- 1Wildlife Conservation Research Unit (WildCRU), Department of Zoology, The Recanati-Kaplan Centre, University of Oxford, Tubney, United Kingdom
- 2IUCN SSC Canid Specialist Group, Oxford, United Kingdom
The Canidae are successful, being a widespread, abundant, speciose, and adaptable family. Several canids in particular have recently experienced rapid expansions in range and abundance, with similar situations mirrored on several continents by different species. Despite extreme behavioral diversity between and within species, monogamy is a common denominator in canid societies. In this review, we ask why canids are monogamous and how monogamy is related to their success. We begin with an overview of canid social monogamy, describing the pair bonding, paternal care, and often alloparental care that is characteristic of the family, and discuss theories on the evolution of mammalian social monogamy. We discuss why and how monogamy is maintained in canids, either voluntarily or enforced, and how ecological conditions influence either the functional advantages of monogamy or ability for enforcement and thus whether social monogamy is maintained. Social monogamy does not necessitate exclusive mating and many canids exhibit extra-pair paternity. We consider the costs and benefits of extra-pair mating for male and female canids and how ecological conditions can shift this cost/benefit balance and thus affect its prevalence. Monogamy may be responsible for many of the unusual canid reproductive characteristics through facilitating alloparental care and monogamy enforcement, and the domestic dogs' departure from monogamy supports our interpretation that it is an adaptation to resource availability. In asking whether monogamy is responsible, at least in part, for their success, we propose the monogamy as pro-cooperative hypothesis, suggesting four characteristics have contributed to canid success: (1) ecological flexibility, (2) high mobility, (3) high reproductive rates, and (4) sociality/cooperation, with the latter two being consequences of monogamy. These four interconnected traits enhance one another and it is their combination, with monogamy at its foundation enabling cooperative sociality and thereby enhanced reproduction and survival, that together comprise the formula of canid success.
Introduction
Of their many notable attributes, three stand out about the Canidae: first, they are remarkably similar; second, they are remarkably different; and third, they are remarkably successful.
First, the similarity lies in the anatomical and behavioral traits that makes all 37 species of the family—from fennec fox (Vulpes zerda) to gray wolf (Canis lupus)—immediately recognizable as dogs (for a dramatis personae see Macdonald and Sillero-Zubiri, 2004). Not only are the largest species essentially morphologically inflated identikits of the smaller ones, but their expressions and demeanours are similar, as are their societies, all built around monogamy (Macdonald et al., 2004). Social monogamy is unusual amongst mammals, adopted by only 3–9% of Mammalia species and 16% of Carnivora species (Kleiman, 1977; Lukas and Clutton-Brock, 2013), yet is found in all canid species studied to date. In no other mammalian family is the pair bond so ubiquitous. In an overview of carnivore societies, Macdonald (1992) emphasized the phylogenetic descent that weaves canidness and monogamy throughout the family (as it does similarly for felidness and polygyny throughout the Felidae) (Macdonald and Kays, 2005).
Second, the canid family is highly diverse. Body sizes range from the 0.8 kg fennec fox to the 60+ kg gray wolf (Nowak, 2005). Their diets range from the almost exclusively insectivorous (e.g., bat-eared fox [Otocyon megalotis]) to almost exclusively carnivorous (e.g., African wild dog [Lycaon pictus], bush dog [Speothos venaticus], Ethiopian wolf [Canis simensis], Marino et al., 2010), with a full spectrum of omnivory between (e.g., red fox [Vulpes vulpes], golden jackal [C. aureus], African golden wolf [C. lupaster]). Such extremes in diets are reflected in their dentition, because while most canids have 42 teeth well-suited for generalist diets (e.g., carnassials for shearing flesh and molars for omnivory), bat-eared foxes have up to 50 less-specialized teeth (the most of any land mammal) for extreme insectivory (Klare et al., 2011), whereas the dholes, bush dogs, and African wild dogs have reduced or absent molars and enhanced carnassials for hypercarnivory (Van Valkenburgh, 1991). Canids are found in nearly all terrestrial habitats, including such extremes as Arctic tundra (Arctic fox [Vulpes lagopus]), desert (fennec fox), tropical forest (dhole [Cuon alpinus]), high-altitude environments (e.g., Ethiopian wolf, Marino, 2003; Himalayan wolf [C. [lupus] himalayensis], Werhahn et al., 2017, 2018) and human cities (e.g., coyote [C. latrans]). Some even partially exploit aquatic (e.g., short-eared dog [Atelocynus microtis], de Oliveira, 2009; British Columbia coastal wolf, Darimont and Paquet, 2002; Stronen et al., 2012) and arboreal (e.g., gray fox [Urocyon cinereoargenteus], Trapp and Hallberg, 1975) habitats. Canid social systems range from generally solitary species like the maned wolf (Chrysocyon brachyurus), where pairs share a territory but associate only during the mating season (Dietz, 1984), through the spatial groups of red and Arctic foxes (e.g., Hersteinsson and Macdonald, 1982, 1992), to species forming large, complex packs (e.g., Ethiopian wolves, Sillero-Zubiri and Gottelli, 1995a; bush dogs, Macdonald, 1996; African wild dogs, Creel et al., 2004; gray wolves, Jedrzejewski et al., 2005). Even within species, canids exhibit substantial variation (Macdonald and Moehlman, 1982; Moehlman, 1989; Creel and Macdonald, 1995; Geffen et al., 1996; Moehlman and Hofer, 1997) and variability within a species may be larger than that between species (Macdonald and Moehlman, 1982; Creel and Macdonald, 1995). For example, gray wolves, coyotes, black-backed jackals (Lupulella mesomelas) and red foxes may live solitarily, in pairs, or in large groups (Kleiman and Brady, 1978; Messier and Barrette, 1982; Mech and Boitani, 2003; Baker and Harris, 2004; Kamler et al., 2019). Red fox home ranges vary between populations by three orders of magnitude, their societies varying between ubiquitous socially monogamous pairs and spatial groups of six adults (Macdonald, 1981), while gray wolves occupy ranges varying from 75 to 2,500 km2 with groups varying from pairs to packs of up to 42 (Mech and Boitani, 2003). Arctic foxes can exist as a “coastal” ecotype, feeding on temporally stable seabirds and marine resources and consequently living a moderate lifestyle with litters averaging five cubs produced yearly. Alternatively, arctic foxes can exist as a “lemming” ecotype, feeding on rodents with extreme cyclic population variations and consequently mirroring this extreme lifestyle, producing litters of up to 18 cubs during rodent peaks and rarely reproducing during years of low prey availability (Tannerfeldt and Angerbjörn, 1998). Though they often exist as a single breeding pair, they may form large social groups of up to 31 individuals, referred to as “fox towns” (Elmhagen et al., 2014). Ethiopian wolves, Afroalpine specialists, also display such plasticity: pairs with large territories dominate in low productivity environments, while packs of up to 18 adults/subadults defend small territories in optimal habitats (Sillero-Zubiri et al., 2004).
Third, canids are successful, both over evolutionary and modern times. Evolutionarily, canids usurped Hyaenidae from the dog-niche in the Pliocene (Macdonald, 1992). Nowadays, many species of canids flourish alongside humanity. A canid currently claims the title of the world's most widely distributed non-domestic terrestrial mammal: the red fox (Macdonald and Sillero-Zubiri, 2004), who usurped this title from another canid, the gray wolf (Mech, 1995; Macdonald and Sillero-Zubiri, 2004), while free-ranging domestic dogs are found across the globe (Lord et al., 2013). Several canids have recently rapidly expanded their ranges: coyotes have become ubiquitous across North and Central America over the past two centuries (Gompper, 2002; Macdonald and Sillero-Zubiri, 2004), while golden jackals (Tóth et al., 2009; Rutkowski et al., 2015) and raccoon dogs (Helle and Kauhala, 1991; Kauhala and Saeki, 2004; Sutor, 2007; Kauhala and Kowalczyk, 2011) mirror this same rapid expansion across Europe. Following introductions of red foxes to Australia and eastern North America, they rapidly spread throughout most of continental Australia and USA (Kamler and Ballard, 2002). Despite intensive human efforts to control populations of red foxes, coyotes, golden jackals and free-ranging domestic dogs, these species continue to survive and thrive. Even gray wolves, driven to the brink of extinction in the 1800s, are now returning to their former range in North America and Europe (Mech, 1995, 2017; Breitenmoser, 1998; Wydeven et al., 1998; Phillips et al., 2004). That these expansions are mirrored across several parts of the world raises the question of what has allowed these canids to achieve such wide distributions and high abundances, rapid expansion, colonization and biological invasion, and resilience to human population control.
Together, these canid attributes—similarity resulting from evolutionary conservatism and differences from behavioral flexibility—prompts the question of what are the limits to variation in each species, and if those limits differ between species, what evolutionary constraints have set them? And since the unusual common denominator of canid societies is monogamy, why are they monogamous and what role has this played in the family's success, both in evolutionary time and the Anthropocene?
Monogamy has long been an area of interest for evolutionary and behavioral ecologists (Orians, 1969; Emlen and Oring, 1977) and numerous early hypotheses attempted to explain its evolution and maintenance (e.g., Orians, 1969; Emlen and Oring, 1977; Wittenberger and Tilson, 1980). Decades of empirical and theoretical research suggest factors affecting monogamy's origin and maintenance can be complex, differ among taxa, and are subject of a constant evolutionary interplay between monogamy and associated traits (Klug, 2018; Lambert et al., 2018). However, most research on monogamy has focused on birds (Reichard and Boesch, 2003), unsurprisingly since ~90% of bird species exhibit social monogamy. In contrast, 95–97% of studied mammals are polygamous (i.e., polygynous, polygynandrous, and less commonly, polyandrous) (Kleiman, 1977; Lukas and Clutton-Brock, 2012)—Canidae are one of the main exceptions (Kleiman, 2011). There are variations on every theme, and some cases in wild canids of polygyny, polyandry, polygynandry, plural breeding, communal breeding, cooperative breeding, and promiscuity exist. Nonetheless, we are aware of no study of any canid species that has not revealed a mated pair at its nucleus. Sexual dimorphism generally correlates with mating system (Weckerly, 1998), and amongst canids monogamous mating and paternal care are associated with little or no sexual dimorphism (Kleiman, 1977, 2011; Bekoff et al., 1981; Johnson et al., 2017). The heavy investment by females in internal fertilization, gestation and lactation leaves females committed to much of the burden of parental care and provides considerable opportunity for males to desert their partners to seek additional mating opportunities (Orians, 1969; Trivers, 1972; Maynard Smith, 1977; Clutton-Brock, 1989). Why then would canids form prolonged, year-long pair bonds, maintained not only outside of the breeding season, but often for many years? And what variations of monogamy are exhibited among and within canid species and what causes these variations?
To begin this review, we first consider social monogamy, then reveal differences between canid social and genetic mating systems, i.e., social monogamy does not dictate exclusive mating. We consider canid-specific reproductive traits that may have developed from social and genetic monogamy and, following a detour around the anomalous case of domestic dogs, we reflect on whether monogamy, and the flexible social systems built around it, is a factor in the success of members of the canid family.
Canid Social Monogamy
Characteristics of Canid Social Monogamy
The fundamental canid social unit, irrespective of group size, is the socially monogamous pair. The primary defining characteristic of social monogamy is spatial congruence of a single breeding male and female. However, this basic criterion is generally exceeded in canid pairs by an affiliative social pair bond, including high rates of social interaction, cooperative territorial defense, mutual offspring care, den sharing, and intrasexual aggression directed at individuals outside the pair bond (Lord et al., 2013). Box 1 provides an overview of variations of social monogamy in canids.
Box 1. Variations of social monogamy in canids.
Social monogamy: Social structure involving a single breeding male and female, which in canids typically involves an affiliative pair bond, shared territory with mutual territory defense, and biparental offspring care. Group-living canids may exhibit social monogamy, with a single breeding male and female pair and additional non-breeding group members (often their offspring).
Genetic monogamy: Exclusive reproduction between one male and one female (i.e., no extra-pair paternity).
Pair bonding: Affiliative bond between a breeding male and female, which in canids is generally maintained for several years, often until the death of one of the pair. In some canids, the mated pair maintains close associations year-round, whereas in maned wolves and many small canids, although a pair shares and defends a territory year-round, close associations and den sharing occur primarily during the breeding and pup-rearing seasons (Dietz, 1984; Kitchen et al., 2005a; Ralls et al., 2007; Kamler and Macdonald, 2014).
Canid variations of social monogamy Pairs: A single pair-bonded mating male and female share a territory year-round and young disperse. Example: swift fox (Kitchen et al., 2006).
Trios: A single pair-bonded dominant mating male and female, plus an additional subordinate non-breeding adult. The additional adult is usually the offspring of at least one of the pair from a previous year, though not always. The third adult may or may not actively help in raising young, and trios may be stable over several years. Example: kit fox (White and Ralls, 1993). Groups: A single pair-bonded dominant male and female, plus additional subordinate non-breeding adults. Additional adults are usually offspring of at least one of the pair from previous years, though not always. In some cases, additional adults may actively help in raising pups and groups may cooperatively hunt and defend resources, in which case it can be considered cooperative breeding (though cooperatively breeding groups could also be polygamous) Example: gray wolves (Bekoff and Wells, 1982). In other cases, additional adults do not actively help in raising pups and group members do not coordinate behavior. Example: Blanford foxes (Geffen and Macdonald, 1992).Double litters/Plural breeding: Multiple (usually two) non-interbreeding pairs of males and females share a den and territory and produce litters. Two litters may be born in the same den (e.g., coyote: Hennessy, 2007), or two litters may later merge (e.g., arctic fox: see Norén et al., 2012). The two females are often close relatives (e.g., mother-daughter). Note that these terms have also been used to describe polygynous/polygynandrous systems. Although double litters are commonly reported in coyotes, more genetic research is needed to distinguish cases where these are in fact multiple litters or large litters with size differences between pups, or whether this represents polygamous systems, though one study has confirmed two genetically monogamous pairs (Hennessy, 2007). If alloparental care is provided to the other litter (e.g., allo-nursing between females can be common), this represents communal breeding (i.e., not social monogamy). Deviations from social monogamy: Group-living canids may instead exhibit social polygyny (e.g., bigamous red foxes: Zabel and Taggart, 1989), social polyandry (e.g., African wild dogs, Spiering et al., 2010), polygynandry/communal breeding (e.g., African wild dogs, Spiering et al., 2010).
Pair Bonding
Most socially monogamous animals practice serial seasonal monogamy, short-term pairing that lasts only a single breeding season, replaced by a new monogamous bond the following year (e.g., ducks of the Anas genus, Mock et al., 1985). Canids, in contrast, often maintain long-term affiliative and cooperative pair bonds and typically remain with the same partner, unless mortality intervenes (e.g., Island fox [Urocyon littoralis], Roemer et al., 2001; swift fox [Vulpes velox], Kitchen et al., 2005a; kit fox [V. macrotis], Ralls et al., 2007; cape fox [V. chama], Kamler and Macdonald, 2014). For example, high mortality rates were responsible for serial monogamy in populations of red foxes (Zabel, 1986), swift foxes (Kamler et al., 2004a) and in intensely hunted gray wolves (Jedrzejewski et al., 2005). Amongst Ethiopian wolves, the dominant female's position changes only with her death, though male turn-over is more frequent (Sillero-Zubiri et al., 1996a, 2004). Pairings as long as 8 years have been reported in coyotes (Hennessy, 2007) and black-backed jackals (Moehlman, 1989) and up to 9 years in gray wolves (Doug Smith, oral communication). The degree to which a pair associates outside the breeding season differs between species. For many large canids, such as gray wolves and African wild dogs, the mated pair remain closely associated year-round, coordinating their behavior and hunting together (Creel and Creel, 1995; Mech and Boitani, 2003). For small species, such as cape foxes, swift foxes, and kit foxes, mated pairs share a territory throughout the year but hunt solitarily; they share dens and closely associate only during the breeding and cub-rearing seasons whereas other times of the year they use different dens and associate with each other less frequently (Kitchen et al., 2005a; Ralls et al., 2007; Kamler and Macdonald, 2014). The maned wolf may be an extreme example of this, as mated pairs apparently do not associate with each other at all outside the of the breeding and pup-rearing season (Dietz, 1984), although intraspecific differences among populations might occur. Medium-sized canids, such as coyotes and jackals, may exhibit variations in year-around associations of mated pairs, possibly related to group size or prey size.
Paternal Care
Although paternal care occurs in only 5–10% of mammalian species (Kleiman and Malcolm, 1981; Clutton-Brock, 1991; Woodroffe and Vincent, 1994), it is nearly ubiquitous in canids (Malcolm, 1985; Asa and Valdespino, 1998; Kleiman, 2011).
Kleiman and Malcolm (1981) categorized mammalian parental care into indirect care, which does not require physical contact with young, and direct, which does. Indirect care includes territory acquisition, maintenance and defense, shelter or den construction, anti-predator defense, and mate care through guarding and provisioning. Direct care includes huddling, grooming, transporting, feeding, active defense against predators or conspecifics and playing and socializing. In some species, indirect paternal care predominates (e.g., Blanford's fox [Vulpes cana], corsac fox [V. corsac], Geffen and Macdonald, 1992; Asa and Valdespino, 1998; Kleiman, 2011); in others, females spend more time with the pups while males provide food (e.g., gray fox, Nicholson et al., 1985; swift fox, Poessel and Gese, 2013); in yet others, males spend more time with pups than the mother and exhibit every care-giving behavior except lactation (e.g., bat-eared fox, Malcolm, 1986; Maas, 1993; Maas and Macdonald, 2004; Wright, 2006; African wild dog, Asa and Valdespino, 1998; raccoon dog [Nyctereutes procyonoides], Kauhala et al., 1998). At an extreme, Kleiman (2011) reports that captive female bush dogs call their mate while giving birth, and the male helps remove pups from the birthing canal, grooms the neonates, and may help in removing the placenta (see also Macdonald, 1996).
Provisioning both the pups and lactating mother is widely documented paternal care amongst canids (Asa and Valdespino, 1998). As Macdonald (1992) noted, regurgitation of partially digested food is widely described in the lupine canid lineage (present in all species in the genera Canis, Lycaon, Cuon, Chrysocyon and Speothos; Biben, 1982; Johnsingh, 1982; Rasmussen and Tilson, 1984; Asa and Valdespino, 1998; Lord et al., 2013) but absent in the vulpine lineage (though see Poessel and Gese, 2013). In these more carnivorous canids, this economical means of transporting prey to the den without the risk of kleptoparasitism is clearly advantageous (e.g., van Lawick-Goodall and Lawick-Goodall, 1970). African wild dogs can carry an estimated 3 days' worth of food in their stomachs to the pups and mothers (Reich, 1981; Creel and Creel, 1995), which allows males to successfully raise pups if the mother dies (Estes and Goddard, 1967). Species feeding on medium-sized prey can carry prey to the den, allowing both parents to provision young once they are old enough to be left alone at the den (e.g., red fox, Macdonald, 1977; arctic fox, Cameron et al., 2011), but for largely insectivorous canids, this is unfeasible. In largely termitivorous bat-eared fox (Klare et al., 2011), nursing mothers must spend >85% of the night foraging (Wright, 2003), leaving males primarily responsible for guarding, huddling and grooming cubs (Lamprecht, 1979; Malcolm, 1986; Maas, 1993; Maas and Macdonald, 2004; Wright, 2006). As bat-eared fox cubs begin foraging, the male accompanies them (Wright, 2006), acting as both protector and teacher, indicating patches of food to the cubs and occasionally pre-chewing larger beetles (Maas and Macdonald, 2004). A similar division of labor is reported amongst other insectivorous canids (e.g., raccoon dog, Kauhala et al., 1998; hoary fox [Lycalopex vetulus], Courtenay et al., 2006), where males compensate for their inability to directly feed cubs by guarding them.
An early theory by Moehlman (1986) posited that requirements for paternal investment in canids, along with other life-history traits, relate to body size. She argued that large canids have relatively smaller infants in larger litters, requiring heavy, prolonged post-partum parental (and alloparental) investment, whereas smaller canids have relatively larger young in smaller litters, requiring less post-partum parental investment. However, other studies concluded female weight was not a strong predictor of canid litter size (Bekoff et al., 1981; Geffen et al., 1996; but see Johnson et al., 2017) and that there was either no correlation between litter size and neonate weight (Geffen et al., 1996) or a positive correlation (Bekoff et al., 1981). Instead of body size, the need and capacity for paternal care is likely determined by diet. The greater energy requirements of large canids mean they must rely more heavily on carnivorous diets and thus larger prey, whereas smaller canids can be more omnivorous (Carbone et al., 1999; Slater, 2015). Large prey not only allow males to directly feed pups, but are also more difficult for young to learn to acquire, thus requiring a longer period of dependency and greater parental investment to ensure pups are fed; offspring even older than 1 year may be directly provisioned by adults by regurgitation in gray wolves (Mech et al., 1999) and black-baked jackals (Moehlman, 1986). With smaller omnivorous species there is less capacity and less need for males to provision young. For example, Blanford's fox males cannot economically carry insects to the cubs so they are entirely reliant on the mother's milk (Geffen and Macdonald, 1992). Diet, and more specifically prey size, therefore probably determines variations in paternal care (Kauhala et al., 1998).
Alloparental Care
Alloparental care by non-breeding adult “helpers” is widespread across canids (see Macdonald et al., 2004). Helpers are usually, but not invariably, related to the pups (e.g., Zabel, 1986, Sillero-Zubiri et al., 2004; Jedrzejewski et al., 2005). There are many examples of “helpers” benefiting pup survival. Cases of alloparenting allowing litter survival despite the mother's death have been documented in red fox (Macdonald, 1979a; von Schantz, 1984) and African wild dogs (Estes and Goddard, 1967). In black-backed jackals, the presence of one additional helper tended to result in survival of one additional pup (R2 = 0.89, Moehlman, 1979). Similar but much weaker associations between pup survival and the number of helpers are found in coyotes (Bekoff and Wells, 1982) and African wild dogs (Malcolm, 1979). The presence of helpers can lead to larger litter sizes in African wild dogs (Gusset and Macdonald, 2010; Angulo et al., 2013), perhaps by increased provisioning of pregnant females. In red wolves (Canis rufus) and Ethiopian wolves, helpers increased female lifetime reproductive success by extending the female's reproductive lifespan (i.e., age of last reproduction), thereby increasing the number of reproductive events and thus lifetime reproductive success (Sillero-Zubiri et al., 2004; Sparkman et al., 2011a). Helpers reduce time pups are left unattended at the den in African wild dogs (Courchamp et al., 2002) and Ethiopian wolves (Sillero-Zubiri et al., 2004) and can actively defend against predators (Macdonald, 1979a; Bekoff and Wells, 1982; Malcolm and Marten, 1982; Creel and Creel, 1995; Kamler and Gipson, 2000; Kamler et al., 2013a).
