- 1Department of Biology, Washington University in St. Louis, St. Louis, MO, United States
- 2Environmental Studies Program, Dartmouth College, Hanover, NH, United States
- 3Institute of Arctic Studies, Dartmouth College, Hanover, NH, United States
- 4Canadian Forest Service—Atlantic Forestry Centre, Corner Brook, NL, Canada
- 5Arctic Research Centre, Aarhus University, Aarhus, Denmark
- 6Department of Wildlife, Fish, and Conservation Biology, University of California, Davis, Davis, CA, United States
- 7Department of Bioscience Kalø, Aarhus University, Aarhus, Denmark
Parasitoids can affect host population dynamics with community-level consequences. In the Arctic, a high diversity of parasitoids relative to potential hosts suggests that parasitoids may exert strong selection pressure on arthropods, but the extent to which these interspecific linkages drive arthropod population dynamics remains unclear. Wolf spiders are dominant and ecologically important arctic predators that experience high rates of egg sac parasitism by wasps. We investigated potential changes in egg sac parasitism rates at two rapidly warming sites in Greenland: a high-arctic site (18 years of data, 1,088 egg sacs) and a low-arctic site (5 years of data, 538 egg sacs). While up to 13% of egg sacs were parasitized annually in the low-arctic site, we found no evidence of it at the high-arctic site despite the presence of congeneric parasitoid species at both locations. The surprising lack of parasitism in the north suggests that populations of this widespread spider species have different eco-evolutionary histories and may respond differentially to climate change.
Introduction
Host-parasite interactions may play a particularly important role in food web dynamics in the Arctic, where arthropod communities exhibit a high diversity of parasitoids relative to overall arthropod diversity (Stahlhut et al., 2013). Parasite impacts on arthropod hosts may even increase in the future, because parasitoids are disproportionately benefitting from rapidly warming temperatures relative to other arthropod groups (Koltz et al., 2018c). Unfortunately, while vertebrate host-parasite dynamics are relatively well-described in the Arctic (e.g., Moerschel, 1999; Hagemoen and Reimers, 2002; Kutz et al., 2002), little is currently known about parasitism among arctic arthropods (Kukal and Kevan, 1987; Bowden and Buddle, 2012a, but see Avery and Post, 2013; Ernst et al., 2016). Addressing this important gap in our knowledge of arctic natural history is critical because arthropods represent the most biodiverse group of multi-cellular organisms in this region, and as herbivores, detritivores, pollinators, and predators, arthropods play important roles in maintaining the health and functioning of arctic ecosystems (Høye and Culler, 2018). Here, we compare the strength of host-parasite interactions in one of the most abundant arctic arthropod groups for two sites in Greenland.
Wolf spiders inhabit nearly all terrestrial ecosystems and are dominant predators in the Arctic (Wyant et al., 2011; Bowden et al., 2018; Koltz et al., 2018a). They commonly prey upon decomposers and thereby indirectly affect decomposition rates and nutrient cycling in the tundra (Koltz et al., 2018b) and elsewhere (Lawrence and Wise, 2000; Lensing and Wise, 2006). Additionally, wolf spiders themselves are preyed upon by a variety of vertebrates (e.g., birds, Wirta et al., 2015) and parasitic wasps, including egg predators and egg parasitoids which target their egg sacs (both generally referred to here as parasitoids; Bowden and Buddle, 2012a). Female wolf spiders display maternal care by tethering their egg sacs to their abdomens for one to several weeks. During this incubation time, eggs develop into juvenile spiders and ultimately leave the egg sac. Egg sacs are conspicuous, being blue-gray or silver in color and large compared to a female's body (Figure 1A). Given the vulnerability of exposed egg sacs and the near global distribution and high local abundances of wolf spiders, it is not surprising that egg sac parasitism is commonly observed in temperate (Edgar, 1971; Cobb and Cobb, 2004) and arctic ecosystems (Bowden and Buddle, 2012a). In some areas of the Arctic, wasps from the genus Gelis, whose juveniles ultimately consume or destroy the entire contents of egg sacs, are found in as many as half of all sampled wolf spider egg sacs (Bowden and Buddle, 2012a). Such high rates of parasitism could impose major selective pressures on the phenology, physiology, and behavior (e.g., Godfray et al., 1994; Fellowes and Godfray, 2000; Low et al., 2014) of wolf spiders, with potential consequences for their population dynamics and ecosystem functioning.
