- 1Biology Department, Siena Heights University, Adrian, MI, United States
- 2Department of Psychological Science, Boise State University, Boise, ID, United States
Almost all publications on the activity of animals have reported average patterns for the observed group or population and provided little information about the variability of these patterns in individual animals. As natural selection operates mainly on the level of individual animals, detailed data about the everyday life of single individuals and field data of high complexity are needed. This study provides low disturbance continuous recording of the diurnal/circadian activity cycle of individual fox squirrels (Sciurus niger) in a natural setting, which allowed us to document the intra-individual and inter-individual pattern variation over the seasons. The daily onsets and offsets of activity were inferred from recordings of skin temperature using iButtons attached to the necks of 14 individual squirrels over almost 4 years resulting in 25 continuous records totalling 1,353 days. All squirrels were clearly diurnal but varied greatly in the number of daily activity bouts showing predominantly unimodal and bimodal patterns. Variations in activity patterns were related mostly to the seasons, followed by the inter-individual variability between squirrels. Fox squirrels spent 66–68% of the day in their nests in spring, summer, and fall, and 77% in winter. In the same season, individual squirrels exited their nests at about the same time every morning. In summer, they left their nests about 3 h earlier in the morning than in winter. The timing of first exits was significantly correlated with the number of daily nest exits and total out-of-nest time (ONT), and both variables were significantly correlated with both photoperiod and daily maximum temperature. Squirrels studied simultaneously showed substantial day-to-day variability in both number of daily exits and total ONT that either matched or exceeded the inter-individual variability. Many squirrels left their nests occasionally during the night, while about half occasionally spent entire nights outside an insulated nest. The timing of nest entries and exits, the modality of the activity pattern, the daily proportion of rest, and the occurrence of activity during the typically inactive time of the activity cycle are all aspects of an animal's activity pattern. They should therefore receive more attention for the characterization of species-specific behavior.
Introduction
The appropriateness of an animal's activity level and pattern over the cycle of day and night and over the time course of the seasons is essential for its survival (DeCoursey et al., 2000; Larivée et al., 2010; Zhang et al., 2017) and reproductive success (Schmidt et al., 2008; Speakman, 2008; Zhao et al., 2013). The selective pressures to forage efficiently while avoiding injury and death lead to trade-offs in an animal's strategies between starvation and predation (Dall and Boyd, 2002; Higginson et al., 2012; Quinn et al., 2012; McNamara et al., 2016) that will affect both the daily proportions of time to be active and rest and the timing and regularity of rest and activity. Research on both aspects of activity is therefore essential to understand the biology of animal species. Locomotor activity has been shown to follow a reproducible daily pattern in numerous animal species, including tree squirrels (Tester, 1978; Adams, 1984; Koprowski and Corse, 2005; Wassmer and Refinetti, 2016). More than half a century ago, Aschoff (1966) observed that many, if not most, studies of locomotor activity had evinced a bimodal pattern with increased activity at dawn and dusk rather than a consolidated bout during the day or a consolidated bout during the night. Fox squirrels (Sciurus niger) are believed to be diurnal with bimodal activity pattern consisting of activity peaks in the early morning and right before sunset (Koprowski, 1994). However, most published data on tree squirrel species have reported average activity patterns for the observed group of animals or population and provided no indications about the variability of these activity patterns in individual animals (Hicks, 1949; Thompson, 1977; Wauters and Dhondt, 1987; Jodice and Humphrey, 1992; Wauters et al., 1992; Bordignon and Monteiro-Filho, 2000; Johnson et al., 2003; Koprowski and Corse, 2005; Ditgen et al., 2007). Only four studies so far have provided data on individual animals in tree squirrels (Zwahlen, 1975; Adams, 1984; Wassmer and Refinetti, 2016; Anders et al., 2017).
It has been suggested that researchers should move away from the “tyranny of the golden mean” (Bennett, 1987) and acknowledge individual behavioral differences as being more than just statistical noise around the group or population mean (Careau et al., 2008; Williams, 2008). This might help to shed light on how selection acts on the inter-individual variation in behavioral and physiological traits as evolution operates at the level of the individual (Roche et al., 2016). In studies on animals' activity cycles and patterns, it is the inter-individual variation in temporal strategies that provides the raw material for natural selection. The “golden mean” is averaging out this variation and obscures our understanding of the fitness impacts of frequency-dependent alternative temporal strategies. Therefore, detailed data about the everyday life of single individuals and field data of high complexity are needed (Halle and Stenseth, 2000).