Other studies, however, have not found helpers to be beneficial. In Blanford's foxes, non-breeding adults were not observed providing direct care to the young (Geffen and Macdonald, 1992), though perhaps they provide indirect care by territory defense or pup guarding. Helpers did not affect offspring production and/or survival in studies of Arctic foxes (Kruchenkova et al., 2009), red foxes (Zabel and Taggart, 1989; Baker et al., 1998) and Ethiopian wolves (Sillero-Zubiri et al., 2004; Marino et al., 2012). It is, however, possible that helpers provided other benefits, such as acting as insurance if a parent dies by adopting the litter (as seen in red foxes: Macdonald, 1979a; von Schantz, 1984), or lightening the work load for the parents (as in Ethiopian wolves: Sillero-Zubiri et al., 2004), which may increase the breeding pair's future reproductive success (Marino et al., 2012, 2013). The benefit provided by helpers likely depends on ecological conditions. In gray wolves (Harrington et al., 1983) and African wild dogs (Malcolm and Marten, 1982), older siblings were observed feeding pups and thereby increasing pup survival only when there was a food availability surplus (Malcolm and Marten, 1982; Harrington et al., 1983). Furthermore, when food is scarce, not only do helpers not feed pups but will even steal food from them (Malcolm and Marten, 1982). Consequently, in lean years, pup survival can be negatively affected by competition with non-breeders (Harrington et al., 1983). Similarly, in red wolves, pup mass and survival positively correlated with the presence of helpers at low population densities, but negatively correlated with pup mass (though not survival) at high population densities. Furthermore, whilst the presence of helpers increases the breeding females' lifetime reproductive success, it can decrease the males' (Sparkman et al., 2011a). In African wild dogs, yearlings and pack size can increase pup survival (Malcolm and Marten, 1982) and there seems to be a minimum pack size threshold below which packs face an increasing probability of extinction due to the need of helpers for hunting, defense, and reproduction (i.e., an Allee effect) (Courchamp and Macdonald, 2001; Angulo et al., 2013). However, there also seems to be an optimal upper limit to pack size above which the increased competition can decrease pup survival (Macdonald and Carr, 1989; Creel and Creel, 1995). Theoretical research shows that if subordinates are related to the dominant pair, they do not need to have a positive effect to be accepted as group members and can even have a slight negative effect, if ecological constraints are such that they are unlikely to survive or reproduce if expelled from the group (Kokko et al., 2002). Dominants can increase their net fitness by allowing unhelpful or even damaging subordinates to remain in a group if it means they survive and can later reproduce (Kokko et al., 2002). Retaining subordinates in a group is often more for their benefit than that of the dominant pair (Kokko et al., 2002).
Evolution of Social Monogamy
Phylogenetic studies suggest that social monogamy has evolved independently perhaps as many as 61 times in mammals (Lukas and Clutton-Brock, 2013). Historically, suggestions for factors influencing its evolution include the need for biparental care, infanticide protection, and male mate guarding, each of which is discussed below.
Need for Biparental Care
The association between paternal care, social monogamy, and pair bonding led to an early emphasis amongst scholars on the need for biparental offspring care as the adaptive significance of monogamy (e.g., Orians, 1969; Kleiman, 1977; Wittenberger and Tilson, 1980; Kleiman and Malcolm, 1981; Clutton-Brock, 1989). These early hypotheses suggested that if females cannot successfully rear young without help and males cannot successfully divide care between multiple litters, both would benefit from social monogamy with biparental care (Kleiman, 1977; Wittenberger and Tilson, 1980; Kleiman and Malcolm, 1981; Malcolm, 1985; Birkhead and Møller, 1996). Canids typically have a single, large litter each year (modal litter size: 3–6, Hayssen et al., 1993) and young are altricial with a long dependency period, relative to other mammals (Asa and Valdespino, 1998; Lord et al., 2013). For example, it takes as long as 8 months for pups to reach independence in black-backed jackals, side-striped jackals (Lupulella adusta), African golden wolves and gray wolves (reviewed in Lord et al., 2013). The commonness of canid paternal care and the prolonged post-partum parental investment required thus supported these early views that the need for male help favored the evolution of canid monogamy (e.g., Kleiman, 1977).
However, several more recent phylogenetic analyses suggest mammalian paternal care likely evolved after monogamy and that paternal care is a consequence of social monogamy, not the cause (Komers and Brotherton, 1997; Brotherton and Komers, 2003; Lukas and Clutton-Brock, 2013; Opie et al., 2013). If both sexes are monogamous for other reasons, paternal care may be the best option to improve fitness (Emlen and Oring, 1977; Lukas and Clutton-Brock, 2013), particularly as in this situation there is high paternity assurance (Trivers, 1972; Queller, 1997; Kvarnemo, 2005; Fromhage and Jennions, 2016). Similarly, rather than large litter sizes requiring paternal care, litter size and paternal care likely coevolved (Stockley and Hobson, 2016). Canid litter sizes can be highly variable and dependent on food availability (Geffen et al., 1996; Marino et al., 2006, 2012), supported by field experiments in Arctic foxes showing provisioning increases litter sizes (Angerbjörn et al., 1991, 1995). Coevolution of paternal care and litter size resulted in larger litters which require paternal and even alloparental care to survive (Stockley and Hobson, 2016). If the need for bi-parental care was not what caused social monogamy to evolve, it is likely crucial to its maintenance (Klug, 2018).
Female choice likely contributed to the evolution of paternal care (Kvarnemo, 2005; Lukas and Clutton-Brock, 2013; Lambert et al., 2018). Kvarnemo (2005) suggested that if females select males that care for young, this can explain the correlation often observed between paternity and male care but in the opposite direction of causation to that often suggested (i.e., caring males are more likely to sire offspring, rather than males that sire offspring are more likely to provide care). This hypothesis has received far less attention than the reverse direction of causation and has been little investigated in mammals (but see Freeman-Gallant, 1996, Kvarnemo, 2005, and Alonzo, 2012 for support from invertebrates, fish, and birds). Nonetheless, there is evidence in at least one mammal: in a group-living monkey where males exhibit extreme degrees of care often toward unrelated young (Campbell, 2019), males that provide more care experience greater future mating success the following breeding season through female choice (Ménard et al., 2001). A male would therefore directly benefit from providing care, regardless of whether he cares for his own offspring or not. Sexual selection can therefore better explain cases where males care for unrelated young (e.g., red foxes: Baker et al., 2004; bat-eared foxes: Wright et al., 2010; wolves: Cassidy et al., 2016) than natural selection (Kvarnemo, 2005; Alonzo, 2012). However, this process would only be possible where females are able to assess males' investment in young before mating and bias mating toward those males (Alonzo, 2012). While this may not apply to the majority of socially monogamous taxa that display seasonal serial monogamy, finding new partners each year (see section Pair Bonding), the long-term partnerships of canids could allow females to bias paternity based on male care provided to the previous year's litter. Male canids can vary in the quality of paternal care bestowed (e.g., bat-eared foxes, Wright, 2006), making this trait subject to sexual selection. Furthermore, even with large litters, a female can compensate for the male's work when he is absent or reduces investment (although at apparent cost to her health and survival, therefore compromising potential future reproductive success) (Sacks and Neale, 2001; Cameron et al., 2011) and females may adjust effort according to litter size (Mech et al., 1999), supporting that male care can be a female preference, rather than pure necessity. Lambert et al. (2018) suggested that monogamy and paternal care co-evolved when selection initially favored affiliative males, which subsequently evolved into paternal care.
Protection Against Male Infanticide
It had been hypothesized that infanticide may select for social monogamy in mammals. If females deter infanticide by mating promiscuously to confuse paternity, males may counter by guarding mates to ensure paternity and protect offspring (e.g., Wolff and Macdonald, 2004; Lukas and Huchard, 2014). However, phylogenetic analysis across mammals concluded that social monogamy did not evolve from high infanticide levels and that social monogamy and infanticide seemingly evolved independently (Lukas and Clutton-Brock, 2013; Lukas and Huchard, 2014).
Male Mate Guarding
Several studies concluded, based on phylogenetic and empirical evidence, that mammalian social monogamy evolved where males could not defend multiple females (Komers and Brotherton, 1997; Brotherton and Komers, 2003; Lukas and Clutton-Brock, 2013), such as when females are solitary and occupy exclusive ranges at low density (Emlen and Oring, 1977, but also see Dobson et al., 2010). High-quality but scarce or patchy resources likely provided the selective pressures leading to social monogamy by increasing female feeding competition, resulting in female territoriality and intolerance. If breeding is also seasonal and synchronized, as with most canids (Asa and Valdespino, 1998), the temporal availability of oestrous females is also limited, such that males cannot effectively guard more than one (Lukas and Clutton-Brock, 2013). This combination of factors, making it more beneficial for males to monopolize their current partner than seeking others, is a powerful explanation for the evolution of mammalian social monogamy (Komers and Brotherton, 1997; Brotherton and Komers, 2003; Lukas and Clutton-Brock, 2013).
Maintenance of Social Monogamy
Social monogamy should be maintained only if (a) it is the optimal strategy for both the male and female, or (b) it is the optimal strategy for one sex and enforcement mechanisms are employed to prevent their mate from adopting other strategies, referred to as “voluntary” and “enforced” monogamy, respectively (Kvarnemo, 2018).
Voluntary Monogamy: Monogamy as the Optimal Strategy
If bi-parental care is highly beneficial or necessary for offspring survival, social monogamy may be the optimal strategy for both sexes. Because most canids are seasonal breeders (Asa and Valdespino, 1998; Lord et al., 2013), investment in one female limits the investment males can make in another (Kleiman and Malcolm, 1981). In bat-eared foxes, the best predictor of the number and proportion of surviving young is the amount of male den attendance (rather than parental age/size, territory quality, and maternal den attendance) (Wright, 2006). Males spend 30–57% of their time at the den, and a 10% increase in attendance corresponds with a 16% increase in cub survival, with complete litter survival when a male spends 49% of his time at the den (Wright, 2006; Wright et al., 2010). Males therefore cannot care for litters at two dens without severely sacrificing offspring survival at one or both. Similarly, male raccoon dogs may be required to keep pups warm while the female forages (Kauhala et al., 1998) and in black-baked jackals, an entire litter died following the male's death (Moehlman, 1986).
Enforced Monogamy: Reproductive Suppression of Subordinates
In group-living canids, social monogamy is commonly enforced by reproductive suppression of subordinates (Moehlman, 1989; Creel and Creel, 1991; Creel and Macdonald, 1995; Moehlman and Hofer, 1997; Asa and Valdespino, 1998). In a review of 25 canid species, Moehlman and Hofer (1997) found reproductive suppression in 44%. The mechanism often involves copulation interference and aggression toward same-sex individuals attempting to breed (e.g., gray wolf, Rabb et al., 1967; Derix et al., 1993; African wild dog: Malcolm, 1979; red fox: Macdonald, 1979a) and infanticide, either by directly killing subordinates' pups (African wild dog: van Lawick, 1973; dingo [C. familiaris dingo]: Corbett, 1988) or indirectly, such as interfering with provisioning of subordinates' pups (African wild dog: Frame et al., 1979) or causing subordinate mothers to become so excessively anxious that their offspring die from the mother's fretfulness (red fox: Macdonald, 1979a; bush dog: Macdonald, 1996).
Physiological mechanisms of reproductive suppression are less documented in canids, but there is evidence for them (e.g., African wild dog: Creel et al., 1997; coyote: Moehlman and Hofer, 1997; Spiering et al., 2010; Ethiopian wolf: van Kesteren et al., 2012, 2013). In Ethiopian wolves, typically only the dominant females breed during a short mating season (Sillero-Zubiri et al., 1998). A study of adjacent Ethiopian wolf packs demonstrated increased oestradiol concentration in feces from eleven dominant females but not in the nine subordinates sampled and no aggression from the dominant female preventing subordinate breeding was documented, indicating hormonal suppression of subordinate females (van Kesteren et al., 2013). Although female gray wolves can breed as yearlings (Medjo and Mech, 1976), they rarely do in the wild before age three, suggesting subordinate females may experience delayed maturation or suppressed oestrus. In African wild dogs, subordinate females were hormonally suppressed, preventing ovulation likely by elevated estrogen and estrogen/progestin ratios (Creel et al., 1997). However, the detailed physiological mechanisms involved in reproductive suppression remain unclear in many species; increased glucocorticoids from social stress is a mechanism of reproductive suppression in some other taxa (e.g., Hackländer et al., 2003), but no evidence of this has been found in canids (African wild dog: Creel et al., 1997; van Kesteren et al., 2013). Glucocorticoid levels in female African wild dogs did not differ according to dominance status (average fecal glucocorticoid concentration for dominant breeding females during the mating season was 207.47 ± 43.69 (SE) ng/g while for subordinate non-breeding females it was 202.5 ± 52.3 ng/g), suggesting other mechanisms were responsible for reproductive suppression (van Kesteren et al., 2013).
Reproductive suppression of subordinates may not be solely for the benefit of the dominant pair. Packard et al. (1983) suggest that deferred reproduction in gray wolves could have evolved by individual selection, as future reproductive fitness may be enhanced by remaining longer in a juvenile role in the native pack. Similarly, delayed dispersal in red wolf males lowers mortality, thereby increasing the chances of becoming reproductive (Sparkman et al., 2011b). Kokko and Johnstone (1999) showed that the delayed benefits of acquiring dominant status in the future (“social queuing”) can provide enough incentive for subordinates to remain peacefully in a group without themselves breeding. Additionally, if larger groups experience greater survival (e.g., African wild dogs, Carbone et al., 1999), individuals may experience greater benefits by remaining as a non-breeding subordinate and helping to raise new group members, rather than dispersing to breed alone (Kokko et al., 2001). Furthermore, subordinates increase their inclusive fitness by helping to raise and improve the survival of their parents' next litter, since they are on average as closely related to their siblings as they would be to their own offspring (Moehlman, 1983, 1986).
Social dominance may play a role in reproductive suppression. Macdonald (1979a, 1987) reported that, although normally only the alpha red fox female bred, when the dominance status of the previously-alpha female waned until becoming equal with another vixen in the group, both conceived the following year. Similarly, Zabel (1986) observed that although a clear dominance relationship existed between dominant breeding females and submissive non-related helpers, social groups with two breeding females had no obvious female dominance hierarchy.
Subordinate reproduction can also be thwarted by expelling them from the group (Jungwirth and Johnstone, 2018) or not allowing subordinates to join (e.g., females “floating” on the periphery of Ethiopian wolf groups, Sillero-Zubiri et al., 1996a; male bat-eared foxes are aggressively territorial toward young males that intrude on the territory, Maas and Macdonald, 2004). Intrasexual aggression is common in canids (e.g., Rabb et al., 1967; Kleiman and Malcolm, 1981; Zabel, 1986), as is the expulsion of same-sex subordinates. The pros and cons of tolerating additional group members are explored by Macdonald and Carr (1989). In Ethiopian wolf packs with more than one subordinate female, the mother expelled the lowest-ranking female at 18–28 months old, with assistance from the dominant sister (Sillero-Zubiri et al., 1996a). Similarly, pregnancy in subordinate Ethiopian wolves can result in pack splitting, thus restoring social monogamy (Marino et al., 2013).
Interestingly, one strategy for subordinate females to avoid reproductive suppression is to raise a litter at the edge of their parent's territory, typically with a subordinate male from a neighboring group, seen in crab-eating foxes (Macdonald and Courtenay, 1996), red foxes (Baker et al., 2000), black-backed jackals (Kamler et al., 2019) and gray wolves (Mech and Boitani, 2003). In this way, subordinates may get the best of both worlds by avoiding risky dispersal into unknown areas yet allowing reproduction. However, the tolerance of alphas letting betas raise litters and use their territory edges might vary according to food abundance and dispersion and kinship.
Ecological Correlates of Social Monogamy
Social monogamy is maintained because either it is the optimal strategy for both sexes, or because polygamy (incl. polygyny, polyandry, polygynandry) is restricted due to monogamy enforcement mechanisms or ecological conditions. Ecological conditions can shift monogamy to other social systems, either by affecting the benefits (motivations) of social monogamy itself or by affecting the ability to enforce it (summarized in Table 1).
Resource Availability: Paternal Care and the Polygyny Threshold Model
One of the primary benefits of social monogamy in canids is benefits to offspring survival due to biparental care (Moehlman, 1989), though resource availability affects the degree to which male care is both necessary and feasible: when resources are plentiful, females may successfully raise pups with less male input (Maas, 1993) and males are able to provide more paternal care (Wright, 2006). The polygyny threshold model (Verner, 1964, Verner and Willson, 1966, Orians, 1969) posits that there is a threshold at which a female can raise as many young sharing a male and territory of higher quality (in a polygynous system) as she could being the sole female with an inferior male/territory (in a monogamous system). Thus, if male help is required and a male cannot share care between multiple litters without decreasing the quality and offspring survival below what could be achieved with his full attention on a single litter, monogamy would be the optimal strategy. If, however, resources are such that a female can raise as many offspring in a polygynous system, either because male help is less needed or because a male can provision multiple litters as well as he could one, the “polygyny threshold” can be crossed. Supporting this, Zabel and Taggart (1989) report that when food availability was high, 71% of the island population of red foxes they studied were bigamous, i.e., a single male cared for the litters of two females, and bigamous females had equal or greater (1.4 times) reproductive success than monogamous females when considering offspring survival in the first year (mean litter size of 4.3 ± 0.29 in bigamous vs. 4.0 ± 1.0 in monogamous females, Zabel and Taggart, 1989). However, when the food supply crashed, the population shifted entirely to monogamy. Red foxes in Sweden also displayed polygyny with multiple breeding females when prey availability was high but a single breeding female when prey availability was low (von Schantz, 1984, see also West, 2014). Similarly, food availability influenced the probability of forming larger groups across four populations of arctic fox, with variations including polygyny, plural breeding and communal breeding where food abundance differed substantially between years, whereas social monogamy is found in areas of stable resources (Angerbjörn et al., 2004; Elmhagen et al., 2014) and in marginal habitats with low food availability (Norén et al., 2012; Elmhagen et al., 2014).
Social Structure
As described in Box 1, canid social monogamy is not restricted to pair-living individuals but also includes group-living variations where social groups contain a single breeding male and female, in addition to non-breeding group members. Alternatively, canid groups may exhibit polygyny, polyandry, or polygynandry with multiple breeding adults. Social monogamy is, unsurprisingly, most common in smaller social groups (Clutton-Brock and Isvaran, 2006; Spiering et al., 2010). A greater availability of potential breeders is more difficult for the dominant pair to suppress (Marino et al., 2013). Spiering et al. (2010) found that many packs of African wild dogs contain only one adult female and thus inevitably only one breeding female. However, in the 30% of groups containing subordinate females, only half were socially monogamous: beta females also bred in 54.5% of years, though theta females never bred. In contrast, subordinate males always secured some paternity, but were only present in 47% of groups. Similarly, in bat-eared foxes studied by Maas and Macdonald (2004), social monogamy depended on the number of females in the group—additional females invariably bred, and in only 1 of 65 breeding events was there a non-breeding adult male in the group. In Ethiopian wolves, packs recovering from disease outbreak can become unusually large and contain more than two subordinate females, increasing the likelihood of pregnancy in subordinate females (Marino et al., 2013).
The mechanisms shaping sociality in carnivores, and in canids specifically, have been reviewed elsewhere (e.g., Macdonald, 1983; Creel and Macdonald, 1995; Macdonald and Sillero-Zubiri, 2004; Macdonald et al., 2004). Canid groups generally form by retention of offspring that do not disperse (e.g., black-backed jackal: Moehlman, 1979, 1983; red fox: Macdonald, 1980; arctic fox: Hersteinsson and Macdonald, 1982; kit fox: Ralls et al., 2001; bat-eared fox: Maas and Macdonald, 2004; hoary fox: Courtenay et al., 2006; Kamler et al., 2013b, 2019; Cape fox: Kamler and Macdonald, 2014) and thereby avoid dispersal costs (Bekoff and Wells, 1982; Macdonald and Carr, 1989; Lucas et al., 1994; Kamler et al., 2019). This results in family groups, though unrelated individuals can sometimes join existing groups (e.g., red fox: Zabel and Taggart, 1989; gray wolf: Jedrzejewski et al., 2005). Macdonald and Carr (1989), drawing heavily on canid examples, presented a profit and loss account of tolerating additional group members. A primary cost is food competition (Schmidt and Mech, 1997; Creel and Creel, 2002), but the list also includes increased risk of infectious disease (e.g., rabies, Macdonald and Bacon, 1982; Loveridge and Macdonald, 2001) and parasite transmission (Hoogland, 1979), and mate sharing (Zabel and Taggart, 1989; Spiering et al., 2010).
The adaptive functions of canid groups include greater hunting success (e.g., African wild dogs: Fanshawe and Fitzgibbon, 1993; Creel and Creel, 1995) and capacity to tackle larger prey (Fanshawe and Fitzgibbon, 1993). Larger African wild dog groups better defend food against spotted hyenas (Crocuta crocuta, Fanshawe and Fitzgibbon, 1993; Carbone et al., 2005), larger golden jackal groups can steal food from smaller groups (Macdonald, 1979b) and packs of dholes can steal prey from leopards (Venkataraman and Johnsingh, 2004). Larger groups also benefit territory defense, as victory in intergroup contests generally goes to the larger group (e.g., Ethiopian wolves: Sillero-Zubiri and Macdonald, 1998; Marino et al., 2012; gray wolves: Cassidy et al., 2017). Grouping can also increase breeding success through alloparental care (e.g., Moehlman, 1979) and decreased predation vulnerability (Kamler et al., 2013a). Sociality can also provide thermoregulatory, energetic and physiological benefits through social thermoregulation (Campbell et al., 2018). Though social thermoregulation is little studied in canids, Hennemann et al. (1983) found that crab-eating foxes reduced heat loss and oxygen consumption (a measure of basal metabolic rate) by 5–18% when huddling with a partner, suggesting huddling can significantly impact daily energy expenditure in this and other canid species (Hennemann et al., 1983).
Large prey can favor cooperative hunting and larger groups (e.g., coyotes: Bowen, 1981). Intense intraspecific and interspecific competition and predation may favor group formation for strength in numbers: recolonizing gray wolves, displaying intra-guild aggression toward coyotes, led coyotes to form larger groups (Arjo and Pletscher, 1999) and higher jackal numbers increased bat-eared fox group sizes (Kamler et al., 2013a). Similarly, arctic foxes tend to form complex groups when facing greater predation pressure from red foxes (Norén et al., 2012) and a mother-daughter pair merged their litters into one den when facing red fox predation, despite low food availability at the time (B. Elmhagen, unpublished data, from Norén et al., 2012).
