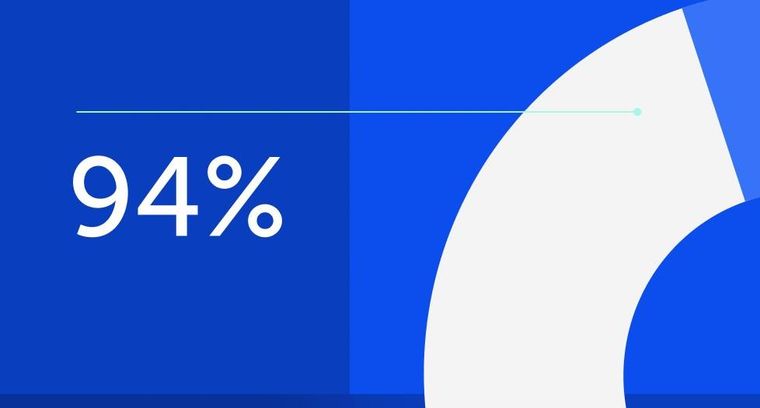
94% of researchers rate our articles as excellent or good
Learn more about the work of our research integrity team to safeguard the quality of each article we publish.
Find out more
ORIGINAL RESEARCH article
Front. Ecol. Evol., 18 June 2019
Sec. Coevolution
Volume 7 - 2019 | https://doi.org/10.3389/fevo.2019.00215
Sexual conflict may result in the escalating coevolution of sexually antagonistic traits. However, our understanding of the evolutionary dynamics of antagonistic traits and their role in association with sex-specific escalation remains limited. Here we study sexually antagonistic coevolution in a genus of water striders called Rhagovelia. We identified a set of male grasping traits and female anti-grasping traits used during pre-mating struggles and show that natural variation of these traits is associated with variation in mating performance in the direction expected for antagonistic coevolution. Phylogenetic mapping detected signal of escalation of these sexually antagonistic traits suggesting an ongoing arms race. Moreover, their escalation appears to be influenced by a trade-off with dispersal through flight in both sexes. Altogether our results highlight how sexual interactions and natural selection may have shaped sex-specific antagonistic trait coevolution.
The evolutionary interests of males and females in reproductive interactions often diverge, leading to the coevolution of sexually antagonistic traits that are favored in one sex at a fitness cost to the other (Arnqvist and Rowe, 2005). Females possess a limited number of eggs and pay increasing costs for multiple mating (Rowe et al., 1994; Arnqvist and Rowe, 2005). On the other hand, males are favored to mate repeatedly because increased mating rate in males results in increased fitness (Rowe et al., 1994; Arnqvist and Rowe, 2005). These opposing interests during mating interactions cause sexual conflict over mating rate (Arnqvist and Rowe, 2005). In this specific case, sexual antagonism drives the evolution of armaments whereby grasping traits allowing males to persist and increase their mating success are often matched by the evolution of anti-grasping traits in females improving their ability to resist and thus escape fitness costs associated with superfluous mating events (Weigensberg and Fairbairn, 1996; Arnqvist and Rowe, 2002b). The repeated evolution of such sex-specific armaments can lead to episodes of escalation and ultimately arms race (Arnqvist and Rowe, 1995, 2002a; Pennell and Morrow, 2013). Sex chromosome evolution (Dean et al., 2012; Pennell and Morrow, 2013), sex biased gene expression (Snook et al., 2010; Mank et al., 2013; Mank, 2017) and intra- and inter-locus sexual conflict (Rowe and Day, 2006; Bonduriansky and Chenoweth, 2009; Crumiere and Khila, 2019), are expected to drive the divergence of the sexes, often resulting in striking cases of sexual dimorphism (Rowe and Day, 2006; Bonduriansky and Chenoweth, 2009).
The dynamics of morphological co-evolution of sexually antagonistic traits has attracted much attention (Arnqvist and Rowe, 2002a; Bergsten and Miller, 2007; Kuntner et al., 2009) and water striders have been widely used as a model to study sexual dimorphism and antagonistic co-evolution in the context of sexual conflict (Arnqvist, 1992; Arnqvist and Rowe, 1995; Weigensberg and Fairbairn, 1996). Despite the rich diversity of this group of semiaquatic insects, the vast majority of these studies have focused on the Gerridae family (Insecta, Heteroptera, Gerromorpha, Veliidae) (Arnqvist, 1992; Arnqvist and Rowe, 1995, 2002a; Weigensberg and Fairbairn, 1996). Here, we investigated the evolution of sexual dimorphism in the tropical genus Rhagovelia from the Veliidae family (Insecta, Heteroptera, Gerromorpha, Veliidae) (Andersen, 1982; Polhemus, 1997; Moreira and Ribeiro, 2009). In this genus, sexual dimorphism is particularly dramatic with both sexes often bearing morphological traits reminiscent to those usually found in species with strong sexual conflict (Andersen, 1982; Polhemus, 1997; Arnqvist and Rowe, 2005; Moreira and Ribeiro, 2009). However, the process of sexual selection; i.e., male competition, mate choice or sexual conflict over mating rate, driving sexual dimorphism in Rhagovelia is unknown (Andersson, 1994; Arnqvist and Rowe, 2005). Through morphological and behavioral observations, we identified a large set of potentially sexually antagonistic armaments in the Rhagovelia genus and tested their role during sexual interactions in a representative species called Rhagovelia antilleana (Andersen, 1982; Polhemus, 1997; Moreira and Ribeiro, 2009). We then tested how variation in sexually antagonistic traits affects male and female success during sexual interactions. We show that this variation is associated with trade-offs with flight capability and egg storage. Finally, we used phylogenetic reconstruction of male and female secondary sexual traits to understand how antagonistic coevolution and diversification may have shaped the divergence of the sexes in this lineage and assess the extent of evolutionary arms race.
