- 1Molecular Phytomedicine, University of Bonn, Bonn, Germany
- 2Business Unit Greenhouse Horticulture, Wageningen University and Research, Bleiswijk, Netherlands
Mirid predators, a special group of plant-feeding omnivorous predators, have become important biological control agents for pest control in greenhouse cropping systems. Their efficacy and behavior may potentially be affected by microorganisms that induce plant defenses or change plant quality. Here we studied the interaction between a root restricted endophytic non-pathogenic strain of Fusarium oxysporum (Fo162) in tomato plants, the greenhouse whitefly Trialeurodes vaporariorum (Westwood) and the plant-feeding mirid predator Macrolophus pygmaeus (Rambur). In the absence of prey, inoculation of tomato plants with the Fo162 endophyte significantly reduced the reproduction of M. pygmaeus compared to plants without the endophyte. In contrast, the population growth of M. pygmaeus was not affected by the Fo162 endophyte in the presence of whiteflies. Moreover, the combination of the predator and endophyte resulted in lower whitefly densities than the predator alone. Whitefly population development was not different between endophyte-inoculated and untreated plants. Thus, endophyte inoculation of tomato plants seems to shift the feeding preference of this omnivorous predator from plant consumption toward relatively more prey consumption, resulting in enhanced suppression of the herbivore. Moreover, the negative effect of the endophyte on M. pygmaeus reproduction could easily be eliminated by providing decapsulated cysts of Artemia franciscana Kellogg as a supplemental food source. Together, this suggests an overall net positive effect of the Fo162 endophyte on a preventive biological control strategy in tomato using M. pygmaeus. Besides the enhanced whitefly control, endophyte-inoculation of tomato plants both with or without the predator also resulted in a higher yield and a reduced number of fruits with blossom-end rot, a disorder caused by limitations in uptake and transport of calcium to the fruits. This suggests that the Fo162 endophyte is also involved in the acquisition of essential nutrients for the benefit for the plant. Since both the Fo162 endophyte and the predator M. pygmaeus can induce plant defense, further studies need to elucidate the exact mechanisms that occur when both organisms are present. Our findings confirm the importance of studying endophytes and induced plant responses in a multi-trophic context with herbivores and their natural enemies.
Introduction
Plant-feeding generalist predators, a specific group of true omnivores, have become important biological control agents in various cropping systems (Coll and Guershon, 2002; Dumont et al., 2018) and particularly in greenhouse crops (Messelink et al., 2012). Plant-feeding generalist predators of the family Miridae, also called zoophytophagous predators, are among the most important predatory bugs used for biocontrol. This family includes well-known species such as Macrolophus pygmaeus (Rambur), Nesidiocoris tenuis (Reuter), Dicyphus errans, and Dicyphus hesperus (Sanchez and Cassis, 2018). They perform well on plants with high trichome densities like tomato and eggplant and have become very successful for two reasons. The first reason is their ability to predate on several important pest species such as whiteflies (Montserrat et al., 2000), aphids (Alvarado et al., 1997), spider mites (Hansen et al., 1999), leaf miners (Arnó et al., 2003) and Lepidopterans, including the important South American moth Tuta absoluta (Urbaneja et al., 2009; Ingegno et al., 2013, 2019). The second reason is their capacity to persist in crops prior to pest invasions by feeding on alternative food and plant resources (Perdikis et al., 2011; Messelink et al., 2014). Preventive releases or “crop inoculations” with generalist omnivorous predators have proven to be very effective for controlling pests that infest crops later in the growing season (Calvo et al., 2012; Messelink et al., 2015). Interestingly, the plant-feeding behavior of mirid predators can also induce plant defense responses by activating the jasmonic acid (JA) signaling pathways (Pappas et al., 2015; Perez-Hedo et al., 2015; Zhang et al., 2018b). Previous exposure of tomato plants to M. pygmaeus caused an increase in mortality and decrease of egg production of the two-spotted spider mite Tetranychus urticae Koch (Pappas et al., 2015). Similarly, in sweet pepper plants, previous feeding by these mirid bugs reduced the reproduction of both western flower thrips Frankliniella occidentalis (Pergande) and T. urticae (Zhang et al., 2018b). Moreover, activation of the JA pathway by mirid predators has been shown to attract the whitefly parasitoid Encarsia formosa (Gahan) and repel some pest species in tomato and sweet pepper plants (Perez-Hedo et al., 2015; Bouagga et al., 2017; Zhang et al., 2018a).
Induced plant responses or plant quality can also influence the feeding behavior of omnivores themselves. Induced plant responses caused a shift in the feeding preferences of the omnivorous thrips F. occidentalis from plant feeding to prey feeding (spider mite eggs) (Agrawal et al., 1999). Studies with host plants from different quality confirmed that low host plant quality increases prey consumption of this omnivore (Magalhães et al., 2005).
A perhaps largely overlooked factor by which plant-feeding predators can be influenced is the effect of microorganisms proliferating inside plants on induced plant defenses and plant quality (Schouten, 2016; Van Overbeek and Saikkonen, 2016). Fungal and bacterial species that are capable of colonizing the root cortex and/or other plant tissues without provoking visual disease symptoms are generally referred to as endophytes (Wilson, 1995). Such endophytes often have beneficial effects on plant performance (Clay and Schardl, 2002; Waller et al., 2005; Rodriguez et al., 2009; Schouten, 2016). Besides increasing nutrient uptake, endophytes may enhance resistance against plant pathogens and herbivores through changes in defensive chemistry (Alabouvette et al., 1993; Vidal, 1996; Backman and Sikora, 2008; Vega et al., 2009; Schouten, 2016). However, these endophyte-generated effects on herbivores can be versatile, depending on plant species, endophytic strain, and the feeding-mode and degree of specialism of the herbivore (Hartley and Gange, 2009).
Besides the herbivores themselves also their natural enemies can be affected by endophytes, and this has been reported for both parasitoids and predators (Omacini et al., 2001; Bultman et al., 2009; Hartley and Gange, 2009; Saari et al., 2014; Garantonakis et al., 2018; Pappas et al., 2018). Plant-mediated effects of endophytes on natural enemies may increase in complexity when the natural enemies are plant-feeding. To date, it is not known if and how endophytes can affect such multitrophic food-web interactions between plants, herbivores and plant-feeding natural enemies. Here we studied how the presence of a root-restricted non-pathogenic endophytic strain of Fusarium oxysporum in tomato plants affects the performance of the greenhouse whitefly Trialeurodes vaporariorum (Westwood) and its control by the plant-feeding mirid predator M. pygmaeus. When inducing plant defense responses or altering plant quality, the endophyte may directly affect the survival and/or reproduction of whiteflies and mirid predators. Moreover, the endophyte may indirectly affect whitefly densities by changing the feeding behavior of the plant-feeding predator. The results of our study may be interesting for both understanding endophyte-mediated multitrophic interactions and for further developing biological pest control strategies.
