- 1Department of Chemical Ecology, Bielefeld University, Bielefeld, Germany
- 2Biodiversity Research/Systematic Botany, University of Potsdam, Potsdam, Germany
- 3Berlin-Brandenburg Institute of Advanced Biodiversity Research (BBIB), Berlin, Germany
- 4Department of Ecology and Ecosystem Management, Restoration Ecology, Technical University of Munich, Freising, Germany
(1) Environmental conditions experienced in the past may lead to intraspecific differences in ecological and chemical traits of plants, which likely affect future responses to altered or new environments. Whether competition by neighbors is such a trait-shaping factor is not yet well-known. We aimed to understand how the level of ancestral plant competition affects traits related to plant fitness and resource allocation, reproduction, and (phyto-)toxin accumulation in offspring, and whether a potential differentiation in these traits can be found in different geographic origins of which one belongs to the native and one to the invaded range. (2) We compared differentiation of the following traits in offspring plants of multiple populations in Erodium cicutarium (Geraniaceae): biomass, seed production, seed traits related to dispersal and germination, and concentrations of foliar mono- and sesquiterpenes. We tested the allelopatic potential of aqueous extracts of the same E. cicutarium plants on seeds of five different plant families. (3) In plants originating from populations that experienced high levels of competition, we found twice as high monoterpene concentrations. These plants also produced more biomass and a higher proportion of ripe to unripe seeds until harvesting. Seeds originating from high competition sites were shorter. Aqueous E. cicutarium leaf extracts with high terpenoid content reduced radicle length of Zea mays and radicle and hypocotyl length of E. cicutarium seedlings. (4) The results of this study provide first evidence that the surrounding vegetation may shape chemo-ecological plant traits that may be fundamental for competitive ability. Our study calls for more research testing whether competition experienced in the native range may lead to an enhanced capability of plants to establish populations and spread in a new range.
Introduction
Plants are strongly influenced by their neighbors within the local environment. It has been shown that persistent competition of plants for above- and belowground resources, such as light (Rajcan and Swanton, 2001), space, nutrients, and water results in a plethora of competition avoidance traits, such as early germination, elongation and pre-emption of space (Weinig, 2000), morphological and physiological adaptation, and the release of phytotoxins (i.e., allelopathy) (Weidenhamer et al., 1989; Fernandez et al., 2016). Such plant traits matching the ancestral competitive environment and thus potentially increasing fitness also for the following generations can be passed on to the offspring (Heger et al., 2014). Plant competition, together with other environmental factors ancestral plant populations experience, can therefore locally shape functional traits and may also influence the future capability of offspring plants to establish and grow in environments, in which the ancestors did not evolve (Saul et al., 2013). Together with general traits that increase the probability for invasion success (van Kleunen et al., 2010), such divergent selection regimes in the native range may lead to trait differences within species and evoke differences in the invasive success among populations. For plants whose ancestors encountered a highly competitive environment i.e., aboveground competition for light, the probability to be likewise exposed to such competition is higher if their regular dispersal distance is small. Local adaptation to competition, e.g., by earlier germination or other trait changes enhancing competition avoidance or competitive strength, could therefore be more efficient if paired with a change in seed morphology, thus enhancing short distance dispersal (Baythavong et al., 2009).
The ability of plants to respond to competitors cannot only be enhanced by morphological and life history traits, but also by chemical traits. Past research revealed an immense intraspecific chemical diversity with regard to specialized plant metabolites (Post and Stenseth, 1999; Pither, 2003; Thuiller et al., 2004; Moore et al., 2014), thus suggesting the potential for local adaptation to the level of competition also in these traits. It was for instance shown in a Brassicaceae species that plants with a history of greater interspecific competition invested more in a toxic allelochemical (i.e., a glucosinolate), even when offspring plants were grown under common conditions (Lankau, 2012). Terpenoids are among the most structurally diverse metabolites in plants (Tholl, 2006) and remarkable levels of intraspecific terpenoid diversity were found in plants from various families, e.g., herbaceous Asteraceae (Johnson et al., 2007; Kleine and Müller, 2011; Wolf et al., 2012), or Fagaceae trees (Loreto et al., 2009). Moreover, mono- and sesquiterpenes in several plant species have been shown to express an allelopathic function (Fischer et al., 1994; Ens et al., 2009), which may also enhance the plants' competitive ability (Callaway and Ridenour, 2004). Monoterpenes function as allelochemicals by inhibiting both cell-nuclear and organelle DNA synthesis and thus cell proliferation in the root apical meristem (Nishida et al., 2005). Furthermore, monoterpenes attack lipophilic layers of the leaf surface and disturb the stomata function, thereby provoking enhanced transpiration (Schulz et al., 2007). Assuming that terpenoids act as allelochemicals and are involved in competition with neighboring plants, competition may lead to a selective pressure and offspring plants which contain higher amounts of allelopathic terpenoids may have a competitive advantage.
Our experimental plant species, the common stork's-bill Erodium cicutarium L' Hér. ex Aiton (Geraniaceae), has been the subject of various chemical and biological studies. E. cicutarium is an annual to biennial herb which is currently distributed globally (Fiz-Palacios et al., 2010), although its native range comprises only Europe, North Africa and Western Asia (Francis et al., 2012). The plant is self-fertile (Baythavong et al., 2009) and each individual can produce up to 9,900 seeds (Blackshaw and Harker, 1998). Thus, E. cicutarium is supposable independent of faunal ecosystem services and main targets of released chemicals may be inter- and intraspecific competitors, or herbivores. Water and alcohol extracts of E. cicutarium plants were shown to act against the Colorado potato beetle Leptinotarsa decemlineata (Lamparski and Wawrzyniak, 2005), the cabbage butterfly Pieris brassicae (Wawrzyniak, 2010) and the tobacco budworm Heliothis virescens (Klocke, 1987). However, less is known about the allelopathic function of E. cicutarium plant extracts and chemicals therein.
Shape, composition and structure of E. cicutarium mericarps (diaspores) are unique and allow for explosive seed dispersal and strong anchorage behavior. The schizocarpic fruits of E. cicutarium contain up to five mericarps (diaspores), which are catapulted up to 100 cm away from the mother plant (Stamp, 1984, 1989; Evangelista et al., 2011). Hygroscopic awns enable the mericarp to drill itself into soil by alternating awn coiling and uncoiling (Stamp, 1989; Evangelista et al., 2011). The mericarps have been characterized from a mechanical perspective (Evangelista et al., 2011), and experiments demonstrated a positive relationship between fruit (i.e., mericarp: seed plus awn) length and ballistic dispersal (Jacobs and Lesmeister, 2012). Secondly, E. cicutarium contains high levels of specialized metabolites such as phenolic compounds (Fecka et al., 2001; Fecka and Cisowski, 2005) and terpenoids (Radulovic et al., 2009; Stojanovic-Radic et al., 2010), which are constitutively contained at remarkably high levels in plant extracts. Moreover, the plant species has been subject of various invasion ecology studies, as it affects biodiversity and agriculture in its invaded range (Blackshaw and Harker, 1998; Brooks, 2000). A long-term study (16 years) revealed a clear suppression of native plants by the presence of invading E. cicutarium, based on competitive plant interactions (Schutzenhofer and Valone, 2006).
Based on these findings, the present study focuses on potential effects of intra- and interspecific plant competition, encountered by ancestral populations collected at two different geographic regions on two continents in which the plant is either native or invasive, on seed traits, fitness and chemical traits of offspring plants. We measured mericarp (= diaspore) morphology and germination ability in the first greenhouse grown generation, and foliar terpenoid concentration, biomass and mericarp number in plants of the second generation.
