- 1Department of Biology, Indiana University, Bloomington, IN, United States
- 2Bren School of Environmental Science and Management, University of California, Santa Barbara, Santa Barbara, CA, United States
- 3National Institute for Mathematical and Biological Synthesis, University of Tennessee, Knoxville, Knoxville, TN, United States
Plant-soil feedback (PSF), the process by which plants influence con- or heterospecifics via alteration of abiotic or biotic soil properties, is a known driver of plant coexistence and invasion. Yet there is limited understanding of how PSF interacts with other important drivers of plant community structure and dynamics, such as aboveground herbivory. Aboveground herbivory and PSFs are ubiquitous processes in plant communities, but traditional PSF experiments in the greenhouse eliminate herbivory as an experimental factor. Aboveground herbivory can affect plant-soil systems in multiple ways and therefore is likely to strongly interact with PSF. Herbivores can be selective, preferring certain species over others, which could influence PSF dynamics. Aboveground herbivory could also affect PSF dynamics by influencing photosynthate allocation, defense compound production, and soil nutrient levels. An existing conceptual framework predicts that aboveground herbivory should generally weaken pathogen-, mutualist- and soil nutrient-driven feedbacks, and a logical extension of these predictions is that aboveground herbivory will weaken PSF as a driver of plant species invasion. Using a Midwest urban woodland study system, we first measured aboveground mammalian herbivore pressure on native woodland perennials used in local restoration efforts. We then simulated these levels of herbivory in a greenhouse experiment to assess whether and how aboveground herbivory alters net pairwise PSF interactions between these native species and Euonymus fortunei, a common invasive plant of Midwest urban woodlands. Results support predictions that aboveground herbivory weakens PSF interactions. In our experiment, simulated herbivory eliminated PSF among E. fortunei and a co-occurring community of native species, although this effect depended on competitive context. When the native community and the invasive species were grown separately, net feedback was neutral regardless of herbivory, but when grown in a competitive mixture, feedback between the native community and the invader switched from negative to neutral when herbivory was imposed. To assess the generality of these findings, future studies are needed that examine herbivory-PSF interactions across multiple native-native and native-invasive species combinations, and for a range of plant community types.
Introduction
While decades of research have established that plant-soil feedbacks (PSFs) can be a key force structuring plant communities and plant invasions (Bever et al., 1997; Klironomos, 2002; Kulmatiski et al., 2008), understanding of the context-dependency of PSFs, i.e., how PSFs change under different environmental conditions—is only beginning to be appreciated (Smith-Ramesh and Reynolds, 2017). The presence or absence of aboveground herbivory as a potentially strong influence on PSFs is our focus here. Plant-soil feedback refers to the process wherein plants affect biotic and/or abiotic conditions of the soil in which they are grown (a process commonly referred to as “soil conditioning”), which influences subsequent growth of con- or heterospecific plants (Bever et al., 1997). Plants affect biotic soil properties through various mechanisms, including root exudates, deposition patterns, and susceptibility to belowground enemies, ultimately generating species-specific microbial associations with roots and/or rhizospheres (Ehrenfeld et al., 2005; Kulmatiski et al., 2008). These associations consist of various symbionts like mutualists and pathogens (Bever et al., 1997; Packer and Clay, 2000; Reynolds et al., 2003; Ehrenfeld et al., 2005). Plants also directly and indirectly affect abiotic soil properties such as nutrient and water availability, pH and allelochemicals through uptake, exudates, and litter inputs (Bertin et al., 2003; Elgersma et al., 2012; Bardgett et al., 2014).
Plant-soil feedbacks can be positive, negative, or neutral. Individual feedbacks refer to plant performance in conspecific vs. heterospecific soil, and net pairwise feedbacks refer to plant performance in conspecific and heterospecific soil relative to that of another species (Smith-Ramesh and Reynolds, 2017). Net pairwise feedbacks are emphasized here because they predict the effects of PSFs on plant community dynamics (Smith-Ramesh and Reynolds, 2017). Net negative PSF, wherein plant species perform relatively better in soil conditioned by heterospecific vs. conspecifics, is a stabilizing mechanism that maintains species diversity (Bever et al., 1997). Net negative PSFs stem from host-specific enemy accumulation in many systems (Packer and Clay, 2000; Reynolds et al., 2003) but could also be driven by uptake of soil resources by niche-partitioned species (Smith-Ramesh and Reynolds, 2017). Net positive PSF, wherein plant species perform relatively better in soil conditioned by conspecifics vs. heterospecifics, is a destabilizing mechanism that can ultimately lead to increased abundance of a particular species or even competitive exclusion of other species (Bever et al., 1997), and may contribute to invasive species spread (Jordan et al., 2008; Zhang et al., 2010; Smith and Reynolds, 2012). Plants that form soil mutualisms could generate positive PSFs (Bever et al., 1997, 2010). Neutral feedbacks occur when plant performance is unaffected by soil conditioning and may indicate that PSFs do not structure plant community dynamics. Neutral PSFs could also promote invasion if native-invasive PSFs are neutral or less negative than native-native PSFs, due for example to complete or partial escape of invasive species from belowground enemies (Smith and Reynolds, 2015).
An existing conceptual framework predicts that aboveground herbivory should generally weaken PSF (Smith-Ramesh and Reynolds, 2017). Aboveground herbivory may weaken pathogen-driven negative PSF through a cross-induction of belowground defenses (Bezemer and van Dam, 2005; Kaplan et al., 2008). (Although if herbivory weakens plant condition and thus increases susceptibility to soil pathogens, it is possible that the strength of negative PSF could increase; Smith-Ramesh and Reynolds, 2017). Aboveground herbivory may weaken nutrient-driven negative PSF by reducing plant size and/or via nutrient inputs (e.g., excreta), either of which could reduce plant nutrient demands (Smith-Ramesh and Reynolds, 2017). Heavy or long-term aboveground herbivory may reduce the ability of plants to supply carbon subsidies to microbial mutualists (Bardgett et al., 1998), which could in turn weaken mutualist-driven PSF [In contrast, intermediate, or short-term herbivory could enhance below-ground resource allocation (Bardgett et al., 1998), strengthening mutualist-driven PSF]. A logical extension of these predictions is that aboveground herbivory will generally weaken PSF as a driver of plant species invasion.
