- 1Department of Forest and Conservation Sciences, University of British Columbia, Vancouver, BC, Canada
- 2Wildlife Research Division, Environment and Climate Change Canada, National Wildlife Research Centre, Ottawa, ON, Canada
- 3Department of Integrative Biology, University of Guelph, Guelph, ON, Canada
- 4Environment and Climate Change Canada, Pacific Wildlife Research Centre, Delta, BC, Canada
For migratory animals, events at one stage of the annual cycle can produce constraints or benefits that carry over to subsequent stages. Differing life-history strategies among individuals can influence the expression of these carry-over effects, leading to pronounced within-population variation in migration. For example, reproductive roles can drive spatiotemporal segregation during the non-breeding season and promote sex-specific carry-over effects, such as reproductive effort affecting autumn migration behavior. For an alpine breeding population of horned larks Eremophila alpestris in northern British Columbia, Canada, we addressed sex-specific variation in migration behavior and carry-over effects during both autumn and spring migration using light-level geolocators. Males spent more time farther north and arrived an average of 6 days earlier at the breeding site in spring. Females delayed autumn departure following greater reproductive effort, in turn demonstrating flexible migration behavior by increasing migration speed and decreasing stopover use. Males maintained autumn migration behavior regardless of reproductive effort or departure date. Finally, both sexes used staging areas in spring (average stopover = 41 days), with consequences for breeding success. Individuals that used staging areas during spring migration exhibited greater nest success and produced 1.8 more fledglings on average than those that migrated directly from their winter site. Consistent use of staging areas may allow individuals to monitor environmental conditions and optimize their breeding arrival date to acquire high-quality territories while avoiding the cost of arriving too early in a harsh alpine habitat. Overall, our results indicate: (1) sex-specific flexibility in migration strategies that carry-over to and from the reproductive period, and (2) spring staging areas may be a critical component of the annual life-cycle for alpine breeding larks. These behaviors may be particularly important for alpine and arctic birds because the stochastic nature of their breeding habitat likely selects for flexible responses to prevailing conditions.
Introduction
Migratory birds can spend up to 75% of the annual cycle away from the breeding site (Webster et al., 2002), often using multiple habitats in different locations and for variable time periods (Marra et al., 1998; Briedis et al., 2018). How individuals disperse spatially across the landscape and the timing of migration events can have important consequences for both individual fitness and population dynamics (Møller et al., 2008; Legagneux et al., 2012; Runge et al., 2014; Wilson et al., 2018). Migratory strategies can vary among populations (Gilroy et al., 2016; Knight et al., 2018; Gow et al., 2019), in response to inclement weather (Morganti et al., 2011; Schmaljohann et al., 2017), and among individuals of different age or sex classes (Tøttrup et al., 2012; McKinnon et al., 2014; Woodworth et al., 2016; Briedis et al., 2019). Understanding drivers of within-population variation in migratory strategies and how differences are linked across seasons is critical to understanding the ecological and evolutionary processes shaping life-history dynamics throughout the full-annual cycle (Marra et al., 2015; Paxton and Moore, 2017).
Spatial and temporal segregation of the sexes during the non-breeding season occur in avian populations, but the drivers of these patterns are not well-understood (McKinnon and Love, 2018). For sexually dimorphic species, the larger sex (often male) may winter farther north or closer to the breeding site (Cristol et al., 1999; Macdonald et al., 2015) and exhibit protandry (i.e., earlier spring arrival for males; Morbey and Ydenberg, 2001). Non-mutually exclusive hypotheses such as the “body size,” “arrival time,” and “social dominance” hypotheses, all predict that larger individuals can withstand harsher winter conditions that enable them to remain closer to the breeding site (Ketterson and Nolan, 1976; Gauthreaux, 1978) and better monitor environmental cues to match breeding site arrival with optimal weather conditions (Saino et al., 2010). The selective advantage of an earlier arrival includes improved territory and mate acquisition (Kokko, 1999; Reudink et al., 2009) and reproductive success (Norris et al., 2004; Smith and Moore, 2005; Gienapp and Bregnballe, 2012), and is most apparent for the more territorial sex (Møller, 2004; Kokko et al., 2006). Thus, the reproductive roles of each sex can produce spatial and temporal differences among individuals during the non-breeding season (Gow and Wiebe, 2014; Meissner, 2015), and consequently may lead to sex-specific fitness consequences of variation in migration behaviors (Saino et al., 2017).
The annual cycle is partitioned into specific life-history stages that are linked across seasons and conditions that influence energy reserves in one season can “carry-over” to influence subsequent stages (Marra et al., 1998). Conditions during the non-breeding season can influence breeding site arrival and success (Norris et al., 2004; Norris, 2005; Harrison et al., 2011). Similarly, breeding success may affect autumn departure date (Stutchbury et al., 2011; Meissner, 2015; van Wijk et al., 2017) which in-turn can influence stopover ecology and arrival at the winter site (Briedis et al., 2016; Gow et al., 2019). Thus, carry-over effects can cascade through temporally-linked phases of the annual cycle during both autumn and spring migration (Piersma, 1987). Behavioral adjustments such as altering stopover duration can allow individuals to buffer cascading effects, but the ability to adjust behavior may be condition-dependent (Gómez et al., 2017). Thus, during autumn migration (post-breeding), individuals that invested more in reproduction may have a reduced capacity for flexible migration behavior, consequently exhibiting greater delays in arrival at non-breeding sites. Similarly, energy-constrained individuals that delay spring migration and arrive late to the breeding site may delay clutch initiation or experience reduced breeding success. Given differences in reproductive investment, males and females may have differing abilities to compensate for delayed migration events.
Variation in migratory strategies among individuals in response to reproductive effort and timing is a type of phenotypic flexibility, or the capacity to reversibly alter phenotypic expression (i.e., behavior) in response to prevailing conditions (Piersma and Drent, 2003). Populations may differ in their capacity for flexible migration behavior based on the breeding and non-breeding habitat to which they are adapted. For example, short-distance migrants exhibit greater flexibility in phenology, potentially by monitoring environmental conditions near the breeding site (Usui et al., 2017; Lehikoinen et al., 2019). Additionally, phenotypic flexibility should be adaptive in stochastic conditions (Piersma and Drent, 2003), and as such birds that breed in highly variable environments, like alpine or arctic habitats, may be more likely to demonstrate and benefit from flexible migration behaviors. Recent studies from far northern latitudes indicate strong potential flexibility in migration timing and stopover behavior (e.g., Krause et al., 2016; Schmaljohann et al., 2017). Addressing within-population variation of migratory traits for alpine birds will advance our understanding of sex-specific flexible migration behaviors and thus the capacity for populations to respond to rapidly changing environments (Shaw, 2016; Beever et al., 2017).
In this study, we examined sex-specific variation in migration behavior and the carry-over effects between reproductive output and migration strategies for an alpine breeding population of horned larks Eremophila alpestris. Specifically, we tested four predictions involving spatial and temporal differences between sexes across the full-annual cycle. First, since larks are sexually dimorphic (Beason, 1995) we predicted spatial sex segregation during the non-breeding season such that the larger males would remain closer to the breeding site. Second, we expected that males would depart and arrive at the breeding site earlier than females, demonstrating protandry. Third, we predicted greater nesting effort or success would influence departure dates from the breeding site, and that a later departure would influence subsequent arrival at non-breeding sites. We expect breeding effort to more strongly influence female migration timing because female larks invest more in reproduction, being solely responsible for nest building, incubation, and half of offspring provisioning (Goullaud et al., 2018). Finally, we predicted that migration distance and departure date from the non-breeding site would influence spring arrival date and subsequent breeding success. We expected this inter-dependency to be most pronounced in males due to the territorial advantage of arriving early on the breeding site (Reudink et al., 2009).
Methods
Study System and Migratory Behavior
From 2015 to 2018, we studied a population of horned lark in ~4 km2 of alpine tundra (elevation: 1,650–2,000 m) on Hudson Bay Mountain (HBM) in northern British Columbia, Canada (54.8°N, 127.3°W). Horned larks are open-country, ground-nesting songbirds (28–40 g) that breed in sparsely-vegetated habitats from 0 to over 4,000 m above sea level (Beason, 1995). Snow-melt at HBM often extends into mid-June, resulting in short breeding seasons from mid-May or early-June to late July (41–57 days; MacDonald et al., 2013; Martin et al., 2017). Females lay 2–5 eggs per nest (average = 3.6) and initiate 1–3 clutches per season, including re-nests (Camfield et al., 2010). Most females produce a single brood; on average 7.7% of females have two broods in one season (de Zwaan, unpublished data).
