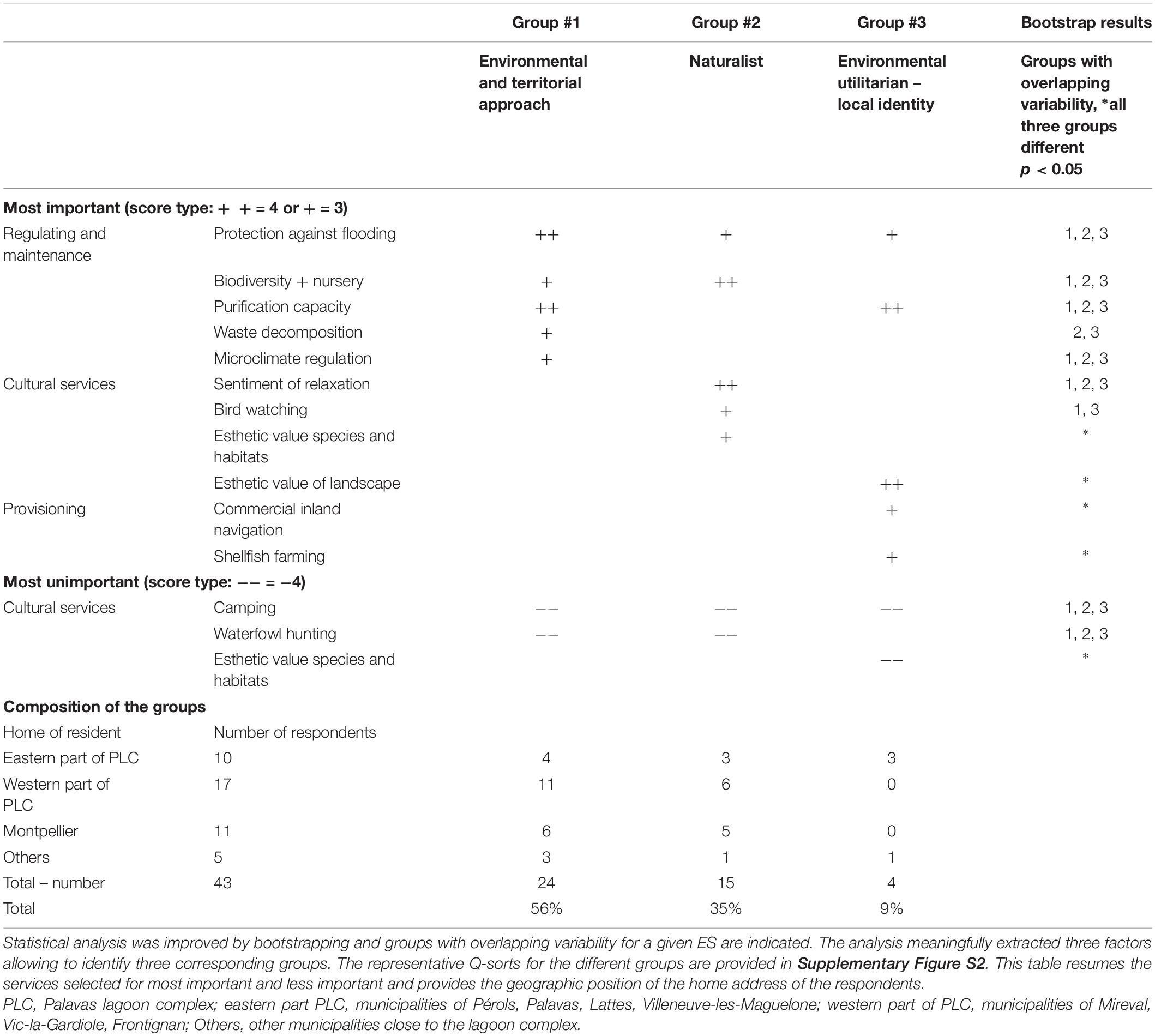
Table 3. Most salient results from analyzing the Q-sorts of the 43 participants in citizen workshops.
General Discussion and Synthesis
Restoration ecology of coastal lagoons should integrate solid knowledge of the biodiversity of the communities and ecosystem functioning. But, as it studies a human activity, i.e., ecological restoration, we argue that it should also take into account the pertinent aspects of legislation, as well as socio-economic aspects concerning perceptions by local stakeholders and their expectations for the future desired state of the ecosystems. Hence, restoration ecology, in addition to sound ecological knowledge, thus has to establish multidisciplinary collaborations with social sciences. In this study, we analyzed by such an integrated multidisciplinary approach, the practice of ecological restoration to combat eutrophication, which has been achieved by reducing the external nutrient loading to shallow Mediterranean coastal lagoons in South France. This action has resulted in oligotrophication of formerly eutrophied coastal lagoons.