Although not currently fully included into the concept of animal personalities (Gosling, 2001; Sih et al., 2004; Stamps, 2007; Wolf and Weissing, 2012), an animal's activity cycle and pattern is of essential ecological and evolutionary importance (Hertel et al., 2017) as it influences, e.g., its temporal selection/avoidance of open vs. protective habitats (Boon et al., 2008; Lone et al., 2015), movement rates (Ciuti et al., 2012), and dispersal (Cooper et al., 2017). Individual variation of activity cycles and patterns is also important at the population level because the degree of variation in temporal strategies renders populations to be more or less resilient to changing conditions (Wolf and Weissing, 2012; Dingemanse and Wolf, 2013). A related concept regarding the importance of activity patterns and cycles is the chronotype. Being originally developed as a concept in human psychology, classifying people along a continuum from “morning types” with a preference to develop daily routines during the first half of the day (also called “larks”) to “evening types,” who prefer daily routines during the second half of the day (also called “owls”) (Horne and Ostberg, 1976; Vink et al., 2001), chronotypes are now also used in comparative psychology (Labyak et al., 1997; Masuda and Zhdanova, 2010; Pfeffer et al., 2015; Refinetti et al., 2016). However, in almost all studies, averaged activity patterns are used and only some aspects of the activity pattern such as the activity onset, acrophase, and spread are considered (Refinetti et al., 2016). Despite a current renewal of attempts to combine chronobiology, which is traditionally conducted in laboratories, with field ecology (Helm et al., 2017), the linkage between the related concepts of personality and chronotype is currently underdeveloped. Exceptions are recent studies of human psychology and medicine finding that chronotype, sleep, and personality are linked and appear to have at least partial genetic foundation (Randler et al., 2017) and a study of circadian behavioral variation by the pearly razorfish, Xyrithchys novacula (Alós et al., 2017), in which the authors suggested that the observed circadian behavioral variation should be viewed as an independent axis of the fish personality, and that the study of chronotypes and their consequences should be regarded as a novel dimension in understanding within-species fish behavioral diversity.
The current study provides low disturbance continuous recording of several hitherto underrepresented parameters of the diurnal/circadian activity cycle [number of nest exits, in-nest time during daylight, total in-nest time during 24 h, nocturnal out-of-nest time (ONT) of individual fox squirrels (Sciurus niger)] in a natural setting. The goal of the present study was to use the recorded patterns of nest entries and exits to study the distribution of unimodal, bimodal, and higher-modal activity patterns and the total ONT during day and night in individual fox squirrels, document the intra-individual (day-to-day) and inter-individual pattern variation over the seasons, and address how the observed variabilities relate to the concepts of animal personalities and chronotype.
Materials and Methods
Location
The study was conducted on an 80-ha area encompassing the campus of Siena Heights University and the adjacent Motherhouse campus of the Adrian Dominican Sisters, in rural Adrian, Lenawee County, in southeastern Michigan, USA (41°54′ N, 84°01′ W, 240-m elevation). Long-term climate data for Adrian are shown in Table 1. Weather conditions during the study were recorded by the Siena Heights University weather station and uploaded to Weather Underground (https://www.wunderground.com/dashboard/pws/KMIADRIA4). Daily averages as well as high and low temperatures for the correlation matrix (Table 2) were retrieved for the Lenawee County Airport (KADG) also via Weather Underground (https://www.wunderground.com/history/airportfrompws/KADG/), while the information on snow fall amounts and snow heights originated from US Climate Data (https://www.usclimatedata.com/climate/adrian/michigan/united-states/usmi0004).
