- 1Quaternary Palaeontology Program, Royal Alberta Museum, Edmonton, AB, Canada
- 2Laboratório de Mastozoologia, Departamento de Zoologia, Instituto de Biociências, Universidade Federal do Estado do Rio de Janeiro (UNIRIO), Rio de Janeiro, Brazil
- 3Jackson Museum of Earth History, University of Texas at Austin, Austin, TX, United States
- 4Museo de Paleontología, Área Académica de Biología, Universidad Autónoma del Estado de Hidalgo, Hidalgo, Mexico
- 5University of Oregon, Earth Sciences Department, Eugene, OR, United States
- 6Programa de Pós-graduação em Biodiversidade e Biologia Evolutiva, Centro de Ciências da Saúde, Instituto de Biologia, Campus Ilha do Fundão, Universidade Federal do Rio de Janeiro (UFRJ), Rio de Janeiro, Brazil
Interest in the origin and evolution of Equus dates back to over a century, but there is still no consensus on the definition of the genus or its phylogenetic position. We review the placement of Equus within several phylogenetic frameworks and present a phylogenetic analysis of derived Equini, including taxa referred to Equus, Haringtonhippus, Dinohippus, Astrohippus, Hippidion, and Boreohippidion. A new, morphology-based phylogenetic tree was used as an initial hypothesis for discussing what taxa Equus encompasses, using four criteria previously used to define the genus category in mammals: phylogenetic gaps, uniqueness of adaptive zone, crown group definition, and divergence time. According to the phylogenetic gaps criterion, Equus encompasses clade 6 (Ha. francisci = E. francisci, E. conversidens, E. quagga, E. hemionus, E. mexicanus, E. ferus, E. occidentalis, and E. neogeus) based on morphological synapomorphies. Equus is assigned to clade 6, or possibly clade 7, according to the uniqueness of adaptive zone criterion. The crown group criterion places Equus at clade 6. Based on the time-calibrated phylogeny of Equini, the divergence time criterion suggests that Equus encompasses clade 9. This clade comprises all taxa traditionally assigned to Equus analyzed in our study, including the eight taxa listed above as well as E. stenonis, E. idahoensis, and E. simplicidens; the latter two are sometimes referred to the subgenus Plesippus and the former to the subgenus Allohippus. With the exception of the divergence time criterion, the results of our evaluation are congruent in identifying clade 6 as the most suitable position for Equus. The taxonomic implications of delimiting Equus to clade 6 in our phylogenetic tree include elevation of Allohippus and Plesippus to generic rank, assignment of a new genus to “Dinohippus” mexicanus, and synonymy of Haringtonhippus with Equus.
Introduction
The origin and evolution of the genus Equus has been of particular interest to paleontologists for over a century (e.g., Marsh, 1879; Gidley, 1907; Matthew, 1924, 1926; Stirton, 1940, 1942; Lance, 1950; Dalquest, 1978, 1988; Bennett, 1980; MacFadden, 1984; Azzaroli, 1988, 1992; Hulbert, 1989; Prado and Alberdi, 1996; Kelly, 1998; MacFadden and Carranza-Castañeda, 2002). There are several evolutionary hypotheses for the genus and the definition of the generic name across those hypotheses has varied. Since the 1980s, discussions concerning the phylogenetic position of the genus have centered primarily on the position of some named species of Dinohippus with respect to Equus (e.g., Bennett, 1980; MacFadden, 1984; Azzaroli, 1988, 1992; Hulbert, 1989; Prado and Alberdi, 1996; Kelly, 1998). In that sense, our understanding of what constitutes the genus has been relatively stable, with discussions of taxonomy restricted to the base of the tree. In contrast, a recent molecular study suggested a taxonomic scheme that departs from previous taxonomies in that E. francisci, a species previously nested within Equus, is placed in a new genus, Haringtonhippus (Heintzman et al., 2017). That interpretation implicitly constrains the definition of the genus to the crown group and has potential implications for the taxonomy of horses traditionally assigned to Equus. In this study, we review the use of the generic name Equus within several phylogenetic frameworks and outline four criteria previously used to define a genus. We then examine the concept and contents of Equus based on a new phylogenetic analysis of derived Equini given those criteria. As is the case with any phylogenetic hypothesis, we acknowledge that the tree we present will be revised as new data and new specimens are studied. Nonetheless, our phylogenetic results offer a working hypothesis for discussing distinct paradigms for understanding higher level taxonomy, particularly in regards to the definition of the genus Equus.
Contextual Framework
Under a Linnaean taxonomic scheme, Equus is included within the subfamily Equinae and the tribe Equini (MacFadden, 1992). Equus is hypothesized to have originated in North America (e.g., Bennett, 1980; MacFadden, 1984; Azzaroli, 1988, 1992; Hulbert, 1989; Prado and Alberdi, 1996; Kelly, 1998; MacFadden and Carranza-Castañeda, 2002). Some early phylogenetic hypotheses proposed that Equus originated from derived species of Pliohippus, within the subgenus Astrohippus (Osborn, 1918; Stirton, 1940). Later studies separated derived species of Pliohippus into two distinct genera, Astrohippus and Dinohippus (Quinn, 1955), and proposed several hypotheses for the origin of Equus. Some studies suggested that Equus evolved from a species of Dinohippus, such as D. mexicanus or D. leidyanus (e.g., Lance, 1950; Webb, 1969; Bennett, 1980; MacFadden, 1984; Azzaroli, 1988, 1992). Others posited a polyphyletic origin from both Astrohippus and Dinohippus (Dalquest, 1978) or from a separate genus, such as Eoequus (Quinn, 1955), a taxon later considered a junior synonym of Protohippus (Hulbert, 1988).
Morphological phylogenetic analyses conducted since the 1980s support the close phylogenetic affinity of derived members of Dinohippus, such as D. mexicanus, to early representatives of Equus, including E. simplicidens (Bennett, 1980; Hulbert, 1989; Prado and Alberdi, 1996; Kelly, 1998). Some of these studies identified “D.” mexicanus as the sister group of Equus (Kelly, 1998). Other studies suggested including “D.” mexicanus within Equus (Prado and Alberdi, 1996) or even including “Dinohippus” s.l. within Equus (Hulbert, 1989). Regardless of placement, the debate over the delimitation of “Equus” has been largely along the stem of the equid tree. Branches of Plio-Pleistocene Equini taxa, other than Hippidion, have traditionally been considered species of Equus.
In contrast to previous studies, the recent naming of a new genus (Haringtonhippus; Heintzman et al., 2017) on the basis of molecular data and estimated divergence times for extant and some fossil equids has potentially significant taxonomic implications for branches of the equid tree. Specifically, the study constrained the definition of Equus to the crown group (Heintzman et al., 2017), implicitly excluding many stem-group species from Equus without explicitly assigning them to any other genus. In that context, we saw an opportunity to explore a deeper philosophical question about the criteria for defining genera, and how those criteria bear on the placement of Equus and the validity of taxa traditionally referred to Equus.
Definition and Delimitation of Mammalian Genera
The literature on species concepts and naming of species is extensive (e.g., Mayr, 1940, 1942, 1963; Simpson, 1961; Ghiselin, 1966, 1974; Van Valen, 1976; Paterson, 1978, 1985; Wiley, 1978; Cracraft, 1983, 1997; de Queiroz and Donoghue, 1988; Templeton, 1989; Mayden, 1997, 2002; de Queiroz, 1998, 2007; Groves, 2004). In contrast, discussions on higher Linnaean taxonomic categories (e.g., the genus) are less numerous. Nonetheless, different authors discussed the meaning and relevance of the genus and proposed various criteria for recognizing and delimiting this higher taxonomic category in a consistent way (e.g., Mayr, 1950, 1969; Cain, 1956; Michener, 1957; Inger, 1958; Simpson, 1961; Hennig, 1966; Dubois, 1987, 1988; Groves, 2001, 2004; Vences et al., 2013). We note that some authors have discussed limitations of the Linnaean classification system and proposed to abandon it (e.g., de Queiroz and Gauthier, 1992; Ereshefsky, 2001; Zachos, 2011) or to combine it with different approaches (Kuntner and Agnarsson, 2006). Therefore, new approaches to nomenclature have been advanced in recent decades (e.g., Papavero et al., 2001; Béthoux, 2007; Cantino and de Queiroz, 2010), but they have not been fully integrated across the Tree of Life. As a result, the Linnaean system continues to form the primary framework used to study and communicate about past and present biodiversity (Vences et al., 2013), particularly in regard to the binomial name (genus and species).
The only widely accepted criterion for delimiting a genus or other higher taxonomic categories is monophyly (Hennig, 1966; Mayr, 1969; Groves, 2001, 2004; Vences et al., 2013). Only monophyletic groups that are well-supported should be named as Linnaean taxa (Vences et al., 2013). Other criteria previously applied to delimitation of extant and extinct genera of mammals are: (1) phylogenetic gaps, (2) uniqueness of adaptive zone, (3) crown group definition, and (4) divergence time (Hennig, 1966; Mayr, 1969; Groves, 2001, 2004; Vences et al., 2013). Some authors advocate for the use of two or more of these criteria, in addition to monophyly, to delimit genera and other higher taxa (e.g., Hennig, 1966; Mayr, 1969), and summary of these criteria is as follows.