When the reduction of nutrient loadings to shallow coastal lagoons was initiated in the early 2000s through the WFD, little scientific knowledge was available for predicting the ecosystem trajectories of shallow coastal lagoons during oligotrophication and ideas were inspired by the experience obtained for the oligotrophication of shallow freshwater lakes that had been initiated two decades earlier. The experience in South France was followed by intensive surveillance monitoring (RSL) and scientific studies (cf. Table 1) focused on improving the understanding of the eutrophication (Souchu et al., 2010, Bec et al., 2011; Le Fur et al., 2018) and of oligotrophication trajectories (Leruste et al., 2016; Pasqualini et al., 2017; Derolez et al., 2019; Le Fur et al., 2019). From this we have obtained a large body of understanding and empirical knowledge to guide the ecological restoration practice. We hereby summarize the main findings. Coastal lagoons show similar attractors at both extremes along the eutrophication gradient as described for freshwater lakes (cf. Scheffer, 2001). At the oligotrophic side, shallow poly- and euhaline lagoons show transparent water and are home to meadows of the marine angiosperms, Z. noltei, Ruppia spp. and C. nodosa together with some slow-growing perennial macroalgae as e.g., A. acetabulum (Le Fur et al., 2018). At the hypertrophic extreme, shallow poly- and euhaline lagoons show turbid water with dense phytoplankton (Bec et al., 2011; Leruste et al., 2016). However, in contrast to freshwater lakes, massive blooming of opportunistic macroalgae represents a third ecosystem state in shallow coastal lagoons (Valiela et al., 1997; De Wit et al., 2001; Viaroli et al., 2008; Le Fur et al., 2018, 2019) in between both extremes. The importance of this third attractor, implies that the attractive bistability scheme proposed by Scheffer for freshwater lakes does not apply straightforwardly for coastal lagoons. Nevertheless, for the poly- and euhaline lagoons, oligotrophication trajectories from hypertrophy to oligotrophy have now been described as a sequence from (i) phytoplankton with bare non-vegetated sediments, (ii) opportunistic macroalgae, and (iii) seagrass and perennial macroalgae, punctuated by regime shifts between the ecosystem states (Le Fur et al., 2019). At present, in these systems, it remains unclear whether domains of multiple stable states occur for the eutrophication – oligotrophication pathways or whether all the regime-shifts represent steep but continuous shifts in equilibrium states. The shift from the phytoplankton-dominated state to the macroalgal-dominated state occurs very quickly upon reduction of the external nutrient loading, i.e., within one or a couple of years (Le Fur et al., 2019), and it has been argued that water-column showed little hysteresis (Derolez et al., 2019). In contrast, ten years of oligotrophication of the hypertrophic lagoons did not yet result in the second regime shift from a macroalgal-dominated state into the SAV state (Le Fur et al., 2019), suggesting strong hysteresis for this final part of the trajectory. Such hysteresis may in part be explained by a time lag to re-establish the positive feedbacks (Maxwell et al., 2017) and mutualistic networks (Van der Geest et al., 2020) in the seagrass systems. Another source of hysteresis during oligotrophication may be due to time lags caused by internal loading from the biogeochemical sinks for nutrients accumulated during the eutrophication phase. Indeed, the poly- and euhaline lagoons showed concomitant increase of N and P with increasing eutrophication (see Figure 7) most likely related to the increase of the organic matter content (average N/P molar ratio of 19.3, which is close to the Redfield ratio). Benthic fluxes of N and P need to be measured (Ouisse et al., 2013) to provide more information on the role of internal loading in delaying oligotrophication. Inertia caused by internal loadings have also been described for the oligotrophication of shallow freshwater lakes, although the focus has been on P alone (Jeppesen et al., 2002), while for the lagoons we show that both N and P play a role.
We can conclude that scientific knowledge and empirical observations are nowadays operational for providing guidance for ecological restoration of the poly- and euhaline Mediterranean coastal lagoons. Nevertheless, insufficient knowledge is still available for the oligo- and mesohaline lagoons. The specific behavior of the aquatic angiosperms forming floating leaves (S. pectinata in oligohaline and Ruppia sp. in mesohaline lagoons) has to be taken into account. Another source of problem is the fact that for hypertrophic poly- and euhaline lagoons it is still not possible to predict whether a complete oligotrophication trajectory will occur and how long it may take. Many of these formerly hypertrophic lagoons appear to remain stuck in the macroalgal stage, even after 10 years of oligotrophication. This raises the question whether in such cases, the ecological restoration should become an active approach by combining the nutrient reduction with the active planting and seeding of the angiosperms (Orth et al., 2012; De Wit et al., 2017). Currently, such an approach is undertaken in Venice lagoon (Sfriso et al., 2019).
