- 1U.S. Geological Survey, Forest and Rangeland Ecosystem Science Center, Boise, ID, United States
- 2Division of Conservation Biology, Institute of Ecology and Evolution, Bern University, Bern, Switzerland
The use of animal-born sensors for location-based tracking and bio-logging in terrestrial systems has expanded dramatically in the past 10 years. This rapid expansion has generated new data on how animals interact with and respond to variation in their environment, resulting in important ecological, physiological, and evolutionary insights. Although understanding the finer details of animal locations has important management relevance, applied studies are not prominent in the movement ecology literature. This is despite the long history of applied studies of animal movement and the urgent and growing need for evidence-based conservation guidance, especially in the challenging field of human-wildlife interactions. The goal of this review is to evaluate the realized contribution of tracking-based animal movement ecology to solving specific conservation problems, and to identify barriers that may hinder expansion of that contribution. To do this, we (a) briefly review the history and technologies used in animal tracking and bio-logging, (b) use a series of literature searches to evaluate the frequency with which movement ecology studies are designed to solve specific conservation problems, and (c) use this information to identify challenges that may limit the applied relevance of the field of movement ecology, and to propose pathways to expand that applied relevance. Our literature review quantifies the limited extent to which research in the field of movement ecology is designed to solve specific conservation problems, but also the fact that such studies are slowly becoming more prevalent. We discuss how barriers that limit application of these principles are likely due to constraints imposed by the types of data used commonly in the field. Problems of scale mismatch, error compounding, and data paucity all create challenges that are relevant to the field of movement ecology but may be especially pertinent in applied situations. Finding solutions to these problems will create new opportunity for movement ecologists to contribute to conservation science.
Introduction
The use of animal-born sensors for location-based tracking and bio-logging in terrestrial systems has expanded dramatically in the recent past (Kays et al., 2015). This rapid expansion has resulted in important insights into ecology and physiology, especially in documenting how animals interact with and respond to variation in their environment. These recent developments have, in many cases, been seen through the theoretical lens of movement ecology (Nathan et al., 2008).
Although this work has led to basic scientific advances, understanding the finer details of animal locations and state also has important applied or management relevance (Wilson et al., 2015; Allen and Singh, 2016; McGowan et al., 2017). Assessing static individual locations (i.e., using habitat selection models and resource selection functions; Johnson, 1980; Kie et al., 2010) and measuring dynamic movement behavior (i.e., how and why organisms change locations and the consequences of that movement; movement ecology; Nathan et al., 2008; Mandel et al., 2011) are prerequisites for building models that accurately predict animal response to, and risks generated by, encounters with anthropogenic infrastructure. In effect, understanding the intimate, species-specific details of resource selection provides insight into how quality and alteration of habitat influences wildlife spatial occurrence and behavior (Sawyer et al., 2009). Likewise, understanding movement relative to humans or anthropogenic obstacles allows quantification of potential behavioral and physiological costs, and projection of associated demographic risks (Miller et al., 2014; Reid et al., 2015). Finally, integrating physiological data delivered by bio-logging together with habitat selection and movement information provides insight into how the environment constrains animal behavioral and demographic response to anthropogenic influences (Shepard et al., 2011; Mosser et al., 2014).
In spite of the applied relevance of understanding animal movement and the long history of animal tracking for applied purposes, applied studies are not prominent in the movement ecology literature. This may be because of ethical concerns associated with tracking rare species (Cooke et al., 2017). However, that is not a universal sentiment and, in some countries rare species may be preferentially tracked [e.g., California condor Gymnogyps californianus (Sheppard et al., 2015) and gray wolf Canis lupus (Bergman et al., 2006) in North America]. Therefore, the goal of this review is to evaluate the realized contribution to conservation science of terrestrial (i.e. non marine), tracking-based animal movement ecology, and to identify barriers that may hinder expansion of that contribution.
Our review begins by briefly covering the history of applied terrestrial animal tracking and bio-logging, and the current sensor technologies and environmental databases used in the field. This history is important because it is highly applied, in contrast to the modern basic emphasis of movement ecology. Likewise, describing sensor technology is important because doing so highlights constraints that may influence the applied potential of work done using these tools. Some of these issues are covered in greater detail in other reviews (Robinson et al., 2010; Tomkiewicz et al., 2010; Kays et al., 2015; Williams et al., 2020), and so we only briefly touch on these topics. However, no one manuscript summarizes all of these components together, and so their inclusion here is important in setting the stage for the concepts we present. Subsequently we use series of literature searches to evaluate, and then summarize, the realized prevalence of research that applies movement ecology principles to solve conservation problems specific to terrestrial ecosystems. This section illustrates the connections and discontinuities between movement ecology and conservation. The final section builds on the findings from the prior two to identify challenges that limit the applied relevance of the field of movement ecology and to propose pathways to expand that applied relevance.
Animal Tracking and Bio-Logging: Applied History and Technology Review
There is a long history of humans tracking animal movement for specific game management goals. Prehistoric communities of hunters-gatherers tracked and anticipated animal movements (Epp, 1988; Shaffer and Gardner, 1997) to enhance access to food (Kornfeld et al., 1999; Benedict, 2005). The first radio-based animal tracking systems were developed in the 1950s and 1960s (LeMunyan et al., 1959; Cochran and Lord, 1963). Although a predominant use of these early systems was to provide track information for wildlife management (Silv, 2012), a few also provided biotelemetry data, for example related to activity, respiration, temperature, cardiovascular, gastroenterological, and neurophysiological function (Lord et al., 1962; Kimmich, 1980). The technology to track animals in a truly remote manner was first applied in the 1960s and 1970s (Craighead et al., 1971, 1972), and was expanded with the development of the ARGOS satellite system in the 1980s. Again, these early remote tracking systems were most frequently used in applied settings.
Communication Protocols
Development of newer communication protocols has accelerated access to a suite of remotely collected sensor data, supplied in large volumes, that has moved animal tracking away from its applied roots. For example, mobile telephony [Global System for Mobile communication (GSM) or Code Division Multiple Access (CDMA)] has been used to transmit high precision Global Positioning Satellite (GPS) locations and other data collected on mammals (Bunnefeld et al., 2011) and birds (Lanzone et al., 2012). There are also tags from which data can be downloaded via other radio connections such as ZigBee (Bouten et al., 2013) or UHF (http://www.e-obs.de/index.html). Finally, when devices must be tiny, dataloggers that fall off (Sapir et al., 2014) or that require recapture and manual removal (Bridge et al., 2013) can provide locational information or monitor physiology and movements (Bevan et al., 1997; Boyd et al., 1999; Mandel et al., 2008; Bächler et al., 2010). Likewise, networks of VHF or other radios (Clements et al., 2005; Campbell et al., 2012; https://motus.org/) can track “pinging” devices attached to animals.
Sensors
There is a rapidly growing suite of miniaturized digital sensors available as off-the-shelf hardware components that can provide detailed information on animals. Although the newest of these sensors have been used primarily to advance basic ecological understanding, they have the potential for extensive use in applied ecology. Likewise, understanding these sensors and their details provides some potential insight into reasons for the limited conservation application of movement ecology principles.
More than 30 types of sensors are commercially available to technology developers and manufacturers (Table 1). Several of these—GPS, accelerometers—are already used with high frequency for bio-logging and sometimes for applied ecology. A second group, including heart rate, blood pressure, and camera sensors, are occasionally used in ecological studies and almost never in applied ecology. A third group, including proximity, current, force, and flex sensors, have potential applications to ecological research but have, to our knowledge, only rarely or never been used for either basic or applied bio-logging. The subset of these devices that are not used to address applied problems are especially important to consider here because their integration in conjunction with principles of movement ecology has the potential to stimulate new growth in applied ecology.