Materials and Methods
Plants, Insects, and Endophyte
All tomato plants (Lycopersicum esculentum L. cv. Capricia, Rijk Zwaan, The Netherlands) for the greenhouse trials were grown in rockwool blocks in a greenhouse compartment with an average temperature of 20°C and a relative humidity of 70%. The predator M. pygmaeus was obtained from Koppert Biological Systems (Berkel & Rodenrijs, The Netherlands) and reared for one generation on tomato plants (cv. Capricia) supplemented with frozen eggs of the flour moth Ephestia kuehniella Zeller. The greenhouse whitefly, T. vaporariorum was reared on tomato plants (cv. Capricia) in small greenhouse compartments. The fungal endophyte used in this study was the non-pathogenic strain Fo162 of Fusarium oxysporum, which was isolated from the cortical tissue of surface-sterilized tomato roots from Kenya (Hallmann and Sikora, 1994). This strain is known to induce plant defense responses in tomato (Bogner et al., 2017) and previous studies have shown effects on phloem feeding insects (Martinuz et al., 2012; Menjivar et al., 2012). Endophyte inoculum was produced on solid potato dextrose agar (PDA) in petri dishes.
Population Dynamics and Tomato Yield
The effect of the endophyte Fo162 on greenhouse whitefly population dynamics was tested both in the presence and in the absence of the predator M. pygmaeus in a greenhouse trial on tomato plants. The four treatment combinations [(1) untreated, (2) the endophyte, (3) the predator, and (4) the endophyte + predator] were organized in a randomized complete block design with 5 replicates: Twenty plants of 6 weeks old were placed individually in cylindrical insect cages (1.6 m diameter, 3 m high) made of fine insect gauze (mesh size 0.22 × 0.31 mm) in a greenhouse compartment of 98 m2 at the Institute of Wageningen University & Research, BU Greenhouse Horticulture in Bleiswijk, the Netherlands. Each plant was placed on a 1 m rockwool slab and supplied with nutrients through drip irrigation without recirculation in order to prevent contamination with endophyte spores among treatments. The nutrient solution was specifically developed for tomato (Sonneveld and Voogt, 2009), which was composed by the following recipe: macronutrient (mM): [Ca(NO3)2.4H2O.0.2NH4NO3] (5.4); KNO3 (6.0) KH2PO4.2H2O (1.5); K2SO4 (2.0); MgSO4.7H2O (2.4); CaCl2.2H2O (1.5); and micronutrients (μM): H3BO3 (25); MnSO4.4H2O (15); ZnSO4.7H2O (5); CuSO4.5H2O (1.5); NaMoO4.2H2O (1.5); and FeDTPA (30). Plants were grown according to standard cultivation methods: each plant was allowed to grow up along a rope attached to the top of the cage (3 m high), which was extended as soon as the plants reached the top of the rope. The plants were subsequently rotated when this stage was reached and the lower leaves were picked to fit the plant in the cage. Picked leaves were left in the cages to allow the remaining predators and whiteflies to move back to the plant. Half of the treatments were inoculated with the endophyte by pipetting 106 spores (harvested from a culture on PDA and solved in 3 ml of water) near the roots in the rockwool blocks right after planting the tomato plants. This procedure was repeated after one week. Five days after the second endophyte application, 6 female and 6 male predatory bugs were introduced per cage in 2 of the 4 treatments. Two weeks after the second endophyte inoculation twenty female whiteflies of mixed age were released per cage in all treatments. Densities of whitefly eggs, larvae and pupae were assessed at 2, 5, 8, and 11 weeks, and predators at 3, 6, 9, and 12 weeks, after their release respectively. Cages were entered through a zip opening and closed again before assessing the plants. Numbers of predators (nymphs and adults) were counted on the whole plant while carefully turning leaves and disturbing the predators as less as possible. The densities of whitefly juveniles were assessed by picking each time 6 terminal leaflets per plant: 3 from the bottom and 3 from the top, and counting all eggs, larvae and pupae under a binocular microscope. Prior to removing the leaves from the cage, adult whiteflies, as well as all M. pygmaeus nymphs and adults, were shaken from the leaves. Tomato fruits were harvested when they were ripe and the number of fruits were counted each time after harvesting. The first tomatoes were ripening 9 weeks after planting. Fruit weight was not measured, but the harvested tomatoes were in general similar sized which makes it plausible to use the number of tomatoes as approximation for the yield. Some of the fruits showed dark rotting spots at the bottom, also referred to as blossom-end rot, which is a physiological disorder caused by a calcium imbalance within the plant (Ho et al., 1993). The number of tomatoes affected by blossom-end rot were counted separately from “healthy” tomatoes. Temperature and relative humidity were registered every 5 min throughout the experiment with a climate recorder (Hoogendoorn Growth Management) inside one of the cages. The experiment was conducted in March-June, and during that period the average daily temperature and relative humidity in the cages was 20.0°C (range 18.2–21.7°C) and 62% (range 47–77%), respectively. Plants were grown under natural light conditions.
Predator Survival and Reproduction
A separate greenhouse experiment was set-up to assess effects of the endophyte Fo162 on the survival and reproduction of M. pygmaeus on plants in the absence of prey. Moreover, it was tested whether potential negative effects of the endophyte can be compensated with supplemental food consisting of decapsulated cysts of Artemia franciscana Kellogg (obtained from Smulders wholesale, Artemia quick HS aqua, Ulestraten, The Netherlands). These decapsulated cysts are known to be a good alternative food source for M. pygmaeus (Vandekerkhove et al., 2009). All four combinations of endophyte and alternative food were tested in a two-factorial randomized complete block design containing 5 replicates. Five-week old tomato plants (cv. Capricia) were placed individually in insect cages (60*60*90 cm, mesh size 0.5 mm) in a greenhouse compartment similar to the one used in the previous experiment. Nutrients were supplied through drip irrigation without recirculation in order to prevent contamination with endophyte spores among treatments. Fertilization was similar to the previous experiment. Also endophyte inoculation was performed in the same way as in the population dynamic experiment. One week after the second endophyte inoculation, twelve couples of 1-week-old M. pygmaeus males and females were introduced in each cage. The supplemental food was added weekly by dusting 0.5 g of A. franciscana cysts with a brush over the entire plant. Twenty-five days after their release, all juvenile and adult predators present on each plant were collected with an aspirator, counted and stored in 70% ethanol. Subsequently, the number of nymphs were counted separately per stage in the laboratory under a binocular microscope (40x) after putting the individuals on a white filter paper. Temperature and relative humidity in the greenhouse compartment were recorded every 5 min throughout the experiment with the same climate recorder as the one used in the population dynamic experiment. The experiment was conducted in September-October and the average daily temperature and relative humidity in the greenhouse during the presence of the predators was 19.4°C (range 18.5–21.2°C) and 74% (range 69–79%), respectively. Natural light was supplemented with 9000 lux artificial light for 2 hours per day during the last 4 weeks of the trial. Artificial lights were also switched on during the day when the natural light intensity was below 300 Watt.
