- U.S. Geological Survey, Forest and Rangeland Ecosystem Science Center, Corvallis, OR, United States
Although biological soil crusts (biocrusts) occur globally in arid and semi-arid environments, most of our knowledge of biocrust cover and ecology is from a relatively small number of locations worldwide. Some plant communities are known to have high cover of biocrusts, but the abundance of biocrusts is largely undocumented in most plant communities. Using a data driven approach, we identified 16 plant communities based on plant cover from the Assessment, Inventory, and Monitoring Strategy data from the Bureau of Land Management (AIM, 5,200 plots). We found that abundance of lichens and mosses varies among communities, but that both components of biocrusts are present in all plant communities. Biocrusts are indicators of two of these communities: one that is defined by high cover of mosses and basin big sagebrush and one that is defined by high cover of lichens and shadscale saltbush. Using non-parametric multiplicative regression, we evaluated a suite of abiotic and disturbance variables to assess the degree to which climate and soils are associated with the abundance of lichens and mosses at the regional scale. At the regional scale, soil depth and maximum vapor pressure deficit were found to be strongly associated with the abundance of lichens and January minimum temperature dictated the abundance of mosses. At the scale of plant communities, community specific metrics of soils and climate were better able to explain the abundance of biocrusts. Our demonstration of the presence of biocrusts across the western US suggests that studies on ecosystem function could include these organisms because they are present in all plant communities, maintain arguably stronger associations with climatic variation, are directly associated with soils, and contribute to ecosystem functions that are not solely maintained by vascular plants.
Significance
Biological soil crusts (biocrusts) contribute to ecosystem functions such as carbon and nitrogen cycling, hydrologic cycling, soil stabilization, and maintenance of albedo in arid and semi-arid environments worldwide. Most studies on biocrusts are from single plant communities in a few locations. The occurrence of biocrusts has not previously been related to plant communities at the regional scale of the western United States (US). We demonstrate that biocrusts occur in all plant communities but with varying abundance across the semi-arid and arid western US. Regionally, moss abundance peaks on lands with January minimum temperatures between 5 and 0°C. Lichen abundance peaks where soil depth is between 0 and 50.8 cm and when annual maximum vapor pressure deficit falls between 15 and 40 hPa. At the scale of plant communities, site-specific factors are generally stronger predictors of abundance of mosses and lichens compared to the regional drivers. These results highlight that biocrusts occur in all plant communities across the semiarid and arid western US and suggest that if management goals include ecosystem functions to which biocrusts contribute, biocrusts could be included.
Introduction
Globally, interspaces among vascular plants in undisturbed semi-arid ecosystems are occupied by biological soil crusts (biocrusts), referring to lichens, mosses, and cyanobacteria that grow on the soil surface (Belnap and Lange, 2001). Many of these organisms contribute to nutrient and hydrologic cycling, dust capture, and all of them contribute to the prevention of soil erosion (Evans and Ehleringer, 1993; Belnap and Gillette, 1998; Reynolds et al., 2001; Barger et al., 2006; Bowker et al., 2011). The occurrence of biocrusts is included in the larger description of biocrusts of North America by Rosentreter and Belnap (2001), highlighting that biocrusts occur in the hot deserts, cold deserts, and chaparral. In the absence of disturbance, where space between plants is large, the abundance of biocrusts increases with increasing moisture availability (Bowker et al., 2006; Büdel et al., 2009; Kidron et al., 2010). However, drivers behind the occurrence and abundance of biocrusts at the biogeographic scale are largely unknown (Steven et al., 2013). This is likely because biocrusts have not been studied in the same way that plant communities have been identified, with studies that span regions but identify areas that are homogenous regarding driving factors of soils and climate. Studies that intersect the occurrence of both biocrusts and plant communities are rare and generally within a single plant community (Hilty et al., 2004; Reisner et al., 2013; Warren et al., 2015; Condon and Pyke, 2018a,b), making generalizations difficult regarding drivers of biocrust abundance. To date, we do not know of any studies on occurrence and abundance of biocrusts that span plant communities at the regional scale of the western US that would allow for the identification of drivers of biocrust occurrence and abundance. Working across scales would allow for a demonstration of the degree to which biocrusts are specific to a particular plant community vs. the degree to which they may be ubiquitous across communities of the western US.
Soils and climate allow plant species to grow in a given location but interactions amongst species, and interactions between species and disturbances, can filter or promote occurrence (Gunderson, 2000). Soils and long-term averages in precipitation are important for determining plant species occurrence and plant communities (Barbour and Billings, 2000). However, it is likely that climatic and edaphic factors that dictate extreme conditions are favoring biocrusts, particularly the lichen component (Hale, 1967). Lichens and most mosses lack protective cuticles, vascular structure and water-transporting roots, leaving them dependent on their surrounding environment for resources (Proctor and Tuba, 2002; McCune and Geiser, 2009). However, they are also poikilohydric, using water as it is available, and with the ability to survive in a desiccated state for years (Breuil Sée, 1993; Proctor and Tuba, 2002; Wasley et al., 2006). They are tolerant of freezing conditions (Lenné et al., 2010) as well as temperatures that are too high for many vascular plants (Hale, 1967). Given these dichotomies, we expect that the extreme ends of the vapor pressure deficit spectrum will favor biocrusts. For example, if there is a high likelihood of dew in the winter but conditions are dry enough to be stressful for vascular plants, cover of biocrusts is expected to be high. Similar relationships are expected with edaphic factors. Sites that are stressful for vascular plants regarding chemistry, water availability, or radiation are expected to favor biocrusts (Garcia-Pichel and Castenholz, 1991; Bowker and Belnap, 2008; Zhao et al., 2010; Rajeev et al., 2013).
The current study aims to demonstrate the occurrence and abundance of biocrusts by plant community across arid and semi-arid, non-forested lands. Although we expect that biocrusts have an affinity for temperature extremes and arid areas, we expect drivers of biocrust abundance to differ at the scale of plant communities, since specific ranges of climatic and edaphic conditions are considered in delineating plant communities. We also expect that land uses and soil characteristics at the scale of a plant community will be more similar and therefore are likely to have a greater influence at this scale as opposed to the regional scale. Although biocrusts are tolerant of climatic and edaphic extremes, we expect that they are less tolerant to disturbances of the soil surface (Condon and Pyke, 2018a,b; Duniway et al., 2018). Under this scenario, soils that are armored with gravel and rocks will be protected from disturbances that perturb the soil surface and are therefore likely to have higher cover of biocrusts. Other driving factors that might influence the occurrence of biocrusts include the affinity of some terricolous lichen species for calcareous soils (McCune and Rosentreter, 2007). We address the following objectives for both the moss and lichen components of biocrusts:
(1) document the occurrence and abundance by plant community across the arid and semi-arid western US, (2) identify drivers of abundance at the regional scale, and (3) compare regional drivers with drivers within each plant community.