Horned lark populations throughout the United States are partially migratory or resident, while those in Canada are obligate migrants (Beason, 1995). Wintering locations are predominantly below 49° latitude and extend as far south as the Chihuahuan grasslands in Mexico (Sullivan et al., 2009). Larks have been observed arriving in southern Canada as early as February (Cannings and Threlfall, 1981). The migratory connectivity or phenology of alpine lark populations is unknown, but they are assumed to be at least altitudinal migrants (Beason, 1995).
Field Methods
Nests were located during the nest building or incubation stage using behavioral observations. If discovered during incubation, clutch initiation was estimated by back-calculating from hatch date using an average incubation period of 12 days (de Zwaan et al., 2019). Nests were monitored every 2 days to record nest fate (fledged, depredated), clutch size, and number of fledglings. Both males and females were captured at the nest during the nestling period using bownets that were triggered when adults entered to provision the nestlings (de Zwaan et al., 2018). Each adult was measured for body size and condition traits (i.e., wing, tarsus, mass, fat) and ringed with one U.S. Geological Survey numbered aluminum ring and three color rings for subsequent identification.
From 2015 to 2017, we fit 37 males and 22 females with Intigeo-P65B1-11 light-level geolocators (Migrate Technology Inc.). The geolocators were attached using a leg-loop harness (Rappole and Tipton, 1990) tied using 45 lb test nylon string (Lee Valley Tools Ltd., Ottawa, Canada), allowing the fit of each harness to be adjusted for individuals in the field. The string was double knotted and glued with epoxy to prevent geolocator loss. The total weight of the geolocator (0.77 g) plus harness (0.20 g) was 0.97 g which, on an average lark for this population (35.1 g; Camfield et al., 2010), is 2.8% of body mass. All birds were captured on fair-weather days and released in under 12 min. We monitored individuals for ~15 min after release to ensure there were no immediate effects of the geolocator. The following year, returning individuals with geolocators were captured at the nest during the nestling stage (males) or the incubation stage (females) using a noose-line trap surrounding the nest (de Zwaan et al., 2018). We removed the geolocator from recaptured birds, measured body size traits, and released them in under 5 min.
Geolocator Analysis
All analyses were performed in R version 3.5.1 (R Core Team, 2018). Based on re-sights, the average return rate for geolocator birds was 59.3% (35 of 59 tagged birds) vs. 68.2% for ringed birds without geolocators. Due to significant trap avoidance in the first season, we retrieved 17 geolocators (8 males, 9 females). Pre-processing of drift-adjusted.lux files and calibration were conducted using the package “GeoLight” (Lisovski and Hahn, 2013). We used a light level threshold of 1 to estimate twilights and removed outliers if they differed by more than 30 min from adjacent twilight estimates. To calibrate the data, we used the “Hill-Ekstrom method” which estimates the zenith angle based on the lowest amount of variance in latitude estimates during stationary periods (Hill and Braun, 2001; Ekstrom, 2004). This method provides more accurate location estimates than both “roof-top” and “on-the-bird” calibration when a lengthy stationary period occurs near an equinox (Lisovski et al., 2012). We identified likely stationary periods (>10 days) during the non-breeding season using the “changeLight” function. Stationary periods were distinguished from movement based on high probability changes in twilight times, where a change point probability greater than the 90th percentile of all probabilities calculated within the entire migration period indicated movement. We then estimated the zenith angle based on the longest stationary period in the non-breeding season.
Location estimates were derived using the package “SGAT” (Sumner et al., 2009), because it allows prior distribution knowledge and bird behavior to be incorporated in a Bayesian framework and tends to be more accurate than location estimates derived from “GeoLight” (Roberto-Charron, 2018). “SGAT” uses the curve method to estimate locations based on the difference between twilight times, in combination with movement behavior priors which apply constraints to the estimates. We first identified likely stopover locations where the bird was stationary for ≥3 days within a distance threshold of 150 km. We created a prior movement model of flight speeds applied to each day of the migration period where most speeds were from 0 to 40 km/h but allowed for speeds up to 80 km/h with rapidly diminishing likelihood. This allows travel distances of 200 km per 5 h flight to be common, up to a maximum of 400 km, which is comparable to migration estimates for other songbirds (Hall-Karlsson and Fransson, 2008; Macdonald et al., 2015; Wright et al., 2018).
We also developed a land mask to restrict the locations of individuals to suitable habitats. We used a 1 km*1 km landcover database from Tuanmu and Jetz (2014) which classifies the globe into 12 categories based on a consensus from multiple remote-sensing sources. Since horned larks are obligate open-country birds that avoid forested areas, we used “herbaceous vegetation or grassland” and “barren” (often alpine) landcover categories as identified by a remote-sensing consensus of >25%. Agricultural fields are also potential non-breeding habitat (Beason, 1995), and therefore we included the category “cultivated or managed vegetation” which includes fallow fields and rangeland. Birds were restricted to stopping in these habitat types but could move freely between stopover sites over all other landcovers, including water.
We fit a group Estelle model incorporating the behavior model and land mask to estimate the location of stationary and movement periods with a multivariate normal error distribution. The model was trained using 1,000 initial iterations with a 20 iteration burn-in, followed by MCMC chains including 10 rounds of 300 iterations each. The final model was run with 2,000 iterations.
The average geolocator error based on location estimates from a known location—the breeding site—was 59 ± 56 (s.d.) km (range: 3–224 km) in latitude and 57 ± 50 km (1–185 km) in longitude. Similarly, the average 95% credible interval for point estimates of non-breeding stationary periods (unknown locations) was 58 km (range: 30–101 km) for latitude and 61 km (range: 34–122 km) for longitude. As a result, we defined the non-breeding season as beginning when an individual moved > 150 km from the breeding site in a consistent direction. Similarly, we considered estimated stopover locations to be distinct if they were separated by at least 150 km, otherwise they were grouped by calculating the centroid weighted by the number of days at each location. Location estimates indicating large latitudinal fluctuations 2 weeks prior-to and after an equinox were removed because at this time estimates of latitude can be inaccurate, although longitude is still relevant (Hill, 1994; Ekstrom, 2004). As a result, if a bird arrived or departed from a stationary point during an equinox period, the arrival and departure times were approximated based on longitudinal shifts and when the longitude stabilized to match the stationary site. Normally, estimates of departure and arrival dates are accurate to ±2 days. However, when estimating phenology during an equinox period based on longitudinal shifts, we assumed accuracy to be ±5 days. This inaccuracy predominately occurred when estimating arrival at prolonged stopovers during autumn migration.
Migration Terminology
Following location estimation, we calculated several migration traits that require definition:
Winter site—The non-breeding site farthest to the south and with the longest duration of stay.
Stopover—Any stationary period ≥ 3 days between the breeding and winter site. Due to location estimate error, stopovers represent general but distinct regions within which an individual may use several locations.
Spring staging area—Stopovers during northward migration where a bird remained stationary for >20 days. The length was chosen to be consistent with other examples in the literature (Renfrew et al., 2013; Gow et al., 2019) and to account for error in location estimates. We acknowledge that staging areas can be considered alternative wintering locations, similar to observations for Neotropical migrants like purple martin (Progne subis; Stutchbury et al., 2016) and veery (Catharus fuscescens; Heckscher et al., 2011). However, since they generally occurred on the migration route between the wintering and breeding site, it is reasonable to treat these locations as staging areas (Warnock, 2010; Bayly et al., 2018).
Absolute distance—Calculated as the linear distance between the breeding and winter site using the Vincenty ellipsoid method to account for curvature in the earth (Vincenty, 1975).
Route distance—Absolute distance does not account for route variation. Therefore, we also calculated the one-way sum of the linear distances between the breeding site, stopovers, and winter site for both southward and northward migration as a measure of realized distance.
Staging distance—The linear distance between the staging area (or winter site if an individual did not demonstrate staging behavior) and the breeding site. This allowed us to address proximity to the breeding site and spring phenology in a manner that is comparable among larks who did or did not exhibit staging behavior.
Migration speed—Measured as the route distance divided by the duration, and thus incorporates flight speed and stopover duration. Due to error in location estimates, distance and speed variables should be considered relative for comparison among individuals and not exact differences.
Statistical Analysis
To test sex-specific differences in spatial distribution and phenology, we used four separate one-way Analysis of Variance (ANOVA) tests with sex as the explanatory variable and absolute distance, staging distance, breeding site departure, or breeding site arrival date as the response variables. Separate ANOVAs were necessary because each response variable reflects different migration events that are distinct in time and space.