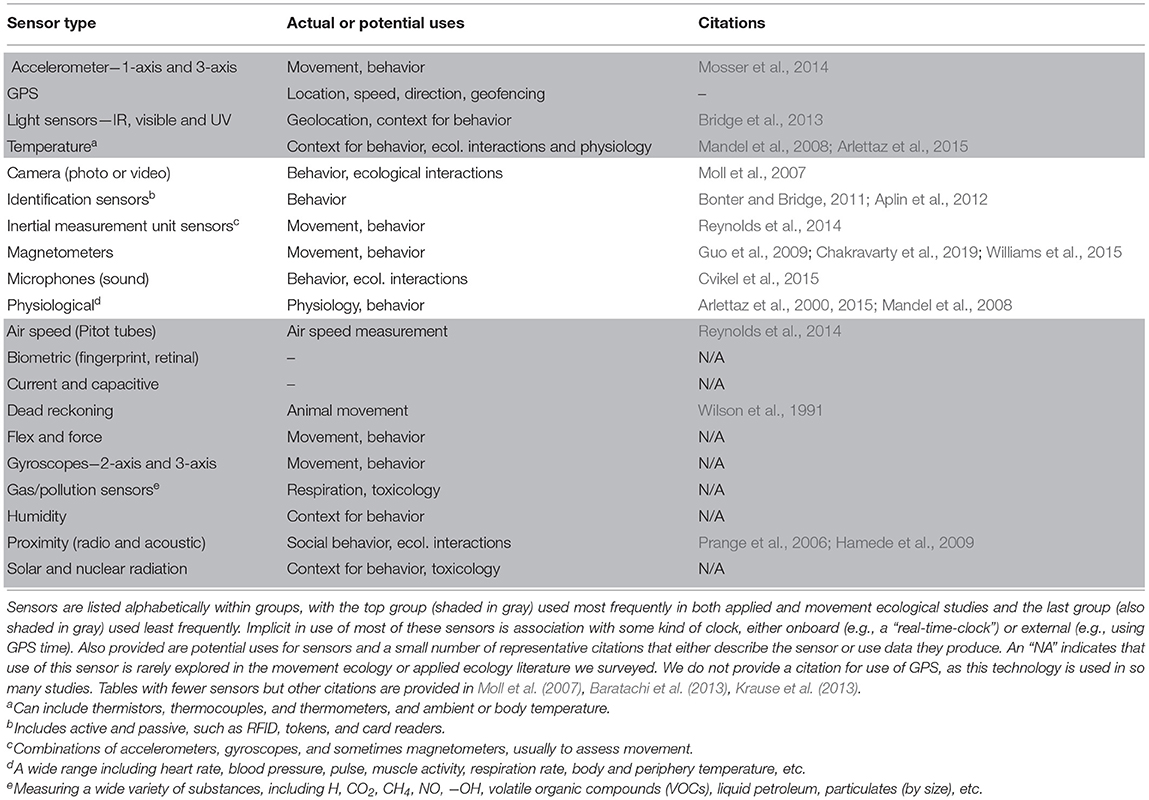
Table 1. Types of sensors available from commercial manufacturers that have been or that could be integrated into on-board animal bio-logging devices in terrestrial systems.
Data Management
A common step in processing sensor data gathered via animal tracking or bio-logging data is to associate them with external data describing the environment animals occupy. GIS and web-based tools have been developed to make these associations (Kemp et al., 2011; Mandel et al., 2011). In this way, terrestrial sensor data have been linked to a wide suite of data on land use and land cover, elevation, topography, weather, the built environment, and other features (Hebblewhite and Haydon, 2010; Table 2). The original development of the tools to statistically evaluate these linkages was often for applied purposes (e.g., Johnson, 1980). However, those applied tools are typically used with a subset of available external data types (the upper, unshaded, rows of Table 2), and less frequently used with newer datasets that are sometimes used to answer basic movement ecology questions (the lower, shaded, rows of Table 2); we return to this issue later in our review.
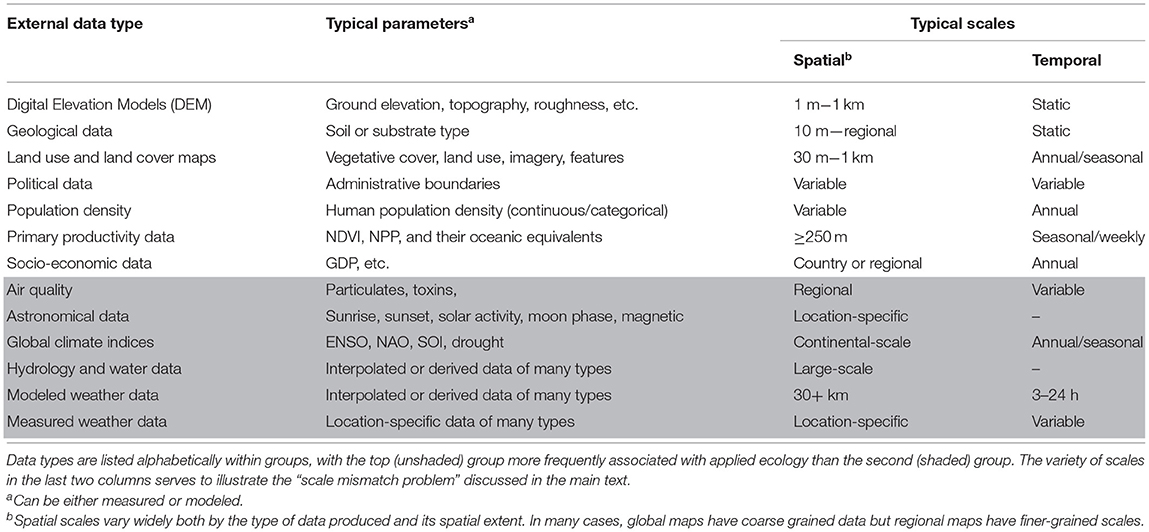
Table 2. External data sets regularly associated with animal tracking or bio-logging data, some of the typical parameters in those datasets that are associated with terrestrial animal movement data, and the typical spatial and temporal scales at which the data are collected.
Literature Review: Applications of Animal Tracking and Bio-Logging to Applied Ecology
Within the field of terrestrial wildlife management, there has been no shortage of studies of animal home range and habitat associations, movements, connectivity, and biogeography (Kie et al., 2010; Urios et al., 2015; Reiners et al., 2017). These form the foundation of modern applications of animal tracking and bio-logging science. That said, it has been suggested that modern movement ecology principles are not strongly integrated into conservation biology (Wilson et al., 2015; Allen and Singh, 2016; McGowan et al., 2017). A recent keyword-based review (Fraser et al., 2018) supports this casual observation. That review noted that many studies discuss the potential relevance to conservation of movement studies, but that a key paper framing the discipline of movement ecology (Nathan et al., 2008) did not mention conservation.
Literature Searches—Methods
To assess this observation, we conducted a series of literature searches to evaluate the realized prevalence of research that applies movement ecology principles to solve specific terrestrial conservation problems. Because different academic search engines may generate different types of results, we conducted 5 different searches using two search engines [GoogleScholar (GS) and Web of Science (WS)]. In our searches, we used four different dyads of search terms, pairing the term “movement ecology” with the terms “wildlife management” (GS + WS), “conservation biology” (WS), “species conservation” (WS), and “conservation” (WS). In our search engine surveys, we limited time frames to the period starting in 2008, when the field of movement ecology was defined (Nathan et al., 2008). Finally, we also evaluated all the articles published to date in the journal “Movement Ecology” (the 184 articles from 1:1 through 7:28).