Methods
Identification of Plant Communities
Beginning in 2011, BLM began using a standardized monitoring framework to collect quantitative data that can be used to make management decisions and to meet management objectives of renewable resources such as vegetation, soils, water, fish and wildlife habitat, the Assessment, Inventory and Monitoring (AIM) program (Toevs et al., 2011). These lands are managed for multiple uses including grazing by livestock, energy development, and recreation. A subset of these data, referred to as the Landscape Monitoring Framework (LMF) includes data on plant cover, characterization of soils and disturbance across the western US excluding Alaska. This massive dataset (5,200 locations as of 2015) gives us the ability to assess the occurrence and abundance of biocrusts, specifically mosses, and lichens in multiple biomes across much of the continent. In the LMF of the AIM program, trained observers visit a randomly assigned location, given specified rejection criteria (see http://aim.landscapetoolbox.org/ accessed 12 May 2017). Rejection criteria for the LMF includes tree cover being >25%, the plot must be <3 miles from the nearest point accessible by vehicle and the land ownership is BLM. If the site is found to be acceptable, observers center two perpendicular 150-foot (45.7 m) transects on the predetermined point. Point-intercept data of plant species and ground cover contacts are recorded for a total of 102 points per plot (Herrick et al., 2005). Point-intercepts from the top of the plant canopy and down to basal intercept can include 5 additional unique intercepts on the way down (for a total of 6 plant intercepts and one ground cover intercept). These possible 7 intercepts are included in our analysis to distinguish communities based on plant composition. For example, Wyoming big sagebrush may be the initial overstory plant intercept species but could have a native or introduced understory. Species taxonomy follows the USDA PLANTS database [United States Department of Agriculture, Natural Resources Conservation Service (USDA, NRCS), 2017] and includes the following non-vascular plant and non-plant categories: none, herbaceous litter, woody litter, artificial litter, organic matter, deposited soil, lichen, moss, rock fragments, and unknown plants. We use all existing data through 2015, resulting in 5200 locations (plots) across 11 states in the western US and summarize data to the plot level. Data are not collected on the occurrence of cyanobacteria and so we do not address the occurrence of cyanobacteria in this analysis.
We use vascular plant species cover data as well as lichen and moss cover to derive plant communities for the region. Plant communities (groups) were formed by hierarchical cluster analysis with indicator species analysis as an objective criterion to select the number of ecologically meaningful groups (Dufrêne and Legendre, 1997). We did not transform cover because we expected that extremes in cover values may strongly favor or discourage lichens and mosses. The most meaningful number of groups demonstrated the highest number of significant indicators (species with p < 0.05) and the lowest average p-value across all species (McCune and Grace, 2002). Indicator species analysis considers both the relative abundance of species and the frequency of species within groups. A species cannot receive a high indicator value for a group unless it is both abundant and faithful to that group. Analyses were performed in PC-ORD version 7.
Identifying the Drivers of Moss and Lichen Abundance
We use non-parametric multiplicative regression (NPMR) to select possible drivers of moss and lichen abundances because NPMR naturally handles interactions amongst predictor variables by testing various ranges of predictor variables on ranges of response variables and employing a multiplicative kernel smoother. We use local mean models with a uniform (rectangular) kernel as we expected precipitous changes in cover, moving from areas with high cover to no detected cover. A consequence of the uniform kernel is that response surfaces tend to be relatively rough, as compared to smoother surfaces produced by the more commonly used Gaussian kernel. NPMR allows for the visualization of relationships that might change over a gradient. For example, perhaps moss cover increases with shrub cover to a certain threshold, at which point moss cover starts to decline or plateau at a site. NPMR naturally identifies these values and relationships and can be more accurate compared to linear models when non-linear relationships exist. Models are assessed with a cross-validated R2 (1- RSS/TSS = xR2) and overfitting is avoided as response surfaces are estimated for each data point, in the absence of that data point. Overfitting controls were used at the medium level, specifically requiring that a minimum of 10 data points be used in prediction, predictor variables were required to improve xR2 by 5%, and the minimum average neighborhood size was 0.05 times the number of plots. Sensitivity analysis was performed on each predictor to evaluate its importance relative to other predictors. Conceptually, sensitivity is the mean difference in the response/range in response divided by the difference in predictor/range in predictor in the equation (McCune, 2009):
In the above equation, ŷi+ is the estimate of the response variable in the case i having increased the predictor by a small value of Δ and ŷi is the estimate of the response variable in the case i having decreased the predictor by a small value of Δ. This calculation for sensitivity gives less weight to large differences and the interpretability is straightforward as a sensitivity = 1 means that a change in the predictor results in a change in the response of equal magnitude (McCune, 2009). We also report the tolerance of each predictor, the half-width of the rectangular. Models with low xR2 are expected to have low sensitivities and high tolerances for the predictors. It is also possible for a model to have a negative xR2 if cross validation results in the residual sum of squares exceeds the total sum of squares. Models were run for cover of mosses and lichens across the region as well as within each plant community type.
Soil and Climate Data
NPMR models were run on a subset of data used to define plant communities because soils data were not collected in 2011, resulting in 3,209 plots with soils data. We expected that both lichens and mosses would be highly sensitive to soil characteristics and so we used measured site-specific data as opposed to pre-existing soil maps, which vary in resolution accuracy. Site-specific soils data includes the depth of the surface horizon, the texture of that horizon, the presence of any texture modifier, and effervescence classes which indicate the amount of calcium carbonate in soil, ranging from 1 for not effervescent to 5 for violently effervescent. Soils texture data were made numeric by using the median values of clay, silt, and sand in each texture class. Texture modifiers were ranked by size class as of the modifier and amount. We used PRISM climate data at the 800 m grid scale (http://prism.oregonstate.edu/ accessed 1 May 2018) including 30 year average annual precipitation, annual maximum vapor pressure deficit, annual minimum vapor pressure deficit, mean dew point temperature in January, average minimum January temperature, average maximum July temperature and elevation. Vapor pressure deficit refers to the difference in the amount of moisture in the air compared with compared with how much moisture the air can hold when saturated in hPa. Dew point is the temperature at which water droplets condense, which varies with barometric pressure and humidity. We anticipated that January temperatures and dew points would be important given the fact that mosses in the semi-arid region of the Intermountain West grow during the winter (Condon and Pyke, 2016) and that mosses often desiccate before freezing (Malek and Bewley, 1978). July is often the hottest month of the year, making July maximum temperature a value that characterizes current temperature extremes (https://www.ncdc.noaa.gov/sotc/national/201707 accessed 13 July 2018). Elevation was also included because in addition to 30 year average annual precipitation, these factors are commonly used to explain occurrence of plant communities (Barbour and Billings, 2000).