Sexual dimorphism in the water strider genus Rhagovelia ranges from subtle to spectacular differences in morphology between the sexes (Figures 1A–D'; Polhemus, 1997; Moreira and Ribeiro, 2009). Rhagovelia populations contain both winged and wingless morphs (Polhemus, 1997; Moreira and Ribeiro, 2009). In our R. antilleana lab population, winged females were present at 27.5% and wingless females at 72.5% while winged males were present at 18.75% and wingless males at 81.25%. In both male morphs, the foreleg tarsi are equipped with prominent, hook-like, combs that are absent in the females (Figures 1E,E'; Polhemus, 1997; Moreira and Ribeiro, 2009). The rearlegs of males possess several rows of spikes of different sizes along the trochanter, femur, and tibia (Figures 1F,F'–G,G'; Polhemus, 1997; Moreira and Ribeiro, 2009). In addition, the femurs of the males' rearlegs are very thick compared to those of the females (Figures 1C,D,F,C',D',F'; Polhemus, 1997; Moreira and Ribeiro, 2009). Staining for actin fibers revealed that the width of the femur is entirely occupied by muscles (Figure S1), suggesting that thicker femurs have the potential to apply stronger grip force. In females, winged morphs possess a prominent spike-like projection of the pronotun (Figure 1H'; Polhemus, 1997) that is not found in wingless females (Figure 1C') or winged males (Figure 1H; Polhemus, 1997). Wingless females have a narrow-shaped abdomen (Figure 2B) that can be even more pronounced in some species such as Rhagovelia obesa (Figure 2C). We have never observed winged females with narrow abdomen or wingless females with pronotum projection indicating that these two traits are mutually exclusive (Figures 2A,B). This observation suggests that morph-specific strategies to reduce mating frequency have evolved in Rhagovelia females.
Figure 1. Sexual dimorphism and secondary sexual traits in the genus Rhagovelia. The outgroup Oiovelia cunucunumana (A,A') does not show strong dimorphism. In the genus Rhagovelia, sexual dimorphism can be subtle as in Rhagovelia plumbea (B,B') or more extreme as in Rhagovelia antilleana (C,C') or Rhagovelia sp.2 (D,D'). This dimorphism affects secondary sexual traits such as the sex combs (in red) present in male fore-legs (E) and absent in females (E'); the presence of spikes (in blue, yellow and red) and the larger femur of the rear-leg femur in males (F) compared to females that only have some small femur spikes (F'); and the presence of spikes (in green) along the rear-leg tibia in males (G) that are also absent in females (G'). Females possess secondary sexual traits such as a narrow abdomen (C') and a pronotum projection (H') that are absent in males (C,H).
Figure 2. Variation in secondary sexual traits in Rhagovelia. Winged R. antilleana females (A) have a large abdomen compared to wingless females (B). The presence of wing muscles (A', in red) in the thorax constrains the eggs to be located in the abdomen (in yellow) while in wingless females (B') the space in the thorax is free from wing muscles and can be occupied by eggs, thus allowing a narrow abdomen. In species such as R. obesa (C), the narrow abdomen is even more pronounced and eggs are located in the thorax (C'), thus confirming our observation. There is also variability in the rear-leg in males, especially in the size of the femur. Winged males (D), have thin femurs while other males have intermediate (E) or large femurs (F).
Pre-mating interactions in R. antilleana include vigorous struggles between males and females, characteristic of sexual conflict over mating rate (Arnqvist and Rowe, 1995, 2005; Khila et al., 2012). Behavioral quantification revealed that both winged and wingless males approach females preferably on the side and the back by grasping female's mid- and rearlegs (Figure S2A). We did not detect a significant male preference for winged or wingless morphs or any differences in the frequency of mating events related to the overall numbers of pre-mating interactions across female morphs (Figures S2B–D). When mounted, males use the sex combs to clasp the pronotum of the female and tighten the grip on her legs using their modified rearlegs (Supplementary Video 1). Females vigorously shake their body and perform repeated somersaults, which frequently result in rejecting the male (Supplementary Video 2). The struggles are vigorous and their duration is typically 0.68 ± 0.99 s in average, but can vary between fractions of a second (<0.15 s) to several seconds (9.75 s) (Figure S2E). These pre-mating struggles are indicative of sexual conflict over mating rate and the sex-specific modifications we observed might be the result of antagonistic interaction between the sexes in this species.
Artificially generated variation in sexually antagonistic traits is known to result in variation in mating success (Khila et al., 2012). Here, we wanted to test whether natural variation in male persistence and female resistance traits is also associated with variation in mating success. In R. antilleana, only winged females have the pronotum projection (Figure 2A) and only wingless females have narrow abdomen (Figure 2B), whereas in males there is considerable natural variation in the degree of elaboration of the rear-legs (Figures 2D–F). To determine the extent to which this variation affects individual performance during pre-mating struggles, we used a tournament design (Figure S3; Ivy and Sakaluk, 2007) composed of 10 replicates with 8 males and 8 females each for a total of 80 males and 80 females. This experiment separated males and females based on the outcome of premating struggles into successful, intermediate, and unsuccessful individuals (Figure 3 and Figure S3). Successful males are those who copulate after a premating struggle and successful females are those who reject males after pre-mating struggle (Figure S3). We found that successful males have significantly more rear-leg tibia spikes and thicker rear-leg femurs compared to unsuccessful males (respectively Wilcoxon test: p = 0.045*; Student t-test: p = 0.02*; Figures 3A,B and Figure S4), however body size, number of spikes on rear-leg femur, and the length of rear-leg tibia and femur did not significantly differ between the two groups (Figures 3C–F and Figure S4). This result highlights the importance of the rear-legs for increasing male mating frequency and therefore, to some extents, male fitness (Andersson, 1994; Danielsson, 2000; Arnqvist and Rowe, 2005). In females, our data indicated that individuals with a pronotum projection were significantly more efficient in rejecting males than females with narrow abdomen (Cochran-Mantel-Haenszel chi-squared test: 23,45; df = 2; p-value: 8.089e-06***; Figure 3G). To further test the validity of this conclusion, we conducted an experiment where the performance of 30 wingless females (narrow abdomen and absence of pronotum projection) was compared to that of 18 winged females (wide abdomen) from which we amputated the pronotum projection. We found that winged females with amputated pronotum projection were less efficient at rejecting males, which resulted in higher mating frequency (Figures 3G,H). This result indicates that in the absence of the pronotum projection, narrow abdomen increases female's ability to resist harassing males. Therefore, alternative morph-specific strategies evolved in the females possibly under selection by male harassment. Altogether, these experiments suggest that natural variation in sexually antagonistic traits is associated with variation in the ability of both sexes to control mating rate.