In our interpretation of search results, we differentiated between research with ecological goals that has the potential to provide information for use in management, and research whose goals was to address specific conservation problems. Our approach builds on recent keyword-based searches that consider larger numbers of papers in less detail (Fraser et al., 2018). For example, we considered a study of home range or habitat associations of a species to be one that provides information with the potential for use in management. Although these papers represent important advances, they were not relevant to the goals of our literature review and we categorized them, together with those that generated theoretical advances, as “Descriptive.” However, we considered a study of change in the home range or habitat associations of that species in response to oil and gas development to be addressing a specific conservation problem. We categorized such papers as “Relevant” to this analysis. Other categories of papers we considered were those that were Perspectives, Viewpoints, Frameworks, or “calls to arms” (“Calls”), those that described new tools for data collection or analysis (“Tool”), review papers (“Review”), and those that were not applicable to any of these categories (“NA,” includes editorials, corrections, or papers not about movement ecology). The results of our searches are predominantly focused on terrestrial studies, but because we knew of no effective mechanism to separate terrestrial and marine studies, our results do contain some marine research papers. We did not filter those papers from our search results.
It should be noted that although we were stringent in considering which papers fit into the “Relevant” category, many papers fit into more than one of the other categories. For instance, some manuscripts reviewed background and proposed, and sometimes even used, new or modified tools. As such, these manuscripts reasonably could have been categorized as “Review,” “Descriptive,” or “Tools.” We were less stringent in assigning papers to these non-relevant categories and, thus, it may not be appropriate to use our summaries of categorization of papers for goals other than those we present here.
Literature Searches—Results
Searching GoogleScholar for the words “movement ecology” yielded about 10,300 results; searching for the words “wildlife management” yielded about 781,000 results. However, searching for the two together, and limiting our search period to the period starting in 2008 yielded about 1,900 results. We reviewed the first 200 of these (when sorted by Google's “relevance”; Table 3, Table S1). Only 14% of these papers could reasonably be classified as research in which elements of movement ecology are used to solve specific conservation problem. An additional 7% of studies were “calls to arms,” some of which discuss the need for animal movement studies to have more applied relevance. The remainder of those first 200 papers were not relevant to this review.
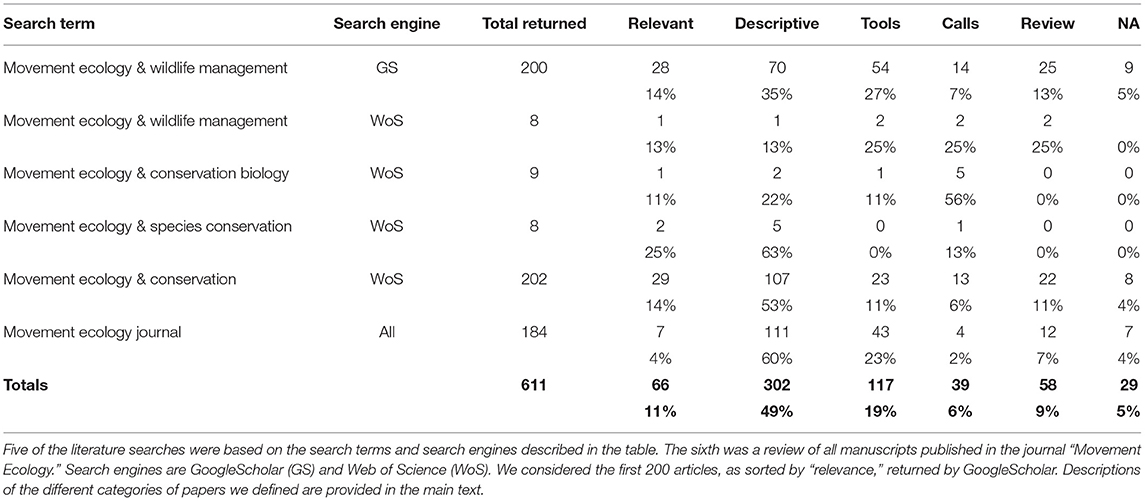
Table 3. Results from six literature searches to assess the degree to which modern movement ecology principles are integrated into conservation biology.
We performed four other searches using Web of Science. Searching for the terms “movement ecology” and “wildlife management” returned eight papers, of which one was relevant (Table 3, Table S2). Searching for the terms “movement ecology” and “conservation biology” returned nine papers, one of which was relevant (Table 3,Table S2). Searching for the terms “movement ecology” and “species conservation” returned eight papers, two of which were relevant (Table 3, Table S2). Finally, searching for the terms “movement ecology” and the general term “conservation” returned 202 papers, of which only 14% were relevant (Table 3, Table S3). Interestingly, the number of these papers has been increasing over time (Figure 1), and 16 of these 29 articles (55%) were published in the last 2 years.
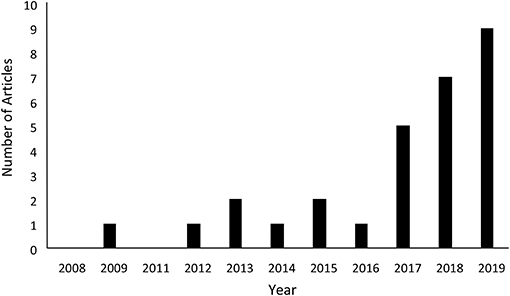
Figure 1. Number of papers published in which movement ecology principles were used to solve specific conservation problems. These data are based on returns from a search of Web of Science for the terms “movement ecology” and “conservation.” The search returned 202 papers, of which 16 were categorized as those in which movement ecology principles were used to solve specific conservation problems.
Finally, we reviewed all 184 articles published in the journal “Movement Ecology” since its creation (Table 3, Table S4). Conservation relevance is not part of the journal aims and scope and so, not surprisingly, we categorized the vast majority of papers as “descriptive” of biology or theory. We categorized only seven papers (4%) as those that used movement ecology principles or tools to solve specific conservation problems. Four of these seven were published in the last 2 years. That said, several of the descriptive papers (at a minimum, #26, 37, 78, and 114, in bold font in Table S4) had the data that would have allowed the authors to tell an applied conservation story. One other paper (#179, also in bold) was a review that drew linkages between movement ecology and biodiversity conservation.
Literature Searches—Summary
Although our literature search illustrated the paucity with which modern movement ecology approaches are used to generate solutions to specific conservation problems, it also highlighted several examples of this being done. Here, to illustrate those examples, we summarize the major themes in these and related publications.
Wildlife management problems have been addressed in a movement ecology framework by overlaying tracking data on data layers describing anthropogenic activity. For example, one paper noted in our literature search maps animal movement in the context of proposed oil and gas development (Sawyer et al., 2009). In other studies, presence data based on snow tracks were used to build spatially explicit models of wildlife abundance and overlay them on maps of projected human recreational activity (Braunisch et al., 2011) and telemetry data illustrated how raptors changed home range size in response to human recreation (Perona et al., 2019). Similarly, models of avian flight behavior have been linked to projections of wind turbine locations to predict risk to birds from blade-strike (Miller et al., 2014; Reid et al., 2015). In fact, the majority of the relevant studies we found in our searches and in the journal evaluated animal movement in the context of data layers describing habitat alterations, roads, resource extraction or anthropogenic influences (Tables S1–S4). One of the salient features of these papers is that many authors seem to struggle with issues related to the scale, accuracy and precision of these external data layers; we return to this issue later in our review.