Disturbances
AIM data include the occurrence of disturbances at two temporal scales (present and past) as well as two spatial scales (on the plot and the larger area that plot resides in, delineated by fence lines, if present, or 1,000 ft from the plot, United States Department of Agriculture, Natural Resources Conservation Service (USDA, NRCS), 2015). Analyzed disturbances were observed within the management unit (larger area). We coded these as ordinal variables where the disturbance was noted as a “1” if it only occurred in either the past or at present but coded as a “2” if it occurred both in the past and in the present. Disturbances fall under the general categories of land uses, land treatments, and natural features. Disturbance types are described in Supplementary Table 1.
Results
A combination of hierarchical cluster analysis and indicator species analysis resulted in 16 plant communities across BLM lands in the western US. This includes one additional group of sites with low cover and as such they did not naturally fit into the other communities. Species that characterized each plant community based on indicator species analyses were used to name the community (Figure 1, Supplementary Table 2). All plant communities had plots that contained lichens and mosses but with varying abundances of these organisms (Figure 1). Two communities are in part defined by cover of biocrusts: big sagebrush-moss and shadscale-lichen because they had high cover of mosses and lichens, respectively.
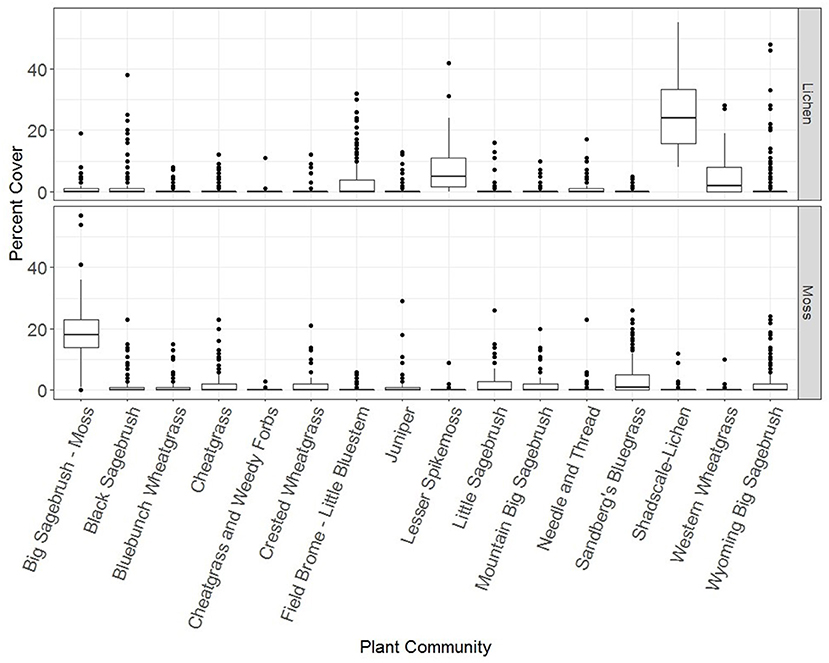
Figure 1. Cover of lichens and mosses within each plant community across the western US summarized as boxplots. Boxplots show the inter quartile range. Whiskers show values within 1.5 the inter-quartile range, the distance between the first and third quantiles. Data beyond this range are plotted as individual points.
Most plant communities fit with those described for the Intermountain West by West and Young (2000) with a few exceptions. Field brome—Little Bluestem Grassland and Needle and Thread—Threadleaf Sedge Grassland are tallgrass and mixed grass prairie, respectively (Sims and Risser, 2000). Lesser spikemoss and associates are common of alpine and meadow communities (Billings, 2000) and these species are also common in the grasslands of the northwestern Great Plains of Montana, North Dakota, and South Dakota (Crane, 1990).
At the regional scale of non-forested drylands in the western US, the best predictors of lichen cover were soil depth, maximum vapor pressure deficit and elevation. Areas with generally shallow soils that are drier and sometimes at elevation are more favorable to the lichen component of biocrusts (xR2 = 0.215, Table 1, Supplementary Figure 1). However, based on xR2, models that were created for each plant community were generally better predictors of lichen abundance, except for the following plant communities: juniper and the cheatgrass- weedy forb community (Table 1). This suggest that factors other than climate and soils, such as past land use, may control the occurrence of biocrusts in these vegetation types, particularly the cheatgrass- weedy forb community which did not result in a useful model. Relationships between soil depth, maximum vapor pressure deficit, elevation and lichen cover are present in many but not all plant communities. To demonstrate the coverage of plots that were classified in each community we show how many plots fell into each plant community, how many were recorded as having mosses and lichens and where each plot was located (Supplementary Figure 1).
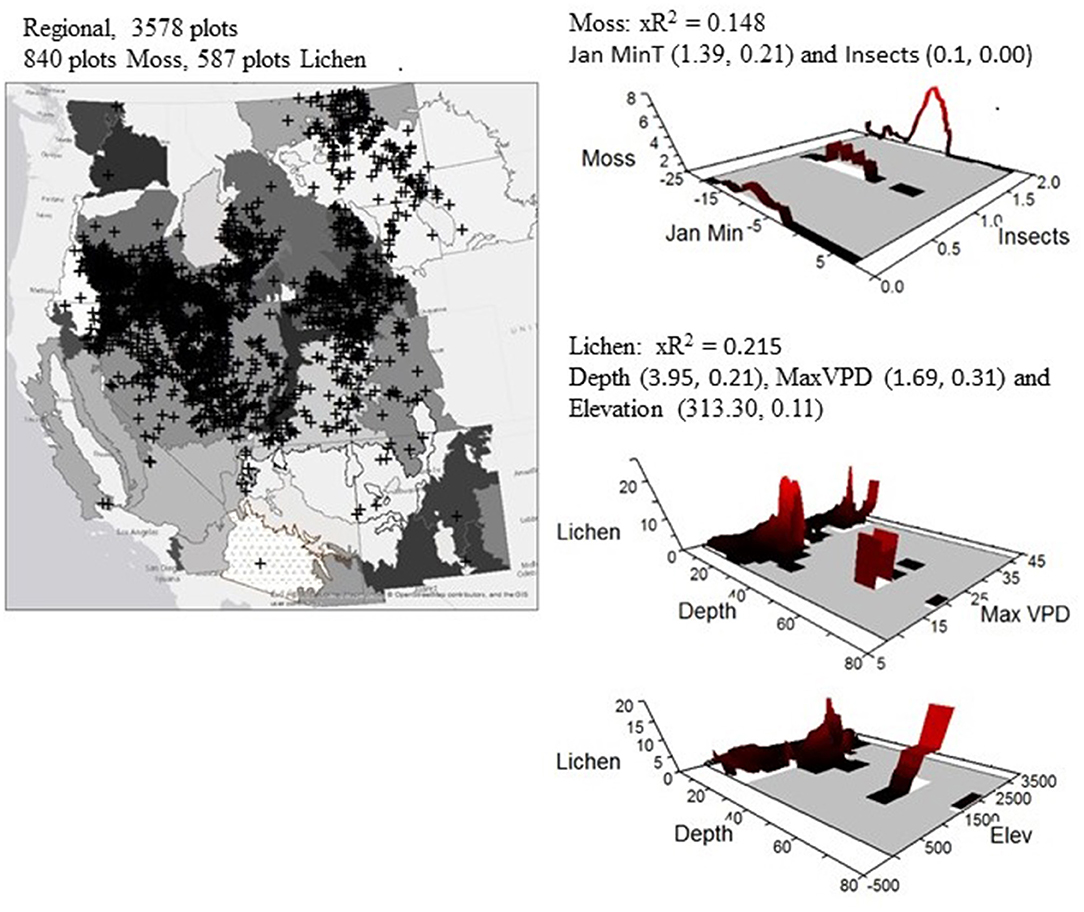
Figure 2. The geographical distribution of the total number of plots classified in each identified plant community and the number of plots that were recorded as having mosses and lichens. The background is the EPA Level 3 ecoregions. Adjacent to the map are NPMR models that of the best regional predictors of lichen cover: maximum vapor pressure deficit (Max VPD), soil depth (Depth) and Elevation and for moss cover: January minimum temperature (JanMinT) and Insects. For each model the cross-validated R2 (xR2), tolerance, and sensitivity are reported.