We fit simple linear models to address: (1) the influence of breeding success on departure date, (2) subsequent effects of departure date on autumn migration speed, stopover use, and non-breeding arrival dates, and (3) effects of using spring staging areas and breeding site arrival date on breeding success. Breeding success was calculated as the proportion of nests that successfully fledged offspring in a season. Total nesting attempts ranged from 1 to 3 but most individuals attempted two nests (12/17 birds; 5 males, 7 females). For autumn stopover behavior, we fit a logistic regression where an individual either did (1) or did not (0) remain stationary at a prolonged stopover for >20 days. Since several individuals migrated during part of the autumn equinox, identifying extended stationary periods was more reliable than the total number of stopovers. All other response variables were fit to a Gaussian distribution.
For all associations, we fit separate models for males and females. If neither were significant, we combined the data from both sexes and report the association from a model with sex as an additive term. We used this process because we were specifically interested in sex-specific differences in flexible migration behaviors. For effects of spring migration behavior on breeding parameters, we addressed two response variables: (1) nest success, and (2) the total number of fledglings in a season. An R2 was calculated for each model to indicate the strength of the association.
Results
Horned larks from an alpine breeding population in northern British Columbia, Canada wintered predominantly east of the Cascade mountain range in Washington and Oregon, USA, with one female overwintering in southeast Idaho (Figure 1). Most males and females exhibited “loop” migrations, traveling southward at higher elevations along the Coast Mountains to the northern Columbia Plateau region before dispersing farther south or east (Figure 1A). Northward migration tended to be at lower elevation through the Thompson-Okanagan and up through the Central Interior Plateau of British Columbia (Figure 1B). Individuals departed the breeding grounds within a span of 31 days from early August to early September and arrived at the wintering grounds over a span of 81 days (Table 1), subsequently spending an average of 170 days or 5.5 months at the wintering site (range: 56–242 days). Both sexes demonstrated faster spring than autumn migration speeds (Table 1).
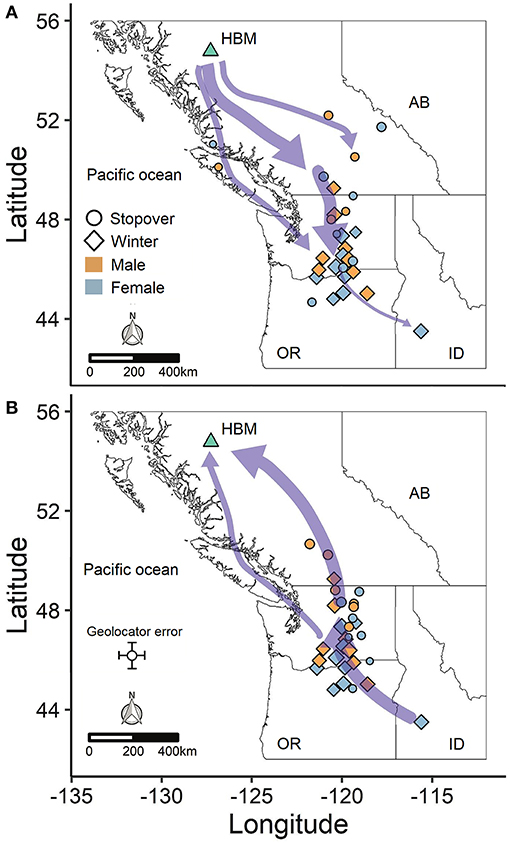
Figure 1. Migration routes between the breeding site (HBM) and winter sites for (A) autumn and (B) spring migration. The purple arrows are weighted to depict the most common migration routes. Points indicate winter sites (diamonds) and stopovers > 14 days long (circles) and are weighted by the number of days at each site. Stopovers < 14 days long are not shown for the sake of clarity. Each individual is represented by at least one winter site and up to one or two stopover sites (n = 17 birds).
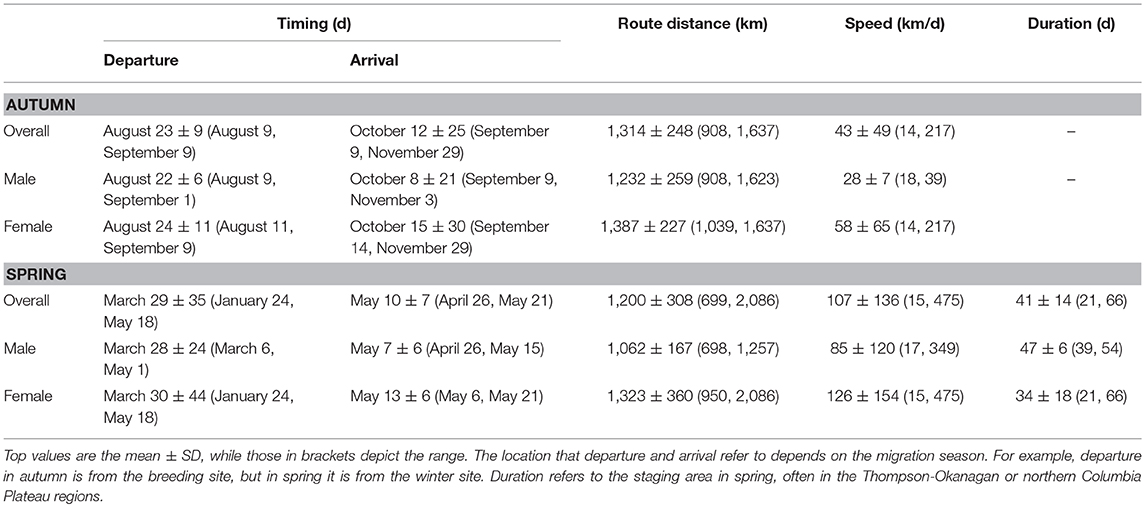
Table 1. Migration behavior for an alpine breeding population of horned larks in northern British Columbia for both autumn and spring migration.
The variation in arrival and duration of stay at winter sites followed from variable stopover use. During autumn migration, at least 11 individuals (~65%) appeared to remain stationary at a prolonged stopover site prior to continuing to the wintering site. However, because this period tended to occur at least partially within the fall equinox, we could not accurately estimate arrival date or exact duration of stay, although it was usually possible to estimate departure date. During spring migration, both males (5/8; 63%) and females (5/9; 56%) stopped at staging areas on their northward route, predominantly within the Thompson-Okanagan or northern Columbia Plateau regions (Figure 2). Individuals remained at staging areas for periods of 21−66 days (Table 1).
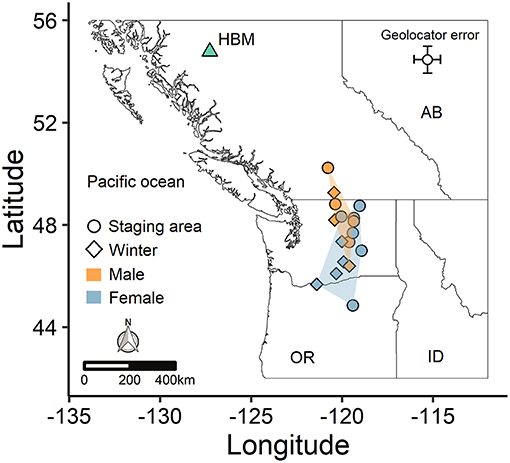
Figure 2. Spatial segregation of male and female non-breeding areas for an alpine population of horned lark. Points indicate the last prolonged stationary period for each individual prior to migrating back to the breeding site (HBM), based on whether the individual used a staging area (circle) or traveled straight from the winter site (diamond). Shaded regions depict the minimum convex polygons for each sex.
Spatial and Phenological Segregation of the Sexes
The mean winter coordinates for males (46.8 ± 1.5°N, −120.1 ± 0.9°W; mean ± SD) tended to be farther north than for females (45.8 ± 1.3°N, −119.6 ± 1.6°W), although the average distance between the breeding and wintering sites did not differ between sexes (Table 2). However, when considering the spring staging area, the spatial segregation between males (48.4 ± 1.3° N, −120.0 ± 0.6°W) and females (46.9 ± 1.3°N, −119.8 ± 0.9°W) was more distinct (Figure 2). Thus, males spent less time at their winter sites and significantly more time closer to the breeding site (Table 2). Autumn departure dates from the breeding grounds were similar for males and females (Table 2). During spring migration, males arrived at the breeding grounds an average of 6 days earlier than females (Table 2).
