- Department of Wildlife and Fisheries Sciences, Texas A&M University, College Station, TX, United States
Animals often adjust their behavior and physiology in response to extreme weather. One reason they do so is because weather can cause sensory impairments but our understanding of this topic is limited. We therefore tested whether wind, a common component of extreme weather, impacts sensory systems in captive great-tailed grackles (Quiscalus mexicanus). In particular, we examined whether wind influences their blinking behavior, a fundamental aspect of visual processing that potentially limits information intake. We exposed the grackles to simulated wind while recording their blinking behavior. We found that the grackles exhibited increased blinking behavior when experiencing windy vs. calm conditions. These results suggest that wind may influence visual processing in birds and potentially impair information gathering.
Introduction
Animals are increasingly exposed to extreme weather events (Beniston and Stephenson, 2004) that are increasing in duration and severity (Rahmstorf and Coumou, 2011). While animals can modify their behavior and physiology in response to these events, we have a limited understanding of how they do so (Buchholz et al., 2019). In particular, windy conditions can influence behavior and physiology. Many species are less active when high wind speeds are present (Grubb, 1978; Robbins, 1981). In one case, 41% fewer avian species were observed at the same location on a windy vs. calm morning (Robbins, 1981). Individuals can also adjust their microhabitat preferences during windy conditions. Birds seek refuge in large trees close to the ground when winds are high (Grubb, 1975), presumably to shield themselves from the wind by remaining on the leeward side of large substrates. By seeking these refuges that minimize wind exposure, individuals can substantially reduce their metabolic costs (Wingfield and Ramenofsky, 2011).
One reason that animals adjust their behavior and physiology in response to windy conditions is because of sensory impairments. Individuals are not always able to hear auditory information above the noise generated by wind. However, animals can adjust their calling behavior when their vocalizations are masked by wind noise (Klump, 1996; Brumm and Slabbekoorn, 2005). For example, king penguins (Aptenodytes patagonicus) emit a higher number of calls and more syllables per call under windy compared to calm conditions (Lengagne et al., 1999). Wind can also limit olfactory abilities because the direction and strength of wind influences chemical dispersal (Finelli et al., 2000). Furthermore, wind can impair individuals' visual abilities. Because wind moves vegetation and other debris, birds can become less sensitive to movement (such as movement from a predator) in their environment (Carr and Lima, 2010). Lizards likely have more difficulty in detecting their rivals' visual displays in windy environments and their rivals therefore increase the speed of their displays under those conditions to most effectively communicate (Ord et al., 2007). Conversely, lizards may sometimes take advantage of the environmental movement that wind generates: lizards are most likely to move between hunting spots during high winds, potentially to reduce their predation rates by moving during times when environmental features are also moving (Jackson, 1974). Wind also affects blinking behavior in humans (Homo sapiens) such that humans blink more frequently when exposed to wind (Nakamori et al., 1997; Wu et al., 2014). We are unaware of any other studies that have examined the impact of wind on visual processing.
The aim of this study was therefore to test the hypothesis that wind impacts blinking behavior, a fundamental aspect of visual processing (Sweeney et al., 2013), in birds. Blinking impairs visual processing in humans (Volkmann et al., 1980; Bristow et al., 2005; Hoppe et al., 2018) but no empirical studies have tested whether it also impairs visual processing in birds or other species. However, indirect evidence suggests that blinking behavior in birds interferes with visual processing because (1) birds strategically inhibit their blinks (Cross et al., 2013; Yorzinski, 2016; Beauchamp, 2017) and (2) the nictitating membrane used for blinking is only semi-transparent in many avian species (Sivak, 1980). We tested the influence of wind on blinking behavior using captive great-tailed grackles (Quiscalus mexicanus), a songbird species that often inhabits open areas and is native to North and South America (Johnson and Peer, 2001). Grackles blink by sweeping their semi-transparent nictitating membranes across their eyes (their eyelids generally remain open when they are alert). The blinking behavior of the birds before, during, and after they were exposed to simulated windy conditions (fan directly blowing toward them) or control conditions was recorded. We tested birds when their heads were restrained and unrestrained. Because blinks are often associated with head movements in other species (Evinger et al., 1994; Gandhi, 2012; Yorzinski, 2016; Beauchamp, 2017), we wanted to test the impact of windy conditions on blinking behavior while controlling for the possible confounding effects of head movements. We expected that the birds' blinking behavior would increase when they were exposed to the simulated wind.