The best predictors of moss cover at the regional scale were January minimum temperature and presence of insects (Table 2). Similar to models predicting lichen cover, models predicting moss cover were generally stronger based on xR2 when created at the scale of the individual plant community. Exceptions to this include models for the field brome-little bluestem community and the western wheatgrass community (Table 2). Relationships between January minimum temperature and moss cover were present in four of the 16 identified plant communities. Additionally, the community with the highest proportion of plots with moss, the big sagebrush—moss community was the only community to have the presence of insects included in its associated model (Table 2).
At the scale of plant communities, predictor variables associated with location were common: county, state, latitude and longitude. These factors were significant in nine of the 16 lichens models and 12 of the 16 moss models (Tables 1, 2).
Discussion
We demonstrate that the lichen and moss components of biocrusts occur throughout BLM lands, in all identified plant communities, across the arid and semi-arid western US. The predictors that are commonly used to explain the occurrence of biocrusts work well for the lichen component: drier sites (increased maximum vapor pressure deficit) with shallow soils (soil depth, Rosentreter and Belnap, 2001). However, winter conditions were the best predictors of the abundance of mosses regionally, specifically January minimum temperature. The cover of these components of biocrusts vary by plant community, and the drivers behind the occurrence of both mosses and lichens were generally a combination of soils and climatic factors.
Two plant communities were defined by the abundance of examined biocrust components: big sagebrush—moss community and shadscale—lichen community (Figure 1, Supplementary Table 2). Both communities inherently have low cover of herbaceous plants but for opposing reasons. Sagebrush can have large canopies, leaving little exposed interspace for herbaceous species (Ellsworth et al., 2016). However, some mosses prefer filtered light, and are often favored by these microclimates (Proctor and Smirnoff, 2000; Durham et al., 2018). Due to dry, salty conditions, shadscale communities are generally harsh sites requiring more saline-tolerant vascular plants and less productive sites, which make them more amenable to biocrusts (West and Young, 2000). In both scenarios, biocrusts are filling a void that is not easily filled by vascular plants.
The factors that drive the abundance of the lichen and moss components of biocrusts varied by plant community. The Wyoming big sagebrush community demonstrated some of the highest maximum cover values of the lichen component outside of the shadscale-lichen community (nearly 45%), even though average cover values were low (Figure 1). In this community, there is a need to manage for cover of biocrusts and particularly the lichen component given historic overgrazing and the association between lichens and reduced cover of cheatgrass (Condon and Pyke, 2018a). The Sandberg's bluegrass community demonstrated relatively low cover values of lichens and moderately high cover values of mosses (Figure 1) corroborating descriptions of this community type and biocrust associations in the northern Great Basin (Rosentreter, 1986). Additionally, Sandberg's bluegrass is commonly seeded in restoration projects (Knutson et al., 2014). It is possible that tillage from seeding efforts led to reductions in cover of lichens as has been seen in sagebrush steppe habitats in Montana (Durham et al., 2018). We were unable to reliably model lichen cover in the cheatgrass-weedy forb community because lichens were only recorded in two of these plots (Figure 1, Table 1). Weedy forbs such as Russian thistle are not very competitive and are characteristic of sites that have recently been heavily disturbed (West and Young, 2000). The high likelihood of recent heavy disturbance in this community may explain the low cover of lichens because lichens are generally sensitive to disturbance (Ponzetti and McCune, 2001; Ponzetti et al., 2007; Condon and Pyke, 2018b). The importance of location-related predictors in the many of plant community specific models of lichen and moss cover suggest that there are environmental or disturbance related predictors that have been missed. Location related predictors may also relate to potentially differing abilities of crews to detect lichens and mosses since crews are often contracted to cover specific areas.
In rangelands of Argentina, Australia, Mexico, Portugal, and Inner Mongolia, biological soil crusts have been evaluated in the relation to grazing practices along gradients or a series of time since disturbance (Liu et al., 2009; Gómez et al., 2012; Concostrina-Zubiri et al., 2014, 2016; Eldridge et al., 2016). Disturbance factors in the AIM dataset are noted as present or absent, possibly masking differences in cover that would be apparent if disturbances were quantified along a continuum. Studies on the effects of grazing have been conducted on biocrusts in portions of the western US but not across the region (Anderson et al., 1982; Ponzetti and McCune, 2001; Condon and Pyke, 2018a,b; Duniway et al., 2018; Condon et al., 2019). Examining the abundance of biocrusts in conjunction with potential stressors could lead to the erroneous conclusion that biocrusts are absent or only present at low levels, when in fact they are being examined under the stressors that keep them as low cover. Under these conditions, it is difficult to separate the effects of current from historic lands uses such as historic overgrazing, abandoned cropland, invasive species, forage seeding, recreation, and changing fire frequencies leading to reductions in cover that are still apparent (Morris and Rowe, 2014; Condon and Pyke, 2018b).