Figure 3. Morphological comparisons and effect on mating performance. Successful males have significantly higher number of tibia spikes (A) and larger femur (B) compared to unsuccessful males. There are no significant differences between the two classes of males for body length (C), number of femur spikes (D), femur length (E) and tibia length (F). Student t-tests were performed for all comparisons except for the number of femur spikes (Wilcoxon test). (G–I) show the distribution of the different morphs ecotype in the different classes of performance with the associated results of Cochran-Mantel-Haenszel Chi-square test. Significant p-values indicate association between the morph ecotype and the performance. Females with pronotum projection are more efficient than females with narrow abdomen in rejecting males (G). Females with amputated pronotum projection lost their ability to efficiently reject males (H). Wingless males are more efficient than winged males in mating (I). A proportional odds logistic regression analysis (polr) for the different phenotypic variables is indicated in all graphs. Significant p-values indicate association between the phenotypic trait measured and success in mating efficiency for males and male rejection for females. n indicates sample size.
Because natural variation in antagonistic traits can result in variation in the performance of males and females during pre-mating struggles, we investigated whether life history traits might influence this variation. Many studies described the presence of trade-offs between flight capability and certain morphological or physiological traits, such as fertility, in a number of insects (Fairbairn and Desranleau, 1987; Langellotto et al., 2000; Simmons and Emlen, 2006; Karlsson and Johansson, 2008). We therefore tested whether wing polymorphism in R. antilleana affects variation in male's and female's sexually antagonistic traits. The pronotum projection, a sexually antagonistic trait that increases the ability of females to resist male harassment, only develops in winged female morphs (Figure 1H'). The presence of the wings is accompanied by the presence of flight muscles, which fill most of the space in the thorax (Figure 2A'; Fairbairn and Desranleau, 1987; Kaitala, 1988). These winged females position their developing eggs mostly in the abdomen (Figure 2A'). In wingless females of both R. antilleana and R. obesa, we found that the absence of flight muscles provides a space in the thorax that is occupied by the developing eggs (Figures 2B',C'). Furthermore, we found that wingless females contained a significantly higher number of developing eggs than winged females, consistent with the trade-off between flight and fertility (mean of 4.15 eggs ± 2.96 for n = 20 wingless females vs. mean of 1.35 eggs ± 2.35 for n = 20 winged females; Student t-test: p-value 0.002094**; Figures 2A'–C') (Fairbairn and Desranleau, 1987; Fairbairn, 1988; Roff and Fairbairn, 1991). These data suggest that the presence of wings is associated with the development of the pronotum projection and constrains the narrowing of the abdomen (Figure S5). These morphological constraints may have driven the evolution of alternative sex-specific and morph-specific strategies to escape fitness costs due to frequent mating in females. In males, we observed that winged morphs typically have the thinnest rear-leg femurs (Figure 2D) and were under-represented in the successful group of our tournament set-up, contrary to wingless morphs that have the largest rear-leg femurs (Cochran-Mantel-Haenszel chi-squared test: 10,957; degree of freedom: 2; p-value: 0.004177**; Figure 3I). Altogether, our observations highlight a trade-off between dispersal and mating success in both males and females.
Coevolution of the sexes due to antagonistic interactions could lead to escalation and arms race that deeply shape the evolutionary trajectory of lineages in nature (Arnqvist and Rowe, 1995, 2002a,b; Bergsten and Miller, 2007; Kuntner et al., 2009). We therefore tested the evolution of armament and presence of arms race in the Rhagovelia genus by analyzing phylogenetic patterns of correlation of male and female phenotypic complexity in terms of secondary sexually antagonistic traits (Arnqvist and Rowe, 2002a; Bergsten and Miller, 2007; Kuntner et al., 2009). We mapped eight male specific traits and two female specific traits on a phylogeny comprising a sample of 13 species to determine the patterns of phenotypic complexity between the sexes (Tables S1, S2, Figure 4, and Figures S6, S7). Our reconstruction showed a strong phylogenetic signal where males of the Rhagovelia genus evolved an increasing number of secondary sexual traits (Figure 4). This pattern indicates substantial escalation of conflict in males (Arnqvist and Rowe, 2002a,b; Bergsten and Miller, 2007; Kuntner et al., 2009). In females, we detected the evolution of anti-grasping traits in a clade where males are the most armed (Figure 4). However, these armaments do not show phylogenetic signal in females and we did not detect correlation between male and female phenotypic complexities (correlation test with phylogenetic independent contrast; rho: 0.28; p-value: 0.37, n.s.). Based on our samples, these results suggest an on-going arms race between males and females in the Rhagovelia genus and more specifically in R. antilleana and R. obesa (Arnqvist and Rowe, 2002a,b; Bergsten and Miller, 2007; Kuntner et al., 2009).
Figure 4. Comparison of male and female phenotypic complexity. The phylogeny of our samples shows a higher complexity of male phenotypes, in terms of number of secondary sexual traits, compared to outgroups (left phylogeny). This complexity is associated with strong phylogenetic signals in males. Male complexity is followed by higher complexity in females in two species R. antilleana and R. obesa (right phylogeny), the ones that possess narrow abdomen and pronotum projection, suggesting an ongoing arms race.
We have shown that sexual conflict over mating rate generates sexual dimorphism via the presence of secondary sexual traits in the Rhagovelia genus. Males have evolved a set of persistence grasping traits aimed at increasing mating frequency, whereas females evolved a set of anti-grasping traits aimed at decreasing mating frequency. Natural variation in these antagonistic traits directly impacts mating frequency and therefore influences the fitness of both sexes. Rhagovelia males possess the sex combs as grasping traits similar to other insects (Spieth, 1952; Markow et al., 1996; Polak et al., 2004; Chesebro et al., 2009; Tanaka et al., 2011). An additional male set of grasping traits consists of elaborations in the rearlegs, which are used as clasps that neutralize female's legs (Supplementary Video 1). Our analysis revealed that variation in number of spines on the tibia and in the width of the femurs results in variation in male mating success. While these two traits have shown significant importance, the legs possess a complete set of secondary sexual modifications that likely act in synergy altogether and increase male mating frequency. Females on the other hand, possess morph-specific modifications consisting of a narrow abdomen in wingless morphs and a pronotum projection in winged morphs. Both of these traits increase the chances for the female to reject harassing males. The projection forms a barrier and increases the distance between the abdomen of the male and the female (Supplementary Video 2), likely making it easier for the female to reject the male. Our experiments also showed that females with narrow abdomen are better at rejecting males than females to which we removed the pronotum projection and that have a wide abdomen. Because males use their rearlegs as clamps on the legs of the female, it is possible that the narrowing of the abdomen allows the female to slide out of this grip. Altogether our observations indicate a role of these sex-specific phenotypes in male persistence and female resistance (Arnqvist and Rowe, 2002a,b).