Methods
We examined the impact of wind on blinking behavior in captive great-tailed grackles (Quiscalus mexicanus) between April and June 2018 in College Station, Texas (30.56°N, 96.41°W). Adult birds were captured from the wild in College Station, Texas and surrounding areas. They were housed in outdoor aviaries (2.1 × 2.1 × 1.9 m) and given food (Purina cat chow and Dumor poultry layer feed) and water ad libitum. We tested 20 adult females and five adult males.
For each trial, a bird was captured from its outdoor aviary (using a butterfly net) and individually transported in a cloth bag to an indoor cage (0.76 × 0.46 × 0.46 m; ~160 m apart). The bird remained within this cage for at least 30 min so that it could acclimate to being indoors (food and water were provided ad libitum). After this acclimation period, the bird was secured in a foam cradle using velcro straps and placed inside a testing arena (Figure S1). The testing arena consisted of a section (0.83 × 0.63 × 0.66 m) within a large plastic box (Wolverine model cooler; Iowa Rotocast Plastics, Inc., Decorah, IA) that contained a speaker (frequency response: 100 Hz to 15 kHz; AN-MINI; Anchor Audio, Carlsbad, CA) connected to a laptop (MacBook Pro; Apple Inc., Cupertino, CA) as well as a bladeless fan (high setting; LOHOME mini bladeless fan). The speaker was placed on the floor of the arena and positioned 10 cm from the foam cradle. The fan was turned upside down, suspended from the roof of the testing arena and positioned immediately above the speaker. An LED light strip on the roof of the plastic box provided lighting (2.2 kLux; light meter positioned directly upwards at the location of the foam cradle; Easyview 31; Extech Instruments, Waltham, MA). In head-restrained trials, the bill of the bird was secured to a wooden dowel (using narrow strips of tape; Gorilla Glue, Inc., Cincinnati, OH) that was attached to the testing arena floor (0.14 m high) to prevent the bird from moving its head; in head-unrestrained trials, the wooden dowel was not present and the head of the bird could freely move. We performed the head-restrained trials to control for possible confounding effects of head movements (see Introduction).
In the head-restrained trials, two video cameras (60 frames/s; VIXIA HF R70; Canon, Inc., Irving, TX) were located on opposite sides of the bird to record each eye. The bird was also monitored in real time using camcorders (SRPRO-T855CAM; Swann Communications, Santa Fe Springs, CA) multiplexed to a DVR (model 2600; Swann Communications). In the head-unrestrained trials, an additional camcorder (60 frames/s; VIXIA HF R70; Canon, Inc.) was placed behind the bird so its eyes could be recorded if it turned its head backwards. In addition, another two camcorders with high frame rates (240 frames/s; Hero 5; GoPro, Inc., San Mateo, CA) were positioned on either side of the bird. The temperature and relative humidity inside the testing arena were continuously recorded (1 s interval; HOBO MX2301; Onset Computer Corporation, Bourne, MA).
Each bird was exposed to a treatment (wind) and control. During the wind treatment, the fan was turned on for 1 min (1.7 m/s at the bird; digital anemometer; LH300 model; Ehdis Car Accessories Co., Ltd, Guangdong, China). Because the fan generated a mechanical noise (75 dB at the bird; sound level meter; A weighting; slow setting; 407730 model; Extech Instruments), it was necessary to demonstrate that the bird was responding to the wind rather than the noise generated by the fan. As such, the bird was also exposed to a control. During the control, the speaker broadcast fan noise (75 dB at the bird) for 1 min but the fan was not turned on. The fan noise was an audio recording of the wind treatment (recorded using a Sennheiser K6 microphone (Sennheiser Electronic Cooperation, Wedemark, Germany) with an ME62 omnidirectional capsule connected to a Tascam DR-100 audio recorder).