Recent work on biocrusts highlights their potential sensitivities to climate change. It is anticipated that warmer climates will result in the mortality of the lichen and moss components of biocrusts and shift communities to more early successional components such as cyanobacteria (Escolar et al., 2012; Ferrenberg et al., 2015; Maestre et al., 2015). These changes in composition are likely to have cascading effects on global nitrogen and carbon cycles given the trend of later successional biocrusts, including lichens and mosses, to fix more carbon and nitrogen (Houseman et al., 2006; Barger et al., 2013). However, we observed an association between the abundance of lichens and increased aridity (maximum vapor pressure deficit and July maximum temperatures). Our finding that the abundance of the moss component of biocrusts is largely driven by minimum temperatures in January, corroborate this potential trend across the western US. It is well-documented that short, precipitation events in warmer months leads to chlorosis and eventual declines in moss cover (Barker et al., 2005; Coe et al., 2012; Reed et al., 2012; Condon and Pyke, 2016) and that ecotypes that are accustomed to summer monsoons are not associated with stress tolerance (Doherty et al., 2017). The effects of climate change on biocrusts may be self-perpetuating given the ability of some biocrust organisms to alter albedo and surface energy (Couradeau et al., 2016; Rutherford et al., 2017).
Higher cover of biocrust organisms may have been detected if a different sampling method had been used because line-point intercept (LPI) is not the best method for detecting organisms with low cover. A greater number of sampling points are needed to quantify species with low cover (Bonham, 2013). Our data likely underestimates the occurrence of these organisms both due to the number of points used with LPI and because there is a tendency for observers misidentify mosses and lichens that are not obvious and to call them soil or litter. Although we anticipate that some observers missed or misidentified some components of biocrusts, our work demonstrates that biocrusts are found throughout the western US.
The contributions of biocrusts to ecosystem functions need to be further explored both regionally and within plant communities where the roles and composition of biocrust components are likely to differ. Corroborating Belnap et al. (2001), mosses make up a greater proportion of biocrust cover in the more northern part of the region, which aligns with the plant communities that fall within the cold deserts of the Snake River Plain, Northern and Central Basin and Range (Supplementary Figure 1). Although mosses have been shown to put on substantial increases in cover every winter in the more northern part of the region (Condon and Pyke, 2016), this trend may not hold in sites that fall outside of the same climatic regimes. Biological soil crusts affect site hydrology with greater infiltration being observed on biocrusts dominated by intact mosses as opposed to disturbed biocrusts or biocrusts dominated by lichens (Brotherson and Rushforth, 1983; Chamizo et al., 2012). Biocrusts may increase runoff in the case of early successional, light cyanobacterial crusts (Faist et al., 2017) but may ultimately reduce runoff, leading to greater net infiltration compared to areas without biocrusts due to the microtopography that they maintain (Warren, 2003). These are critical relationships in arid and semi-arid environments where plant establishment is limited by available water (Bainbridge, 2007). Biological soil crusts are also contributors to the ecosystem function nutrient cycling which is of paramount importance in these semi-arid ecosystems. Bromus tectorum L. and other ruderal and weedy plant species benefit from self-perpetuating nutrient cycles, particularly excess nitrogen (Sperry et al., 2006). Scientists continue to explore management actions that will reduce labile soil nutrients in efforts to reduce B. tectorum (Jones et al., 2015). The restoration of biocrusts could be considered in these efforts as biocrusts fix carbon (Evans and Lange, 2003) and carbon additions increase the carbon/nitrogen ratio, reducing the amount of nitrogen that is available for uptake by vegetation (Mazzola et al., 2008). Our study demonstrates the potential for biocrusts to be in all plant communities across the western United States justifying their inclusion in restoration and management targets.
Conclusions
We demonstrate the presence of the lichen and moss components of biocrusts in all plant communities across the semi-arid western US, concluding that the absence of the lichen and moss components of biocrusts is not a result of their being unable to grow in the environmental setting in any of our identified plant communities. Maximum vapor pressure deficits, soil depth and elevation are regionally important for lichen cover, but moss cover is largely driven by minimum temperatures in January suggesting that potential changes in these associated climatic variables are likely to influence the prevalence of the biocrust components.
Throughout the region, plant communities are being related to potential vegetation types or Ecological Site Descriptions (ESDs), based on information about soils and climate. Existing ESDs are currently being used to understand ecosystem dynamics and responses to disturbance allowing for the prediction of changes in dominant plant species following thresholds of disturbance (Stringham et al., 2003; Brown and Bestelmeyer, 2016). Our work provides a foundation for the inclusion of biocrusts in the ESD framework with reported maximum values of moss and lichen cover in each identified plant community. Future examinations of disturbance thresholds with the objective of understanding the recovery of ecosystem processes would be more complete with the inclusion of biocrusts.
Data Availability Statement
The datasets generated for this study can be found in the Bureau of Land Management https://landscape.blm.gov/geoportal/catalog/AIM/AIM.page.
Author Contributions
LC and DP conceived the study and wrote the paper. LC analyzed the data.
Conflict of Interest
The authors declare that the research was conducted in the absence of any commercial or financial relationships that could be construed as a potential conflict of interest.
Acknowledgments
We thank the Bureau of Land Management and everyone who has contributed to the Assessment, Inventory and Monitoring dataset. This work was funded by the US Geological Survey Coordinated Intermountain Restoration Project, US Fish and Wildlife Service, Nevada State Office, Bureau of Land Management, Nevada State Office, and the US Forest Service. The manuscript benefited from comments by Emily Kachergis, Dr. Peter Weisberg and Dr. Bruce McCune. This report has been peer reviewed and approved for publication consistent with USGS Fundamental Science Practices (http://pubs.usgs.gov/circ/1367/). Any use of trade, firm, or product names is for descriptive purposes only and does not imply endorsement by the U.S. government.
Supplementary Material
The Supplementary Material for this article can be found online at: https://www.frontiersin.org/articles/10.3389/fevo.2019.00449/full#supplementary-material
References
Anderson, D. C., Harper, K. T., and Rushforth, S. R. (1982). Recovery of cryptogamic soils from grazing on Utah winter ranges. J. Range Manage. 35, 355–359. doi: 10.2307/3898317
Barbour, M. G., and Billings, W. D. (Eds.). (2000). North American Terrestrial Vegetation, 2nd Edn. Cambridge: Cambridge University Press.
Barger, N. N., Castle, S. C., and Dean, G. N. (2013). Denitrification from nitrogen-fixing biologically crusted soils in a cool desert environment, southeast Utah, U.S.A. Ecol. Process. 2, 1–9. doi: 10.1186/2192-1709-2-16
Barger, N. N., Herrick, J. E., VanZee, J. W., and Belnap, J. (2006). Impacts of biological soil crust disturbance and composition on C and N loss from water erosion. Biogeochemistry 77, 247–263. doi: 10.1007/s10533-005-1424-7
Barker, D. H., Stark, L. R., Zimpfer, J. F., McLetchie, N. D., and Smith, S. D. (2005). Evidence of drought-induced stress on biotic crust moss in the Mojave Desert. Plant Cell Environ. 28, 939–947. doi: 10.1111/j.1365-3040.2005.01346.x
Belnap, J., and Gillette, D. A. (1998). Vulnerability of desert biological soil crusts to wind erosion: the influences of crust development, soil texture, and disturbance. J. Arid Environ. 39, 133–142. doi: 10.1006/jare.1998.0388
Belnap, J., Kaltenecker, J. H., Rosentreter, R., Williams, J., Leonard, S., and Eldridge, D. (2001). Biological Soil Crusts: Ecology and Management. U.S. Department of the Interior, Bureau of Land Management.