Statistical Analyses
All whitefly and predator density data in the population dynamic trial were log (+1) transformed to adjust the data for homogeneity of variance and normality, followed by a repeated measures ANOVA, with predator and endophyte presence as factors. Differences among treatments were analyzed by Fisher's Least Significant Difference (LSD) post hoc tests (p < 0.05). Differences among treatments in the total number of produced tomato fruits per plant and the percentage of tomato fruit with blossom-end rot was analyzed with a two-way ANOVA with predator and endophyte as factors. Fruit numbers were log (+1) transformed and fractions of fruit with blossom-end rot arcsine transformed prior to this analyses to meet the requirements for a normal distribution of the data, followed by Fisher's LSD post hoc tests (p < 0.05). A two-way ANOVA was also used to analyse the data of the predator survival and reproduction trial, with endophyte and supplemental food application as the two factors. Because a faster juvenile development would result in a larger proportion of the older nymphal stages, we also looked at differences in the proportion of third nymphal stages among treatments (the oldest nymphal stage found after 25 days). The proportion of surviving adults and third nymphal stages were arcsin transformed and the total number of offspring were log transformed to get a normal distribution of the data prior the ANOVA analyses, followed by Fisher's LSD post hoc tests (p < 0.05). All statistical analyses were performed using the statistical package GenStat, Release 19.1.
Results
Population Dynamics and Tomato Yield
We found a significant interaction effect between the endophyte and the M. pygmaeus treatment on the number of whiteflies over time [F(1, 16) = 4.68, p = 0.046]. The factor predator alone was statistically significant [F(1, 16) = 182.49, p < 0.001], whereas the factor endophyte alone was not significant [F(1, 16) = 1.4, p = 0.255]. The endophyte treatments had no significant effect on whitefly population development, but combining the two factors enhanced control of whiteflies significantly compared to the treatment with predators alone (Figure 1A). In other words, the endophyte showed an effect on whiteflies only in the presence of predators. Overall predator densities were not significantly different on plants inoculated with the endophyte compared to untreated plants [F(1, 8) = 1.29, p = 0.289, Figure 1B]. The factor predator did not have a significant effect on both the total number of produced tomato fruits per plant [F(1, 16) = 1.19, p = 0.292] and the number of fruits with blossom-end rot [F(1, 16) = 0.05 p = 0.825], neither was the interaction with the endophyte factor significant. After removing the predator factor from the model, the factor endophyte did show a significant effect on both the total number of produced tomato fruits per plant [F(1, 18 = 4.95, p = 0.039] and the number of fruits with blossom-end rot [F(1, 18) = 4.54, p = 0.047]. There were no differences among treatments in the appearance of the first ripe fruits, so this was not further analyzed. Plants treated with the Fo162 endophyte gave a significant higher yield (numbers of fruit) and the tomato fruits were less affected by blossom-end rot (Figures 2A,B).
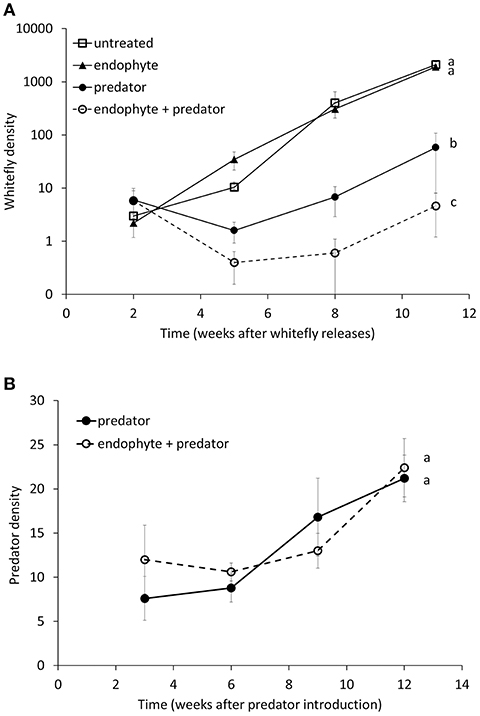
Figure 1. Population dynamics of (A) the greenhouse whitefly Trialeurodes vaporariorum and (B) the predator Macrolophus pygmaeus on tomato plants treated with the endophyte Fo162, the predator M. pygmaeus, both, or none of them (untreated). Predators were added 1 week and the endophyte inoculations were done 2 and 3 weeks prior to the whitefly release, respectively. Data shown are the mean (± SE) (Log) densities of juvenile whiteflies per 6 leaflets and predator densities per plant. Different letters next to the curves indicate overall significant differences among treatments (Fisher's LSD-tests, p < 0.05).
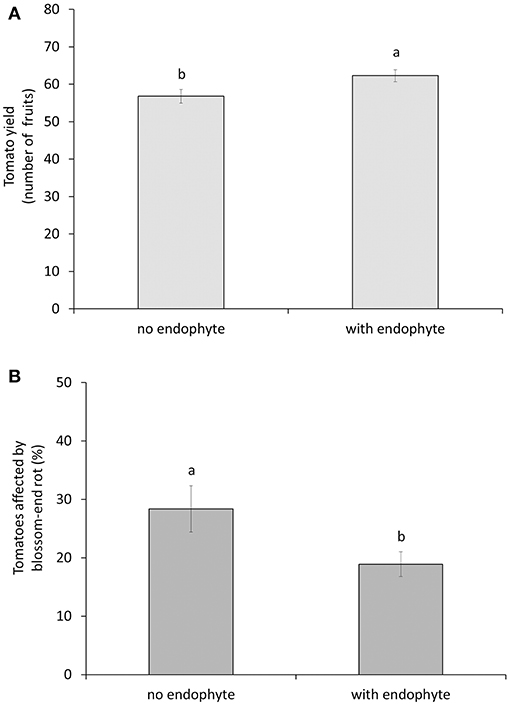
Figure 2. Total number (±SE) of fruits (A) and the percentage (±SE) of these fruits with blossom-end rot (B) from tomato plants without or with inoculation of the endophyte Fo162. Different letters above bars indicate significant differences between treatments (ANOVA, p < 0.05).