Phylogenetic Gaps
The identification of phylogenetic gaps is a criterion that has been used for delimiting genera as well as other higher taxonomic categories (Mayr, 1969). The gaps between taxa and the relative size of those gaps are the result of evolutionary processes (e.g., speciation, extinction, evolutionary and adaptive radiations, and unequal rates of evolution; Mayr, 1969). Specifically, Mayr (1969) defined the genus as “a taxonomic category containing a single species, or a monophyletic group of species, which is separated from other taxa of the same rank [other genera] by a decided gap” (Mayr, 1969, p. 92). In the context of phylogenetic analyses, the gaps between taxa can be measured by the number of synapomorphic traits. In order to facilitate information retrieval and limit redundancy in taxonomic classifications, Mayr (1969) suggested the size of the gap could vary depending on the size of the taxon.
Uniqueness of Adaptive Zone
Simpson (1944) proposed the concept of adaptive zone as a key component of evolutionary change. An adaptive zone corresponds to a particular mode of life or a unique ecological situation (e.g., Simpson, 1944, 1953; Mayr, 1950, 1969; Wood and Collard, 1999; Vences et al., 2013). Under this concept, the occupation of a new adaptive zone by a taxon results in significant evolutionary change (Simpson, 1944). Some authors suggest that this evolutionary change should be reflected at one or more higher categories in the taxonomic classification (Mayr, 1969). Under this perspective, the difference in the occupation of an adaptive zone contributes to the width and the sharpness of phylogenetic gaps between taxa (Mayr, 1969). Therefore, a genus is considered to consist of a species or group of species of common ancestry that occupy a different adaptive zone from the one occupied by species of another genus (Mayr, 1950, 1969).
Crown Group Definition
With the advent of phylogenetic systematics the definition of taxa shifted to a nominalist perspective (de Queiroz, 1994). The essentialist perspective starts from the assumption that the taxon exists, then tries to discover its essential traits, and then refers all organisms with these traits to the taxon in question (de Queiroz, 1994). The nominalist perspective instead assumes that the limits of named taxa are arbitrary conventions, and then proceeds to spell these conventions out. Thus, phylogenetic nomenclature defines taxon names explicitly by anchoring them to defined points on the phylogenetic Tree of Life (de Queiroz, 1994). For example, Mammalia has been defined as the last common ancestor of monotremes and therians plus all descendants of that ancestor (Rowe, 1988; Rowe and Gauthier, 1992). This is a crown group definition of Mammalia (de Queiroz, 1994). In the case of Equus, the last common ancestor of all extant species assigned to Equus and all descendants of that ancestor, is the crown group definition of the genus.
Divergence Time
Some researchers suggest that a taxonomic arrangement above the species level should ideally not only provide information about evolutionary relationships, but should also reflect the approximate divergence times of the different taxonomic ranks (e.g., Hennig, 1966; Avise and Johns, 1999; Groves and Grubb, 2011). In the particular case of the genus, Groves (2001, 2004) and Groves and Grubb (2011) indicated that the cutoff point for assigning generic status to monophyletic groups of species could be placed at about the Miocene-Pliocene boundary (4–7 Ma). This cutoff point is based on a principle of least violence (which aims at preserving as many traditional genera as possible) and a survey of mammals whose fossil record or molecular divergence estimates are well-known (Groves, 2001, 2004). Under the divergence time criterion, species are regarded as distinct genera if they diverged well-before the Miocene-Pliocene boundary. This approach was intended to make the delimitation of genera a more objective endeavor (Groves, 2001, 2004; Groves and Grubb, 2011).
What is Equus? Delimitation of the Genus Based on Four Criteria
The question “What is Equus?” is a philosophical one that ultimately relates to the evolutionary paradigm under which individual researchers are operating and the research questions that are being asked. In this sense, the question we pose in the title of the paper may have different answers depending on the paradigm under consideration. What Equus is, under these varying paradigms, has implications for how we communicate knowledge about the evolutionary and taxonomic history of horses. Perhaps the more valuable question is not “What is Equus?,” but rather “How variable is the taxonomic content of Equus in a given phylogenetic tree, under very distinct paradigms for understanding higher-level taxonomy?” Therefore, we conducted a phylogenetic analysis of derived Equini and examined the placement of the name “Equus” within the resultant phylogenetic tree given the four criteria discussed above for delimiting extant and extinct genera of mammals.
Phylogenetic Analysis
We performed a phylogenetic analysis of derived Equini using a matrix of 32 morphological characters and 21 Equini taxa, including Astrohippus stocki, Boreohippidion galushai, Dinohippus leardi, D. leidyanus, D. interpolatus, D. mexicanus, Equus conversidens, E. ferus, E. hemionus, E. idahoensis, E. mexicanus, E. neogeus, E. occidentalis, E. quagga, E. simplicidens, E. stenonis, Haringtonhippus francisci, Hippidion saldiasi, Hi. principale and two outgroup taxa, Acritohippus stylodontus, and Pliohippus pernix (Table 1). Our study sample included holotype and referred specimens (Table A1). We gathered data from the literature for E. idahoensis (Scott, 2005) and E. stenonis (Athanassiou, 2001; Palombo and Alberdi, 2017); all other specimens in our study were directly examined by us (Table A1). We analyzed the holotype and referred specimens of E. conversidens separately from the holotype of Ha. francisci (= E. francisci), as they are considered distinct taxa in some studies (e.g., Lundelius and Stevens, 1970; Dalquest, 1979; Scott, 1996; Azzaroli, 1998; Bravo-Cuevas et al., 2011; Barrón-Ortiz et al., 2017; Priego-Vargas et al., 2017). We note, however, that some researchers considered E. conversidens a senior synonym of Ha. francisci (= E. francisci) (Dalquest and Hughes, 1965), or a nomen dubium (e.g., Winans, 1985, 1989; Heintzman et al., 2017). Equus ferus is represented in our study by a sample of caballine equids of late Pleistocene age, some of which were previously referred to this taxon (Barrón-Ortiz et al., 2017). For E. hemionus and E. quagga we studied the remains of wild animals. The phylogenetic analysis was performed using TNT 1.1 (Goloboff et al., 2008) with the implicit enumeration option (exhaustive search), using equal weighting for the characters, and without a collapsing rule. We treated all characters as unordered in the analysis reported here. Two additional analyses (one without a collapsing rule and another with collapsing rule 1) that differed by including ordering of characters 1, 29, 30, and 31 break down the distinction between clades 12, 13, 14, and 17 (Figure S1), but leave the topology of the other clades intact (as shown in Figures 1, 2). We used the strict (= Nelsen) consensus option to calculate the consensus tree.
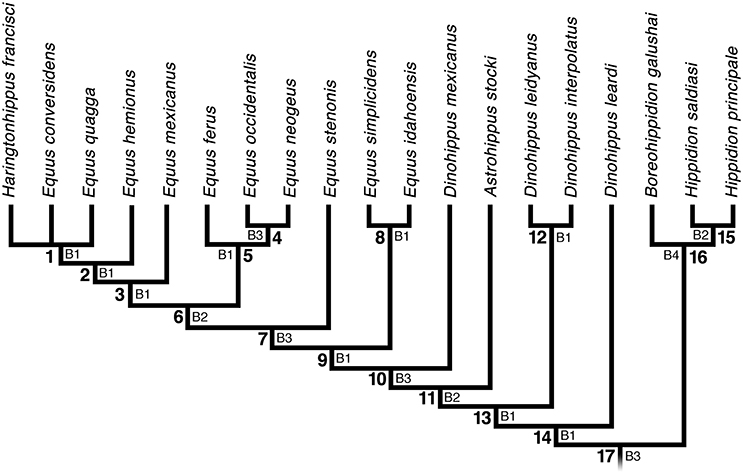
Figure 1. Strict consensus of three equally most parsimonious cladograms of 85 steps, consistency index (CI) of 0.57 and retention index (RI) of 0.80 using a matrix of 32 morphological characters and 21 Equini taxa (Table 1). Numbers beside nodes indicate Bremer support values. Outgroups (Acritohippus stylodontus and Pliohippus pernix) are not shown in the figure. Characters and character states are described in the Appendix.
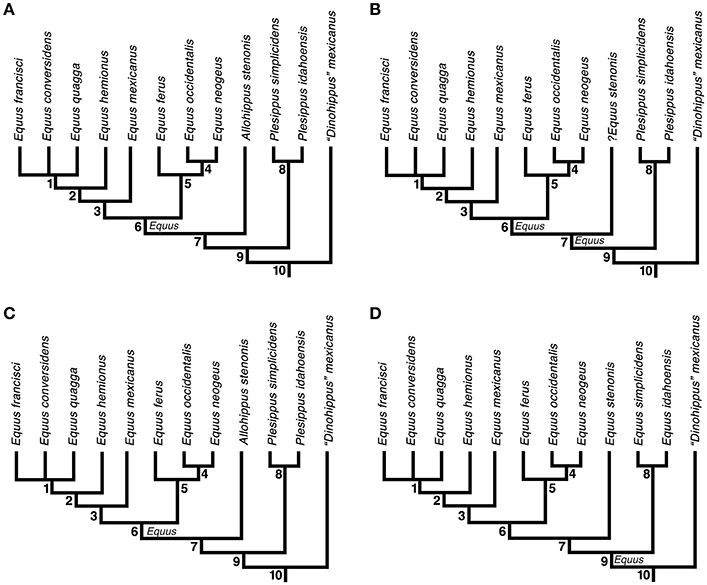
Figure 2. Taxonomic position of Equus in the strict consensus tree (Figure 1) based on four explicit criteria (in addition to monophyly) previously used to delimit mammalian genera: phylogenetic gaps (A), uniqueness of adaptive zone (B), crown group definition (C), and divergence time (D).