The order of the treatment and control was randomized within birds. The first treatment or control began 5 min after the bird was secured in the foam cradle to allow the bird time to acclimate to the testing arena. The second treatment or control began 6 min after the first treatment or control ended (this allowed for a 1-min period following the first treatment or control as well as an additional 5 min before the next treatment or control). The bird was then removed from the testing arena 1 min after the second treatment or control ended. To ensure that the experimenter did not create disturbances when turning on or off the fan or noise, the fan and noise were digitally programmed to turn on and off at the appropriate times. The fan was turned on and off automatically using a digital timer outlet (model: T319; Nearpow); the noise was turned on and off automatically using an audio player (version 7; Quicktime; Apple, Inc.). Each bird was tested in two trials (head-restrained and head-unrestrained; separated by at least 9 days) and the order of these two trials was randomized across birds (the treatment-control order in the head-restrained and head-unrestrained trials was the same for a given bird).
The blinking behavior of the birds was measured from the videos using Quicktime (version 7; Apple, Inc.). All of the videos from a given trial were synchronized and a 3-min clip was extracted from each trial that included three time periods: a 1-min period before the fan/noise turned on, a 1-min period while the fan/noise was turned on, and a 1-min period after the fan/noise was turned off. For each trial, the frame at which each blink began and ended during the 3-min clip was recorded (Figure S2). A blink start was defined as the first frame when the nictitating membrane was visible and the blink end was defined as the first frame when the nictitating membrane was no longer visible. The birds kept their eyelids open continuously during the trials. The blinks in the left and right eye were recorded separately because the birds did not always synchronize their blinks between the eyes. In the head-unrestrained trials, the videos that were recorded at the high frame rate were consulted when the birds moved their heads quickly (their eyes appeared blurry using the videos with the lower frame rate during quick head turns so blinks sometimes needed to be confirmed using the videos with the higher frame rate).
To ensure reliability in coding the blinking behavior, both of the two coders practiced their scoring methods on a video from one of the trials. After an initial training period in which they scored at least 20 blinks and received feedback on their scoring from a trainer (JLY), they scored another 20 blinks and these blinks were compared to those scored by the trainer. The blinks from the coders and the trainer were scored similarly (over 98% of the blinks of each coder were scored in the same way as the blinks scored by the trainer).
Using customized scripts (Matlab; Mathworks, Inc., Natick, MA), the blink rate, blink duration, and percentage of time spent blinking were calculated for each time period. The blink rate was calculated as the sum of the number of blinks in the left eye and the number of blinks in the right eye divided by two and then divided by the time period (e.g., if the bird blinked 57 times with the left eye and 61 times with the right eye during the 1-min period before the wind began, the blink rate would be 59 blinks per minute). The blink duration was calculated as the sum of the mean duration of each blink in the left eye and mean duration of each blink in the right eye and then divided by two (e.g., if the bird's blinks each lasted, on average, 0.080 s in the left eye and 0.074 s in the right eye during the 1-min period before the wind began, the blink duration would be 0.077 s). The percentage of time the birds spent blinking was calculated as the sum of the percentage of time the left eye was blinking and the percentage of time the right eye was blinking divided by two (e.g., if the bird spent 7.50% of its time with its left eye blinking and 7.60% of its time with its right eye blinking during the 1-min period before the wind began, the percentage of time the bird spent blinking would be 7.55%). We used the mean values of the left and right eyes because the blinking behavior in the left and right eyes were highly correlated [blink rate: F(1, 24) = 6177.8, p < 0.0001; blink duration: F(1, 24) = 362.14, p < 0.0001; percentage of time the birds spent blinking: F(1, 24) = 2952.38, p < 0.0001]. In fact, the birds synchronized their blinks most of the time (mean ± SE: 96.0 ± 0.1%; range: 89.1–99.4%) and blink synchronization did not differ between the wind treatment (mean ± SE: 95.8 ± 0.2%; range: 89.1–99.4%) and control [mean ± SE: 96.1 ± 0.2%; range: 89.5–99.4%; F(1, 24) = 1.35, p = 0.26].