Belnap, J., and Lange, O. L. (Eds.). (2001). Biological Soil Crusts: Structure, Function and Management. Berlin: Springer, Verlag.
Billings, W. D. (2000). “Alpine vegetation,” in North American Terrestrial Vegetation, 2nd Edn, eds M. G. Barbour and W. D. Billings (Cambridge: Cambridge University Press), 537–572.
Bonham, C. D. (2013). Measurements for Terrestrial Vegetation, 2nd Edn. West Sussex: Wiley Blackwell, 246.
Bowker, M. A., and Belnap, J. (2008). A simple classification of soil types as habitats of biological soil crusts on the Colorado Plateau, U.S.A. J. Veg. Sci. 19, 831–840. doi: 10.3170/2008-8-18454
Bowker, M. A., Belnap, J., Davidson, D. W., and Goldstein, H. (2006). Correlates of biological soil crust abundance across a continuum of scales: support for a hierarchical conceptual model. J. Appl. Ecol. 43, 152–163. doi: 10.1111/j.1365-2664.2006.01122.x
Bowker, M. A., Mau, R. L., Maestre, F. T., Escolar, C., and Castillo-Monroy, A. P. (2011). Functional profiles reveal unique ecological roles of various biological soil crust organisms. Funct. Ecol. 25, 787–795. doi: 10.1111/j.1365-2435.2011.01835.x
Breuil Sée, A. (1993). Recorded dessication-survival times in bryophytes. J. Bryol. 17, 679–684. doi: 10.1179/jbr.1993.17.4.679
Brotherson, J. D., and Rushforth, S. R. (1983). Influences of cryptogammic crusts on moisture relationships of soils in Navajo National Monument, Arizona. Great Basin Nat. 53, 40–47.
Brown, J. R., and Bestelmeyer, B. T. (2016). An introduction to the special issue “Ecological Sites for Landscape Management”. Rangelands 38, 311–312. doi: 10.1016/j.rala.2016.11.001
Büdel, B., Darienko, T., Deutschewitz, K., Dojani, S., Friedl, T., Mohr, K. I., et al. (2009). Southern African biological soil crusts are ubiquitous and highly diverse in drylands, being restricted by rainfall frequency. Microb. Ecol. 57, 229–247. doi: 10.1007/s00248-008-9449-9
Chamizo, S., Cantón, Y., Lázaro, R., Solé-Benet, A., and Domingo, F. (2012). Crust composition and disturbance drive infiltration through biological soil crusts in semiarid ecosystems. Ecosystems 15, 148–161. doi: 10.1007/s10021-011-9499-6
Coe, K. K., Belnap, J., and Sparks, J. P. (2012). Precipitation driven carbon balance controls survivorship of desert mosses. Ecology 93, 1626–1636. doi: 10.1890/11-2247.1
Concostrina-Zubiri, L., Huber-Sannwald, E., Martínez, I., Flores, J. F., Reyes-Aguero, J. A., Escudero, A., et al. (2014). Biological soil crusts across disturbance-recovery scenarios: effect of grazing regime on community dynamics. Ecol. Appl. 24, 1863–1877. doi: 10.1890/13-1416.1
Concostrina-Zubiri, L., Molla, I., Velizarova, E., and Branquinho, C. (2016). Grazing or not grazing: Implications for ecosystem services provided by biocrusts in Mediterranean cork oak woodlands. Land. Degrad. Dev. 28, 1345–1353. doi: 10.1002/ldr.2573
Condon, L. A., Pietrasiak, N., Rosentreter, R., and Pyke, D. A. (2019). Passive restoration of vegetation and biological soil crusts following 80 years of exclusion from grazing across the Great Basin. Restor. Ecol. doi: 10.1111/rec.13021. [Epub ahead of print].
Condon, L. A., and Pyke, D. A. (2016). Filling the interspace- restoring arid land mosses: source populations, organic matter, and overwintering govern success. Ecol. Evol. 6, 7623–7632. doi: 10.1002/ece3.2448
Condon, L. A., and Pyke, D. A. (2018a). Fire and grazing influence site resistance to cheatgrass invasion through their effects on shrub, bunchgrass, and biocrust communities. Ecosystems 21, 1416–1431. doi: 10.1007/s10021-018-0230-8
Condon, L. A., and Pyke, D. A. (2018b). Resiliency of biological soil crusts and vascular plants varies among morphogroups with disturbance intensity. Plant Soil 433, 271–287. doi: 10.1007/s11104-018-3838-8
Couradeau, E., Karaoz, U., Lim, H. C., Nunes da Rocha, U., Northen, T., Brodie, E., et al. (2016). Bacteria increase arid-land soil surface temperature through the production of sunscreens. Nat. Commun. 7:10373. doi: 10.1038/ncomms10373
Crane, M. F. (1990). “Selaginella densa,” in Fire Effects Information System. U.S. Department of Agriculture, Forest Service, Rocky Mountain Research Station, Fire Sciences Laboratory. Available online at: www.fs.fed.us/database/feis//plants/fern/selden/all.html (accessed June 21, 2019).
Doherty, K. D., Bowker, M. A., Antoninka, A. J., Johnson, N. C., and Wood, T. E. (2017). Biocrust moss populations differ in growth rates, stress response, and microbial associates. Plant Soil. 429, 187–198. doi: 10.1007/s11104-017-3389-4
Dufrêne, M., and Legendre, P. (1997). Species assemblages and indicator species: the need for a flexible asymmetrical approach. Ecol. Monogr. 67, 345–366. doi: 10.2307/2963459
Duniway, M. C., Geiger, E. L., Minnick, T. J., Phillips, S. L., and Belnap, J. (2018). Insights from long-term ungrazed and grazed watersheds in a salt desert Colorado Plateau Ecosystem. Rangeland Ecol. Manage. 71, 492–505. doi: 10.1016/j.rama.2018.02.007
Durham, R. A., Doherty, K. D., Antoninka, A. J., Ramsey, R. W., and Bowker, M. A. (2018). Insolation and disturbance history drive biocrust diversity in western Montana rangelands. Plant Soil 430, 151–169. doi: 10.1007/s11104-018-3725-3
Eldridge, D. J., Delgado-Baquerizo, M., Travers, S. K., Val, J., and Oliver, I. (2016). Do grazing intensity and herbivore type affect soil health? Insights from a semi-arid productivity gradient. J. Appl. Ecol. 54, 976–985. doi: 10.1111/1365-2664.12834
Ellsworth, L. M., Wrobleski, D. W., Kauffman, J. B., and Reis, S. A. (2016). Ecosystem resilience is evident 17 years after fire in Wyoming big sagebrush ecosystems. Ecosphere 7:e01618. doi: 10.1002/ecs2.1618
Escolar, C., Martinez, I., Bowker, M. A., and Maestre, F. T. (2012). Warming reduces the growth and diversity of biological soil crusts in a semi-arid environment: implications for ecosystem structure and functioning. Philos. Trans. R. Soc. B Biol. Sci. 367, 3087–3099. doi: 10.1098/rstb.2011.0344
Evans, R. D., and Ehleringer, J. R. (1993). A break in the nitrogen cycle in arid lands? Evidence from δ15N of soils. Oecologia 94, 314–317. doi: 10.1007/BF00317104
Evans, R. D., and Lange, O. L. (2003). “Biological soil crusts and ecosystem nitrogen and carbon dynamics,” in Biological Soil Crusts: Structure, Function, and Management, eds J. Belnap and L. Lange (Berlin: Springer, Verlag), 263–279.