Relatively few studies have examined herbivory in the context of PSFs. Several studies on herbivory and PSFs have investigated the effects of PSFs on herbivores, specifically herbivore growth and performance (Kos et al., 2015a,b; Heinen et al., 2017). However, research is needed to assess the effects of herbivory on PSFs. One study examined the effects of moth and beetle herbivory on PSF interactions with Jacobia vulgaris, finding that belowground (beetle) herbivory weakened negative PSFs (measured with respect to unconditioned soil) whereas aboveground (moth) herbivory strengthened negative PSFs (Bezemer et al., 2013). Another study examined the effects of aboveground insect herbivory on individual PSF in three native grasses and found that herbivory neutralized negative PSFs (Heinze and Joshi, 2017). The nature of herbivory-PSF interactions in other systems, and for native-invasive dynamics, is unknown.
Urban woodlands are highly disturbed systems that pose a unique opportunity to study herbivory-PSF interactions with native and invasive species. Urban woodlands are typically small, habitat fragments, with high edge to surface area ratios, close proximity to conventionally landscaped properties, and high populations of urban-adapted mammals such as rabbits and deer (Bauer and Reynolds, 2016). These conditions promote high levels of both plant invasions and herbivore pressure. Furthermore, in an instance of the general phenomena of enemy escape (Keane and Crawley, 2002; Shea and Chesson, 2002; Mitchell and Power, 2003), invasive woodland plant species may be consumed to a lesser extent by mammalian herbivores than are native woodland species (Knight et al., 2009; Relva et al., 2010; Averill et al., 2016). Concern for biodiversity loss and appreciation of ecosystem services has motivated efforts to restore urban ecosystems (DiCicco, 2014; Elmqvist et al., 2015). Besides advancing basic science understanding of context-dependency in PSF, study of how mammalian grazing pressure interacts with native-invasive PSF dynamics in urban woodlands may contribute to more successful restoration efforts.
We used field exclosure experiments to assess the intensity of mammalian herbivore pressure on seven native Midwest U.S. woodland perennials commonly used in urban woodland restorations in Bloomington, IN, U.S. We then simulated these levels of mammalian herbivory in a full reciprocal PSF greenhouse study to determine their effect on net pairwise PSF dynamics amongst a community of three native woodland perennials and Euonymus fortunei, a common perennial invasive species in Midwest U.S. urban woodlands (Smith and Reynolds, 2015). Based on common findings of weakened negative or positive PSF for introduced species (Klironomos, 2002; Callaway et al., 2004) and our previous findings (Smith and Reynolds, 2012, 2015), we predicted that PSFs would be neutral to positive favoring the invader in the absence of herbivory. Furthermore, consistent with conceptual predictions (Smith-Ramesh and Reynolds, 2017), we also expected that PSF dynamics between native and invasive species would weaken in the presence of herbivory.
Materials and Methods
Herbivore Pressure Experiment
Aboveground herbivore pressure from mammals such as deer and rabbits was assessed in two predominantly beech-maple urban woodlands in Bloomington, IN, U.S.: Dunn's Woods on Indiana University's campus and Latimer Woods, a municipal woodland preserve. Both of these woodlands are a focus of invasive species mitigation and native species restoration efforts by the Bloomington Urban Woodlands Project, a consortium of local non-profit, city government and Indiana University partners (https://sustain.iu.edu/buwp.html). Three separate exclosure experiments were conducted to assess herbivore pressure on transplanted native plants in these woodlands. In each of these experiments, native plants were transplanted into closed or open cages or uncaged control plots established in eight (Dunn's Woods) or 10 blocks (Latimer Woods) randomly located throughout the woodlands in areas without invasive plants. As an index of herbivore pressure, aboveground biomass of plugs after 12–16 months was measured.
Native plants for all experiments were propagated under natural light in a temperature-controlled greenhouse at Indiana University, from Indiana-genotype seed purchased from Spence Restoration Nursery (Muncie, IN, U.S.). Seeds were germinated in 10 cm × 10 cm flats filled with MetroMix 360 (Sun Gro Horticulture, Agawam, MA, U.S.). Once large enough to handle without breaking (~4 weeks), seedlings were transplanted to stubby cone-tainers (Steuwe & Sons, Corvallis, OR, U.S.) filled with additional MetroMix and grown to the mature plug stage (~2–3 months), wherein roots fully filled the cone-tainer. Seven perennials native to central Indiana, U.S. deciduous woodlands were used across the three experiments. Experiment one, conducted in Dunn's Woods from May 2013-September 2014, involved Solidago flexicaulis (zig-zag goldenrod), Elymus hystrix (bottle-brush grass) and Aster cordifolius (heart-leaved aster). Experiment two, conducted in Dunn's Woods from October 2013-September 2014, involved Aster lateriflorus (calico aster), Carex normalis (spreading oval sedge) and Conoclinum coelestinum (blue mistflower). Experiment three, conducted in Latimer Woods from October 2013-September 2014, involved Lobelia siphilitica (great blue lobelia) Elymus hystrix (bottlebrush grass) and Conoclinum coelestinum (blue mistflower).
Herbivore exclosures were 40-cm diameter, 1-m tall cylindrical cages constructed from hardware cloth (Midwest Air Tech Import, Grandview, MO, U.S.) fastened with cable ties (Gardner Bender, Milwaukee, WI, U.S.). Closed cages did not have openings and were not accessible to mammalian herbivores such as deer and rabbits. Open cages had two entrances (18 cm × 15 cm) opposite one another at the bottom of each cage and were accessible to small mammals such as rabbits. Open cages served as cage controls and also enabled the effects of small mammal vs. deer herbivory to be distinguished. Closed and open cages were secured to the forest floor with four ground staples (Easy Gardner Inc., Waco, TX). Uncaged plots (40 cm × 40 cm) were marked with four 30 cm bamboo stakes and were accessible to all herbivores. Within each block, caged and control areas were spaced approximately 1 m apart from one another. Mature plugs were removed from cone-tainers and transplanted into caged and uncaged plots using a dibble sized for the cone-tainer volume (Steuwe & Sons, Corvallis, OR, U.S.). Three plugs of each of three species were transplanted into each caged or uncaged plot, for a total of nine plugs per plot. Plugs were planted 5 cm apart in a 3 × 3 array, and plug locations were randomized.