Human-wildlife conflict issues also have been addressed with real-time alerts generated by tracking technologies commonly used in movement ecology. For example, alerts were created when California condors (Sheppard et al., 2015) and African elephants (Loxodonta africana; Wall et al., 2014) were near to human-driven threats (wind turbines and subsistence farms, respectively). These alerts can lead to actions to reduce risk (by shutting down wind turbines) or to change the animal's trajectory (aversive conditioning to keep the elephant out of a farm). In the case of the elephants, there was additional wildlife health benefit because alerts were generated when elephants altered or stopped movements in ways that indicated illness or death. In another applied study, real-time tracking of gulls was used to identify illegal waste dumping (Navarro et al., 2016). However, in spite of their novelty and utility as an applied tool, real-time alerts have technological constraints and are only relevant to the individual or groups of animals being tracked. As such, although alerts can be useful in solving an individual-specific management problem, they are rarely relevant to broad population-level conservation solutions.
Movement ecology approaches have been used in a few other applied settings. For example, statistical modeling of remotely gathered bio-logging data can provide new insight about the environment an animal experiences. In this vein, analysis of data from soaring birds has been used as a tool to infer information about wind velocity and scale that may contribute to high-resolution weather observations (Treep et al., 2016; Weinzierl et al., 2016). Likewise, data from GPS sensors or accelerometry, together or separately, can be interpreted to characterize animal behaviors (e.g., Wilson et al., 2006; Shepard et al., 2011; Nathan et al., 2012; Katzner et al., 2015; Sur et al., 2017) that can then be modeled as a function of either energy expenditure or response to disturbance (Wilson et al., 2015). In contrast to real-time alerts, integration of bio-logging data into these types of statistical models is applicable to populations and therefore has greater, often unrealized, potential for broad applied ecological relevance.
Finally, a recurring theme in our review was the presence of a fairly large number of papers pointing out the relevance to conservation biology of principles developed via movement ecology (i.e., “call to arms”). These pieces illustrate a broader recognition that there is value to using principles of movement ecology for conservation purposes. That said, the fact that such papers still are being published suggests that there is room for improvement in this regard.
Discussion: Constraints That Limit Application of Movement Ecology to Conservation Biology
Movement ecology aims to triangulate among the organism's internal state, its capacity to move, and the external factors it experiences (Nathan et al., 2008). Our review highlights both some of the ways this triangulation has been used in applied settings but also how technological and other constraints may influence applied interpretation of movement data. Evaluating those constraints highlights potential reasons why applied studies are not prominent in the movement ecology literature and this process identifies potential solutions to that problem.
Constraints Due to Data Scale, Accuracy, and Precision
Literature review suggests that making linkages between animal movement and the environment is prone to bias imposed by variation in the scale, accuracy, and precision of environmental data types. These biases manifest themselves in at least three different and highly relevant ways. The first of these is the problem of mismatches in scale between different sources of data used in movement research (i.e., a “scale mismatch problem”; see Table 2; Neumann et al., 2015; Remelgado et al., 2018). For example, although GPS data often are accurate to a spatial scale of meters or centimeters and a temporal scale of seconds, land cover data are at time scales of seasons or years and spatial scales of 10s or 100s of meters. Likewise, weather data are modeled over temporal scales of hours and spatial scales of tens of kilometers (e.g., Mandel et al., 2011). This constrains analyses to inference at the scale of the coarsest dataset. However, behavior often is the result of decisions at finer scales, creating challenges in identifying drivers of animal behavior.
A second bias can be imposed by compounding of measurement errors in data sets commonly used in movement ecology (i.e., an “error compounding problem”). For example, when estimating an animal's location in 3D, GPS measurement error combines with error and averaging within an elevation model (Péron et al., 2017; Poessel et al., 2018). As a consequence, small errors of measurement in GPS data from a bird flying 100 m above ground and 5 m from a cliff may cause the animal to appear to be in the cliff and, therefore, below ground. Such infrequent errors may be of limited consequence to the process of detecting ecological patterns in data, but they may be substantially more relevant in an applied situation where rare events (e.g., collisions of birds with wind turbine blades or airplanes) can dictate policy. Furthermore, although their presence can be evaluated with modern ecological statistics, minimizing, rather than characterizing, errors is of key importance to managers.
A third bias may be imposed by the paucity of data describing ecologically relevant resources (e.g., distribution of food, nests, or den sites; a “data paucity problem”). This is akin to the knowledge gap problem identified in previous reviews (Fraser et al., 2018). Without such ecologically relevant data, interpretation relies on inference from proxies rather than direct measurement. As an example, mapping potential breeding areas for cavity nesting birds might be best evaluated by measuring snag density. This is difficult though and, instead, potential nesting areas are usually identified by inference, using land cover class or tree species richness as a proxy for snag density (van der Hoek et al., 2017).
Addressing all three of these problems would have relevance to both fundamental and applied ecology. However, because so many management problems are scale- and measurement-specific, solving these issues may be especially relevant to applied ecology. With this in mind, one way to give the field of movement ecology more applied relevance may be through integration of data from less frequently used on-board or intrinsic sensors that can describe and map the environment the animal experiences (e.g., Treep et al., 2016). Integrating cameras, weather sensors and GPS data could provide dramatic new insights into micro-habitat selection and its consequences for conservation-dependent wildlife. Similarly, in wildlife disease studies, samples collected from an animal's environment (e.g., toxins in potential food) are linked to those collected from the animal itself (e.g., toxins in blood). Here again, rarely-used on-board sensors that sample toxins or disease in the animal and in the environment or the food an animal ate (Loyd et al., 2013; Lavelle et al., 2015; McGregor et al., 2015) could make explicit, and more valuable, the linkages between the location, experience, and the internal state of an animal.
All of these solutions though share a common theme. In each case, they illustrate the value of onboard sensors to measure the actual environment the animal experiences, contributing to potential resolution of the data mismatch, error compounding, and data paucity problems. Such an approach would reduce reliance on coarse-scale environmental data layers that may limit application of movement ecology principles to conservation settings.
Constraints Due to Data Availability, Integration, Validation, and Transmission
It was evident in our review that miniaturization of new generations of sensors has created reams of data that yield both opportunity and overload. The first ARGOS telemetry systems used a single sensor to measure location. Modern devices use a suite of sensors to measure repeatedly the location, the environment, and the animal's condition, actions, and physiology. Integration of these data from multiple sensors, although rarely achieved, allows users to refine understanding not only of animal movement but also of error in any one sensor. These refinements make data more accurate and precise and, thus, more relevant to both fundamental and applied ecology.
Developing the conceptual and quantitative tools to integrate, validate, analyze, and interpret these data is non-trivial. As a consequence, it is not unusual for users of sensor data in basic ecological studies to classify behaviors without externally validating their classification algorithms (e.g., Bishop et al., 2015). Although such an approach may be acceptable for basic ecology, it is not well-suited to the applied arena. Consider, for example, the sociological consequences of even a low rate of false positives or false negatives associated with real-time alerts for crop-raiding elephants (Wall et al., 2014). Therefore, two important steps in bridging the gap between basic and applied animal movement science are (a) developing methodological tools to integrate, analyze and interpret data from multiple sensors (for example, drawing from tools used to evaluate human mobility data; Thums et al., 2018), and (b) moving to consistent use of supervised classification and validation of remotely gathered biotelemetry data (e.g., Nathan et al., 2012).