Predator Survival and Reproduction
The survival of M. pygmaeus adults 25 days after their release on plants was not significantly affected by the factor endophyte [F(1, 16) = 0.3, p = 0.593], nor was the interaction between supplemental food application and endophyte treatment significant [F(1, 16) = 3.15, p = 0.095]. In contrast, the application of supplemental food increased survival significantly [F(1, 16) = 26.11, p < 0.001, Figure 3A]. The total number of offspring was significantly affected by the interaction of the factors endophyte and supplemental food [F(1, 16) = 10.08, p = 0.006]. In the absence of supplemental food, the endophyte reduced the predator's offspring, but this negative effect of the endophyte completely disappeared when Artemia was provided as supplemental food source (Figure 3B). The juvenile stages present at the day of assessment all belonged to the first, second and third nymphal stage (Figure 3B). The application of supplemental food also enhanced juvenile developmental time, since a significantly larger proportion of third nymphal stages were present in these treatments than in the treatments without food [F(1, 16) = 9.71, p = 0.007]. Juvenile developmental time, reflected by the proportion of third nymphal stages, was not affected by the factor endophyte [F(1, 16) = 2.2, p = 0.157], nor was the interaction between the factors endophyte and food significant [F(1, 16) = 0, p = 0.974].
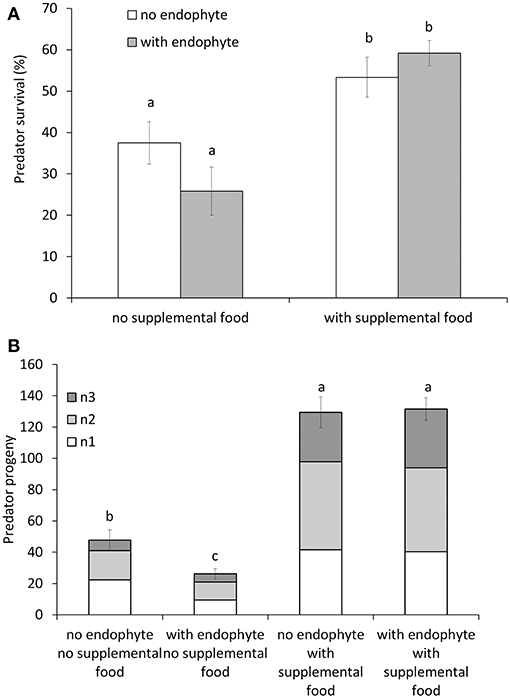
Figure 3. Survival (A) and reproduction (B) of the predator Macrolophus pygmaeus on tomato plants treated with the endophyte Fo162, the supplemental food source Artemia franciscana or a combination of both compared to untreated plants. Shown is the mean (±SE) percentage of survival of 24 released adult predators 2 weeks after their introduction (A) and the mean (±SE) total number of first, second and third nymphal stages (n1, n2, n3) produced (B). Different letters above bars indicate significant differences among treatments (Fisher's LSD-tests, p < 0.05).
Discussion
Here we show for the first time that plants inoculated with a root-associated endophyte can shift the feeding preference of an omnivorous predator from plant feeding toward relatively more prey consumption, resulting in enhanced suppression of the herbivore. The presence of the endophyte Fo162 can thus further improve the whitefly control efficacy of the already rather effective predation by M. pygmaeus. This enhanced control is remarkable, because reproduction of the predator was significantly reduced on endophyte treated plants without prey or supplemental food, and we also observed a clear trend (although statistically not significant) of lower adult survival on endophyte-treated plants compared to untreated plants. Thus, this negative effect of the endophyte on predator reproduction shifted to a positive effect in terms of pest control, because of the predator's flexible feeding behavior. In the presence of whiteflies, the predator population remained unaffected by the endophyte. Providing Artemia cysts as a supplemental food source also eliminated the negative effect of the endophyte on predator survival and reproduction. Hence, providing these supplemental food sources could be used to increase predator populations in the absence of pests on endophyte inoculated plants. Plant feeding by M. pygmaeus was probably not completely abandoned on endophyte-treated plants because of the considerable amount of water they need for their extra-oral digestion when feeding on prey (Casta et al., 2011). Moreover, the predator M. pygmaeus is known to develop and reproduce on plants even without prey (Lykouressis et al., 2008), suggesting that plant nutrients form an important part of their diet (Portillo et al., 2012). It has been shown before that reduced host plant quality can induce a feeding shift of omnivores (Agrawal et al., 1999; Magalhães et al., 2005), but that such a shift from plant to prey feeding can be mediated by an endophyte was unknown.
An unexpected additional positive effect of the endophyte in this study was the reduction we observed in the number of fruits with blossom–end rot in endophyte-treated plants compared to plants without endophyte inoculation. Blossom-end rot is a disorder caused by limitations in uptake and transport of calcium to the fruits (Ho et al., 1993). The endophyte Fo162 apparently enhanced the uptake of essential nutrients, including calcium, which reduced the number of fruits with disorders. Moreover, the endophyte also increased the total number of produced fruits during the experimental time. This all indicates that the Fo162 F. oxysporum strain can be beneficial for the tomato plants in several ways, similar to what was observed for the Fo162-Arabidopsis interaction (Martinuz et al., 2015). It is generally accepted that, although many strains of F. oxysporum are notorious for being pathogenic (Gordon and Martyn, 1997), non-pathogenic F. oxysporum strains can be beneficial for plants by suppressing Fusarium wilt disease (Ogawa and Komada, 1985; Postma and Rattink, 1992) and nematode infestations (Martinuz et al., 2013; Schouten, 2016). Overall, Fo162 not only positively affected the whitefly control by a plant-feeding predator, but also reduced blossom-end rot and increased yield.
As omnivorous predators also use plant nutrients for their development (Portillo et al., 2012), it may have seemed logical to expect a positive effect of endophyte-facilitated nutrient acquisition on the survival and reproduction of M. pygmaeus due to the expected better nutritional value of the plants. In fact, this has also been shown for studies with other root-colonizing fungi: tomato plants colonized by Trichoderma longibrachiatum promoted population growth of M. pygmaeus and were more attractive for these predators (Battaglia et al., 2013) and similar results were found for the arbuscular mycorrhizal fungus Rhizophagus irregularis (Prieto et al., 2017). Yet, in our study we found a negative effect of the endophyte on the reproduction of M. pygmaeus, and a positive effect on fruit quality and yield, indicating that other mechanisms than changes in nutritional value of the plant are involved as well. Other studies with the Fo162 endophyte showed this fungus may both induce plant defense responses and release various secondary metabolites by itself (Bogner et al., 2017), including indole acetic acid and 4-hydroxybenzoic acid, which may directly or indirectly have affected the survival, reproduction and feeding behavior of M. pygmaeus, similar to what was found for sedetary plant parasitic nematodes (Martinuz et al., 2013; Le et al., 2016).