Herbivory-PSF Experiment
Propagation of Study Species and Greenhouse Conditions
We focused on examining herbivory-PSF interactions amongst Euonymus and the three native perennial woodland species, Solidago flexicaulis, Elymus hystrix, and Aster cordifolius, shown to be most vulnerable to herbivory in our herbivore pressure experiment (see Results). Plants were germinated, propagated and grown in a temperature-controlled greenhouse at Indiana University. Supplemental light was provided by 1,000-watt high pressure sodium lights set to a summer photoperiod of 15 h light, 9 h dark. Native seeds were purchased from Spence Restoration Nursery (Muncie, IN) and Euonymus cuttings (one-node shoot tips) were collected from three urban woodland sites where soil was obtained (described below in Phase I—soil conditioning). Seeds and Euonymus cuttings were surface sterilized with 10% bleach. Likewise, all containers used to grow plants, as well as greenhouse bench surfaces, were surface-sterilized with 0.52% Physan 2.0 (Maril Products, Inc., Tustin, CA). Seeds were cold stratified for 4 weeks in moist fine sand that had been autoclaved twice at 120°C, 24 h apart, 2 h intervals then germinated in 10 × 10 cm flats filled with MetroMix 360 (Sun Gro Horticulture, Agawam, MA; autoclaved twice at 120°C, 24 h apart, 2 h intervals). Four-week old seedlings and Euonymus cuttings were rooted in 5 cm deep, 128-cell trays (Hummert International, St. Joseph, MO) filled with a 50/50 mix of river-washed sand (Rogers Group Inc., Martinsville, IN) and Indiana topsoil (sourced from Bloomington, IN) that had been autoclaved twice (120°C, 24 h apart, for 2 h intervals). Seedlings and cuttings grew in cell trays for a 4 week period.
Phase I—Soil Conditioning
For phase I pots, live soil was collected June 20th through June 22nd, 2017 from Latimer Woods and two other urban, predominantly beech-maple woodlands in Bloomington, Indiana: a woodland on Indiana University's campus near Ballantine Hall and Wapehani Woods, a municipal woodland preserve. At each site, a spade-tipped shovel was used to collect soil to a 21.5 cm depth in equal amounts from areas that were uninvaded and areas invaded by Euonymus. Soil from uninvaded and invaded areas was combined and thoroughly mixed by passing it through a 6 mm sieve. Mixed soil was stored in 5-gallon plastic tubs with lids. One plastic tub was collected from each site per day and used to establish a block of 12 experimental pots (four pots for each of the three sites), such that one block was established per day for 3 consecutive days. Soil was not mixed between sites to allow for site-specific effects to be observed. Shovels, sieves, tubs, gloves, and all other materials were washed with soap and sprayed with 70% ethanol in between sites to prevent cross contamination.
Phase I vegetation treatments were established in 16-cm diameter, 18-cm deep pots (Dillen Products Inc., Middlefield, OH) that had been surface-sterilized as described above and bottom-lined with 10-cm squares of clean newspaper. The native community treatment consisted of one individual of each species planted equidistant in each pot, and the Euonymus vegetation treatment consisted of three equidistant Euonymus cuttings per pot. To guard against cross contamination, pots were spaced at least 50 cm apart on greenhouse benches. After being planted, all pots were given an acclimation period of 2 months (June-August 2017) before herbivory treatments were assigned. Plants of each vegetation treatment were assigned to an herbivory treatment group, for which plants were clipped with scissors to simulate mammalian herbivory, and an unclipped control group (Figure 1). Scissors were washed with soap and rinsed with water between pots to prevent cross contamination. In the herbivore pressure experiment, we observed that plugs in open cages or uncaged controls were heavily browsed (see Results, Figure 2A). To simulate this strong degree of herbivory, plants were cut twice weekly to maintain a height of 5 cm for 3 months (September-November 2017) prior to collection of phase I soil. Each block consisted of one pot for each vegetation and herbivory group and soil site (12 pots per block). In total there were nine replicates for each vegetation and herbivory group for a total of thirty-six phase I pots. Pots were randomized within each of the three blocks.
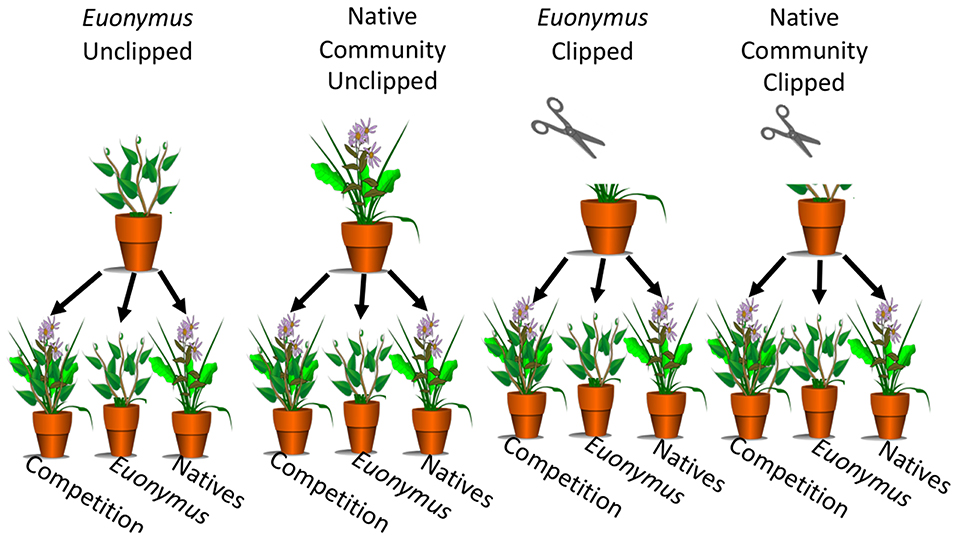
Figure 1. Phase I vegetation groups included natives and Euonymus. Herbivory groups included unclipped controls (left) and simulated herbivory by clipping (right). In phase II, conditioned soil was used to grow the native community, monocultures of Euonymus, and a competition treatment with native community-Euonymus mixtures.