Perhaps the greatest technological limitation to data collection via remotely-sensed animal bio-logging is the narrow bandwidth pipe connecting the animal in the field to the computing servers that host the data. Such constraints are especially relevant to applied ecology because conservation-dependent terrestrial species are only sometimes well-suited to intensive study. Thus, massive amounts of accelerometry data from griffon vultures in Europe and the Middle East can be sent over VHF systems, in part because these birds are colonial and regularly return to a set of nest cliffs (Nathan et al., 2012). In contrast, it would be far more difficult to collect such data from ecologically similar but non-colonial California condors that often occupy more remote and roadless areas (Poessel et al., 2018). This limitation explains why accelerometry and animal-borne video studies are often performed on wildlife that are faithfully colonial or easily recaptured, or on captive or domestic animals (Moll et al., 2007; Loyd et al., 2013; McGregor et al., 2015; den Uijl et al., 2017; Hernández-Pliego et al., 2017; Hicks et al., 2017; le Roux et al., 2017).
In certain areas the use of mobile phone telemetry can greatly expand the volume and speed of data transfer from bio-logging devices to scientists. That said, even 4G and LTE systems often are insufficient to transfer the large quantities of data collected at many measurements per second. This is likely why wildlife accelerometry that uses GSM systems is, to date, largely experimental (Yuan et al., 2010; Gor et al., 2017; Sur et al., 2017). Likewise, for those animals that live outside mobile phone coverage, the only option to recover large volumes of data is animal recapture and removal of data loggers. A potential solution to this problem are hybrid solutions that send GPS data via satellite but other data via other systems (e.g., GSM, ZigBee, VHF, or satellite internet services). Eventual deployment of 5G mobile phone systems also has the potential to speed up data transfer rates to address this issue.
Constraints and Conservation
The constraints we highlight here are all influential for movement ecology in general, and not exclusively for solving conservation problems. In fact, the early research into animal movement was more strongly influenced by these constraints than is modern movement ecology, yet it was still highly applied. Similarly, rapid advances have been made linking movement ecology and conservation management in the marine environment, despite greater data constraints than in terrestrial settings.
Since these constraints didn't influence historical or marine studies, it is therefore not easy to understand why the constraints we identify in literature may limit applied relevance of movement ecology to terrestrial conservation management. One possible explanation is that, in the subset of marine ecological and early animal movement studies that are relevant to conservation, measurements are collected and models built at relatively large spatial and temporal scales (e.g., hours and kilometers, see Tables 1, 2; Reisinger et al., 2018). As such, the coarse scales of movement match the coarse scale of environmental datasets, and are thus relatively uninfluenced by error rates, and data transmission and management constraints. In contrast, terrestrial movement ecology collects measurements and build models at precise spatial and temporal scales (e.g., meters and seconds, see Tables 1, 2; Kays et al., 2015). In those situations, the constraints we note here have a greater influence both on the ability to conduct the work, and also on the conservation relevance of the data.
Conclusions
Our review highlights the fact that principles of movement ecology are sometimes used to solve specific conservation problems. However, there is immense potential for expansion in this regard and that potential is starting to be realized, as illustrated by the increase in papers in this area in the past 2 years. There are constraints that may limit the continued expansion in this area. Some of these constraints include those highlighted by others, such as the availability of data or results, improved dialogue between scientists and practitioners, and development of frameworks for incorporating movement ecology into conservation (Fraser et al., 2018). Others we identify here are linked to issues of the transmission and validation of massive amounts of telemetry data, others to the scale, accuracy, precision, error rates, availability and integration of external data layers that are used to infer drivers of behavior. Nonetheless, solutions or work-arounds are already being developed to overcome many of these social and technological constraints. As these solutions come on-line, there will be new opportunities for movement ecologists to meet the urgent and growing need for evidence-based conservation. It is our hope that, by highlighting these issues, this review and commentary will spur growth in applied movement ecology studies.
Author Contributions
The concept for this manuscript was developed as a result of discussions between the two authors. TK led writing. Both authors participated in revising and editing the document.
Funding
The authors' institutions provided support for this work.
Conflict of Interest
The authors declare that the research was conducted in the absence of any commercial or financial relationships that could be construed as a potential conflict of interest.
Acknowledgments
The authors thank V. Braunisch, A. Duerr, T. Miller, and S. Poessel for insightful comments on drafts of the manuscript. Any use of trade, product, or firm names is for descriptive purposes only and does not imply endorsement by the U.S. Government.
Supplementary Material
The Supplementary Material for this article can be found online at: https://www.frontiersin.org/articles/10.3389/fevo.2019.00519/full#supplementary-material
References
Allen, A. M., and Singh, N. J. (2016). Linking movement ecology with wildlife management and conservation. Front. Ecol. Evol. 3:166. doi: 10.3389/fevo.2015.00155
Aplin, L. M., Farine, D. R., Morand-Ferron, J., and Sheldon, B. C. (2012). Social networks predict patch discovery in a wild population of songbirds. Proc. R. Soc. B. 279, 4199–4205. doi: 10.1098/rspb.2012.1591
Arlettaz, R., Nusslé, S., Baltic, M., Vogel, P., Palme, R., Jenni-Eiermann, S., et al. (2015). Disturbance of wildlife by outdoor winter recreation: allostatic stress response and altered activity-energy budgets. Ecol. App. 25, 1197–1212. doi: 10.1890/14-1141.1
Arlettaz, R., Ruchet, C., Aeschimann, J., Brun, E., Genoud, M., and Vogel, P. (2000). Physiological traits affecting the distribution and wintering strategy of the bat Tadarida teniotis. Ecology 81, 1004–1014 doi: 10.1890/0012-9658(2000)081[1004:PTATDA]2.0.CO;2
Bächler, E., Hahn, S., Schaub, M., Arlettaz, R., Jenni, L., Fox, J. W., et al. (2010). Year-round tracking of small trans-Saharan migrants using light-level geolocators. PLoS ONE. 5:e9566. doi: 10.1371/journal.pone.0009566
Baratachi, M., Meratnia, N., Havinga, P. J. M., Skidmore, A. K., and Toxopeus, B. A. G. (2013). Sensing solutions for collecting spatio-temporal data for wildlife monitoring applications: a review. Sensors 13, 6054–6088. doi: 10.3390/s130506054
Benedict, J. B. (2005). Tundra game drives: an Arctic – Alpine comparison. Arct. Antarct. Alp. Res. 37, 425–434. doi: 10.1657/1523-0430(2005)037[0425:TGDAAC]2.0.CO;2
Bergman, E. J., Garrott, R. A., Creel, S., Borkowski, J. J., Jaffe, R., and Watson, F. G. R. (2006). Assessment of prey vulnerability through analysis of wolf movements and kill sites. Ecol. Apps. 16, 273–284. doi: 10.1890/04-1532
Bevan, R. M., Boyd, I. L., Butler, P. J., Reid, K., Woakes, A. J., and Croxall, J. P. (1997). Heart rates and abdominal temperatures of free-ranging South Georgian Shags, Phalacrocorax georgianus. J. Exper. Biol. 200, 661–675.