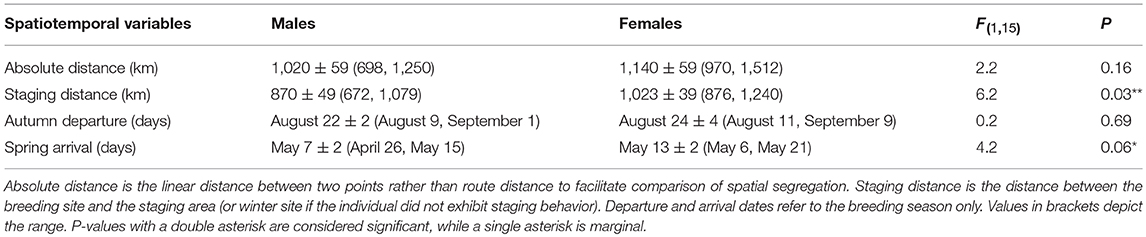
Table 2. Analysis of Variance (ANOVA) results for sex-specific differences in spatial distribution and phenology during the non-breeding season.
Effects of Reproductive Success on Autumn Migration Behavior
Females that had greater breeding success departed earlier from the breeding site (t = −2.4, P = 0.04, R2 = 0.46), while males did not exhibit an association (t = 0.1, P = 0.90, R2 = 0.00; Figure 3A). Departure date was positively associated with arrival at the first prolonged stationary site (i.e., stopover > 20 days or wintering site) for males (t = 3.2, P = 0.02, R2 = 0.63), but not for females (t = 1.2, P = 0.26, R2 = 0.17; Figure 3B). However, when just the winter site was considered, arrival was not associated with departure from the breeding site for either sex (t = −1.5, P = 0.16, R2 = 0.15; Figure 3B), likely due to variable stopover use. Later departing females increased migration speed to the first stopover site (t = 2.4, P = 0.05, R2 = 0.45; Figure 3C), and were less likely to exhibit prolonged stopover behavior (t = −5.3, P < 0.01, R2 = 0.82; Figure 3D). In contrast, regardless of departure date, males maintained migration speed (t = 0.2, P = 0.83, R2 = 0.01; Figure 3C) and stopover use (t = 0.0, P = 1.00, R2 = 0.00; Figure 3D).
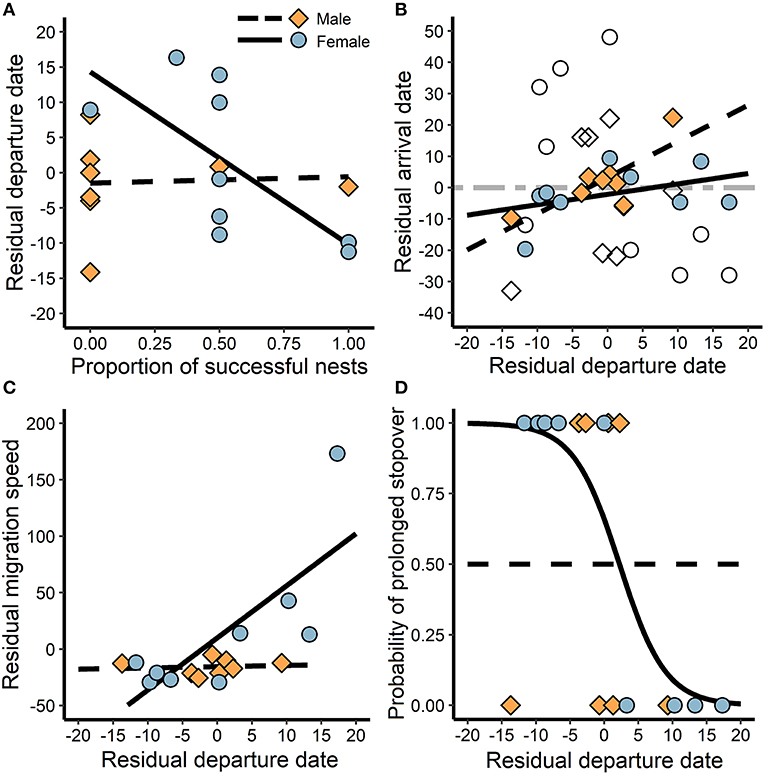
Figure 3. The association between breeding success and cascading migration behaviors during autumn migration. (A) The relationship between breeding success and timing of departure from the breeding site, and (B) the subsequent association between departure date and arrival at the first prolonged non-breeding site (black lines) and the winter site (dashed gray line). The gray line depicts the relationship for both males and females because there were no sex differences, but the empty points indicate males and females separately for comparison. Additionally, (C) late departing females increased migration speed to the first stopover, and (D) reduced use of prolonged stopovers following delayed departure. Residuals were calculated by subtracting from the mean such that positive is greater and negative is less than average.
Effects of Spring Migration Behavior on Breeding Success
While breeding season arrival date was positively associated with departure date from the last prolonged stationary site (i.e., staging area or wintering site) for both sexes (t = 4.6, P < 0.01, R2 = 0.69; Figure 4A), arrival date did not correlate with clutch initiation date (Pearson's product-moment correlation: rp = 0.32, t = 1.3, d.f. = 15, P = 0.21). However, larks that stopped at staging areas while traveling northward demonstrated greater breeding success (t = 2.7, P = 0.02, R2 = 0.39; Figure 4B) and consequently produced more fledglings than those that stopped farther south or migrated directly from the winter site (t = 2.4, P = 0.03, R2 = 0.30). On average, larks that stopped at staging areas farther north produced 1.8 more fledglings over the season (2.2 ± 0.6; mean + SE; n = 10) than individuals that remained farther south (0.4 ± 0.4; n = 7).
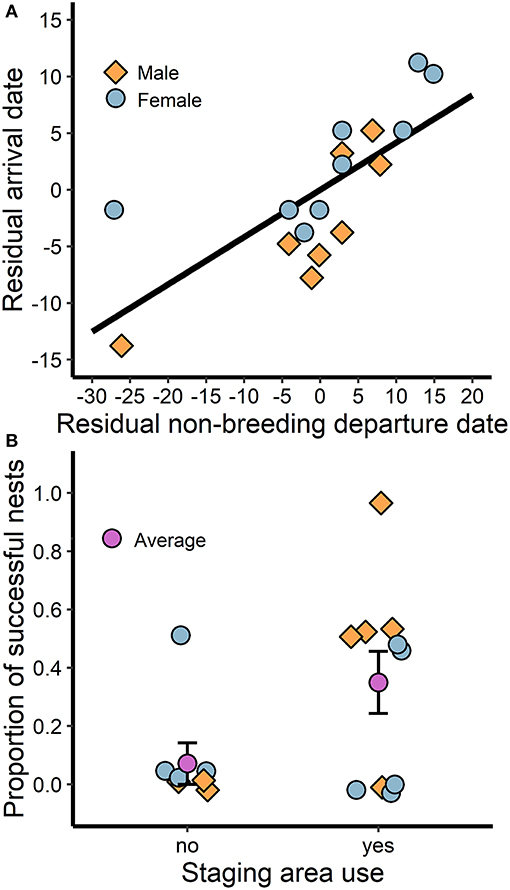
Figure 4. The association between spring migration behavior and breeding success: (A) individuals that depart the last stationary site (i.e., staging area or winter site) later arrive late to the breeding site, and (B) individuals that use staging areas in the Thompson-Okanagan or northern Columbia Plateau regions en route to the breeding site have greater breeding success. Residual date was calculated by subtracting from the mean such that positive values are delayed and negative values are advanced.
Discussion
Sex-Specific Differences in Spatial Distribution and Phenology
In an alpine breeding population of horned larks, males spent a significantly longer time farther north and closer to the breeding site than females. In contrast to expectations for a short-distance migrant, males arrived on the breeding grounds an average of 6 days earlier, demonstrating relatively minor protandry. Short-distance migrants often exhibit greater protandry (~2 weeks) compared to long-distance migrants (2–8 days; Tøttrup and Thorup, 2008; Briedis et al., 2019). Closer proximity to the breeding site should allow individuals to monitor environmental cues (Ouwehand and Both, 2017; Lehikoinen et al., 2019), to arrive as early as possible and gain territorial benefits (Reudink et al., 2009). However, the competitive advantage of arriving early is balanced with the cost of arriving too early when harsher weather may limit available resources and deplete energy reserves (Kokko et al., 2006; Coppack and Pulido, 2009). In a stochastic alpine habitat, the cost-to-benefit ratio of arriving early may be greater than in a less seasonal and more environmentally consistent low elevation habitat. At our alpine site, daytime temperatures often remain at or below 0°C throughout the first half of May, with frequent storms and high wind speeds (Camfield and Martin, 2009; Martin et al., 2017). Therefore, both males and females may experience stabilizing selection to arrive at similar times.