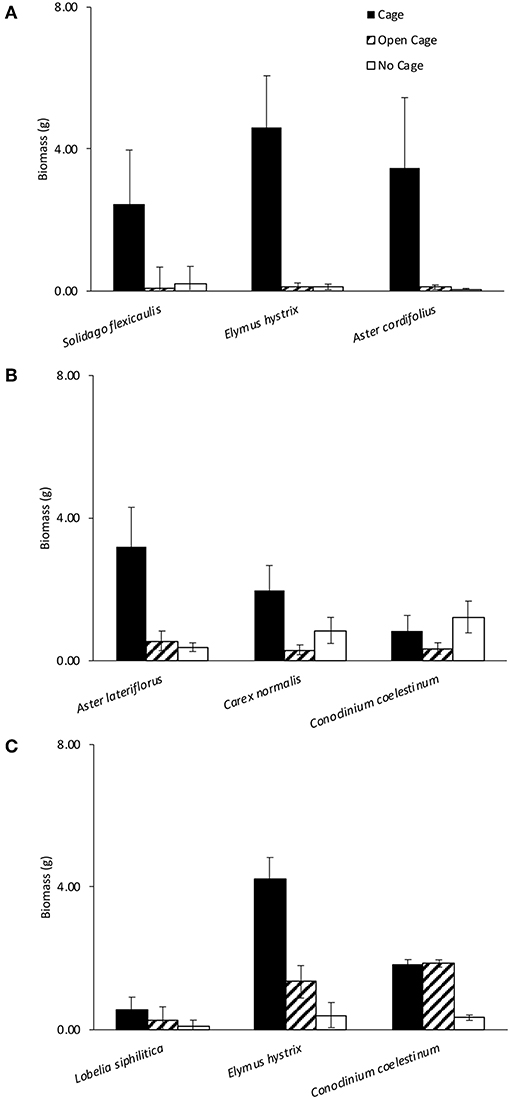
Figure 2. Cage treatments had a significant effect on biomass at Dunn's (A,B) and Latimer (C) woods. Bars show +/– 1 SE.
Phase II—Feedback Response
A net pairwise design was implemented where vegetation treatments were grown in conspecific and heterospecific soil (Figure 1). Aboveground phase I plants were clipped, placed in paper bags, and dried at 60°C for 72 h. Belowground biomass was sieved from soil, placed in Ziplock bags, then washed, blotted dry on paper towels, placed in paper bags, and dried at 60°C for 72 h. Dried above- and belowground biomass was weighed for inclusion as covariates in phase II analyses. Phase I soil from each pot was passed through a 4 mm sieve into a clean plastic tub and stored in new Ziplock bag in a refrigerator for <12 h. Equipment was washed between pots and all pots and greenhouse benches were surface-sterilized as described in phase I. Once an entire block was sieved, soil from each phase I pot was divided into three 950 mL pots lined with 10 cm squares of clean newspaper. Each set of three pots was then planted with one of three vegetation groups: a native community with one new seedling of each of the native species used in phase I, an invasive monoculture of three Euonymus cuttings, and a native-Euonymus additive design mixture of each of the three native seedlings and three E. fortunei cuttings (Figure 1). An additive design was used to best represent the process of plant invasion and native-invasive competition. Initial data including height, longest leaf length, and number of leaves was obtained from seedlings within 24 h after transplanting, for inclusion as covariates in Phase II analyses. Pots were randomized within each of nine blocks in the greenhouse. After a 3 month growth period (December 2017 to February 2018), above- and belowground biomass of phase II plants were harvested, dried and weighed following the same protocols as for phase I plants.
Statistical Analyses
To assess the degree of herbivore pressure that native woodland perennials experienced, we used nlme in R Core Development Team (2017, v3.4) to construct a mixed effects linear model for each experiment. with aboveground biomass as the response variable, exclosure treatment (cage, open cage, no cage), species identity, and their interaction as fixed effects, and block as a random effect. Based on graphical diagnostics of residuals, aboveground biomass was log-transformed [ln(x+min/2)] to meet normality and homogeneity of variance assumptions. Because there was no significant interaction between plant species identity and exclosure treatment, the interaction was dropped from the models. A priori contrasts were performed using R's contrast package to assess differences between exclosure treatments for each experiment.
In order to determine how herbivory influenced plant-soil feedback under different competitive conditions, we used PROC-MIXED in SAS Institute (2017, v9.4m5) to construct mixed-effects linear models. Given that our interest was mainly in examining herbivory × PSF interactions and that we had limited power to run a four-way model, we chose to run two separate three-way models, one for phase II plants grown in non-competitive mixtures and one for phase II plants grown in competitive mixtures. Phase II biomass was used as the response variable and herbivory, phase I vegetation group (i.e., conditioned soil type), and phase II vegetation group were included as fixed effects with all possible interactions. Phase II seedling size measurements (height, longest leaf length, and number of leaves) and phase I biomass (above- and belowground) were used as covariates. Soil collection sites (Ballantine, Latimer, Wapehani) and block were random effects. Graphical diagnostics of residuals were used to test for normality and heterogeneity of variance. No transformations were needed to meet model assumptions.
Plant-soil feedback is reflected in the interaction between phase I and phase II vegetation group, with non-neutral PSF indicated where the interaction is significant. A significant three-way interaction between herbivory, phase I vegetation group, and phase II vegetation group indicates that PSF was significantly different in the simulated herbivory treatment compared to the no-herbivory treatment. A priori contrasts were used to estimate net pairwise feedback (sensu Mangan et al., 2010; Smith and Reynolds, 2015), reflected as the interaction coefficient (Is) developed by Bever et al. (1997):
where AA represents species A growth in soil conditioned by species A and AB refers to species A growth in soil conditioned by species B. Likewise, BB refers to species B growth in conspecific soil and BA refers to species B growth in heterospecific soil.