In this study, we were not able to detect any effect of the endophyte on the whitefly population growth, whereas Menjivar et al. (2012) showed that the Fo162 endophyte negatively affected the feeding preferences of greenhouse whiteflies. This endophyte may be able to induce plant volatiles that influence the plant preference of whiteflies, but this study shows that it does not affect reproduction of whiteflies in a situation where the whiteflies were forced to feed on endophyte treated plants. These results suggest that due to being an obligate herbivore, whitefly may have adapted to certain levels of plant defenses in the course of co-evolution, e.g., by tolerating or degrading toxic secondary plant metabolites. Since M. pygmaeus is a zoophytophagus predatory bug, such selection pressure might be less pronounced or even absent in this species. At the same time, however, specialist natural enemies, like aphid parasitoids, can also experience a negative effect of endophytes through their host (Omacini et al., 2001). In general it has been observed that pests can adapt to plant defense responses, whereas their natural enemies still encounter negative effects (Ode, 2006). Another possible reason for the difference in endophyte effects on whiteflies and plant-feeding predators is their completely different mode of plant feeding. Whiteflies rarely damage mesophyll cells in their search for phloem sieve elements, thus remaining unaffected by defensive secondary metabolites stored in mesophyll cells (Walling, 2008). Omnivorous predatory bugs, however, use their stylets to lacerate the mesophyll cells, solubilizing cell contents and consuming the cellular slurry (Wheeler, 2001), and are therefore probably more exposed to the endophyte-induced plant defensive compounds. In addition to the endophyte induced plant defenses, the omnivorous predator may also induce defenses (Pappas et al., 2015). However, previous studies showed that the JA-signaling pathway induced by M. pygmaeus did not affect whitefly and aphid phloem feeders (Pappas et al., 2015; Zhang et al., 2018b). Thus, a synergistic effect of the predator induced and endophyte-induced plant defenses on whiteflies is not likely. However, how those two defense inducers interact is not known and deserves further studies.
Plant feeding by mirid predators can not only induce plant defenses, but in some cases also cause considerable plant damage (Casta et al., 2011; Puentes et al., 2018). Although M. pygmaeus is in general considered as a safe predator, it can cause serious plant damage at high predator levels and low prey densities (Sanchez et al., 2018). Greenhouse observations indicate this damage may even increase when plant are infected by the Pepino Mosaic Virus (Moerkens et al., 2016). More serious plant damage can be caused by the related omnivorous predator N. tenuis (Arnó et al., 2010), making this predator a controversial biological control agent (Pérez-Hedo and Urbaneja, 2016). The results of our study suggest that plant feeding by omnivorous predators can be reduced by endophytes, which may potentially also reduce crop damage caused by the predator's plant feeding. Indeed, another non-pathogenic Fusarium strain [Fusarium solani strain K (FsK)] was shown to reduce feeding damage by N. tenuis in young tomato plants (Garantonakis et al., 2018). Hence, this reduction of plant feeding damage by omnivorous predators might be another benefit of inoculating plants with fungal endophytes. In addition, a recent study by Pappas et al. (2018) showed increased attraction of M. pygmaeus to tomato plants that were colonized by this same endophyte (FsK), even in the absence of prey. This attraction to pest-free plants that are colonized by endophytes might result in an additional negative effect on M. pygmaeus reproduction. However, any negative effects of endophytes on population growth of omnivorous predators can probably be eliminated rather easily by providing supplemental food sources, as was shown in our study.
Overall, this work shows that the trophic level, on which an omnivorous predator operates can be influenced by a fungal endophyte. Our findings confirm the importance of studying endophytes and induced plant responses in a multi-trophic context with herbivores and their natural enemies, as unexpected interactions may occur (Bezemer et al., 2005; Kaplan and Thaler, 2011). Moreover, it confirms the importance of studying the impact of below-aboveground interactions on predator-pest interactions (Hooper et al., 2000; Van Der Putten et al., 2001, 2009; Wardle et al., 2004), even in simplified ecosystems as described by us. Changing plant quality by endophytes may also be a useful tool for enhancing biological pest control with omnivorous predators and reducing plant damage caused by omnivorous plant feeding.
Data Availability
The datasets generated for this study are available on request to the corresponding author.
Author Contributions
GM, JE, and AS designed the study. GM, JE, and RvH-S conducted the greenhouse trials. GM and HK analyzed the data. JE, HK, AS, and GM wrote the paper.
Conflict of Interest Statement
The authors declare that the research was conducted in the absence of any commercial or financial relationships that could be construed as a potential conflict of interest.
Acknowledgments
This study was funded by the European Regional Development Fund and part of the project Healthy greenhouse. JE would like to acknowledge Prof. Florian Grundler for his professional guidance and valuable support; Prof. Richard Sikora, who provided insight and expertise that greatly assisted the research and Gisela Sichtermann for her key support in the preliminary work.
References
Agrawal, A. A., Kobayashi, C., and Thaler, J. S. (1999). Influence of prey availability and induced host-plant resistance on omnivory by western flower thrips. Ecology 80, 518–523. doi: 10.1890/0012-9658(1999)080[0518:IOPAAI]2.0.CO;2
Alabouvette, C., Lemanceau, P., and Steinberg, C. (1993). Recent advances in the biological control of fusarium wilts. Pesticide Sci. 37, 365–373. doi: 10.1002/ps.2780370409
Alvarado, P., Balta, O., and Alomar, O. (1997). Efficiency of four heteroptera as predators of Aphis gossypii and Macrosiphum euphorbiae (hom.: Aphididae). Entomophaga 42, 215–226. doi: 10.1007/BF02769899
Arnó, J., Alonso, E., and Gabarra, R. (2003). Role of the parasitoid Diglyphus isaea (Walker) and the predator Macrolophus caliginosus Wagner in the control of leafminers IOBC/WPRS 26, 79–84.