Our results also indicated that some life history traits such as fertility and dispersal influence sexually antagonistic armaments in both sexes (Fairbairn and Desranleau, 1987; Fairbairn, 1988; Roff and Fairbairn, 1991). Dispersal, manifested by the presence of wings (Andersen, 1982; Spence, 1989; Fairbairn and King, 2009), is associated with variation in the expression of sexually antagonistic traits in both sexes. Variation in the elaboration of these traits in turn influences the ability of the sexes to control mating frequency. Therefore, there is a tradeoff between dispersal and the expression of sexually antagonistic traits in Rhagovelia. In many wing-dimorphic gerrids, winged morphs are larger, less reproductively active, and mate and lay eggs at reduced rates compared with wingless morphs (Roff and Fairbairn, 1991; Fairbairn and Preziosi, 1996). In the case of R. antilleana winged females, the presence of wings is associated with the extension of the pronotum, which suggests that the development of this antagonistic structure might be contingent on the activation of the wing development program, although this hypothesis remains to be experimentally tested. This structure is an efficient female resistance trait that allows winged females to reduce mating events and potentially increase their fitness. Inversely, winged males develop relatively simple antagonistic traits associated with a low mating success but with the dispersal capability. In wingless morphs, males have evolved more elaborated secondary sexual traits that are associated with increased in mating success and the narrow abdomen of females allows male rejection but at a lower efficiency compared to winged females. These differences in mating success correlate with the higher egg numbers observed in wingless females that could potentially need to replenish sperm more often than winged females. However, how the decision to develop into winged or wingless morphs and whether sexual conflict affects this decision in Rhagovelia is unknown. Rhagovelia is a tropical genus and populations occur in large groups that can reach hundreds of individuals, suggesting that male harassment is frequent (Ditrich and Papacek, 2010). It is possible that under these conditions, winged morphs are favored to allow for dispersal and occupation of niches with lower population densities. However, winged morphs may not be favored in other contexts because of the trade-offs we observed between the presence of wings, the number of eggs produced by females, and the low success in pre-mating interactions we observed for males. It is more likely that trade-offs, between mating frequency, dispersal, and fertility are associated with differences in benefits and costs that maintain the variation of these traits and have shaped phenotypic evolution in this group of insects (Roff and Fairbairn, 1991).
Our study also highlights that antagonistic sexual interactions and morphological constraints might have driven evolutionary escalation in the Rhagovelia genus, similar to the dynamics observed in many species of the Gerridae (Arnqvist and Rowe, 2002a,b). Our analysis has shown that, across this genus, males have evolved an arsenal of traits across different species while females only evolved two morph-specific armaments (i.e., narrow abdomen and pronotum projection) in only two species from a single clade in our sample. These species, R. antilleana and R. obesa both belong to the collaris complex in the genus Rhagovelia (Polhemus, 1997). This complex of 44 species, out of the 396 Rhagovelia species identified, is characterized by the presence of pronotum projection in winged females and narrow abdomen in wingless females (Polhemus, 1997; Polhemus and Chordas, 2010). This suggests that sexual antagonism had strongly impacted the evolution of the genus. In addition to morphological modifications, the females have evolved complex behavior among their resistance arsenal through shaking and vigorous somersaults. In our study we have conducted behavioral observation in one species and our arms race hypothesis is based on morphological traits only. It would be interesting to expand the analysis to the difference in female resistance behavior across Rhagovelia species and assess to which extant females rely on behavior rather than morphological modifications in this genus (Rowe and Arnqvist, 2002). These observations could provide important insights about how behavioral modifications could participate and influence escalation and arms race. This could also explain why females from only some species evolved morphological secondary sexual traits, and why females generally evolved less traits than males. At a broader scale, comparison across families and therefore across different ecological niches, with gerrids inhabiting stagnant water (Crumiere et al., 2016) and Rhavogelia inhabiting stream water (Santos et al., 2017), would provide interesting insights about how habitats and lifestyles can influence behavioral and morphological coevolution (Perry and Rowe, 2018).
Species were collected during fieldwork in the locations indicated in Table S1. Specimens from Brazil were collected under SISBIO permit # 43105-1. Specimens from other locations did not request any official authorizations. Lab populations were established for R. antilleana. They were kept in water tanks at 25°C, 50% humidity, 14 h of daylight and fed daily on crickets. Styrofoam floaters were provided for adult female egg laying. Adults and nymphs were raised in independent tanks to decrease nymph cannibalism.
Picture acquisition and observation of secondary sexual traits were performed using a SteREO Discovery V12 (Zeiss), a Stereomicroscope M205 FA (Leica) and using Scanning Electron Microscopy at Centre Technologique des Microstructures (UCBL).
Male rear-leg femurs were dissected and opened using forceps and scalpel to remove the cuticle. Femurs were fixed with Formaldehyde 4% in PTW 1% during 20 min. Femurs were then washed in 5 successive 10-min baths of PTW 1%. The muscles were marked with 1/1,000 Phalloidine 488 (Invitrogen) for 1 h followed by 5 successive 10-min baths in PTW 1%. The femurs were mounted in 50% glycerol + Dapi.