Results
For each of the three herbivore pressure experiments, the mixed effects linear models revealed a significant effect of exclosure treatment and a priori contrasts indicated significant pairwise differences amongst exclosure treatments (Table 1). Although the exclosure × plant species identity interaction was dropped from all models due to non-significance, the effect of exclosure treatment was most consistent across species for Dunn's Woods experiment one, where on average, aboveground biomass of Solidago flexicaulis, Elmus hystrix, and Aster cordifolius was over 95% greater in closed cages compared to open cages or no-cage controls (Figure 2A, Table 1). Similar trends were evident for Aster laterifolius and Carex normalis, but not Conoclinum coelestinum in Dunn's Woods experiment two (Figure 2B) and for Elymus hystrix, but not Lobelia siphilitica or Conoclinum coelestinum in experiment three at Latimer Woods (Figure 2C), although the data were considerably more variable across species and a priori contrasts did not detect a significant difference between closed cages and no-cage controls in experiment two (Table 1).
In the herbivory × PSF experiment, when native communities and Euonymus were grown separately (non-competitive conditions), there was no significant two-way interaction between phase I and phase II vegetation type (i.e., PSF, aka “neutral PSF”) and no significant three-way interaction between phase I vegetation group, phase II response group and herbivory group in the mixed effects GLM (i.e., no effect of herbivory on PSF, Table 2). Consistent with these non-significant interactions, mean interaction coefficients were not significantly different from zero regardless of herbivory treatment (Figure 3). There was a significant main effect of phase I vegetation group and a significant two-way interaction between herbivory group and phase I vegetation group (Table 2).
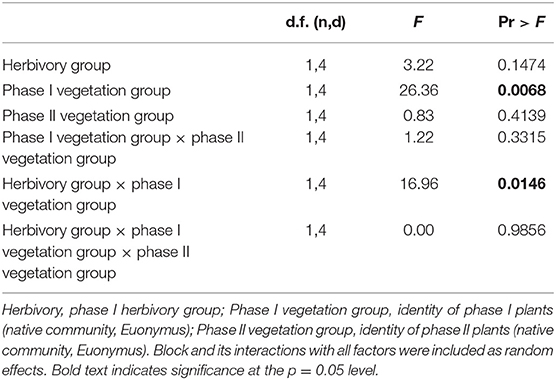
Table 2. Results of mixed effects linear model for phase II plants grown in non-competitive conditions.
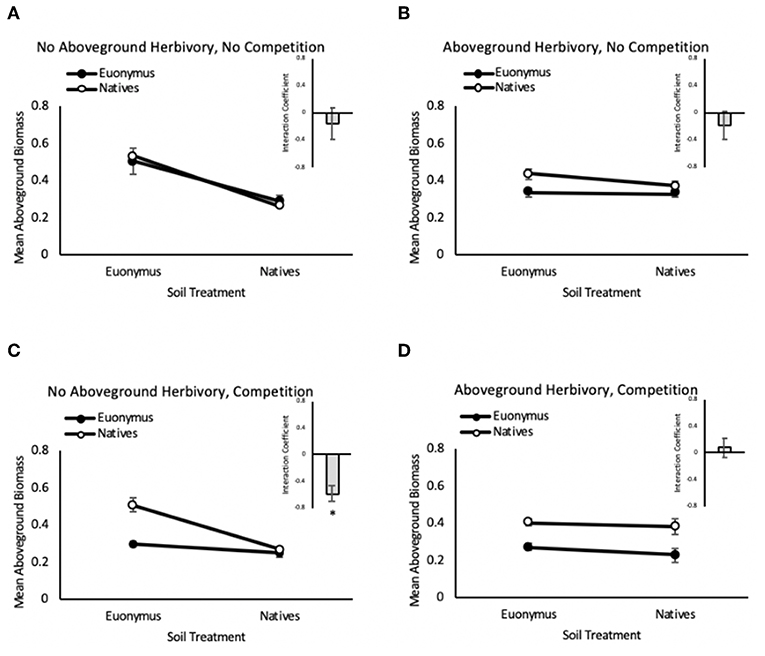
Figure 3. Feedback between Euonymus (closed circles) and the native community (open circles) in the no aboveground herbivory, no competition (A), no aboveground herbivory, no competition (B), aboveground herbivory, competition (C), and herbivory, competition (D) groups. Insets are mean interaction coefficients for each group. Bars show +/– 1 SE.
In contrast, when native communities and Euonymus were grown together (competitive conditions), the mixed effects GLM revealed a significant three-way interaction for herbivory group × phase I vegetation group × phase II vegetation group (Figure 3, Table 3). This indicates that PSF was significantly different in the simulated herbivory treatment compared to the no-herbivory treatment. Mean interaction coefficients reveal the nature of this shift; net pairwise PSF was neutral (0.081 ± 0.146, p = 0.635) under conditions of simulated herbivory and negative (−0.580 ± 0.130, p = 0.047) in the absence of herbivory. There were also significant main effects of phase I and phase II vegetation group and a significant two-way interaction for herbivory × phase I vegetation group (Table 3). In the absence of herbivory growth of both the native community and Euonymus was higher in soil conditioned by Euonymus, but this effect disappeared in the presence of herbivory.
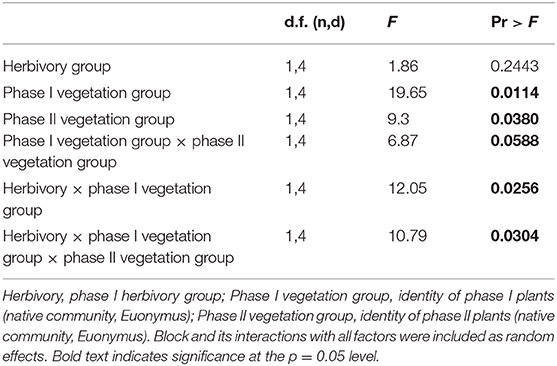
Table 3. Results of mixed effects general linear model for phase II plants grown in competitive conditions.