Arnó, J., Castãné, C., Riudavets, J., and Gabarra, R. (2010). Risk of damage to tomato crops by the generalist zoophytophagous predator Nesidiocoris tenuis (Reuter) (Hemiptera: Miridae). Bull. Entomol. Res. 100, 105–115. doi: 10.1017/S0007485309006841
Backman, P. A., and Sikora, R. A. (2008). Endophytes: an emerging tool for biological control. Biol. Control 46, 1–3. doi: 10.1016/j.biocontrol.2008.03.009
Battaglia, D., Bossi, S., Cascone, P., Digilio, M. C., Prieto, J. D., Fanti, P., et al. (2013). Tomato below ground-above ground interactions: Trichoderma longibrachiatum affects the performance of Macrosiphum euphorbiae and its natural antagonists. Mol. Plant-Microbe Interactions 26, 1249–1256. doi: 10.1094/MPMI-02-13-0059-R
Bezemer, T. M., De Deyn, G. B., Bossinga, T. M., Van Dam, N. M., Harvey, J. A., and Van Der Putten, W. H. (2005). Soil community composition drives aboveground plant-herbivore-parasitoid interactions. Ecol. Lett. 8, 652–661. doi: 10.1111/j.1461-0248.2005.00762.x
Bogner, C. W., Kamdem, R. S. T., Sichtermann, G., Matthäus, C., Hölscher, D., Popp, J., et al. (2017). Bioactive secondary metabolites with multiple activities from a fungal endophyte. Microbial Biotechnol. 10, 175–188. doi: 10.1111/1751-7915.12467
Bouagga, S., Urbaneja, A., Rambla, J. L., Flors, V., Granell, A., Jaques, J. A., et al. (2017). Zoophytophagous mirids provide pest control by inducing direct defences, antixenosis and attraction to parasitoids in sweet pepper plants. Pest Manag. Sci. 74:1286–1296 doi: 10.1002/ps.4838
Bultman, T. L., Rodstrom, J. L., Radabaugh, K. R., Vandop, J. D., Librizzi, J. M., Longwell, L. L., et al. (2009). Influence of genetic variation in the fungal endophyte of a grass on an herbivore and its parasitoid. Entomologia Experimentalis Et Applicata 130, 173–180. doi: 10.1111/j.1570-7458.2008.00802.x
Calvo, F. J., Lorente, M. J., Stansly, P. A., and Belda, J. E. (2012). Preplant release of Nesidiocoris tenuis and supplementary tactics for control of Tuta absoluta and Bemisa tabaci in greenhouse tomato. Entomologia Experimentalis Et Applicata 143, 111–119. doi: 10.1111/j.1570-7458.2012.01238.x
Castañé, C., Arno, J., Gabarra, R., and Alomar, O. (2011). Plant damage to vegetable crops by zoophytophagous mirid predators. Biol. Control 59, 22–29. doi: 10.1016/j.biocontrol.2011.03.007
Clay, K., and Schardl, C. (2002). Evolutionary origins and ecological consequences of endophyte symbiosis with grasses. A. Naturalist 160, 99–127. doi: 10.2307/3079271Coll
Coll, M., and Guershon, M. (2002). Omnivory in terrestrial arthropods: mixing plant and prey diets. Ann. Rev. Entomol. 47, 267–297. doi: 10.1146/annurev.ento.47.091201.145209
Dumont, F., Aubry, O., and Lucas, E. (2018). From evolutionary aspects of zoophytophagy to biological control. Front. Ecol. Evol. 6:221. doi: 10.3389/fevo.2018.00221
Garantonakis, N., Pappas, M. L., Varikou, K., Skiada, V., Broufas, G. D., Kavroulakis, N., et al. (2018). Tomato inoculation with the endophytic strain Fusarium solani K results in reduced feeding damage by the zoophytophagous predator Nesidiocoris tenuis. Front. Ecol. Evol. 6:126. doi: 10.3389/fevo.2018.00126
Gordon, T. R., and Martyn, R. D. (1997). The evolutionary biology of Fusarium oxysporum. Ann. Rev. Phytopathol. 35, 111–128. doi: 10.1146/annurev.phyto.35.1.111
Hallmann, J., and Sikora, R. A. (1994). Occurrence of plant-parasitic nematodes and nonpathogenic species of Fusarium in tomato plants in Kenya and their role as mutualistic synergists for biological-control of root-knot nematodes. Int. J. Pest Manage. 40, 321–325. doi: 10.1080/09670879409371907
Hansen, D. L., Brødsgaard, H. F., and Enkegaard, A. (1999). Life table characteristics of Macrolophus caliginosus preying upon Tetranychus urticae. Entomologia Experimentalis Et Applicata 93, 269–275. doi: 10.1046/j.1570-7458.1999.00587.x
Hartley, S. E., and Gange, A. C. (2009). Impacts of plant Symbiotic Fungi on insect herbivores: mutualism in a multitrophic context. Ann. Rev. Entomol. 54, 323–342. doi: 10.1146/annurev.ento.54.110807.090614
Ho, L. C., Belda, R., Brown, M., Andrews, J., and Adams, P. (1993). Uptake and transport of calcium and the possible causes of blossom-end rot in tomato. J. Experiment. Botany 44, 509–518. doi: 10.1093/jxb/44.2.509
Hooper, D. U., Bignell, D. E., Brown, V. K., Brussaard, L., Dangerfield, J. M., Wall, D. H., et al. (2000). Interactions between aboveground and belowground biodiversity in terrestrial ecosystems: patterns, mechanisms, and feedbacks. Bioscience 50, 1049–1061. doi: 10.1641/0006-3568(2000)050[1049:IBAABB]2.0.CO;2
Ingegno, B. L., Ferracini, C., Gallinotti, D., Alma, A., and Tavella, L. (2013). Evaluation of the effectiveness of Dicyphus errans (Wolff) as predator of Tuta absoluta (Meyrick). Biol. Control 67, 246–252. doi: 10.1016/j.biocontrol.2013.08.002
Ingegno, B. L., Messelink, G. J., Bodino, N., Iliadou, A., Driss, L., Woelke, J. B., et al. (2019). Functional response of the mirid predators Dicyphus bolivari and Dicyphus errans and their efficacy as biological control agents of Tuta absoluta on tomato. J. Pest Sci. doi: 10.1007/s10340-019-01079-8
Kaplan, I., and Thaler, J. S. (2011). Do plant defenses enhance or diminish prey suppression by omnivorous Heteroptera? Biol. Control 59, 53–60. doi: 10.1016/j.biocontrol.2010.12.005
Le, H. T. T., Padgham, J. L., Hagemann, M. H., Sikora, R. A., and Schouten, A. (2016). Developmental and behavioural effects of the endophytic Fusarium moniliforme Fe14 towards Meloidogyne graminicola in rice. Ann. Applied Biol. 169, 134–143. doi: 10.1111/aab.12287
Lykouressis, D., Giatropoulos, A., Perdikis, D., and Favas, C. (2008). Assessing the suitability of noncultivated plants and associated insect prey as food sources for the omnivorous predator Macrolophus pygmaeus (Hemiptera : Miridae). Biol. Control 44, 142–148. doi: 10.1016/j.biocontrol.2007.11.003