Animals
Fox squirrels were caught with double-door Tomahawk Deluxe Transfer traps (Tomahawk Live Traps, Hazelhurst, WI) permanently attached to the trunk of four tall trees on custom-made platforms (Huggins and Gee, 1995). Squirrels were weighed with Pesola spring scales and ear-tagged with Monel type 5 tags (National Band and Tag Company, Newport, KY). Age was categorized as juvenile, sub-adult, and adult according to weight and fur characteristics and by visual examination of external reproductive organs. Sex of adult and sub-adult squirrels was determined by visual examination of the external reproductive organs (Reighard et al., 2004; Schroeder and Robb, 2005). All work was conducted with approval of the Institutional Animal Care and Use Committee of Siena Heights University and followed appropriate guidelines as outlined by (Schmidt et al., 2008).
Data Collection
Each squirrel was equipped with a data-logger collar containing a temperature-sensitive iButton (DS1922L, Maxim Integrated Products, Inc., San Jose, CA) and released at the site of capture immediately thereafter. Data-logger collars weighed between 8 and 12 g and did not exceed 2% of the animals' body mass. The collar design is described in detail elsewhere (Wassmer, 2017). The monitoring of collar temperature (Tc) is particularly suited to provide an index of location (in-nest vs. out-of-nest), as the temperature of the collar quickly rises to core temperature levels after the animals enter their thermally insulated nests and drops rapidly when the animals leave their nests (Lamb, 1995; Kanda et al., 2005; Lazerte and Kramer, 2011; Murray and Smith, 2012; Wassmer and Refinetti, 2016). These assumptions were confirmed by aligning Tc-records with behavioral logs (Wassmer and Refinetti, 2016) including nest entries. A recent study using a very similar setup with a large population of North American red squirrels (Tamiasciurus hudsonicus) confirmed the paradigm with about 700 observations of nest exits and entries (Studd et al., 2016). The iButtons were programmed to record one Tc reading every 5, 10, or 30 min with a thermal accuracy of 0.5 or 0.06°C. The limited memory of the iButton allowed data to be recorded for a minimum of 28 days (at 5-min intervals) to a maximum of 6 months (at 30-min intervals). We obtained data from 25 distinct timeseries from 14 individual squirrels, across the four seasons (Figure 1). The corresponding ambient temperatures (Ta) at the times of Tc recordings were obtained by the nearby Siena Heights weather station (<500 m away from the squirrel habitat; see the URL above).
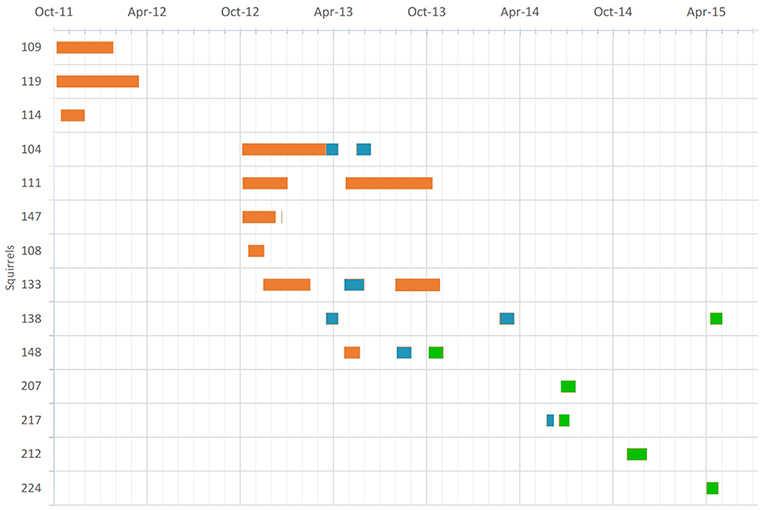
Figure 1. Temporal distribution of 25 individual time series over 14 individual squirrels (y-axis) tagged with thermo-sensitive dataloggers. Orange bars indicate 30-min recording intervals, teal indicates 5-min intervals, and green 10-min intervals.
Exact times of entry into and exit from the nest were often easily identified by visual inspection of collar temperature records. To avoid potential subjectivity bias, however, the time series was analyzed by a custom-made computer program developed by one of the authors (RR). The computer algorithm defined a nest entry as the data point when the current temperature was higher than the preceding temperature and also higher than or equal to the mean nightly temperature of the individual squirrel. Similarly, the algorithm defined a nest exit as the data point when the current temperature was lower than the preceding temperature and also lower than the mean nightly temperature of the individual squirrel.