Ecological restoration is a human activity that is clearly identified as a Response in the DPSIR framework (see Figure 3). The link between DPSIR and ecological restoration has been invoked in studies focusing on the ecological restoration of rivers (Song and Frostell, 2012; Lalande et al., 2014) although their focus has been more upstream underscoring the importance of identifying drivers and pressures for designing ecological restoration rather than analyzing what is actually targeted by the different practices of ecological restoration. Collectively, these studies together with our study highlight the paramount importance of clearly identifying the pressures on aquatic ecosystems and that ecological restoration should target a reduction of these pressures in the first place. Hence improved sanitation and appropriate management of non-point sources of nutrient in watersheds is paramount for the ecological restoration of eutrophied lagoons. Ecological restoration measures only targeting the state of the ecosystems are not efficient without acting on the pressures.
Surveillance monitoring is a key aspect to follow the state of the ecosystem (S in the DPSIR). The principle “one out all out” was designed for surveillance monitoring of the ecological status of aquatic ecosystems in the WFD, particularly to check if a system is and remains in better than moderate, i.e., good or high conditions. The application of this principle is probably a reason why (i) the perception of local populations is more optimistic than that of WFD, (ii) the assessment systems have been judged by 8% of the interviewed managers as “overly strict to define success” (Carvalho et al., 2019), and (iii) it appears as particularly problematic for assessing the impact of specific actions as ecological restoration. For example, it does not identify correctly if variables move in the right direction (the aggregated score only moves until the worst valued has also moved in the positive direction). Thus, the surveillance monitoring that has been used in the frame of the WFD is very conservative and does not give a sufficient indication of minor to major advances in ecological status. Hence, it is important to introduce specific action monitoring for following the impact of ecological restoration that should be designed to detect more responsively (i) whether the ecosystem state moves in the right direction, (ii) identify possible transient states, and (iii) assess whether the different targets for the ecological restoration project have been achieved.
For the conservation and management of coastal lagoons, there is a clear need to study the juxtaposition particularly of the WFD and Habitats Directives, as well as the Birds Directive in some cases. Hence, complementarities and possible incongruences between these Directives should be identified and solutions proposed. For protected areas, the WFD approach has clear advantages with respect to more conventional nature conservation approaches, because it adopts the concept of the aquatic continuum, underscores importance of land-use in the watershed for ecological processes in the lagoon, explicitly considers chemical pollutants and provides a framework that accommodates the concepts of restoration ecology. This approach works well for addressing water quality and the aquatic biota. However, the WFD does not consider the terrestrial habitats as the coastal barrier with beaches and dune systems, the salt marshes and wetlands in its immediate surroundings, as part of the lagoon ecosystem. Moreover, in addition to nutrient reduction targeting oligotrophication, ecological restoration in coastal lagoons includes an array of different actions, e.g., including restoring the freshwater-salt water ecoclines and ecotones through hydrological measures (Yáñez-Arancibia et al., 2013; Feola et al., 2018), restoration of dune vegetation on the coastal barrier (Buisson et al., 2014) and the restoration of meadows of submerged marine angiosperms (Sfriso et al., 2019). In these cases, the Natura 2000 network offers workable sites comprising different habitats to pursue such management actions and the Life program a funding mechanism for ecological restoration demonstration projects. Hence, the ecological restoration projects that are being developed in coastal lagoon territories have to adapt to the Habitats Directive and for the aquatic compartment also to the WFD. This may represent a challenge as in the Habitats Directive, the assessment of the conservation status of priority habitats is very much rooted in phytosociological approaches, and the possibilities offered by more modern ecological approaches like restoration ecology need to be included more explicitly. While, the WFD promotes a highly integrated vision and is explicitly linked to the Habitats Directive, the differences of approaches and implementation by different public bodies in member states can result in rather sectorial approaches. Hence, WFD is not fully congruent with the Habitats Directive as a consequence of different objectives and concepts. Both have their advantages and inconveniences and an intelligent application of both should be based on integrative non-sectorial application of national legislation and pragmatic management. The active restoration of angiosperm meadows and ecological restoration of aquatic connectivity are examples where co-implementation of both Directives is particularly beneficial. We also recommend the integration of some of the valuable concepts of the WFD into the conservation management of temporal and smaller (i.e., in France <50 ha) lagoons requested by the Habitats Directive.
Knowledge of social representation of the ecosystem and perceptions of management actions are of paramount importance for managers engaged in ecological restoration projects. In fact, public understanding and appreciation lead to more legitimate and accepted public policies such as coastal lagoon ecological restoration projects. In contrast, a low level of congruency will imply a high risk of conflict for implementing such policies. Nevertheless, the results of our studies are encouraging as the ecological restoration practice is highly valued by human populations and stakeholders. Stakeholders have a positive perception of the ecological and societal importance of coastal lagoons and of the need of their ecological restoration, i.e., more than 85% of both residents and tourists close to Montpellier expressed this point of view (De Wit et al., 2017). However, the social representation of the lagoons is more focused on biodiversity aspects than on water quality sensu stricto (Audouit et al., 2019). There is consensus among both the highly involved stakeholders (Sy et al., 2018) and among the local residents, who participated in our citizen workshops, on giving highest importance to the regulation and maintenance ecosystem services. While primarily characterized by the consensus on the role of regulating services, stakeholder profile groups’ preferences mainly varied based on cultural services and more rarely on provisioning services. Many stakeholders have a sort of collective appreciation of the importance of these regulation and maintenance services for the local surrounding of the lagoons (territorial approach). Other enjoyments as a personal experience provided by contemplating nature, are also important wishes as e.g., the coastal lagoons should contribute to the sentiment of relaxation, the esthetics of species and landscapes, and possibilities for birdwatching. Some groups showing a more naturalist type of profile (citizens) compared to others with a more environmentalist profile (highly involved stakeholders). These studies allow to conclude that coastal lagoons ecosystem services are very important for the stakeholders and that public policies aiming at the ecological restoration of these fragile ecosystems may count on public support.