We did not find a relationship between breeding site arrival and clutch initiation date, which contradicts patterns observed in migrating songbirds breeding at low elevation (Norris et al., 2004; Woodworth et al., 2016). A selective advantage for early spring arrival is predicated on expected high-quality territory acquisition and subsequent benefits for breeding success (Morbey and Ydenberg, 2001). In the alpine, regardless of arrival date, ground-nests cannot be initiated until late May due to extensive snow cover. Even after onset of snowmelt, the higher probability of severe and cold early-season storms that lead to nest failure can limit the fitness benefits of breeding early (Martin et al., 2017). Alpine and arctic birds likely have greater flexibility to respond to proximate environmental conditions to advance or delay both breeding site arrival and clutch initiation date (Bears et al., 2009; Boelman et al., 2017). As a result, a relationship between arrival and clutch initiation date is likely moderated by variable environmental conditions, regardless of any potential competitive advantage to arriving early.
Cascading Effects of Breeding Success on Autumn Migration
Breeding effort and success showed sex-specific associations with autumn migration behavior, indicating potentially different mechanisms driving the timing of autumn migration, and subsequently different migration strategies. During autumn migration, breeding site departure date was delayed with reduced breeding success for females but not males. Since females will re-nest multiple times following failed attempts, this suggests greater energy investment during the breeding season can influence timing of autumn migration. Although similar evidence is limited, individuals that invest more in reproduction may be energetically constrained to depart later (Wojczulanis-Jakubas et al., 2013). For example, dunlin (Calidris alpina) delay breeding site departure following greater breeding success (Meissner, 2015), and wood thrush (Hylocichla mustelina) in low body condition at the end of the breeding season tend to remain farther north for longer, potentially because these individuals have delayed molting behavior (Stutchbury et al., 2011). Horned larks molt at the breeding site before departing (Beason, 1995), and thus molt could exacerbate departure delays for females considering the substantial energy investment required when late reproduction and molt coincide (Flinks et al., 2008; Borowske et al., 2017). Regardless of the mechanism, our results indicate that greater breeding effort has the potential to delay departure from the breeding site.
Males and females commonly differ in their capacity for flexibility across a wide range of behaviors (Nakagawa et al., 2007), including migration strategies (Both et al., 2016). We demonstrated sex-specific abilities to compensate for delayed departure during autumn migration, or to buffer cascading constraints so they did not carry over indefinitely (Conklin and Battley, 2012; Senner et al., 2014). Termed “reversible state effects,” conditions that influence energy reserves in one stage of the annual cycle can produce temporary constraints that influence subsequent stages but are corrected over time (Senner et al., 2015). While males maintained consistent stopover behavior, later departing females increased migration speed and decreased stopover use, providing some support for reversible state effects. Short-distance migrants likely have a greater capacity for flexible stopover behavior (Schmaljohann and Both, 2017), which can compensate for a late departure (Stutchbury et al., 2011). Speeding up migration implies an urgency to arrive at a destination and this selective pressure is thought to be greater in spring than autumn (Horton et al., 2016). It is unclear why female larks speed up autumn migration, but one possibility is that poor weather conditions at northern stopovers later in autumn may cause less territorial individuals, like females, to continue migrating south (Stutchbury et al., 2016; Schmaljohann et al., 2017).
Spring Staging Behavior
We observed exceptionally prolonged staging behavior during spring migration, with some individuals remaining at northern staging areas for up to 2 months. The traditional expectation for stopovers is that their duration should be minimized, such that birds stay just long enough to refuel (Alerstam et al., 2003). While evidence for staging in songbirds originally stemmed from long-distance migrants preparing to cross major landscape features (e.g., the Sahara; Arlt et al., 2015), recent observations indicate this behavior may be more prevalent in songbirds than once thought (Bayly et al., 2018). For example, bobolink Dolichonyx oryzivorus may stop for more than 20 days during both southward and northward migration (Renfrew et al., 2013), while rusty blackbirds Euphagus carolinus exhibited average stationary periods of 25.5 days (max = 62 days) prior to arriving at the breeding site (Wright et al., 2018). Interestingly, staging behavior is most commonly observed in capital breeders like snow goose Anser caerulescens when preparing to arrive at unpredictable, high latitude breeding sites (Bêty et al., 2004). While songbirds are income breeders, staging behavior may allow larks to improve fat reserves to respond to variable, early season conditions upon arriving in the alpine.
For larks, staging areas may be key components of the annual cycle (Pledger et al., 2009), particularly because individuals that exhibited staging behavior farther north demonstrated greater reproductive success. The use of a specific staging area may have intrinsic value that carries over to reproduction. For example, high-quality individuals may be able to move earlier to northern staging areas to maximize limited resources in a harsher environment and thus be in a better position to arrive at the breeding site at an optimal time to acquire high-quality territories and mates. This may explain why two males remained in the region for the entire non-breeding season. In support of this mechanism, black-and-white warblers Mniotilta varia that arrive early at stopovers close to the breeding grounds remain longer and accumulate more fat than later arriving birds (Paxton and Moore, 2017). Future research that addresses the habitat quality of staging areas, as well as, the body condition of individuals that remain at these sites for prolonged periods of time would help identify the drivers of this behavior in an alpine population of horned larks.
Conclusion
We report sex differences in spatial distribution during the non-breeding season and flexibility in migration behavior. Since our sample size is modest, with 17 individuals over 3 years, we acknowledge that our results, although intriguing, may not capture the full range of variability in certain parameters measured in this study (e.g., departure date, nest success). Nevertheless, the sex-specific patterns we observed indicate the potential importance of flexible migration behaviors in shaping individual life-history strategies and fitness, with implications for population dynamics. If individuals from one sex are more constrained to specific migration behaviors or non-breeding sites, they may be more susceptible to changing environmental and land-use conditions which may influence sex-biased mortality or dispersal rates. As a result, identifying critical non-breeding sites, as well as, within-population variation in how these locations are used is an important step in the conservation of declining open-country birds (Cohen et al., 2017). To this end, we observed striking spring staging behavior and its potential influence on subsequent breeding success, suggesting staging areas in the Thompson-Okanagan and Columbia Plateau may be critical components of the annual cycle. Staging behavior is difficult to recognize and poorly understood among songbirds owing to their small size and often greater dispersion across the landscape, but future studies like ours will improve our ability to identify staging areas and the factors influencing this behavior.
Data Availability
Raw geolocator data will be made available in the Movebank data repository. All other datasets are available upon request. The data supporting the conclusions of this manuscript will be made available by the authors, without undue reservation, to any qualified researcher.
Ethics Statement
All procedures and protocols for this study were approved by the University of British Columbia's Animal Care Committee (A15-0027) and are in accordance with the Canadian Council on Animal Care's national guidelines. All data were also collected under a Scientific Permit for Capture and Banding of Migratory Birds from Environment and Climate Change Canada (10365 DS and 10761 J).
Author Contributions
DdZ, EG, SW, and KM conceived the ideas and designed the methodology. DdZ collected and analyzed the data, and led writing of the manuscript. All authors contributed critically to the drafts and gave final approval for publication.
Funding
Funding for this research was provided to DdZ by the Northern Scientific Training Program, Werner and Hildegard Hesse Research Award (University of British Columbia), Northwest Scientific Association, American Ornithological Society, and Society of Canadian Ornithologists (Taverner Award), to EG by the Izaak Walton Killam Memorial Fund, to KM and DdZ by the Natural Sciences and Engineering Research Council of Canada and to KM and SW by Environment and Climate Change Canada.
Conflict of Interest Statement
The authors declare that the research was conducted in the absence of any commercial or financial relationships that could be construed as a potential conflict of interest.
Acknowledgments
We thank A. Sulemanji, D. Maucieri, S. Hudson, N. Morrel, T. Altamirano, N. Froese, N. Bennett, K. Hicks, and C. Rivas for their contributions to data collection. Special thanks to J. Fox and S. Lisovski for helpful feedback on geolocator application and analysis.