Although this simple algorithm worked well for data sets with a temperature gradient of more than 10–15°C between Tc and Ta, additional specifications had to be included to ensure successful data analysis during warmer periods that resulted in more noisy data sets (e.g., Figure 9D). In addition, some collar preparations showed a lower amplitude of Tc fluctuations (e.g., Figure 10D), and we recognized that the recording interval (5, 10, and 30 min) has an influence on the selection of meaningful criteria. We therefore re-evaluated the algorithm used in Wassmer and Refinetti (2016) and established several sets of criteria for high/low amplitude, short/long intervals, and clear/noisy patterns (Table S1) to include the most recording days possible without compromising the event detection and shifting the detection frame, which would result in incorrect detections for many days after falsely determining an event (nest entry or exit).
The first nest exit on each day was defined as the onset of a squirrel's daily activity, whereas the last nest entry on each day was an indication of the daily activity offset. The number of nest exits each day was used to quantify the modality of the activity pattern for each squirrel. Other dependent variables were diurnal nest time (time a squirrel stayed inside of its nest during daylight hours), total nest time (in-nest time per 24 h), and nocturnal ONT (time spent outside of the nest during the night). For the analyses of seasonality, the meteorological definition of seasons was used: Summer starts June 1, lasting until August 31; fall runs from September 1 until November 30, followed by winter from December 1 through February 28, and finally the spring season lasting from March 1 to May 31.
Data Analysis
Presence of statistically significant 24-h rhythmicity was determined by two methods: chi-square periodogram (Sokolove and Bushell, 1978) and cosinor rhythmometry (Nelson et al., 1979). The daily and seasonal timing of nest exits and entries were analyzed and compared using circular statistics (Batschelet, 1981; Fisher, 1993; Mardia and Jupp, 2000). Standard statistical tests were used for comparisons of group means, standard deviations, and standard errors (Siegel and Castellan, 1988; Moore et al., 2013; Zar, 2018). Unless specifically stated, means are presented together with their standard deviation (mean ± SD).
We compared the intra-individual (day-to-day) variation to the inter-individual variation contrasting the standard errors of the means of two data sets of equal length assembled either from consecutive or randomly chosen days.
Results
General Results
In this study, we deployed 27 data-logger collars on 16 individual fox squirrels over almost 4 years. One of the collared animals was never seen again while the data-logger collars of four squirrels that were believed to have been lost were found a year later after the leaf nests of these squirrels deteriorated and the collars fell to the ground. Only the data of one of these collars were lost due to an exhausted iButton battery. In summary, we were able to analyze 25 individual time series of 14 individual animals (Figure 1, Table S2). All fox squirrels exhibited statistically significant 24-h rhythmicity (p < 0.0001) as determined by the chi-square periodogram procedure and by cosinor rhythmometry. Representative records for four fox squirrels are shown in Figure 2. For all 14 animals, neck temperature was consistently high (37–38°C) during the night, when the animals remained curled up inside their nests, but oscillated noticeably during the day, when the animals exited the nest one or more times a day. Although Ta oscillated daily, the daily variations in Tc were independent from variations in Ta (Figures 2, 9, 10). When correlating 2 weeks of recordings of Tc at a temporal resolution of 0.5 h (672 data points) to simultaneous recordings of Ta of equal duration and resolution, we found low negative correlations in winter (highest r = −0.061, p > 0.1) and to some extent higher negative correlations in summer (r = −0.139, p < 0.05). This indicates that Tc's were slightly higher when Ta's were lower.