Nomenclature
Taxonomic nomenclature followed AlgaeBase (Guiry and Guiry, 2016) and World Register of Marine Species (WoRMS Editorial Board, 2016).
Data Availability Statement
This article was based on review of the ecological publications listed in Table 1 and social science data (publications cited in the text). The ecological monitoring data are available in the French data bases “Banque Hydro” (http://www.hydro.eaufrance.fr/), Naïade (http://www.naiades.eaufrance.fr/acces-donnees), and “Surval,” which among others hosts the data of the Réseau Suivi Lagunaire (RSL) monitoring program (Ifremer, https://wwz.ifremer.fr/surval/). Data of the citizens’ workshop are available in Supplementary Material.
Ethics Statement
The citizen workshop was based on voluntary participation and the data have been treated anonymously in compliance with the EU General Data Protection Regulation (GDPR).
Author Contributions
AL, IL, MS, BB, VO, VD, and RD contributed to the field and laboratory work, and ecological data compilation and interpretation. VD hold responsibilities for the Lagoon Monitoring Network “RSL,” and Water Framework Directive (WFD) monitoring programs. MS, HR-V, and RD organized the citizen workshops. MS analyzed the data. BB, VO, HR-V, and RD contributed to supervising the Ph.D. thesis of AL, IL, and MS. RD wrote this publication with the help of all co-authors.
Funding
Ecological data used in this article have been collected through (1) the Lagoon Monitoring Network (RSL), financed by Région LR, AERMC, Ifremer, (2) the Water Framework Directive (WFD) financed by AERMC and Ifremer, (3) the DRIIHM LabEx (ANR-11-LABX-0010_DRIIHM), “Device for Interdisciplinary Research on human-environments Interactions” within the framework of the Human-environment observatory “Mediterranean coastline,” (4) Ph.D. studies at the University of Montpellier, and (5) additional studies (PNEC, INTERREG II/PNOC, and RLC programs). The DRIIHM LabEx supported the studies with the citizens’ workshops. The Laboratory for Excellence project (DRIIHM LabEx) was supported by the program “vestment in the future” (ANR). AL, IL, and MS were supported by Ph.D. fellowships by the Doctoral School in Montpellier, Ifremer and AERMC, and the DRIIHM Labex, respectively.
Conflict of Interest
The authors declare that the research was conducted in the absence of any commercial or financial relationships that could be construed as a potential conflict of interest.
Acknowledgments
Philippe Souchu, Thierry Laugier, Nathalie Malet, Annie Fiandrino, Dominique Munaron, Jocelyne Oheix, Martine Fortuné, Grégoire Messiaen, Elise Hatey, and Michel Lauret are thanked for their major contributions to the Lagoon Monitoring Network “RSL.” The staff of the Ifremer LER laboratories (LER “Languedoc Roussillon” and LER “Provence Azur Corse”) are thanked for their contributions to field work and laboratory analysis. The Cépralmar provided logistic help for the RSL. Many coastal lagoon managers in South France are thanked for their interest and contribution to discussions. We thank the reviewers for their constructive comments and suggestions.
Supplementary Material
The Supplementary Material for this article can be found online at: https://www.frontiersin.org/articles/10.3389/fevo.2020.00108/full#supplementary-material
Footnotes
- ^ http://www.hydro.eaufrance.fr/
- ^ http://www.naiades.eaufrance.fr/acces-donnees
- ^ https://wwz.ifremer.fr/surval/
References
Audouit, Ch, Pasqualini, V., De Wit, R., Flanquart, H., Deboudt, Ph, and Rufin-Soler, C. (2019). Comparing social representation of water quality in coastal lagoons with normative use of ecological indicators. Mar. Polic. 101, 137–146. doi: 10.1016/j.marpol.2017.08.023
Bec, B., Collos, Y., Souchu, P., Vaquer, A., Lautier, J., Fiandrino, A., et al. (2011). Distribution of picophytoplankton and nanophytoplankton along an anthropogenic eutrophication gradient in French Mediterranean coastal lagoons. Aqua. Microb. Ecol. 63, 29–45. doi: 10.3354/ame01480
Beisner, B. E., Haydon, D. T., and Cuddington, K. (2003). Alternative stable states in ecology. Front. Ecol. Environ. 1:376–382.