Male behavior during pre-mating interactions has been analyzed through 4 different experiments. Experiment 1: 5 pairs of 2 males (4 pairs of 2 wingless males and 1 pair of 2 winged males) and 5 pairs of 2 females (1 winged and 1 wingless females) have been isolated during 5 days prior experiment in order to standardize mating receptivity in females and mating motivation in males. All individuals were taken from the stock population and were potentially non-virgin individuals. The experiment was performed during 5 consecutive days. Each day each pair of males were placed with a different pair of females in a common 14 × 10 cm plastic container with water, no food and no resting place. They were recorded during 10 min using a NIKON D7100 camera. We stopped trials when mating event occurred. Videos were analyzed using QuickTime software (Apple). Between each days/trials the different pairs were maintained isolated. With this set-up we obtained 5 biological replicates with 5 technical replicates for every pairs. Experiment 2: In this experiment we placed 1 male and 1 female from the general stock population in 8 × 6 cm plastic box containing water without food or resting area and captured every interaction using a Phantom Miro M310 Digital High-Speed Camera and PCC Software (Vision research, Ametek) for video acquisition. We recorded interactions until we reached 20 videos per couples or until a mating event occurred. We used 3 couples (biological replicates) with 3 trials each (technical replicates) for the 4 different possible morph combinations: wingless male with wingless female, winged male with winged female, wingless male with winged female, and winged male with wingless female. Experiment 3: In this experiment we took males and females from the general stock population. In each trial we placed 2 males (1 winged and 1 wingless) with 1 female (winged or wingless) in a 14 × 10 cm plastic container with water, no food and no resting place and we counted the number of interactions of each male with the female during 10 min. We used 3 different females for each morph type (biological replicates) with 3 replicates for each female (technical replicates). Experiment 4: We took males and females from the general stock population. For each trial we placed 1 male with 1 female in a 14 × 10 cm plastic container with water, no food and no resting place and using a stopwatch with centisecond option we recorded the duration of interactions over 10-min observation or until mating event occurred. Interactions shorter than 0.15 s were too fast to take note and were not consider in the analysis of data. We used 3 couples (biological replicates) with 3 trials each (technical replicates) for the 4 different possible morph combinations: wingless male with wingless female, winged male with winged female, wingless male with winged female, and winged male with wingless female. Data analysis: Data collected from experiments 1 and 2 were used to analyse the way males approach females by counting and classifying the different types of approach into “anteriorly” if males approach from the front and block the female T1-legs (fore-legs), “posteriorly” if males approach from the back and block the female T3-legs (rear-legs), and from the side if the males block female T1- and T2-legs (mid-legs), T1- and T3-legs or T2- and T3 legs (Figure S2A). Data from experiments 1 and 3 were used to evaluate the interaction and mating frequencies by counting the total number of interactions and mating events and by calculating the ratio between these two variables (Figures S2B–D). Data from experiment 4 were used to quantify the duration of pre-mating interactions (Figure S2E).
Non-virgin males and females were isolated into two different buckets (one for each sex), feed with frozen crickets, during 5 days to increase the motivation to mate. We set-up 10 tournaments between 8 males and 8 females (80 males and 80 females in total; with 22 winged females and 58 wingless females) to split them in three different categories: successful, intermediate and unsuccessful, depending the ability in copulating for males and the ability in rejecting male for females. Both males and females were introduced at the same time in a common 26 × 35 cm bucket of water without food or resting place. During the first round, for each mating we removed the couple and interrupted the mating by separating the male and the female in order to preserve the motivation to mate for the second round. The male was classified as successful and the female as unsuccessful. Inversely, the remaining males that did not succeed to mate, despite interactions with females, were classified as unsuccessful in mating. Remaining females that were never mated, despite interactions with males, were classified as successful in rejecting. The first round goes on until we obtained 4 successful males and 4 unsuccessful females (Figure S3). For the second round, we used two different buckets. In the first bucket, we introduced, at the same time, the 4 successful males with the 4 successful females, and in the second bucket we introduced, at the same time, the 4 unsuccessful males with the 4 unsuccessful females. When mating occurred, we removed the couple from the bucket, as in the first round. The second round goes on until we obtained 2 successful males and 2 unsuccessful females per buckets. The different rounds of this experiment occurred for few minutes to several hours depending on the activity of the bugs. For each replicate males were actively harassing females. At the end, we were able to obtain the following rank for both males and females: successful for males that have mated twice and females that have never mated, intermediate for individuals that mated once, and unsuccessful for males that have never mated and for females that mated twice (Figure S3). Then, using SteREO Discovery V12 (Zeiss) with ZEN 2011 software (Zeiss) we took pictures of each individual to quantify the individual phenotypes. We measured the length and the width of the rear-leg femur, the length of the rear-leg tibia and we counted the number of spikes on the rear-leg femur and the number of spikes on the rear-leg tibia. We also recorded the presence of a pronotum projection, narrow abdomen and body length in females. To further test the role of the pronotum projection, we manually removed the pronotum projection from 18 winged females using forceps and kept these females in lab condition during 1 week to allow them to recover and assess whether they behaved normally. Then we set-up 6 new tournaments to compare the ability of these 18 winged females in rejecting males against 30 wingless females. Prior experiment, all females were kept isolated from males. All data are available in the Dryad Digital Repository (doi: 10.5061/dryad.8cq6427) (Crumière et al., 2019).
Cochran-Mantel-Haenszel chi-squared tests (Figures 3G–I) were performed on data of the tournament using only the replicates where both morph types were present in order to compare performance [i.e., winged vs. wingless; 9 replicates (72 females) for pronotum projection vs. narrow abdomen; 6 replicates (48 females) for amputated pronotum vs. narrow abdomen; 8 replicates (64 males) for winged vs. wingless morphs]. Proportional odds logistic regression (MASS package) (Venables and Ripley, 2002) were performed on data of the tournament using all individuals in order to analyse phenotypes related to mating performance. Shapiro tests, and Student t-tests or Wilcoxon tests with fdr correction for multiple comparisons were performed using RStudio version 1.0.153 for analysis of male preference, duration of interactions, comparison of trait values for successful and unsuccessful males, depending whether variables followed normal distributions. R Script used in this study is available in the Dryad Digital Repository (doi: 10.5061/dryad.8cq642710.5061/dryad.8cq6427) (Crumière et al., 2019).
Sequences were retrieved from in house transcriptome databases and from Crumiere et al. (2016) for the following markers: 12S RNA; 16S RNA; 18S RNA; 28S RNA; Cytochrome Oxydase subunit I (COI); Cytochrome Oxydase subunit II (COII); Cytochrome Oxydase subunit III (COIII); Cytochrome b (cyt b); NADH-ubiquinone oxidoreductase chain 1 (ND1); Ultrabithorax (Ubx); Sex combs reduced (Scr); Gamma interferon inducible thiol reductase (gilt); Antennapedia (Antp); Distal-less (dll); Doublesex (dsx). All these markers were submitted to GenBank and their accession numbers can be found in the Dryad Digital Repository (doi: 10.5061/dryad.8cq6427) (Crumière et al., 2019). Phylogenetic reconstruction (Figure S5) was performed using MrBayes version 3.2.6 (GTR model; one million generations; 25% burnin) and PhyML version 3.0 (GTR model with 100 bootstraps) in Geneious 7.1.9 as described in Crumiere et al. (2016). Concatenation of sequence alignments and phylogenetic tree in Newick format are also available in the Dryad Digital Repository (doi: 10.5061/dryad.8cq6427) (Crumière et al., 2019).