Magalhães, S., Janssen, A., Montserrat, M., and Sabelis, M. W. (2005). Host-plant species modifies the diet of an omnivore feeding on three trophic levels. Oikos 111, 47–56. doi: 10.1111/j.0030-1299.2005.13897.x
Martinuz, A., Schouten, A., Menjivar, R. D., and Sikora, R. A. (2012). Effectiveness of systemic resistance toward Aphis gossypii (Hom., Aphididae) as induced by combined applications of the endophytes Fusarium oxysporum Fo162 and Rhizobium etli G12. Biol. Control 62, 206–212 doi: 10.1016/j.biocontrol.2012.05.006
Martinuz, A., Schouten, A., and Sikora, R. A. (2013). Post-infection development of Meloidogyne incognita on tomato treated with the endophytes Fusarium oxysporum strain Fo162 and Rhizobium etli strain G12. BioControl 58, 95–104. doi: 10.1007/s10526-012-9471-1
Martinuz, A., Zewdu, G., Ludwig, N., Grundler, F., Sikora, R. A., and Schouten, A. (2015). The application of Arabidopsis thaliana in studying tripartite interactions among plants, beneficial fungal endophytes and biotrophic plant-parasitic nematodes. Planta 241, 1015–1025. doi: 10.1007/s00425-014-2237-5
Menjivar, R. D., Cabrera, J. A., Kranz, J., and Sikora, R. A. (2012). Induction of metabolite organic compounds by mutualistic endophytic fungi to reduce the greenhouse whitefly Trialeurodes vaporariorum (Westwood) infection on tomato. Plant Soil 352, 233–241. doi: 10.1007/s11104-011-0991-8
Messelink, G. J., Bennison, J., Alomar, O., Ingegno, B. L., Tavella, L., Shipp, L., et al. (2014). Approaches to conserving natural enemy populations in greenhouse crops: current methods and future prospects. BioControl 59, 377-393. doi: 10.1007/s10526-014-9579-6
Messelink, G. J., Bloemhard, C. M. J., Hoogerbrugge, H., Van Schelt, J., Ingegno, B. L., and Tavella, L. (2015). Evaluation of mirid predatory bugs and release strategy for aphid control in sweet pepper. Journal of Applied Entomology 139, 333–341. doi: 10.1111/jen.12170
Messelink, G. J., Sabelis, M. W., and Janssen, A. (2012). “Generalist predators, food web complexities and biological pest control in greenhouse crops,” in Integrated pest management and pest control - current and future tactics, eds. M.L. Larramendy and S. Soloneski. (Rijeka: InTech), 191–214.
Moerkens, R., Berckmoes, E., Van Damme, V., Ortega-Parra, N., Hanssen, I., Wuytack, M., et al. (2016). High population densities of Macrolophus pygmaeus on tomato plants can cause economic fruit damage: interaction with Pepino mosaic virus? Pest Manage. Sci. 72, 1350–1358. doi: 10.1002/ps.4159
Montserrat, M., Albajes, R., and Casta,ñé, C. (2000). Functional response of four Heteropteran predators preying on greenhouse whitefly (Homoptera: Aleyrodidae) and western flower thrips (Thysanoptera : Thripidae). Environment. Entomol. 29, 1075–1082. doi: 10.1603/0046-225X-29.5.1075
Ode, P. J. (2006). Plant chemistry and natural enemy fitness: Effects on herbivore and natural enemy interactions. Annual Rev. Entomol. 51, 163–185. doi: 10.1146/annurev.ento.51.110104.151110
Ogawa, K., and Komada, H. (1985). Biological control of Fusarium wilt of sweet potato with cross-protection by prior inoculation with nonpathogenic Fusarium oxysporum. Jarq-Japan Agricultural Research Quarterly 19, 20–31.
Omacini, M., Chaneton, E. J., Ghersa, C. M., and Müller, C. B. (2001). Symbiotic fungal endophytes control insect host-parasite interaction webs. Nature 409, 78–81. doi: 10.1038/35051070
Pappas, M. L., Liapoura, M., Papantoniou, D., Avramidou, M., Kavroulakis, N., Weinhold, A., et al. (2018). The beneficial endophytic fungus Fusarium solani strain K alters tomato responses against spider mites to the benefit of the plant. Front. Plant Sci. 9:1603. doi: 10.3389/fpls.2018.01603
Pappas, M. L., Steppuhn, A., Geuss, D., Topalidou, N., Zografou, A., Sabelis, M. W., et al. (2015). Beyond predation: The zoophytophagous predator Macrolophus pygmaeus induces tomato resistance against spider mites. PLoS ONE 10:e0127251. doi: 10.1371/journal.pone.0127251
Perdikis, D., Fantinou, A., and Lykouressis, D. (2011). Enhancing pest control in annual crops by conservation of predatory Heteroptera. Biol. Control 59, 13–21. doi: 10.1016/j.biocontrol.2011.03.014
Perez-Hedo, M., Bouagga, S., Jaques, J. A., Flors, V., and Urbaneja, A. (2015). Tomato plant responses to feeding behavior of three zoophytophagous predators (Hemiptera: Miridae). Biol. Control 86, 46–51. doi: 10.1016/j.biocontrol.2015.04.006
Pérez-Hedo, M., and Urbaneja, A. (2016). “The zoophytophagous predator Nesidiocoris tenuis: a successful but controversial biocontrol agent in tomato crops,” in Advances in Insect Control and Resistance Management, eds. A.R. Horowitz & I. Ishaaya. (Cham: Springer International Publishing), 121–138. doi: 10.1007/978-3-319-31800-4_7
Portillo, N., Alomar, O., and Wackers, F. (2012). Nectarivory by the plant-tissue feeding predator Macrolophus pygmaeus Rambur (Heteroptera: Miridae): Nutritional redundancy or nutritional benefit? J. Insect Physiol. 58, 397–401. doi: 10.1016/j.jinsphys.2011.12.013
Postma, J., and Rattink, H. (1992). Biological control of Fusarium wilt of carnation with a nonpathogenic isolate of Fusarium oxysporum. Canad. J. Botany-Revue Canadienne De Botanique 70, 1199–1205. doi: 10.1139/b92-150
Prieto, J. D., Castane, C., Calvet, C., Camprubi, A., Battaglia, D., Trotta, V., et al. (2017). Tomato belowground-aboveground interactions: Rhizophagus irregularis affects foraging behavior and life history traits of the predator Macrolophus pygmaeus (Hemiptera: Miridae). Arthropod-Plant Interactions 11, 15–22. doi: 10.1007/s11829-016-9465-5
Puentes, A., Stephan, J. G., and Björkman, C. (2018). A systematic review on the effects of plant-feeding by omnivorous arthropods: time to catch-up with the mirid-tomato bias? Front. Ecol. Evol. 6:218. doi: 10.3389/fevo.2018.00218