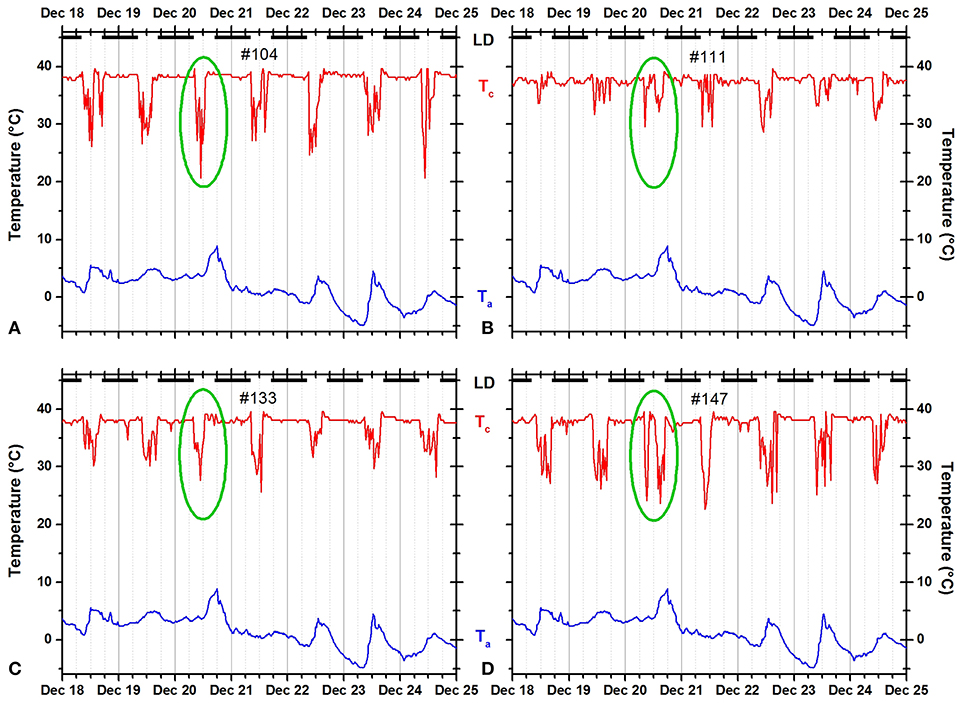
Figure 2. Records of ambient temperature (Ta, blue) and neck skin temperature (Tc, red) for four fox squirrels 104 (A), 111 (B), 133 (C), and 147 (D) living outdoors during the winter. The dark horizontal bars at the top of each panel indicate the duration of the dark phase of the natural light–dark cycle. The green ovals mark the time course of body temperature at the same day illustrating individual variability under the same weather conditions. Squirrels 104 and 133 showed a unimodal pattern, whereas squirrels 111 and 147 showed a bimodal pattern.
In the combined first-order effects on our dependent variables (number of daily nest exits, in-nest time during daylight hours, total daily in-nest time and ONT during night hours), season had the strongest effect [Wilks lambda = 0.287, F(21, 3, 808) = 98.9, p < 0.0001] followed by differences between individual squirrels [Wilks lambda = 0.565, F(84, 8, 129) = 9.4, p < 0.0001], age [Wilks lambda = 0.846, F(7, 1, 326) = 34.629, p < 0.0001], and recording interval [5, 10, or 30 min, Wilks lambda = 0.908, F(14, 2, 652) = 9.417, p < 0.00001]. Although a MANOVA revealed no main effect of sex (Wilks lambda = 1.0), it showed an interaction of sex and season [F(3, 1, 344) = 9.243, p < 0.0005].
Number of Nest Exits
In the pooled data, the average number of nest exits per day was 1.610 ± 0.788 suggesting a slightly higher proportion of bimodal and higher-modal days with two or more daily activity periods separated by one or more rest period in their nest (e.g., squirrels 111 and 147 in Figure 2). However, a histogram of the distribution of unimodal, bimodal, and higher-modal days for all 14 individual squirrels (Figure 3) shows that most squirrels had more unimodal than bimodal days, with the exceptions of squirrels 111 and 108. There were much fewer days during which the animals exited three or more times a day indicating fewer trimodal and even less polymodal activity patterns. Individual squirrels differed significantly in the mean number of exits ranging from 1.335 ± 0.537 to 1.875 ± 0.833.