The costs of dispersal increase when the journey is hazardous and/or the availability of vacancies is low (Ballard et al., 1987; Norén et al., 2012). Therefore, population density, likely linked to food availability, affects the advantages of group formation such that polygamy can be associated with high population density (e.g., swift foxes, Kamler et al., 2004a; red foxes, Baker et al., 2004; Iossa et al., 2008a; gray foxes, Weston Glenn et al., 2009). Iossa et al. (2008a) found 60% of red fox groupings were socially monogamous at low population density but 23% at high population density. Similarly, in swift foxes at high population density from low predation pressure, 30% of social groups exhibited polygyny with communal denning and 40% included non-breeding females (in 10 social groups), whereas in low density/high predation pressure, only monogamy was observed, with no non-breeding helpers (16 groups, Kamler et al., 2004a).
Finally, group formation may occur not only when groups are beneficial or dispersal is costly, but rather when grouping carries little cost (Macdonald and Carr, 1989; Macdonald and Johnson, 2015). The resource dispersion hypothesis (RDH, Macdonald, 1981, 1983; Carr and Macdonald, 1986) posits that when resources are dispersed heterogeneously, the minimum territory needed to meet a breeding pair's resource requirements can often support additional group members with little or no cost to the dominant pair. Greater heterogeneity leads to larger group sizes. Macdonald (1980, 1987) reviewed the early literature to show that monogamous red fox pairs are associated with spatio-temporally homogeneous resources (e.g., farmlands of USA Midwestern states, Storm et al., 1976), often at low population density, whereas groups more commonly form where food availability is more spatio-temporally heterogeneous and foxes that exploit cyclic rodent populations may accommodate additional group members in peak rodent years (Macdonald, 1984; von Schantz, 1984; Elmhagen et al., 2014; see also Macdonald et al., 2016). Similarly, if the cost of tolerance is low, its benefits may be minimal: Geffen and Macdonald (1992) report dominant Blanford's fox pairs tolerating non-breeding subordinate vixens, but found no evidence that they act as helpers.
The balance of these costs and benefits of group formation, determined by local ecological conditions, will therefore influence the social structure of canids and thus whether they exist as a socially monogamous pair, one of the variations on social monogamy described above, or depart from monogamy altogether.
Contrasting Canid Social and Genetic Mating Systems: Potential Functions and Ecological Correlates of Extra-pair Mating in Canids
Social monogamy is no guarantee of genetic monogamy, i.e., exclusive mating (Klug, 2018; Lambert et al., 2018), and, indeed, almost every genetically studied canid species has revealed extra-pair paternities (EPP) (see Hennessy, 2007 for an exception). For example, extra-pair males sired 25% of 16 offspring in Island foxes (Roemer et al., 2001), 52% of 19 offspring from 15 litters in swift foxes (Kitchen et al., 2006), 31% of 176 offspring in arctic foxes (Cameron et al., 2011) and in red foxes from 38% of 38 offspring (Iossa et al., 2008b) to as much as 80% of 30 offspring (Baker et al., 2004). Many of these examples are drawn from pairs, rather than larger social groups.
In group-living canids, extra-pair mating can occur both within-pack and with extra-pack individuals. In Ethiopian wolves, despite the dominant pair's apparent social monogamy, extra-pair copulations (EPC) happen both within (rarely) and outside (more commonly) the pack (Gottelli et al., 1994): Sillero-Zubiri et al. (1996a) observed that 70% of copulations were between a female and male in adjoining packs and Randall et al. (2007) found that 50% of litters had offspring sired by an extra-pack male, with 28% of offspring with resolved paternities sired by extra-pack males. Though red wolves were found to be highly genetically monogamous, with only 4 of 174 litters (2%) showing EPP, these rare cases included extra-pair mating within and outside of the pack (Sparkman et al., 2012). In contrast, two studies in African wild dogs found that, although extra-pair mating was common, extra-pack males never sired offspring (of 226 offspring, Spiering et al., 2010, and 39 offspring, Moueix, 2006); when subordinate males existed in a pack, levels of mixed paternity in litters were 53% (of 15 litters, Spiering et al., 2010) and 100% (of 5 litters, Moueix, 2006).
Benefits and Costs of Genetic Polygyny
For males, whose reproductive success is generally limited by access to females, the benefit of extra-pair mating is obvious: mating with additional females can directly increase reproductive success by producing more offspring, and especially when these are cared for by another male. For example, male red foxes studied by Baker et al. (2004) sired more offspring with extra-pair females than with their social mate and traveled as far as 2.7 territories away during extra-territorial forays; consequently, they could have sired offspring in as many as 32 neighboring groups (Baker et al., 2004). Such males benefit doubly, genetically and from the parental investment of cuckolded males, thus extra-territorial forays are widely recorded amongst canids during the courtship and mating periods (e.g., Macdonald, 1981; Zoellick and Smith, 1992; Baker et al., 2004; Deuel et al., 2017; Kamler et al., 2017, 2019). However, the costs of male philandering include leaving their mate unguarded and therefore increasing their own risk of being cuckolded, increased exposure to sexually transmitted disease and parasites (Poiani and Wilks, 2000; McLeod and Day, 2014), and risks of mortality, predation, intraspecific conflict and stress when traveling in unfamiliar areas (Harris and Smith, 1987; Young and Monfort, 2009).
Benefits of Genetic Polyandry
For females, whose reproductive output is limited, the benefits of extra-pair mating are less obvious. Various hypotheses have been proposed to explain why females engage in extra-pair mating (summarized in Table 2).
Increase Genetic Quality
A favored explanation for extra-pair mating in birds is increased genetic fitness of offspring by mating with the highest quality males (Birkhead and Møller, 1996; Jennions and Petrie, 2000; Westneat and Stewart, 2003). In monogamous social systems where most individuals are paired, mate choice is constrained and thus most females would be partnered with suboptimal males. The majority of females would therefore benefit from seeking EPC with superior males. This may be the motivation for extra-pair mating by female red foxes studied by Iossa et al. (2008a,b). Red fox females typically engaged in EPC with dominant males from adjoining territories (Iossa et al., 2008a) and extra-pair males that sired offspring were always larger than the female's cuckolded social partner (Iossa et al., 2008b). By mating with males of higher quality than their partner, females can increase the genetic quality of their offspring. Similarly, three of four cases of EPP observed in Island foxes were by the two largest males in the study (Roemer, 1999, 2004), suggesting females engaged in extra-pair mating with high-quality males. Furthermore, multiple mating may also increase genetic quality of offspring by inciting sperm competition and allowing cryptic female choice (e.g., Kvarnemo and Simmons, 2013; Annavi et al., 2014).
Genetic quality, however, is not the only factor, as illustrated by female Ethiopian wolves that mate outside their pack being notably unselective about the dominance status of these mates (Sillero-Zubiri et al., 1996a; Randall et al., 2007) and similar observations of other red foxes being unselective outside, but selective within, the group in regards to male dominance status (Baker et al., 2004). While it is possible that females select for genetic quality using indicators other than dominance status (which is often influenced by size, health, strength), these studies suggest that, at times, other explanations are involved besides quality of the extra-pair mates (especially considering females are likely well-informed of their neighbours' social status). The case of Bristol's urban red foxes is revealing in showing how motivations for extra-pair mating can change: in 1992–1994, females appeared to be unselective in the quality of extra-group males, mating with both dominant and subordinate males (Baker et al., 2004). In 1994–1996, the population declined by 80% due to mange (Baker et al., 2000; Iossa et al., 2008a). Subsequently, in 2002–2004, females became highly selective, reducing the frequency of extra-pair mating and mating only with males that appeared to be of higher quality than their social mate (Iossa et al., 2008b). Furthermore, rates of mixed paternity dropped from 38–69% pre-outbreak to 0% post-outbreak (Baker et al., 2004; Iossa et al., 2008b). Thus, it seems that following substantial pressure from disease, females changed their reproductive strategy to emphasize genetic quality, which may increase the probability of their offspring surviving disease.
Increase Genetic Diversity
Canid litters can be sired by multiple males. Thus, polyandry might function to increase within-litter genetic diversity (Yasui, 1998; Jennions and Petrie, 2000; Slatyer et al., 2012). This was proposed to explain polyandry in arctic foxes, where 26% of litters were sired by multiple males (Cameron et al., 2011). In fluctuating environments, such as the harsh arctic, the fittest genes may be unpredictable and thus increased within-litter genetic diversity may increase the probability that at least some offspring survive (Yasui, 1998; Jennions and Petrie, 2000). The majority of cases where female canids engage in EPC result in mixed paternity litters (e.g., Baker et al., 2004; Moueix, 2006; Randall et al., 2007; Spiering et al., 2010; Cameron et al., 2011; Converse, 2013, but see Iossa et al., 2008a,b and Cameron et al., 2011 for exceptions). Increased within-litter genetic diversity may therefore be a common motivation for extra-pair mating in canids.
Inbreeding Avoidance
Extra-pair mating may function to prevent inbreeding (Stockley et al., 1993; Jennions and Petrie, 2000; Tregenza and Wedell, 2002; Annavi et al., 2014; Arct et al., 2015). This may be particularly important where territories are inherited by successive generations or there is a lack of dispersal (e.g., Ethiopian wolf, Sillero-Zubiri et al., 1996a; bat-eared fox, Maas and Macdonald, 2004), leading to highly related groups. However, in most canids that have been genetically investigated, mated pairs are generally unrelated (e.g., African wild dog: McNutt, 1996; Girman et al., 1997 [average relatedness of social pairs: 0.05 ± 0.11, N = 5]; gray wolf: Smith et al., 1997 [0.01 ± 0.14, N = 16]; arctic fox: Cameron et al., 2011 [0.01 ± 0.14, N = 13]; kit fox: Ralls et al., 2001 [−0.07 ± 0.07, N = 10]; coyote: Hennessy, 2007 [0.00 ± 0.14, N = 7]; swift fox: Kitchen et al., 2006 [−0.01 ± 0.23, N = 48]; red wolf: Sparkman et al., 2012, R < 0.50 for 95% of 174 mating events). Although there are occasional instances where social pairs are closely related (e.g., Hennessy, 2007: 1/7 coyote pairs, R = 0.26; Kitchen et al., 2006: 1/48 swift fox pairs, R = 0.48; Weston Glenn et al., 2009: one gray fox pair, R = 0.36; Roemer, 1999: 4/15 Island fox pairs, R = 0.19, 0.35, 0.35, 0.52; Jedrzejewski et al., 2005: one gray wolf half-sibling pair), there are also cases where extra-pair mating occurs between relatives. For example, Baker et al. (2004) found four incestuous EPPs between close relatives with experiential histories (mother-son for 2 years, father-daughter, and half-brother-half-sister) and three additional pairings between more distantly related individuals (e.g., R = 0.13) in red foxes, Cameron et al. (2011) found one incestuous mother-son case of EPP (of 13 mated pairs, 7.6%) between arctic foxes that were socially paired with non-relatives, and Sparkman et al. (2012) found 4 parent-offspring and 4 full-sibling matings (of 174 mated pairs, 9%). There may be greater risk of this where there is neighborhood settlement by dispersers, as in crab-eating foxes (Macdonald and Courtenay, 1996), bat-eared foxes (Kamler et al., 2013b), swift foxes (Kitchen et al., 2005b) and black-backed jackals (Kamler et al., 2019). When it has been investigated, relatedness between social mates and extra-pair mates did not differ (e.g., Cameron et al., 2011: mean ± SD relatedness between social mates: 0.05 ± 0.12, N = 9; between extra-pair mates: −0.09 ± 0.11, N = 4). It therefore seems that canids achieve inbreeding avoidance by other mechanisms, such as avoiding mating within ones' natal pack, sex-biased dispersal and adult dispersal (Kamler et al., 2004c, 2013b; Geffen et al., 2011; Sparkman et al., 2012; Kamler and Macdonald, 2014).
One exception, however, could be in Ethiopian wolves. A lack of dispersal opportunities from shrinking habitat, coupled with male philopatry, results in highly related packs (Sillero-Zubiri et al., 1996a; Randall et al., 2007). Sillero-Zubiri et al. (1996a) observed that the majority (70%) of copulations by female Ethiopian wolves were with males from adjoining packs, rather than her own pack, and thus extra-pack mating was suggested to be an inbreeding avoidance strategy. Females rejected advances from all males within their packs except those from the alpha male, yet were unselective concerning the status of extra-pack males with which they mated, suggesting outbreeding was of importance rather than mate quality (Sillero-Zubiri et al., 1996a). However, subsequent research found that, although packs are indeed highly related (mean pairwise relatedness within packs was 0.39) and there is a high prevalence of incestuous pairing (22% of mating pairs within packs were closely related [R = 0.18–0.44]), members of neighboring packs were also closely related so incestuous pairing occurred with both within-pack and extra-pack mating (33% of extra-pack mating pairs were closely related [R = 0.42–0.44]). In this case, female dispersal appears to contribute more than extra-pack mating to reduce inbreeding (Randall et al., 2007). Was this an artifact of the unusual, modern, circumstances of these wolves? Perhaps extra-pack mating evolved as an inbreeding avoidance strategy, but modern conditions, exacerbated by recurrent rabies outbreaks (Mebatsion et al., 1992; Sillero-Zubiri et al., 1996b; Whitby et al., 1997; Randall et al., 2004, 2006; Marino et al., 2006), changes in dispersal and demographic events led to neighboring packs and breeding pairs being more closely related than during evolutionary time (Randall et al., 2007).
Infanticide Protection by Paternity Confusion
Based on evidence across 33 mammal families, Wolff and Macdonald (2004) concluded that the most convincing explanation for polyandry across mammals is paternity confusion to deter infanticide. This hypothesis, originally proposed by Hrdy (1974, 1979), relies on female promiscuity being an effective counterstrategy against male infanticide (Lukas and Huchard, 2014) and predicts that females mate with many males. However, a complication is that, in contrast to some taxa (notably felids, see Macdonald et al., 2010), male infanticide in canids would not hasten female oestrus because most canids are seasonal breeders (Asa and Valdespino, 1998; Valdespino et al., 2002; Lord et al., 2013), notwithstanding some possible exceptions in African wild dogs (Frame et al., 1979), bat-eared foxes (Rosenberg, 1971), and bush dogs (Porton et al., 1987). This raises the question of what male canids could gain by infanticide. Indeed, amongst canids infanticide appears most commonly practiced by females (e.g., African wild dog: van Lawick, 1973; coyote: Camenzind, 1978; dingo: Corbett, 1988; gray wolf: McLeod, 1990; Ethiopian wolf: Sillero-Zubiri et al., 1996a; Girman et al., 1997), either as suppression of subordinate breeding attempts by the dominant female (Corbett, 1988; McLeod, 1990; Sillero-Zubiri et al., 1996a; Girman et al., 1997) or perhaps to increase availability of breeding territories or dens (see Zabel, 1986). Although examples of male infanticide are numerous amongst ursids and felids (e.g., Loveridge et al., 2007), we know of none in canids. The closest reports seem to be a description of a lone female red fox being harassed by males who intruded on her den and eventually the entire litter died (Zabel, 1986; Zabel and Taggart, 1989) and Latham and Boutin (2011) suggested that the death of a gray wolf pup may have been infanticide by a male, though the evidence was inconclusive and might best be explained by intergroup resource competition. Furthermore, in contrast to infanticidal male takeovers in other taxa (e.g., Loveridge et al., 2007), quite the opposite has been reported in gray wolves: when a new and unrelated alpha wolf takes over a pre-existing pack, he provisions and cares for pups that are not his own, which may increase his acceptance by the pack (Cassidy et al., 2016). Thus, paternity confusion to prevent infanticide is an unconvincing explanation for extra-pair mating in canids.
Fertilization Assurance
Females may engage in EPC for fertilization assurance to guard against male infertility (Wetton and Parkin, 1991; Hoogland, 1998; Hasson and Stone, 2009). Canids are unusual among Carnivora in that they are monoestrous, having only one ovulation event each season (Asa and Valdespino, 1998; see section Monoestrum), making the stakes high if a female's mate is infertile. Multiple mating may guard against this possibility.
Alloparental Care From Paternity Confusion/Dilution
In communal or cooperative breeding situations, selective female promiscuity with group members could be beneficial by confusing or diluting paternity and thus potentially increasing offspring care, particularly when paternal care is indivisible. This would not apply to most cases of EPC in canids where mating occurs outside of the social group (e.g., Sillero-Zubiri et al., 1996a; Baker et al., 2004). However, in African wild dogs, despite previous beliefs that only the alpha pair breeds, research found females frequently mate with subordinate males and documented high levels of paternity sharing, though none of the offspring analyzed (39 pups, Moueix, 2006; 226 pups, Spiering et al., 2010) were sired by extra-pack males. Spiering et al. (2010) found that the three top-ranking males always sired pups, or, if there were only two males in a pack, they shared the litter's paternity equally (similarly see Moueix, 2006). Male African wild dogs invest heavily in offspring care (Creel et al., 2004) so by mating with multiple males in a group, females may dilute paternity and increase the amount of care for her offspring while also increasing within-litter genetic diversity.
Costs of Genetic Polyandry
Like males, females engaging in extra-pair mating risk increased exposure to sexually transmitted disease and parasites (Poiani and Wilks, 2000; McLeod and Day, 2014) and increased stress (Young and Monfort, 2009) and mortality (Harris and Smith, 1987). Additionally, females may lose investment in her offspring by her mate or other group members.
Loss of Paternal Care
If males adjust investment according to confidence in paternity (Trivers, 1972; Møller and Birkhead, 1993; Sheldon, 2002), females should be less likely to seek EPCs when paternal care is important (Mulder et al., 1994; Westneat and Stewart, 2003; Lambert et al., 2018). Such reduced paternal investment by males with unfaithful partners is observed in arctic foxes: Cameron et al. (2011) found that, in faithful partnerships, den attendance rates were similar for males and females, whereas cuckolded males showed a 56% reduction in den attendance compared to non-cuckolded males and a non-significant 52% reduction in food provisioning. Overall rates of food provisioning did not differ between litters, meaning greater burden of care was placed on unfaithful females. The potential costs to males were substantial: 11% of litters were cared for by a male that did not sire any of the offspring. In contrast, in bat-eared foxes, cuckolded males did not invest less than other males (Wright et al., 2010). One explanation for this difference is that, because canids can have mixed paternity litters, when male care is indivisible among pups, such as vigilance against predators, females may be able to get away with some EPP without reducing male investment. Amongst bat-eared foxes, male den attendance is important and cannot be split amongst the young, whether sired by that male or not; conversely, food provisioning by male arctic foxes could be preferentially directed toward their own progeny, although this is untested (Wright et al., 2010; Cameron et al., 2011). However, this presupposes males can recognize their own offspring, which may be unlikely considering success of cross-fostering in coyotes (Kitchen and Knowlton, 2006), red wolves (Gese et al., 2015), gray wolves (Goodman, 1990; US Fish Wildlife Service, 2004) and dingoes and African wild dogs (Kitchen and Knowlton, 2006) and cases where cuckolded male foxes care for litters sired entirely by other males (Baker et al., 2004; Cameron et al., 2011). An alternative explanation for this difference between arctic and bat-eared foxes relates to the potential risk and costs. Comparative research across taxa suggests males reduce investment when cuckolded only when there is high cost and high risk of cuckoldry (Griffin et al., 2013). EPP was twice as frequent in arctic foxes as in bat-eared foxes and arctic males faced high potential costs of caring for litters sired entirely by other males (Wright et al., 2010; Cameron et al., 2011). A third explanation relates to female choice: if females preferentially mate with males that provide care, males can increase future breeding success by caring even for unrelated young (Kvarnemo, 2005; Alonzo, 2012; see section Need for Biparental Care). If females adjust the amount of extra-pair mating according to levels of male care (as in some birds: Freeman-Gallant, 1996), this can even paradoxically result in greater male investment when cuckolded to avoid losing future breeding opportunities.
Loss of Alloparental Care
A similar potential cost to engaging in extra-pair mating is the possible loss of alloparental care by philopatric young (who might otherwise be assumed to be related to new pups as full sibs). However, although there is little research on how EPP affects alloparental care, increased inclusive fitness is not the only motivation for alloparenting. Helpers are not always related to pups (Zabel, 1986) and may receive other benefits, such as inheritance of dens/territories (Lindström, 1986; Zabel, 1986; Kokko et al., 2002; Marino et al., 2012, 2013; Converse, 2013) or dominance status (Baker et al., 1998; Kokko and Johnstone, 1999), or where individuals achieve greater fitness by being in larger groups (e.g., African wild dogs: Carbone et al., 1999), thus making alloparental care beneficial even when helpers are unrelated to the young (“group augmentation”; Kokko et al., 2001).
Ecological Correlates of Extra-Pair Mating
Ecological conditions can affect the balance of these costs and benefits of extra-pair mating and thus its prevalence (summarized in Table 1).
Resource Availability and Reliance on Paternal Care
When resources are abundant, offspring survival may be less dependent on male care and thus the potential costs of EPCs may be outweighed by potential benefits (Norén et al., 2012). Though desirable, there are cases where paternal care is not essential and females can at least sometimes raise litters without male assistance (e.g., bat-eared fox: Maas, 1993; coyote: Sacks and Neale, 2001; swift fox: Kamler et al., 2004b; cape fox: Kamler and Macdonald, 2014). This leads to the prediction that in socially monogamous species with biparental care, EPCs should increase with increased resource availability; a prediction supported for birds (Møller, 2000; Griffith et al., 2002). A comparative analysis of 15 mammal species (including three canids) found that rates of EPP correlated with levels of paternal care (Huck et al., 2014; see also Dillard and Westneat, 2016). Similarly, EPCs may be more common in mammals than in birds because paternal care is more common in birds (Isvaran and Clutton-Brock, 2006).
Within canids, among the lowest reported values of EPP thus far found is in bat-eared foxes (9.8–15.6%, Wright et al., 2010), a species wherein male care can be highly beneficial for offspring survival (Wright, 2006). The frequency of EPP in arctic foxes studied by Cameron et al. (2011) was argued to reflect variations in the need for paternal care: EPP correlated with spatial variation in food availability, being more frequent when closer to a goose colony. However, this study did not control for effects of population density, which often correlates with resource availability (e.g., Clark, 1972; White and Garrott, 1997) and thus could have been responsible for greater EPP closer to the goose colony. However, this hypothesis was not supported in a study of urban coyotes, wherein despite optimal food availability, pairs were strictly genetically monogamous (96 offspring from 18 litters, Hennessy et al., 2012).