Rodriguez, R. J., White, J. F., Arnold, A. E., and Redman, R. S. (2009). Fungal endophytes: diversity and functional roles. New Phytologist 182, 314–330. doi: 10.1111/j.1469-8137.2009.02773.x
Saari, S., Richter, S., Robbins, M., and Faeth, S. H. (2014). Bottom-up regulates top-down: the effects of hybridization of grass endophytes on an aphid herbivore and its generalist predator. Oikos 123, 545–552. doi: 10.1111/j.1600-0706.2013.00690.x
Sanchez, J. A., and Cassis, G. (2018). Towards solving the taxonomic impasse of the biocontrol plant bug subgenus Dicyphus (Dicyphus) (Insecta: Heteroptera: Miridae) using molecular, morphometric and morphological partitions. Zoolog. J. Linnean Soc. 184:330–406. doi: 10.1093/zoolinnean/zly005
Sanchez, J. A., López-Gallego, E., Pérez-Marcos, M., Perera-Fernández, L. G., and Ramírez-Soria, M. J. (2018). How safe is it to rely on Macrolophus pygmaeus (Hemiptera: Miridae) as a biocontrol agent in tomato crops? Frontiers in Ecology and Evolution 6:132. doi: 10.3389/fevo.2018.00132
Schouten, A. (2016). “Mechanisms involved in nematode control by endophytic fungi,” in Annual Review of Phytopathology, Vol 54, eds. J.E. Leach & S. Lindow. (Palo Alto, CA: Annual Reviews), 121–142. doi: 10.1146/annurev-phyto-080615-100114
Sonneveld, C., and Voogt, W. (2009). “Nutrient solutions for soilless cultures,” in plant nutrition of greenhouse crops, eds. C. Sonneveld & W. Voogt. (Dordrecht: Springer), 277–312. doi: 10.1007/978-90-481-2532-6_13
Urbaneja, A., Monton, H., and Molla, O. (2009). Suitability of the tomato borer Tuta absoluta as prey for Macrolophus pygmaeus and Nesidiocoris tenuis. J. Applied Entomol. 133, 292–296. doi: 10.1111/j.1439-0418.2008.01319.x
Van Der Putten, W. H., Bardgett, R. D., De Ruiter, P. C., Hol, W. H., Meyer, K. M., Bezemer, T. M., et al. (2009). Empirical and theoretical challenges in aboveground-belowground ecology. Oecologia 161, 1–14. doi: 10.1007/s00442-009-1351-8
Van Der Putten, W. H., Vet, L. E. M., Harvey, J. A., and Wackers, F. L. (2001). Linking above- and belowground multitrophic interactions of plants, herbivores, pathogens, and their antagonists. Trends Ecol. Evol. 16, 547–554. doi: 10.1016/S0169-5347(01)02265-0
Van Overbeek, L. S., and Saikkonen, K. (2016). Impact of bacterial-fungal interactions on the colonization of the endosphere. Trends Plant Sci. 21, 230–242. doi: 10.1016/j.tplants.2016.01.003
Vandekerkhove, B., Parmentier, L., Van Stappen, G., Grenier, S., Febvay, G., Rey, M., et al. (2009). Artemia cysts as an alternative food for the predatory bug Macrolophus pygmaeus. J. Applied Entomol. 133, 133–142. doi: 10.1111/j.1439-0418.2008.01332.x
Vega, F. E., Goettel, M. S., Blackwell, M., Chandler, D., Jackson, M. A., Keller, S., et al. (2009). Fungal entomopathogens: new insights on their ecology. Fungal Ecol.2, 149–159. doi: 10.1016/j.funeco.2009.05.001
Vidal, S. (1996). Changes in suitability of tomato for whiteflies mediated by a non-pathogenic endophytic fungus. Entomologia Experimentalis Et Applicata 80, 272–274. doi: 10.1111/j.1570-7458.1996.tb00933.x
Waller, F., Achatz, B., Baltruschat, H., Fodor, J., Becker, K., Fischer, M., et al. (2005). The endophytic fungus Piriformospora indica reprograms barley to salt-stress tolerance, disease resistance, and higher yield. Proceedings of the National Academy of Sciences U.S.A. 102, 13386–13391. doi: 10.1073/pnas.0504423102
Walling, L. L. (2008). Avoiding effective defenses: Strategies employed by phloem-feeding insects. Plant Physiol. 146, 859–866. doi: 10.1104/pp.107.113142
Wardle, D. A., Bardgett, R. D., Klironomos, J. N., Setälä, H., Van Der Putten, W. H., and Wall, D. H. (2004). Ecological linkages between aboveground and belowground biota. Science 304, 1629–1633. doi: 10.1126/science.1094875
Wheeler, J. G. (2001). Biology of the plant bugs (Hemiptera: Miridae) : pests, predators, opportunists. Ithaca, NY: Comstock Publishing Associates.
Wilson, D. (1995). Endophyte: The evolution of a term, and clarification of its use and definition. Oikos 73, 274–276. doi: 10.2307/3545919
Zhang, N. X., Messelink, G. J., Alba, J. M., Schuurink, R. C., Kant, M. R., and Janssen, A. (2018b). Phytophagy of omnivorous predator Macrolophus pygmaeus affects performance of herbivores through induced plant defences. Oecologia 186, 101–113. doi: 10.1007/s00442-017-4000-7
Keywords: biological control, endophytic fungi, multitrophic interactions, Macrolophus pygmaeus, Trialeurodes vaporariorum
Citation: Eschweiler J, van Holstein-Saj R, Kruidhof HM, Schouten A and Messelink GJ (2019) Tomato Inoculation With a Non-pathogenic Strain of Fusarium oxysporum Enhances Pest Control by Changing the Feeding Preference of an Omnivorous Predator. Front. Ecol. Evol. 7:213. doi: 10.3389/fevo.2019.00213
Received: 27 February 2019; Accepted: 22 May 2019;
Published: 06 June 2019.
Edited by:
George Broufas, Democritus University of Thrace, GreeceReviewed by:
Maria Cristina Digilio, University of Naples Federico II, ItalyAndreas Walzer, University of Natural Resources and Life Sciences Vienna, Austria
Apostolos Kapranas, Benaki Phytopathological Institute, Greece
Copyright © 2019 Eschweiler, van Holstein-Saj, Kruidhof, Schouten and Messelink. This is an open-access article distributed under the terms of the Creative Commons Attribution License (CC BY). The use, distribution or reproduction in other forums is permitted, provided the original author(s) and the copyright owner(s) are credited and that the original publication in this journal is cited, in accordance with accepted academic practice. No use, distribution or reproduction is permitted which does not comply with these terms.
*Correspondence: Gerben J. Messelink, Z2VyYmVuLm1lc3NlbGlua0B3dXIubmw=
†Present Address: Alexander Schouten, Laboratory of Phytopathology, Wageningen University and Research, Wageningen, Netherlands