The phylogenetic analysis resulted in three equally most parsimonious trees of 85 steps, consistency index (CI) and retention index (RI) of 0.57 and 0.80, respectively. The strict consensus tree is shown in Figure 1. Of particular relevance to the present study are the phylogenetic relationships among Dinohippus mexicanus, Haringtonhippus francisci, and species of Equus. Our phylogenetic analysis identified Dinohippus as a paraphyletic group, and “D.” mexicanus as the sister group to the clade including all species of Equus and Ha. francisci. The sister group relationship of “D.” mexicanus and Equus is consistent with some previously proposed phylogenetic hypotheses (Prado and Alberdi, 1996; Kelly, 1998). Our results also suggests that E. idahoensis, E. simplicidens, and E. stenonis lie outside of crown group Equus. This pattern is consistent with the morphometric analysis of Eisenmann and Baylac (2000), but disagrees with the phylogenetic analysis of Bennett (1980).
Haringtonhippus francisci is nested within crown group Equus in our phylogenetic tree, forming a polytomy with E. conversidens and E. quagga (Figure 1). Haringtonhippus francisci (= E. francisci) is situated in the crown group in the morphological phylogenetic analysis of Bennett (1980). A closer phylogenetic relationship of Ha. francisci (= E. francisci) to extant Equus than primitive species of the genus, such as E. simplicidens (= E. shoshonensis) and E. stenonis, is also indicated by cranial proportions (Eisenmann and Baylac, 2000). Ancient mitochondrial DNA analyses suggested that the lineage which was later named Haringtonhippus was the sister species to extant and fossil caballine equids (Weinstock et al., 2005; Orlando et al., 2008; Vilstrup et al., 2013; Der Sarkissian et al., 2015; Barrón-Ortiz et al., 2017). In contrast to these results, a recent genomic analysis concluded that Ha. francisci lies outside of crown group Equus (Heintzman et al., 2017), but its relationship to E. simplicidens and E. stenonis was not studied, as molecular data for these species are presently unknown. To evaluate the consistency between our phylogenetic results and the genomic study by Heintzman et al. (2017), we performed a second analysis in which Ha. francisci was constrained to lie outside of crown group Equus. This analysis resulted in eleven equally most parsimonious trees of 91 steps; 6 steps longer than the most parsimonious trees that we obtained in the unconstrained analysis. In eight of the 11 equally most parsimonious trees, Ha. francisci was the sister group of crown Equus, whereas in the three remaining trees Ha. francisci was the sister group of the clade formed by E. idahoensis + crown Equus. The discrepancy between the morphological and genomic analyses with regards to the phylogenetic position of Ha. francisci is notable, and needs to be investigated in further studies.
Extent of Equus Based on Four Criteria for Delimiting Genera
Phylogenetic Gaps
Application of this criterion to our phylogenetic tree (Figure 1) suggests that the name Equus best encompasses clade 6 on the basis of the number of morphological synapomorphies (Figure 2A). This taxonomic arrangement excludes E. stenonis, E. idahoensis, E. simplicidens, and “Dinohippus” mexicanus from the genus and renders Haringtonhippus as a junior synonym of Equus. There are six synapomorphies for clade 6 (Table A2). In contrast, clade 7 (which includes E. stenonis in addition to the taxa in clade 6), and clade 10 (which includes “D.” mexicanus, all taxa traditionally assigned to Equus, and Haringtonhippus) each possess five synapomorphies (Table A2). Equus idahoensis and E. simplicidens have at times been referred to Plesippus, at either the generic or subgeneric rank (Eisenmann and Baylac, 2000). Similarly, E. stenonis has been referred to Allohippus at the subgeneric rank (Eisenmann and Baylac, 2000), although we note that some researchers consider Allohippus a nomen dubium (Azzaroli, 1992). Under the phylogenetic gaps criterion, Allohippus and Plesippus should be elevated to generic rank. Furthermore, “D.” mexicanus should be assigned to a new genus, and Haringtonhippus synonymized with Equus.
Uniqueness of Adaptive Zone
An adaptive zone is defined in the literature as a particular mode of life or a unique ecological situation (e.g., Simpson, 1944, 1953; Mayr, 1950, 1969; Wood and Collard, 1999; Vences et al., 2013). This criterion has been used in the definition of our own genus, Homo (Wood and Collard, 1999; Collard and Wood, 2007; Holliday, 2012; Antón et al., 2014). Specializations in body size and shape, locomotor behavior, rate, and pattern of development, among other traits, are argued to have allowed Homo to play a unique ecological role relative to other hominins (e.g., Leakey et al., 1964; Tobias, 1991; Wood and Collard, 1999; Collard and Wood, 2007; Holliday, 2012; Antón et al., 2014). Therefore, these traits have been considered important in the delimitation of the genus Homo by some researchers (e.g., Leakey et al., 1964; Tobias, 1991; Wood and Collard, 1999; Collard and Wood, 2007; Antón et al., 2014; Wood, 2014), although we note that a consensus on the definition of the genus has not been reached (Wood and Collard, 1999; Collard and Wood, 2007; Holliday, 2012; Antón et al., 2014; Wood, 2014).
In this context, the unique mode of life of extant equids could be defined as that of ungulate mammals that are adapted to live in generally open, arid habitats and that can thrive on low-quality, high-fiber foods such as grasses and other coarse and tough vegetation (Janis, 1976, 1988; Mihlbachler et al., 2011; Rubenstein et al., 2016; Schoenecker et al., 2016). Potential morphological adaptations for this mode of life comprise modifications of the locomotory and digestive systems. Possible locomotor adaptations to living in open habitats include the elongation of distal autopodial bones as well as the development of monodactyly and the reduction or loss of metapodials II and IV (Simpson, 1951; Shotwell, 1961; Janis and Wilhelm, 1993); however, we note that alternative explanations for digit reduction have been proposed (Thomason, 1986; Biewener, 1998; McHorse et al., 2017). The enhanced development of the stay-apparatus, which allows the individual to conserve energy while standing, is also potentially an adaptation to living in open habitats (Hermanson and MacFadden, 1992, 1996). Potential adaptations of the digestive system, particularly the dentition, to feeding on low-quality, high-fiber vegetation in open environments include increased crown-height of cheek teeth and incisors (Janis, 1976, 1988; Damuth and Janis, 2011; Mihlbachler et al., 2011; Schoenecker et al., 2016), increased enamel complexity (Famoso and Davis, 2014; including increased implications of the occlusal enamel [(Gromova, 1949; Simpson, 1951; Rensberger et al., 1984; Eisenmann and David, 1990; Kaiser, 2002)]), elongation of the protocones of the upper molars and premolars (Eisenmann, 1982; Guadelli and Prat, 1995), increased separation of the metastylid and metaconid, and enlargement of the metastylid to the point of being equal or subequal in size to the metaconid in the lower molars (MacFadden and Carranza-Castañeda, 2002).
The phylogenetic result reported here indicates that the locomotory and dental traits mentioned above did not appear at the same time. Three of these morphological traits are synapomorphies for Clade 6: oval protocone outline on P2 (character 22, state 1); oblong protocone outline on P3-P4 (character 23, state 5); and a high, well-developed intermediate tubercle, which is key in the development of the stay apparatus (character 30, state 2) (Table A2). Three of the remaining morphological traits are synapomorphies for Clade 7: pli-protoloph and/or pli-hypostyle common and persistent in the upper molars (character 18, state 1); oblong protocone outline on M1-2 (character 24, state 3); and metaconid and metastylid persistently well-separated from each other on p3-m3 (character 27, state 2). Metapodials II and IV reduced to less than half the length of metapodial III (character 31, state 1) is a synapomorphy for clades 10 and 16 (Table A2). Absence of metapodials II and IV (character 31, state 2) is a synapomorphy for clade 4 (Table A2). An oblong protocone outline in the M3 (character 25, state 2), and a metaconid and metastylid of equal or subequal size in the lower molars (character 28, state 1) are synapomorphies of clade 11 (Table A2). An unworn molar crown height > 60 mm (character 19, state 1) is a synapomorphy of clade 14 (Table A2). Based on the position of the majority of purported, adaptive zone-related characteristics, Equus is assigned in the phylogenetic tree to clade 6, or possibly clade 7, under the adaptive zone criterion (Figure 2B). Under this paradigm, Haringtonhippus is considered a synonym of Equus, Plesippus is elevated to generic rank (the same would apply to Allohippus if Equus were delimited to clade 6), and “D.” mexicanus should be assigned to a new genus.