Diet (Foraging Strategy and Time Budget)
Mate guarding is a common strategy to prevent EPCs, though the feasibility is affected by mate proximity during foraging and/or foraging time budgets. Solitary foragers (e.g., Island fox: Roemer, 1999; red fox: Baker et al., 2004; swift fox: Kitchen et al., 2006; Iossa et al., 2008a; Arctic fox: Cameron et al., 2011) may have greater opportunity to engage in clandestine EPCs, allowing relatively high levels of EPP (Island foxes: 25%, Roemer, 1999; red fox: 38–80%, Baker et al., 2004; Iossa et al., 2008b; swift fox: 52%, Kitchen et al., 2006; Arctic foxes: 31%, Cameron et al., 2011). These typically solitary foragers apparently try to reduce EPCs by spending more time closer to their partners during the breeding season (Kitchen et al., 2005a). In contrast, the relatively low levels of EPP in bat-eared foxes (9.8–15.6%, Wright et al., 2010) may reflect their insectivorous diet which enables partners to forage together (Wright, 2003). Additionally, insectivorous species with high foraging requirements may not have time to search for extra-pair mates: bat-eared foxes spend 80% of the night feeding (Wright et al., 2010), leaving little free time to search for extra-pair mates.
Population Density
Population density and female dispersion can be key factors affecting levels of EPC (Iossa et al., 2008a). High population density decreases the potential risks of embarking on extra-territorial forays to search for mating opportunities by decreasing distances between individuals, increasing encounter rates between males and females seeking EPCs and allowing males to assess the reproductive conditions of neighboring females (Gorman and Trowbridge, 1989). High population densities are associated with high levels of EPP in red foxes (up to 80% of cubs sired by extra-pair males at a density of 19.6–27.6 adults/km2; Baker et al., 2004) and Island foxes (25% of 16 offspring sired by extra-group males with population density of 2.4–15.9 foxes/km2; Roemer et al., 2001).
Particularly compelling evidence of the effect of population density on extra-pair mating comes from a population of red fox that experienced severe population declines while food availability remained constant. In a shift from high density (19.6–27.6 adults/km2) to low (4.0–5.5 adults/km2) from mange outbreak, EPP rates decreased from 80% (30 offspring) to 38% (38 offspring) and multiple paternity rates of litters decreased from 38–69% (16 litters) to 0% (10 litters, Baker et al., 2004; Iossa et al., 2008a). Fox body mass did not differ between the high and low density periods, indicating the population was not resource-limited at high densities (Soulsbury et al., 2008), suggesting food availability was not responsible for these differences.
However, population density did not seem to affect EPP in swift foxes studied by Kitchen et al. (2006) and urban coyotes living at high density with high resource availability were entirely genetically monogamous (Hennessy et al., 2012).
Social Structure
The number of potential breeders in a group influences the probability of extra-pair mating in canids (Spiering et al., 2010) and in mammals more generally (Clutton-Brock and Isvaran, 2006; Isvaran and Clutton-Brock, 2006; Lambert et al., 2018). Thus, the factors affecting group formation (described in section Ecological Correlates of Social Monogamy: Social Structure) can influence EPC.
The Unusual Canid Reproductive System: Anomalous Canid Reproductive Traits and Their Potential Relationship With Monogamy
Canids exhibit a suite of reproductive and physiological features that are unusual or even unique among mammals (Asa and Valdespino, 1998). The ultimate cause of these unusual traits could relate to the monogamous social system of canids by facilitating alloparental care and enforcing monogamy.
Facilitation of Alloparental Care
Long-term monogamous mating results in high levels of kinship between group members, an important factor in the evolution of mammalian alloparental care and cooperative breeding (Lukas and Clutton-Brock, 2012). Phylogenetic research shows that mammalian cooperative breeding evolved from social monogamy (Lukas and Clutton-Brock, 2012; Dillard and Westneat, 2016). Canids have developed several physiological characteristics that facilitates caring of offspring by individuals other than the mother, including the ability to provide food through regurgitation, obligate pseudopregnancy with potential spontaneous lactation, and seasonal prolactin surges. The conflict between whether to help raise siblings or breed is minimized since older offspring of a monogamous pair are equally related to their full-siblings as they would be to their own offspring.
Regurgitation
An innovation in canids is the ability to directly feed both pups and mother by regurgitation of partially digested food. This ability is found in all wolf-like canids (Canis, Cuon, and Lycaon genera, Johnsingh, 1982; Lord et al., 2013) as well as maned wolves (Rasmussen and Tilson, 1984) and bush dogs (Biben, 1982). Regurgitation is generally absent from vulpine canids, although it was recently reported in the swift fox (Poessel and Gese, 2013). Regurgitation may be seen as an evolutionary adaptation facilitating paternal care, alloparental care and cooperative breeding, which would be advantageous in a closely-related monogamous social system.
Hormonal Priming of Alloparental Care
Canid ovulation that does not result in pregnancy is followed by a remarkably long dioestrous phase of nearly the same duration as pregnancy (2 months, Asa and Valdespino, 1998), during which time progesterone and prolactin are elevated, similar to pregnancy. This is therefore called pseudopregnancy. Spontaneous ovulation followed by obligate pseudopregnancy with hormonally-primed allomaternal care and the potential for additional lactating females has clear benefits for helping the pack and caring for the dominants' offspring.
All canid species whose reproductive physiology have thus far been studied exhibit obligate pseudopregnancy, including gray wolves, coyotes, arctic foxes, red foxes, Ethiopian wolves and culpeos (Lycalopex culpaeus, Asa, 1997; Asa and Valdespino, 1998; van Kesteren et al., 2013). The endocrine similarity of obligate pseudopregnancy to true pregnancy hormonally primes all females that have ovulated for maternal behavior, regardless of whether they conceived, thereby encouraging allomaternal care by non-breeding subordinate females. The hormonal similarity of pseudopregnancy and pregnancy can even cause spontaneous lactation, providing the possibility for females aside from the mother to nurse pups (Jöchle, 1997; Asa and Valdespino, 1998; van Kesteren et al., 2013). Allonursing has been reported in all Canis species except golden jackals (Lord et al., 2013) and can increase pup survival (Sillero-Zubiri et al., 2004). The potential for allo-suckling may be an adaptive function of pseudopregnancy (Macdonald, 1992; Jöchle, 1997). In gray wolves, all pack members experience seasonal peaks in prolactin coinciding with pup birth. All adult wolves, even gonadectomized individuals, experience this prolactin peak, which is identical for males and females (Kreeger et al., 1991). Prolactin is associated with parental care in other species (reviewed by Angelier and Chastel, 2009) and thus this is likely related to the parental care exhibited by all pack members, including males (Kreeger et al., 1991; Jöchle, 1997).
Monogamy Enforcement
Post-copulatory Lock
A post-copulatory tie has been reported for all canid species where copulation was observed, though of varying duration (Asa and Valdespino, 1998). In African wild dogs, the lock can be very brief (Asa and Valdespino, 1998), while in fennec foxes, locks can last as long as 2.75 h (average 1.8 h, Valdespino et al., 2002). The function may be to increase the probability of fertilization and enhance sperm transport. It has also been suggested to be an anatomical adaptation to encourage monogamy as a form of post-copulatory mate-guarding, since no other males can access the female during the period of sperm transport (Gomendio, 1998).
Monoestrum
The only carnivores to exhibit monoestrum, the restriction of seasonal reproduction to a single ovulatory cycle (Asa and Valdespino, 1998), are canids and their close relatives, ursids (Hayssen et al., 1993; Agnarsson et al., 2010). Polyoestrum, which is typical of most other mammals, is characterized by successive cycles of oestrus and ovulation without an intervening period of anoestrus (reproductive quiescence), which can be seasonal or year-round. Thus, if a female fails to conceive at one ovulation she has additional opportunities. Seasonal monoestrum limits females to a single conception opportunity per year—potentially a very risky reproductive strategy.
In ursids, the risk of monoestrous leading to missed mating opportunities is reduced by induced ovulation, meaning females only ovulate in the presence of appropriate stimuli (e.g., a male or copulation), similar to many other Carnivora species (e.g., felids, mustelids, Hayssen et al., 1993). Canids, however, not only have a single ovulatory cycle per season, but also exhibit spontaneous ovulation (Conaway, 1971; Asa and Valdespino, 1998), seemingly a derived trait as there is evidence of induced oestrus and ovulation in the most basal canid genus, Urocyon (Lindblad-Toh et al., 2005): Island foxes (U. littoralis) ovulate only in the presence of males (Asa et al., 2007) (whether there is induced oestrus and ovulation in the other member of the Urocyon genus [e.g., gray fox, U. cinereoargenteus] is unknown). Canids are thus unique in that they exhibit both spontaneous ovulation and monoestrum. This combination may increase the value of long-term pair bonding. Although the risks of monoestrous are reduced by the long oestrous period in canids (lasting ~1 week, contrasting with the 1-day oestrous of many mammals, Asa and Valdespino, 1998), with only a single spontaneous oestrous cycle per year, there would be considerable risk if a female does not find a partner during the limited window of reproductive opportunity or if a female unknowingly paired with an infertile or genetically incompatible mate; long-term successful pairing may provide assurance against these possibilities.
Asa and Valdespino (1998) argue that the ultimate cause for monoestrum could be the canid social system, facilitating social monogamy and cooperative breeding through reproductive suppression. Monoestrum eliminates the opportunity for additional periods of oestrus in subordinates, which could cause social tension. They argue that if canids were polyoestrous, the dominant female would likely conceive on the first cycle but subordinates would continue cycling. However, due to canids' long oestrous period (Asa and Valdespino, 1998), the duration of time in oestrus may be equivalent between monoestrum and polyoestrum, resulting in the same amount of effort needed for reproductive suppression, regardless if over several cycles or one.
The Exception Proves the Rule: Social and Mating System of the Domestic Dog
The domestic dog presents an interesting case because its social and mating system differs from all other members of the Canis genus. Domestic dogs derived from the gray wolf an estimated 11,000–40,000 years ago (see reviews by Driscoll et al., 2009; Driscoll and Macdonald, 2010; Wang et al., 2013; Frantz et al., 2016; Botigué et al., 2017). Despite their close evolutionary history, the general Canis pattern of social monogamy, pair bonding, extended paternal and alloparental care and monoestrous seasonal reproduction is conspicuously absent from dogs (Lord et al., 2013).
Free-living dogs generally exhibit a promiscuous mating system with no breeding hierarchy (Lord et al., 2013), though they can exhibit a range of mating systems (Pal, 2011). All adults can have the opportunity to breed and thus dog social groups can contain multiple lactating females with litters, in addition to other male and female group members (Macdonald and Carr, 1995; Pal, 2011; Paul et al., 2014). Though free-living dogs often live in groups, they do not always form a structured pack (Macdonald and Carr, 1995; Kamler et al., 2003a; Majumder et al., 2014). A social group may defend a territory together, but groups can be dynamic in composition, influenced by mating interests, resource availability, and closeness to source populations (Macdonald and Carr, 1995; Kamler et al., 2003a; Majumder et al., 2014). Some groups hunt cooperatively (Kamler et al., 2003b; Fleming et al., 2006) while others seemingly do not (Macdonald and Carr, 1995). Care is predominantly provided by the mother (Macdonald and Carr, 1995; Lord et al., 2013), though there are some observations of paternal (Pal, 2005) and alloparental (Paul et al., 2014) care. Although regurgitation and provisioning of offspring by males and helpers is characteristic of all other Canis species, it is rare in domestic dogs and mainly exhibited by the mother (Malm, 1995; though see Pal, 2005; Lord et al., 2013). Dogs reach independence much earlier than other Canis species, at 10–13 weeks, compared to an approximate average age of 6 months for other members of the genus (Pal, 2005; Lord et al., 2013). Dog pups do not receive extended parental care. After weaning, dog pups are independent of parenting and no longer directly fed; they instead must find their own food and compete with adults and juveniles. Wild Canis all exhibit reproductive seasonality, including dingoes, with births coinciding with seasonal increases in food availability; dogs are the only exception, with females coming into oestrous approximately every 7 months and males always being capable of reproducing, though there can be concentrations of breeding during certain times of the year (Lord et al., 2013). Thus, dogs exhibit approximately two oestrus per year, unlike all other monoestrous Canis (Asa and Valdespino, 1998; Lord et al., 2013). This vastly different social and mating behavior of domestic dogs compared to wild Canis is associated with different ecological conditions, providing an opportunity for understanding the ecological conditions that shape monogamy in other canids.
Are These Differences Adaptive?
Although Macdonald and Carr (1995) cautioned against interpreting the behavior of a domesticated species as adaptive, Lord et al. (2013) argue that humans have had little reproductive control over the vast majority of dogs because most are free-ranging. They argue that the differences in the reproductive systems and behavior of dogs compared to other Canis are adaptations to a new ecological niche created by the permanent and stationary settlement of humans and the associated food resource availability, rather than by artificial selection or reduced natural selection. The proposition that their behavior is adaptive is supported by findings that two different dog communities behaved very differently when exposed to contrasting ecological circumstances (Macdonald and Carr, 1995).
Dogs tend to cluster in areas of human waste and the diet of most free-ranging dogs originates from humans, either directly through provisioning or indirectly from scavenging (Kamler et al., 2003a; Vanak and Gompper, 2009). Dogs are therefore released from seasonal fluctuations in resource availability, avoid high costs of having to hunt and generally experience high resource abundance (Macdonald and Carr, 1995; Kamler et al., 2003a; Pal, 2008; Lord et al., 2013). The ease of finding and processing food decreases the necessity for energetically-expensive parental care behaviors, making male care less valuable for domestic dogs than for wild canids. The reliable, year-round availability of human-derived food likely favored the loss of reproductive seasonality (Lord et al., 2013). Freed from seasonality in resource availability, dogs can breed continuously throughout the year, avoiding competition with other dog litters even within the same social group. This also allows early age at first reproduction as dogs can breed as soon as they come into maturity, rather than waiting for the next breeding season (Lord et al., 2013). By avoiding energetically-costly parenting behavior, dog parents can redirect energy into breeding year-round and multiple times per year, thereby increasing fecundity (Lord et al., 2013).
Although for wild canids the optimal strategy for maximizing reproductive success is often monogamy with biparental care, for domestic dogs paternal and alloparental care is not necessary due to more stable resource availabilities and thus they benefit from adopting an entirely different strategy. The genus-atypical reproductive and parental behavior of domestic dogs supports the hypothesis that monogamy in canids is largely an adaptation allowing wild canids to make the most of fluctuations in resource availability (Lord et al., 2013).
Canid Success: Cause, Corollary or Consequence of Monogamy, the Pro-cooperative Hypothesis
Canid Success
This essay was prompted by the question of whether monogamy is a cause, consequence, or correlate of Canidae success, as individuals, species and family. Unlike many other carnivore families, canids have thrived in the rapidly changing conditions of the Anthropocene (Wang et al., 2007; Wang and Tedford, 2008). The world's most widely distributed wild terrestrial mammal is a canid: the red fox, found from the Arctic Circle to North Africa, North America and Eurasia and introduced and now widespread in Australia and USA (Macdonald and Sillero-Zubiri, 2004). Prior to the ascent of red foxes, this title was held by another canid, the gray wolf, originally distributed throughout the Northern Hemisphere in every habitat large ungulates were found (Mech, 1995; Macdonald and Sillero-Zubiri, 2004), until widespread human persecution caused their near-complete extirpation by the late 1800s (Mech, 1995; Phillips et al., 2004). But canids are resilient and gray wolves are now returning to their former range in both North America and Europe (Mech, 1995, 2017; Breitenmoser, 1998; Wydeven et al., 1998; Phillips et al., 2004). Coyotes have dramatically increased their range over the past two centuries. Previously found only in the prairies and deserts of western North America, they are now ubiquitous in every country and state from Alaska to Panama, found in nearly all available habitats, including forest, prairie, desert, mountain, tropical habitats, and cities (Gompper, 2002; Macdonald and Sillero-Zubiri, 2004). Coyote range expansion was likely catalyzed by the extermination of gray wolves, thus reducing intra-guild competition (Macdonald and Sillero-Zubiri, 2004), and land conversion through logging and agriculture which opened up additional habitat (Méndez–Carvajal and Moreno, 2014). The rapid expansion of coyotes in North and Central America is mirrored by that of golden jackals in Europe. Native to the Middle East and southern Asia, golden jackals arrived at the southern edge of Central and Eastern Europe around 8,000 years ago and began slowly expanding in the nineteenth century, but since the 1950's their expansion has accelerated into the north and west of Europe (Tóth et al., 2009; Rutkowski et al., 2015). They are now found as far north as Finland, four degrees below the Arctic Circle (Banea and Giannatos, 2019), and as far west as Switzerland (Arnold et al., 2012; Trouwborst et al., 2015) and their continued expansion, for reasons paralleling those for coyotes, seems likely (Arnold et al., 2012). In addition to these natural rapid expansions, introductions of canids by humans allowed several to thrive as invasive species. Raccoon dogs, originally from Siberia, East Asia and Japan, were introduced as a furbearing species in the Soviet Union from 1928 to 1955 and within 50 years had colonized 1.4 million km2 of northern and eastern Europe (Helle and Kauhala, 1991; Kauhala and Saeki, 2004; Sutor, 2007; Kauhala and Kowalczyk, 2011). The reintroduction and subsequent rapid spread of red foxes in mainland Australia and USA is another classic example of biological invasion (Kamler and Ballard, 2002; Fleming et al., 2006). The domestic dog, a few genes adrift from gray wolves, is one of the world's most successful mammal: population estimates range from 700 million (Hughes and Macdonald, 2013) to over one billion (Lord et al., 2013), roughly 80% of which are estimated to be free-ranging (Lord et al., 2013). Despite considerable efforts, humans have been unable to control the populations of these canids.
What has allowed these canids—red fox, gray wolf, coyote, golden jackal, raccoon dog, and domestic dog—to be so successful as to rapidly colonize new areas, dramatically increase in abundance, and continue to do so despite human efforts to control populations, and could their monogamous lifestyle be partially to blame? We suggest that canid success may be attributed to four main characteristics: (1) their generalist nature, adaptability, flexibility, and intelligence, allowing them to adapt to diverse habitats, diets and circumstances; (2) their high mobility and capacity for long-distance travel, facilitating fast colonization, expansive gene flow and genetic diversity, creating a selective advantage in changing environments and minimizing the risk of inbreeding from founder effects (Reed and Frankham, 2003); (3) their high reproductive rate, allowing them to quickly increase in number and recover following population declines from disease and persecution; and (4) their sociality and ability to cooperate, which can provide numerous benefits (see section Ecological Correlates of Social Monogamy: Social Structure; Macdonald and Carr, 1989; Macdonald et al., 2004). We argue that while the first and second attributes on this list are conserved traits that arose early in canids' phylogenetic history, the third and fourth are consequences of monogamy, and that it is the combination of these four characteristics that contribute to canids' success.
Canid Success Traits That Are Consequences of Monogamy
High Reproductive Rates
Co-evolution of paternal care and litter size resulted in the large litters that are characteristic of canids, thus increasing reproductive output (Stockley and Hobson, 2016). Canids can also reproduce in their first year and breed annually (Lord et al., 2013). In comparison to other omnivorous Carnivores, canids are distinguished by the platform provided by monogamy for benefiting from paternal care, cooperative breeding and allopaternal care, all of which can enhance lifetime reproductive success (Lukas and Clutton-Brock, 2013; Opie et al., 2013). Rapid reproduction allows canids to withstand high mortality rates (from human persecution and disease, itself often anthropogenic; Goltsman et al., 1996; Laurenson et al., 1998; Rhodes et al., 1998) and quickly colonize new areas. Despite widespread persecution, red foxes, coyotes, golden and black-backed jackals and raccoon dogs are able to thrive, while wolves continue to recover in North America and Europe despite ongoing illegal killings (Mech, 1995, 2017).
High reproductive rates may also allow for rapid phenotypic and genotypic adaptations to cope with new or changing environments and prevents inbreeding depression (Reed and Frankham, 2003). Furthermore, socially monogamous mating systems are predicted to produce greater reproductive output and genetic diversity compared to polygynous or polyandrous systems, leading to larger effective population sizes (Parker and Waite, 1997; Waite and Parker, 1997).
Sociality and Cooperation
The complex, cooperative social systems of canids that evolved from monogamy (Lukas and Clutton-Brock, 2013; Dillard and Westneat, 2016) provides numerous benefits discussed in section Canid Social Monogamy (e.g., cooperative hunting, food defense, reproductive success). Furthermore, the cooperation that first developed between members of the monogamous pair can spill over to other individuals, generally kin, when resource dispersion facilitates cohabitation by a spatial group (see Macdonald and Johnson, 2015). While this might originally be focused on young, it is a small step to cooperating with, and even assisting, other adult group members (e.g., adult red foxes caught in traps may be fed by other foxes [Garcelon et al., 1999]).
Canids have among the largest relative brain sizes in Carnivora (Gittleman, 1986; Swanson et al., 2012) and an enlarged pre-frontal cortex compared to felids and other carnivores (Rakinsky, 1969), associated with increased intelligence and behavioral complexity and flexibility. Across carnivore species, experiments show that greater relative brain size is associated with greater problem solving (Benson-Amram et al., 2016) and across mammals, larger brain sizes are associated with the ability to successfully adapt to, colonize, and invade novel habitats (Sol et al., 2008). The sociality and cooperation that evolved in canids from monogamy may have increased canid brain size through influence on diet. Cooperative hunting allows canids to tackle larger vertebrate prey and increases hunting success (Fanshawe and Fitzgibbon, 1993; Creel and Creel, 1995). Carnivore species that consume vertebrates have the largest brains, omnivores intermediate, and insectivores the smallest (Swanson et al., 2012). This could be because hunting vertebrate prey is more cognitively demanding than omnivory or insectivory, and particularly when synchronizing hunting behavior with pack mates, or because consuming higher-energy foods allows evolution of metabolically expensive brain tissue (Swanson et al., 2012).