Bishop, C. M., Spivey, R. J., Hawkes, L. A., Batbayar, N., Chua, B., Frappell, P. B., et al. (2015). The roller coaster flight strategy of bar-headed geese conserves energy during Himalayan migrations. Science 347, 250–254. doi: 10.1126/science.1258732
Bonter, D. N., and Bridge, E. S. (2011). Applications of radio frequency identification (RFID) in ornithological research: a review. J. Field Ornith. 82, 1–10. doi: 10.1111/j.1557-9263.2010.00302.x
Bouten, W., Baaij, E. W., Shamoun-Baranes, J., and Camphuysen, K. C. J. (2013). A flexible GPS tracking system for studying bird behavior at multiple scales. J. Ornith. 154, 571–580. doi: 10.1007/s10336-012-0908-1
Boyd, I. L., Bevan, R. M., Woakes, A. J., and Butler, P. J. (1999). Heart rate and behavior of fur seals: implications for measurement of field energetics. Am. J. Physiol. 276, H844–H857. doi: 10.1152/ajpheart.1999.276.3.H844
Braunisch, V., Patthey, P., and Arlettaz, R. (2011). Spatially explicit modeling of conflict zones between wildlife and snow sports: prioritizing areas for winter refuges. Ecol. App. 21, 955–967. doi: 10.1890/09-2167.1
Bridge, E. S., Kelly, J. F., Contina, A., Gabrielson, R. M., MacCurdy, R. B., and Winkler, D. W. (2013). Advances in tracking small migratory birds: a technical review of light-level geolocation. J. Field Ornith. 84, 121–137. doi: 10.1111/jofo.12011
Bunnefeld, N., Börger, L., van Moorter, B., Rolandsen, C. M., Dettki, H., Solberg, E. J., et al. (2011). A model-driven approach to quantify migration patterns: individual, regional and yearly differences. J. Anim. Ecol. 80, 466–476. doi: 10.1111/j.1365-2656.2010.01776.x
Campbell, H. A., Hewitt, M., Watts, M. E., Peverell, S., and Franklin, C. E. (2012). Short- and long-term movement patterns in the freshwater whipray (Himantura dalyensis) determined by the signal processing of passive acoustic telemetry data. Marine Freshwater Res. 63, 341–350. doi: 10.1071/MF11229
Chakravarty, P., Maalberg, M., Cozzi, G., Ozgul, A., and Aminian, K. (2019). Behavioural compass: animal behaviour recognition using magnetometers. Mov. Ecol. 7:28. doi: 10.1186/s40462-019-0172-6
Clements, S., Jepsen, D., Karnowski, M., and Schreck, C. (2005). Optimization of an acoustic telemetry array for detecting transmitter-implanted fish. North Am. J. Fish Manage. 25, 429–436 doi: 10.1577/M03-224.1
Cochran, W. W., and Lord, R. D. (1963). A radio-tracking system for wild animals. J. Wild. Manage. 27, 9–24. doi: 10.2307/3797775
Cooke, S. J., Nguyen, V. M., Kessel, S. T., Hussey, N. E., Young, N., and Ford, A. T. (2017). Troubling issues at the frontier of animal tracking for conservation and management. Cons. Biol. 31, 1205–1207. doi: 10.1111/cobi.12895
Craighead, F. C. Jr., Craighead, J. J., Cote, C. E., and Beuchner, H. K. (1972). “Satellite and ground radio tracking of elk,” in Proceedings of a Conference on Animal Orientation and Navigation, Vol. 262, eds S. R. Galler, K. Schmidt-Koenig, G. J. Jacobs, and R. E. Belleville (NASA Scientific Publications), 99–111.
Craighead, J. J., Craighead, F. C. Jr., Varney, R., and Cote, C. E. (1971). Satellite monitoring of black bear. BioScience 21, 1206–1212. doi: 10.2307/1296018
Cvikel, N., Berg, K. E., Levin, E., Hurme, E., Borissov, I., Boonman, A., et al. (2015). Bats aggregate to improve prey search but might be impaired when their density becomes too high. Curr. Biol. 25, 206–211. doi: 10.1016/j.cub.2014.11.010
den Uijl, I., Gómez Álvarez, C. B., Bartram, D., Dror, Y., Holland, R., and Cook, A. (2017). External validation of a collar-mounted triaxial accelerometer for second-by-second monitoring of eight behavioural states in dogs. PLoS ONE. 12:e0188481. doi: 10.1371/journal.pone.0188481
Epp, H. T. (1988). Way of the migrant herds: dual dispersion strategy among bison. Plains Anthropol. 33, 309–320. doi: 10.1080/2052546.1988.11909402
Fraser, K. C., Davies, K. T. A., Davy, C. M., Ford, A. T., Flockhart, D. T. T., and Martins, E. G. (2018). Tracking the conservation promise of movement ecology. Front. Ecol. Evol. 6:150. doi: 10.3389/fevo.2018.00150
Gor, M., Vora, J., Tanwar, S., Tyagi, S., Kumar, N., Obaidat, M. S., et al. (2017). “GATA: GPS-arduino based tracking and alarm system for protection of wildlife animals,” in 2017 International Conference on Computer, Information and Telecommunication Systems (CITS), eds M. S. Obaidat, Z. Chen, K.-F. Hsiao Petros Nicopolitidis, and D. Cascado (Dailan). doi: 10.1109/CITS.2017.8035325
Guo, Y., Poulton, G., Corke, P., Bishop-Hurley, G. J., Wark, T., and Swain, D. L. (2009). Using accelerometer, high sample rate GPS and magnetometer data to develop a cattle movement and behaviour model. Ecol. Modelling. 220, 2068–2075. doi: 10.1016/j.ecolmodel.2009.04.047
Hamede, R. K., Bashford, J., McCallum, H., and Jones, M. (2009). Contact networks in a wild Tasmanian devil (Sarcophilus harrisii) population: using social network analysis to reveal seasonal variability in social behaviour and its implications for transmission of devil facial tumour disease. Ecol. Lett. 12, 1147–1157. doi: 10.1111/j.1461-0248.2009.01370.x
Hebblewhite, M., and Haydon, D. T. (2010). Distinguishing technology from biology: a critical review of the use of GPS telemetry data in ecology. Phil. Trans. R. Soc. B. 365, 2303–2312. doi: 10.1098/rstb.2010.0087
Hernández-Pliego, J., Rodríguez, C., Dell'Omo, G., and Bustamante, J. (2017). Combined use of tri-axial accelerometers and GPS reveals the flexible foraging strategy of a bird in relation to weather conditions. PLoS ONE. 12:e0177892. doi: 10.1371/journal.pone.0177892
Hicks, O., Burthe, S., Daunt, F., Butler, A., Bishop, C., and Green, J. A. (2017). Validating accelerometry estimates of energy expenditure across behaviours using heart rate data in a free-living seabird. J. Exper. Biol. 220, 1875–1881. doi: 10.1242/jeb.152710
Johnson, D. H. (1980). The comparison of usage and availability measurements for evaluating resource preference. Ecology 61, 65–71. doi: 10.2307/1937156
Katzner, T. E., Turk, P. J., Duerr, A. E., Miller, T. A., Lanzone, M. J., Cooper, J. L., et al. (2015). Use of multiple modes of flight subsidy by a soaring terrestrial bird, the golden eagle Aquila chrysaetos, when on migration. J. R. Soc. Interface. 12:20150530. doi: 10.1098/rsif.2015.0530
Kays, R., Crofoot, M. C., Jetz, W., and Wikelski, M. (2015). Terrestrial animal tracking as an eye on life and planet. Science 348:1222. doi: 10.1126/science.aaa2478
Kemp, M. U., van Loon, E. E., Shamoun-Baranes, J., and Bouten, W. (2011). RNCEP: global weather and climate data at your fingertips. Methods Ecol. Evol. 3, 65–70. doi: 10.1111/j.2041-210X.2011.00138.x
Kie, J. G., Matthiopoulos, J., Fieberg, J., Powell, R. A., Cagnacci, F., Mitchell, M. S., et al. (2010). The home range concept are traditional estimators still relevant with modern telemetry technology. Phil. Trans. R. Soc. B. 365, 2221–2231. doi: 10.1098/rstb.2010.0093
Kimmich, H. P. (1980). “Artifact free measurement of biological parameters: biotelemetry, a historical review and layout of modern developments,” in A Handbook on Biotelemetry and Radio Tracking, eds C. J. Amlaner Jr., D. W. MacDonald (Oxford: Pergamon Press), 3–20. doi: 10.1016/B978-0-08-024928-5.50006-3