We hypothesized (1). mericarp awns from populations that had experienced high levels of plant competition to be shorter, leading to short distance dispersal and thereby enabling the offspring to make use of inherited competition-avoidance traits as suggested by (Baythavong et al., 2009). In addition, we expected a higher proportion of germination and a higher ratio of ripe to unripe schizocarps (= fruits, consisting of up to five mericarps) as a measure for earlier development under persistent competition. We further hypothesized (2). higher foliar terpenoid concentrations in offspring plants from high competition-experienced mother plants compared to offspring plants from low-competition ancestors. In many plant species, terpenoids have been found to show an allelopathic function and thus counteract competition. We therefore hypothesized (3). decreased hypocotyl and radicle growth of seeds treated with foliar extracts of terpene-rich E. cicutarium plants, i.e., from high-competition ancestors, Finally, we hypothesized (4). intraspecific differences in the concentrations of foliar terpenoids in the second laboratory generation of plants, as a measure of biochemical adaptations of plants to the local environments (e.g., climatic conditions, presence of herbivores) at the two distinct geographic regions, of which one belongs to the native and one to the invaded range. We expected plants with higher terpenoid concentrations to compromise the additional biochemical costs by allocating fewer resources to biomass and reproductive outcome.
Materials and Methods
Origin of Seeds
In 2011 and 2012, 14 populations in Bavaria (Germany, continental climate, native range, see Table S1) and 14 populations in California (USA, Mediterranean climate, invaded range, see Table S1) were chosen, and seeds were collected from up to 20 mother plants per population (Heger et al., unpublished data). At each collection site, the level of competition was assessed in three 50 × 50 cm plots by estimating the cover and measuring aboveground biomass of vegetation surrounding E. cicutarium. The populations have been chosen to represent a gradient in the level of competition they are exposed to, ranging from situations where E. cicutarium was growing among a dense cover of grasses and other plant neighbors to situations with sparse cover of neighbors. Out of this pool of populations and maternal families, we selected four populations in each of both regions (i.e., California and Germany) and five maternal families per population to be included in the current study. For each region, we chose two populations facing a comparably high level of competition, mostly interspecific but also intraspecific, and two with a low level of competition. The categorization of collection sites into “high competition” or “low competition” was done based on the mean cover of vegetation: Collection sites with a mean cover of 73% and more were classified as “high competition sites,” and those with a cover of 35% and less as “low competition sites,” independent of the surrounding plant species (Table S1; see also Heger et al., 2014).
When choosing the populations for sampling, we made sure that the environments were as similar as possible with respect to abiotic conditions, and mainly differed with respect to the level of competition. In each region, we therefore included a high and a low competition population occurring within the same area (i.e., Erlangen, Exerzierplatz in Germany, and McLaughling Natural Reserve in California, Table S1). Soil samples confirmed that sites with a high level of competition were not systematically richer in soil nutrients; also, temperature and precipitation do not differ systematically among the two groups high and low competition (see Table S1).
In order to minimize maternal effects, the seeds were grown for one generation under standardized conditions in a greenhouse. These plants were allowed to self-fertilize, and the seeds were harvested. The produced mericarps (1st generation) were weighed and measured before they were used to grow the experimental plants for this study (2nd generation) in a climate chamber. From the mericarps produced by the 2nd generation, we measured both parts separately, the seed and the uncoiled awn, which was still connected to the seed. Uncoiling was achieved by soaking the mericarps for a few seconds in water.
Germination and Plant Cultivation
To investigate the effect of the level of ancestral competition and geographic region of seed origin, we used the mericarps (1st generation, see above) in a germination assay and thus placed them on 1.2% agar-agar (Bioscience, Roth, Germany) in transparent plastic boxes with semi-transparent lids (l × h × w = 200 × 65 × 200 mm, 20–25 seeds of at least 5–6 populations per box). A 4 cm interspaced grid was drawn underneath each box and letters and numbers were assigned to rows and columns which allowed distinct labeling. Mericarps from different populations (i.e., geographic region and competition level) were randomly distributed among boxes onto the fields of the grids, thus minimizing effects due to potential microclimatic differences within each box and between the boxes without causing confusion of positions. The boxes were placed into a climate chamber at 20°C and 70% humidity 16:8 h light: dark. Seed germination was recorded thrice a day. After 7 days, we planted emerged seedlings into substrate (2:1 river sand: garden mold) in individual pots (l × h × w = 7 × 8 × 7 cm, polypropylene). The seedlings remained in the same climate chamber but received direct light. Plants were watered thrice a week. After 2 weeks, the plants were additionally fertilized twice a week with a standard formulation of mineral fertilizer (Wuxal, Manna, Düsseldorf, Germany).
Plant Harvest and Phenotyping
The numbers of ripe mericarps (diaspores, up to five per schizocarp, consisting of a seed and a connected awn) and unripe (green) schizocarps were counted at day 112 after placing the seeds on agar (see above). Plants which had already produced flowers were harvested on this day; plants which did not flower yet were harvested 14 days later in order to reach a comparable ontogenetic state. All plants had produced at least the first buds until harvest. Shoots were cut off and the fresh biomass was determined. Of each plant, the two youngest leaves above 6 cm length and the three largest leaves of the bottom of the rosette, which are likely the oldest leaves, were pooled, placed on ice and subsequently frozen at × 80°C. The pooled leaf samples were freeze-dried for 24 h and coarsely crushed to homogenize samples before terpenoid extraction and preparation of aqueous extracts to test allelopathy.
Foliar Terpenoid Extraction and Analysis
The extraction and analysis of terpenoids was conducted using a modified protocol of Kleine and Müller (2013). Of the lyophilized leaf samples, 40 mg were supplemented by a stainless steel ball (Ø 5 mm) and ground for 30 s at 30 Hz (Retsch MM 301, Haan, Germany), each in 1 mL n-heptane (Roth, 99% HPLC grade), containing 5 ng μL−1 1-bromodecane (97%, Sigma Aldrich, Karlsruhe, Germany) as internal standard. The samples were incubated for 15 min in an ultrasonic bath at room temperature and centrifuged for 5 min at 13,200 rpm. The supernatants were concentrated under nitrogen flow to a volume of ~30 μL and analyzed by gas chromatography coupled with mass spectroscopy (GC-MS) (Focus GC coupled to a DSQII MS instrument, Thermo, 515 Electron Corporation, S.o.A. Rodano, Italy) in electron impact ionization mode. Helium was used as a carrier gas (flow rate = 1.1 mL min−1). A VF-5 ms column (30 m × 0.2 mm ID, 10 m guard column, Varian, Palo Alto, CA, USA) was used. The GC-injection port was kept at 240°C and operated in split mode (10 mL min−1, ratio = 10:1). The GC oven program started at 50°C for 5 min and increased at 280°C with 10°C min−1 (total run duration 28 min). An alkane standard mix (C8-C20, Sigma Aldrich, Karlsruhe, Germany) was analyzed under the same conditions in order to calculate retention indices (RI) for each targeted compound (Kováts, 1958). The identification of volatiles was accomplished by comparison of RI and mass spectra to the National Institute of Standards and Technology library and the Pherobase (El-Sayed, 2019). Relative concentrations of mono- and sesquiterpenes were calculated by converting the obtained peak areas to sample dry weights and by normalizing the sample to the internal standard peak area (1-bromodecane).
To test allelopathic potential of E. cicutarium leaves, aqueous leaf extract samples were produced (see section Allelopathic potential of foliar extracts). The terpenoid content of these extracts was checked prior to conducting a germination assay by absorption of compounds on conditioned pieces of polydimethylsiloxane-(PDMS) (Eilers et al., 2015). The PDMS- pieces were 4 mm long and left for 1 h in the filtered extracts in Erlenmeyer flasks. Terpenoids were analyzed by a thermal desorption unit (TDU), coupled to a gas-chromatograph and mass spectrometer (GS-MS; TD-20, GC-MS QP2020, Shimadzu, Kyoto, Japan). After insertion of one PDMS sampling tube, the TDU temperature increased from 30 to 230°C at a rate of 30°C min−1 under a helium flow of 60 ml min−1, kept for 8 min. Compounds were desorbed onto a Tenax® adsorbent trap at a temperature of −20°C. The trap was then heated to 250°C for 3 min. The interface heat trap and line temperature were set to 250°C and the split ratio at the injection port was 1:3. Compounds were separated on a Rtx-5MS column (30 m × 0.25 mm, 0.25 μm film thickness, Restek, Bad Homburg, Germany) with a column flow of 1.6 ml min−1 of helium. The oven temperature programme was the same as for liquid injection of heptane extracts.