Increased brain size is also argued to be a consequence of the complex social relationships that monogamy requires. Shultz and Dunbar (2007) found that larger relative brain size is correlated with socially monogamous pair bonding in Carnivora, other mammalian orders, and birds. Furthermore, bird species with long-term monogamous pair bonds (like that of most canids) have larger brains than species with short-term seasonal monogamy (Shultz and Dunbar, 2007; West, 2014). Shultz and Dunbar (2010) concluded that increased brain size evolved in birds as a result of long-term pair bonding, not that larger brains allowed long-term pair bonding. Three hypotheses have been proposed for why monogamous pair bonding may select for larger brains. Shultz and Dunbar (2007) and Dunbar (2009) argue that the cognitive demands of behavioral coordination, synchronization, and negotiation necessary for navigating and maintaining stable pair bonded partnerships is responsible for increased brain sizes seen with monogamy. Alternatively, the potentially high costs of selecting an unreliable or infertile mate, particularly in species forming long-term monogamous pair bonds where there is reduced availability of alternative mates, may have selected for cognitively-demanding mate selection processes (Dunbar, 2009). A third hypothesis relates to the pressures of mate guarding and procuring fitness-increasing extra-pair copulations while maintaining social partnerships (West, 2014). This is supported in birds, where larger brains not only correlate with social monogamy but also with extra-pair paternity: as rates of extra-pair paternity increase, so does brain size. This suggests there is an intersexual co-evolutionary arms race with both sexes trying to outsmart each other in trying to sneak extra-pair copulations while preventing their mate from doing the same, leading to larger brains in both sexes (West, 2014).
Sociality beyond the pair bond may also contribute to increased brain sizes. Swanson et al. (2012) found that carnivoran social complexity is positively correlated with relative cerebrum volume (but not total brain volume), in line with the social brain hypothesis in primates which posits that larger brains evolved due to the cognitive demands posed by complex social systems (Dunbar, 1992; Shultz and Dunbar, 2007; but see DeCasien et al., 2017) (studies on the relationship between total relative brain volume and carnivore sociality have found conflicting results, see Gittleman, 1986; Dunbar and Bever, 1998; Pérez-Barbería et al., 2007; Shultz and Dunbar, 2007; Finarelli and Flynn, 2009; Swanson et al., 2012).
Thus, the sociality and cooperation that evolved in canids as a result of monogamy also likely made them more intelligent and adaptable compared to other carnivore families, which would have further facilitated the relative dominance of canids and allowed several canid species to prosper even during the Anthropocene.
In this sense, monogamy primed pro-cooperative, pro-social behaviors in ancestral canids that were, and largely remain, facultative rather than obligate. This facility for cooperation offers canids a selective advantage to maximize opportunities more readily than would have been the case without the pro-cooperative bonus brought by monogamy.
Conserved Traits for Success Amongst the Canidae
Intra-Specific Ecological Flexibility
Canids are highly flexible and adaptable in their ecology, able to exploit a wide range of diets, habitats, and social structures. Although the central theme of canid social and mating systems is monogamy, their extreme flexibility leads to intraspecific variation in social behavior as adaptations to varying ecological conditions, allowing them to take advantage of superior strategies when opportunity permits. Consider the finding of Robertson (2016) that in areas with low resource availability, female coyotes delay reproduction and instead bide at home as helpers, more than half breeding only after their third year. In areas with high resource availability, almost half bred as yearlings, and almost all did so by their third birthday. This flexibility allows rapid increases in numbers, contributing to resilience to intense hunting pressure (Berger, 2006). Interspecifically, consider the domestic dog, which abandoned the Canis pattern of monogamy and its associated social and reproductive traits to take advantage of the abundant resources in its niche, allowing domestic dogs to become the most abundant carnivore on earth (Hughes and Macdonald, 2013; Lord et al., 2013). The remarkable intra-specific flexibility of canids means that while monogamy, and the cooperation it facilitates, is their norm, they can survive and even sometimes thrive alone when needed (much like omnivorous viverrids and musteloids) or adopt polygamy when ecological conditions present that as a superior strategy. They can be flexible as necessity or circumstance requires or permits.
High Mobility
Canids are capable of fast and wide-ranging movements. Movements of 86 km in a little over a month and 230 km in ~3 months have been recorded in the African golden wolf (Karssene et al., 2018), gray wolves disperse as far as 1,000 km in search of new territories and mates (Mech et al., 1995; Ciucci et al., 2009) and minimum dispersal distances documented for coyotes are 94 km for females and 113 km for males (Harrison, 1992). The interplay of this high mobility with their ecological flexibility allows canids to quickly move to new areas and adjust to local conditions.
Formula for Canid Success: the Monogamy as Pro-cooperative Hypothesis
The four attributes presented above may be the keys to the formula of canid success among carnivores. This hypothesis might be termed the monogamy as pro-cooperative hypothesis (Figure 1). In short, monogamy appears to have arisen when females are dispersed between sharable territories that arise as a result of the dispersion of available resources (Macdonald and Johnson, 2015), preventing males from defending multiple females but nonetheless allowing them to cohabit with one, such that guarding a single female is the most efficient male strategy (Komers and Brotherton, 1997; Brotherton and Komers, 2003; Lukas and Clutton-Brock, 2013), emerging from the ancestral canids' ecological circumstances. Monogamy together with biparental and alloparental care allowed for high reproductive output and cooperative sociality and intelligence. This perspective is congruent with Lukas and Clutton-Brock (2013) conclusion, based on phylogenetic analysis, that biparental care evolved from social monogamy, and cooperative breeding systems evolved from that. Their high mobility allows canids to quickly expand to new areas, their intelligence and generalist nature allows them to adapt to new diets and habitats in these new areas, and together with their high reproductive output and cooperative sociality allows them to quickly increase in numbers and successfully colonize.
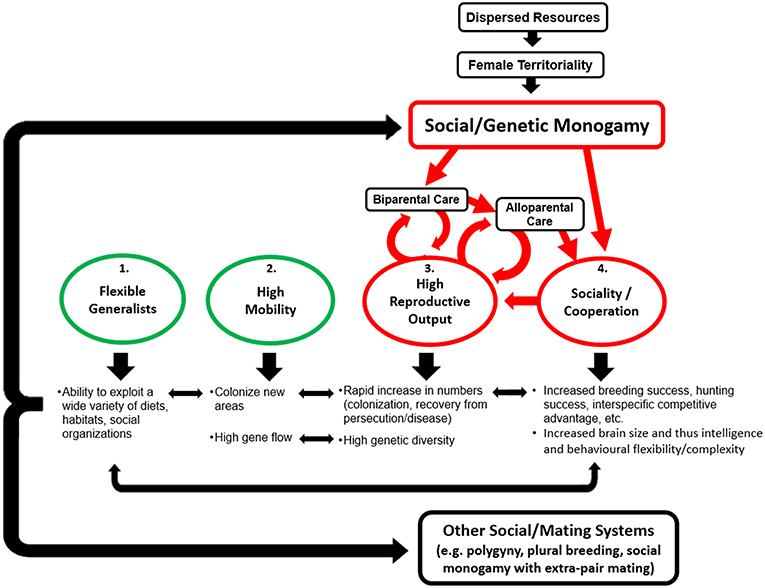
Figure 1. The monogamy as pro-cooperative hypothesis: Canid success may be attributed to four characteristics: (1) their flexible, generalist, adaptable nature, (2) high mobility, (3) high reproductive rates, (4) sociality and cooperation. Traits 1 and 2 appear to be early traits of canids' phylogenetic history, while 3 and 4 arose from monogamy. Dispersed resources, insufficient to support multiple breeding females but sufficient for a female and male within a territory, seem to have led to the evolution of social monogamy, which in turn led to the evolution of biparental care and, thus, where ecological circumstances allow, alloparental care and sociality/cooperation (trait 4) and high reproductive rates (trait 3). These create feedback loops, where biparental/alloparental care, sociality and cooperation lead to higher reproductive output, which, in turn require biparental/alloparental care, sociality and cooperation. These four canid traits are interconnected and enhance one another. For example, high mobility allows canids to move to new areas, their generalist flexible nature allows them to adapt to these new areas, their ability to quickly increase in number through high reproductive rates allows them to establish in these new areas, with survival and reproduction further enhanced by their sociality and cooperation. The interplay of rapid reproduction and high mobility, allowing gene flow and enhancing genetic diversity, contributes to their adaptability and flexibility, and sociality further contributes to their flexibility through its association with enhanced intelligence, in line with the social brain hypothesis. This flexibility means that canids can adapt social and mating systems to suit local ecological conditions, which may result in social monogamy, or, if ecological conditions are such that superior strategies exist, canids can exploit alternative social/mating strategies yet retain the benefits of high reproductive rates and sociality/cooperation that monogamy afforded (see section Ecological Correlates of Social Monogamy for ecological conditions affecting maintenance of social monogamy vs. alternative strategies, such as polygyny, plural breeding, etc., and section Ecological Correlates of Extra-Pair Mating for ecological conditions affecting genetic monogamy vs. extra-pair mating). It is thus the combination of these four traits, with monogamy at its foundation, that together make up the formula of canid success, explaining the rapid expansion, colonization, and invasion of multiple canid species in recent years.
Comparison Within Canidae
To consider further the role these four traits play in canid success, we explore how some of the most successful wild canids, as outlined above—red fox, golden jackal, coyote, raccoon dog—differ from those species that are not faring so well. Of the 37 extant Canidae species, one is listed by the IUCN as Critically Endangered (red wolf) and four as Endangered (Ethiopian wolf, African wild dog, dhole and Darwin's fox); no species are listed as Vulnerable and only one canid has gone extinct in historical times (Falkland islands wolf, Dusicyon australis, in 1876). How do these species differ? For the Falkland islands wolf and Darwin's fox, their small and isolated ranges, was and is their main downfall. For the other species mentioned here, they lack a critical piece of the puzzle for canid success—their generalist, adaptable nature. African wild dogs and dholes are hypercarnivorous, with specialized dentition for a diet primarily of large vertebrate prey. Their large body size coincides with high energy requirements (Carbone et al., 1999; Slater, 2015), meaning they must consume large prey for foraging to be energetically economical. This results in a greater reliance on cooperative hunting, such that, unlike other canids that can adjust social structure and thrive alone, as pairs, or in groups, these species may have a minimum pack size threshold for successful hunting and breeding (Carbone et al., 1999). The African wild dog and dhole are also among the least monogamous canids—dholes tend to exhibit communal breeding, living in large clans and more often with multiple breeding females in a group (Fox, 1984), while African wild dogs are often polyandrous or polygynandrous with multiple litters in a pack (see section Ecological Correlates of Extra-Pair Mating). African wild dogs deviate from other canid patterns—they exhibit the shortest recorded post-copulatory lock (Frame et al., 1979; Asa and Valdespino, 1998), which we suggested could be a monogamy enforcement adaptation (section Post-copulatory Lock). The Ethiopian wolf has also increased dietary specialization, but in the opposite direction—rather than specializing on large prey, this species specializes on Afroalpine rodents, which can comprise as much as 97% of prey volume of their diet (Sillero-Zubiri and Gottelli, 1995b). Afroalpine rodent communities can therefore limit the distribution of Ethiopian wolves (Sillero-Zubiri et al., 1995a,b).
Comparison Among Carnivora
To isolate the importance of monogamy within the canid syndrome of breeding fast and cooperating as opportunity allows requires solving the algebra of alternative evolutionary pathways that led other generalist, omnivorous carnivores to life histories that do not involve monogamy. How do they fare?
Consider the feliform solution to the same evolutionary problem, the Viverridae (genets and civets). Although viverrid biology is not well-known (e.g., Ross et al., 2017) they too excel at omnivory, but exhibit classic carnivorean polygyny, produce small litters, and lack the pioneering adaptability and cooperative tendencies of canids. The same might be said of most omnivorous musteloids and ursids, themselves carrying much of the caniform phylogenetic baggage shared by canids (Koepfli et al., 2017), but with societies conspicuously lacking monogamy (Macdonald and Newman, 2017). The comparison is not flawless, but sufficiently compelling that the role of monogamy in the syndrome of canid attributes is part of their particular success. Perhaps the extinction of the tenth family of caniforme Carnivores, the Amphicyonidae (the “bear-dogs”), 2.6 million years ago, probably due to competition with true dogs, lay in the trump card of monogamy. The point might even be stretched to explain how, five million years ago, canids ousted the dog-like Hyeanidae that once outnumbered them (Macdonald, 1992). Amongst the Caniforme suborder of Carnivora, despite their close phylogenetic relationships, Canidae are the only family to exhibit monogamy. Of the 37 extant canid species, only 5—13.5%—are listed as threatened (Critically Endangered, Endangered, or Vulnerable) on the IUCN Red List. This is the lowest proportion among all Caniforme families. An avenue worth exploring may be associations between the conservation status of species in a family and the number of the critical traits identified here that they exhibit (amongst Caniforme families we think that none other than canids display all four).
Author Contributions
The review was developed by DM and LC with substantial input from GW. All authors (DM, LC, JK, JM, GW, and CS-Z) participated in refining the manuscript.
Funding
LC was supported by the Oxford Newton Abraham Fund and the International Fund for Animal Welfare and GW by an Oxford-Lady Margaret Hall-NaturalMotion Graduate Scholarship.
Conflict of Interest Statement
The authors declare that the research was conducted in the absence of any commercial or financial relationships that could be construed as a potential conflict of interest.
Acknowledgments
DM was grateful to Nancy Solomon for the invitation to write this review for Frontiers in Ecology and Evolution, and also grateful to the editorial staff for their professionalism and forbearance. He was also grateful to Christopher O'Kane for meticulous help throughout, and in addition to the current doctoral students and post-docs who have joined this exercise as co-authors, DM has reflected with great warmth on the total of 26 successful doctoral, masters, and diploma students who, over 40 years, he has supervised specifically on canids and whose wisdom and friendship is reflected in this review.
References
Agnarsson, I., Kuntner, M., and May–Collado, L. J. (2010). Dogs, cats, and kin: a molecular species–level phylogeny of Carnivora. Mol. Phylogenet. Evol. 54, 726–745. doi: 10.1016/j.ympev.2009.10.033
Alonzo, S. H. (2012). Sexual selection favours male parental care, when females can choose. Proc. R. Soc. Lond. B Biol. Sci. 279, 1784–1790. doi: 10.1098/rspb.2011.2237
Angelier, F., and Chastel, O. (2009). Stress, prolactin and parental investment in birds: a review. Gen. Comp. Endocrinol. 163, 142–148. doi: 10.1016/j.ygcen.2009.03.028
Angerbjörn, A., Arvidson, B., Norén, E., and Strömgren, L. (1991). The effect of winter food on reproduction in the arctic fox, Alopex lagopus: a field experiment. J. Anim. Ecol. 60, 705–714. doi: 10.2307/5307
Angerbjörn, A., Hersteinsson, P., and Tannerfeldt, M. (2004). “Arctic foxes,” in Canid Biology and Conservation, eds D. W. Macdonald and C. Sillero-Zubiri (Oxford: Oxford University Press), 163–172.
Angerbjörn, A., Tannerfeldt, M., Bjärvall, A., Ericson, M., From, J., and Norén, E. (1995). “Dynamics of the arctic fox population in Sweden,” in Annales Zoologici Fennici, Vol. 32, 55–68.
Angulo, E., Rasmussen, G. S. A., Macdonald, D. W., and Courchamp, F. (2013). Do social groups prevent Allee effect related extinctions?: the case of wild dogs. Front. Zool. 10:11. doi: 10.1186/1742-9994-10-11
Annavi, G., Newman, C., Dugdale, H. L., Buesching, C. D., Sin, Y. W., Burke, T., et al. (2014). Neighbouring-group composition and within-group relatedness drive extra-group paternity rate in the European badger (Meles meles). J. Evol. Biol. 27, 2191–2203. doi: 10.1111/jeb.12473
Arct, A., Drobniak, S. M., and Cichon, M. (2015). Genetic similarity between mates predicts extrapair paternity—a meta–analysis of bird studies. Behav. Ecol. 26, 959–968. doi: 10.1093/beheco/arv004
Arjo, W. M., and Pletscher, D. H. (1999). Behavioral responses of coyotes to wolf recolonization in northwestern Montana. Can. J. Zool. 77, 1919–1927. doi: 10.1139/z99-177
Arnold, J., Humer, A., Heltai, M., and Murariu, D. (2012). Current status and distribution of golden jackals Canis aureus in Europe. Mammal. Rev. 42, 1–11. doi: 10.1111/j.1365-2907.2011.00185.x
Asa, C. S. (1997). “Hormonal and experiential factors in the expression of social and parental behavior in canids,” in Cooperative Breeding in Mammals, eds N. G. Solomon and J. A. French (Cambridge: Cambridge University Press, 129–149.
Asa, C. S., Bauman, J. E., Coonan, T. J., and Gray, M. M. (2007). Evidence for induced estrus or ovulation in a canid, the island fox (Urocyon littoralis). J. Mammal. 88, 436–440. doi: 10.1644/05-MAMM-A-295R2.1
Asa, C. S., and Valdespino, C. (1998). Canid reproductive biology: an integration of proximate mechanisms and ultimate causes. Am. Zool. 38, 251–259. doi: 10.1093/icb/38.1.251
Baker, P. J., Funk, S. M., Bruford, M. W., and Harris, S. (2004). Polygynandry in a red fox population: implications for the evolution of group living in canids? Behav. Ecol. 15, 766–778. doi: 10.1093/beheco/arh077
Baker, P. J., Funk, S. M., Harris, S., and White, P. C. (2000). Flexible spatial organization of urban foxes, Vulpes vulpes, before and during an outbreak of sarcoptic mange. Anim. Behav. 59, 127–146. doi: 10.1006/anbe.1999.1285
Baker, P. J., and Harris, S. (2004). “Red foxes,” in The Biology and Conservation of Wild Canids, eds D. W. Macdonald and C. Sillero-Zubiri (Oxford: Oxford University Press), 207–216.
Baker, P. J., Robertson, C. P. J., Funk, S. M., and Harris, S. (1998). Potential fitness benefits of group living in the red fox, Vulpes vulpes. Anim. Behav. 56, 1411–1424. doi: 10.1006/anbe.1998.0950
Ballard, W. B., Whitman, J. S., and Gardner, C. L. (1987). Ecology of an exploited wolf population in south–central Alaska. Wildl Monogr 98, 3–54.
Banea, O. C., and Giannatos, G. (2019). Finland and “The Battle of the Jackal”. Reykjavik: GOJAGE News.
Bekoff, M., Diamond, J., and Mitton, J. B. (1981). Life–history patterns and sociality in canids: body size, reproduction, and behavior. Oecologia 50, 386–390. doi: 10.1007/BF00344981
Bekoff, M., and Wells, M. C. (1982). Behavioral ecology of coyotes: social organization, rearing patterns, space use, and resource defense. Z. Tierpsychol. 60, 281–305. doi: 10.1111/j.1439-0310.1982.tb01087.x
Benson-Amram, S., Dantzer, B., Stricker, G., Swanson, E. M., and Holekamp, K. E. (2016). Brain size predicts problem-solving ability in mammalian carnivores. Proc. Natl. Acad. Sci. U.S.A. 113, 2532–2537. doi: 10.1073/pnas.1505913113
Berger, K. M. (2006). Carnivore-livestock conflicts: Effects of subsidized predator control and economic correlates on the sheep industry. Conserv. Biol. 20, 751–761. doi: 10.1111/j.1523-1739.2006.00336.x
Biben, M. (1982). Ontogeny of social behaviour related to feeding in the crab-eating fox (Cerdocyon thous) and the Bush dog (Speothos venaticus). J. Zool. 196, 207–216. doi: 10.1111/j.1469-7998.1982.tb03501.x
Birkhead, T. R., and Møller, A. P. (1996). “Monogamy and sperm competition in birds,” in Partnerships in Birds: The Study of Monogamy, ed J. M. Black (Oxford: Oxford University Press), 323–343.
Botigué, L. R., Song, S., Scheu, A., Gopalan, S., Pendleton, A. L., Oetjens, M., et al. (2017). Ancient European dog genomes reveal continuity since the early Neolithic. Nat. Commun. 8:16082. doi: 10.1038/ncomms16082
Bowen, W. D. (1981). Variation in coyote social organization: the influence of prey size. Can. J. Zool. 59, 639–652. doi: 10.1139/z81-094
Breitenmoser, U. (1998). Large predators in the Alps: the fall and rise of man's competitors. Biol. Conserv. 83, 279–289. doi: 10.1016/S0006-3207(97)00084-0
Brotherton, P. N., and Komers, P. E. (2003). “Mate guarding and the evolution of social monogamy in mammals,” in Monogamy: Mating Strategies and Partnerships in Birds, Humans and Other Mammals, eds U. H. Reichard and C. Boesch (Cambridge: Cambridge University Press), 42–58.
Camenzind, F. J. (1978). Behavioral ecology of coyotes (Canis latrans) on the National Elk Refuge, Jackson, Wyoming (PhD dissertation), University of Wyoming, Laramie, WY, United States.
Cameron, C., Berteaux, D., and Dufresne, F. (2011). Spatial variation in food availability predicts extrapair paternity in the arctic fox. Behav. Ecol. 22, 1364–1373. doi: 10.1093/beheco/arr158
Campbell, L. A. D. (2019). Fostering of a wild, injured, juvenile by a neighbouring group: implications for rehabilitation and release of Barbary macaques confiscated from illegal trade. Primates 60, 339–345. doi: 10.1007/s10329-019-00729-w
Campbell, L. A. D., Tkaczynski, P. J., Lehmann, J., Mouna, M., and Majolo, B. (2018). Social thermoregulation as a potential mechanism linking sociality and fitness: barbary macaques with more social partners form larger huddles. Sci. Rep. 8:6074. doi: 10.1038/s41598-018-24373-4
Carbone, C., Frame, L., Frame, G., Malcolm, J., Fanshawe, J., FitzGibbon, C., et al. (2005). Feeding success of African wild dogs (Lycaon pictus) in the Serengeti: the effects of group size and kleptoparasitism. J. Zool. 266, 153–161. doi: 10.1017/S0952836905006710
Carbone, C., Mace, G. M., Roberts, S. C., and Macdonald, D. W. (1999). Energetic constraints on the diet of terrestrial carnivores. Nat. Lond. 402, 286–288. doi: 10.1038/46266
Carr, G. M., and Macdonald, D. W. (1986). The sociality of solitary foragers: a model based on resource dispersion. Anim. Behav. 34, 1540–1549. doi: 10.1016/S0003-3472(86)80223-8
Cassidy, K. A., Mech, L. D., MacNulty, D. R., Stahler, D. R., and Smith, D. W. (2017). Sexually dimorphic aggression indicates male gray wolves specialize in pack defense against conspecific groups. Behav Proc 136, 64–72. doi: 10.1016/j.beproc.2017.01.011
Cassidy, K. A., Smith, D. W., Mech, L. D., MacNulty, D. R., Stahler, D. R., and Metz, M. C. (2016). Territoriality and inter-pack aggression in gray wolves: shaping a social carnivore's life history. Yellowstone Sci. 24, 37–43.