We created a matrix of presence/absence of secondary sexual traits for both males and females (Table S2) in 13 species (4 outgroups and 9 Rhagovelia species). Based on morphological observation, we found eight traits exclusively present in males; the sex combs in the forelegs, the different rows of spines (up to 5 rows) and the shape of the different segments of rear-legs. Traits exclusively present in females include the pronotum projection and the narrow abdomen shape. Then, we mapped each sexually antagonistic trait individually and wing polymorphism on our phylogeny to infer their ancestral states (Figure S6). Finally, we performed a reconstruction using the combination of all traits, in males and females separately, to determine the pattern of phylogenetic complexity in this sample. Reconstruction of ancestral state was performed using ML reconstruction in Mesquite version 3.2 (Maddison and Maddison, 2018). Mapping of phenotypic complexity (ML reconstruction) and estimation of phylogenetic signal were performed using, respectively contMap and phylosig (package phytools, Revell, 2012). Correlation test with phylogenetic independent contrast has been conducted using the package ape (Paradis et al., 2004). These different analysis have been performed in RStudio version 1.0.153.
We used CT Scan to observe egg position without disrupting internal morphology of our samples. We fixed females of R. antilleana (n = 5 winged; n = 5 wingless) and R. obesa (n = 5 wingless) during 2 days using Bouin solution (MM France). Then, samples were washed twice with PBS 1X and were emerged 4 days in 0.3% phosphotungstic acid + 70% ethanol + 1% Tween20 solution to improve the contrast during microtomography acquisition (Metscher, 2009). Specimens were scanned using Phoenix Nanotom S (General Electrics) using the following parameters: 30 kV tensions, 200 μA intensity, 2,400 images with time exposure of 2,000 ms and a 2.5 μM voxel size. Three-dimensional images were reconstructed with the software attached to the machine (data rec) and then visualized with VGStudioMax (Volume Graphics). Longitudinal pictures were acquired using this same software to assess the presence of wing muscles and the position of eggs. Further egg counting was performed after dissection of 20 winged and 20 wingless R. antilleana females.
Pictures of adult females of R. antilleana (n = 10 winged; n = 10 wingless) and R. obesa (n = 9 wingless) were acquired using SteREO Discovery V12 (Zeiss). The body outline of each individual was extracted using Adobe Illustrator CC 2017 (Adobe). The analysis of outlines of the different conditions and Principal Component Analysis were performed using the package Momocs (Bonhomme et al., 2014) in Rstudio version 1.0.153.
Sexual conflict is ubiquitous in nature and is thought to be a potent force driving the evolution of sexually dimorphic traits. Under sexual conflict, the evolutionary interests of males and females in reproductive interactions often diverge, leading to the coevolution of sexually antagonistic traits that are favored in one sex at a fitness cost to the other. However, our understanding of the role of sexually antagonistic selection in driving the evolution of this dimorphism has been acquired through the study of some historical model systems. In this work, we studied the coevolution of males and females in the water strider genus Rhagovelia, to understand the evolutionary dynamics and the ecological factors that may influence the escalation of antagonistic traits between the sexes. In these insects, sexual dimorphism ranges from subtle to spectacular morphological differences between males and females; with various traits having a role during pre-mating struggles. By integrating morphological, behavioral and phylogenetic comparisons, we highlighted how sexual interactions and natural selection can influence and trigger diversification of secondary sexually antagonistic traits between males and females. Finally, our study promotes a new model system for the study of sexual conflict, which offers new perspectives for future studies.
The data collected and resources used in this study are available on Dryad Digital Depository (doi: 10.5061/dryad.8cq6427) (Crumière et al., 2019).
AC and AK: conception and design of experiments, analysis and interpretation, and drafting manuscript. AC, DA, AV-L, MK, FM, and AK: acquisition of data, revising manuscript.
This work was supported by the European Research Council (ERC-CoG # 616346), by the Conselho Nacional de Desenvolvimento Científico e Tecnológico (CNPq-PVE # 400751/2014-3) and by the Centre d'Etude de la Biodiversité Amazonienne (CEBA:ANR-10-LABX-25-01) to AK. The European Research Council (ERC-CoG # 616346) supports open access policies.
The authors declare that the research was conducted in the absence of any commercial or financial relationships that could be construed as a potential conflict of interest.
We thank A. Herrel, N. Nadeau, J. Abbott, and L. Rowe for helpful discussions. A. Le Bouquin, S. Viala, C. Finet, F. Bonneton, A. Decaras, R. Arbore, and W. Toubiana for comments on the manuscript. S. Viala for help with scanning electron microscopy and Centre Technologiques des Microstructure at Universite Claude Bernard Lyon 1 for access to SEM microscope, M. Bouchet for help with CT Scan, L. Souquet for help with shape analysis, and A. Le Bouquin and P. Joncour for help with statistics. This work was supported by ERC-CoG # 616346, CNPq-PVE # 400751/2014-3 and by CEBA:ANR-10-LABX-25-01 to AK. This manuscript has been released as a Pre-Print at https://www.biorxiv.org/content/10.1101/430322v1 (Crumière et al., 2018).
The Supplementary Material for this article can be found online at: https://www.frontiersin.org/articles/10.3389/fevo.2019.00215/full#supplementary-material
Supplementary Video S1. Interaction between a winged male and a winged female. The winged male attacks the female from the side by catching her by the mid- and rear-leg. Then the male climbs on the back of the female and block her mid- and rear-legs using his own rear legs as clamps. The male uses the fore-legs to catch the female pronotum, close to the head. The female resists by shaking its body to reject the male. This movie is a slow-motion video.
Supplementary Video S2. Interaction between wingless male and a winged female. The wingless male attacks the female by the back and climb on her while trying to block her mid- and rear-legs using its own rear-legs. The pronotum projection of the female forms a barrier increasing the distance between the male and the female thus facilitating male rejection. This movie is a slow-motion video.
Andersen, N. M. (1982). The Semiaquatic Bugs (Hemiptera: Gerromorpha). Klampenborg.: Scandinavian Science Press LTD.