Allelopathic Potential of Foliar Extracts
We tested the allelopathic potential of aqueous E. cicutarium leaf extracts by using a modified protocol after Fernandez et al. (2016) and Yu et al. (2003). The use of aqueous extracts in this context is admissible, due to high aqueous solubility for several monoterpenes, comparable to the common allelopathic phenolics and organic acids (Weidenhamer et al., 1993; Fischer et al., 1994). Germination rates, radicle and hypocotyl length of the following plant species were tested after application of aqueous leaf extracts of E. cicutarium: Medicago sativa (Fabaceae), Zea mays (variety Badischer Gelber, Poaceae), Daucus carota (variety Nominator, Apiaceae), and Sinapis alba (Brassicaceae) (all Kiepenkerl, Everswinkel, Germany). In addition, E. cicutarium (Geraniaceae; Appels Wilde Samen, Darmstadt, Germany) seeds were tested to investigate auto-allelopathic potential. The leaves for this experiment originated from the same samples as used for foliar terpenoid extraction and analysis (harvesting procedure described in section Plant harvest and phenotyping). We pooled 2 g lyophilized leaf sample for each of the four treatment combinations: “high competition” or “low competition” in region “Germany” or “California.” More specifically, 200 μg lyophilized leaf sample from each of the five maternal families within the two populations for each competition level were combined for each pooled sample, yielding 200 μg × 5 × 2 = 2 g leaf material. Each pooled sample was macerated in borosilicate glassware in 200 mL ultrapure water (Milli-Q, Merck, Germany) by shaking for 24 h on an orbital shaker at 200 rpm in dark conditions at room temperature. The leaves were removed from extracts with folded filters (MN 615, Macherey-Nagel, Düren, Germany). Afterwards, the extracts were immediately transferred to Petri dishes, lined with 55 mm filter paper (MN 615, Macherey-Nagel, Düren, Germany). Four plant seeds were placed individually with forceps in equal distance on the filter papers (n = 8 Petri dishes for each treatment and plant species). The Petri dishes were sealed with Parafilm M (Bemis, Neenah, United States of America) and kept at room temperature in the dark. After 6 days, all seedlings were measured. To quantify the relative amount of terpenoids in aqueous extracts, the method of Eilers et al. (2015) was applied. A polydimethylsiloxane-(PDMS) tube piece was added as absorbance to a 1 mL subsample of each aqueous extracts in glass vials, left for 1 h at 20°C and was analyzed with a thermal desorption-gas-chromatograph coupled to a mass spectrometer (TD-GS-MS, for details see section Foliar terpenoid extraction and analysis).
Statistical Analyses
We used the software “R” version 3.2.3 (R Core Team, 2019) for statistical data analysis. Most of the data were not normally distributed and required logarithmic (log) or square root (sqrt) transformation. We analyzed the effect of level of plant competition at the seed collection site (i.e., high or low) and geographic region of seed origin (i.e., California, USA or Bavaria, Germany) (fixed effects) on the continuous and/or transformed data for seed length, mericarps weight and awn length of the first greenhouse reared generation using linear mixed models (LMM) with Gaussian distribution and a nested design (i.e., populations within competition level and region; function “lmer” in package “lme4”). The non-transformed data for the length of mericarps and awns was tested for correlation (Spearman's rank correlation). We furthermore tested the effect of ancestral competition and geographic region of seed origin on the continuous and/or transformed data for shoot biomass (fresh weight), number of unripe schizocarps, number of ripe mericarps, ratio of ripe to unripe schizocarps and foliar monoterpene and sesquiterpene concentrations in the second laboratory reared generation of plants, using LMM with Gaussian distribution (function “lmer”). To test the effect of region and competition (fixed effects) on the proportion of germinated seeds, we used a generalized linear mixed model (GLMM) with binomial distribution (function “glmer” in package “lme4”). The number of produced mericarps per schizocarp was analyzed by generalized linear mixed-effects models with Poisson distribution. In all mixed-effects models, we included the random effects seed collection site (three groups in each of the two regions, Table S1) and family (five families within each population). To test the effect of aqueous leaf extracts of E. cicutarium plants from different ancestral regions and competition levels on root and shoot length of seedlings from different plant families in the allelopathy experiment, we first averaged the numbers for the four seeds in each of eight Petri dishes. We then used Kruskal-Wallis rank sum tests to compare the five treatment groups and, if differences between groups were significant (p < 0.05), conducted post-hoc pairwise comparisons using Wilcoxon rank sum tests with “BH” adjustment of p- values (Benjamini and Hochberg, 1995).
Results
In the first plant generation, we found an interaction effect of the level of ancestral competition and geographic region of seed origin on the length of seeds (Table 1, Figure 1A). For the plants originating from Californian mother plants, a high level of ancestral competition was associated with a minor (1.9% difference in medians) but significant reduction in seed length of offspring plants. The region of seed origin did not affect the weight of mericarps (Table 1). Seed length and mericarp weight were positively correlated for seeds originating from plants with low competition (Spearman's rank correlation, S = 1,06,150, rho = 0.51, P-value < 0.001, see Figure S1), but there was no such correlation for seeds from high competition sites (Spearman's rank correlation, S = 260,600, rho = 0.14, P-value = 0.13). For plants originating from both competition levels, the length of seeds and awns was positively correlated (Spearman's rank correlation, S = 1,733,900, rho = 0.22, P-value < 0.001, see Figure S2). Awns of those mericarps from high competition sites tended to be longer, and there also tended to be an interaction between ancestral competition and region (Table 1, Figure 1B). Plants originating from populations in California produced significantly longer awns (median length = 3.5 cm) than plants from Germany (median length = 3.1 cm). The proportion of germinated seeds from Californian ancestor plants was significantly lower than of seeds from German ancestor plants (37.5% compared to 62.5%).
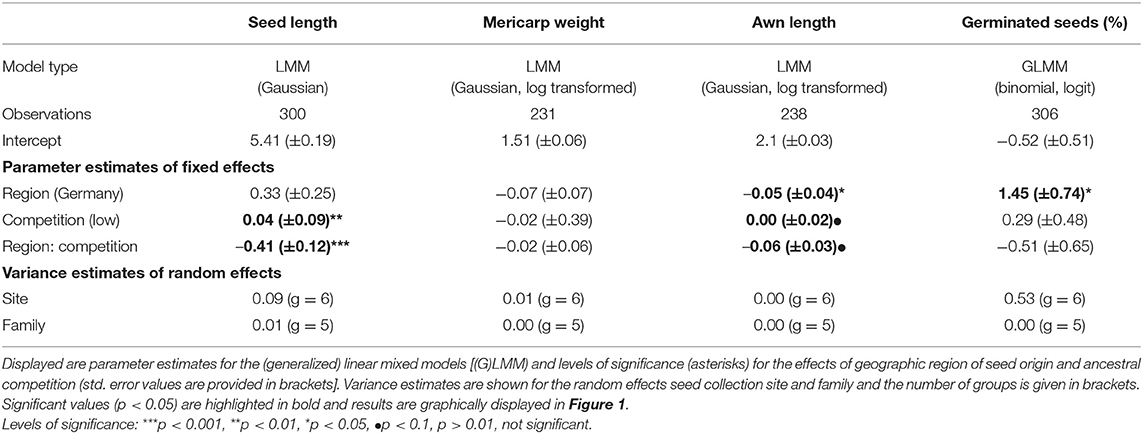
Table 1. Statistical analyses of the effects of geographic region of seed origin and level of ancestral competition on reproductive traits and proportion of germinated seeds of Erodium cicutarium plants (1st laboratory generation).