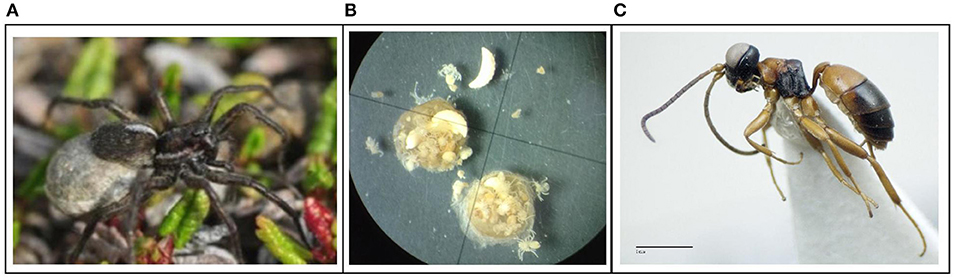
Figure 1. Egg sac parasitism of wolf spiders by parasitoid wasps is common in arctic and temperate ecosystems, including in SW Greenland, where populations of P. glacialis are parasitized by the wasp G. micrurus. (A) Female P. glacialis with egg sac; (B) Egg sac contents with a mix of wolf spider eggs and parasitoid larvae; (C) adult parasitoid G. micrurus. Photo credits: (A) Oskar L.P. Hansen; (B) Lauren E. Culler; (C) Ika Osterblad, Station Linné/Swedish Museum of Natural History, License: CreativeCommons Attribution, Non-commercial ShareAlike (CC BY-NC-SA 3.0).
Wolf spiders in the Arctic are becoming larger as a consequence of the longer growing seasons that have resulted from recent climate change (Høye et al., 2009). Because female spider body size is positively associated with the number of eggs per egg sac (Bowden and Buddle, 2012b), larger female spider bodies are expected to result in higher fecundity (Marshall and Gittleman, 1994) and potentially higher adult wolf spider abundances. Similarly, access to more eggs and/or reproductive females should presumably favor parasitoid wasps that feed upon wolf spider eggs, but the extent to which parasitoid behavior and abundances are being affected by changes in their typical prey are currently unknown.
We investigated the spatial and long-term temporal variability of egg sac parasitism in the dominant species of wolf spider across most of Greenland, Pardosa glacialis (Lycosidae) (Høye and Hammel, 2010; Bowden et al., 2018) at two sites that are warming rapidly under climate change. Specifically, we quantified parasitism using museum samples of wolf spider egg sacs that were collected over 18 years between 1996 and 2014 in Zackenberg, Greenland, a High-Arctic site (Meltofte and Rasch, 2008). Additionally, in 5 years between 2010 and 2017, we assessed parasitism rates on P. glacialis egg sacs in Kangerlussuaq, Greenland, a low-arctic site (Post, 2013). We affirmed the identities of parasitoid species by using DNA barcoding methods on a portion of parasitized egg sacs. We also measured the body sizes of a subset of egg sac – carrying female spiders to test whether parasitic wasps preferentially parasitize egg sacs produced by larger individuals (Bowden and Buddle, 2012a). Prior studies have shown that the abundance of parasitoid wasps in Zackenberg is higher in years with warmer summers (Koltz et al., 2018c). Given that body sizes, and presumably fecundity, of wolf spiders are increasing at Zackenberg as well (Høye et al., 2009), we predicted a general increase in parasitism rates over our 18-year study period. Additionally, we predicted that overall parasitism rates should be higher at Kangerlussuaq than at Zackenberg because growing seasons and hence, potential temporal overlap in host and parasitoid phenology are longer at Kangerlussuaq.
Materials and Methods
Study Sites
We sampled female arctic wolf spiders (Pardosa glacialis) and their egg sacs from two locations in Greenland (Figure 2). Samples from the first site were collected near the Zackenberg Research Station (74.28°N 20.34°W), which is located in Northeast Greenland in the high-arctic and is at the most northern range of P. glacialis (Dondale and Redner, 1990). The second site is an inland, mountainous tundra system in West Greenland near Kangerlussuaq (67.11°N 50.37°W) and is considered low-arctic (Bliss, 1981). Both sites are characterized by continental climates with cold winters and generally dry conditions, although overall climatic conditions are harsher at Zackenberg. Over the study period, the average temperature in July at Zackenberg was 6.5°C, whereas the average July temperature in Kangerlussuaq was 13.2°C (Figure S1). There are general warming trends of similar magnitude at Zackenberg and Kangerlussuaq (Zackenberg: +9.0 summed degree days/year between 1996 and 2017; Kangerlussuaq: +4.6 summed degree days/year between 1996 and 2013; Figure S1). P. glacialis is the only wolf spider species present in the samples collected at Zackenberg and is the most commonly collected species in Kangerlussuaq (In 2017 at Kangerlussuaq, 89% of all wolf spiders collected were P. glacialis, 11% were Arctosa insignita and <1% were Pardosa groenlandica, L. Culler unpublished data).