Buisson, E., Bravet, P., Mieusset, J., Baillot, N., Dubreucq, C., Sadonès, L., et al. (2014). Plant communities of a coastal lagoon foredune: definition of the reference and restoration after compaction. Acta Bot. Gall. 161, 277–286. doi: 10.1080/12538078.2014.919875
Carvalho, L., Mackay, E. B., Cardoso, A. C., Baattrup-Pedersen, A., Birk, S., Blackstock, K. L., et al. (2019). Protecting and restoring Europe’s waters: an analysis of the future development needs of the water framework directive. Sci. Total Environ. 658, 1228–1238. doi: 10.1016/j.scitotenv.2018.12.255
Clewell, A. F., and Aronson, J. (2013). Ecological Restoration, Principles, Values, and Structure of an Emerging Profession. 2nd Edn. Washington, DC: Island Press.
Covelli, S. (2012). The MIRACLE Project: an integrated approach to understanding biogeochemical cycling of mercury and its relationship with lagoon clam farming. Estuar. Coast. Shelf Sci. 113, 1–6. doi: 10.1016/j.ecss.2012.08.011
Cunha, A. H., Marbá, N., Van Katwijk, M., Pickerell, C., Henriques, M., Bernard, G., et al. (2012). Changing paradigms in seagrass restoration. Restor. Ecol. 20, 427–430. doi: 10.1111/j.1526-100x.2012.00878.x
De Groot, R., Blignaut, J., Van der Ploeg, S., Aronson, J., Elmqvist, T., and Farley, J. (2013). Benefits of investing in ecosystem restoration. Conserv. Biol. 27, 1286–1293. doi: 10.111/cobi.12158
De Wit, R. (2011). “Biodiversity of coastal lagoon ecosystems and their vulnerability to global change,” in Ecosystems Biodiversity, eds O. Grillo and G. Venore (London: InTech Open Access Publisher), 29–40.
De Wit, R., Rey-Valette, H., Balavoine, J., Ouisse, V., and Lifran, R. (2017). Ecological restoration of coastal lagoons; prediction of ecological trajectories and economic valuation. Aqua. Conserv. 27, 137–157. doi: 10.1002/aqc.2601
De Wit, R., Stal, L. J., Lomstein, B. A., Herbert, R. A., Van Gemerden, H., Viaroli, P., et al. (2001). ROBUST: The ROle of BUffering capacities in STabilising coastal lagoon ecosystems. Cont. Shelf Res. 21, 2021–2041.
De Wit, R., Vincent, A., Foulc, L., Klesczewski, M., Scher, O., Loste, C., et al. (2019). Seventy-year chronology of Salinas in southern France: coastal surfaces managed for salt production and conservation issues for abandoned sites. J. Nat. Conserv. 49, 95–107. doi: 10.1016/j.jnc.2019.03.003
Derolez, V., Bec, B., Munaron, D., Fiandrino, A., Pete, R., Simier, M., et al. (2019). Recovery trajectories following the reduction of urban nutrient inputs along the eutrophication gradient in French Mediterranean lagoons. Ocean Coast. Manag. 171, 1–10. doi: 10.1016/j.ocecoaman.2019.01.012
Dolbeth, M., Stålnacke, P., Alves, F. L., Sousa, L. P., Gooch, G. D., Khokhlov, V., et al. (2016). An integrated Pan-European perspective on coastal Lagoons management through a mosaic-DPSIR approach. Sci. Rep. 6:19400. doi: 10.1038/srep19400
EU Birds Directive (2009). Directive 2009/147/EC of the European Parliament and of the Council on the conservation of wild birds (the first version of this Directive was adopted in 1979 as Directive 79/409/EEC that was amended in 2009). Brussels: EU.
EU Habitats Directive (1992). Directive 92/43/EEC of the European Parliament and of the Council on the Conservation of Natural Habitats and of Wild Fauna and Flora. Available online at: http://ec.europa.eu/environment/nature/legislation/habitatsdirective/index_en.htm (accessed February 26, 2020).
EU Water Framework Directive (2000). Directive 2000/60/EC of the European Parliament and of the Council Establishing a Framework for the Community Action in the Field of Water Policy. Available online at: http://ec.europa.eu/environment/water/water-framework/ (accessed February 26, 2020).
European Commission DG Environment (2013). The Interpretation Manual of European Union Habitats - EUR28. Available online at: http://ec.europa.eu/environment/nature/legislation/habitatsdirective/docs/Int_Manual_EU28.pdf (accessed February 26, 2020).
European Environment Agency (1999). Technical Report No 25, Environmental Indicators: Typology and Overview. Copenhague: European Environment Agency.
Feola, A., Bonometto, A., Ponis, E., Cacciatore, F., Oselladore, F., Matticchio, B., et al. (2018). LIFE LAGOON REFRESH. Ecological restoration in Venice Lagoon (Italy): Concrete Actions Supported by Numerical Modeling and Stakeholder Involvement. Available online at: http://www.lifelagoonrefresh.eu/file/pubblicazioni/COWM_2018_Extendedabstract.pdf (accessed February 26, 2020).