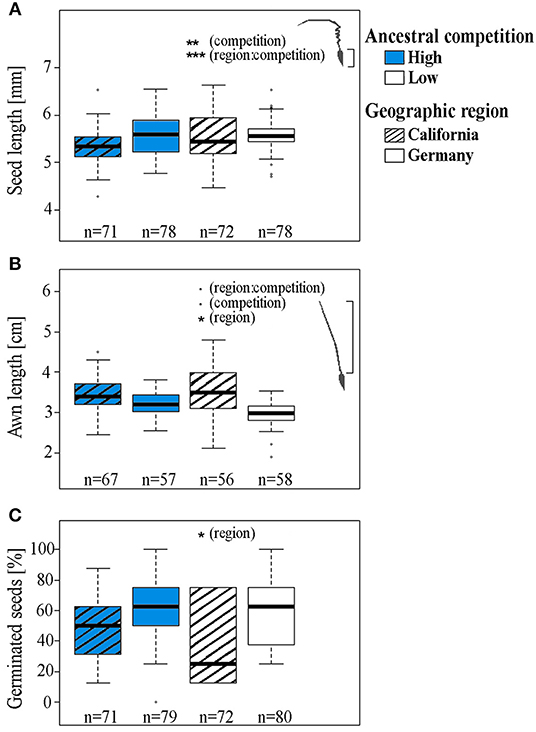
Figure 1. (A) Seed length, (B) awn length (moistened, extended), and (C) proportion of germinated seeds from Erodium cicutarium plants (1st laboratory generation). Data for the proportion of germinated seeds were pooled for all maternal families per region prior to display. Data are shown for two geographic areas of seed origin: California, USA (hatched boxes) and Bavaria, Germany, and two levels of ancestral competition: high (blue boxes), and low. The box and whisker plots show the median (horizontal line), quartiles (boxes), 5 and 95% percentiles (whiskers), and outliers of each data set (open circles). Levels of significance: ***p < 0.001, **p < 0.01, *p < 0.05, •p < 0.1, p > 0.01, not significant. The results of statistical analyses are displayed in Table 1.
In the second plant generation, the interaction of the factors ancestral competition and region of seed origin had a significant impact on the amount of aboveground biomass the plants produced until harvesting (Table 2, Figure 2A). Within the Californian populations, plants originating from high competition sites produced more aboveground biomass (median: 12.59 g) compared to offspring from low competition sites (median: 11.37 g). The number of produced unripe schizocarps until harvest (day 112 for early flowering plants, day 126 for late flowering plants) differed by trend in response to each of the factors ancestral region and competition: plants originating from sites with a high level of competition and from Germany produced on average 2.33 times the number of schizocarps that offspring plants from low competition sites and from California produced (Table 2, Figure 2B). The higher number of schizocarps in offspring plants from Germany corresponds to our observations of first flowering date: Flowering was first observed after 71 days in offspring plants from German sites; the first blossoms on plants from Californian mother plants appeared 14 days later (data not shown). The ratio of ripe to unripe schizocarps (a measure of the progress of phenological development of seeds) differed significantly in relation to ancestral competition; plants from populations that had experienced high levels of competition had a higher ripe to unripe schizocarp ratio at harvesting (median ratio: 0.77) than plants from low competition sites (median ratio: 0.0), indicating a faster life cycle. The number of ripe mericarps and the number of mericarps per schizocarp were neither influenced by ancestral competition, nor geographic region. The foliar concentration of monoterpenes in leaves of plants originating from high ancestral competition sites was on average 2.14 times higher than the foliar monoterpene concentration of plants originating from low competition sites (Table 2, Figure 3A), whereas the sesquiterpene concentration tended to be influenced by geographic region and competition in interaction. Plants from the German populations contained on average 1.86 times higher sesquiterpene concentrations than plants from Californian populations (Table 2, Figure 3B).
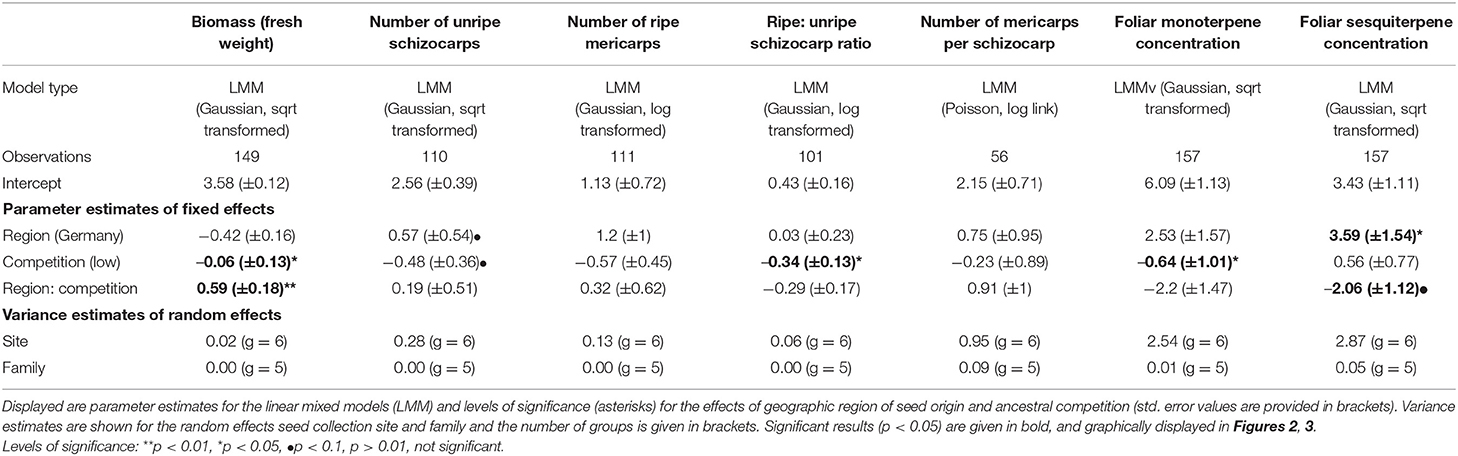
Table 2. Overview of statistical analyses testing the effect of geographic region of seed origin and ancestral level of competition on biomass, reproductive traits and foliar terpenoid content of Erodium cicutarium plants (2nd laboratory generation).
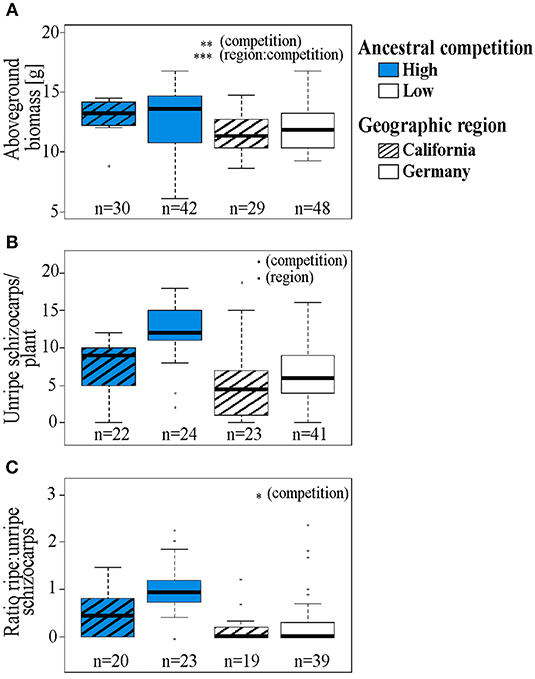
Figure 2. (A) Aboveground fresh biomass, (B) number of unripe (green) schizocarps at harvesting, and (C) ratio of ripe to unripe schizocarps produced until harvesting of Erodium cicutarium plants (2nd laboratory generation). Data are shown for two geographic areas of seed origin: California, USA (hatched boxes) and Bavaria, Germany and two levels of ancestral competition: high (blue boxes), and low. The box and whisker plots show the median (horizontal line), quartiles (boxes), 5 and 95% percentiles (whiskers), and outliers of each data set (open circles). Levels of significance: ***p < 0.001, **p < 0.01, *p < 0.05, •p < 0.1, p > 0.01, not significant. The results of statistical analyses are displayed in Table 2.