Crown Group Definition
Under this criterion Equus is defined as the most recent common ancestor of all extant species assigned to Equus, and all descendants of that ancestor. In our phylogenetic tree, a crown-group definition of Equus is constrained to clade 6 (Figure 2C). This clade includes the extant taxa E. quagga, E. hemionus, and E. ferus, which represent each lineage of extant Equus (zebrines, hemionines [including asses], and caballines, respectively) as well as four extinct taxa (E. conversidens, E. occidentalis, E. neogeus, and Ha. francisci). This taxonomic arrangement results in the synonymy of Haringtonhippus with Equus and excludes “D.” mexicanus and both plesippine and stenonine equids from the genus. The latter two taxa would then be best assigned to Plesippus and Allohippus, respectively.
Divergence Time
The divergence time criterion states that species should be regarded as distinct genera if they diverged well-before the Miocene-Pliocene boundary (Groves, 2001, 2004; Groves and Grubb, 2011). Application of the time depth criterion to the time-calibrated phylogeny of Equini (Figure 3) retains the traditional taxonomic arrangement of Equus. Clade 9, which comprises E. idahoensis, E. simplicidens, and the remaining taxa traditionally assigned to Equus, is identified as having originated within the Pliocene (Figure 3). Therefore, under the divergence time criterion, taxa within clade 9 should be assigned to the same genus (i.e., Equus; Figure 2D). In contrast, “D.” mexicanus is identified to have originated well-before the Miocene-Pliocene boundary (Figure 3) and should be assigned to a new genus (Figure 2D).
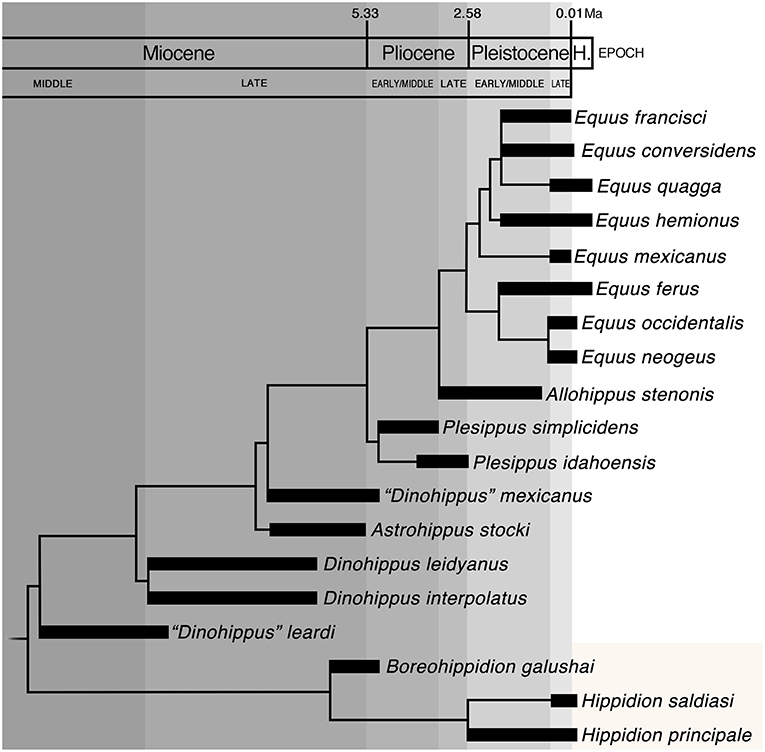
Figure 3. Time-calibrated phylogeny of Equini based on fossil occurrences and the most parsimonious cladogram of 85 steps, consistency index (CI) of 0.57 and retention index (RI) of 0.80 using a matrix of 32 morphological characters and 21 Equini taxa (Table 1). Outgroups (Acritohippus stylodontus and Pliohippus pernix) are not shown in the figure. H., Holocene.
The time-calibrated phylogeny (Figure 3) is, of course, based on fossil occurrences. An independent assessment of divergence times for some Equini taxa, including crown group Equus, Haringtonhippus, and Hippidion, is provided by molecular analyses (Der Sarkissian et al., 2015; Heintzman et al., 2017). These analyses place the divergence time between the lineage leading to Haringtonhippus and that leading to crown group Equus at ~4.1–5.7 Ma (Heintzman et al., 2017). This estimate spans the Miocene-Pliocene boundary, which is currently recognized at 5.33 Ma (Cohen et al., 2013) and is in the upper range of the cutoff interval (4–7 Ma) proposed by Groves (2001, 2004). Molecular estimates of the time of divergence between Hippidion and Haringtonhippus + Equus (~5.2–7.7 Ma) overlap the lower range of the 4–7 Ma cutoff interval (Der Sarkissian et al., 2015; Heintzman et al., 2017). Based on these estimated divergence times, Haringtonhippus and potentially Hippidion should be synonymized with Equus under the divergence time criterion.
Discussion
Delimitation of the genus Equus depends on at least two important factors: (1) identifying well-supported phylogenetic hypotheses, and (2) identifying which clade in a given phylogenetic tree should be considered to comprise the genus Equus. Our study infers the first factor and emphasizes the second by evaluating the consistency in the content of “Equus” across four explicit criteria (phylogenetic gaps, uniqueness of adaptive zone, crown group definition, and divergence time) previously used to delimit genera. Ideally, discussions of what “Equus” is should also consider taxonomic stability, except where evidence suggests that some aspect of a traditional classification is fundamentally flawed from an evolutionary perspective.
Application of the four criteria for delimiting genera results in slightly different positions for the generic name on the phylogenetic tree (Figure 2). Under the phylogenetic gaps criterion Equus should be equated with clade 6 based on the number of morphological synapomorphies (Figure 2A). The same is true for the crown group criterion, as extant taxa of Equus here analyzed (E. ferus, E. hemionus, and E. quagga) fall within clade 6 (Figure 2C). The adaptive-zone criterion places Equus at clade 6 or possibly clade 7, depending on how the characters are evaluated in relation to the adaptive zone (Figure 2B). At a minimum, clade 6 is supported by morphological synapomorphies related to the unique mode of life of extant equids, which represent adaptations for living in open, generally arid, habitats characterized by low-quality, high-fiber foods such as grasses and other coarse vegetation (Janis, 1976, 1988; Mihlbachler et al., 2011; Rubenstein et al., 2016; Schoenecker et al., 2016). The divergence time criterion suggests that Equus encompasses clade 9 (Figure 2D), based on the time-calibrated phylogeny of Equini (Figure 3).
With the exception of the divergence time criterion, the results of our evaluation are overall congruent in identifying clade 6 as the most suitable position of the genus Equus. We note that the aim of the divergence time criterion is to provide an operational definition of genera that incorporates time-depth information (Groves, 2001, 2004; Groves and Grubb, 2011). Nevertheless, whether a time interval of 4–7 Ma is a reasonable cutoff point for all taxonomic groups of mammals is debatable, given the disparate evolutionary rates for different groups of mammals (Carroll, 1998). Moreover, the objectivity of this criterion breaks down for clades that diverged very close to the Miocene-Pliocene boundary, as is potentially the case for Haringtonhippus and the crown group of Equus (Heintzman et al., 2017). In such cases, the taxonomist has to decide whether to keep the sister taxa in one genus or split them into separate genera. Another point to consider about the divergence time criterion is that it relies on the availability of either a very dense fossil record, or very precise molecular divergence estimates (often calibrated by a rather dense fossil record). The fossil record of Neogene equids is particularly dense (MacFadden, 1992). Therefore, the discrepancies between the time-calibrated phylogeny of Equini presented here (Figure 3) and the molecular divergence estimates for Haringtonhippus and Hippidion in two recent molecular analyses (Der Sarkissian et al., 2015; Heintzman et al., 2017) are surprising. Resolution of these differences is beyond the scope of the present study, but this is a topic that should be investigated in future studies. Lastly, the divergence time criterion is designed for extant species and is generally difficult to apply to past biodiversity.
The remaining criteria (phylogenetic gaps, adaptive zone, and crown group) consistently support a clade 6 position for the generic name, with less support for a clade 7 position (in the case of the adaptive zone criterion). We found the phylogenetic gaps and crown group criteria for delimiting genera to be more readily evaluated than the adaptive zone criterion. The broad definition of an adaptive zone implies that it consists of a hyperdimensional space, which in turn makes it difficult to consistently define and quantify. Describing the unique mode of life of extant equids as that of ungulate mammals that are adapted to live in open, generally arid, habitats, and that can thrive on low-quality, high-fiber foods such as grasses and other coarse and tough vegetation (Janis, 1976, 1988; Mihlbachler et al., 2011; Rubenstein et al., 2016; Schoenecker et al., 2016) seems reasonable, but likely captures only a small portion of the complete adaptive zone they occupy. The adaptive zone criterion may also be criticized because it looks for key (essential) traits that allow a taxon or group of taxa to occupy a unique adaptive zone. Mosaic evolution is a widespread phenomenon in vertebrate taxa, including equids (MacFadden, 1992); therefore, it is not rare for characters to have evolved at different times, raising debates about which one should be considered the “essential” character. This problem is exemplified in the present study, as both clades 6 and 7 possess synapomorphies that are relevant for the unique adaptive zone of extant equids, as defined here. Another difficulty in applying the adaptive zone criterion is that the exact ecology of extinct organisms is often hard to determine. In the particular case of equids, some aspects of their ecology, such as feeding ecology, are more readily inferred than others (e.g., MacFadden et al., 1999; Fortelius and Solounias, 2000; Solounias and Semprebon, 2002; Kaiser and Solounias, 2003; Merceron et al., 2004; Sánchez et al., 2006; Mihlbachler et al., 2011). As more complete ecological information becomes available, it will allow researchers to better define the adaptive zone or particular mode of life of extinct equid species. This is particularly relevant if researchers consider that Equus should have both phylogenetic and adaptive significance.