Faist, A. M., Herrick, J. E., Belnap, J., Van Zee, J. W., and Barger, N. N. (2017). Biological soil crusts and disturbance controls on surface hydrology in a semi-arid ecosystem. Ecosphere 8, 1–13. doi: 10.1002/ecs2.1691
Ferrenberg, S., Reed, S. C., and Belnap, J. (2015). Climate change and physical disturbance cause similar community shifts in biological soil crusts. Proc. Natl. Acad. Sci. U.S.A. 112, 12116–12121. doi: 10.1073/pnas.1509150112
Garcia-Pichel, F., and Castenholz, R. W. (1991). Characterization and biological implications of scytonemin, a cyanobacterial sheath pigment. J. Phycol. 409, 395–409. doi: 10.1111/j.0022-3646.1991.00395.x
Gómez, D. A., Aranibar, J. N., Tabeni, S., Villagra, P. E., Garibotti, I. A., and Atencio, A. (2012). Biological soil crust recovery after long-term grazing exclusion in the Monte Desert (Argentina). Changes in coverage, spatial distribution and soil nitrogen. Acta. Oecol. 38, 33–40. doi: 10.1016/j.actao.2011.09.001
Gunderson, L. H. (2000). Ecological resilience- in theory and application. Annu. Rev. Ecol. Syst. 31, 425–439. doi: 10.1146/annurev.ecolsys.31.1.425
Herrick, J. E., Van Zee, J. W., Havstad, K. M., Burkett, L. M., and Whitford, W. G. (2005). Monitoring Manual for Grassland, Shrubland and Savanna Ecosystems, Vol. I. Las Cruces, NM: Quick Start; USDA Agricultural Research Service; Jornada Experimental Range.
Hilty, J. H., Eldridge, D. J., Rosentreter, R., Wicklow-Howard, M. C., and Pellant, M. (2004). Recovery of biological soil crusts following wildfire in Idaho. J. Range Manage. 57, 89–96. doi: 10.2307/4003959
Houseman, D. C., Powers, H. H., Collins, A. D., and Belnap, J. (2006). Carbon and nitrogen fixation differ between successional stages of biological soil crusts in the Colorado Plateau and Chihuahuan Desert. J. Arid Environ. 66, 620–34. doi: 10.1016/j.jaridenv.2005.11.014
Jones, R., Chambers, J. C., Johnson, D. W., Blank, R. R., and Board, D. I. (2015). Effects of repeated burning on plant and soil carbon and nitrogen in cheatgrass (Bromus tectorum) dominated systems. Plant Soil 386, 47–64. doi: 10.1007/s11104-014-2242-2
Kidron, G. J., Vonshak, A., Dor, I., Barinova, S., and Abeliovich, A. (2010). Properties and spatial distribution of microbiotic crusts in the Negev Desert, Israel. Catena 82, 92–101. doi: 10.1016/j.catena.2010.05.006
Knutson, K. C., Pyke, D. A., Wirth, T. A., Arkle, R. S., Pilliod, D. S., Brooks, M. L., et al. (2014). Long-term effects of seeding after wildfire on vegetation in Great Basin shrubland ecosystems. J. Appl. Ecol. 51, 1414–1424. doi: 10.1111/1365-2664.12309
Lenné, T., Bryant, G., Hocart, C. H., Huang, C. X., and Ball, M. C. (2010). Freeze avoidance: a dehydrating moss gathers no ice. Plant Cell Environ. 33, 1731–1741. doi: 10.1111/j.1365-3040.2010.02178.x
Liu, H., Han, X., Li, L., Huang, J., Liu, H., and Li, X. (2009). Grazing density effects on cover, species composition, and nitrogen fixation of biological soil crusts in an Inner Mongolia steppe. Rangeland Ecol. Manage. 62, 321–327. doi: 10.2111/08-179.1
Maestre, F. T., Escolar, C., and Bardgett, R. D. (2015). Warming reduces the cover and diversity of biocrust-forming mosses and lichens, and increases the physiological stress of soil microbial communities in a semi-arid Pinus halepensis plantation. Front. Microbiol. 6:865. doi: 10.3389/fmicb.2015.00865
Malek, L., and Bewley, J. D. (1978). Effects of various rates of freezing on the metabolism of a drought-tolerant plant, the moss Tortula ruralis. Plant Physiol. 61, 334–338. doi: 10.1104/pp.61.3.334
Mazzola, M. B., Allcock, K. G., Chambers, J. C., Blank, R. R., Schupp, E. W., Doescher, P. S., et al. (2008). Effects of nitrogen availability and cheatgrass competition on the establishment of Vavilov Siberian Wheatgrass. Rangeland Ecol. Manage. 61, 475–484. doi: 10.2111/07-133.1
McCune, B. (2009). Nonparametric Multiplicative Regression for Habitat Modeling. Corvallis, OR: Oregon State University.
McCune, B., and Geiser, L. (2009). Macrolichens of the Pacific Northwest, 2nd Edn. Corvallis, OR: Oregon State University Press, 464.
McCune, B., and Grace, J. B. (2002). Analysis of Ecological Communities. Gleneden Beach, OR: MJM Software Design.
McCune, B., and Rosentreter, R. (2007). Biotic Soil Crust Lichens of the Columbia Basin. Monographs in North American Lichenology. Vol. 1, 1–105. Corvallis, OR: Northwest Lichenologists.