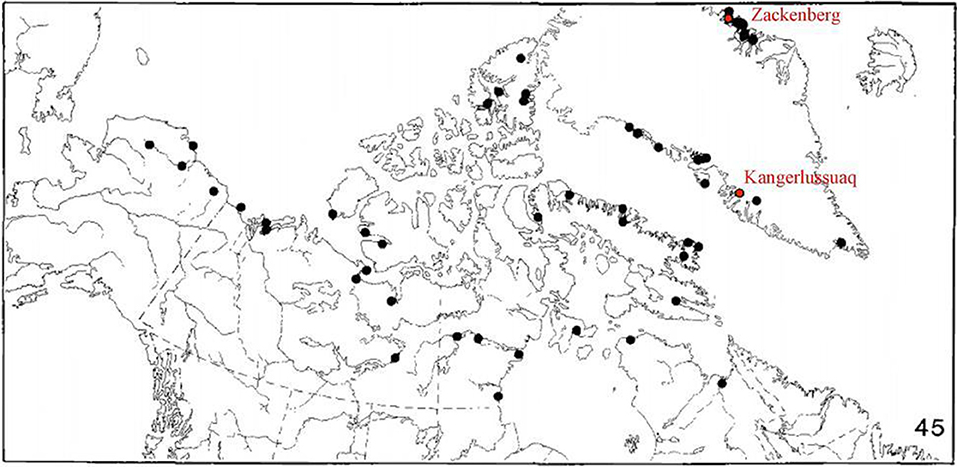
Figure 2. Map of the distribution of the arctic wolf spider Pardosa glacialis (Figure modified and reproduced with permission from Dondale and Redner, 1990). Egg sacs of P. glacialis were sampled and inspected for evidence of parasitism from Zackenberg (74.47°N 20.57°W), a high-arctic site in NE Greenland, and Kangerlussuaq (67.11°N 50.37°W) in low-arctic W Greenland.
Wolf spider egg sacs from Zackenberg were extracted from samples of ground-dwelling arthropods that were collected using pitfall traps from late May through at least the end of August from 1996 to 2014 (excluding 2010) as part of the BioBasis Monitoring Programme (Jensen et al., 2013). This sampling period includes almost the entire active period for both wolf spiders and potential parasitoid wasps; Table S1 contains specific information on sampling dates and the number of trapping days per year. Sampling plots were located in three different habitat types: mesic heath, arid heath, and wet fen (Høye and Forchhammer, 2008). Pitfall traps were 10 cm in diameter, yellow, and 1/3 filled with water and a few drops of detergent. Traps were emptied once per week. All captured specimens were stored in 70% ethanol. Samples were subsequently sorted and counted by technicians from the Department of Bioscience at Aarhus University, Denmark and spiders were identified and measured by J.J.B.
We collected spiders in Kangerlussuaq in 2010, 2011, 2012, 2016, and 2017. In 2010-2012, sampling occurred from May 30 through July 21. During these 3 years, twenty pitfall traps were deployed in mixed dwarf shrub / graminoid tundra habitat at each of six trapping sites (Table S2) that were evenly distributed between north- and south-facing slopes. Of the 20 pitfall traps at each site, 10 were located within a large herbivore exclosure treatment and the other 10 were outside the treatment in open tundra. In 2011 and 2012, additional sticky traps were deployed within 10 meters from the established pitfall sampling plots. The contents of all traps were collected every 24–48 h, preserved in 70% ethanol, and sorted at Pennsylvania State University by Michael Avery before further processing. Sampling in Kangerlussuaq in 2016 and 2017 was part of two studies documenting the abundance and composition of ground-dwelling arthropods in habitats of varying vegetation cover and soil moisture (Table S3). Sampling was conducted over approximately 2.5 weeks during the peak of the summer season in 2016 (30 June to 16 July) and during a longer period of 9 weeks in 2017 (22 May to 25 July). In 2016, 72 pitfall traps were evenly distributed among dwarf shrub and unvegetated tundra at three distinct sites (total trap days = 1,152; Table 1, Table S3). In 2017, 144 pitfall traps were distributed among dwarf shrub, dry graminoid, wet graminoid, and unvegetated tundra at eight distinct sites (total trap days = 3,117; Table 1, Table S3). In each year, sample contents were collected every 4–5 days and preserved in 70% ethanol for later processing at Dartmouth College. Although these samples do not capture the entirety of the growing season, in combination with the sampling from 2010 to 2012, they provide the best information available on wolf spider phenology and potential overlap with parasitoids in the Kangerlussuaq area.