Kornfeld, M., Frison, G. C., Larson, M. L., Miller, J. C., and Saysette, J. (1999). Paleoindian bison procurement and paleoenvironments in Middle Park of Colorado. Geoarchaeology 14, 655–674. doi: 10.1002/(SICI)1520-6548(199910)14:7<655::AID-GEA3>3.0.CO;2-L
Krause, J., Krause, S., Arlinghaus, R., Psorakis, I., Roberts, S., and Rutz, C. (2013). Reality mining of animal social systems. Trends Ecol. Evol. 28, 541–551. doi: 10.1016/j.tree.2013.06.002
Lanzone, M., Miller, T., Turk, P., Brandes, D., Halverson, C., Maisonneuve, C., et al. (2012). Flight responses by a migratory soaring raptor to changing meteorological conditions. Biol. Lett. 8, 710–713. doi: 10.1098/rsbl.2012.0359
Lavelle, M. J., Blass, C. R., Fischer, J. W., Hygnstrom, S. E., Hewitt, D. G., and VerCauteren, K. C. (2015). Food habits of adult male white-tailed deer determined by camera collars. Wild. Soc. Bull. 39, 651–657. doi: 10.1002/wsb.556
le Roux, S. P., Marias, J., Wolhuter, R., and Niesler, T. (2017). Animal-borne behaviour classification for sheep (Dohne Merino) and Rhinoceros (Ceratotherium simum and Diceros bicornis). Anim. Biotelem. 5:25. doi: 10.1186/s40317-017-0140-0
LeMunyan, C. D., White, W., Nyberg, E., and Christian, J. J. (1959). Design of a miniature radio transmitter for use in animal studies. J. Wild. Manage. 23, 107–110. doi: 10.2307/3797755
Lord, R. D. Jr., Bellrose, F. C., and Cochran, W. W. (1962). Radio-telemetry of the respiration of a flying duck. Science 137, 39–40. doi: 10.1126/science.137.3523.39
Loyd, K. A. T., Hernandez, S. M., Carroll, J. P., Abernathy, K. J., and Marshall, G. J. (2013). Quantifying free-roaming domestic cat predation using animal-borne video cameras. Biol. Cons. 160, 183–189. doi: 10.1016/j.biocon.2013.01.008
Mandel, J. T., Bildstein, K. L., Bohrer, G., and Winkler, D. W. (2008). Movement ecology of migration in turkey vultures. Proc. Nat. Acad. Sci. U.S.A. 105, 19102–19107. doi: 10.1073/pnas.0801789105
Mandel, J. T., Bohrer, G., Winkler, D. W., Barber, D. R., Houston, C. S., and Bildstein, K. L. (2011). Migration path annotation: cross-continental study of migration-flight response to environmental conditions. Ecol. App. 21, 2258–2268. doi: 10.1890/10-1651.1
McGowan, J., Beger, M., Lewison, R. L., Harcourt, R., Campbell, H., Priest, M., et al. (2017). Integrating research using animal-borne telemetry with the needs of conservation management. J. Appl. Ecol. 54, 423–429. doi: 10.1111/1365-2664.12755
McGregor, H., Legge, S., Jones, M. E., and Johnson, C. N. (2015). Feral cats are better killers in open habitats, revealed by animal-borne video. PLoS ONE. 10:e0133915. doi: 10.1371/journal.pone.0133915
Miller, T. M., Brooks, R. P., Lanzone, M., Brandes, D., Cooper, J., O'Malley, K., et al. (2014). Assessing risk to birds from industrial wind energy development via paired resource selection models. Cons. Biol. 28, 745–755. doi: 10.1111/cobi.12227
Moll, R. J., Millspaugh, J. J., Beringer, J., Sartwell, J., and He, Z. (2007). A new ‘view' of ecology and conservation through animal-borne video systems. Trends Ecol. Evol. 22, 660–668 doi: 10.1016/j.tree.2007.09.007
Mosser, A. A., Avgar, T., Brown, G. S., Walker, C. S., and Fryxell, J. M. (2014). Towards an energetic landscape: broad-scale accelerometry in woodland caribou. J. Anim. Ecol. 83, 916–922. doi: 10.1111/1365-2656.12187
Nathan, R., Getz, W. M., Revilla, E., Holyoak, M., Kadmon, R., Saltz, D., et al. (2008). A movement ecology paradigm for unifying organismal movement research. Proc. Nat. Acad. Sci. U.S.A. 105, 19052–19059. doi: 10.1073/pnas.0800375105
Nathan, R., Spiegel, O., Fortmann-Roe, S., Harel, R., Wikelski, M., and Getz, W. M. (2012). Using tri-axial acceleration data to identify behavioral modes of free-ranging animals: general concepts and tools illustrated for griffon vultures. J. Exper. Biol. 215, 986–996. doi: 10.1242/jeb.058602
Navarro, J., Grémillet, D., Afán, I., Ramírez, F., Bouten, W., and Forero, M. G. (2016). Feathered detectives: real-time GPS tracking of scavenging gulls pinpoints illegal waste dumping. PLoS ONE 11:e0159974. doi: 10.1371/journal.pone.0159974
Neumann, W., Martinuzzi, S., Estes, A. B., Pidgeon, A. M., Dettki, H., Ericsson, G., et al. (2015). Opportunities for the application of advanced remotely-sensed data in ecological studies of terrestrial animal movement. Mov. Ecol. 3:8. doi: 10.1186/s40462-015-0036-7
Péron, G., Fleming, C. H., Duriez, O., Fluhr, J., Itty, C., Lambertucci, S., et al. (2017). The energy landscape predicts flight height and wind turbine collision hazard in three species of large soaring raptor. J. Appl. Ecol. 54, 1895–1906. doi: 10.1111/1365-2664.12909
Perona, A. M., Urios, V., and López-López, P. (2019). Holidays? Not for all. Eagles have larger home ranges on holidays as a consequence of human disturbance. Biol. Cons. 231, 59–66. doi: 10.1016/j.biocon.2019.01.010
Poessel, S. A., Duerr, A. E., Hall, J. C., Braham, M. A., and Katzner, T. E. (2018). Improving estimation of flight altitude in wildlife telemetry studies. J. Appl. Ecol. 55, 2064–2070. doi: 10.1111/1365-2664.13135
Prange, S., Jordan, T., Hunter, C., and Gehrt, S. D. (2006). New radiocollars for the detection of proximity among individuals. Wild. Soc. Bull. 34, 1333–1344. doi: 10.2193/0091-7648(2006)34[1333:NRFTDO]2.0.CO;2
Reid, T., Kruger, S., Whifield, D. P., and Amar, A. (2015). Using spatial analyses of bearded vulture movements in southern Africa to inform wind turbine placement. J. Appl. Ecol. 52, 881–892. doi: 10.1111/1365-2664.12468
Reiners, W. A., Lockwood, J. A., Reiners, D. S., and Prager, S. D. (2017). 100 years of ecology: what are our concepts and are they useful? Ecol. Monogr. 87, 260–277. doi: 10.1002/ecm.1243
Reisinger, R. R., Raymond, B., Hindell, M. A., Bester, M. N., Crawford, R. J., Davies, D., et al. (2018). Habitat modelling of tracking data from multiple marine predators identifies important areas in the Southern Indian Ocean. Divers. Distrib. 24, 535–550. doi: 10.1111/ddi.12702
Remelgado, R., Leutner, B., Safi, K., Sonnenschein, R., Kuebert, C., and Wegmann, M. (2018). Linking animal movement and remote sensing–mapping resource suitability from a remote sensing perspective. Remote Sens. Ecol. Cons. 4, 211–224. doi: 10.1002/rse2.70
Reynolds, K. V., Thomas, A. L. R., and Taylor, G. (2014). Wing tucks are a response to atmospheric turbulence in the soaring flight of the steppe eagle Aquila nipalensis. J. R. Soc. Interface 11:20140645. doi: 10.1098/rsif.2014.0645
Robinson, W. D., Bowlin, M. S., Bisson, I., Shamoun-Baranes, J., Thorup, K., Diehl, R. H., et al. (2010). Integrating concepts and technologies to advance the study of bird migration. Front. Ecol. Envir. 8, 354–361. doi: 10.1890/080179
Sapir, N., Horvitz, N., Dechmann, D. K. N., Fahr, J., and Wikelski, M. (2014). Commuting fruit bats beneficially modulate their flight in relation to wind. Proc. R. Soc. B 281:20140018. doi: 10.1098/rspb.2014.0018
Sawyer, H., Kauffman, M. J., Kielson, R. M., and Horne, J. S. (2009). Identifying and prioritizing ungulate migration routes for landscape-level conservation. Ecol. App. 19, 2016–2025. doi: 10.1890/08-2034.1
Shaffer, B. S., and Gardner, K. M. (1997). Reconstructing animal exploitation by Puebloan peoples of the southwestern United States using Mimbres pottery, AD 1000-1150. Anthropozoologica 25, 263–268.