Ciucci, P., Reggioni, W., Maiorano, L., and Boitani, L. (2009). Long-distance dispersal of a rescued wolf from the northern apennines to the Western Alps. J. Wildl. Manag. 73, 1300–1306. doi: 10.2193/2008-510
Clark, F. W. (1972). Influence of jackrabbit density on coyote population change. J. Wildl. Manag. 36, 343–356. doi: 10.2307/3799064
Clutton-Brock, T. H. (1989). Review lecture: mammalian mating systems. Proc. R. Soc. Lond. B Biol. Sci. 236, 339–372. doi: 10.1098/rspb.1989.0027
Clutton-Brock, T. H., and Isvaran, K. (2006). Paternity loss in contrasting mammalian societies. Biol. Lett. 2, 513–516. doi: 10.1098/rsbl.2006.0531
Conaway, C. H. (1971). Ecological adaptation and mammalian reproduction. Biol. Reprod. 4, 239–247. doi: 10.1093/biolreprod/4.3.239
Converse, K. E. (2013). Genetic mating system and territory inheritance in the Sacramento Valley red fox (MSc dissertation), California State University, Sacramento, CA, United States.
Corbett, L. K. (1988). Social dynamics of a captive dingo pack: population regulation by dominant female infanticide. Ethology 78, 177–198. doi: 10.1111/j.1439-0310.1988.tb00229.x
Courchamp, F., and Macdonald, D. W. (2001). Crucial importance of pack size in the African wild dog Lycaon pictus. Anim. Conserv. 4, 169–174.
Courchamp, F., Rasmussen, G. S., and Macdonald, D. W. (2002). Small pack size imposes a trade–off between hunting and pup–guarding in the painted hunting dog Lycaon pictus. Behav. Ecol. 13, 20–27. doi: 10.1093/beheco/13.1.20
Courtenay, O., Macdonald, D. W., Gillingham, S., Almeida, G., and Dias, R. (2006). First observations on South America's largely insectivorous canid: the hoary fox (Pseudalopex vetulus). J. Zool. 268, 45–54. doi: 10.1111/j.1469-7998.2005.00021.x
Creel, S., and Creel, N. M. (1995). Communal hunting and pack size in African wild dogs, Lycaon pictus. Anim. Behav. 50, 1325–1339. doi: 10.1016/0003-3472(95)80048-4
Creel, S., and Creel, N. M. (2002). The African Wild Dog: Behavior, Ecology, and Conservation. Princeton, NJ: Princeton University Press.
Creel, S., Creel, N. M., Mills, M. G., and Monfort, S. L. (1997). Rank and reproduction in cooperatively breeding African wild dogs: behavioral and endocrine correlates. Behav. Ecol. 8, 298–306. doi: 10.1093/beheco/8.3.298
Creel, S., Mills, M. G., and McNutt, J. W. (2004). “African wild dogs,” in The Biology and Conservation of Wild Canids, eds D. W. Macdonald and C. Sillero-Zubiri (Oxford: Oxford University Press), 337–352.
Creel, S. R., and Creel, N. M. (1991). Energetics, reproductive suppression and obligate communal breeding in carnivores. Behav. Ecol. Sociobiol. 28, 263–270. doi: 10.1007/BF00175099
Creel, S. R., and Macdonald, D. W. (1995). Sociality, group size, and reproductive suppression among carnivores. Adv. Study Behav. 24, 203–257. doi: 10.1016/S0065-3454(08)60395-2
Darimont, C. T., and Paquet, P. C. (2002). Gray wolves, Canis lupus, of British Columbia's Central and North Coast: distribution and conservation assessment. Can. Field Nat. 116, 416–422.
de Oliveira, T. G. (2009). Distribution, habitat utilization and conservation of the vulnerable bush dog Speothos venaticus in northern Brazil. Oryx 43, 247–253. doi: 10.1017/S0030605307002347
DeCasien, A. R., Williams, S. A., and Higham, J. P. (2017). Primate brain size is predicted by diet but not sociality. Nat. Ecol. Evol. 1:0112. doi: 10.1038/s41559-017-0112
Derix, R., van Hooff, J., de Vries, H., and van hooff, J. (1993). Male and female mating competition in wolves: female suppression vs. male intervention. Behaviour 127, 141–174. doi: 10.1163/156853993X00461
Deuel, N. R., Conner, L. M., Miller, K. V., Chamberlain, M. J., Cherry, M. J., and Tannenbaum, L. V. (2017). Gray fox home range, spatial overlap, mated pair interactions and extra–territorial forays in southwestern Georgia, USA. Wildl. Biol. 2017:wlb.00326. doi: 10.2981/wlb.00326
Dietz, J. M. (1984). Ecology and Social Organization of the Maned Wolf (Chrysocyon brachyurus). Washington, DC: Smithsonian Institution Press.
Dillard, J. R., and Westneat, D. F. (2016). Disentangling the correlated evolution of monogamy and cooperation. Trends Ecol. Evol. 31, 503–513. doi: 10.1016/j.tree.2016.03.009
Dobson, F. S., Way, B. M., and Baudoin, C. (2010). Spatial dynamics and the evolution of social monogamy in mammals. Behav. Ecol. 21, 747–752. doi: 10.1093/beheco/arq048
Driscoll, C. A., and Macdonald, D. W. (2010). Top dogs: wolf domestication and wealth. J. Biol. 9:10. doi: 10.1186/jbiol226
Driscoll, C. A., Macdonald, D. W., and O'Brien, S. J. (2009). From wild animals to domestic pets, an evolutionary view of domestication. Proc. Natl. Acad. Sci. U.S.A. 106, 9971–9978. doi: 10.1073/pnas.0901586106
Dunbar, R. I. (1992). Neocortex size as a constraint on group size in primates. J. Hum. Evol. 22, 469–493. doi: 10.1016/0047-2484(92)90081-J
Dunbar, R. I. (2009). The social brain hypothesis and its implications for social evolution. Ann. Hum. Biol. 36, 562–572. doi: 10.1080/03014460902960289
Dunbar, R. I., and Bever, J. (1998). Neocortex size predicts group size in carnivores and some insectivores. Ethology 104, 695–708. doi: 10.1111/j.1439-0310.1998.tb00103.x
Elmhagen, B., Hersteinsson, P., Norén, K., Unnsteinsdottir, E. R., and Angerbjörn, A. (2014). From breeding pairs to fox towns: the social organisation of arctic fox populations with stable and fluctuating availability of food. Polar Biol. 37, 111–122. doi: 10.1007/s00300-013-1416-3
Emlen, S. T., and Oring, L. W. (1977). Ecology, sexual selection, and the evolution of mating systems. Science 197, 215–223. doi: 10.1126/science.327542
Estes, R. D., and Goddard, J. (1967). Prey selection and hunting behavior of the African wild dog. J Wildl Manag 31, 52–70. doi: 10.2307/3798360
Fanshawe, J. H., and Fitzgibbon, C. D. (1993). Factors influencing the hunting success of an African wild dog pack. Anim. Behav. 45, 479–490. doi: 10.1006/anbe.1993.1059
Finarelli, J. A., and Flynn, J. J. (2009). Brain-size evolution and sociality in Carnivora. Proc. Natl. Acad. Sci. U.S.A. 106, 9345–9349. doi: 10.1073/pnas.0901780106
Fleming, P. J. S., Allen, L. R., Lapidge, S. J., Robley, A., Saunders, G. R., and Thomson, P.C (2006). A strategic approach to mitigating the impacts of wild canids: proposed activities of the Invasive Animals Cooperative Research Centre. Aust. J. Exp. Agric. 46, 753–762. doi: 10.1071/EA06009
Fox, M. W. (1984). The Whistling Hunters: Field Studies of the Asiatic Wild Dog (Cuon Alpinus). Albany, NY: State University of New York Press.
Frame, L. H., Malcolm, J. R., Frame, G. W., and Van Lawick, H. (1979). Social organization of African wild dogs (Lycaon pictus) on the Serengeti Plains, Tanzania 1967–1978. Z. Tierpsychol. 50, 225–249. doi: 10.1111/j.1439-0310.1979.tb01030.x
Frantz, L. A., Mullin, V. E., Pionnier-Capitan, M., Lebrasseur, O., Ollivier, M., Perri, A., et al. (2016). Genomic and archaeological evidence suggest a dual origin of domestic dogs. Science 352, 1228–1231. doi: 10.1126/science.aaf3161
Freeman-Gallant, C. R. (1996). DNA fingerprinting reveals female preference for male parental care in Savannah Sparrows. Proc. R. Soc. Lond. B Biol. Sci. 263, 157–160. doi: 10.1098/rspb.1996.0025
Fromhage, L., and Jennions, M. D. (2016). Coevolution of parental investment and sexually selected traits drives sex–role divergence. Nat. Commun. 7:12517. doi: 10.1038/ncomms12517
Garcelon, D. K., Roemer, G. W., Philips, R. B., et al. (1999). Food provisioning by island foxes, Urocyon littoralis, to conspecifics caught in traps. Southwest. Nat. 44, 83–86.
Geffen, E., Gompper, M. E., Gittleman, J. L., Luh, H.-K., MacDonald, D. W., and Wayne, R. K. (1996). Size, life–history traits, and social organization in the Canidae: a reevaluation. Am. Nat. 147, 140–160. doi: 10.1086/285844
Geffen, E., Kam, M., Hefner, R., Hersteinsson, P., Angerbjörn, A., Dalèn, L., et al. (2011). Kin encounter rate and inbreeding avoidance in canids. Mol. Ecol. 20, 5348–5358. doi: 10.1111/j.1365-294X.2011.05358.x
Geffen, E., and Macdonald, D. W. (1992). Small size and monogamy: spatial organization of Blanford's foxes, Vulpes cana. Anim. Behav. 44, 1123–1130. doi: 10.1016/S0003-3472(05)80324-0
Gese, E. M., Knowlton, F. F., Adams, J. R., Beck, K., Fuller, T. K., Murray, D. L., et al. (2015). Managing hybridization of a recovering endangered species: the red wolf Canis rufus as a case study. Curr. Zool. 61, 191–205. doi: 10.1093/czoolo/61.1.191
Girman, D. J., Mills, M. G. L., Geffen, E., and Wayne, R. K. (1997). A molecular genetic analysis of social structure, dispersal, and interpack relationships of the African wild dog (Lycaon pictus). Behav. Ecol. Sociobiol. 40, 187–198. doi: 10.1007/s002650050332
Gittleman, J. L. (1986). Carnivore brain size, behavioral ecology, and phylogeny. J. Mammal. 67, 23–36. doi: 10.2307/1380998
Goltsman, M., Kruchenkova, E. P., and Macdonald, D. W. (1996). The Mednyi Arctic foxes: treating a population imperilled by disease. Oryx 30, 251–258. doi: 10.1017/S0030605300021748
Gomendio, M. (1998). “Sperm competition in mammals,” in Sperm Competition and Sexual Selection, eds T. R. Birkhead and A. Møller (London: Academic Press), 667–756.
Gompper, M. E. (2002). Top Carnivores in the Suburbs? Ecological and Conservation Issues Raised by Colonization of North–eastern North America by Coyotes: The expansion of the coyote's geographical range may broadly influence community structure, and rising coyote densities in the suburbs may alter how the general public views wildlife. Bioscience 52, 185–190. doi: 10.1641/0006-3568(2002)052[0185:TCITSE]2.0.CO;2
Goodman, P. A. (1990). A History of Wolf Park 1972–1999 Ethology Series, Vol. 5. Battle Ground, IN: Institute of Ethology, North American Wildlife Park Foundation.
Gorman, M. L., and Trowbridge, B. J. (1989). “The role of odor in the social lives of carnivores,” in Carnivore Behavior, Ecology, and Evolution, ed J. L. Gittleman (Boston, MA: Springer), 57–88.
Gottelli, D., Sillero-Zubiri, C., Applebaum, G. D., Roy, M. S., Girman, D. J., Garcia-Moreno, J., et al. (1994). Molecular genetics of the most endangered canid: the Ethiopian wolf Canis simensis. Mol. Ecol. 3, 301–312. doi: 10.1111/j.1365-294X.1994.tb00070.x
Griffin, A. S., Alonzo, S. H., and Cornwallis, C. K. (2013). Why do cuckolded males provide paternal care? PLoS Biol. 11:e1001520. doi: 10.1371/journal.pbio.1001520
Griffith, S. C., Owens, I. P., and Thuman, K. A. (2002). Extra pair paternity in birds: a review of interspecific variation and adaptive function. Mol. Ecol. 11, 2195–2212. doi: 10.1046/j.1365-294X.2002.01613.x
Gusset, M., and Macdonald, D. W. (2010). Group size effects in cooperatively breeding African wild dogs. Anim. Behav. 79, 425–428. doi: 10.1016/j.anbehav.2009.11.021
Hackländer, K., Möstl, E., and Arnold, W. (2003). Reproductive suppression in female Alpine marmots, Marmota marmota. Anim. Behav. 65, 1133–1140. doi: 10.1006/anbe.2003.2159
Harrington, F. H., Mech, L. D., and Fritts, S. H. (1983). Pack size and wolf pup survival: their relationship under varying ecological conditions. Behav. Ecol. Sociobiol. 13, 19–26. doi: 10.1007/BF00295072
Harris, S., and Smith, G. C. (1987). Demography of two urban fox (Vulpes vulpes) populations. J. Appl. Ecol. 24, 75–86. doi: 10.2307/2403788
Harrison, D. J. (1992). Dispersal characteristics of juvenile coyotes in Maine. J. Wildl. Manag. 56, 128–138. doi: 10.2307/3808800
Hasson, O., and Stone, L. (2009). Male infertility, female fertility and extrapair copulations. Biol. Rev. 84, 225–244. doi: 10.1111/j.1469-185X.2008.00068.x
Hayssen, V. D., Van Tienhoven, A., and Van Tienhoven, A. (1993). Asdell's patterns of mammalian reproduction: a compendium of species-specific data. Can. Vet. J. 35, 658–659.
Helle, E., and Kauhala, K. (1991). Distribution history and present status of the raccoon dog in Finland. Ecography 14, 278–286. doi: 10.1111/j.1600-0587.1991.tb00662.x
Hennemann, I. I. I. W. W, Thompson, S. D., and Konecny, M. J. (1983). Metabolism of crab-eating foxes, Cerdocyon thous: ecological influences on the energetics of canids. Physiol. Zool. 56, 319–324. doi: 10.1086/physzool.56.3.30152596
Hennessy, C. (2007). Genetic aspects of a coyote population in a suburban landscape (MS thesis), The Ohio State University, Columbus, OH, United States.
Hennessy, C. A., Dubach, J., and Gehrt, S. D. (2012). Long–term pair bonding and genetic evidence for monogamy among urban coyotes (Canis latrans). J. Mammal. 93, 732–742. doi: 10.1644/11-MAMM-A-184.1
Hersteinsson, P., and Macdonald, D. W. (1982). Some comparisons between red and arctic foxes, Vulpes vulpes and Alopex lagopus, as revealed by radio tracking. Symp. Zool. Soc. Lond. 49, 259–289.
Hersteinsson, P., and Macdonald, D. W. (1992). Interspecific competition and the geographical distribution of red and arctic foxes Vulpes vulpes and Alopex lagopus. Oikos 64, 505–515. doi: 10.2307/3545168
Hoogland, J. L. (1979). Aggression, ectoparasitism, and other possible costs of prairie dog (Sciuridae, Cynomys spp.) coloniality. Behaviour 69, 1–35. doi: 10.1163/156853979X00377
Hoogland, J. L. (1998). Why do female Gunnison's prairie dogs copulate with more than one male? Anim. Behav. 55, 351–359. doi: 10.1006/anbe.1997.0575
Hrdy, S. B. (1974). Male-male competition and infanticide among the langurs (Presbytis entellus) of Abu, Rajasthan. Folia Primatol. 22, 19–58. doi: 10.1159/000155616
Hrdy, S. B. (1979). Infanticide among animals: a review, classification, and examination of the implications for the reproductive strategies of females. Ethol. Sociobiol. 1, 13–40. doi: 10.1016/0162-3095(79)90004-9
Huck, M., Fernandez-Duque, E., Babb, P., and Schurr, T. (2014). Correlates of genetic monogamy in socially monogamous mammals: insights from Azara's owl monkeys. Proc. R. Soc. Lond. B Biol. Sci. 281:20140195. doi: 10.1098/rspb.2014.0195
Hughes, J., and Macdonald, D. W. (2013). A review of the interactions between free-roaming domestic dogs and wildlife. Biol. Conserv. 157, 341–351. doi: 10.1016/j.biocon.2012.07.005
Iossa, G., Soulsbury, C. D., Baker, P. J., Edwards, K. J., and Harris, S. (2008a). Behavioral changes associated with a population density decline in the facultatively social red fox. Behav. Ecol. 20, 385–395. doi: 10.1093/beheco/arn149
Iossa, G., Soulsbury, C. D., Baker, P. J., Edwards, K. J., and Harris, S. (2008b). Body mass, territory size, and life–history tactics in a socially monogamous canid, the red fox Vulpes vulpes. J. Mammal. 89, 1481–1490. doi: 10.1644/07-MAMM-A-405.1
Isvaran, K., and Clutton-Brock, T. (2006). Ecological correlates of extra–group paternity in mammals. Proc. R. Soc. Lond. B Biol. Sci. 274, 219–224. doi: 10.1098/rspb.2006.3723
Jedrzejewski, W., Branicki, W., Veit, C., MeÐugorac, I., Pilot, M., Bunevic, A. N., et al. (2005). Genetic diversity and relatedness within packs in an intensely hunted population of wolves Canis lupus. Acta Theriol. 50, 3–22. doi: 10.1007/BF03192614
Jennions, M. D., and Petrie, M. (2000). Why do females mate multiply? A review of the genetic benefits. Biol. Rev. 75, 21–64. doi: 10.1017/S0006323199005423
Jöchle, W. (1997). Prolactin in canine and feline reproduction. Reprod. Dom. Anim. 32, 183–193. doi: 10.1111/j.1439-0531.1997.tb01280.x
Johnsingh, A. J. T. (1982). Reproductive and social behaviour of the dhole, Cuon alpinus (Canidae). J. Zool. 198, 443–463. doi: 10.1111/jzo.1982.198.4.443
Johnson, P. J., Noonan, M. J., Kitchener, A. C., Harrington, L. A., Newman, C., and Macdonald, D. W. (2017). Rensching cats and dogs: feeding ecology and fecundity trends explain variation in the allometry of sexual size dimorphism. R. Soc. Open Sci. 4:170453. doi: 10.1098/rsos.170453
Jungwirth, A., and Johnstone, R. A. (2018). Multiple evolutionary routes to monogamy: modelling the coevolution of mating decisions and parental investment. Am. Nat. 193:E000. doi: 10.1086/700698
Kamler, J. F., and Ballard, W. B. (2002). A review of native and nonnative red foxes in North America. Wildl. Soc. Bull. 30, 370–379. doi: 10.2307/3784493
Kamler, J. F., Ballard, W. B., Gese, E. M., Harrison, R. L., Karki, S., and Mote, K. (2004b). Adult male emigration and a female-based social organisation in swift foxes, Vulpes velox. Anim. Behav. 67, 699–702. doi: 10.1016/j.anbehav.2003.08.012
Kamler, J. F., Ballard, W. B., Gese, E. M., Karrison, R. L., Karki, S., and Mote, K. (2004c). Dispersal characteristics of swift foxes. Can. J. Zool. 82, 1837–1842. doi: 10.1139/z04-187
Kamler, J. F., Ballard, W. B., and Gipson, P. S. (2003a). Occurrence of feral dogs (Canis lupus familiaris) in Northwest Texas: an observation. Tex. J. Agric. Nat. Resour. 16, 75–77.
Kamler, J. F., Ballard, W. B., and Lemons, P. R. (2004a). Variation in mating system and group structure in two populations of swift foxes, Vulpes velox. Anim. Behav. 68, 83–88. doi: 10.1016/j.anbehav.2003.07.017
Kamler, J. F., and Gipson, P. S. (2000). Space and habitat use by resident and transient coyotes. Can. J. Zool. 78, 2106–2111. doi: 10.1139/z00-153
Kamler, J. F., Gray, M. M., Oh, A., and Macdonald, D. W. (2013b). Genetic structure, spatial organization, and dispersal in two populations of bat-eared foxes. Ecol. Evol. 3, 2892–2902. doi: 10.1002/ece3.683
Kamler, J. F., Keeler, K., Wiens, G., and Richardson, C. (2003b). Feral dogs, Canis familiaris, kill coyote, Canis latrans. Can. Field Nat. 117, 123–124.
Kamler, J. F., and Macdonald, D. W. (2014). Social organization, survival, and dispersal of cape foxes (Vulpes chama) in South Africa. Mammal. Biol. 79, 64–70. doi: 10.1016/j.mambio.2013.09.004
Kamler, J. F., Rostro-García, S., and Macdonald, D. W. (2017). Seasonal changes in social behavior and movements of bat-eared foxes in South Africa: disease implications. J. Mammal. 98, 1426–1433. doi: 10.1093/jmammal/gyx092
Kamler, J. F., Stenkewitz, U., Gharajehdaghipoor, T., and Macdonald, D. (2019). Social organization, home ranges, and extraterritorial forays of black-backed jackals on a game reserve in South Africa. J. Wildl. Manag. 83, 1–9. doi: 10.1002/jwmg.21748
Kamler, J. F., Stenkewitz, U., and Macdonald, D. W. (2013a). Lethal and sublethal effects of black-backed jackals on cape foxes and bat-eared foxes. J. Mammal. 94, 295–306. doi: 10.1644/12-MAMM-A-122.1
Karssene, Y., Chammem, M., Nowak, C., de Smet, K., Castro, D., Eddine, A., et al. (2018). Noninvasive genetic assessment provides evidence of extensive gene flow and possible high movement ability in the African golden wolf. Mammal. Biol. 92, 94–101. doi: 10.1016/j.mambio.2018.05.002
Kauhala, K., Helle, E., and Pietilä, H. (1998). Time allocation of male and female raccoon dogs to pup rearing at the den. Acta Theriol. 43, 301–310. doi: 10.4098/AT.arch.98-25
Kauhala, K., and Kowalczyk, R. (2011). Invasion of the raccoon dog Nyctereutes procyonoides in Europe: history of colonization, features behind its success, and threats to native fauna. Curr. Zool. 57, 584–598. doi: 10.1093/czoolo/57.5.584
Kauhala, K., and Saeki, M. (2004). “Raccoon dogs,” in Biology and Conservation of Wild Canids, eds D. W. Macdonald and C. Sillero-Zubiri (New York, NY: Oxford University Press), 217–226.