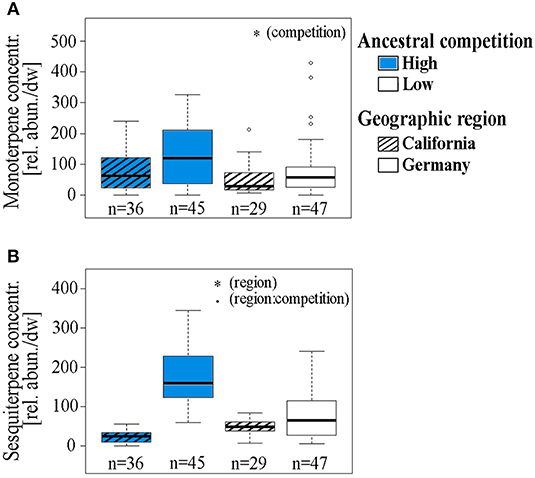
Figure 3. (A) Foliar monoterpene and (B) sesquiterpene concentrations of Erodium cicutarium plants (2nd laboratory generation) displayed as sum of relative compound abundances, normalized by sample dry weight and peak area of the internal standard. Data are shown for two geographic regions of seed origin: California, USA (hatched boxes) and Bavaria, Germany and two levels of ancestral competition: high (blue boxes), and low. The box and whisker plots show the median (horizontal line), quartiles (boxes), 5 and 95% percentiles (whiskers), and outliers of each data set (open circles). Levels of significance: *p < 0.05, •p < 0.1, p > 0.01, not significant. The results of statistical analyses are displayed in Table 2.
The relative proportions of terpenoid concentrations in pooled samples for the allelopathy experiments (Ca, H: 43.07 relative units, De, H: 104.41 r.u., Ca, L: 51.74 r.u., De, L: 41.67 r.u.) were comparable to the mean terpenoid contents for the individual samples, displayed in Figure 3. Aqueous extracts of E. cicutarium leaves significantly affected the germination of E. cicutarium and Z. mays seeds (Table 3). Both E. cicutarium and Z. mays seeds germinated with the highest proportion on water controls, compared to foliar extracts. The seedlings had the highest radicle length and hypocotyl length on water controls. For these seedlings, the length difference of radicles to those of controls was highest for extracts from plants originating from Germany with high ancestral competition, i.e., the extracts which contained the highest terpenoid concentrations. However, the radicle length did not differ significantly between the four extract treatments.
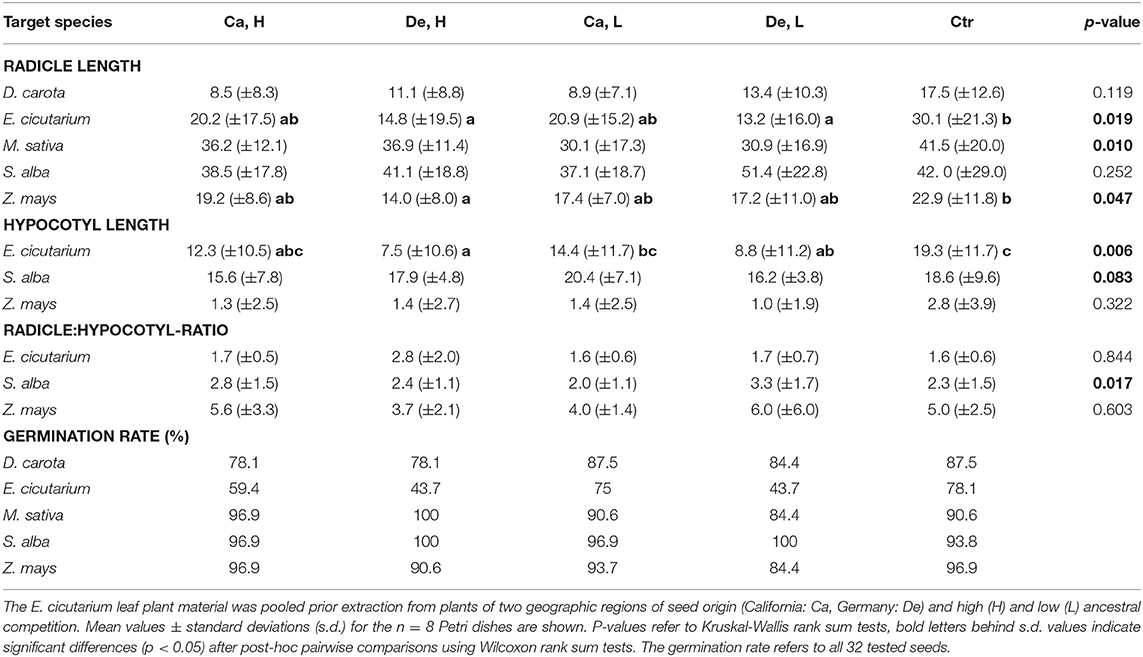
Table 3. Results of allelopathy experiment, testing the effect of Erodium cicutarium aqueous leaf extracts on radicle and hypocotyl length and germination rate of five target species.
Discussion
Our experiments indicate that the level of competition experienced in the field influences fruit morphology, aboveground biomass, proportion of ripe to unripe fruits, and foliar terpenoid concentrations of Erodium cicutarium plants of subsequent generations. Further, we found significant differences in most of these traits among the plants grown under uniform conditions related to the geographic region of seed origin (i.e., California or Germany). Aqueous foliar E. cicutarium extracts exhibit allelopathic function in Z. mays and E. cicutarium (auto-allelopathy).
Ancestral Competition Level and Reproductive Traits
We found that a high level of ancestral competition of E. cicutarium plants was related to shorter seeds produced by the offspring, i.e., the first greenhouse generation. However, the length of awns, which are presumably mainly responsible for seed dispersal and self-burial, was not affected by ancestral competition. Additionally, the effect size in seed length difference was rather small. Thus, our first hypothesis can only partially be supported and further experiments are essential.
Plants stemming from sites with high levels of competition produced more aboveground biomass, in trend an increased number of unripe schizocarps, and the ratio of ripe to unripe schizocarps was significantly higher in these plants. These traits suggest an earlier development and reproduction of plants, whose ancestors experienced high levels of aboveground competition. In previous studies on E. cicutarium including the same German seed families used in this study (corresponding to the 1st greenhouse generation investigated in this study), E. cicutarium originating from high competition populations produced less biomass than those from low competition (Heger et al., 2014; Heger, 2016). Indicators for the velocity of the life cycle as time to first flowering and proportion of unripe fruits at harvesting did not differ consistently among high and low ancestral competition levels. In these previous experiments, all (Heger et al., 2014) or half (Heger, 2016) of the used seeds had been collected in the field.
Geographic Origin and Germination
We found a significantly lower proportion of germinated seeds for offspring plants from the Californian region. In a previous germination experiment with E. cicutarium seeds from Germany, California and Chile (Heger et al., 2018; some of the maternal families used there were included in our experiments), results were similar: seeds from German mother plants germinated fastest and in highest proportions. In those experiments, seeds originating from Californian populations displayed a 2-fold dormancy, which was not the case for German seeds. It is very likely that the same mechanism also slowed down germination in Californian seeds in our experiment. Delayed germination may be an adaptation to the Mediterranean climate (see climatic data of sampling sites in Table S1), which either evolved in the new range, or more likely is carried over from the original source region, i.e., most likely Spain for Californian E. cicutarium populations. The difference in seed length among offspring from high and low competition exposed mother plants was more pronounced in Californian populations, which may indicate a local adaptation to competition.