Fox squirrels showed the highest number of nest exits in summer (1.982 ± 0.968) and least in winter (1.263 ± 0.489). All seasons were significantly different from each other except fall and spring (Tukey HSD test, p < 0.05). Individual squirrels were predominantly unimodal in winter with average numbers of nest exits between 1.157 and 2.000 and predominantly bimodal in summer with values between 1.519 and 3.115. Some animals showed more exits in fall and/or spring than in summer. Female fox squirrels showed significantly more nest exits in spring and summer (1.976 ± 0.886, 2.250 ± 1.223) than male squirrels [1.600 ± 0.722, 1.848 ± 0.781, F(1, 255) = 13.969, p < 0.0005, Figure 4]. On the contrary, there was no sex difference in nest exits in either fall or winter nor during the pooled data for these two seasons [F(1, 418) = 1.166, p = 0.281]. In addition to the seasonal and individual variability, the number of nest exits also showed considerable day-to-day fluctuations in individual squirrels recorded during the same days (Figure 5A).
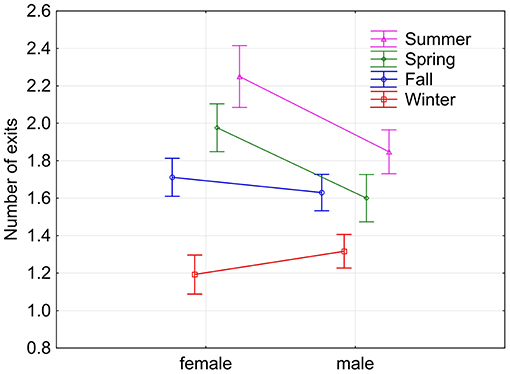
Figure 4. Means (and 95% confidence intervals) of the number of exits by female and male squirrels during the four seasons. Current effect: F(3, 1, 344) = 9.2425, p < 0.00001.
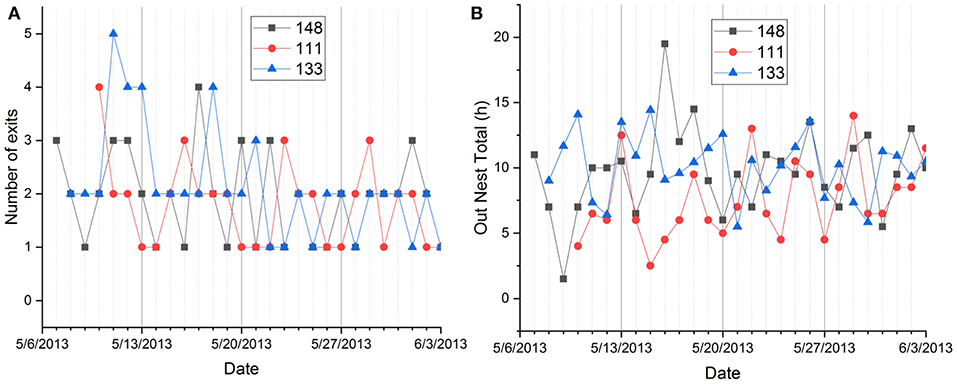
Figure 5. Day-to-day fluctuation of nest exits (A) and total out-of-nest time (B) in individual squirrels recorded during the same 28 days in spring and summer.
The number of nest exits showed comparable significant correlations to the daily hours of daylight (Pearson's r = 0.360, p < 0.001) and daily high temperatures (r = 0.345, p < 0.001). The correlation between the number of exits and snow depth was less pronounced but still significant, while average wind speed, precipitation, and snowfall were not significantly correlated (Table 2).
In-nest and Out-of-nest Times
From fall through summer, the animals spent increasingly longer periods inside of their nests during daytime, starting at 3.318 ± 2.485 h in fall to 7.430 ± 2.943 h in summer (Figure 6A). All seasons were significantly different from each other regarding time spent in the nest during light hours (Tukey HSD test, p < 0.05). Total daily time spent in the nest was highest in winter (18.466 ± 2.129 h) and lowest in spring (15.936 ± 3.060 h); however, only winter was significantly different from every other season (Tukey HSD test, p < 0.05, Figure 6B). The daily proportion of ONT during civil daylight hours ranged from 0% (observed only once) to 96% with an average of 43 ± 23%. Total nest time allows a rough estimate of the proportion of rest per 24 h. In the pooled data, squirrels spend about 66–68% of 24 h in their nests in spring, summer, and fall, and 77% in winter. This difference was significant (one-way ANOVA and Tukey post-test, p < 0.00001).