References
Alerstam, T., Hedenström, A., and Åkesson, S. (2003). Long-distance migration: evolution and determinants. Oikos 103, 247–260. doi: 10.1034/j.1600-0706.2003.12559.x
Arlt, D., Olsson, P., Fox, J. W., Low, M., and Pärt, T. (2015). Prolonged stopover duration characterises migration strategy and constraints of a long-distance migrant songbird. Anim. Migr. 2, 47–62. doi: 10.1515/ami-2015-0002
Bêty, J., Giroux, J. F., and Gauthier, G. (2004). Individual variation in timing of migration: causes and reproductive consequences in greater snow geese (Anser caerulescens atlanticus). Behav. Ecol. Sociobiol. 57, 1–8. doi: 10.1007/s00265-004-0840-3
Bayly, N. J., Rosenberg, K. V., Easton, W. E., Gomez, C., Carlisle, J., Ewert, D. N., et al. (2018). Major stopover regions and migratory bottlenecks for Nearctic-Neotropical landbirds within the Neotropics: a review. Bird Conserv. Inter. 28, 1–26. doi: 10.1017/S0959270917000296
Bears, H., Martin, K., and White, G. C. (2009). Breeding in high-elevation habitat results in shift to slower life-history strategy within a single species. J. Anim. Ecol. 78, 365–375. doi: 10.1111/j.1365-2656.2008.01491.x
Beason, R. C. (1995). “Horned lark (Eremophila alpestris),” in The Birds of North America, Vol. 195, eds A. Poole and F. Gill (Philadelphia, PA; Washington, DC: Academy of Natural Sciences/American Ornithologists' Union), 1–21. doi: 10.2173/bna.195
Beever, E. A., Hall, L. E., Varner, J., Loosen, A. E., Dunham, J. B., Gahl, M. K., et al. (2017). Behavioral flexibility as a mechanism for coping with climate change. Front. Ecol. Environ. 15, 299–308. doi: 10.1002/fee.1502
Boelman, N. T., Krause, J. S., Sweet, S. K., Chmura, H. E., Perez, J. H., Gough, L., et al. (2017). Extreme spring conditions in the Arctic delay spring phenology of long-distance migratory songbirds. Oecologia 185, 69–80. doi: 10.1007/s00442-017-3907-3
Borowske, A., Gjerdrum, C., and Elphick, C. (2017). Timing of migration and prebasic molt in tidal marsh sparrows with different breeding strategies: comparisons among sexes and species. Auk 134, 51–64. doi: 10.1642/AUK-16-116.1
Both, C., Bijlsma, R. G., and Ouwehand, J. (2016). Repeatability in spring arrival dates in pied flycatchers varies among years and sexes. Ardea 104, 3–22. doi: 10.5253/arde.v104i1.a1
Briedis, M., Bauer, S., Adamík, P., Alves, J. A., Costa, J. S., Emmenegger, T., et al. (2019). A full annual perspective on sex-biased migration timing in long-distance migratory birds. Proc. R. Soc. B 286, 2821–2829. doi: 10.1098/rspb.2018.2821
Briedis, M., Hahn, S., Gustafsson, L., Henshaw, I., Träff, J., Král, M., et al. (2016). Breeding latitude leads to different temporal but not spatial organization of the annual cycle in a long-distance migrant. J. Avian Biol. 47, 743–748. doi: 10.1111/jav.01002
Briedis, M., Krist, M., Král, M., Voigt, C. C., and Adamík, P. (2018). Linking events throughout the annual cycle in a migratory bird—wintering period buffers accumulation of carry-over effects. Behav. Ecol. Sociobiol. 72, 93–104. doi: 10.1007/s00265-018-2509-3
Camfield, A. F., and Martin, K. (2009). The influence of ambient temperature on Horned Lark incubation behaviour in an alpine environment. Behaviour 146, 1615–1633. doi: 10.1163/156853909X463335
Camfield, A. F., Pearson, S. F., and Martin, K. (2010). Life history variation between high and low elevation subspecies of horned larks Eremophila spp. J. Avian Biol. 41, 273–281. doi: 10.1111/j.1600-048X.2009.04816.x
Cannings, R. J., and Threlfall, W. (1981). Horned lark breeding biology at Cape St. Mary's, Newfoundland. Wilson Bull. 93, 519–530.
Cohen, E. B., Barrow, W. C. Jr, Buler, J. J., Deppe, J. L., Farnsworth, A., Marra, P. P., et al. (2017). How do en route events around the Gulf of Mexico influence migratory landbird populations? Condor 119, 327–343. doi: 10.1650/CONDOR-17-20.1
Conklin, J. R., and Battley, P. F. (2012). Carry-over effects and compensation: late arrival on non-breeding grounds affects wing moult but not plumage or schedules of departing bar-tailed godwits Limosa lapponica baueri. J. Avian Biol. 43, 252–263. doi: 10.1111/j.1600-048X.2012.05606.x
Coppack, T., and Pulido, F. (2009). Proximate control and adaptive potential of protandrous migration in birds. Integr. Comp. Biol. 49, 493–506. doi: 10.1093/icb/icp029
Cristol, D. A., Baker, M. B., and Carbone, C. (1999). Differential migration revisited: latitudinal segregation by age and sex class. Curr. Ornithol. 15, 33–88. doi: 10.1007/978-1-4757-4901-4_2
de Zwaan, D. R., Camfield, A. F., MacDonald, E. C., and Martin, K. (2019). Variation in offspring development is driven more by weather and maternal condition than predation risk. Func. Ecol. 33, 447–456. doi: 10.1111/1365-2435.13273
de Zwaan, D. R., Trefry, S. A., and Martin, K. (2018). Efficiency and fitness consequences of two trapping methods for recapturing ground-nesting songbirds. J. Field Ornithol. 89, 363–371. doi: 10.1111/jofo.12270
Ekstrom, P. A. (2004). “An advance in geolocation by light,” in Memoirs of the National Institute of Polar Research, ed Y. Naito (Tokyo: National Institute of Polar Research), 201–226.
Flinks, H., Helm, B., and Rothery, P. (2008). Plasticity of moult and breeding schedules in migratory European stonechats Saxicola rubicola. Ibis 150, 687–697. doi: 10.1111/j.1474-919X.2008.00833.x
Gauthreaux, S. A. Jr. (1978). “The ecological significance of behavioural dominance,” in Perspectives in Ethology Vol. 3: Social Behavior, eds P. P. G. Bateson and P. H. Kopfer (Boston, MA: Springer), 17–54. doi: 10.1007/978-1-4684-2901-5_2
Gienapp, P., and Bregnballe, T. (2012). Fitness consequences of timing of migration and breeding in cormorants. PLoS ONE 7:e46165. doi: 10.1371/journal.pone.0046165
Gilroy, J. J., Gill, J. A., Butchart, S. H., Jones, V. R., and Franco, A. M. (2016). Migratory diversity predicts population declines in birds. Ecol. Lett. 19, 308–317. doi: 10.1111/ele.12569
Gómez, C., Bayly, N. J., Norris, D. R., Mackenzie, S. A., Rosenberg, K. V., Taylor, P. D., et al. (2017). Fuel loads acquired at a stopover site influence the pace of intercontinental migration in a boreal songbird. Sci. Rep. 7, 3405–3415. doi: 10.1038/s41598-017-03503-4
Goullaud, E. L., de Zwaan, D. R., and Martin, K. (2018). Predation risk-induced adjustments in provisioning behavior for Horned Lark (Eremophila alpestris) in British Columbia. Wilson J. Ornithol. 130, 180–190. doi: 10.1676/16-150.1
Gow, E. A., Burke, L., Winkler, D. W., Knight, S. M., Bradley, D. W., Clark, R. G., et al. (2019). A range-wide domino effect and resetting of the annual cycle in a migratory songbird. Proc. R. Soc. B 286, 1916–1924. doi: 10.1098/rspb.2018.1916
Gow, E. A., and Wiebe, K. L. (2014). Males migrate farther than females in a differential migrant: an examination of the fasting endurance hypothesis. R. Soc. Open Sci. 1, 140346–140353. doi: 10.1098/rsos.140346
Hall-Karlsson, K. S. S., and Fransson, T. (2008). How far do birds fly during one migratory flight stage? Ring. Migr. 24, 95–100. doi: 10.1080/03078698.2008.9674381
Harrison, X. A., Blount, J. D., Inger, R., Norris, D. R., and Bearhop, S. (2011). Carry-over effects as drivers of fitness differences in animals. J. Anim. Ecol. 80, 4–18. doi: 10.1111/j.1365-2656.2010.01740.x
Heckscher, C. M., Taylor, S. M., Fox, J. W., and Afanasyev, V. (2011). Veery (Catharus fuscescens) wintering locations, migratory connectivity, and a revision of its winter range using geolocator technology. AUK 128, 531–542. doi: 10.1525/auk.2011.10280
Hill, C., and Braun, M. J. (2001). “Geolocation by light level – the next step: latitude,” in Electronic Tagging and Tracking in Marine Fisheries, eds J. R. Sibert and J. Nielsen (Dordrecht: Kluwer Academic Publishers), 315–330. doi: 10.1007/978-94-017-1402-0_17
Hill, R. D. (1994). “Theory of geolocation by light levels,” in Elephant Seals: Population Ecology, Behavior, and Physiology, eds L. Boeuf and R. M. Laws (Berkely, CA: University of California Press, 228–237.