Under the phylogenetic gaps criterion Equus should be delimited to clade 6 in our phylogenetic tree. This is the clade that has the most synapomorphies, resulting in a “phylogenetic gap.” A potential criticism of this criterion is that the positions and relative sizes of gaps among taxa may reflect not only evolutionary processes (e.g., speciation, extinction, evolutionary and adaptive radiations, and unequal rates of evolution; Mayr, 1969), but also gaps in our knowledge. As more fossils are discovered and more specimens are studied, existing gaps will be subdivided. In this regard, our phylogenetic analysis did not include taxa traditionally assigned to Equus of Pliocene and early Pleistocene age other than E. idahoensis, E. simplicidens, and E. stenonis. Inclusion of additional Plio-Pleistocene taxa could potentially make application of this taxonomic criterion more difficult, if it were to “even out” the phylogenetic gaps between clades. Nevertheless, the six currently recognized synapomorphies of clade 6 (Table A2), many of which are also relevant to the adaptive zone criterion, strongly suggest to us that Equus should encompass this clade, pending further phylogenetic analyses that include more Plio-Pleistocene equids traditionally assigned to Equus (e.g., E. cumminsi; E. enormis; E. huanghoensis; E. koobiforensis; E. livenzovensis; E. qingyangensis; E. sanmeniensis; E. yunnanensis; Azzaroli, 1992; Azzaroli and Voorhies, 1993; Downs and Miller, 1994; Eisenmann and Deng, 2005; Palombo and Alberdi, 2017).
As noted above, application of the crown group criterion also supports the idea that Equus encompasses all of clade 6 in our phylogenetic tree. These results are at odds with a recent genomic analysis that concluded Haringtonhippus lies outside of crown group Equus (Heintzman et al., 2017). The crown group criterion has been used in the literature to define genera and other higher taxonomic categories (such as Panthera, see King and Wallace, 2014; and Tapirus, see Holanda and Ferrero, 2013). Furthermore, extant taxa generally allow us to make robust inferences within a crown group about traits that generally do not fossilize. However, one criticism of the crown definition of higher taxa is that it is based on the extinction criterion (Lucas, 1992). As a result, there is great emphasis on living taxa and, instead of promoting stability, this criterion could lead to taxonomic confusion. This is especially true for relict groups, such as Homo. In those instances, other criteria for defining genera may be more relevant. In the case of Homo, the adaptive zone criterion has been used by different researchers to delimit the genus (e.g., Wood and Collard, 1999; Collard and Wood, 2007; Holliday, 2012; Antón et al., 2014).
Clade 6 also meets the three primary taxon naming criteria proposed by Vences et al. (2013) to promote economy of change in Linnaean classification schemes and reduce subjective taxonomic instability. These criteria are monophyly, clade stability, and phenotypic diagnosability (Vences et al., 2013). Clade 6 is characterized by six synapomorphies (Table A2), discussed above under the phylogenetic gaps criterion, and is a fairly well-supported clade (Figure 1). These characteristics identify clade 6 as a stable clade. Furthermore, the six synapomorphies of clade 6 also meet the phenotypic diagnosability criterion, which states that “a taxon to which a Linnaean rank is assigned should be diagnosable and identifiable phenotypically” (Vences et al., 2013, p. 228). The six synapomorphies of clade 6 are visible in both sexes and in many life-history stages of the organism; these are important requirements for this criterion (Vences et al., 2013). Equally important, considering clade 6 as encompassing the genus promotes overall taxonomic stability as traditionally considered in taxonomic treatments. Given that interpretation, our study suggests that Haringtonhippus should be synonymized with Equus, because the former is situated within clade 6.
Outside clade 6, there are some taxonomic considerations for some taxa. First, “Dinohippus” mexicanus would have to be assigned to a new genus. In our study “D.” mexicanus is identified as the sister species to the Equus + Allohippus + Plesippus clade. Our results contrast with previous studies that suggested including “D.” mexicanus within Equus (Prado and Alberdi, 1996) or even including “Dinohippus” s.l. within Equus (Hulbert, 1989), and also with studies in which “D.” mexicanus was identified as the sister species of Equus (Kelly, 1998). Second, by restricting Equus to clade 6, stenonine (i.e., akin to E. stenonis) and plesippine (i.e., akin to E. simplicidens) equids are excluded from Equus and would be recognized as distinct lineages. The recognition of stenonine and plesippine equids as distinct lineages from other Equus taxa was previously suggested by morphometric analyses of cranial proportions (Eisenmann and Baylac, 2000; Eisenmann and Deng, 2005). Based on those analyses, the extant species of Equus and at least some fossil taxa, such as Ha. francisci (= E. francisci) and E. occidentalis, are grouped under the subgenus Equus, whereas stenonine and plesippine equids are referred to the subgenera Allohippus and Plesippus, respectively (Eisenmann and Baylac, 2000). Based on our results, Allohippus and Plesippus should be given full generic status.
The recognition that Ha. francisci (= E. francisci) and extant species of Equus form a distinct clade (clade 6) from stenonine and plesippine equids is consistent with a previous morphometric study (Eisenmann and Baylac, 2000). However, this phylogenetic arrangement is not supported by some phylogenetic analyses (Bennett, 1980; Heintzman et al., 2017). In the morphological study by Bennett (1980), E. stenonis and E. simplicidens (= E. shoshonensis) are nested within the clade that comprises extant zebras. Moreover, Ha. francisci (= E. francisci) was found to be more closely related to E. onager (Bennett, 1980), whereas in our phylogenetic analysis Ha. francisci forms a polytomy with E. conversidens and E. quagga (Figure 1). In the genomic study by Heintzman et al. (2017), Ha. francisci lies outside the clade that comprises extant Equus, but its relationship to stenonine and plesippine equids was not tested because molecular data for these species are presently unknown. The discrepancies between those studies and ours emphasize that the definition of Equus remains a work in progress.
From the morphological side, the study of additional characters, including those relating to postcranial and cranial anatomy, will continue to refine phylogenetic hypotheses of horses. The character matrix that we used is undoubtedly biased toward dental and craniofacial characters, and incorporation of internal cranial characters and more postcranial characters may alter the resulting topology. On the molecular side, better taxonomic sampling is needed. Ultimately, using an integrative, total-evidence approach may provide a better understanding of the evolution and systematics of Plio-Pleistocene equids and a more resolved taxonomy.
As new phylogenetic datasets and hypotheses develop, we have no doubt that there will be additional discussions related to defining Equus and how best to reconcile taxonomy and evolutionary history. We view the deeper value of this endeavor to be stimulation of discussion around naming of genera. As long as binomial nomenclature is retained, naming of new genera (or retaining previously named genera) will almost certainly have a cascade effect, impacting how we view and discuss the evolutionary history of horses.
Conclusions
Two factors are particularly relevant in the delimitation of the genus Equus: (1) identifying well-supported phylogenetic hypotheses, and (2) identifying which clade in a given phylogenetic tree should be considered to comprise the genus Equus. In this study, we inferred a phylogenetic tree and addressed the second factor by considering the consistency in delimitation of Equus on our strict consensus tree across four explicit criteria previously used in combination with monophyly to delimit genera. In our phylogenetic tree, an Equus that encompasses clade 6 has the strongest support on the basis of the most mutually consistent criteria (i.e., phylogenetic gaps, crown group, and, to a lesser extent, adaptive zone), and results in the most taxonomically stable placement of the genus. As such, our results suggest that Haringtonhippus should be considered a synonym of Equus, Allohippus and Plesippus should be elevated to generic rank, and “Dinohippus” mexicanus assigned to a new genus.
Data Availability
All datasets generated for this study are included in the manuscript and/or the Supplementary Files.
Author Contributions
CB-O, LA, and CJ conceived the study and prepared an earlier version of the manuscript. LA, DM, HM, VB-C, and CB-O collected and analyzed the data. All authors contributed to the final version of the manuscript.
Funding
The Royal Alberta Museum provided funding for open access publication fees. This study was funded in part by a post-doctoral scholarship (202.375/2018) to DM, from the Fundação Carlos Chagas Filho de Amparo à Pesquisa do Estado do Rio de Janeiro (FAPERJ) and a post-doctoral scholarship (248772/2013-9) to LA, from the Conselho Nacional de Desenvolvimento Científico e Tecnológico (CNPq).
Conflict of Interest Statement
The authors declare that the research was conducted in the absence of any commercial or financial relationships that could be construed as a potential conflict of interest.