Monoterpenes and Potential Allelopathy
We found foliar monoterpene concentrations in E. cicutarium to respond to the level of ancestral competition, but foliar sesquiterpenes were responsive to the geographic region of seed origin. Depending on the plant species, mono- and sesquiterpenes may be phytotoxic: monoterpenes released by false rosemary (Conradina canescens, Lamiaceae) (Fischer et al., 1994) and sesquiterpenes released by the invasive bitou bush (Chrysanthemoides monilifera, Asteraceae) (Ens et al., 2009) have been shown to act as allelochemicals. The higher concentration of foliar monoterpenes in plants originating from high competition sites is in line with our second hypothesis. Similar results were shown by Lankau (2012), who found higher concentrations of allelopathic glucosinolates in Brassicaceae plants with high interspecific competition at the source site. In line with our third hypothesis, we found a suppression of radicle growth after treatment with terpenoid-rich E. cicutarium leaf extract on Z. mays and E. cicutarium and a trend in the same direction for M. sativa. Whole leaf extracts were tested in our allelopathy experiment, presumably containing a mixture of different terpenoids and other water-soluble primary and specialized metabolites. In addition to terpenoid concentrations of foliar extracts, other factors may play a major role in the allelopathic potential of leaf extracts. For instance, composition of terpenoids may be different and not all compounds within this class function as equally strong allelochemicals (Singh et al., 2002; Nishida et al., 2005). Furthermore, the allelopathic function of chemical compounds depends highly on the target plant family and species. We tested five different target species of which two responded with significant radicle growth inhibition and a trend for inhibition in a third species was observed. E. cicutarium contains high levels of phenolic compounds (Fecka et al., 2001; Fecka and Cisowski, 2005) in addition to terpenoids, which are also known as allelochemicals (Cheng and Cheng, 2015). It is yet unknown, in which way the concentrations of terpenoids and phenolics are related in E. cicutarium. In our allelopathy experiment, the same number of seeds was placed in each Petri dish. However, phytotoxicity may decrease with increasing plant density by dilution (Weidenhamer et al., 1989). Thus, it remains to be tested if the allelopathic effect of E. cicutarium leaf extracts is overall diminished at sites with very high density of surrounding plants, i.e., high intra- and interspecific competition, even though the plants produce higher amounts of terpenoids. Furthermore, the production of allelopathic compounds depends on various abiotic and biotic factors, such as plant size (Weidenhamer et al., 2019). For the sake of simplicity, the plants that were tested for their allelopathic potential in this study were grown under the same standardized conditions and thus further factors that may influence the allelopathic potential of E. cicutarium remain to be tested.
Seed Origin and Terpenoid Contents
The increased concentration of monoterpenes in plants with high ancestral competition did not lead to a decrease in biomass production and reproductive outcome, opposed to our fourth hypothesis. In contrast, plants with high ancestral competition and higher concentrations of monoterpenes yielded more biomass, produced in trend an increased number of unripe schizocarps and the ratio of ripe to unripe schizocarps was significantly higher in these plants. An increased monoterpene production (= qualitative defense) could result in a diminished investment of the plant to other (quantitative) defense compounds and the ability to allocate more resources to growth and reproduction. Likely candidates of other defense compounds may be flavonoids (Saleh et al., 1983) and phenolic acids (Fecka et al., 2001), which are contained in E. cicutarium at high concentrations. Our results do not allow for a comparison of biochemical costs in relation to biomass and reproductive outcome, as we only measured one (i.e., terpenoids) out of several important compound classes. Furthermore, it remains to be determined, whether sesquiterpenes in E. cicutarium indeed belong to the category of qualitative defense. The attempt to classify terpenoids by cost-dependent categories caused controversy in the past, as it appears to be highly species-specific (Stamp, 2003). The involvement of terpenoids in a broad range of ecological functions, such as defense against herbivores and pathogens, allelopathy, nutrient cycling, and attraction of pollinators (Gershenzon, 1994), may as well point toward qualitative defense. The higher sesquiterpene concentrations in plants with German origin may be explained as an adaptation to the different climate and environment at the geographic origin of the seeds (see Table S1) or may have evolved as defense against specialist herbivores within the native but not the exotic regime (enemy release hypothesis, ERH, Keane and Crawley, 2002). Overall, the variance estimates of the mixed models calculated for the contents of both measured types of foliar terpenoids are comparatively high for the random effect “seed origin” (Table 2), indicating that a high intraspecific diversity of terpenes exists, not only with regard to the tested variables region and source site competition. Future experiments therefore will have to cover a broader range of populations in both ranges and should ideally also include more samples per population. Additionally, other important defense compounds, such as phenolics, should be considered and measured simultaneously with terpenoids.
Conclusions
Our data indicate intraspecific differences in plant traits relating to experienced environmental conditions of the grandparental plant generation of E. cicutarium, such as aboveground competition for light. High levels of competition experienced by ancestral populations were connected to early seed production, more aboveground biomass, and increased concentrations of foliar monoterpenes in the offspring. These differences were accompanied by shorter seeds in the populations from high competition, and the potentially resulting shorter dispersal distance could have enhanced the formation of trait differentiation. Since we selected the collection sites within each region to be as similar as possible in terms of abiotic conditions, with two out of four populations per region being located close to each other (see Table S1), it is very likely that the trait differences within each region are really driven by the level of competition experienced by the populations.
Interestingly, trait differentiation in response to ancestral competition is consistent across the two sampled ranges. The higher monoterpene concentrations found in offspring plants from high competition sites may act as allelochemicals and enhance the competitive ability, but still these plants showed accelerated biomass and fruit production compared to plants from low competition sites. Monoterpenes may act as allelochemicals, as shown for other plant species, and could therefore be a competition avoidance trait, employed by offspring plants from high competition sites. In our allelopathy experiment, we found aqueous E. cicutarium leaf extracts containing the highest monoterpene concentration to inhibit radicle and hypocotyl growth in seedlings of E. cicutarium (auto-allelopathy) and to inhibit radicle growth in Z. mays (Poaceae). Future experiments are however required, to investigate which terpenoids are expressing the allelopathic function and if their function interacts with other metabolites, such as phenolic compounds. In E. cicutarium, sesquiterpenes were found in lower relative concentrations than monoterpenes. They may rather function as antiherbivore or antipathogen defense and may therefore be less important for competition avoidance in E. cicutarium, but linked to the presence or absence of respective antagonists at the geographic origin of ancestor plants. Lower foliar concentrations of sesquiterpenes in our experimental plants originating from California (i.e., an invaded region) may indicate the importance of reduced terpenoid concentrations in the invasion success of the plant. However, these interpretations have to be treated with care, since due to logistic and other constraints our sample size was not very high.
In our experiments, increased monoterpene concentrations were accompanied by an increased production of aboveground biomass, which may be interpreted as an additional trait that could potentially enhance competitive ability. As the function of terpenoids and the biochemical costs for their production in E. cicutarium remain largely unknown and supposedly differ between mono- and sesquiterpenes, additional experiments are essential to gain further clarity. Hence, our results call for more studies investigating the effects of past competition on intraspecific trait variation, and on the invasion success of plants with high intraspecific chemodiversity, such as E. cicutarium.
Data Availability Statement
All analyzed datasets for this study were uploaded to the Knowledge Network for Biocomplexity (KNB) [https://knb.ecoinformatics.org/view/urn:uuid:b7790731-2701-41a4-97e9-7e7970df94ee].
Author Contributions
EE and TH conceived, designed, performed the experiments, and wrote the paper. EE performed chemical analyses, analyzed the data, and prepared the figures and tables.
Funding
TH received financial support for seed collection from the Deutsche Forschungsgemeinschaft (DFG; HE 5893/2-1) and the Bavaria California Technology Center [BaCaTeC, Nr. 9 (2001-1)].