Discussion
Our results demonstrate that herbivore pressure on native understory plants can be intense in urban woodlands, and this can weaken net pairwise PSFs between native and invasive species, which is consistent with our original predictions. This is the first report of interactive herbivory × PSF effects in woodlands and for native and invasive species. Other findings support herbivory (from insects) as a factor that reduces the importance of PSF as a driver of plant community dynamics among native grassland species (Heinze and Joshi, 2017). There are several mechanisms that could explain aboveground herbivory weakening negative PSFs, including increased root growth (Bardgett et al., 1998) leading to an increase in soil mutualisms (Smith-Ramesh and Reynolds, 2017), a cross-induction of belowground defenses (Bezemer and van Dam, 2005; Kaplan et al., 2008), and a reduction in plant size and nutrient demands (Smith-Ramesh and Reynolds, 2017). Comparing microbial communities, soil defense compounds, and nutrient concentrations in phase I soils may yield insights into which mechanism operates. An important factor to consider is that our experiment did not pose an aboveground enemy escape scenario for Euonymus, which would be expected in nature even for more palatable invasive plant species (at least in the case of host-specific and/or specialist herbivores). In an aboveground enemy escape scenario, the native community would experience aboveground herbivory and any of its knock on effects to soil variables, while the invasive species would not. Depending on whether and how aboveground herbivory on the native community influenced soil microbial communities, defense compounds, and/or nutrient concentrations associated with native plants, net pairwise PSF among native and invasive plant species could range from negative to positive.
We observed net negative pairwise PSF dynamics for competitive mixtures of the native community grown with the invader Euonymus in the absence of herbivory. This result is inconsistent with general expectations from the literature (Kulmatiski et al., 2008) and with previous studies examining PSF dynamics between native woodland species and Euonymus, which have found net neutral to positive feedback (Smith and Reynolds, 2012, 2015). Something overlooked, however, is that while neutral or positive PSF among native and invasive species is expected if either enhanced mutualisms or novel weapons are the driver of invasion (Figures 4A,B), negative PSF among native and invasive species is the expectation if belowground enemy escape is the main driver of invasion (Figure 4C). Our findings might therefore be explained if belowground enemy escape was operating for Euonymus under the conditions of our experiment.
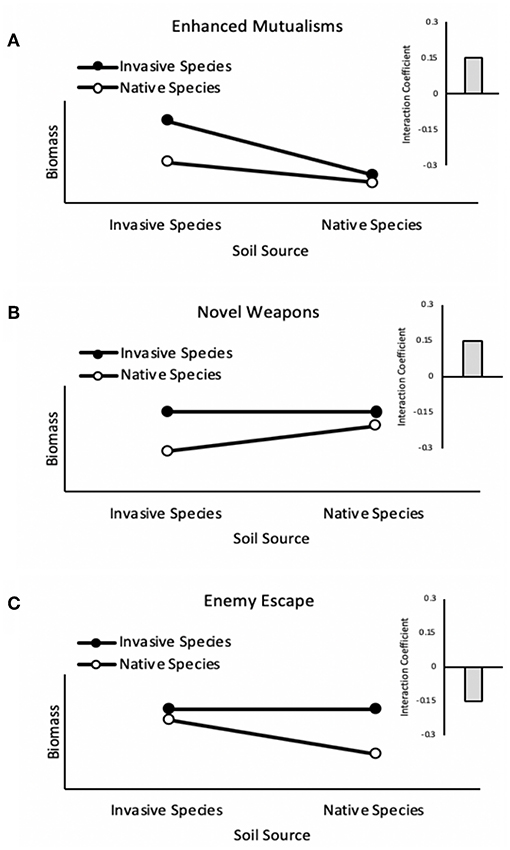
Figure 4. Plant-soil feedback dynamics for enhanced mutualisms, novel weapons, and enemy escape hypotheses. If an invasive species exhibits enhanced mutualisms (A), growth of the invasive species could be sufficiently greater in conspecific soil relative to heterospecific soil (positive individual feedback) to dominate the outcome of net pairwise feedback. An invader that exhibits novel weapons (B) would presumably be adapted to its own weapons and thus exhibit neutral individual feedback, whereas the invader's novel weapons would hinder native species growth in heterospecific soil, resulting in positive individual feedback for the native. Net pairwise feedback would therefore also be positive under the novel weapons hypothesis. In contrast, if an invasive species exhibits belowground enemy escape (C), its growth would not be expected to be affected by soil conditioning (neutral individual feedback) whereas native species would exhibit negative individual feedback from host specific enemies, resulting in negative net pairwise feedback.
Interestingly, our results also indicate that soil conditioned by Euonymus may have a general promoting effect on plant growth, although this effect disappeared in the presence of herbivory. While this finding that Euonymus-conditioned soil promoted greater phase II plant growth than native-conditioned soil may at first seem surprising, it could simply reflect greater soil resource drawdown by the three-species native community, perhaps due to niche complementarity of niche-partitioned species, ultimately reducing nutrient availability for phase II plants. The fact that this effect was lost in the herbivory treatment may be expected given that biomass—and presumably soil resource demand—of all species was kept consistently low by the simulated herbivory. Further studies are needed to confirm whether Euonymus has a promoting effect and the mechanisms by which this might occur.
Plant-soil feedbacks tend to be weaker in field studies compared to greenhouse experiments (Kulmatiski et al., 2008) and our results suggest that herbivore pressure in the field may contribute to such weakened PSF. Still, several limitations to our study may have prevented the magnitude of PSFs observed in our results from accurately reflecting field conditions. Cutting aboveground plant tissues with scissors may have a different effect on plant defense compounds or may damage plant tissues differently than an herbivore. In natural systems, herbivores may trample herbaceous vegetation and release waste products, both of which could potentially affect soil conditions (Bardgett and Wardle, 2003; Schrama et al., 2013) and consequently PSFs. Field PSF experiments that manipulate natural herbivores are therefore needed. While we used an additive design to best simulate the invasion process, results may have been different with a substitutive design (which holds total plant density constant).