Acknowledgments
We would like to thank the curators and collection managers who provided access to specimens in their care: T. Deng and S. Wang (Institute of Vertebrate Paleontology and Paleoanthropology), S. Wang (Tianjin Hoangho-Paiho Museum), K. Fedyniak and M. Edwards (Royal Alberta Museum), V. Rhue and S. McLeod (Natural History Museum of Los Angeles County), A. Farrell (La Brea Tar Pits and Museum), P. Holroyd (University of California, Museum of Paleontology), E. Scott (San Bernardino County Museum), S. Lucas and G. Morgan (New Mexico Museum of Natural History and Science), J. C. Sagebiel (Jackson School Museum of Earth History, University of Texas at Austin), J. Meng and J. Galkin (American Museum of Natural History), B. MacFadden and R. Hulbert (Florida Museum of Natural History), J. Arroyo-Cabrales (Instituto Nacional de Antropología e Historia), O. Carranza-Castañeda and J. Alvarado-Ortega (Universidad Nacional Autónoma de México), R. Aguilar and G. Viramontes (Museo de Paleontología de Guadalajara), C. Laurito and A. Valerio (Museo Nacional de Costa Rica), J. L. Román-Carrión (Museo de Historia Natural Gustavo Orcés V), I. Alvarado and R. Sallas (Museo de Historia Natural), O. Aguilar Condemayta (Museo de Historia Natural de la Universidad Nacional de San Antonio Abad del Cusco), N. Guidon (Fundação Museu do Homem Americano), C. Cartelle (Museu de Ciências Naturais da Pontifícia Universidade Católica de Minas Gerais), R. Rocha Machado (Departamento Nacional de Produção Mineral), L. Carvalho, O. Grillo and U. Gomes (Museu Nacional), R. Fariña, A. Rojas, M. Ubilla, and D. Perea (Universidad de la República), A. Rinderknecht (Museo Nacional de Historia Natural y Antropología), A. Kramarz (Museo Argentino de Ciencias Naturales Bernardino Rivadavia), M. Reguero (Museo de La Plata), R. Vezzosi (Museo Provincial de Ciencias Naturales Florentino Ameghino), F. Paredes-Ríos (Museo Nacional de Paleontología y Arqueología de Tarija), I. Martínez (Universidad Internacional SEK), A. Prieto (Instituto de la Patagonia), K. Moreno and R. LaBarca (Laboratorio de Paleoecología de la Universidad Austral de Chile), D. Rubilar-Rogers (Museo Nacional de Historia Natural), G. Peralta (Museo Histórico Municipal de Osorno), P. Brewer and A. Lister (Natural History Museum), P. Tassy (Muséum national d'Histoire naturelle). We thank P. J. Lewis (Sam Houston State University) for assisting in the study of the holotype of Haringtonhippus francisci. We also thank J. C. Sagebiel (Jackson School Museum of Earth History, University of Texas at Austin) for kindly photographing the skull and mandible of the holotype of Haringtonhippus francisci. R. L. Bernor and M. Montellano-Ballesteros provided helpful discussions. We also thank the reviewers, P. Heintzman and D. Marjanović, and the associate editor, C. Sullivan, for providing constructive criticism and comments that greatly improved the manuscript. The author DM thanks the Fundação Carlos Chagas Filho de Amparo à Pesquisa do Estado do Rio de Janeiro (FAPERJ) for the Post-doctoral scholarship 202.375/2018; the author LA is grateful to the Conselho Nacional de Desenvolvimento Científico e Tecnológico (CNPq) for the Post-doctoral scholarship 248772/2013-9. Both scholarships were crucial for studying the specimens included in this manuscript.
Supplementary Material
The Supplementary Material for this article can be found online at: https://www.frontiersin.org/articles/10.3389/fevo.2019.00343/full#supplementary-material
References
Antón, S. C., Potts, R., and Aiello, L. C. (2014). Evolution of early Homo: an integrated biological perspective. Science 345:1236828. doi: 10.1126/science.1236828
Athanassiou, A. (2001). New data on the Equus stenonis Cocchi, 1867 from the late Pliocene locality of Sésklo (Thessaly, Greece). Geodiversitas 23, 439–469.
Avise, J. C., and Johns, G. C. (1999). Proposal for a standardized temporal scheme of biological classification for extant species. Proc. Natl. Acad. Sci. U.S.A. 96, 7358–7363.
Azzaroli, A. (1988). On the equid genera Dinohippus Quinn 1955 and Pliohippus Marsh 1874. Boll. Soc. Paleontol. Italiana. 27, 61–72.
Azzaroli, A. (1992). Ascent and decline of monodactyl equids: a case for prehistoric overkill. Ann. Zool. Fenn. 28, 151–163.
Azzaroli, A. (1998). The genus Equus in North America – The Pleistocene species. Paleontogr. Ital. 85, 1–60.
Azzaroli, A., and Voorhies, M. (1993). The genus Equus in North America: the Blancan species. Palaeontogr. Ital. 80, 175–198.
Barrón-Ortiz, C. I., Rodrigues, A. T., Theodor, J. M., Kooyman, B. P., Yang, D. Y., and Speller, C. F. (2017). Cheek tooth morphology and ancient mitochondrial DNA of late Pleistocene horses from the western interior of North America: implications for the taxonomy of North American late Pleistocene Equus. PLoS ONE 12:e0183045. doi: 10.1371/journal.pone.0183045
Bennett, D. K. (1980). Stripes do not a zebra make. Part I: a cladistic analysis of Equus. Syst. Zool. 29, 272–287.
Béthoux, O. (2007). Propositions for a character-state-based biological taxonomy. Zool. Script. 36, 409–416. doi: 10.1111/j.1463-6409.2007.00287.x
Biewener, A. A. (1998). Muscle-tendon stresses and elastic energy storage during locomotion in the horse. Comp. Biochem. Physiol. B Biochem. Mol. Biol. 120, 73–87. doi: 10.1016/S0305-0491(98)00024-8
Bravo-Cuevas, V. M., Jiménez-Hidalgo, E., and Priego-Vargas, J. (2011). Taxonomía y hábito alimentario de Equus conversidens (Perissodactyla, Equidae) del Pleistocene tardío de Hidalgo, centro de México. Rev. Mexic. Ciencias Geol. 28, 65–82.
Cantino, P. D., and de Queiroz, K. (2010). PhyloCode: International Code of Phylogenetic Nomenclature (Version 4c). Available online at: http://www.ohio.edu/phylocode.
Carroll, R. L. (1998). Patterns and Processes of Vertebrate Evolution. Cambridge Paleobiology Series, Cambridge University Press, 448.
Cohen, K. M., Finney, S. C., Gibard, P. L., and Fan, J.-X. (2013). The ICS international chronostratigraphic chart. Episodes 36, 199–204.
Collard, M., and Wood, B. (2007). “Defining the genus Homo,” in Handbook of Paleoanthropology, vol. 3 of Phylogeny of Hominids, eds W. Henke and I. Tattersall (Berlin: Springer), 1575–1611.
Cracraft, J. (1997). “Species concepts in systematics and conservation biology—an ornithological viewpoint,” in Species: The Units of Biodiversity, M. F. Claridge, A. A. Dawah, and M. R. Wilson (New York, NY: Chapman and Hall), 325–339.
Dalquest, W. W. (1978). Phylogeny of American horses of Blancan and Pleistocene age. Acta Zool. Fennica. 15, 191–199.
Dalquest, W. W. (1979). The little horses (genus Equus) of the Pleistocene of North America. Am. Midland Nat. 101, 241–244.
Dalquest, W. W. (1988). Astrohippus and the Origin of Blancan and Pleistocene Horses. Occas: Papers, The Museum Texas Tech Univ. 116, 1–23.
Dalquest, W. W., and Hughes, J. T. (1965). The Pleistocene horse, Equus conversidens. Am. Midland Nat. 74, 408–417.
Damuth, J., and Janis, C. (2011). On the relationship between hypsodonty and feeding ecology in ungulate mammals, and its utility in paleoecology. Biol. Rev. 86, 733–758. doi: 10.1111/j.1469-185X.2011.00176.x
de Queiroz, K. (1994). Replacement of an essentialistic perspective on taxonomic definitions as exemplified by the definition of “Mammalia”. Syst. Biol. 43, 497–510.
de Queiroz, K. (1998). “The general lineage concept of species, species criteria, and the process of speciation: a conceptual unification and terminological recommendations,” in Endless Forms: Species and Speciation, eds D. J. Howard and S. H. Berlocher (New York, NY: Oxford University Press),57–75.
de Queiroz, K. (2007). Species concepts and species delimitation. Syst. Biol. 56, 879–886. doi: 10.1080/10635150701701083
de Queiroz, K., and Donoghue, M. J. (1988). Phylogenetic systematics and the species problem. Cladistics. 4, 317–338.
de Queiroz, K., and Gauthier, J. (1992). Phylogenetic taxonomy. Annu. Rev. Ecol. Syst. 23, 449–480. doi: 10.1146/annurev.es.23.110192.002313
Der Sarkissian, C., Vilstrup, J. T., Schubert, M., Seguin-Orlando, A., Eme, D., Weinstock, J., et al. (2015). Mitochondrial genomes reveal the extinct Hippidion as an outgroup to all living equids. Biol. Lett. 11:20141058. doi: 10.1098/rsbl.2014.1058
Downs, T., and Miller, G. J. (1994). Late Cenozoic equids from the Anza-Borrego Desert of California. Nat. Hist. Mus. Los Angeles Co. Contrib. Sci. 440, 1–90.