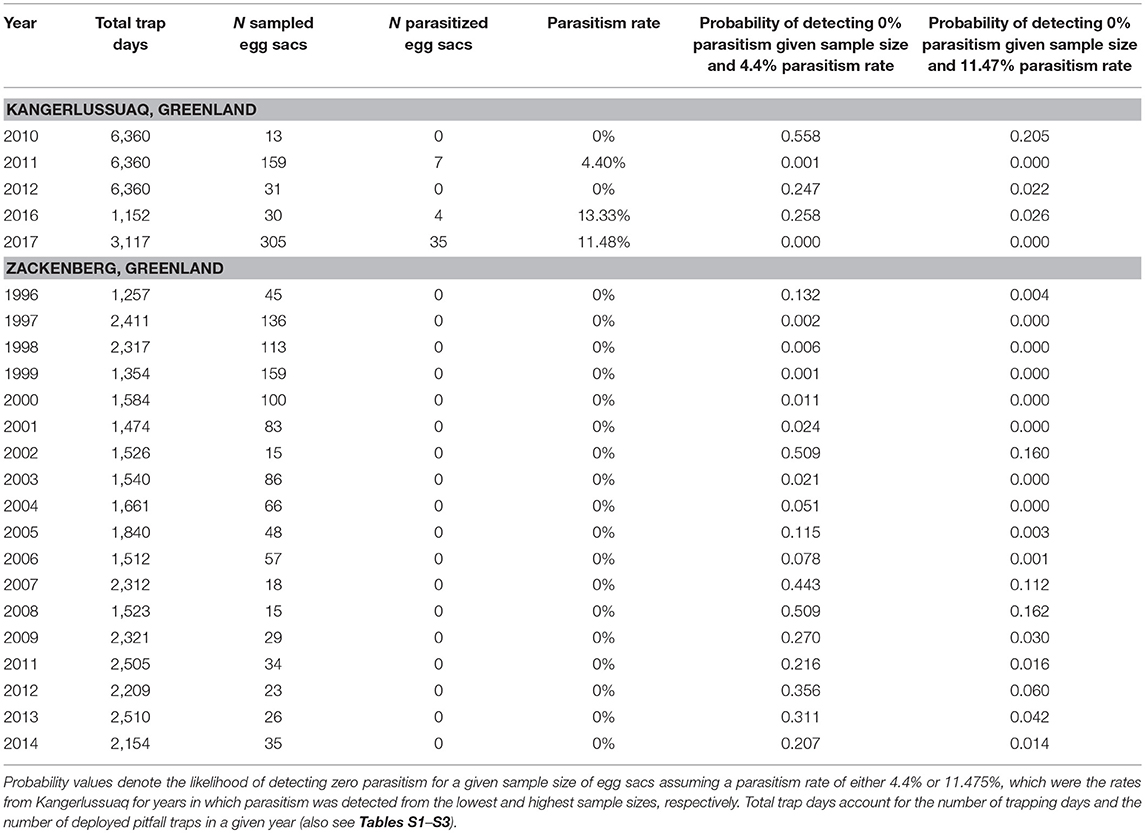
Table 1. Number of sampled wolf spider (Pardosa glacialis) egg sacs and egg sac parasitism rates for each study year from the Greenland sites of Kangerlussuaq (southern site) and Zackenberg (northern site).
Determining Egg Sac Parasitism Rates
We inspected a total of 1,088 wolf spider egg sacs from Zackenberg (high-arctic site) and 538 egg sacs from Kangerlussuaq (low-arctic site) for evidence of parasitism (Table 1). To investigate the extent to which sample sizes may have compromised our ability to detect egg sac parasitism events in certain sites and years, we evaluated the likelihood of observing a given number of events under different assumptions of parasitism rates. Specifically, we stochastically simulated 100,000 samples from a population with either 4.4 or 11.5% parasitism rates (i.e., the rates of parasitism observed in the southern site in years in which parasitism was detected and sampling effort was, respectively, smallest and largest) and compared the resulting distribution with the observed value.
Body Size as a Predictor of Egg Sac Parasitism
We used binary logistic regression models to test whether the egg sacs of larger females have higher probabilities of being parasitized. The body size of adult female P. glacialis that were clearly identified as egg sac carriers was quantified by measuring carapace width (Hagstrum, 1971) using digital calipers (Diesella, Kolding, Denmark). All egg-sac carrying adult females from Kangerlussuaq were measured in samples from 2010 to 2012 (N = 203). From Zackenberg, we were only able to measure female body sizes for 292 of the 1,088 samples, as egg sacs were frequently separated from the adult females. Sampling year and date at which the egg sacs were obtained were also included as predictors in our models. All statistical analyses were performed in RCoreTeam (2017).
Identification of Egg Sac Parasitoids
We submitted the parasitoid tissue (either eggs or larvae) from seven parasitized egg sacs that were collected from Kangerlussuaq in 2017 to LifeScanner (http://lifescanner.net/) for sequence analysis. LifeScanner is a DNA barcoding service that DNA barcodes user-provided samples using standardized sampling kits, a mobile app, and standardized laboratory workflow. The project is based at the Centre for Biodiversity Genomics (http://biodiversitygenomics.net/) at the University of Guelph; Taxonomic identifications are generated through the Barcode of Life Data (BOLD) Systems ID Engine (Ratnasingham and Hebert, 2007).
Results
At Kangerlussuaq, we found evidence of egg sac parasitism in 3 of the 5 sampling years. Rates of egg sac parasitism varied between 0 and 13.3% from these collection years (Table 1). In 2017, the year with the largest sample size (N = 305), parasitism was observed in 11.5% of all collected egg sacs (Table 1). Incidences of egg sac parasitism were most commonly detected in wet graminoid habitat at Kangerlussuaq (Table S4). While we did not find evidence of parasitism in egg sacs from Kangerlussuaq in 2010 or 2012, our stochastic simulations suggest that these absences are likely to be a product of low sample sizes in those years (Table 1). Results from the binomial generalized linear model indicated that incidences of egg sac parasitism at Kangerlussuaq were not significantly related to female body size, sampling date, or sampling year (all p > 0.087; Table S5).