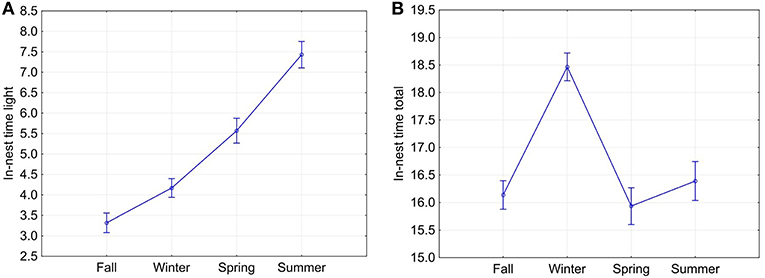
Figure 6. Means (and 95% confidence intervals) of the seasonal in-nest time during daylight (A) and in-nest time during the entire 24-h cycle of day and night (B). Current effect: Wilks lambda = 0.30196, F(6, 2, 694) = 368.09, p = 0.0000.
There were also significant differences between individual squirrels in nest time during daylight hours ranging from 1.698 ± 1.213 to 9.05 ± 2.981 h, total nest time ranging from 12.523 ± 1.899 to 18.488 ± 2.581 h, and nocturnal out of nest time ranging from 0.0285 ± 0.181 to 1.106 ± 2.035 h (Tukey HSD tests, p < 0.05). As nest exits, total ONT showed considerable day-to-day fluctuations in individual squirrels recorded during the same days (Figure 5B).
Total ONT was best correlated with the daily high temperatures (Pearson's r = 0.342) and less to the hours of daylight (r = 0.275). In addition, snow depth (r = −0.236) and snowfall (r = −0.176) were also significantly but less strongly correlated (all probabilities, p < 0.001), whereas average wind speed and precipitation did not seem to influence total ONT (Table 2).
Timing of First Exits
In all seasons, individual squirrels showed highly significant mean vectors for the timing of their first exits with mean vector lengths (r) between 0.76 and 0.99. Individual squirrels differed significantly in the timing of their first exits (Figure 7, Table 3). None of the animals was consistently an early or late riser throughout all seasons. In summer, fox squirrels left their nests 3 h earlier in the morning than in winter (Mardia–Watson–Wheeler test on the pooled data of all 14 animals, p < 0.05). The range between early and late risers was highest in summer (4.8 h) and lowest in winter (1.83 h). In summer, first exits of individual squirrels were, on average, 1.72 h apart from each other—in winter, the average spacing was only 37 min. Three individual fox squirrels (#104, 111, 133) were recorded throughout all seasons and could be compared to the pooled data. While #104 and #111 showed similar results to the pooled data with first exits in summer occurring about 4:37 and 3:42 h earlier than in winter, respectively, #133 showed different results with first exits earliest in fall (6:48 EST) and spring (7:36 EST) and late exits in summer (9:57 EST) and winter (10:11 EST) only about 0:14 h apart.