Conflict of Interest
The authors declare that the research was conducted in the absence of any commercial or financial relationships that could be construed as a potential conflict of interest.
Acknowledgments
We thank Caroline Müller (Bielefeld University, Department of Chemical Ecology) for constructive discussion and valuable comments on this manuscript. Lydia Dück assisted in germination experiments and potting of experimental plants.
Supplementary Material
The Supplementary Material for this article can be found online at: https://www.frontiersin.org/articles/10.3389/fevo.2019.00392/full#supplementary-material
References
Baythavong, B. S., Stanton, M. L., and Rice, K. J. (2009). Understanding the consequences of seed dispersal in a heterogeneous environment. Ecology 90, 2118–2128. doi: 10.1890/08-0307.1
Benjamini, Y., and Hochberg, Y. (1995). Controlling the false discovery rate: a practical and powerful approach to multiple testing. J. R. Stat. Soc. Ser. B 57, 289–300. doi: 10.1111/j.2517-6161.1995.tb02031.x
Blackshaw, R. E., and Harker, K. N. (1998). Erodium cicutarium density and duration of interference effects on yield of wheat, oilseed rape, pea and dry bean. Weed Res. 38, 55–62. doi: 10.1046/j.1365-3180.1998.00071.x
Brooks, M. L. (2000). Competition between alien annual grasses and native annual plants in the Mojave Desert. Am. Midland Nat. 144, 92–108. doi: 10.1674/0003-0031(2000)144[0092:CBAAGA]2.0.CO;2
Callaway, R. M., and Ridenour, W. M. (2004). Novel weapons: invasive success and the evolution of increased competitive ability. Front. Ecol. Environ. 2, 436–443. doi: 10.1890/1540-9295(2004)002[0436:NWISAT]2.0.CO;2
Cheng, F., and Cheng, Z. (2015). Research progress on the use of plant allelopathy in agriculture and the physiological and ecological mechanisms of allelopathy. Front. Plant Sci. 6:1020. doi: 10.3389/fpls.2015.01020
Eilers, E. J., Pauls, G., Rillig, M. C., Hansson, B. S., Hilker, M., and Reinecke, A. (2015). Novel set-up for low-disturbance sampling of volatile and non-volatile compounds from plant roots. J. Chem. Ecol. 41, 253–266. doi: 10.1007/s10886-015-0559-9
El-Sayed, A. M. (2019). The Pherobase: Database of Pheromones and semiochemicals. Available online at: http://www.pherobase.com (accessed February 12, 2019).
Ens, E. J., Bremner, J. B., French, K., and Korth, J. (2009). Identification of volatile compounds released by roots of an invasive plant, bitou bush (Chrysanthemoides monilifera spp. rotundata), and their inhibition of native seedling growth. Biol. Invas. 11, 275–287. doi: 10.1007/s10530-008-9232-3
Evangelista, D., Hotton, S., and Dumais, J. (2011). The mechanics of explosive dispersal and self-burial in the seeds of the filaree, Erodium cicutarium (Geraniaceae). J. Exp. Biol. 214, 521–529. doi: 10.1242/jeb.050567
Fecka, I., and Cisowski, W. (2005). Tannins and flavonoids from the Erodium cicutarium herb. Zeitschrift für Naturforschung Sect. B J. Chem. Sci. 60, 555–560. doi: 10.1515/znb-2005-0513
Fecka, I., Kowalczyk, A., and Cisowski, W. (2001). Phenolic acids and depsides from some species of the Erodium genera. Zeitschrift für Naturforschung Sect. C J. Biosci. 56, 943–950. doi: 10.1515/znc-2001-11-1205
Fernandez, C., Monnier, Y., Santonja, M., Gallet, C., Weston, L. A., Prevosto, B., et al. (2016). The impact of competition and allelopathy on the trade-off between plant defense and growth in two contrasting tree species. Front. Plant Sci. 7:594. doi: 10.3389/fpls.2016.00594
Fischer, N. H., Williamson, G. B., Weidenhamer, J. D., and Richardson, D. R. (1994). In search of allelopathy in the Florida scrub - the role of terpenoids. J. Chem. Ecol. 20, 1355–1380. doi: 10.1007/BF02059812
Fiz-Palacios, O., Vargas, P., Vila, R., Papadopulos, A. S. T., and Aldasoro, J. J. (2010). The uneven phylogeny and biogeography of Erodium (Geraniaceae): radiations in the Mediterranean and recent recurrent intercontinental colonization. Ann. Bot. 106, 871–884. doi: 10.1093/aob/mcq184
Francis, A., Darbyshire, S. J., Legere, A., and Simard, M. J. (2012). The biology of Canadian weeds. 151. Erodium cicutarium (L.) L'Her. ex Aiton. Can. J. Plant Sci. 92, 1359–1380. doi: 10.4141/cjps2012-076
Gershenzon, J. (1994). Metabolic costs of terpenoid accumulation in higher plants. J. Chem. Ecol. 20, 1281–1328. doi: 10.1007/BF02059810
Heger, T. (2016). Light availability experienced in the field affects ability of following generations to respond to shading in an annual grassland plant. J. Ecol. 104, 1432–1440. doi: 10.1111/1365-2745.12607
Heger, T., Jacobs, B. S., Latimer, A. M., Kollmann, J., and Rice, K. J. (2014). Does experience with competition matter? Effects of source competitive environment on mean and plastic trait expression in Erodium cicutarium. Perspect. Plant Ecol. Evol. Syst. 16, 236–246. doi: 10.1016/j.ppees.2014.06.002
Heger, T., Nikles, G., and Jacobs, B. S. (2018). Differentiation in native as well as introduced ranges: germination reflects mean and variance in cover of surrounding vegetation. Aob Plants 10:ply009. doi: 10.1093/aobpla/ply009
Jacobs, B. S., and Lesmeister, S. A. (2012). Maternal environmental effects on fitness, fruit morphology and ballistic seed dispersal distance in an annual forb. Funct. Ecol. 26, 588–597. doi: 10.1111/j.1365-2435.2012.01964.x
Johnson, R. H., Hull-Sanders, H. M., and Meyer, G. A. (2007). Comparison of foliar terpenes between native and invasive Solidago gigantea. Biochem. Syst. Ecol. 35, 821–830. doi: 10.1016/j.bse.2007.06.005
Keane, R. M., and Crawley, M. J. (2002). Exotic plant invasions and the enemy release hypothesis. Trends Ecol. Evol. 17, 164–170. doi: 10.1016/S0169-5347(02)02499-0
Kleine, S., and Müller, C. (2011). Intraspecific plant chemical diversity and its relation to herbivory. Oecologia 166, 175–186. doi: 10.1007/s00442-010-1827-6
Kleine, S., and Müller, C. (2013). Differences in shoot and root terpenoid profiles and plant responses to fertilisation in Tanacetum vulgare. Phytochemistry 96, 123–131. doi: 10.1016/j.phytochem.2013.09.018
Klocke, J. A. (1987). Natural plant compounds useful in insect control. ACS Sympos. Ser. 330, 396–415. doi: 10.1021/bk-1987-0330.ch036
Kováts, E. (1958). Gas-chromatographische Charakterisierung organischer Verbindungen. Teil 1: retentionsindices aliphatischer Halogenide, Alkohole, Aldehyde und Ketone. Helvetica Chim. Acta 41, 1915–1932. doi: 10.1002/hlca.19580410703
Lamparski, R., and Wawrzyniak, M. (2005). Effect of water extracts from Geraniaceae plants with adjuvant added on feeding and development of colorado potato beetle Leptinotarsa decemlineata. J. Plant Protect. Res. 45, 115–122. Available online at: http://www.plantprotection.pl/Effect-of-water-extracts-from-Geraniaceae-plants-with-adjuvant-added-on-feeding-and,90228,0,2.html
Lankau, R. A. (2012). Coevolution between invasive and native plants driven by chemical competition and soil biota. Proc. Natl. Acad. Sci. U.S.A. 109, 11240–11245. doi: 10.1073/pnas.1201343109
Loreto, F., Bagnoli, F., and Fineschi, S. (2009). One species, many terpenes: matching chemical and biological diversity. Trends Plant Sci. 14, 416–420. doi: 10.1016/j.tplants.2009.06.003
Moore, B. D., Andrew, R. L., Kulheim, C., and Foley, W. J. (2014). Explaining intraspecific diversity in plant secondary metabolites in an ecological context. New Phytol. 201, 733–750. doi: 10.1111/nph.12526
Nishida, N., Tamotsu, S., Nagata, N., Saito, C., and Sakai, A. (2005). Allelopathic effects of volatile monoterpenoids produced by Salvia leucophylla: inhibition of cell proliferation and DNA synthesis in the root apical meristem of Brassica campestris seedlings. J. Chem. Ecol. 31, 1187–1203. doi: 10.1007/s10886-005-4256-y
Pither, J. (2003). Climate tolerance and interspecific variation in geographic range size. Proc. R. Soc. B Biol. Sci. 270, 475–481. doi: 10.1098/rspb.2002.2275
Post, E., and Stenseth, N. C. (1999). Climatic variability, plant phenology, and northern ungulates. Ecology 80, 1322–1339. doi: 10.1890/0012-9658(1999)080[1322:CVPPAN]2.0.CO;2
R Core Team (2019). R: A Language and Environment for Statistical Computing. Vienna: R Foundation for Statistical Computing, ISBN 3-900051-07-0. Available online at: https://www.r-project.org/.