Kitchen, A. M., Gese, E. M., Karki, S. M., and Schauster, E. R. (2005a). Spatial ecology of swift fox social groups: From group formation to mate loss. J. Mammal. 86, 547–554. doi: 10.1644/1545-1542(2005)86[547:SEOSFS]2.0.CO;2
Kitchen, A. M., Gese, E. M., Waits, L. P., Karki, S. M., and Schauster, E. R. (2005b). Genetic and spatial structure within a swift fox population. J. Anim. Ecol. 74, 1173–1181. doi: 10.1111/j.1365-2656.2005.01017.x
Kitchen, A. M., Gese, E. M., Waits, L. P., Karki, S. M., and Schauster, E. R. (2006). Multiple breeding strategies in the swift fox, Vulpes velox. Anim. Behav. 71, 1029–1038. doi: 10.1016/j.anbehav.2005.06.015
Kitchen, A. M., and Knowlton, F. F. (2006). Cross–fostering in coyotes: evaluation of a potential conservation and research tool for canids. Biol. Conserv. 129, 221–225. doi: 10.1016/j.biocon.2005.10.036
Klare, U., Kamler, J. F., and Macdonald, D. W. (2011). The bat-eared fox: A dietary specialist? Mamm. Biol. 76, 646–650. doi: 10.1016/j.mambio.2011.06.005
Kleiman, D. G. (2011). Canid mating systems, social behavior, parental care and ontogeny: are they flexible? Behav. Gen. 41:803. doi: 10.1007/s10519-011-9459-0
Kleiman, D. G., and Brady, C. A. (1978). “Coyote behavior in the context of recent canid research: problems and perspectives,” in Coyotes: Biology, Behavior and Management, ed M. Bekoff (New York, NY: Academic Press), 163–188.
Kleiman, D. G., and Malcolm, J. R. (1981). “The evolution of male parental investment in mammals,” in Parental Care in Mammals, eds N. G. Solomon and J. A. French (Boston, MA: Spring), 129–149.
Klug, H. (2018). Why monogamy? A review of potential ultimate drivers. Front. Ecol. Evol. 6:30. doi: 10.3389/fevo.2018.00030
Koepfli, K. P., Dragoo, J. W., and Wang, X. (2017). “The evolutionary history and molecular systematics of the Musteloidea,” in Biology and Conservation of Musteloids, eds D. W. Macdonald, C. Newman, and L. A. Harrington (Oxford: Oxford University Press), 75–91.
Kokko, H., and Johnstone, R. A. (1999). Social queuing in animal societies: a dynamic model of reproductive skew. Proc. R. Soc. Lond. B Biol. Sci. 266, 571–578. doi: 10.1098/rspb.1999.0674
Kokko, H., Johnstone, R. A., and Clutton-Brock, T. H. (2001). The evolution of cooperative breeding through group augmentation. Proc. R. Soc. Lond. B Biol. Sci. 268, 187–196. doi: 10.1098/rspb.2000.1349
Kokko, H., Johnstone, R. A., and Wright, J. (2002). The evolution of parental and alloparental effort in cooperatively breeding groups: when should helpers pay to stay? Behav. Ecol. 13, 291–300. doi: 10.1093/beheco/13.3.291
Komers, P. E., and Brotherton, P. N. (1997). Female space use is the best predictor of monogamy in mammals. Proc. R. Soc. Lond. B Biol. Sci. 264, 1261–1270. doi: 10.1098/rspb.1997.0174
Kreeger, T. J., Seal, U. S., Cohen, Y., Plotka, E. D., and Asa, C. S. (1991). Characterization of prolactin secretion in gray wolves (Canis lupus). Can. J. Zool. 69, 1366–1374. doi: 10.1139/z91-192
Kruchenkova, E. P., Goltsman, M., Sergeev, S., and Macdonal, D.W. (2009). Is alloparenting helpful for Mednyi Island arctic foxes, Alopex lagopus semenovi? Naturwissenschaften 96, 457–466. doi: 10.1007/s00114-008-0494-5
Kvarnemo, C. (2005). Evolution and maintenance of male care: is increased paternity a neglected benefit of care? Behav. Ecol. 17, 144–148. doi: 10.1093/beheco/ari097
Kvarnemo, C. (2018). Why do some animals mate with one partner rather than many? A review of causes and consequences of monogamy. Biol. Rev. 93, 1795–1812. doi: 10.1111/brv.12421
Kvarnemo, C., and Simmons, L. W. (2013). Polyandry as a mediator of sexual selection before and after mating. Phil. Trans. R. Soc. B 368:20120042. doi: 10.1098/rstb.2012.0042
Lambert, C. T., Solomon, N. G., and Sabol, A. C. (2018). Genetic monogamy in socially monogamous mammals is primarily predicted by multiple life history factors. Front. Ecol. Evol. 6:139. doi: 10.3389/fevo.2018.00139
Lamprecht, J. (1979). Field observations on the behaviour and social system of the bat-eared fox Otocyon megalotis Desmarest. Z. Tierpsychol. 49, 260–284. doi: 10.1111/j.1439-0310.1979.tb00292.x
Latham, A. D. M., and Boutin, S. (2011). Wolf, Canis lupus, pup mortality: interspecific predation or non–parental infanticide? Can. Field Nat. 125, 158–161. doi: 10.22621/cfn.v125i2.1199
Laurenson, K., Sillero–Zubiri, C., Thompson, H., Shiferaw, F., Thirgood, S., and Malcolm, J. (1998). Disease as a threat to endangered species: Ethiopian wolves, domestic dogs and canine pathogens. Anim. Conserv. 1, 273–280. doi: 10.1111/j.1469-1795.1998.tb00038.x
Lindblad-Toh, K., Wade, C. M., Mikkelsen, T. S., Karlsson, E. K., Jaffe, D. B., Kamal, M., et al. (2005). Genome sequence, comparative analysis and haplotype structure of the domestic dog. Nature 438, 803–819. doi: 10.1038/nature04338
Lindström, E. (1986). Territory inheritance and the evolution of group–living in carnivores. Anim. Behav. 34, 1825–1835. doi: 10.1016/S0003-3472(86)80268-8
Lord, K., Feinstein, M., Smith, B., and Coppinger, R. (2013). Variation in reproductive traits of members of the genus Canis with special attention to the domestic dog (Canis familiaris). Behav. Proc. 92, 131–142. doi: 10.1016/j.beproc.2012.10.009
Loveridge, A. J., and Macdonald, D. W. (2001). Seasonality in spatial organization and dispersal of sympatric jackals (Canis mesomelas and C. adustus): implications for rabies management. J. Zool. 253, 101–111. doi: 10.1017/S0952836901000097
Loveridge, A. J., Searle, A. W., Murindagomo, F., and Macdonald, D. W (2007). The impact of sport–hunting on the population dynamics of an African lion population in a protected area. Biol. Conserv. 134, 548–558. doi: 10.1016/j.biocon.2006.09.010
Lucas, J. R., Waser, P. M., and Creel, S. R. (1994). Death and disappearance: estimating mortality risks associated with philopatry and dispersal. Behav. Ecol. 5, 135–141. doi: 10.1093/beheco/5.2.135
Lukas, D., and Clutton-Brock, T. (2012). Life histories and the evolution of cooperative breeding in mammals. Proc. R. Soc. Lond. B Biol. Sci. 279, 4065–4070. doi: 10.1098/rspb.2012.1433
Lukas, D., and Clutton-Brock, T. H. (2013). The evolution of social monogamy in mammals. Science 341, 526–530. doi: 10.1126/science.1238677
Lukas, D., and Huchard, E. (2014). The evolution of infanticide by males in mammalian societies. Science 346, 841–844. doi: 10.1126/science.1257226
Maas, B. (1993). Behavioural ecology and social organisation of the bat-eared fox in the Serengeti National Park, Tanzania (PhD dissertation), University of Cambridge, Cambridge, United Kingdom.
Maas, B., and Macdonald, D. W. (2004). “Bat-eared foxes,” in The Biology and Conservation of Wild Canids, eds D. W. Macdonald and C. Sillero-Zubiri (New York, NY: Oxford University Press), 227–242.
Macdonald, D. W. (1977). On food preference in the red fox. Mammal. Rev. 7, 7–23. doi: 10.1111/j.1365-2907.1977.tb00359.x
Macdonald, D. W. (1979b). The flexible social system of the golden jackal, Canis aureus. Behav. Ecol. Sociobiol. 5, 17–38. doi: 10.1007/BF00302692
Macdonald, D. W. (1980). “Social factors affecting reproduction amongst red foxes,” in The Red Fox, ed E. Zimen (Dordrecht: Springer), 123–175.
Macdonald, D. W. (1981). “Resource dispersion and the social organization of the red fox (Vulpes vulpes),” in Worldwide Furbearer Conference Proceedings (Maryland), 918–949.
Macdonald, D. W. (1983). The ecology of carnivore social behaviour. Nature 301, 379–384. doi: 10.1038/301379a0
Macdonald, D. W. (1984). Carnivore social behaviour—does it need patches? Nature 307, 390–390. doi: 10.1038/307390a0
Macdonald, D. W. (1996). Social behaviour of captive bush dogs (Speothos venaticus). J. Zool. 239, 525–543. doi: 10.1111/j.1469-7998.1996.tb05941.x
Macdonald, D. W., and Bacon, P. J. (1982). Fox society, contact rate and rabies epizootiology. Comp. Immunol. Microbiol. Infect. Dis. 5, 247–256. doi: 10.1016/0147-9571(82)90045-5
Macdonald, D. W., and Carr, G. M. (1989). “Food security and the rewards of tolerance,” in Comparative Socioecology: The Behavioural Ecology of Humans and Animals, eds V. Standen and R. A. Foley (Oxford: Blackwell Scientific Publications), 75–99.
Macdonald, D. W., and Carr, G. M. (1995). “Variation in dog society: between resource dispersion and social flux,” in The Domestic Dog: Its Evolution, Behaviour and Interactions With People, eds J. Serpell and P. Barrett (Cambridge: Cambridge University Press), 199–216.
Macdonald, D. W., and Courtenay, O. (1996). Enduring social relationships in a population of crab-eating zorros, Cerdocyon thous, in Amazonian Brazil (Carnivora, Canidae). J. Zool. 239, 329–355. doi: 10.1111/j.1469-7998.1996.tb05454.x
Macdonald, D. W., Creel, S., and Mills, M. G. (2004). “Canid society,” in The Biology and Conservation of Wild Canids, eds D. W. Macdonald and C. Sillero-Zubiri (Oxford: Oxford University Press), 85–106.
Macdonald, D. W., Doncaster, P., Newdick, M., Hofer, H., Mathews, F., and Johnson, P. J. (2016). “Foxes in the landscape: ecology and sociology,” in Wildlife Conservation on Farmland: Managing for Nature on Lowland Farms, eds D. W. Macdonald and R. A. Feber (Oxford: Oxford University Press), 20–46.
Macdonald, D. W., and Johnson, D. D. P. (2015). Patchwork planet: the resource dispersion hypothesis, society, and the ecology of life. J. Zool. 295, 75–107. doi: 10.1111/jzo.12202
Macdonald, D. W., and Kays, R. W. (2005). “Carnivores of the world: an introduction,” in Carnivores of the World, ed R. M. Nowak (Baltimore;London: The John's Hopkins University Press), 1–67.
Macdonald, D. W., and Moehlman, P. D. (1982). “Co-operation, altruism, and restraint in the reproduction of carnivores,” in Perspectives in Ethology: Ontogeny, eds P. P. G. Bateson and P. H. Klopfer (New York, NY: Plenum Press), 443–467.
Macdonald, D. W., Mosser, A., and Gittleman, J. L. (2010). “Felid society,” in The Biology and Conservation of Wild Felids, eds D. W. Macdonald and A. Loveridge (Oxford: Oxford University Press), 125–160.
Macdonald, D. W., and Newman, C. (2017). “Musteloid sociality: the grass –roots of society,” in Biology and Conservation of Musteloids, eds D. W. Macdonald, C. Newman, and L. A. Harrington (Oxford: Oxford University Press), 167–188.
Macdonald, D. W., and Sillero-Zubiri, C. (2004). “Dramatis personae. Wild Canids–an introduction and dramatis personae,” in The Biology and Conservation of Wild Canids, eds D. W. Macdonald and C. Sillero-Zubiri (Oxford: Oxford University Press), 3–38.
Majumder, S. S., Bhadra, A., Ghosh, A., Mitra, S., Bhattacharjee, D., Chatterjee, J., et al. (2014). To be or not to be social: foraging associations of free-ranging dogs in an urban ecosystem. Acta Ethol. 17, 1–8. doi: 10.1007/s10211-013-0158-0
Malcolm, J. (1979). The African wild dog, Lycaon pictus (PhD dissertation), Harvard University, Cambridge, MA, United States.
Malcolm, J. R. (1986). Socio-ecology of bat-eared foxes (Otocyon megalotis). J. Zool. 208, 457–469. doi: 10.1111/j.1469-7998.1986.tb01907.x
Malcolm, J. R., and Marten, K. (1982). Natural selection and the communal rearing of pups in African wild dogs (Lycaon pictus). Behav. Ecol. Sociobiol. 10, 1–13. doi: 10.1007/BF00296390
Malm, K. (1995). Regurgitation in relation to weaning in the domestic dog: a questionnaire study. Appl. Anim. Behav. Sci. 43, 111–122. doi: 10.1016/0168-1591(95)00556-8
Marino, J. (2003). Threatened Ethiopian wolves persist in small isolated Afroalpine enclaves. Oryx 37, 62–71. doi: 10.1017/S0030605303000139
Marino, J., Mitchel, R., and Johnson, P. J. (2010). Dietary specialization and climatic-linked variations in extant populations of Ethiopian wolves. Afr. J. Ecol. 48, 517–525. doi: 10.1111/j.1365-2028.2009.01140.x
Marino, J., Sillero-Zubiri, C., Gottelli, D., and Macdonald, D. W. (2013). The fall and rise of Ethiopian wolves: lessons for conservation of long-lived, social predators. Anim. Conserv. 16, 621–632. doi: 10.1111/acv.12036
Marino, J., Sillero-Zubiri, C., Johnson, P. J., and Macdonald, D. W. (2012). Ecological bases of philopatry and cooperation in Ethiopian wolves. Behav. Ecol. Sociobiol. 66, 1005–1015. doi: 10.1007/s00265-012-1348-x
Marino, J., Sillero-Zubiri, C., and Macdonald, D. W. (2006). Trends, dynamics and resilience of an Ethiopian wolf population. Anim. Conserv. 9, 49–58. doi: 10.1111/j.1469-1795.2005.00011.x
Maynard Smith, J. (1977). Parental investment: a prospective analysis. Anim. Behav. 25, 1–9. doi: 10.1016/0003-3472(77)90062-8
McLeod, D. V., and Day, T. (2014). Sexually transmitted infection and the evolution of serial monogamy. Proc. R. Soc. Lond. B Biol. Sci. 281:20141726. doi: 10.1098/rspb.2014.1726
McNutt, J. W. (1996). Sex-biased dispersal in African wild dogs, Lycaon pictus. Anim. Behav. 52, 1067–1077. doi: 10.1006/anbe.1996.0254
Mebatsion, T., Sillero-Zubiri, C., Gottelli, D., and Cox, J. H. (1992). Detection of rabies antibody by ELISA and RFFIT in unvaccinated dogs and in the endangered Simien jackal (Canis simensis) of Ethiopia. J. Vet. Med. B 39, 233–235. doi: 10.1111/j.1439-0450.1992.tb01162.x
Mech, L. D. (1995). The challenge and opportunity of recovering wolf populations. Conserv. Biol. 9, 270–278. doi: 10.1046/j.1523-1739.1995.9020270.x
Mech, L. D. (2017). Where can wolves live and how can we live with them? Biol. Conserv. 210, 310–317. doi: 10.1016/j.biocon.2017.04.029
Mech, L. D., and Boitani, L. (2003). Wolves: Behavior, Ecology, and Conservation. Chicago, IL: The University of Chicago Press.
Mech, L. D., Fritts, S. H., and Wagner, D. (1995). Minnesota Wolf Dispersal to Wisconsin and Michigan. Am. Midl. Nat. 133, 368–370. doi: 10.2307/2426402
Mech, L. D., Wolf, P. C., and Packard, J. M. (1999). Regurgitative food transfer among wild wolves. Can. J. Zool. 77, 1192–1195. doi: 10.1139/z99-097
Medjo, D. C., and Mech, L. D. (1976). Reproductive activity in nine- and ten-month-old wolves. J. Mammal. 57, 406–408. doi: 10.2307/1379708
Ménard, N., von Segesser, F., Scheffrahn, W., Pastorini, J., Vallet, D., Gaci, B., et al. (2001). Is male–infant caretaking related to paternity and/or mating activities in wild Barbary macaques (Macaca sylvanus)? C. R. Acad. Sci. 324, 601–610. doi: 10.1016/S0764-4469(01)01339-7
Méndez–Carvajal, P., and Moreno, R. (2014). Mammalia, Carnivora, Canidae, Canis latrans (Say, 1823): actual distribution in Panama. Check List 10, 376–379. doi: 10.15560/10.2.376
Messier, F., and Barrette, C. (1982). The social system of the coyote (Canis latrans) in a forested habitat. Can. J. Zool. 60, 1743–1753. doi: 10.1139/z82-227
Mock, D. W., Gowaty, P. A., Burley, N., Bluhm, C. K., Anderson, M. G., McKinney, F., et al. (1985). Avian monogamy. Ornithol. Monogr. 37, 1–121. doi: 10.2307/40166785
Moehlman, P. D. (1983). Socioecology of silverbacked and golden jackals (Canis mesomelas and Canis aureus). Adv Study Mammal Behav 7, 423–453.
Moehlman, P. D. (1986). “Ecology of cooperation in canids,” in Ecological Aspects of Social Evolution, eds D. I. Rubenstein and R. Wrangham (Princeton, NJ: Princeton University Press), 64–86.
Moehlman, P. D. (1989). “Intraspecific variation in canid social systems,” in Carnivore Behavior, Ecology, and Evolution, ed J. L. Gittleman (Boston, MA: Springer), 143–163.
Moehlman, P. D., and Hofer, H. (1997). “Cooperative breeding, reproductive suppression, and body mass in canids,” in Cooperative Breeding in Mammals (Cambridge: Cambridge University Press).
Møller, A. P. (2000). Male parental care, female reproductive success, and extrapair paternity. Behav. Ecol. 11, 161–168. doi: 10.1093/beheco/11.2.161
Møller, A. P., and Birkhead, T. R. (1993). Certainty of paternity covaries with paternal care in birds. Behav. Ecol. Sociobiol. 33, 261–268. doi: 10.1007/BF02027123
Moueix, C. H. M. (2006). Genetic verification of multiple paternity in two free–ranging isolated populations of African wild dogs (Lycaon pictus) (PhD dissertation), University of Pretoria, Pretoria, South Africa.
Mulder, R. A., Dunn, P. O., Cockburn, A., Lazenby Cohen, K. A., and Howell, M. J. (1994). Helpers liberate female fairy–wrens from constraints on extra–pair mate choice. Proc. R. Soc. Lond. B Biol. Sci. 255, 223–229. doi: 10.1098/rspb.1994.0032
Nicholson, W. S., Hill, E. P., and Briggs, D. (1985). Denning, pup–rearing, and dispersal in the gray fox in east–central Alabama. J. Wildl. Manag. 49, 33–37. doi: 10.2307/3801836
Norén, K., Hersteinsson, P., Samelius, G., Eide, N. E., Fuglei, E., and Elmhagen, B. (2012). From monogamy to complexity: social organization of arctic foxes (Vulpes lagopus) in contrasting ecosystems. Can. J. Zool. 90, 1102–1116. doi: 10.1139/z2012-077
Nowak, R. M. (2005). Walkers's Carnivores of the World. Baltimore, MD: The Johns Hopkins University Press.
Opie, C., Atkinson, Q. D., Dunbar, R. I., and Shultz, S. (2013). Male infanticide leads to social monogamy in primates. Proc. Natl. Acad. Sci. U.S.A. 110, 13328–13332. doi: 10.1073/pnas.1307903110
Orians, G. H. (1969). On the evolution of mating systems in birds and mammals. Am. Nat. 103, 589–603. doi: 10.1086/282628
Packard, J. M., Mech, L. D., and Seal, U. S. (1983). Social Influences on Reproduction in Wolves. Edmonton, AB: Candian Wildlife Service Reports.
Pal, S. K. (2005). Parental care in free–ranging dogs, Canis familiaris. Appl. Anim. Behav. Sci. 90, 31–47. doi: 10.1016/j.applanim.2004.08.002
Pal, S. K. (2008). Maturation and development of social behaviour during early ontogeny in free–ranging dog puppies in West Bengal, India. Appl. Anim. Behav. Sci. 111, 95–107. doi: 10.1016/j.applanim.2007.05.016
Pal, S. K. (2011). Mating system of free-ranging dogs (Canis familiaris). Int. J. Zool. 2011:314216. doi: 10.1155/2011/314216
Parker, P. G., and Waite, T. A. (1997). “Mating systems, effective population size, and conservation of natural populations,” in Behavioral Approaches to Conservation in the Wild, eds J. R. Clemmons and R. Buchholz (Cambridge: Cambridge University Press), 243–261.
Paul, M., Majumder, S. S., and Bhadra, A. (2014). Grandmotherly care: a case study in Indian free–ranging dogs. J. Ethol. 32, 75–82. doi: 10.1007/s10164-014-0396-2
Pérez-Barbería, F. J., Shultz, S., and Dunbar, R. I. (2007). Evidence for coevolution of sociality and relative brain size in three orders of mammals. Evolution 61, 2811–2821. doi: 10.1111/j.1558-5646.2007.00229.x
Phillips, M. K., Bangs, E. E., and Mech, L. D. (2004). “Grey wolves–yellowstone,” in The Biology and Conservation of Wild Canids, eds D. W. Macdonald and C. Sillero-Zubiri (New York, NY: Oxford University Press), 297–310.