The data were analyzed using linear mixed-effects models with repeated measures in SAS (PROC MIXED; Version 9.4; SAS Institute Inc., Cary, NC). Because the blinking variables were highly correlated, we performed a factor analysis on the blinking variables (blink rate, blink duration, and percentage of time spent blinking) to extract a single principal-component factor (“blinking behavior”) derived from varimax rotation that explained 68.7% of the variance (Minitab version 18.1; Minitab Inc., State College, PA); the three variables loaded positively on a single factor. The factor score coefficients were highest for blink rate and percentage of time spent blinking (blink rate: 0.41; blink duration: 0.29; percentage of time spent blinking: 0.48); similarly, the proportion of variability explained by the factor (communality) was highest for blink rate and percentage of time spent blinking (blink rate: 0.72; blink duration: 0.35; percentage of time spent blinking: 0.99). We used this principal-component factor (natural log transformed to meet underlying assumptions of normality) as the dependent variable. The independent variables were the treatment-control (wind or noise), time period (before, during, or after the fan/noise was turned on), type (head restrained or head unrestrained), and their interaction as well as the sex of the bird, treatment order (wind or noise first), ambient temperature (mean across each minute time period) and ambient relative humidity (mean across each minute time period). Bird identity (random factor) was included within the model to account for repeated measures. A priori contrasts were performed to compare the blinking behavior between treatments, time periods, and type; 12 comparisons were performed and the false discovery rate correction was used to evaluate statistical significance [the false discovery rate was controlled at q* = 0.05; (Benjamini and Hochberg, 1995)]. We also reran this model using each blinking variable (blink rate, blink duration, and percentage of time spent blinking) as a dependent variable.
Results
The birds modified their blinking behavior when exposed to windy conditions (Table 1; Figure 1; Movie S1). Regardless of whether their heads were restrained or not, the birds exhibited increased blinking behavior during the wind compared to the noise [head restrained: t(1, 22) = 13.86, p < 0.0001; head unrestrained: t(1, 22) = 13.08, p < 0.0001]. Before the wind or noise, the birds' blinking behavior was similar [head restrained: t(1, 22) = 0.68, p = 0.51; head unrestrained: t(1, 22) = 1.23, p = 0.23]. The birds exhibited increased blinking behavior after the wind compared to after the noise [head restrained: t(1, 22) = 3.08, p = 0.0054; head unrestrained: t(1, 22) = 5.29, p < 0.0001]. The birds blinking behavior before, during, and after the wind or noise was similar in the head-restrained and head-unrestrained trials (p > 0.05). Their blinking behavior increased when the temperature was high and the relative humidity was low (Table 1); however, these environmental variables explained very little of the variation in blinking behavior (temperature: R2 = 0.8%; relative humidity: R2 = 0.1%). Blinking behavior was similar regardless of whether the birds' heads were restrained or not, whether they were exposed to the wind or noise first, and whether the birds were male or female (Table 1). Blink rate and percentage of time spent blinking explained more of the variability in blinking behavior than blinking duration. However, the results were qualitatively similar when the analysis was performed on the individual blinking variables (blink rate, blink duration, and percentage of time spent blinking; Table S1; Figures S3–S5).
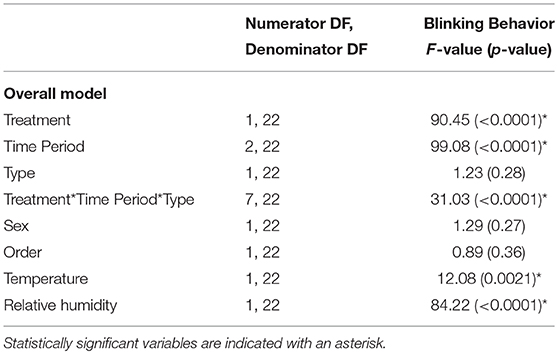
Table 1. The effect of treatment, time period, type, and their interaction as well as sex, order, temperature, and humidity on blinking behavior (composite factor including blink rate, blink duration, and percentage of time spent blinking).