Dubois, A. (1988). The genus in zoology: a contribution to the theory of evolutionary systematics. Mémoires Muséum Nat. d'Histoire Naturelle 140, 1–123.
Eisenmann, V. (1982). Le Cheval et ses Proches Parents: Évolution et Phylogénie. 8e Journée d'étude équine. Paris: CEREOPA, 9–26.
Eisenmann, V., and Baylac, M. (2000). Extant and fossil Equus (Mammalia, Perissodactyla) skulls: a morphometric definition of the subgenus Equus. Zool. Scripta. 29, 89–100. doi: 10.1046/j.1463-6409.2000.00034.x
Eisenmann, V., and David, F. (1990). “Les chevaux des niveaux XI et Xb-c de la grotte du renne à Arcy-sur-Cure (Yonne),” in Paléolithique Moyen Récent et Paléolithique Supérieur Ancien en Europe. Mémoires du Musée de Préhistoire d'Ile-de-France, Colloque international de Nemours, 9–11.
Eisenmann, V., and Deng, T. (2005). Equus qingyangensis (Equidae, Perissodactyla) of the Upper Pliocene of Bajiazui. China: evidence for the North American origin of an Old World lineage distinct from E. stenonis. Quaternaire Hors-série 2, 113–122.
Ereshefsky, M. (2001). The Poverty of the Linnaean hierarchy. A Philosophical Study of Biological Taxonomy. Cambridge University Press.
Famoso, N. A., and Davis, E. B. (2014). Occlusal enamel complexity in middle Miocene to Holocene equids (Equidae: Perissodactyla) of North America. PLoS ONE 9:e90184. doi: 10.1371/journal.pone.0090184
Fortelius, M., and Solounias, N. (2000). Functional characterization of ungulate molars using the abrasion-attrition wear gradient: a new method for reconstructing paleodiets. Am. Museum Novitates 3301, 1–36.
Ghiselin, M. T. (1966). On psychologism in the logic of taxonomic controversies. Syst. Zool. 15, 207–215.
Gidley, J. W. (1907). Revision of the miocene and pliocene equidae of North Am. Bull. Amer. Mus. Nat. Hist. 23, 865–934.
Goloboff, P., Farris, J., and Nixon, K. (2008). TNT, a free program for phylogenetic analysis. Cladistics 24, 774–786.
Gromova, V. (1949). Histoire des chevaux (genre Equus) de l'ancien monde. Première partie: revue et description des formes. Travaux Inst. Paléontol. Acad. Sci. URSS, t. 17, n° 1, 373 p., Trad. Pietresson de Saint-Aubin. Annales du Centre d'Études et de Documentation Paléontologiques, 1955, n° 13.
Groves, C. P., and Grubb, P. (2011). Ungulate Taxonomy. Baltimore: The Johns Hopkins University Press.
Guadelli, J.-L., and Prat, F. (1995) Le cheval du gisement pléistocène moyen de Camp-de-Peyre (Sauveterre-la-Lémance, Lot-et-Garonne). Paleo 7, 85–121.
Heintzman, P. D., Zazula, G. D., MacPhee, R. D. E., Scott, E., Cahill, J. A., McHorse, B. K., et al. (2017). A new genus of horse from Pleistocene North America. eLife 6:e29944. doi: 10.7554/eLife.29944
Hermanson, J. W., and MacFadden, B. J. (1992). Evolutionary and functional morphology of the shoulder region and stay-apparatus in fossil and extant horses (Equidae). J. Vertebr. Palaeontol. 12, 377–386.
Hermanson, J. W., and MacFadden, B. J. (1996). Evolutionary and functional morphology of the knee in fossil and extant horses (Equidae). J. Vertebr. Palaeontol. 16, 349–357.
Holanda, E. C., and Ferrero, B. S. (2013). Reappraisal of the genus Tapirus (Perissodactyla, Tapiridae): systematics and phylogenetic affinities of the South American Tapirs. J. Mammal. Evol. 20, 33–44. doi: 10.1007/s10914-012-9196-z
Holliday, T. W. (2012). Body size, body shape, and the circumscription of the genus Homo. Curr. Anthropol. 53, S330–S345. doi: 10.1086/667360
Hulbert, R. C. (1988). Calippus and Protohippus (Mammalia, Perissodactyla, Equidae) from the Miocene (Barstovian-Early Hemphillian) of the Gulf Coastal Plain. Bull. Florida State Museum Biol. Sci. 32, 221–340.
Hulbert, R. C. (1989). “Phylogenetic interrelationships of North American late Neogene Equinae,” in The Evolution of Perissodactyls, eds D. R. Prothero and R. M. Schoch (New York, NY: Oxford Univ. Press), l76–196.
Janis, C. (1976). The evolutionary strategy of the Equidae and the origins of rumen and cecal digestion. Evolution 30, 757–774.
Janis, C. (1988). “An estimation of tooth volume and hypsodonty indices in ungulate mammals and the correlation of these factors with dietary preference,” in Teeth Revisited: Proceedings of the Vllth International Symposium on Dental Morphology, Paris, 1986. Mémoires du Musée National d'Histoire Naturelle, série C, Paris, eds D. E. Russell, J.-P. Santoro, and D. Sigogneau-Russell, 367–387.
Janis, C. M., and Wilhelm, P. B. (1993). Were there mammalian pursuit predators in the Tertiary? Dances with wolf avatars. J. Mamm. Evol. 1, 103–125. doi: 10.1007/BF01041590
Kaiser, T. M. (2002). Functional significance of ontogenetic gradients in the enamel ridge pattern of the upper cheek dentition of the Miocene hiparionin horse Cormohipparion occidentale (Equidae, Perissodactyla). Senckenbergiana Lethaea. 82, 167–180. doi: 10.1007/bf03043782
Kaiser, T. M., and Solounias, N. (2003). Extending the tooth mesowear method to extinct and extant equids. Geodiversitas 25, 321–345.
Kelly, T. S. (1998). New middle Miocene equid crania from California and their implications for the phylogeny of the Equini. Nat. Hist. Mus. Contrib. Sci. 473, 1–43.
King, L. M., and Wallace, S. C. (2014). Phylogenetics of Panthera, including Panthera atrox, based on craniodental characters. Historic. Biol. 26, 827–833. doi: 10.1080/08912963.2013.861462
Kuntner, M., and Agnarsson, I. (2006). Are the Linnaean and phylogenetic nomenclatural systems combinable? Recommendations for biological nomenclature. Syst. Biol. 55, 774–784. doi: 10.1080/10635150600981596
Lance, J. F. (1950). Paleontología y estratigrafía del Plioceno de Yepómera, Estado de Chihuahua - Ia parte: equidos, excepto Neohipparion. lnst. Geol. Univ. Autónoma México Bol. 54, 1–81.
Leakey, L. S. B., Tobias, P. V., and Napier, J. R. (1964). A new species of the genus Homo from Olduvai Gorge. Nature 202, 7–9.
Lundelius, E. L., and Stevens, M. S. (1970). Equus francisci Hay, a small stilt-legged horse, Middle Pleistocene of Texas. J. Paleontol. 44, 148–153.
MacFadden, B. J. (1984). Astrohippus and Dinohippus from the Yepómera Local Fauna (Hemphillian, Mexico) and implications for the phylogeny of one-toed horses. Jour. Vert. Paleontol. 4, 273–283.
MacFadden, B. J. (1992). Fossil Horses: Systematics, Paleobiology, and Evolution of the Family Equidae. New York, NY: Cambridge Univ. Press, 369.
MacFadden, B. J., and Carranza-Castañeda, O. (2002). Cranium of Dinohippus mexicanus (Mammalia: Equidae) from the early Pliocene (latest Hemphillian) of central Mexico, and the origin of Equus. Bull. Florida Museum Nat. History 43, 163–185.
MacFadden, B. J., Solounias, N., and Cerling, T. E. (1999). Ancient diets, ecology, and extinction of 5 million-year-old horses from Florida. Science 283, 824–827.
Matthew, W. D. (1926). The evolution of the horse. A record and its interpretation. Quart. Rev. BioI. 1, 139–185.
Mayden, R. L. (1997). “A hierarchy of species concepts: the denouement in the saga of the species problem,” in Species: The Units of Biodiversity, eds M. F. Claridge, H. A. Dawah, and M. R. Wilson (London: Chapman and Hall, 381–424.
Mayden, R. L. (2002). On biological species, species concepts and individuation in the natural world. Fish Fish. 3, 171–196. doi: 10.1046/j.1467-2979.2002.00086.x
Mayr, E. (1942). Systematics and the Origin of Species from the Viewpoint of a Zoologist. New York, NY: Columbia University Press.