Poessel, S. A., and Gese, E. M. (2013). Den attendance patterns in swift foxes during pup rearing: varying degrees of parental investment within the breeding pair. J. Ethol. 31, 193–201. doi: 10.1007/s10164-013-0368-y
Poiani, A., and Wilks, C. (2000). Cloacal microparasites and sexual selection in three Australian passerine species. Ethol. Ecol. Evol. 12, 251–258. doi: 10.1080/08927014.2000.9522799
Porton, I. J., Kleiman, D. G., and Rodden, M. (1987). Aseasonality of bush dog reproduction and the influence of social factors on the estrous cycle. J. Mammal. 68, 867–871. doi: 10.2307/1381569
Queller, D. C. (1997). Why do females care more than males? Proc. R. Soc. Lond. B Biol. Sci. 264, 1555–1557. doi: 10.1098/rspb.1997.0216
Rabb, G. B., Woolpy, J. H., and Ginsburg, B. E. (1967). Social relationships in a group of captive wolves. Am. Zool. 7, 305–311. doi: 10.1093/icb/7.2.305
Rakinsky, L. B. (1969). Outlines of canid and felid brain evolution. Ann. N. Y. Acad. Sci. 167, 277–288. doi: 10.1111/j.1749-6632.1969.tb20450.x
Ralls, K., Cypher, B., and Spiegel, L. K. (2007). Social monogamy in kit foxes: formation, association, duration, and dissolution of mated pairs. J. Mammal. 88, 1439–1446. doi: 10.1644/06-MAMM-A-348R.1
Ralls, K., Pilgrim, K. L., White, P. J., Paxinos, E. E., Schwartz, M. K., and Fleischer, R. C. (2001). Kinship, social relationships, and den sharing in kit foxes. J. Mammal. 82, 858–866. doi: 10.1644/1545-1542(2001)082<0858:KSRADS>2.0.CO;2
Randall, D. A., Marino, J., Haydon, D. T., Silero-Zubiri, C., Knobel, D. L., Tallents, L. A., et al. (2006). An integrated disease management strategy for the control of rabies in Ethiopian wolves. Biol. Conserv. 131, 151–162. doi: 10.1016/j.biocon.2006.04.004
Randall, D. A., Pollinger, J. P., Wayne, R. K., Tallents, L. A., Johnson, P. J., and Macdonald, D. W. (2007). Inbreeding is reduced by female–biased dispersal and mating behavior in Ethiopian wolves. Behav. Ecol. 18, 579–589. doi: 10.1093/beheco/arm010
Randall, D. A., Williams, S. D., Kuzmin, I. V., Rupprecht, C. E., Tallents, L. A., Tefera, Z., et al. (2004). Rabies in endangered Ethiopian wolves. Emerging Infect. Dis. 10, 2214–2217. doi: 10.3201/eid1012.040080
Rasmussen, J. L., and Tilson, R. L. (1984). Food provisioning by adult maned wolves (Chrysocyon brachyurus). Z. Tierpsychol. 65, 346–352. doi: 10.1111/j.1439-0310.1984.tb00109.x
Reed, D. H., and Frankham, R. (2003). Correlation between fitness and genetic diversity. Conserv. Biol. 17, 230–237. doi: 10.1046/j.1523-1739.2003.01236.x
Reich, A. (1981). The behavior and ecology of the African wild dog (Lycaon picrus) in the Kruger National Park (PhD dissertation), Yale University, New Haven, CT, United States.
Reichard, U. H., and Boesch, C. (2003). Monogamy: Mating Strategies and Partnerships in Birds, Humans and Other Mammals. Cambridge: Cambridge University Press.
Rhodes, C. J., Atkinson, R. P., Anderson, R. M., and Macdonald, D. W. (1998). Rabies in Zimbabwe: reservoir dogs and the implications for disease control. Philos. Trans. R. Soc. Lond. Biol. 353, 999–1010. doi: 10.1098/rstb.1998.0263
Robertson, S. M. (2016). Mammalian Reproductive Plasticity in Response to Resource Availability (PhD dissertation), North Carolina State University, Raleigh, NC, United States.
Roemer, G. W. (1999). The ecology and conservation of the island fox (Urocyon littoralis) (PhD dissertation), University of California, Los Angeles, CA, United States.
Roemer, G. W. (2004). “Island foxes,” in The Biology and Conservation of Wild Canids, eds D. W. Macdonald and C. Sillero-Zubiri (New York, NY: Oxford University Press), 173–184.
Roemer, G. W., Smith, D. A., Garcelon, D. K., and Wayne, R. K. (2001). The behavioural ecology of the island fox (Urocyon littoralis). J. Zool. 255, 1–14. doi: 10.1017/S0952836901001066
Rosenberg, H. (1971). Breeding the bat-eared fox at Utlca Zoo. Int. Zool. Yearb. 11, 101–102. doi: 10.1111/j.1748-1090.1971.tb01865.x
Ross, J., Hearn, A. J., and Macdonald, D. W. (2017). “The Bornean carnivore community: lessons from a little-known guild,” in Biology and Conservation of Musteloids, eds D. W. Macdonald, C. Newman, and L. A. Harrington (Oxford: Oxford University Press), 326–339.
Rutkowski, R., Krofel, M., Giannatos, G., Cirović, D., Männil, P., Volokh, A. M., et al. (2015). A European concern? Genetic structure and expansion of golden jackals (Canis aureus) in Europe and the Caucasus. PLoS ONE 10:e0141236. doi: 10.1371/journal.pone.0141236
Sacks, B. N., and Neale, J. C. (2001). Does paternal care of pups benefit breeding female coyotes? Southwest. Nat. 46, 121–126. doi: 10.2307/3672388
Schmidt, P. A., and Mech, L. D. (1997). Wolf pack size and food acquisition. Am. Nat. 150, 513–517. doi: 10.1086/286079
Sheldon, B. C. (2002). Relating paternity to paternal care. Philos. Trans. R. Soc. Lond. Biol. 357, 341–350. doi: 10.1098/rstb.2001.0931
Shultz, S., and Dunbar, R. I. (2007). The evolution of the social brain: anthropoid primates contrast with other vertebrates. Proc. R. Soc. Lond. B Biol. Sci. 274, 2429–2436. doi: 10.1098/rspb.2007.0693
Shultz, S., and Dunbar, R. I. (2010). Social bonds in birds are associated with brain size and contingent on the correlated evolution of life-history and increased parental investment. Biol. J. Linn. Soc. 100, 111–123. doi: 10.1111/j.1095-8312.2010.01427.x
Sillero-Zubiri, C., and Gottelli, D. (1995b). Diet and feeding behavior of Ethiopian wolves (Canis simensis). J. Mammal. 76, 531–541. doi: 10.2307/1382361
Sillero-Zubiri, C., Gottelli, D., and Macdonald, D. W. (1996a). Male philopatry, extra-pack copulations and inbreeding avoidance in Ethiopian wolves (Canis simensis). Behav. Ecol. Sociobiol. 38, 331–340. doi: 10.1007/s002650050249
Sillero-Zubiri, C., Johnson, P. J., and Macdonald, D. W. (1998). A hypothesis for breeding synchrony in Ethiopian wolves (Canis simensis). J. Mammal. 79, 853–858. doi: 10.2307/1383093
Sillero-Zubiri, C., King, A. A., and Macdonald, D. W. (1996b). Rabies and mortality in Ethiopian wolves (Canis simensis). J. Wildl. Dis. 32, 80–86. doi: 10.7589/0090-3558-32.1.80
Sillero-Zubiri, C., and Macdonald, D. W. (1998). Scent-marking and territorial behaviour of Ethiopian wolves Canis simensis. J. Zool. 245, 351–361. doi: 10.1111/j.1469-7998.1998.tb00110.x
Sillero-Zubiri, C., Marino, J., Gottelli, D., and Macdonald, D. W. (2004). “Ethiopian wolves: Afroalpine ecology, solitary foraging and intense sociality amongst Ethiopian wolves,” in The Biology and Conservation of Wild Canids, eds D. W. Macdonald and C. Sillero-Zubiri (Oxford: Oxford University Press), 311–320.
Sillero-Zubiri, C., Tattersall, F. H., and Macdonald, D. W. (1995a). Habitat selection and daily activity of giant mole-rats (Tachyorycies macrocephalus): significance to the Ethiopian wolf (Canis simensis) in the Afroalpine ecosystem. Biol. Conserv. 72, 77–84. doi: 10.1016/0006-3207(94)00067-Z
Sillero-Zubiri, C., Tattersall, F. H., and Macdonald, D. W. (1995b). Bale Mountains rodent communities and their relevance to the Ethiopian wolf (Canis simensis). Afr. J. Ecol. 33, 301–320. doi: 10.1111/j.1365-2028.1995.tb01041.x
Sillero-Zubiri, C. S., and Gottelli, D. (1995a). Spatial organization in the Ethiopian wolf Canis simensis: large packs and small stable home ranges. J. Zool. 237, 65–81. doi: 10.1111/j.1469-7998.1995.tb02747.x
Slater, G. J. (2015). Iterative adaptive radiations of fossil canids show no evidence for diversity-dependent trait evolution. PNAS 112:4897–4902. doi: 10.1073/pnas.1403666111
Slatyer, R. A., Mautz, B. S., Backwell, P. R., and Jennions, M. D. (2012). Estimating genetic benefits of polyandry from experimental studies: a meta-analysis. Biol Rev 87, 1–33. doi: 10.1111/j.1469-185X.2011.00182.x
Smith, D., Meier, T., Geffen, E., Mech, L. D., Burch, J. W., Adams, L. G., et al. (1997). Is incest common in gray wolf packs? Behav. Ecol. 8, 384–391. doi: 10.1093/beheco/8.4.384
Sol, D., Bacher, S., Reader, S. M., and Lefebvre, L. (2008). Brain size predicts the success of mammal species introduced into novel environments. Am. Nat. 172, S63–S71. doi: 10.1086/588304
Soulsbury, C. D., Baker, P. J., Iossa, G., and Harris, S. (2008). Fitness costs of dispersal in red foxes (Vulpes vulpes). Behav. Ecol. Sociobiol. 62, 1289–1298. doi: 10.1007/s00265-008-0557-9
Sparkman, A. M., Adams, J. R., Steury, T. D., Waits, L. P., and Murray, D. L. (2011a). Direct fitness benefits of delayed dispersal in the cooperatively breeding red wolf (Canis rufus). Behav. Ecol. 22, 199–205. doi: 10.1093/beheco/arq194
Sparkman, A. M., Adams, J. R., Steury, T. D., Waits, L. P., and Murray, D. L. (2012). Pack social dynamics and inbreeding avoidance in the cooperatively breeding red wolf. Behav. Ecol. 23, 1186–1194. doi: 10.1093/beheco/ars099
Sparkman, A. M., Waits, L. P., and Murray, D. L. (2011b). Social and demographic effects of anthropogenic mortality: a test of the compensatory mortality hypothesis in the red wolf. PLoS ONE 6:e20868. doi: 10.1371/journal.pone.0020868
Spiering, P. A., Somers, M. J., Maldonado, J. E., WildtMicaela, D. E., and Gunthe, S. (2010). Reproductive sharing and proximate factors mediating cooperative breeding in the African wild dog (Lycaon pictus). Behav. Ecol. Sociobiol. 64, 583–592. doi: 10.1007/s00265-009-0875-6
Stockley, P., and Hobson, L. (2016). Paternal care and litter size coevolution in mammals. Proc. R. Soc. Lond. B Biol. Sci. 283:20160140. doi: 10.1098/rspb.2016.0140
Stockley, P., Searle, J. B., MacDonald, D. W., and Jones, C. S. (1993). Female multiple mating behaviour in the common shrew as a strategy to reduce inbreeding. Proc. R. Soc. Lond. B Biol. Sci. 254, 173–179. doi: 10.1098/rspb.1993.0143
Storm, G. L., Andrews, R. D., Phillips, R. L., Bishop, R. A., Siniff, D. B., Tester, J. R., et al. (1976). Morphology, reproduction, dispersal, and mortality of midwestern red fox populations. Wildl Monogr 49, 3–82.
Stronen, A. V., Tessier, N., Jolicoeur, H., Paquet, P. C., Hénault, M., Villemure, M., et al. (2012). Canid hybridization: Contemporary evolution in human-modified landscapes. Ecol. Evol. 2, 2128–2140. doi: 10.1002/ece3.335
Sutor, A. (2007). Dispersal of the alien raccoon dog Nyctereutes procyonoides in Southern Brandenburg, Germany. Eur. J. Wildl. Res. 54, 321–326. doi: 10.1007/s10344-007-0153-8
Swanson, E. M., Holekamp, K. E., Lundrigan, B. L., Arsznov, B. M., and Sakai, S. T. (2012). Multiple determinants of whole and regional brain volume among terrestrial carnivorans. PLoS ONE 7:e38447. doi: 10.1371/journal.pone.0038447
Tannerfeldt, M., and Angerbjörn, A. (1998). Fluctuating resources and the evolution of litter size in the arctic fox. Oikos 83, 545–559. doi: 10.2307/3546681
Tóth, T., Krecsák, L., Szcs, E., Heltai, M., and Huszár, G (2009). Records of the golden jackal (Canis aureus Linnaeus, 1758) in Hungary from 1800th until 2007, based on a literature survey. Northwest J. Zool. 5, 386–405.
Trapp, G. R., and Hallberg, D. L. (1975). Ecology of the Gray Fox (Urocyon cinereoargenteus): A Review. The wild canids. New York, NY: Van Nostrand–Reinhold.
Tregenza, T., and Wedell, N. (2002). Polyandrous females avoid costs of inbreeding. Nature 415:71. doi: 10.1038/415071a
Trivers, R. (1972). “Parental investment and sexual selection.” in Sexual Selection and the Descent of Man, ed B. Campbell (Chicago, IL: Aldine Publishing Company), 136–179.
Trouwborst, A., Krofel, M., and Linnell, J. D. (2015). Legal implications of range expansions in a terrestrial carnivore: the case of the golden jackal (Canis aureus) in Europe. Biodiv. Conserv. 24, 2593–2610. doi: 10.1007/s10531-015-0948-y
Valdespino, C., Asa, C. S., and Bauman, J. E. (2002). Estrous cycles, copulation, and pregnancy in the fennec fox (Vulpes zerda). J. Mammal. 83, 99–109. doi: 10.1644/1545-1542(2002)083<0099:ECCAPI>2.0.CO;2
van Kesteren, F., Paris, M., Macdonald, D. W., Millar, R., Argaw, K., Johnson, P. J., et al. (2013). The physiology of cooperative breeding in a rare social canid; sex, suppression and pseudopregnancy in female Ethiopian wolves. Physiol. Behav. 122, 39–45. doi: 10.1016/j.physbeh.2013.08.016
van Kesteren, F., Sillero-Zubiri, C., Millar, R., Argaw, K., Macdonald, D. W., and Paris, M. (2012). Sex, stress and social status: patterns in fecal testosterone and glucocorticoid metabolites in male Ethiopian wolves. Gen. Comp. Endocrinol. 179, 30–37. doi: 10.1016/j.ygcen.2012.07.016
van Lawick-Goodall, H. V., and Lawick-Goodall, J. V. (1970). Innocent Killers. New York, NY: Ballantine Books.
Van Valkenburgh, B. (1991). Iterative evolution of hypercarnivory in canids (Mammalia: Carnivora): evolutionary interactions among sympatric predators. Paleobiology 17, 340–362. doi: 10.1017/S0094837300010691
Vanak, A. T., and Gompper, M. E. (2009). Dogs Canis familiaris as carnivores: their role and function in intraguild competition. Mammal Rev 39, 265–283. doi: 10.1111/j.1365-2907.2009.00148.x
Venkataraman, A. B., and Johnsingh, A. J. T. (2004). “The behavioural ecology of dholes in India,” in The Biology and Conservation of Wild Canids, eds D. W. Macdonald and C. Sillero-Zubiri (Oxford: Oxford University Press), 323-336.
Verner, J. (1964). Evolution of polygamy in the long-billed marsh wren. Evolution 18, 252–261. doi: 10.1111/j.1558-5646.1964.tb01597.x
Verner, J., and Willson, M. F. (1966). The influence of habitats on mating systems of North American passerine birds. Ecology 47, 143–147. doi: 10.2307/1935753
von Schantz, T. (1984). ‘Non-breeders’ in the red fox Vulpes vulpes: a case of resource surplus. Oikos 42, 59–65. doi: 10.2307/3544609
Waite, T. A., and Parker, P. G. (1997). Extrapair paternity and the effective size of socially monogamous populations. Evolution 51, 620–622. doi: 10.1111/j.1558-5646.1997.tb02450.x
Wang, G. D., Zhai, W., Yang, H. C., Fan, R. X., Cao, X., Zhong, L., et al. (2013). The genomics of selection in dogs and the parallel evolution between dogs and humans. Nat. Commun. 4:1860. doi: 10.1038/ncomms2814
Wang, X., and Tedford, R. H. (2008). Dogs: Their Fossil Relatives and Evolutionary History. New York, NY: Columbia University Press.
Wang, X., Tedford, R. H., and Jensen, P. (2007). “Evolutionary history of canids,” in The Behavioural Biology of Dogs, ed P. Jensen (Trowbridge: Cromwell Press), 3–20.
Weckerly, F. W. (1998). Sexual–size dimorphism: influence of mass and mating systems in the most dimorphic mammals. J. Mammal. 79, 33–52. doi: 10.2307/1382840
Werhahn, G., Senn, H., Ghazali, M., Karmacharya, D., Sherchan, A. M., Joshi, J., et al. (2018). The unique genetic adaptation of the Himalayan wolf to high–altitudes and consequences for conservation. Glob. Ecol. Conserv. 16:e00455. doi: 10.1016/j.gecco.2018.e00455
Werhahn, G., Senn, H., Kaden, J., Joshi, J., Bhattarai, S., Kusi, N., et al. (2017). Phylogenetic evidence for the ancient Himalayan wolf: towards a clarification of its taxonomic status based on genetic sampling from western Nepal. R. Soc. Open Sci. 4:170186. doi: 10.1098/rsos.170186
West, R. J. (2014). The evolution of large brain size in birds is related to social, not genetic, monogamy. Biol. J. Linn. Soc. 111, 668–678. doi: 10.1111/bij.12193
Westneat, D. F., and Stewart, I. R. (2003). Extra–pair paternity in birds: causes, correlates, and conflict. Ann. Rev. Ecol. Evol. Syst. 34, 365–396. doi: 10.1146/annurev.ecolsys.34.011802.132439
Weston Glenn, J. L., Civitello, D. J., and Lance, S. L. (2009). Multiple paternity and kinship in the gray fox (Urocyon cinereoargenteus). Mamm. Biol. 74, 394–402. doi: 10.1016/j.mambio.2008.10.003
Wetton, J. H., and Parkin, D. T. (1991). An association between fertility and cuckoldry in the house sparrow, Passer domesticus. Proc. R. Soc. Lond. B Biol. Sci. 245, 227–233. doi: 10.1098/rspb.1991.0114
Whitby, J. E., Johnstone, P., and Sillero–Zubiri, C. (1997). Rabies virus in the decomposed brain of an Ethiopian wolf detected by nested reverse transcription–polymerase chain reaction. J. Wildl. Dis. 33, 912–915. doi: 10.7589/0090-3558-33.4.912
White, P. J., and Garrott, R. A. (1997). Factors regulating kit fox populations. Can. J. Zool. 75, 1982–1988. doi: 10.1139/z97-830
White, P. J. and Ralls, K. (1993). Reproduction and spacing patterns of kit foxes relative to changing prey availability. J. Wildl. Manag. 57, 861–867.
Wittenberger, J. F., and Tilson, R. L. (1980). The evolution of monogamy: hypotheses and evidence. Annu. Rev. Ecol. Syst. 11, 197–232. doi: 10.1146/annurev.es.11.110180.001213
Wolff, J. O., and Macdonald, D. W. (2004). Promiscuous females protect their offspring. Trends Ecol. Evol. 19, 127–134. doi: 10.1016/j.tree.2003.12.009
Woodroffe, R., and Vincent, A. (1994). Mother's little helpers: patterns of male care in mammals. Trends Ecol. Evol. 9, 294–297. doi: 10.1016/0169-5347(94)90033-7
Wright, H. (2003). Monogamy in the bat–eared fox, Otocyon megalotis (PhD dissertation), University of Warwick, Coventry, United Kingdom.
Wright, H. W., Gray, M. M., Wayne, R. K., and Woodroffe, R. B. (2010). Mating tactics and paternity in a socially monogamous canid, the bat–eared fox (Otocyon megalotis). J. Mammal. 91, 437–446. doi: 10.1644/09-MAMM-A-046.1
Wright, H. W. Y. (2006). Paternal den attendance is the best predictor of offspring survival in the socially monogamous bat-eared fox. Anim. Behav. 71, 503–510. doi: 10.1016/j.anbehav.2005.03.043
Wydeven, A. P., Fuller, T. K., Weber, W., and MacDonald, K. (1998). The potential for wolf recovery in the northeastern United States via dispersal from southeastern Canada. Wildl. Soc. Bull. 26, 776–784.
Yasui, Y. (1998). The genetic benefits of female multiple mating reconsidered. Trends Ecol. Evol. 13, 246–250. doi: 10.1016/S0169-5347(98)01383-4
Young, A. J., and Monfort, S. L. (2009). Stress and the costs of extra–territorial movement in a social carnivore. Biol. Lett. 5, 439–441. doi: 10.1098/rsbl.2009.0032
Zabel, C. J. (1986). Reproductive behavior of the red fox (Vulpes vulpes): a longitudinal study of an island population (PhD dissertation), University of California, Santa Cruz, CA, United States.
Zabel, C. J., and Taggart, S. J. (1989). Shift in red fox (Vulpes vulpes) mating system associated with El Niño in the Bering Sea. Anim. Behav. 38, 830–838. doi: 10.1016/S0003-3472(89)80114-9
Keywords: paternal care, alloparental care, extra-pair mating, sociality, cooperation, canidae, carnivores, pair bond
Citation: Macdonald DW, Campbell LAD, Kamler JF, Marino J, Werhahn G and Sillero-Zubiri C (2019) Monogamy: Cause, Consequence, or Corollary of Success in Wild Canids? Front. Ecol. Evol. 7:341. doi: 10.3389/fevo.2019.00341
Received: 26 April 2019; Accepted: 26 August 2019;
Published: 13 September 2019.
Edited by:
Nancy G. Solomon, Miami University, United StatesReviewed by:
Eduardo Fernandez-Duque, Yale University, United StatesF. Stephen Dobson, Auburn University, United States
Copyright © 2019 Macdonald, Campbell, Kamler, Marino, Werhahn and Sillero-Zubiri. This is an open-access article distributed under the terms of the Creative Commons Attribution License (CC BY). The use, distribution or reproduction in other forums is permitted, provided the original author(s) and the copyright owner(s) are credited and that the original publication in this journal is cited, in accordance with accepted academic practice. No use, distribution or reproduction is permitted which does not comply with these terms.
*Correspondence: David W. Macdonald, ZGF2aWQubWFjZG9uYWxkJiN4MDAwNDA7em9vLm94LmFjLnVr
†These authors share joint first authorship