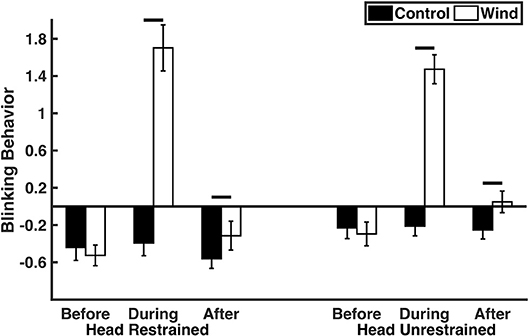
Figure 1. Blinking behavior (composite factor including blink rate, blink duration, and percentage of time spent blinking) before, during, and after the control or wind treatment (n = 25). Means ± standard errors are displayed. Horizontal lines indicate statistically significant comparisons.
Discussion
Great-tailed grackles modify their blinking behavior during windy conditions, supporting the hypothesis that wind impacts blinking behavior. The grackles increased their blinking behavior (including blink rate, blink duration, and percentage of time spent blinking) when exposed to windy conditions compared to control conditions. Furthermore, their blinks in the left and right eyes were highly synchronized.
Given that ocular irritants increase blinking behavior (Nakamori et al., 1997; Yang et al., 2001; Wu et al., 2014), we would expect windy conditions to increase blinking behavior. In fact, the grackles increased their blink rate by 45% when their heads were unrestrained during the windy compared to control conditions (their blink rate increased by 65% when their heads were restrained in the windy conditions). We are aware of only one other species (humans) in which the effect of windy conditions on blink rate has been investigated (Nakamori et al., 1997; Wu et al., 2014). In humans, blink rate increases by 35% in windy conditions (Nakamori et al., 1997). The wind speed in this grackle study (1.7 m/s) was greater than the wind speed in the human study [1.4 m/s; (Nakamori et al., 1997)]; because wind speed impacts blink rates (Wu et al., 2014), differences in wind speed between the studies could explain variation between the species in the magnitude of the wind's effect on blinking behavior (Wu et al., 2014). Future studies that examine the impact of additional wind speeds, including high wind speeds that birds would encounter during severe storms, would be valuable. Given that birds are exposed to high winds during flight, additional studies could also explore how birds modify their blinking behavior while flying. Even after the wind ended, the grackles exhibited slightly elevated blink rates, potentially because eye physiology cannot always return to equilibrium immediately after disturbances (Quallo et al., 2015).
Head movements had limited effects on the grackles' blinking behavior: their blinking behavior was similar regardless of whether their heads were restrained or not. Given that birds (Yorzinski, 2016; Beauchamp, 2017) and primates (Evinger et al., 1994; Gandhi, 2012) often blink when they move their heads, we expected that grackles would exhibit higher blink rates when their heads were unrestrained. However, even when their heads were unrestrained, they did not always exhibit many head movements because their bodies were still constrained. Additional studies that examine the relationship between head movements and blinks in grackles would be informative.
Interestingly, grackle blinking behavior in the left and right eyes was highly synchronized but the birds did have the ability to blink in one eye while not blinking in the other. These results are consistent with previous work showing that birds can sleep with one eye open and one eye closed because the two hemispheres of their brains can largely operate independently (Rattenborg et al., 1999a,b). Future experiments that expose only one eye to windy conditions would be informative to assess whether birds can minimize any costs associated with blinking by blinking more in the eye exposed to the wind but maintaining normal blinking behavior in the eye not exposed to the wind. Even if birds positioned themselves such that only one eye was exposed to wind (to minimize blinking costs in one eye), they may encounter tradeoffs. For example, when birds face away from high winds, their thermoregulation costs can be higher (Gebremedhin, 1987).