The majority of parasitized egg sacs from Kangerlussuaq were found in mid-July. However, we also detected parasitism as early as June 21 in 1 year (Table S4). Each parasitized egg sac contained between 1 and 10 wasp eggs, larvae, or pupae (Figure 1B, Table S4). Of the seven samples from 2017 from Kangerlussuaq that were submitted for DNA barcoding, three specimens were successfully identified as Gelis micrurus (Forster, 1850; Figure 1C) with 100% confidence using the BOLD database (Ratnasingham and Hebert, 2013). The sequences and associated data for identified parasitoids (i.e., GPS coordinates of collection locality and images of parasitoid tissues) are available from BOLD (www.boldsystems.org) through the following Barcode Index Number: BOLD:ACF8182 and DOI: dx.doi.org/10.5883/DS-LSGNLD18. We note that G. micrurus is a new species record for the area, which is not included in the recent identification manual for Greenland (Böcher et al., 2015). Identification attempts for the other four samples were not successful, perhaps due to the limited amount of tissue submitted for wasp eggs and/or larvae.
In sharp contrast, we did not find any evidence of egg sac parasitism at Zackenberg, the high-arctic site, despite having sampled at that site over a longer time period (18 years) and generally having larger samples than at Kangerlussuaq (Table 1). Simulation tests indicate that failure to detect parasitized egg sacs would have been highly unlikely given our sample sizes if Zackenberg's parasitism rate was similar to either the highest or lowest parasitism rate measured at Kangerlussuaq (Table 1).
Discussion
We found no evidence that the wolf spider P. glacialis, a dominant tundra predator in Greenland, has experienced any measurable predation pressure by egg sac parasitoids at the northern edge of its range over an 18-year period of the recent past. This finding is surprising because wolf spider egg sacs in general, are commonly parasitized across arctic (Bowden and Buddle, 2012a) and temperate (Edgar, 1971) ecosystems, and because our own measurements indicate that P. glacialis in particular, is exposed to parasitism in southwestern Greenland. Whether arctic wolf spiders have “escaped” parasitism as a result of local extinction of the parasitoid at the northern site or because this population of wolf spiders has never been exposed to parasitism is unclear. Nevertheless, our results demonstrate that geographic variability in the rate of parasitism is high for this widespread wolf spider species. In the context of a rapidly warming Arctic, such high variability in the strength of host-parasite species interactions also suggests that population-level responses to environmental change are unlikely to be uniform throughout a species' range.
Understanding what drives wolf spider population dynamics is important because these predators comprise a large proportion of worldwide spider abundance and diversity (World Spider Catalog, 2018). In the Arctic, this endeavor is even more relevant, because wolf spiders are one of the most widespread and locally abundant arthropods (Bowden and Buddle, 2010; Wyant et al., 2011; Hansen et al., 2016a; Koltz et al., 2018b). In terms of host-parasite dynamics, the absence of parasitism in Zackenberg is especially surprising when considering that this site hosts two wasp species (Wirta et al., 2016) of a genus known to parasitize wolf spiders in North America (Bowden and Buddle, 2012a) and Kangerlussuaq (this study). At least one of these species, however, is a Lepidopteran parasitoid (on the family Erebidae; Wirta et al., 2015) that is unlikely to prey on wolf spider egg sacs. Given that the population viability of a species is typically most fragile at the edge of its range (Lawton, 1993), it is possible that a local absence of parasitism has enabled P. glacialis to occur further north than would otherwise be expected. If that is the case, then the possibility of parasitoid range expansions under climate change (Chen et al., 2011) could threaten the continued persistence of this species in the northern High Arctic. Because wolf spiders generate trophic cascades at multiple levels within the food web that can ultimately affect important processes like decomposition and nutrient cycling (Wise et al., 1999; Scheu, 2001; Koltz et al., 2018b), such distributional changes, although seemingly insignificant, could have important ecological impacts.
There are a number of possible explanations for the local absence of a viable parasitoid of wolf spider egg sacs at Zackenberg, including the fact that Zackenberg has a more extreme climate than Kangerlussuaq (Figure S1). Likewise, slight differences in plant community composition and other abiotic conditions contribute to which arthropod species are present at a given site (Hansen et al., 2016a,b). Local geological and glacial histories also strongly affect the distribution of species, the interactions between them, and their co-evolutionary dynamics (Anderson and Ferree, 2010; Stewart et al., 2010; Yeakel et al., 2013; Ávila-Jiménez et al., 2019). Zackenberg is more recently deglaciated compared to other areas of the Arctic where wolf spider egg sac parasitism rates are high, such as NW North America (Tarasov et al., 2012; Lecavalier et al., 2014). Various barriers to dispersal, such as the Greenland ice sheet, may have prohibited potential egg sac parasitoids from accessing NE Greenland. Such dispersal barriers are likely to be particularly limiting to apterous, or wingless, arthropods, including females from many Gelis species, which may contribute to these parasitoids having more narrow spatial distributions.
The geographic variability in the strength of predation pressure by egg sac parasitoids on P. glacialis that we demonstrate here suggests that life history strategies of this wolf spider species and in turn, its population-level responses to climate change, may vary across its range. For example, although sampling date was not a significant predictor of parasitism (Table S5), our data suggest that incidences of egg sac parasitism occur during the peak of the growing season (Table S4). Early season production of egg sacs by female wolf spiders could thus be one strategy to avoid parasitism, but the timing and duration of this period is expected to shift as the Arctic continues to warm. The degree to which parasitism pressure plays a role in dictating various life history traits of this abundant predator and other arctic arthropods, including their phenology, behavior, and foraging decisions (e.g., Schmid Hempel, 2011), warrants further investigation, particularly where climate change may affect the timing and strength of these species interactions.