Mayr, E. (1950). Taxonomic categories in fossil hominids. Cold Spring Harbor Symp. Quant. Biol. 15, 109–118. doi: 10.1101/SQB.1950.015.01.013
McHorse, B. K., Biewener, A. A., and Pierce, S. E. (2017). Mechanics of evolutionary digit reduction in fossil horses (Equidae). Proc. R. Soc. B 284:20171174. doi: 10.1098/rspb.2017.1174
Merceron, G., Blondel, C., Brunet, M., Sen, S., Solounias, N., Viriot, L., and Heintz, E. (2004). The Late Miocene paleoenvironment of Afghanistan as inferred from dental microwear in artiodactyls. Palaeogeogr. Palaeoclimatol. Palaeoecol. 207, 143–163. doi: 10.1016/j.palaeo.2004.02.008
Michener, C. D. (1957). Some bases for higher categories in classification. Syst. Zool. 6, 160–173. doi: 10.2307/2411422
Mihlbachler, M. C., Rivals, F., Solounias, N., and Semprebon, G. M. (2011). Dietary change and evolution of horses in North America. Science 331, 1178–1181. doi: 10.1126/science.1196166
Orlando, L., Male, D., Alberdi, M. T., Prado, J. L., Prieto, A., Cooper, A., et al. (2008). Ancient DNA clarifies the evolutionary history of American late Pleistocene equids. J. Mol. Evol. 66, 533–538. doi: 10.1007/s00239-008-9100-x
Osborn, H. F. (1918). Equidae of the Oligocene, Miocene, and Pliocene of North America. Iconographic type revision. Mem. Amer. Mus. Nat. Hist. 2, 1–326.
Palombo, M. R., and Alberdi, M. T. (2017). Light and shadows in the evolution of South European stenonoid horses. Fossil Imprint. 73, 115–140. doi: 10.2478/if-2017-0006
Papavero, N., Llorente-Bousquets, J., and Minoro Abel, J. (2001). Proposal of a new system of nomenclature for phylogenetic systematics. Arquivos Zool. 36, 1–145. doi: 10.11606/issn.2176-7793.v36i1p1-145
Paterson, H. E. (1978). More evidence against speciation by reinforcement. S. Afr. J. Sci. 14, 369–371.
Paterson, H. E. (1985). “The recognition concept of species,” in Species and Speciation, ed E. S. Vrba (Pretoria: Transvaal Museum) (Monograph No. 4), 176.
Prado, J., and Alberdi, M. T. (1996). A cladistic analysis of the horses of the Tribe Equini. Palaeontology. 39, 663–680.
Priego-Vargas, J., Bravo-Cuevas, V. M., and Jiménez-Hidalgo, E. (2017). Revisión taxonómica de los équidos del Pleistoceno de México con base en la morfología dental. Rev. Brasil. Paleontol. 20, 239–268. doi: 10.4072/rbp.2017.2.07
Quinn, J. H. (1955). Miocene Equidae of the Texas Gulf Coastal Plain. Univ. Texas Bur. Econ. Geol. 5516, 1–102.
Rensberger, J. M., Forsten, A., and Fortelius, M. (1984). Functional evolution of the cheek tooth pattern and chewing direction in Tertiary horses. Paleobiology 10, 439–452.
Rowe, T., and Gauthier, J. (1992). Ancestry, paleontology, and definition of the name Mammalia. Syst. Biol. 41, 372–378.
Rubenstein, D. I., Cao, Q., and Chiu, J. (2016). “Equids and ecological niches: behavioral and life history variations on a common theme,” in Wild Equids: Ecology, Management, and Conservation, eds J. I. Ransom and P. Kaczensky (Baltimore: Johns Hopkins University Press, 58–67.
Sánchez, B., Prado, J. L., and Alberdi, M. T. (2006). Ancient feeding, ecology and extinction of Pleistocene horses from the Pampean Region, Argentina. Ameghiniana 43, 427–436.
Schoenecker, K. A., King, S. R. B., Nordquist, M. K., Nandintsetseg, D., and Cao, Q. (2016). “Habitat and diet of Equids,” in Wild Equids: Ecology, Management, and Conservation, eds J. I. Ransom and P. Kaczensky (Baltimore: Johns Hopkins University Press, 41–57.
Scott, E. (1996). “The small horse from Valley Wells, San Bernardino County, California,” in Punctuated Chaos in the Northeastern Mojave Desert. SBCM Association Quarterly 43(1,2), eds R. E. Reynolds and J. Reynolds (Redland, CA: J, San Bernardino County Museum Association, 85–89.
Scott, E. (2005). Equus idahoensis From the Pliocene of Arizona, and Its Role in Plesippine Evolution in the American Southwest. Available online at: http://www.sbcounty.gov/museum/discover/divisions/geo/pdf/SVP_2005b_EquusIdahoensis.pdf.
Shotwell, J. A. (1961). Late Tertiary biogeography of horses in the northern Great Basin. J. Paleontol. 35, 203–217.
Simpson, G. G. (1951). Horses: The Story of the Horse Family in the Modern World and Through Sixty Million Years. Oxford: Oxford University Press.
Solounias, N., and Semprebon, G. (2002). Advances in the reconstruction of ungulate ecomorphology with application to early fossil equids. Am. Museum Novitates 3366, 1–49. doi: 10.1206/0003-0082(2002)366<0001:AITROU>2.0.CO;2
Stirton, R. A. (1940). Phylogeny of North American Equidae. Univ. California Pub., Bull. Dept. Geol. Sci. 25, 165–198.
Stirton, R. A. (1942). Comments on the origin and generic status of Equus. J. Paleontol. 16, 627–637.
Templeton, A. R. (1989). “The meaning of species and speciation: a genetic perspective,” in Speciation and its Consequences, eds D. Otte and J. A. Endler (Sunderland, MA: Sinauer Associates).
Thomason, J. J. (1986). The functional morphology of the manus in the tridactyl equids Merychippus and Mesohippus: paleontological inferences from neontological models. J. Vertebr. Paleontol. 6, 143–161. doi: 10.1080/02724634.1986.10011607
Tobias, P. V. (1991). The Skulls, endocasts and teeth of Homo habilis. Olduvai Gorge Volume 4. Cambridge: Cambridge Univ. Press.
Vences, M., Guayasamin, J. M., Miralles, A., and de la Riva, I. (2013). To name or not to name: criteria to promote economy of change in Linnaean classification schemes. Zootaxa 3636, 201–244. doi: 10.11646/zootaxa.3636.2.1
Vilstrup, J. T., Seguin-Orlando, A., Stiller, M., Ginolhac, A., Raghavan, M., Nielsen, S. C. A., et al. (2013). Mitochondrial phylogenomics of modern and ancient equids. PLoS ONE 8:e55950. doi: 10.1371/journal.pone.0055950
Webb, S. D. (1969). The Burge and Minnechaduza Clarendonian mammalian faunas of north-central Nebraska. Univ. Calif. Pub. Geol. Sc. 78, 1–191.
Weinstock, J., Willerslev, E., Sher, A., Tong, W., Ho, S. Y. W., Rubenstein, D., et al. (2005). Evolution, systematics, and phylogeography of Pleistocene horses in the New World: a molecular perspective. PLoS Biol. 3:e241. doi: 10.1371/journal.pbio.0030241
Wiley, E. O. (1978). The evolutionary species concept reconsidered. Syst. Zool. 27, 17–26. doi: 10.2307/2412809
Winans, M. C. (1985). Revision of North American fossil species of the genus Equus (Mammalia: Perissodactyla: Equidae). Ph.D. Dissertation, University of Texas at Austin.
Winans, M. C. (1989). “A quantitative study of North American fossil species of the genus Equus,” in The Evolution of Perissodactyls, eds D. R. Prothero and R. M. Schoch (Oxford: Clarendon Press), 262–297.
Wood, B. A., and Collard, M. (1999). The human genus. Science 284, 65–71. doi: 10.1126/science.284.5411.65
Keywords: phylogeny, taxonomy, Equidae, Equus, Dinohippus, Haringtonhippus, Allohippus, Plesippus
Citation: Barrón-Ortiz CI, Avilla LS, Jass CN, Bravo-Cuevas VM, Machado H and Mothé D (2019) What Is Equus? Reconciling Taxonomy and Phylogenetic Analyses. Front. Ecol. Evol. 7:343. doi: 10.3389/fevo.2019.00343
Received: 07 March 2019; Accepted: 27 August 2019;
Published: 12 September 2019.
Edited by:
Corwin Sullivan, University of Alberta, CanadaReviewed by:
Peter Heintzman, Arctic University of Norway, NorwayDavid Marjanović, Museum für Naturkunde, Germany
Copyright © 2019 Barrón-Ortiz, Avilla, Jass, Bravo-Cuevas, Machado and Mothé. This is an open-access article distributed under the terms of the Creative Commons Attribution License (CC BY). The use, distribution or reproduction in other forums is permitted, provided the original author(s) and the copyright owner(s) are credited and that the original publication in this journal is cited, in accordance with accepted academic practice. No use, distribution or reproduction is permitted which does not comply with these terms.
*Correspondence: Christina I. Barrón-Ortiz, Y2hyaXN0aW5hLmJhcnJvbi1vcnRpeiYjeDAwMDQwO2dvdi5hYi5jYQ==