Because grackles increased their blinking behavior in the windy compared to control conditions, they likely experience limitations in visual processing during windy conditions. When the birds blink, their semi-transparent nictitating membranes move across their eyes. While it is not known whether birds have suppressed neural processing during blinks, studies in humans have shown that blinks suppress neural activity in areas of the brain associated with perceiving environmental change (Volkmann et al., 1980; Bristow et al., 2005). Regardless, given the semi-transparent nature of the nictitating membrane, the grackles likely have some visual impairments during blinks. Furthermore, previous studies have demonstrated that birds strategically modify their blinking behavior (Cross et al., 2013; Yorzinski, 2016; Beauchamp, 2017), suggesting that blinking imposes a cost. However, it is also possible that birds can compensate for any costs associated with blinking behavior using specialized neural processing abilities or performing behavioral adjustments. Future experiments that test whether blinking behavior impairs visual processing, or incurs other behavioral costs, will be essential to fully understanding how blinking impacts visual processing in birds.
If blinking does impair visual processing in birds, birds may experience reduced behavioral abilities during windy conditions because they are blinking more. Evidence suggests that birds are more likely to collide with obstacles (e.g., airplanes) during windy conditions (Steele, 2001), potentially because their ability to rapidly detect these obstacles is limited due to increased blinking behavior. Furthermore, birds have a lower tendency to flee from predators on windy days (Carr and Lima, 2010); their reduced tendency to flee could partially result from them being less aware of threats because they are blinking more. Predators could therefore potentially benefit by having greater hunting success during windy conditions while prey may be more vulnerable.
Our results demonstrate that environmental conditions can impact sensory systems. Additional studies are needed to examine whether individuals modify their behavior in weather extremes because they are experiencing sensory impairments. For example, songbirds often reduce their activity in windy conditions (Robbins, 1981) and they could be doing so to compensate for reduced visual abilities. Given that environmental extremes are expected to become more frequent (Easterling et al., 2000; Min et al., 2011), a better understanding of how animals adjust their behavior to varying environmental conditions will be valuable.
Data Availability
All datasets generated for this study are included in the manuscript/Supplementary Files (Supplementary Data Sheet 2).
Ethics Statement
The study was approved by Texas A&M University's Animal Care and Use Committee (#2016-0250).
Author Contributions
JY designed the study, collected the data, analyzed the data, and wrote the manuscript. SA coded the videos. All authors commented on and approved the manuscript.
Funding
This work was supported by the College of Agriculture and Life Sciences at Texas A&M University and Texas A&M AgriLife Research.
Conflict of Interest Statement
The authors declare that the research was conducted in the absence of any commercial or financial relationships that could be construed as a potential conflict of interest.
Acknowledgments
We thank Sabrien Wheeler for help with coding some of the videos.
Supplementary Material
The Supplementary Material for this article can be found online at: https://www.frontiersin.org/articles/10.3389/fevo.2019.00330/full#supplementary-material