García-Nieto, A. P., Quintas-Soriano, C., García-Llorente, M., Paloma, I., Montes, C., and Martín-López, B. (2015). Collaborative mapping of ecosystem services : the role of stakeholders’ profiles. Ecosyst. Serv. 13, 141–152. doi: 10.1016/j.ecoser.2014.11.006
Guiry, M. D., and Guiry, G. M. (2016). AlgaeBase. World-wide electronic publication. Galway: National University of Ireland.
Gulati, R. D., and Van Donk, E. (2002). Lakes in the Netherlands, their origin, eutrophication and restoration: state-of-the-art review. Hydrobiologia 478, 73–106. doi: 10.1007/978-94-017-1335-1_5
Haines-Young, R., and Potschin, M. B. (2018). Common International Classification of Ecosystem Services (CICES) V5.1 and Guidance on the Application of the Revised Structure. Brussel: European Environment Agency.
IFREMER (2014). Réseau de Suivi Lagunaire du Languedoc-Roussillon. Bilan des résultats 2013. Issy-les-Moulineaux: IFREMER.
Jeppesen, E., Jensen, J. P., and Søndergaard, M. (2002). Response of phytoplankton, zooplankton, and fish to re-oligotrophication: an 11 year study of 23 Danish lakes 31-43. Ecosyst. Health Manag. 5, 31–43. doi: 10.1080/14634980260199945
Jeppesen, E., Søndergaard, M., Jensen, J. P., Havens, K. E., Anneville, O., Carvalho, L., et al. (2005). Lake responses to reduced nutrient loading—an analysis of contemporary long-term data from 35 case studies. Freshw. Biol. 50, 1747–1771. doi: 10.1111/j.1365-2427.2005.01415.x
Kjerfve, B. (1994). “Coastal lagoons,” in Coastal Lagoon Processes, ed. B. Kjerfve (Amsterdam: Elsevier Oceanographic Series), 1–8. doi: 10.1016/s0422-9894(08)70006-0
Lalande, N., Cernesson, F., Decherf, A., and Tournoud, M.-G. (2014). Implementing the DPSIR framework to link water quality of rivers to land use: methodological issues and preliminary field test. Int. J. River Basin Manag. 12, 201–217.
Larue, J. P., and Rouquet, J. (2016). La lagune de La Palme (Aude, France) face au comblement et à l’eutrophisation. Physiol. Géol. 10, 45–60. doi: 10.4000/physio-geo.4761
Le Fur, I. (2018). Rôle Des Macrophytes Dans la Restauration Des Milieux Lagunaires : Successions Écologiques. Montpellier: Université de Montpellier, 210.
Le Fur, I., De Wit, R., Plus, M., Oheix, J., Derolez, V., Simier, M., et al. (2019). Re-oligotrophication trajectories of macrophyte assemblages in Mediterranean coastal lagoons based on 17-year time-series. Mar. Ecol. Progr. Ser. 608, 13–32. doi: 10.3354/meps12814
Le Fur, I., De Wit, R., Plus, M., Oheix, J., Simier, M., and Ouisse, V. (2018). Submerged benthic macrophytes in Mediterranean lagoons: distribution patterns in relation to water chemistry and depth. Hydrobiologia 808, 175–200. doi: 10.1007/s10750-017-3421-y
Leruste, A. (2016). Trajectoires D’évolution des Communautés Phytoplanctoniques au Cours du Processus de Restauration Écologique des Milieux Lagunaires Méditerranéens. Montpellier: Université de Montpellier, 314.
Leruste, A., Guilhaumon, F., De Wit, R., Malet, N., Collos, Y., and Bec, B. (2019a). Phytoplankton strategies to exploit nutrients in coastal lagoons with different eutrophication status and involved in a re-oligotrophication process. Aqua. Microb. Ecol. 83, 131–146. doi: 10.3354/ame01906
Leruste, A., Pasqualini, V., Garrido, M., Malet, N., De Wit, R., and Bec, B. (2019b). Physiological and behavioral responses of phytoplankton communities to nutrient availability in a disturbed Mediterranean coastal lagoon. Estuar. Coast. Shelf Sci. 219, 176–188. doi: 10.1016/j.ecss.2019.02.014
Leruste, A., Malet, N., Munaron, D., Derolez, V., Hatey, E., Collos, Y., et al. (2016). First steps of ecological restoration in Mediterranean lagoons: shifts in phytoplankton communities. Estuar. Coast. Shelf Sci. 180, 190–203. doi: 10.1016/j.ecss.2016.06.029
Li, W. K. W. (1995). Composition of ultraphytoplankton in the central North Atlantic. Mar. Ecol. Progr. Ser. 122, 1–8. doi: 10.3354/meps122001
Life programme (2020). Available online at: https://ec.europa.eu/easme/en/life (accessed February 28, 2020).