Arnqvist, G. (1992). spatial variation in selective regimes: sexual selection in the water strider, gerris odontogaster. Evolution 46, 914–929. doi: 10.1111/j.1558-5646.1992.tb00609.x
Arnqvist, G., and Rowe, L. (1995). Sexual conflict and arms races between the sexes-a morphological adaptation for control of mating in a female insect. Proc. R. Soc. B Biol. Sci. 261, 123–127. doi: 10.1098/rspb.1995.0126
Arnqvist, G., and Rowe, L. (2002a). Antagonistic coevolution between the sexes in a group of insects. Nature 415, 787–789. doi: 10.1038/415787a
Arnqvist, G., and Rowe, L. (2002b). Correlated evolution of male and female morphologles in water striders. Evolution 56, 936–947. doi: 10.1111/j.0014-3820.2002.tb01406.x
Arnqvist, G., and Rowe, L. (2005). Sexual Conflict. Princeton, NJ: Princeton University Press. doi: 10.1515/9781400850600
Bergsten, J., and Miller, K. B. (2007). Phylogeny of diving beetles reveals a coevolutionary arms race between the sexes. PLoS ONE 2:e522. doi: 10.1371/journal.pone.0000522
Bonduriansky, R., and Chenoweth, S. F. (2009). Intralocus sexual conflict. Trends Ecol. Evol. 24, 280–288. doi: 10.1016/j.tree.2008.12.005
Bonhomme, V., Picq, S., Gaucherel, C., and Claude, J. (2014). Momocs: outline analysis using R. J. Stat. Softw. 56, 1–24. doi: 10.18637/jss.v056.i13
Chesebro, J., Hrycaj, S., Mahfooz, N., and Popadic, A. (2009). Diverging functions of Scr between embryonic and post-embryonic development in a hemimetabolous insect, Oncopeltus fasciatus. Dev. Biol. 329, 142–151. doi: 10.1016/j.ydbio.2009.01.032
Crumière, A. J. J., Armisén, D., Vargas-Lowman, A., Kubarakos, M., Moreira, F. F. F., and Khila, A. (2018). Escalation and constraints of antagonistic armaments in water striders. bioRxiv. doi: 10.1101/430322
Crumière, A. J. J., Armisén, D., Vargas-Lowman, A., Kubarakos, M., Moreira, F. F. F., and Khila, A. (2019). Data from: escalation and morphological constraints of antagonistic armaments in water striders. Dryad Digital Repository. doi: 10.5061/dryad.8cq6427
Crumiere, A. J. J., and Khila, A. (2019). Hox genes mediate the escalation of sexually antagonistic traits in water striders. Biol. Lett. 15, 20180720. doi: 10.1098/rsbl.2018.0720
Crumiere, A. J. J., Santos, M. E., Semon, M., Armisen, D., Moreira, F. F. F., and Khila, A. (2016). Diversity in morphology and locomotory behavior is associated with niche expansion in the semi-aquatic bugs. Curr. Biol. 26, 3336–3342. doi: 10.1016/j.cub.2016.09.061
Danielsson, I. (2000). Antagonistic pre- and post-copulatory sexual selection on male body size in a water strider (Gerris lacustris). Proc. R. Soc. B 268, 77–81. doi: 10.1098/rspb.2000.1332
Dean, R., Perry, J. C., Pizzari, T., Mank, J. E., and Wigby, S. (2012). Experimental evolution of a novel sexually antagonistic allele. PLoS Genet. 8:e1002917. doi: 10.1371/journal.pgen.1002917
Ditrich, T., and Papacek, M. (2010). Effect of population density on the development of Mesovelia furcata (Mesoveliidae), Microvelia reticulata and Velia caprai (Veliidae) (Heteroptera: Gerromorpha). Eur. J. Entomol. 107, 579–587. doi: 10.14411/eje.2010.067
Fairbairn, D., and Desranleau, L. (1987). Flight threshold, wing muscle histolysis, and alary polymorphism: correlated traits for dispersal tendency in the Gerridae. Ecol. Entomol. 12, 13–24. doi: 10.1111/j.1365-2311.1987.tb00980.x
Fairbairn, D., and Preziosi, R. F. (1996). Sexual selection and the evolution of sexual size dimorphism in the water strider, aquarius remigis. Evolution 50, 1549–1559. doi: 10.1111/j.1558-5646.1996.tb03927.x
Fairbairn, D. J. (1988). Adaptive significance of wing dimorphism in the absence of dispersal-a comparative-study of wing morphs in the waterstrider, gerris-remigis. Ecol. Entomol. 13, 273–281. doi: 10.1111/j.1365-2311.1988.tb00357.x
Fairbairn, D. J., and King, E. (2009). Why do Californian striders fly? J. Evol. Biol. 22, 36–49. doi: 10.1111/j.1420-9101.2008.01619.x
Ivy, T. M., and Sakaluk, S. K. (2007). Sequential mate choice in decorated crickets: females use a fixed internal threshold in pre- and postcopulatory choice. Anim. Behav. 74, 1065–1072. doi: 10.1016/j.anbehav.2007.01.017
Kaitala, A. (1988). Wing muscle dimorphism: two reproductive pathways of the waterstrider GerrisThoracicus in relation to habitat instability. Oikos 53, 222–228. doi: 10.2307/3566066
Karlsson, B., and Johansson, A. (2008). Seasonal polyphenism and developmental trade-offs between flight ability and egg laying in a pierid butterfly. Proc. Biol. Sci. 275, 2131–2136. doi: 10.1098/rspb.2008.0404
Khila, A., Abouheif, E., and Rowe, L. (2012). Function, developmental genetics, and fitness consequences of a sexually antagonistic trait. Science 336, 585–589. doi: 10.1126/science.1217258
Kuntner, M., Coddington, J. A., and Schneider, J. M. (2009). Intersexual arms race? Genital coevolution in nephilid spiders (Araneae, Nephilidae). Evolution 63, 1451–1463. doi: 10.1111/j.1558-5646.2009.00634.x
Langellotto, G. A., Denno, R. F., and Ott, J. R. (2000). A trade-off between flight capability and reproduction in males of a wing-dimorphic insect. Ecology 81, 865–875. doi: 10.1890/0012-9658(2000)081[0865:ATOBFC]20.CO;2
Maddison, W. P., and Maddison, D. R. (2018). Mesquite: A Modular System for Evolutionary Analysis. Version 3.40. Available online at: http://mesquiteproject.org (accessed November, 2018)
Mank, J. E. (2017). The transcriptional architecture of phenotypic dimorphism. Nat. Ecol. Evol.1, 6. doi: 10.1038/s41559-016-0006
Mank, J. E., Wedell, N., and Hosken, D. J. (2013). Polyandry and sex-specific gene expression. Philos. Trans. R. Soc. Lond. B Biol. Sci. 368, 20120047. doi: 10.1098/rstb.2012.0047
Markow, T. A., Bustoz, D., and Pitnick, S. (1996). Sexual selection and a secondary sexual character in two Drosophila species. Anim. Behav. 52, 759–766. doi: 10.1006/anbe.1996.0220
Metscher, B. D. (2009). MicroCT for comparative morphology: simple staining methods allow high-contrast 3D imaging of diverse non-mineralized animal tissues. BMC Physiol. 9:11. doi: 10.1186/1472-6793-9-11
Moreira, F. F. F., and Ribeiro, J. R. I. (2009). Two new Rhagovelia (Heteroptera: Veliidae) and new records for twelve species in southeastern Brazil. Aquat. Insects 31, 45–61. doi: 10.1080/01650420802444449
Paradis, E., Claude, J., and Strimmer, K. (2004). APE: analyses of phylogenetics and evolution in r language. Bioinformatics 20, 289–290. doi: 10.1093/bioinformatics/btg412
Pennell, T. M., and Morrow, E. H. (2013). Two sexes, one genome: the evolutionary dynamics of intralocus sexual conflict. Ecol. Evol. 3, 1819–1834. doi: 10.1002/ece3.540
Perry, J. C., and Rowe, L. (2018). Sexual conflict in its ecological setting. Philos. Trans. R. Soc. Lond. B Biol. Sci. 373. doi: 10.1098/rstb.2017.0418
Polak, M., Starmer, W. T., and Wolf, L. L. (2004). Sexual selection for size and symmetry in a diversifying secondary sexual character in Drosophila bipectinata duda (Diptera: Drosophilidae). Evolution 58, 597–607. doi: 10.1111/j.0014-3820.2004.tb01682.x
Polhemus, D. A. (1997). Systematics of the Genus Rhagovelia Mayr (Heteroptera: Veliidae) in the Western Hemisphere (Exclusive of the Angustipes Complex). Lanham, MD: Entomological Society of America.