Horton, K. G., Van Doren, B. M., Stepanian, P. M., Farnsworth, A., and Kelly, J. F. (2016). Seasonal differences in landbird migration strategies. AUK 133, 761–769. doi: 10.1642/AUK-16-105.1
Ketterson, E. D., and Nolan, V. Jr. (1976). Geographic variation and its climate correlates in the sex ratio of eastern-wintering dark-eyed juncos (Junco hyemalis hyemalis). Ecology 57, 679–693. doi: 10.2307/1936182
Knight, S. M., Bradley, D. W., Clark, R. G., Gow, E. A., Bélisle, M., Berzins, L. L., et al. (2018). Constructing and evaluating a continent-wide migratory songbird network across the annual cycle. Ecol. Monog. 88, 445–460. doi: 10.1002/ecm.1298
Kokko, H. (1999). Competition for early arrival in migratory birds. J. Anim. Ecol. 68, 940–950. doi: 10.1046/j.1365-2656.1999.00343.x
Kokko, H., Gunnarsson, T. G., Morrell, L. J., and Gill, J. A. (2006). Why do female migratory birds arrive later than males? J. Anim. Ecol. 75, 1293–1303. doi: 10.1111/j.1365-2656.2006.01151.x
Krause, J. S., Pérez, J. H., Chmura, H. E., Sweet, S. K., Meddle, S. L., Hunt, et al. (2016). The effect of extreme spring weather on body condition and stress physiology in Lapland longspurs and white-crowned sparrows breeding in the Arctic. Gen. Comp. Endocr. 237, 10–18. doi: 10.1016/j.ygcen.2016.07.015
Legagneux, P., Fast, P. L., Gauthier, G., and Bêty, J. (2012). Manipulating individual state during migration provides evidence for carry-over effects modulated by environmental conditions. Proc. R. Soc. B 279, 876–883. doi: 10.1098/rspb.2011.1351
Lehikoinen, A., Lindén, A., Karlsson, M., Andersson, A., Crewe, T. L., Dunn, E. H., et al. (2019). Phenology of the avian spring migratory passage in Europe and North America: asymmetric advancement in time and increase in duration. Ecol. Indic. 101, 985–991. doi: 10.1016/j.ecolind.2019.01.083
Lisovski, S., and Hahn, S. (2013). GeoLight - processing and analysing light-based geolocation in R. Methods Ecol. Evol. 3, 1055–1059. doi: 10.1111/j.2041-210X.2012.00248.x
Lisovski, S., Hewson, C. M., Klaassen, R. H., Korner-Nievergelt, F., Kristensen, M. W., and Hahn, S. (2012). Geolocation by light: accuracy and precision affected by environmental factors. Methods Ecol. Evol. 3, 603–612. doi: 10.1111/j.2041-210X.2012.00185.x
Macdonald, C. A., McKinnon, E. A., Gilchrist, H. G., and Love, O. P. (2015). Cold tolerance, and not earlier arrival on breeding grounds, explains why males winter further north in an Arctic-breeding songbird. J. Avian Biol. 46, 7–15. doi: 10.1111/jav.00689
MacDonald, E. C., Camfield, A. F., Jankowski, J. E., and Martin, K. (2013). Extended incubation recesses by alpine-breeding Horned Larks: a strategy for dealing with inclement weather? J. Field Ornithol. 84, 58–68. doi: 10.1111/jofo.12006
Marra, P. P., Cohen, E. B., Loss, S. R., Rutter, J. E., and Tonra, C. M. (2015). A call for full annual cycle research in animal ecology. Biol. Lett. 11, 552–555. doi: 10.1098/rsbl.2015.0552
Marra, P. P., Hobson, K. A., and Holmes, R. T. (1998). Linking winter and summer events in a migratory bird by using stable-carbon isotopes. Science 282, 1884–1886. doi: 10.1126/science.282.5395.1884
Martin, K., Wilson, S., MacDonald, E. C., Camfield, A. F., Martin, M., and Trefry, S. A. (2017). Effects of severe weather on reproduction for sympatric songbirds in an alpine environment: interactions of climate extremes influence nesting success. AUK 134, 696–709. doi: 10.1642/AUK-16-271.1
McKinnon, E. A., Fraser, K. C., Stanley, C. Q., and Stutchbury, B. J. M. (2014). Tracking from the Tropics reveals behaviour of juvenile songbirds on their first spring migration. PLoS ONE 9:e105605. doi: 10.1371/journal.pone.0105605
McKinnon, E. A., and Love, O. P. (2018). Ten years tracking the migrations of small landbirds: lessons learned in the golden age of bio-logging. AUK 135, 834–856. doi: 10.1642/AUK-17-202.1
Meissner, W. (2015). Immature dunlins Calidris alpina migrate towards wintering grounds later than adults in years of low breeding success. J. Ornithol. 156, 47–53. doi: 10.1007/s10336-014-1132-y
Møller, A. P. (2004). Protandry, sexual selection and climate change. Glob. Change Biol. 10, 2028–2035. doi: 10.1111/j.1365-2486.2004.00874.x
Møller, A. P., Rubolini, D., and Lehikoinen, E. (2008). Populations of migratory bird species that did not show a phenological response to climate change are declining. PNAS 105, 16195–16200. doi: 10.1073/pnas.0803825105
Morbey, Y. E., and Ydenberg, R. C. (2001). Protandrous arrival timing to breeding areas: a review. Ecol. Lett. 4, 663–673. doi: 10.1046/j.1461-0248.2001.00265.x
Morganti, M., Mellone, U., Bogliani, G., Saino, N., Ferri, A., Spina, F., et al. (2011). Flexible tuning of departure decisions in response to weather in black redstarts Phoenicurus ochruros migrating across the Mediterranean Sea. J. Avian Biol. 42, 323–334. doi: 10.1111/j.1600-048X.2011.05331.x
Nakagawa, S., Gillespie, D. O. S., Hatchwell, B. J., and Burke, T. (2007). Predictable males and unpredictable females: sex difference in repeatability of parental care in a wild bird population. J. Evol. Biol. 20, 1674–1681. doi: 10.1111/j.1420-9101.2007.01403.x
Norris, D. R. (2005). Carry-over effects and habitat quality in migratory populations. Oikos 109, 178–186. doi: 10.1111/j.0030-1299.2005.13671.x
Norris, D. R., Marra, P. P., Kyser, T. K., Sherry, T. W., and Ratcliffe, L. M. (2004). Tropical winter habitat limits reproductive success on the temperate breeding grounds in a migratory bird. Proc. R. Soc. B 271, 59–64. doi: 10.1098/rspb.2003.2569
Ouwehand, J., and Both, C. (2017). African departure rather than migration speed determines variation in spring arrival in pied flycatchers. J. Anim. Ecol. 86, 88–97. doi: 10.1111/1365-2656.12599
Paxton, K. L., and Moore, F. R. (2017). Connecting the dots: stopover strategies of an intercontinental migratory songbird in the context of the annual cycle. Ecol. Evol. 7, 6716–6728. doi: 10.1002/ece3.3227
Piersma, T. (1987). Hop, skip or jump? Constraints on migration of Arctic waders by feeding, fattening and flight speed. Limosa 60, 185–194.
Piersma, T., and Drent, J. (2003). Phenotypic flexibility and the evolution of organismal design. Trends Ecol. Evol. 18, 228–233. doi: 10.1016/S0169-5347(03)00036-3
Pledger, S., Efford, M., Pollock, K., Collazo, J., and Lyons, J. (2009). “Stopover duration analysis with departure probability dependent on unknown time since arrival,” in Modeling Demographic Processes in Marked Populations, eds D. L. Thomson, E. G. Cooch, and M. J. Conroy (New York, NY: Springer), 349–363. doi: 10.1007/978-0-387-78151-8_15
R Core Team (2018). R: A Language and Environment for Statistical Computing. Vienna: R Foundation for Statistical Computing.