Further research is also needed to confirm whether herbivory-PSF interactions affect invasion success. For example, future studies could examine Euonymus-native PSFs in the presence and absence of herbivory and quantify Euonymus invasion success in each treatment. Additionally, future studies should assess whether herbivore type (e.g., insect, mammalian) differentially affect PSFs. Herbivory-PSF interactions across a gradient of lower to higher herbivore pressure should also be examined. Exploring herbivory-PSF interactions among other native-native and native-invasive combinations, including palatable vs. unpalatable invasive species, and across different habitat types is also needed to assess the generalizability of our findings.
Conclusions
Our results suggest that herbivore pressure can be intense in urban woodlands and that such herbivore pressure can reduce the strength of PSFs, affecting species interactions with an invader and potentially influencing invasion success. Thus, plant-soil feedbacks may be less important in driving plant community dynamics in systems with high herbivore pressure. Our results also suggest that Euonymus' invasion success may in part be attributed to belowground enemy escape, although research to confirm this mechanism of feedback is required. Further research is needed to determine whether herbivory-PSF interactions affect invasion success, identify the mechanisms of herbivory-PSF interactions, and to assess the generality of our results under more complex and varied field conditions.
Data Availability Statement
The datasets generated for this study are available on request to the corresponding author.
Author Contributions
HR, CH, and RA designed and executed the herbivore pressure experiments. HR and SB designed and SB executed the PSF × herbivory experiment. LS-R and SB did the statistical analyses. SB and HR wrote the manuscript, with input from LS-R. All authors contributed to the development of ideas.
Funding
This work was supported by Indiana University Biology funds awarded to HR. LS-R was supported by a NIMBioS postdoctoral fellowship.
Conflict of Interest
The authors declare that the research was conducted in the absence of any commercial or financial relationships that could be construed as a potential conflict of interest.
Acknowledgments
We thank reviewers for comments that improved the manuscript. We thank the Indiana University greenhouse staff for assistance with experimental setup and maintenance and the City of Bloomington Parks and Recreation Department for allowing us to collect soil on their properties.
References
Averill, K. M., Mortensen, D. A., Smithwick, E. A. H., and Post, E. (2016). Deer feeding selectively for invasive plants. Biol. Invasions. 18, 1247–1263. doi: 10.1007/s10530-016-1063-z
Bardgett, R. D., Mommer, L., and De Vries, F. T. (2014). Going underground: root traits as drivers of ecosystem processes. Trends Ecol. Evol. 29, 692–699. doi: 10.1016/j.tree.2014.10.006
Bardgett, R. D., and Wardle, D. A. (2003). Herbivore-mediated linkages between aboveground and belowground communities. Ecology 84, 2258–2268. doi: 10.1890/02-0274
Bardgett, R. D., Wardle, D. A., and Yeates, G. W. (1998). Linking above-ground and below-ground interactions: how plant responses to foliar herbivory influence soil organisms. Soil Biol. Biochem. 30, 1867–1878. doi: 10.1016/S0038-0717(98)00069-8
Bauer, J. T., and Reynolds, H. L. (2016). Restoring native understory to a woodland invaded by Euonymus fortunei: multiple factors affect success. Restor. Ecol. 24, 45–52. doi: 10.1111/rec.12285
Bertin, C., Yang, X., and Weston, L. A. (2003). The role of root exudates and allelochemicals in the rhizosphere. Plant Soil 256, 67–83. doi: 10.1023/A:1026290508166
Bever, J. D., Dickie, I. A., Facelli, E., Facelli, J. M., Klironomos, J., Moora, M., et al. (2010). Rooting theories of plant community ecology in microbial interactions. Trends Ecol. Evol. 25, 468–478. doi: 10.1016/j.tree.2010.05.004
Bever, J. D., Westover, K. M., and Antonovics, J. (1997). Incorporating the soil community into plant population dynamics: the utility of the feedback approach. J. Ecol. 85, 561–573. doi: 10.2307/2960528
Bezemer, T. M., and van Dam, N. M. (2005). Linking aboveground and below-ground interactions via induced plant defenses. Trends Ecol. Evol. 20, 617–624. doi: 10.1016/j.tree.2005.08.006
Bezemer, T. M., van der Putten, W. H., Martens, H., van de Voorde, T. F. J., Mulder, P. P. J., and Kostenko, O. (2013). Above- and below-ground herbivory effects on below- ground plant-fungus interactions and plant-soil feedback responses. J. Ecol. 101, 325–333. doi: 10.1111/1365-2745.12045
Callaway, R. M., Thelen, G. C., Rodriguez, A., and Holben, W. E. (2004). Soil biota and exotic plant invasion. Nature 427, 731–733. doi: 10.1038/nature02322
DiCicco, J. M. (2014). Long-term urban park ecological restoration: a case study of Prospect Park, Brooklyn, New York. Ecol. Restor. 32, 314–326. doi: 10.3368/er.32.3.314
Ehrenfeld, J. G., Ravit, B., and Elgersma, K. (2005). Feedback in the plant-soil system. Annu. Rev. Environ. Resour. 30, 75–115. doi: 10.1146/annurev.energy.30.050504.144212
Elgersma, K. J., Yu, S., Vor, T., and Ehrenfeld, J. G. (2012). Microbial-mediated feedbacks of leaf litter on invasive plant growth and interspecific competition. Plant Soil 356, 341–355. doi: 10.1007/s11104-011-1117-z
Elmqvist, T., Setälä, H., Handel, S. N., van der Ploeg, S., Aronson, J., Blignaut, J. N., et al. (2015). Benefits of restoring ecosystem services in urban areas. Curr. Opin. Environ. Sustain. 14, 101–108. doi: 10.1016/j.cosust.2015.05.001