References
Beauchamp, G. (2017). Half-blind to the risk of predation. Front. Ecol. Evol. 5:131. doi: 10.3389/fevo.2017.00131
Beniston, M., and Stephenson, D. B. (2004). Extreme climatic events and their evolution under changing climatic conditions. Glob. Planet. Change 44, 1–9. doi: 10.1016/j.gloplacha.2004.06.001
Benjamini, Y., and Hochberg, Y. (1995). Controlling the false discovery rate: a practical and powerful approach to multiple testing. J. R. Stat. Soc. 57, 289–300. doi: 10.1111/j.2517-6161.1995.tb02031.x
Bristow, D., Haynes, J. D., Sylvester, R., Frith, C. D., and Rees, G. (2005). Blinking suppresses the neural response to unchanging retinal stimulation. Curr. Biol. 15, 1296–1300. doi: 10.1016/j.cub.2005.06.025
Brumm, H., and Slabbekoorn, H. (2005). Acoustic communication in noise. Adv. Study Behav. 35:151–209. doi: 10.1016/S0065-3454(05)35004-2
Buchholz, R., Banusiewicz, J. D., Burgess, S., Crocker-Buta, S., Eveland, L., and Fuller, L. (2019). Behavioural research priorities for the study of animal response to climate change. Anim. Behav. 150, 127–137. doi: 10.1016/j.anbehav.2019.02.005
Carr, J. M., and Lima, S. L. (2010). High wind speeds decrease the responsiveness of birds to potentially threatening moving stimuli. Anim. Behav. 80, 215–220. doi: 10.1016/j.anbehav.2010.04.021
Cross, D. J., Marzluff, J. M., Palmquist, I., Minoshima, S., Shimizu, T., and Miyaoka, R. (2013). Distinct neural circuits underlie assessment of a diversity of natural dangers by American crows. Proc. R. Soc. B Biol. Sci. 280:20131046. doi: 10.1098/rspb.2013.1046
Easterling, D. R., Evans, J. L., Groisman, P. Y., Karl, T. R., Kunkel, K. E., and Ambenje, P. (2000). Observed variability and trends in extreme climate events: a brief review. Bull. Am. Meteorol. Soc. 81, 417–425. doi: 10.1175/1520-0477(2000)081<0417:OVATIE>2.3.CO;2
Evinger, C., Manning, K. A., Pellegrini, J. J., Basso, M. A., Powers, A. S., and Sibony, P. A. (1994). Not looking while leaping: the linkage of blinking and saccadic gaze shifts. Exp. Brain Res. 100, 337–344. doi: 10.1007/BF00227203
Finelli, C. M., Pentcheff, N. D., Zimmer, R. K., and Wethey, D. S. (2000). Physical constraints on ecological processes: a field test of odor-mediated foraging. Ecology 81, 784–797. doi: 10.1890/0012-9658(2000)081[0784:PCOEPA]2.0.CO;2
Gandhi, N. J. (2012). Interactions between gaze-evoked blinks and gaze shifts in monkeys. Exp. Brain Res. 216, 321–339. doi: 10.1007/s00221-011-2937-z
Gebremedhin, K. G. (1987). Effect of animal orientation with respect to wind direction on convective heat loss. Agric. Forest Meteorol. 40, 199–206. doi: 10.1016/0168-1923(87)90007-4
Grubb, T. C. Jr. (1975). Weather-dependent foraging behavior of some birds wintering in a deciduous woodland. Condor 77, 175–182. doi: 10.2307/1365788
Grubb, T. C. Jr. (1978). Weather-dependent foraging rates of wintering woodland birds. Auk 95, 370–376.
Hoppe, D., Helfmann, S., and Rothkopf, C. A. (2018). Humans quickly learn to blink strategically in response to environmental task demands. Proc. Natl. Acad. Sci. U.S.A. 115, 2246–2251. doi: 10.1073/pnas.1714220115
Jackson, J. F. (1974). Utilization of periods of high sensory complexity for site change in two lizards. Copeia 1974, 785–787 doi: 10.2307/1442696
Johnson, K., and Peer, B. D. (2001). “Great-tailed grackle (Quiscalus mexicanus), version 2.0,” in The Birds of North America, eds A. F. Poole and F. B. Gill. (Ithaca, NY: Cornell Lab of Ornithology).
Klump, G. M. (1996). “Bird communication in the noisy world,” in Ecology and Evolution of Acoustic Communication in Birds, eds D. E. Kroodsma, and E. H. Miller. (Ithaca, NY: Cornell University Press, 321–338.