Rappole, J. H., and Tipton, A. (1990). New harness design for attachment of radio transmitters to small passerines. J. Field Ornithol. 62, 335–337.
Renfrew, R. B., Kim, D., Perlut, N., Smith, J., Fox, J., and Marra, P. P. (2013). Phenological matching across hemispheres in a long-distance migratory bird. Divers. Distrib. 19, 1008–1019. doi: 10.1111/ddi.12080
Reudink, M. W., Marra, P. P., Kyser, T. K., Boag, P. T., Langin, K. M., and Ratcliffe, L. M. (2009). Non-breeding season events influence sexual selection in a long-distance migratory bird. Proc. R. Soc. B 276, 1619–1626. doi: 10.1098/rspb.2008.1452
Roberto-Charron, A. (2018). Investigating methods of geolocator analysis in songbird migration research and their application to the study of a threatened, neotropical songbird (Master's thesis). University of Manitoba, Winnipeg, MA, Canada.
Runge, C. A., Martin, T. G., Possingham, H. P., Willis, S. G., and Fuller, R. A. (2014). Conserving mobile species. Front. Evol. Environ. 12, 395–402. doi: 10.1890/130237
Saino, N., Ambrosini, R., Caprioli, M., Romano, A., Romano, M., Rubolini, D., et al. (2017). Sex-dependent carry-over effects on timing of reproduction and fecundity of a migratory bird. J. Anim. Ecol. 86, 239–249. doi: 10.1111/1365-2656.12625
Saino, N., Ambrosini, R., Rubolini, D., von Hardenberg, J., Provenzale, A., Hüppop, K., et al. (2010). Climate warming, ecological mismatch at arrival and population decline in migratory birds. Proc. R. Soc. B 278, 835–842. doi: 10.1098/rspb.2010.1778
Schmaljohann, H., and Both, C. (2017). The limits of modifying migration speed to adjust to climate change. Nat. Clim. Change 7, 573–576. doi: 10.1038/nclimate3336
Schmaljohann, H., Lisovski, S., and Bairlein, F. (2017). Flexible reaction norms to environmental variables along the migration route and the significance of stopover duration for total speed of migration in a songbird migrant. Front. Zool. 14:17. doi: 10.1186/s12983-017-0203-3
Senner, N. R., Conklin, J. R., and Piersma, T. (2015). An ontogenetic perspective on individual differences. Proc. R. Soc. B 282, 1050–1058. doi: 10.1098/rspb.2015.1050
Senner, N. R., Hochachka, W. M., Fox, J. W., and Afanasyev, V. (2014). An exception to the rule: carry-over effects do not accumulate in a long-distance migratory bird. PLoS ONE 9:e86588. doi: 10.1371/journal.pone.0086588
Shaw, A. K. (2016). Drivers of animal migration and implications in changing environments. Evol. Ecol. 30, 991–1007. doi: 10.1007/s10682-016-9860-5
Smith, R. J., and Moore, F. R. (2005). Arrival timing and seasonal reproductive performance in a long-distance migratory landbird. Behav. Ecol. Sociobiol. 57, 231–239. doi: 10.1007/s00265-004-0855-9
Stutchbury, B. J., Gow, E. A., Done, T., MacPherson, M., Fox, J. W., and Afanasyev, V. (2011). Effects of post-breeding moult and energetic condition on timing of songbird migration into the tropics. Proc. R. Soc. B 278, 131–137. doi: 10.1098/rspb.2010.1220
Stutchbury, B. J., Siddiqui, R., Applegate, K., Hvenegaard, G. T., Mammenga, P., Mickle, N., et al. (2016). Ecological causes and consequences of intratropical migration in temperate-breeding migratory birds. Am. Nat. 188, S28–S40. doi: 10.1086/687531
Sullivan, B. L., Wood, C. L., Iliff, M. J., Bonney, R. E., Fink, D., and Kelling, S. (2009). eBird: a citizen-based bird observation network in the biological sciences. Biol. Conserv. 142, 2282–2292. doi: 10.1016/j.biocon.2009.05.006
Sumner, M. D., Wotherspoon, S. J., and Hindell, M. A. (2009). Bayesian estimation of animal movement from archival and satellite tags. PLoS ONE 4:e7324. doi: 10.1371/journal.pone.0007324
Tøttrup, A. P., Klaassen, R. H. G., Strandberg, R., Thorup, K., Kristensen, M. W., Jørgensen, P. S., et al. (2012). The annual cycle of a transequatorial Eurasian–African passerine migrant: different spatio-temporal strategies for autumn and spring migration. Proc. R. Soc. B 279, 1008–1016. doi: 10.1098/rspb.2011.1323
Tøttrup, A. P., and Thorup, K. (2008). Sex-differentiated migration patterns, protandry and phenology in North European songbird populations. J. Ornithol. 149, 161–167. doi: 10.1007/s10336-007-0254-x
Tuanmu, M.-N., and Jetz, W. (2014). A global 1-km consensus land-cover product for biodiversity and ecosystem modeling. Glob. Ecol. Biogeogr. 23, 1031–1045. doi: 10.1111/geb.12182
Usui, T., Butchart, S. H. M., and Phillimore, A. B. (2017). Temporal shifts and temperature sensitivity of avian spring migratory phenology: a phylogenetic meta-analysis. J. Anim. Ecol. 86, 250–261. doi: 10.1111/1365-2656.12612
van Wijk, R. E., Schaub, M., and Bauer, S. (2017). Dependencies in the timing of activities weaken over the annual cycle in a long-distance migratory bird. Behav. Ecol. Sociobiol. 71, 73–80. doi: 10.1007/s00265-017-2305-5
Vincenty, T. (1975). Direct and inverse solutions of geodesics on the ellipsoid with application of nested equations. Surv. Rev. 23, 88–93. doi: 10.1179/sre.1975.23.176.88
Warnock, N. (2010). Stopping vs. staging: the difference between a hop and a jump. J. Avian Biol. 41, 621–626. doi: 10.1111/j.1600-048X.2010.05155.x
Webster, M. S., Marra, P. P., Haig, S. M., Bensch, S., and Holmes, R. T. (2002). Links between worlds: unraveling migratory connectivity. TREE 17, 76–83. doi: 10.1016/S0169-5347(01)02380-1
Wilson, S., Smith, A. C., and Naujokaitis-Lewis, I. (2018). Opposing responses to drought shape population dynamics of declining grassland birds. Divers. Distrib. 24, 1687–1698. doi: 10.1111/ddi.12811
Wojczulanis-Jakubas, K., Jakubas, D., Foucher, J., Dziarska-Pałac, J., and Dugué, H. (2013). Differential autumn migration of the aquatic warbler Acrocephalus paludicola. Naturwissenschaften 100, 1095–1098. doi: 10.1007/s00114-013-1108-4
Woodworth, B. K., Newman, A. E. M., Turbek, S. P., Dossman, B. C., Hobson, K. A., Wassenaar, L. I., et al. (2016). Differential migration and the link between winter latitude, timing of migration, and breeding in a songbird. Oecologia 181, 413–422. doi: 10.1007/s00442-015-3527-8
Keywords: alpine, flexible life-history, Eremophila alpestris, light-level geolocation, phenology, plasticity, protandry, stopover ecology
Citation: de Zwaan DR, Wilson S, Gow EA and Martin K (2019) Sex-Specific Spatiotemporal Variation and Carry-Over Effects in a Migratory Alpine Songbird. Front. Ecol. Evol. 7:285. doi: 10.3389/fevo.2019.00285
Received: 27 March 2019; Accepted: 15 July 2019;
Published: 31 July 2019.
Edited by:
Nathan R. Senner, University of South Carolina, United StatesReviewed by:
Rien Van Wijk, Van Wijk Eco Research, DenmarkKristina Paxton, University of Hawaii at Hilo, United States
Copyright © 2019 de Zwaan, Wilson, Gow and Martin. This is an open-access article distributed under the terms of the Creative Commons Attribution License (CC BY). The use, distribution or reproduction in other forums is permitted, provided the original author(s) and the copyright owner(s) are credited and that the original publication in this journal is cited, in accordance with accepted academic practice. No use, distribution or reproduction is permitted which does not comply with these terms.
*Correspondence: Devin R. de Zwaan, ZHJkZXp3YWFuQGdtYWlsLmNvbQ==