Data Availability
All datasets analyzed for this study are included in the manuscript and the Supplementary Files.
Author Contributions
AK conceived of the idea for the research. LC collected field samples. AK, JB, LC completed the lab work. AK, TH, LC, JB, and EP wrote the paper.
Funding
AK was supported by a grant from Aarhus University Research Foundation and the National Geographic Society Committee for Research and Exploration. This research was also supported by U.S. National Science Foundation Award numbers 1106401 to AK, 1506155 to LC, and 1107381 and 0902125 to EP. Grace Stillwell, supported by Dartmouth's Women In Science Program, assisted with lab work. This work is a contribution to the Arctic Science Partnership (ASP), asp-net.org.
Conflict of Interest Statement
The authors declare that the research was conducted in the absence of any commercial or financial relationships that could be construed as a potential conflict of interest.
Acknowledgments
We are grateful to Michael Avery and the Greenland Ecosystem Monitoring programme for providing us access to the samples for this study and to the Natural History Museum, Aarhus for curation of the Zackenberg specimens.
Supplementary Material
The Supplementary Material for this article can be found online at: https://www.frontiersin.org/articles/10.3389/fevo.2019.00250/full#supplementary-material
References
Anderson, M. G., and Ferree, C. E. (2010). Conserving the stage: climate change and the geophysical underpinnings of species diversity. PLoS ONE 5:e11554. doi: 10.1371/journal.pone.0011554
Avery, M., and Post, E. (2013). Record of a Zoophthora sp.(Entomophthoromycota: Entomophthorales) pathogen of the irruptive noctuid moth Eurois occulta (Lepidoptera) in West Greenland. J. Invertebr. Pathol. 114, 292–294. doi: 10.1016/j.jip.2013.09.005
Ávila-Jiménez, M. L., Solhøy, T., Gwiazdowicz, D. J., Fjellberg, A., Dózsa-Farkas, K., Monson, F., et al. (2019). The terrestrial invertebrate fauna of Edgeøya, Svalbard: Arctic landscape community composition reflects biogeography patterns. Polar Biol. 42, 837–850. doi: 10.1007/s00300-019-02471-x
Bliss, L. (1981). 1.2 North American and Scandinavian tundras and polar deserts. Tundra Ecosyst. 25:8.
Böcher, J., Kristensen, N. P., Pape, T., and Vilhelmsen, L. (2015). The Greenland Entomofauna: An Identification Manual of Insects, Spiders and Their Allies. Leiden: Brill. doi: 10.1163/9789004261051
Bowden, J., and Buddle, C. (2012a). Egg sac parasitism of Arctic wolf spiders (Araneae: Lycosidae) from northwestern North America. J. Arachnol. 40, 348–350. doi: 10.1636/P11-50.1
Bowden, J., and Buddle, C. (2012b). Life history of tundra-dwelling wolf spiders (Araneae: Lycosidae) from the Yukon Territory, Canada. Can. J. Zool. 90, 714–721. doi: 10.1139/z2012-038
Bowden, J. J., and Buddle, C. M. (2010). Determinants of ground-dwelling spider assemblages at a regional scale in the Yukon Territory, Canada. Ecoscience 17, 287–297. doi: 10.2980/17-3-3308
Bowden, J. J., Hansen, O. L. P., Olsen, K., Schmidt, N. M., and Høye, T. T. (2018). Drivers of inter-annual variation and long-term change in High-Arctic spider species abundances. Polar Biol. 41, 1635–1649. doi: 10.1007/s00300-018-2351-0
Chen, I.-C., Hill, J. K., Ohlemüller, R., Roy, D. B., and Thomas, C. D. (2011). Rapid range shifts of species associated with high levels of climate warming. Science 333, 1024–1026. doi: 10.1126/science.1206432
Cobb, L. M., and Cobb, V. A. (2004). Occurrence of parasitoid wasps, Baeus sp. and Gelis sp., in the egg sacs of the wolf spiders Pardosa moesta and Pardosa sternalis (Araneae, Lycosidae) in southeastern Idaho. Can. Field-Nat. 118, 122–123. doi: 10.22621/cfn.v118i1.894
Dondale, C., and Redner, J. (1990). “Part-17: The insects and arachnids of Canada,” in The wolf spiders, Nurseryweb Spiders, and Lynx Spiders of Canada and Alaska. Araneae: Lycosidae, Pisauridae, and Oxyopidae. Ottawa, ON: Agriculture Canada.