Lengagne, T., Aubin, T., Lauga, J., and Jouventin, P. (1999). How do king penguins (Aptenodytes patagonicus) apply the mathematical theory of information to communicate in windy conditions? Proc. Royal. Soc. B 266, 1623–1628. doi: 10.1098/rspb.1999.0824
Min, S.-K., Zhang, X., Zwiers, F. W., and Hegerl, G. C. (2011). Human contribution to more intense precipitation extremes. Nature 470, 378–381. doi: 10.1038/nature09763
Nakamori, K., Odawara, M., Nakajima, K., Mizutani, T., and Tsubota, K. (1997). Blinking is controlled primarily by ocular surface conditions. Am. J. Ophthalmol. 124, 24–30. doi: 10.1016/S0002-9394(14)71639-3
Ord, T. J., Peters, R. A., Clucas, B., and Stamps, J. A. (2007). Lizards speed up visual displays in noisy motion habitats. Proc. Royal. Soc. B 274, 1057–1062. doi: 10.1098/rspb.2006.0263
Quallo, T., Vastani, N., Horridge, E., Gentry, C., Parra, A., Moss, S., et al. (2015). TRPM8 is a neuronal osmosensor that regulates eye blinking in mice. Nat. Comm. 6:7150. doi: 10.1038/ncomms8150
Rahmstorf, S., and Coumou, D. (2011). Increase of extreme events in a warming world. Proc. Natl. Acad. Sci. U.S A. 108, 17905–17909. doi: 10.1073/pnas.1101766108
Rattenborg, N. C., Lima, S. L., and Amlaner, C. J. (1999a). Half-awake to the risk of predation. Nature 397, 397–398. doi: 10.1038/17037
Rattenborg, N. C., Lima, S. L., and Amlaner, C. J. (1999b). Facultative control of avian unihemispheric sleep under the risk of predation. Behav. Brain Res. 105, 163–172.
Sivak, J. G. (1980). Avian mechanisms for vision in air and water. Trends Neurosci. 3, 314–317. doi: 10.1016/0166-2236(80)90113-7
Steele, W. K. (2001). “Factors influencing the incidence of bird-strikes at Melbourne Airport, 1986–2000,” in Proceedings of the 3rd Bird Strike Committee-USA/Canada Joint Annual Meeting (Calgary, AB), 144–157.
Sweeney, D. F., Millar, T. J., and Raju, S. R. (2013). Tear film stability: a review. Exp. Eye Res. 117, 28–38. doi: 10.1016/j.exer.2013.08.010
Volkmann, F. C., Riggs, L. A, and Moore, R. K. (1980). Eyeblinks and visual suppression. Science 207, 900–902. doi: 10.1126/science.7355270
Wingfield, J. C., and Ramenofsky, M. (2011). “Hormone-behavior interrelationships of birds in response to weather,” in Advances in the Study of Behavior, Vol. 43, eds H. Jane Brockmann, T. J. Roper, M. Naguib, J.C. Mitani, and L.W. Simmons (Burlington: Elsevier Inc, Academic Press), 93–188. doi: 10.1016/B978-0-12-380896-7.00003-4
Wu, Z., Begley, C. G., Situ, P., and Simpson, T. (2014). The effects of increasing ocular surface stimulation on blinking and sensation. Invest. Ophthalmol. Vis. Sci.. 55, 1555–1563. doi: 10.1167/iovs.13-13780
Yang, X., Zhang, Y. P., Chen, D., Chen, W. G., and Wang, R. (2001). Eye irritation caused by formaldehyde as an indoor air pollution—A controlled human exposure experiment. Biomed. Environ. Sci. 14, 229–236.
Keywords: attention, eye blink, head movement, wind, weather
Citation: Yorzinski JL and Argubright S (2019) Wind Increases Blinking Behavior in Great-Tailed Grackles (Quiscalus mexicanus). Front. Ecol. Evol. 7:330. doi: 10.3389/fevo.2019.00330
Received: 10 June 2019; Accepted: 16 August 2019;
Published: 03 September 2019.
Edited by:
Guy Gilles Beauchamp, Independent Researcher, Montréal, QC, CanadaReviewed by:
Jennie Carr, Washington College, United StatesSteven Lima, Indiana State University, United States
Copyright © 2019 Yorzinski and Argubright. This is an open-access article distributed under the terms of the Creative Commons Attribution License (CC BY). The use, distribution or reproduction in other forums is permitted, provided the original author(s) and the copyright owner(s) are credited and that the original publication in this journal is cited, in accordance with accepted academic practice. No use, distribution or reproduction is permitted which does not comply with these terms.
*Correspondence: Jessica L. Yorzinski, anlvcnppbnNraSYjeDAwMDQwO3RhbXUuZWR1