Liquete, C., Piroddi, C., Drakou, E. G., Gurney, L., Katsanevakis, S., Charef, A., et al. (2013). Current status and future prospects for the assessment of marine and coastal ecosystem services: a systematic review. PLoS One 8:e67737. doi: 10.1371/journal.pone.0067737
Marsden, M. (1989). Lake restoration by reducing external phosphorus loading: the influence of sediment phosphorus release. Freshw. Biol. 21, 139–162.
Maxwell, P. S., Eklof, J. S., Van Katwijk, M. M., O’brien, K. R., De La Torre-Castro, M., Bostrom, C., et al. (2017). The fundamental role of ecological feedback mechanisms for the adaptive management of seagrass ecosystems - a review. Biol. Rev. 92, 1521–1538. doi: 10.1111/brv.12294
Meinesz, C., Derolez, V., and Bouchoucha, M. (2013). Base de Données “Pressions Sur Les Lagunes Méditérranéennes” - Analyse des liens état - pression. Report in French. Montpellier: Agence de l’Eau Rhône-Méditeranée- Corse.
Munaron, D., Tapie, N., Budzinski, H., Andral, B., and Gonzalez, J. L. (2012). Pharmaceuticals, alkylphenols and pesticides in Mediterranean coastal waters: results from a pilot survey using passive samplers. Estuar. Coast. Shelf Sci. 114, 82–92. doi: 10.1016/j.ecss.2011.09.009
Newton, A., Brito, A. C., Icely, J. D., Derolez, V., Clara, I., Angus, S., et al. (2018). Assessing, quantifying and valuing the ecosystem services of coastal lagoons. J. Nat. Conserv. 44, 50–65. doi: 10.1016/j.jnc.2018.02.009
Nixon, S. W. (2009). Eutrophication and the macroscope. Hydrobiologia 629, 5–19. doi: 10.1007/978-90-481-3385-7_2
Orth, R. J., McGlathery, K. J., and Heck, K. L. Jr. (eds) (2012). Theme section: eelgrass recovery induces state changes in a coastal bay system. Mar. Ecol. Progr. Ser. 434, 171–301.
Ouisse, V., Fiandrino, A., De Wit, R., and Malet, N. (2013). Restauration des écosystèmes lagunaires : évaluation du rôle du sédiment et des herbiers à phanérogames. Rapport Final du Contrat n 2012 1835. Report in French. France: Agence de l’Eau Rhône-Méditeranée-Corse.
Pasqualini, V., Derolez, V., Garrido, M., Orsoni, V., Baldi, Y., Etourneau, S., et al. (2017). Spatiotemporal dynamics of submerged macrophyte status and watershed exploitation in a Mediterranean coastal lagoon: understanding critical factors in ecosystem degradation and restoration. Ecol. Eng. 102, 1–14. doi: 10.1016/j.ecoleng.2017.01.027
Petraitis, P. S., and Hoffman, C. (2010). Multiple stable states and relationship between thresholds in processes and states. Mar. Ecol. Progr. Ser. 413, 189–200. doi: 10.3354/meps08691
Rouillard, J. J., Lago, M., Roeschel, L., Abhold, K., Kafyeke, T., Klimmek, H., et al. (2017). Protecting and restoring aquatic biodiversity: is the existing EU policy framework fit for purpose? Environ. Policy Gov. 28, 114–128. doi: 10.1002/eet.1793
Scheffer, M. (2001). Alternative attractors of shallow lakes. Sci. World 1, 254–263. doi: 10.1100/tsw.2001.62
Scheffer, M., Hosper, S. H., Meijer, M.-L., Moss, B., and Jeppesen, E. (1993). Alternative equilibria in shallow lakes. Trends Ecol. Evol. 8, 275–279. doi: 10.1016/0169-5347(93)90254-m
Schramm, W. (1999). Factors influencing seaweed responses to eutrophication: some results from EU-project EUMAC. J. Appl. Phycol. 11, 69–78.
Sfriso, A., Buosi, A., Tomio, Y., Juhmani, A.-S., Facca, C., Sfriso, A. A., et al. (2019). Aquatic angiosperm transplantation: a tool for environmental management and restoring in transitional water systems. Water 11:2135. doi: 10.3390/w11102135
Society for Ecological Restoration International Science and Policy Working Group (2004). The SER International Primer on Ecological Restoration. Available online at: https://www.ctahr.hawaii.edu/littonc/PDFs/682_SERPrimer.pdf (accessed July 11, 2019).