Polhemus, D. A., and Chordas, S. W. III. (2010). A new species of Rhagovelia in the armata group from Belize (Heteroptera: Veliidae). Aquat. Insects 32, 135–142. doi: 10.1080/01650421003649182
Revell, L. J. (2012). phytools: an R package for phylogenetic comparative biology (and other things). Methods Ecol. Evol. 3, 217–223. doi: 10.1111/j.2041-210X.2011.00169.x
Roff, D. A., and Fairbairn, D. (1991). Wing dimorphisms and the evolution of migratory polymorphisms among the insecta. Am. Zool. 31, 243–251. doi: 10.1093/icb/31.1.243
Rowe, L., and Arnqvist, G. (2002). Sexually antagonistic coevolution in a mating system: combining experimental and comparative approaches to address evolutionary processes. Evolution 56, 754–767. doi: 10.1111/j.0014-3820.2002.tb01386.x
Rowe, L., Arnqvist, G., Sih, A. J., and Krupa, J. (1994). Sexual conflict and the evolutionary ecology of mating patterns: water striders as a model system. Trends Ecol. Evol. 9, 289–293. doi: 10.1016/0169-5347(94)90032-9
Rowe, L., and Day, T. (2006). Detecting sexual conflict and sexually antagonistic coevolution. Philos. Trans. R. Soc. Lond. B Biol. Sci. 361, 277–285. doi: 10.1098/rstb.2005.1788
Santos, M. E., Le Bouquin, A., Crumière, A. J. J., and Khila, A. (2017). Taxon-restricted genes at the origin of a novel trait allowing access to a new environment. Science 358, 386–390. doi: 10.1126/science.aan2748
Simmons, L. W., and Emlen, D. J. (2006). Evolutionary trade-off between weapons and testes. Proc. Natl. Acad. Sci. U.S.A. 103, 16346–16351. doi: 10.1073/pnas.0603474103
Snook, R. R., Bacigalupe, L. D., and Moore, A. J. (2010). The quantitative genetics and coevolution of male and female reproductive traits. Evolution 64, 1926–1934. doi: 10.1111/j.1558-5646.2010.00958.x
Spence, J. R. (1989). The habitat templet and life-history strategies of Pond Skaters (Heteroptera, Gerridae)-reproductive potential, phenology, and wing dimorphism. Can. J. Zool. Revue Can. De Zool. 67, 2432–2447. doi: 10.1139/z89-344
Spieth, H. T. (1952). Mating behavior within the genus Drosophila (Diptera). Bull. Am. Mus. Nat. Hist. 99, 401–474.
Tanaka, K., Barmina, O., Sanders, L. E., Arbeitman, M. N., and Kopp, A. (2011). Evolution of sex-specific traits through changes in HOX-dependent doublesex expression. PLoS Biol. 9:e1001131. doi: 10.1371/journal.pbio.1001131
Venables, W. N., and Ripley, B. D. (2002). Modern Applied Statistics with S. New York, NY: Springer. doi: 10.1007/978-0-387-21706-2
Keywords: sexual conflict, antagonistic armaments, coevolution, water striders, sexual dimorphism
Citation: Crumière AJJ, Armisén D, Vargas-Lowman A, Kubarakos M, Moreira FFF and Khila A (2019) Escalation and Morphological Constraints of Antagonistic Armaments in Water Striders. Front. Ecol. Evol. 7:215. doi: 10.3389/fevo.2019.00215
Received: 09 March 2019; Accepted: 23 May 2019;
Published: 18 June 2019.
Edited by:
Jingchun Li, University of Colorado Boulder, United StatesReviewed by:
Jessica Goodheart, University of California, Santa Barbara, United StatesCopyright © 2019 Crumière, Armisén, Vargas-Lowman, Kubarakos, Moreira and Khila. This is an open-access article distributed under the terms of the Creative Commons Attribution License (CC BY). The use, distribution or reproduction in other forums is permitted, provided the original author(s) and the copyright owner(s) are credited and that the original publication in this journal is cited, in accordance with accepted academic practice. No use, distribution or reproduction is permitted which does not comply with these terms.
*Correspondence: Abderrahman Khila, YWJkZXJyYWhtYW4ua2hpbGFAZW5zLWx5b24uZnI=
†Present Address: Antonin Jean Johan Crumière, Section for Ecology and Evolution, Department of Biology, University of Copenhagen, Copenhagen, Denmark
David Armisén, INRA-Université Clermont-Auvergne, UMR 1095 GDEC, Clermont-Ferrand, France
Martha Kubarakos, Department of Pharmaceutical Sciences, University of Toronto, Toronto, ON, Canada
Disclaimer: All claims expressed in this article are solely those of the authors and do not necessarily represent those of their affiliated organizations, or those of the publisher, the editors and the reviewers. Any product that may be evaluated in this article or claim that may be made by its manufacturer is not guaranteed or endorsed by the publisher.
Research integrity at Frontiers
Learn more about the work of our research integrity team to safeguard the quality of each article we publish.