Morris, L. R., and Rowe, R. J. (2014). Historical land use and altered habitats in the Great Basin. J. Mamm. 95, 1144–1156. doi: 10.1644/13-MAMM-S-169
Ponzetti, J., and McCune, B. (2001). Biotic soil crusts of Oregon's shrub steppe: community composition in relation to soil chemistry, climate and livestock activity. Bryologist 104, 212–225. doi: 10.1639/0007-2745(2001)104[0212:BSCOOS]2.0.CO;2
Ponzetti, J. M., McCune, B., and Pyke, D. A. (2007). Biotic soil crusts in relations to topography, cheatgrass and fire in the Columbia Basin, Washington. Bryologist 110, 706–22. doi: 10.1639/0007-2745(2007)110[706:BSCIRT]2.0.CO;2
Proctor, M. C. F., and Smirnoff, N. (2000). Rapid recovery of photosystems on rewetting dessication-tolerant mosses: chlorophyll fluorescence and inhibitor experiments. J. Exp. Bot. 51, 1695–1704. doi: 10.1093/jexbot/51.351.1695
Proctor, M. C. F., and Tuba, P. (2002). Poikilohydry and homoihydry: antithesis or spectrum of possibilities. New Phytol. 156, 327–349. doi: 10.1046/j.1469-8137.2002.00526.x
Rajeev, L., da Rocha, U. N., Klitgord, N., Luning, E. G., Fortney, J., Axen, S. D., et al. (2013). Dynamic cyanobacterial response to hydration and dehydration in a desert biological soil crust. ISME J. 7, 2178–2191. doi: 10.1038/ismej.2013.83
Reed, S. C., Coe, K. K., Sparks, J. P., Housman, D. C., Zelikova, T. J., and Belnap, J. (2012). Changes to dryland rainfall result in rapid moss mortality and altered soil fertility. Nat Clim Change 2, 752–755. doi: 10.1038/nclimate1596
Reisner, M. D., Grace, J. B., Pyke, D. A., and Doescher, P. S. (2013). Conditions favouring Bromus tectorum dominance of endangered sagebrush steppe ecosystems. J. Appl. Ecol. 50, 1039–1049. doi: 10.1111/1365-2664.12097
Reynolds, R., Belnap, J., Reheis, M., Lamothe, P., and Luiszer, F. (2001). Aeolian dust in Colorado Plateau soils: nutrient inputs and recent change in source. Proc. Natl. Acad. Sci. U.S.A. 98, 7123–7127. doi: 10.1073/pnas.121094298
Rosentreter, R. (1986). “Compositional patterns within a rabbitbrush (Chrysothamnus) community of the Idaho Snake River Plain,” in Proceedings- Symposium on the Biology of Artemisia and Chrysothamnus. GTR-INT-2000 (Ogden, UT: USDA USFS Intermountain Research Station).
Rosentreter, R., and Belnap, J. (2001). “Biological soil crusts of North America,” in Biological Soil Crusts: Structure, Function and Management, eds J. Belnap, O. L. Lange (Berlin: Springer, Verlag), 31–50.
Rutherford, W. A., Painter, T. H., Ferrenberg, S., Belnap, J., Okin, G. S., Flagg, C., et al. (2017). Albedo feedbacks to future climate via climate change impacts on dryland biocrusts. Sci. Rep. 7:44188. doi: 10.1038/srep44188
Sims, P. L., and Risser, P. G. (2000). “Grasslands,” in North American Terrestrial Vegetation, 2nd Edn, eds M. G. Barbour and W. D. Billings (Cambridge: Cambridge University Press), 323–356.
Sperry, L. J., Belnap, J., and Evans, R. D. (2006). Bromus tectorum invasion alters nitrogen dynamics in an undisturbed arid grassland ecosystem. Ecology 87, 603–615. doi: 10.1890/05-0836
Steven, B., Gallegos-Graves, L. V., Belnap, J., and Kuske, C. R. (2013). Dryland soil microbial communities display spatial biogeographic patterns associated with soil depth and soil parent material. FEMS Microb. Ecol. 86, 101–113. doi: 10.1111/1574-6941.12143
Stringham, T. Z., Krueger, W. C., and Shaver, P. L. (2003). State and transition modeling: an ecological process approach. J. Range Manage. 56, 106–113. doi: 10.2307/4003893
Toevs, G. R., Taylor, J. J., Spurrier, C. S., MacKinnon, W. C., and Bobo, M. R. (2011). Bureau of Land Management Assessment, Inventory and Monitoring Strategy: For Integrated Renewable Resource Management. Denver, CO: Bureau of Land Management, National Operations Center.
United States Department of Agriculture Natural Resources Conservation Service (USDA, NRCS). (2015). National Resources Inventory Grazing Land On-Site Data Collection Handbook of Instructions. Washington, DC: USDA NRCS.
United States Department of Agriculture Natural Resources Conservation Service (USDA, NRCS). (2017). The PLANTS Database. Greensboro, NC: National Plant Data Team. Avaliable online at: http://plants.usda.gov (accessed March 21, 2017).
Warren, S. D. (2003). “Biological soil crusts and hydrology in North American Deserts,” in Biological Soil Crusts: Structure, Function, and Management, eds J. Belnap and L. Lange (Berlin: Springer, Verlag), 327–337.
Warren, S. D., St. Clair, L. L., Johansen, J. R., Kugrens, P., Baggett, L. S., and Bird, B. J. (2015). Biological soil crust response to late season prescribed fire in a Great Basin juniper woodland. Rangeland Ecol. Manag. 68, 241–247. doi: 10.1016/j.rama.2015.03.007
Wasley, J., Robinson, S. A., Lovelock, C. E., and Popp, M. (2006). Some like it wet- biological characteristics underpinning tolerance of extreme water stress events in Antarctic bryophytes. Funct. Plant Biol. 33, 443–455. doi: 10.1071/FP05306
West, N. E., and Young, J. A. (2000). “Intermountain valleys and lower mountain slopes,” in North American Terrestrial Vegetation, eds M. G. Barbour and W. D. Billings (Cambridge: Cambridge University Press), 255–284.
Keywords: AIM data, biocrusts, climate, disturbance, lichen, moss, non-parametric multiplicative regression, soils
Citation: Condon LA and Pyke DA (2020) Components and Predictors of Biological Soil Crusts Vary at the Regional vs. Plant Community Scales. Front. Ecol. Evol. 7:449. doi: 10.3389/fevo.2019.00449
Received: 21 June 2019; Accepted: 07 November 2019;
Published: 08 January 2020.
Edited by:
Vincent John Martin Noah Linus Felde, University of Kassel, GermanyReviewed by:
Chongfeng Bu, Institute of Soil and Water Conservation (CAS), ChinaGianalberto Losapio, ETH Zürich, Switzerland
This work is authored by Condon and Pyke on behalf of the U.S. Government and, as regards Condon, Pyke and the U.S. Government, is not subject to copyright protection in the United States. Foreign and other copyrights may apply. This is an open-access article distributed under the terms of the Creative Commons Attribution License (CC-BY). The use, distribution or reproduction in other forums is permitted, provided the original author(s) and the copyright owner(s) are credited and that the original publication in this journal is cited, in accordance with accepted academic practice. No use, distribution or reproduction is permitted which does not comply with these terms.
*Correspondence: Lea A. Condon, lcondon@usgs.gov