Shepard, E. L. C., Lambertucci, S. A., Vallmitjana, D., and Wilson, R. P. (2011). Energy beyond food: foraging theory informs time spent in thermals by a large soaring bird. PLoS ONE 6:e27375. doi: 10.1371/journal.pone.0027375
Sheppard, J. K., McGann, A., Lanzone, M., and Swaisgood, R. R. (2015). An autonomous GPS geofence alert system to curtail avian fatalities at wind farms. Anim. Biotelem. 3:43. doi: 10.1186/s40317-015-0087-y
Silv, N. J., (ed.). (2012). The Wildlife Techniques Manual. V.1, 2, 7th Edn. Baltimore, MD: Johns Hopkins University Press.
Sur, M., Suffredini, T., Wessells, S. M., Bloom, P. H., Lanzone, M., Blackshire, S., et al. (2017). Improved supervised classification of accelerometry data to distinguish behaviors of soaring birds. PLoS ONE 12:e0174785. doi: 10.1371/journal.pone.0174785
Thums, M., Fernández-Gracia, J., Sequeira, A. M. M., Eguíluz, V. M., Duarte, C. M., and Meekan, M. G. (2018). How big data fast tracked human mobility research and the lessons for animal movement ecology. Front. Mar. Sci. 5:21. doi: 10.3389/fmars.2018.00021
Tomkiewicz, S. M., Fuller, M. R., Kie, J. G., and Bates, K. K. (2010). Global positioning system and associated technologies in animal behaviour and ecological research. Philos. Trans. R. Soc. B Biol. Sci. 365, 2163–2176.
Treep, J., Bohrer, G., Shamoun-Baranes, J., Duriez, O., de Moraes Frasssson, R. P., and Bouten, W. (2016). Using high-resolution GPS tracking data of bird flight for meteorological observations. Bull. Am. Meteorol. Soc. 2016, 951–961. doi: 10.1175/BAMS-D-14-00234.1
Urios, V., Romero, M., and Mellone, U. (2015). The Use of Satellite Telemetry for the Study of the Movement Ecology of Raptors. Alicante: Publications University of Alicante.
van der Hoek, Y., Gaona, G. V., and Martin, K. (2017). The diversity, distribution and conservation status of the tree-cavity-nesting birds of the world. Divers. Distrib. 23, 1120–1131. doi: 10.1111/ddi.12601
Wall, J., Wittemyer, G., Klinkenberg, B., and Douglas-Hamilton, I. (2014). Novel opportunities for wildlife conservation and research with real-time monitoring. Ecol. App. 24, 593–601. doi: 10.1890/13-1971.1
Weinzierl, R., Bohrer, G., Kranstauber, B., Fieldler, W., Wikelski, M., and Flack, A. (2016). Wind estimation based on thermal soaring of birds. Ecol. Evol. 6, 8706–8718. doi: 10.1002/ece3.2585
Williams, H. J., Shepard, E. L. C., Duriez, O., and Lambertucci, S. A. (2015). Can accelerometry be used to distinguish between flight types in soaring birds? Anim. Biotelem. 3:45. doi: 10.1186/s40317-015-0077-0
Williams, H. J., Taylor, L. A., Benhamou, S., Bijleveld, A. I., Clay, T. A., de Grissac, S., et al. (2020). Optimising the use of bio-loggers for movement ecology research. J. Anim. Ecol. 89, 186–206. doi: 10.1111/1365-2656.13094.
Wilson, A. D. M., Wikelski, M., Wilson, R. P., and Cooke, S. J. (2015). Utility of biological sensor tags in animal conservation. Cons. Biol. 29, 1065–1075. doi: 10.1111/cobi.12486
Wilson, R. P., White, C. R., Quintana, F., Halsey, L. G., Liebsch, N., Martin, G. R., et al. (2006). Moving towards acceleration for estimates of activity-specific metabolic rate in free-living animals: the case of the cormorant. J. Anim. Ecol. 75, 1081–1090. doi: 10.1111/j.1365-2656.2006.01127.x
Wilson, R. P., Wilson, M.-P. T., Link, R., Mempel, H., and Adams, N. J. (1991). Determination of movements of African penguins Spheniscus demersus using a compass system: dead reckoning may be an alternative to telemetry. J. of Exper. Biol. 157, 557–564.
Keywords: animal movement, biologging, conservation biology, data paucity, error compounding, radio telemetry, scale mismatch, wildlife management
Citation: Katzner TE and Arlettaz R (2020) Evaluating Contributions of Recent Tracking-Based Animal Movement Ecology to Conservation Management. Front. Ecol. Evol. 7:519. doi: 10.3389/fevo.2019.00519
Received: 02 October 2019; Accepted: 20 December 2019;
Published: 24 January 2020.
Edited by:
Deseada Parejo, University of Extremadura, SpainReviewed by:
Andrew Mark Allen, Radboud University Nijmegen, NetherlandsVicente Urios, University of Alicante, Spain
At least a portion of this work is authored by Todd E. Katzner on behalf of the U.S. Government and, as regards Dr. Katzner and the U.S. Government, is not subject to copyright protection in the United States. Foreign and other copyrights may apply. This is an open-access article distributed under the terms of the Creative Commons Attribution License (CC BY). The use, distribution or reproduction in other forums is permitted, provided the original author(s) and the copyright owner(s) are credited and that the original publication in this journal is cited, in accordance with accepted academic practice. No use, distribution or reproduction is permitted which does not comply with these terms.
*Correspondence: Todd E. Katzner, tkatzner@usgs.gov; Raphaël Arlettaz, raphael.arlettaz@iee.unibe.ch