Edgar, W. D. (1971). Aspects of the ecology and energetics of the egg sac parasites of the wolf spider Pardosa lugubris (Walckenaer). Oecologia 7, 155–163. doi: 10.1007/BF00346357
Ernst, C. M., Hanelt, B., and Buddle, C. M. (2016). Parasitism of ground beetles (Coleoptera: Carabidae) by a new species of hairworm (Nematomorpha: Gordiida) in Arctic Canada. J. Parasitol. 102, 327–335. doi: 10.1645/15-863
Fellowes, M. D. E., and Godfray, H. C. J. (2000). The evolutionary ecology of resistance to parasitoids by Drosophila. Heredity 84:1. doi: 10.1046/j.1365-2540.2000.00685.x
Godfray, H., Hassell, M., and Holt, R. (1994). The population dynamic consequences of phenological asynchrony between parasitoids and their hosts. J. Anim. Ecol. 63, 1-10. doi: 10.2307/5577
Hagemoen, R. I. M., and Reimers, E. (2002). Reindeer summer activity pattern in relation to weather and insect harassment. J. Anim. Ecol. 71, 883–892. doi: 10.1046/j.1365-2656.2002.00654.x
Hagstrum, D. W. (1971). Carapace width as a tool for evaluating the rate of development of spiders in the laboratory and the field. Ann. Entomol. Soc. Am. 64, 757–760. doi: 10.1093/aesa/64.4.757
Hansen, R. R., Hansen, O. L. P., Bowden, J. J., Normand, S., Bay, C., Sørensen, J. G., et al. (2016a). High spatial variation in terrestrial arthropod species diversity and composition near the Greenland ice cap. Polar Biol. 39, 2263–2272. doi: 10.1007/s00300-016-1893-2
Hansen, R. R., Hansen, O. L. P., Bowden, J. J., Treier, U. A., Normand, S., and Høye, T. (2016b). Meter scale variation in shrub dominance and soil moisture structure Arctic arthropod communities. PeerJ 4:e2224. doi: 10.7717/peerj.2224
Høye, T. T., and Culler, L. E. (2018). Tundra arthropods provide key insights into ecological responses to environmental change. Polar Biol. 41, 1523–1529. doi: 10.1007/s00300-018-2370-x
Høye, T. T., and Forchhammer, M. C. (2008). Phenology of high-arctic arthropods: effects of climate on spatial, seasonal, and inter-annual variation. Adv. Ecol. Res. 40, 299–324. doi: 10.1016/S0065-2504(07)00013-X
Høye, T. T., and Hammel, J. U. (2010). Climate change and altitudinal variation in sexual size dimorphism of arctic wolf spiders. Clim. Res. 41, 259–265. doi: 10.3354/cr00855
Høye, T. T., Hammel, J. U., Fuchs, T., and Toft, S. (2009). Climate change and sexual size dimorphism in an Arctic spider. Biol. Lett. 5, 542–544. doi: 10.1098/rsbl.2009.0169
Jensen, L. M., Rasch, M., and Schmidt, N. M. (ed) (2013). Zackenberg Ecological Research Operations, 18th Annual Report, 2012. Aarhus University; DCE-Danish Centre for Environment and Energy, 122.
Koltz, A. M., Asmus, A., Gough, L., Pressler, Y., and Moore, J. C. (2018a). The detritus-based microbial-invertebrate food web contributes disproportionately to carbon and nitrogen cycling in the Arctic. Polar Biol. 41, 1531–1545. doi: 10.1007/s00300-017-2201-5
Koltz, A. M., Classen, A. T., and Wright, J. P. (2018b). Warming reverses top-down effects of predators on belowground ecosystem function in Arctic tundra. Proc. Natl. Acad. Sci. U.S.A. 115, E7541–E7549. doi: 10.1073/pnas.1808754115
Koltz, A. M., Schmidt, N. M., and Høye, T. T. (2018c). Differential arthropod responses to warming are altering the structure of Arctic communities. R. Soc. Open Sci. 5:171503. doi: 10.1098/rsos.171503
Kukal, O., and Kevan, P. G. (1987). The influence of parasitism on the life history of a high arctic insect, Gynaephora groenlandica (Wöcke) (Lepidoptera: Lymantriidae). Can. J. Zool. 65, 156–163. doi: 10.1139/z87-022
Kutz, S. J., Hoberg, E. P., Nishi, J., and Polley, L. (2002). Development of the muskox lungworm, Umingmakstrongylus pallikuukensis (Protostrongylidae), in gastropods in the Arctic. Can. J. Zool. 80, 1977–1985. doi: 10.1139/z02-197
Lawrence, K. L., and Wise, D. H. (2000). Spider predation on forest-floor Collembola and evidence for indirect effects on decomposition. Pedobiologia 44, 33–39. doi: 10.1078/S0031-4056(04)70026-8
Lawton, J. H. (1993). Range, population abundance and conservation. Trends Ecol. Evol. 8, 409–413. doi: 10.1016/0169-5347(93)90043-O
Lecavalier, B. S., Milne, G. A., Simpson, M. J., Wake, L., Huybrechts, P., Tarasov, L., et al. (2014). A model of Greenland ice sheet deglaciation constrained by observations of relative sea level and ice extent. Quat. Sci. Rev. 102, 54–84. doi: 10.1016/j.quascirev.2014.07.018
Lensing, J. R., and Wise, D. H. (2006). Predicted climate change alters the indirect effect of predators on an ecosystem process. Proc. Natl. Acad. Sci. U.S.A. 103, 15502–15505. doi: 10.1073/pnas.0607064103
Low, P. A., McArthur, C., and Hochuli, D. F. (2014). Dealing with your past: experience of failed predation suppresses caterpillar feeding behaviour. Anim. Behav. 90, 337–343. doi: 10.1016/j.anbehav.2014.02.020
Marshall, S. D., and Gittleman, J. L. (1994). Clutch size in spiders: is more better? Funct. Ecol. 8, 118–124. doi: 10.2307/2390120
Meltofte, H., and Rasch, M. (2008). The study area at Zackenberg. Adv. Ecol. Res. 40, 101–110. doi: 10.1016/S0065-2504(07)00005-0
Moerschel, F. M. (1999). Use of climatic data to model the presence of oestrid flies in carbiou herds. J. Wildl. Manage. 63, 588–593. doi: 10.2307/3802647
Post, E. (2013). Ecology of Climate Change: The Importance of Biotic Interactions. Princeton, NJ: Princeton University Press. doi: 10.23943/princeton/9780691148472.001.0001
Ratnasingham, S., and Hebert, P. D. (2007). BOLD: the barcode of life data system (http://www.barcodinglife.org). Mol. Ecol. Notes 7, 355–364. doi: 10.1111/j.1471-8286.2007.01678.x
Ratnasingham, S., and Hebert, P. D. (2013). A DNA-based registry for all animal species: the Barcode Index Number (BIN) system. PLoS ONE 8:e66213. doi: 10.1371/journal.pone.0066213
RCoreTeam (2017). R: A Language and Environment for Statistical Computing. R Foundation for Statistical Computing. Vienna.