Søndergaard, M., Jensen, J. P., and Jeppesen, E. (2003). Role of sediment and internal loading of phosphorus in shallow lakes. Hydrobiologia 50, 135–145. doi: 10.1023/b:hydr.0000008611.12704.dd
Song, X., and Frostell, B. (2012). The DPSIR framework and a pressure-oriented water quality monitoring approach to ecological river restoration. Water 4, 670–682. doi: 10.3390/w4030670
Souchu, P., Bec, B., Smith, V. H., Laugier, T., Fiandrino, A., Benau, L., et al. (2010). Patterns in nutrient limitation and chlorophyll a along an anthropogenic eutrophication gradient in French Mediterranean coastal lagoons. Can. J. Fish. Aqua. Sci. 67, 743–753. doi: 10.1139/F10-018
Sy, M. M. (2019). Evaluation Des Services Écosystémiques Fournis Par les Complexes Lagunaires Dans un Processus de Restauration Écologique. Montpellier: Université de Montpellier, 175.
Sy, M. M., Rey-Valette, H., Simier, M., Pasqualini, V., Figuières, Ch, and De Wit, R. (2018). Identifying consensus on coastal lagoons ecosystem services and conservation priorities for an effective decision making: A Q approach. Ecol. Econ. 154, 1–13. doi: 10.1016/j.ecolecon.2018.07.018
Valiela, I., McLelland, J., Hauxwell, J., Behr, P. J., Hersh, D., and Foreman, K. (1997). Macroalgal blooms in shallow estuaries: controls and ecophysiological and ecosystem consequences. Limnol. Oceanogr. 42, 1105–1118. doi: 10.4319/lo.1997.42.5_part_2.1105
Van der Geest, M., Van der Heide, T., Holmer, M., and De Wit, R. (2020). First field-based evidence that the seagrass-lucinid mutualism can mitigate sulfide stress in seagrasses. Front. Mar. Sci. 7:11. doi: 10.3389/fmars.2020.00011
Van Katwijk, M. M., Bos, A. R., De Jonge, V. N., Hanssen, L. S. A., Hermus, D. C. R., and De Jong, D. J. (2009). Guidelines for seagrass restoration: importance of habitat selection and donor population, spreading of risks, and ecosystem engineering effects. Mar. Pollut. Bull. 58, 179–188. doi: 10.1016/j.marpolbul.2008.09.028
Van Katwijk, M. M., Thorhaug, A., Marba, N., Orth, R. J., Duarte, C. M., Kendrick, G. A., et al. (2016). Global analysis of seagrass restoration: the importance of large-scale planting. J. Appl. Ecol. 53, 567–578. doi: 10.1111/1365-2664.12562
Venice System. (1958). The Venice system for the classification of marine waters according to salinity. Limnol. Oceanogr. 3, 346–347. doi: 10.4319/lo.1958.3.3.0346
Viaroli, P., Bartoli, M., Giordano, G., Naldi, M., Orfanidis, S., and Zaldivar, J. M. (2008). Community shifts, alternative stable states, biogeochemical controls and feedbacks in eutrophic coastal lagoons: a brief overview. Aqua. Conserv. 18, 105–117. doi: 10.1002/aqc.956
Voulvoulis, N., Arpon, K. D., and Giakoumis, T. (2017). The EU Water Framework Directive: from great expectations to problems with implementation. Sci. Total Environ. 575, 358–366. doi: 10.1016/j.scitotenv.2016.09.228
WoRMS Editorial Board (2016). World Register of Marine Species. doi: 10.14284/170 Available online at: http://www.marinespecies.org at VLIZ (accessed July 23, 2019).
Yáñez-Arancibia, A., Day, J. W., Sánchez-Gil, P., Day, J. N., Lane, R. R., Zárate-Lomelí, D., et al. (2013). Ecosystem functioning: the basis for restoration and management of a tropical coastal lagoon, Pacific coast of Mexico. Ecol. Eng. 65, 88–100. doi: 10.1016/j.ecoleng.2013.03.007
Keywords: restoration ecology, ecological restoration, water quality, WFD, ecosystem trajectories, DPSIR, conservation, ecological indices
Citation: De Wit R, Leruste A, Le Fur I, Sy MM, Bec B, Ouisse V, Derolez V and Rey-Valette H (2020) A Multidisciplinary Approach for Restoration Ecology of Shallow Coastal Lagoons, a Case Study in South France. Front. Ecol. Evol. 8:108. doi: 10.3389/fevo.2020.00108
Received: 23 July 2019; Accepted: 01 April 2020;
Published: 20 May 2020.
Edited by:
Sofia Reizopoulou, Hellenic Centre for Marine Research (HCMR), GreeceReviewed by:
Christos Dimitrios Arvanitidis, Hellenic Centre for Marine Research (HCMR), GreeceSarah Faulwetter, University of Patras, Greece
Copyright © 2020 De Wit, Leruste, Le Fur, Sy, Bec, Ouisse, Derolez and Rey-Valette. This is an open-access article distributed under the terms of the Creative Commons Attribution License (CC BY). The use, distribution or reproduction in other forums is permitted, provided the original author(s) and the copyright owner(s) are credited and that the original publication in this journal is cited, in accordance with accepted academic practice. No use, distribution or reproduction is permitted which does not comply with these terms.
*Correspondence: Rutger De Wit, cnV0Z2VyLmRlLXdpdEB1bW9udHBlbGxpZXIuZnI=