Radulovic, N., Dekic, M., Stojanovic-Radic, Z., and Palic, R. (2009). Volatile constituents of Erodium cicutarium (L) L'Hérit (Geraniaceae). Cent. Eur. J. Biol. 4, 404–410. doi: 10.2478/s11535-009-0026-0
Rajcan, I., and Swanton, C. J. (2001). Understanding maize–weed competition: resource competition, light quality and the whole plant. Field Crops Res. 71, 139–150. doi: 10.1016/S0378-4290(01)00159-9
Saleh, N. A. M., Elkaremy, Z. A. R., Mansour, R. M. A., and Fayed, A. A. A. (1983). A chemosystematic study of some Geraniaceae. Phytochemistry 22, 2501–2505. doi: 10.1016/0031-9422(83)80148-4
Saul, W. C., Jeschke, J. M., and Heger, T. (2013). The role of eco-evolutionary experience in invasion success. Neobiota 17, 57–74. doi: 10.3897/neobiota.17.5208
Schulz, M., Kussmann, P., Knop, M., Kriegs, B., Gresens, F., Eichert, T., et al. (2007). Allelopathic monoterpenes interfere with Arabidopsis thaliana cuticular waxes and enhance transpiration. Plant Signal. Behav. 2, 231–239. doi: 10.4161/psb.2.4.4469
Schutzenhofer, M. R., and Valone, T. J. (2006). Positive and negative effects of exotic Erodium cicutarium on an arid ecosystem. Biol. Conserv. 132, 376–381. doi: 10.1016/j.biocon.2006.04.031
Singh, H. P., Batish, D. R., and Kohli, R. K. (2002). Allelopathic effect of two volatile monoterpenes against bill goat weed (Ageratum conyzoides L.). Crop Protect. 21, 347–350. doi: 10.1016/S0261-2194(01)00096-5
Stamp, N. E. (1984). Self-burial behavior of Erodium cicutarium seeds. J. Ecol. 72, 611–620. doi: 10.2307/2260070
Stamp, N. E. (1989). Seed dispersal of four sympatric grassland annual species of Erodium. J. Ecol. 77, 1005–1020. doi: 10.2307/2260819
Stamp, N. E. (2003). Out of the quagmire of plant defense hypotheses. Q. Rev. Biol. 78, 23–55. doi: 10.1086/367580
Stojanovic-Radic, Z., Comic, L., Radulovic, N., Dekic, M., Randelovic, V., and Stefanovic, O. (2010). Chemical composition and antimicrobial activity of Erodium species: E. ciconium L., E. cicutarium L., and E. absinthoides Willd. (Geraniaceae). Chem. Papers 64, 368–377. doi: 10.2478/s11696-010-0014-x
Tholl, D. (2006). Terpene synthases and the regulation, diversity and biological roles of terpene metabolism. Curr. Opin. Plant Biol. 9, 297–304. doi: 10.1016/j.pbi.2006.03.014
Thuiller, W., Lavorel, S., Midgley, G., Lavergne, S., and Rebelo, T. (2004). Relating plant traits and species distributions along bioclimatic gradients for 88 Leucadendron taxa. Ecology 85, 1688–1699. doi: 10.1890/03-0148
van Kleunen, M., Weber, E., and Fischer, M. (2010). A meta-analysis of trait differences between invasive and non-invasive plant species. Ecol. Lett. 13, 235–245. doi: 10.1111/j.1461-0248.2009.01418.x
Wawrzyniak, M. (2010). Effect of extracts from Geraniaceae plants on Pieris brassicae. J. Central Eur. Agric. 10, 361–365. Available online at: https://hrcak.srce.hr/index.php?show=clanak&id_clanak_jezik=80021
Weidenhamer, J. D., Hartnett, D. C., and Romeo, J. T. (1989). Density-dependent phytotoxicity: distinguishing resource competition and allelopathic interference in plants. J. Appl. Ecol. 26, 613–624. doi: 10.2307/2404086
Weidenhamer, J. D., Macias, F. A., Fischer, N. H., and Williamson, G. B. (1993). Just how insoluble are monoterpenes? J. Chem. Ecol. 19, 1827–1835. doi: 10.1007/BF00982309
Weidenhamer, J. D., Montgomery, T. M., Cipollini, D. F., Weston, P. A., and Mohney, B. K. (2019). Plant density and rhizosphere chemistry: does marigold root exudate composition respond to intra-and interspecific competition?. J. Chem. Ecol. 45, 525–533. doi: 10.1007/s10886-019-01073-5
Weinig, C. (2000). Differing selection in alternative competitive environments: shade-avoidance responses and germination timing. Evolution 54, 124–136. doi: 10.1111/j.0014-3820.2000.tb00013.x
Wolf, V. C., Gassmann, A., Clasen, B. M., Smith, A. G., and Müller, C. (2012). Genetic and chemical variation of Tanacetum vulgare in plants of native and invasive origin. Biol. Control 61, 240–245. doi: 10.1016/j.biocontrol.2012.01.009
Keywords: resource allocation, specialized metabolites, gas chromatography-mass spectrometry, seed morphology, plant interactions, eco-evolutionary experience
Citation: Eilers EJ and Heger T (2019) Past Competition Affects Offspring Foliar Terpenoid Concentrations, Seed Traits, and Fitness in the Invasive Forb Erodium cicutarium (Geraniaceae). Front. Ecol. Evol. 7:392. doi: 10.3389/fevo.2019.00392
Received: 05 March 2019; Accepted: 01 October 2019;
Published: 17 October 2019.
Edited by:
Juergen Gross, Julius Kühn-Institut, GermanyReviewed by:
Martin James Steinbauer, La Trobe University, AustraliaAlexandra C. U. Furch, Friedrich Schiller University Jena, Germany
Copyright © 2019 Eilers and Heger. This is an open-access article distributed under the terms of the Creative Commons Attribution License (CC BY). The use, distribution or reproduction in other forums is permitted, provided the original author(s) and the copyright owner(s) are credited and that the original publication in this journal is cited, in accordance with accepted academic practice. No use, distribution or reproduction is permitted which does not comply with these terms.
*Correspondence: Elisabeth J. Eilers, ZWxpc2FiZXRoLmVpbGVyc0B1bmktYmllbGVmZWxkLmRl