Heinen, R., van der Sluijs, M., Biere, A., Harvey, J. A., and Bezemer, T. M. (2017). Plant community composition but not plant traits determine the outcome of soil legacy effects on plants and insects. J. Ecol. 106, 1217–1229. doi: 10.1111/1365-2745.12907
Heinze, J., and Joshi, J. (2017). Plant-soil feedback effects can be masked by aboveground herbivory under natural field conditions. Oecologia 186, 235–246. doi: 10.1007/s00442-017-3997-y
Jordan, N. R., Larson, D. L., and Huerd, S. C. (2008). Soil modification by invasive plants: effects on native and invasive species of mixed-grass prairies. Biol. Invasions 10, 177–190. doi: 10.1007/s10530-007-9121-1
Kaplan, I., Halitschke, R., Kessler, A., Sardanelli, S., and Denno, R. F. (2008). Constitutive and induced defenses to herbivory in above- and belowground plant tissues. Ecology 89, 392–406. doi: 10.1890/07-0471.1
Keane, R. M., and Crawley, M. J. (2002). Exotic plant invasions and the enemy release hypothesis. Trends Ecol. Evol. 17, 164–170. doi: 10.1016/S0169-5347(02)02499-0
Klironomos, J. N. (2002). Feedback with soil biota contributes to plant rarity and invasiveness in communities. Nature 417, 67–70. doi: 10.1038/417067a
Knight, T. M., Dunn, J. L., Smith, L. A., Davis, J., and Kalisz, S. (2009). Deer facilitate invasive plant success in a Pennsylvania forest understory. Nat. Areas J. 29, 110–116. doi: 10.3375/043.029.0202
Kos, M., Tuijl, M. A. B., de Roo, J., Mulder, P. P. J., and Bezemer, M. T. (2015b). Species-specific plant-soil feedback effects on above-ground plant-insect interactions. J. Ecol. 103, 908–914. doi: 10.1111/1365-2745.12402
Kos, M., Tuijl, M. A. B., de Roo, J., Mulder, P. P. J., and Bezemer, T. M. (2015a). Plant-soil feedback effects on plant quality and performance of an aboveground herbivore interact with fertilization. Oikos 124, 658–667. doi: 10.1111/oik.01828
Kulmatiski, A., Beard, K. H., Stevens, J. R., and Cobbold, S. M. (2008). Plant-soil feedbacks: a meta analytical review. Ecol. Lett. 11, 908–992. doi: 10.1111/j.1461-0248.2008.01209.x
Mangan, S. A., Schnitzer, S. A., Herre, E. A., Mack, K. M. L., Valencia, M. C., Sanchez, E. I., et al. (2010). Negative plant-soil feedback predicts tree species relative abundance in a tropical forest. Nature. 466, 752–755. doi: 10.1038/nature09273
Mitchell, C. E., and Power, A. G. (2003). Release of invasive plants from fungal and viral pathogens. Nature 625, 625–627. doi: 10.1038/nature01317
Packer, A., and Clay, K. (2000). Soil pathogens and spatial patterns of seedling mortality in a temperate tree. Nature 404, 278–821. doi: 10.1038/35005072
R Core Development Team (2017). R: A Language and Environment for Statistical Computing, Version 3.4. Vienna: R Foundation for Statistical Computing. Available online at: http://www.R-project.org/
Relva, M. A., Nuñez, M. A., and Simberloff, D. (2010). Introduced deer reduce native plant cover and facilitate invasion of non-native tree species: evidence for invasional meltdown. Biol. Invasions 12, 303–311. doi: 10.1007/s10530-009-9623-0
Reynolds, H. L., Packer, A., Bever, J. D., and Clay, K. (2003). Grassroots ecology: plant-microbe-soil interactions as drivers of plant community structure and dynamics. Ecology 84, 2281–2291. doi: 10.1890/02-0298
Schrama, M., Heijning, P., Bakker, J. P., van Wijnen, H. J., Berg, M. P., and Olff, H. (2013). Herbivore trampling as an alternative pathway for explaining differences in nitrogen mineralization in moist grasslands. Oecologia 172, 321–243. doi: 10.1007/s00442-012-2484-8
Shea, K., and Chesson, P. (2002). Community ecology theory as a framework for biological invasions. Trends Ecol. Evol. 17, 170–176. doi: 10.1016/S0169-5347(02)02495-3
Smith, L. M., and Reynolds, H. L. (2012). Positive plant-soil feedback may drive dominance of a woodland invader, Euonymus fortunei. Plant Ecol. 213, 853–860. doi: 10.1007/s11258-012-0047-z
Smith, L. M., and Reynolds, H. L. (2015). Euonymus fortunei dominance over native species may be facilitated by plant-soil feedback. Plant Ecol. 216, 1401–1406. doi: 10.1007/s11258-015-0518-0
Smith-Ramesh, L. M., and Reynolds, H. L. (2017). The next frontier of plant-soil feedback research: unraveling context dependence across biotic and abiotic gradients. J. Veg. Sci. 28, 484–494. doi: 10.1111/jvs.12519
Keywords: plant-soil feedback, herbivory, invasion, Midwest deciduous forest, native-invasive competition
Citation: Bennett SI, Howard C, Albrecht R, Smith-Ramesh LM and Reynolds H (2020) Simulated Herbivory Weakens Plant-Soil Feedbacks in Competitive Mixtures of Native and Invasive Woodland Plants. Front. Ecol. Evol. 7:497. doi: 10.3389/fevo.2019.00497
Received: 13 June 2019; Accepted: 04 December 2019;
Published: 08 January 2020.
Edited by:
Jasmin Joshi, University of Applied Sciences Rapperswil, SwitzerlandReviewed by:
Justin Wright, Duke University, United StatesIlias Travlos, Agricultural University of Athens, Greece
Copyright © 2020 Bennett, Howard, Albrecht, Smith-Ramesh and Reynolds. This is an open-access article distributed under the terms of the Creative Commons Attribution License (CC BY). The use, distribution or reproduction in other forums is permitted, provided the original author(s) and the copyright owner(s) are credited and that the original publication in this journal is cited, in accordance with accepted academic practice. No use, distribution or reproduction is permitted which does not comply with these terms.
*Correspondence: Savannah I. Bennett, c2F2YWJlbm5AaW5kaWFuYS5lZHU=