Scheu, S. (2001). Plants and generalist predators as links between the below-ground and above-ground system. Basic Appl. Ecol. 2, 3–13. doi: 10.1078/1439-1791-00031
Schmid Hempel, P. (2011). Evolutionary Parasitology: The Integrated Study of Infections, Immunology, Ecology, and Genetics (Oxford; New York: Oxford University Press).
Stahlhut, J. K., Fernández-Triana, J., Adamowicz, S. J., Buck, M., Goulet, H., Hebert, P. D., et al. (2013). DNA barcoding reveals diversity of Hymenoptera and the dominance of parasitoids in a sub-arctic environment. BMC Ecol. 13:2. doi: 10.1186/1472-6785-13-2
Stewart, J. R., Lister, A. M., Barnes, I., and Dalén, L. (2010). Refugia revisited: individualistic responses of species in space and time. Proc. R. Soc. Lond. B. Biol. Sci 277, 661–671. doi: 10.1098/rspb.2009.1272
Tarasov, L., Dyke, A. S., Neal, R. M., and Peltier, W. R. (2012). A data-calibrated distribution of deglacial chronologies for the North American ice complex from glaciological modeling. Earth Planet. Sci. Lett. 315-316, 30–40. doi: 10.1016/j.epsl.2011.09.010
Wirta, H., Várkonyi, G., Rasmussen, C., Kaartinen, R., Schmidt, N. M., Hebert, P., et al. (2016). Establishing a community-wide DNA barcode library as a new tool for arctic research. Mol. Ecol. Resour. 16, 809–822. doi: 10.1111/1755-0998.12489
Wirta, H. K., Vesterinen, E. J., Hambäck, P. A., Weingartner, E., Rasmussen, C., Reneerkens, J., et al. (2015). Exposing the structure of an Arctic food web. Ecol. Evol. 5, 3842–3856. doi: 10.1002/ece3.1647
Wise, D., Snyder, W., Tuntibunpakul, P., and Halaj, J. (1999). Spiders in decomposition food webs of agroecosystems: theory and evidence. J. Arachnol. 27, 363–370.
World Spider Catalog (2018). The World Spider Catalog, Version 18.5.in Museum. Bern. Available online at: http://wsc.nmbe.ch/
Wyant, K. A., Draney, M. L., and Moore, J. C. (2011). Epigeal spider (Araneae) communities in moist acidic and dry heath tundra at Toolik Lake, Alaska. Arctic Antarctic Alp. Res. 43, 301–312. doi: 10.1657/1938-4246-43.2.301
Keywords: Arctic, Hymenoptera, Greenland, parasitism, parasitoid, wasp, wolf spider, Pardosa glacialis
Citation: Koltz AM, Culler LE, Bowden JJ, Post E and Høye TT (2019) Dominant Arctic Predator Is Free of Major Parasitoid at Northern Edge of Its Range. Front. Ecol. Evol. 7:250. doi: 10.3389/fevo.2019.00250
Received: 19 February 2019; Accepted: 17 June 2019;
Published: 03 July 2019.
Edited by:
Gina Marie Wimp, Georgetown University, United StatesReviewed by:
Clay Cressler, University of Nebraska-Lincoln, United StatesMarcelo Oliveira Gonzaga, Federal University of Uberlandia, Brazil
Copyright © 2019 Koltz, Culler, Bowden, Post and Høye. This is an open-access article distributed under the terms of the Creative Commons Attribution License (CC BY). The use, distribution or reproduction in other forums is permitted, provided the original author(s) and the copyright owner(s) are credited and that the original publication in this journal is cited, in accordance with accepted academic practice. No use, distribution or reproduction is permitted which does not comply with these terms.
*Correspondence: Amanda M. Koltz, akoltz@wustl.edu