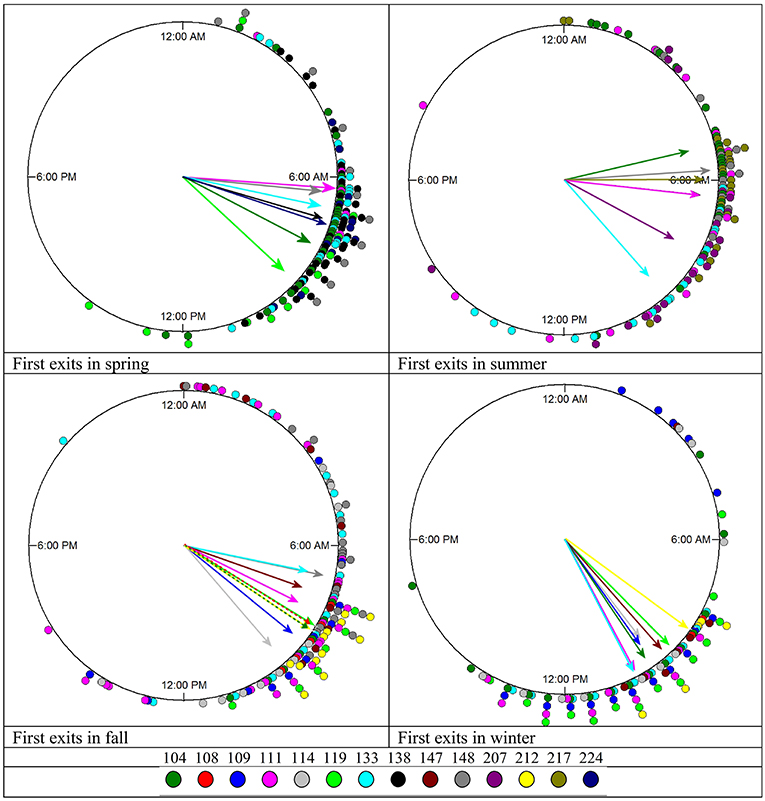
Figure 7. Timing of first exits in individual squirrels during each season. The mean vectors point at the average timing of first entries for each squirrel and are in the same color as the data points plotted on the periphery of the circle (see the color key on the bottom). The length of the mean vector indicates how concentrated or spread the first exits are around this mean time. The exact values can be found in Table 3.
In the pooled data for all seasons, the timing of first exits was inversely correlated with the number of daily exits (Pearson's r = −0.708, p = 0.005) and total time outside nests (Pearson's r = −0.685, p = 0.007), meaning that squirrels that exited the nest earlier also exited the nest more times each day and spent longer times outside the nest (Table 4, Figure 8). In addition to the results for the pooled seasons, total time spent outside the nest in fall was also significantly inversely correlated to the timing of first exits in fall.
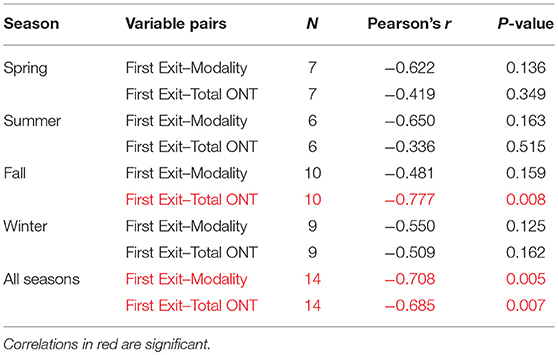
Table 4. Linear correlations between the timing of first exits and modality (number of nest exits per day) and timing of first exits and total time spent outside of the nest per 24 h (total ONT).
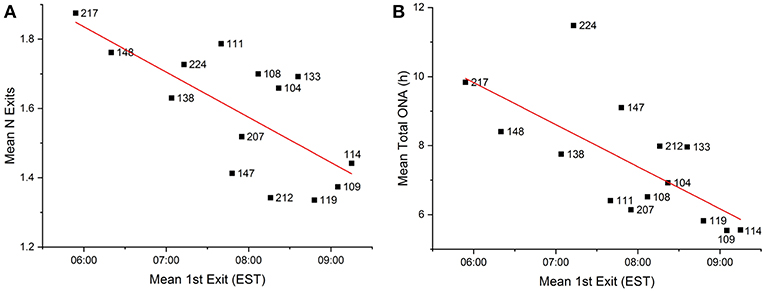
Figure 8. (A) Correlation between the mean timing of first exits and the mean number of exits (modality) of all recorded fox squirrel (numbers) in all seasons (Pearson's r = −0.708, p = 0.005). (B) Correlation between the mean timing of first exits and the mean total time spent outside the nest per 24 h of all recorded squirrels in all seasons of the year (Pearson's r = −0.685, p = 0.007).
Continuous Nest Occupancy for More Than 24 h and Nocturnal Out-of-nest Time
Some squirrels occasionally remained in the nest for more than 24 h and/or occasionally failed to return to the nest at night (Figures 9, 10). The green oval in Figure 9B documents that squirrel #104 did not leave the insulated nest for more than 36 h. In Figure 10B, the green oval marks a 35-h time period during which the animal was not resting in an insulated shelter.
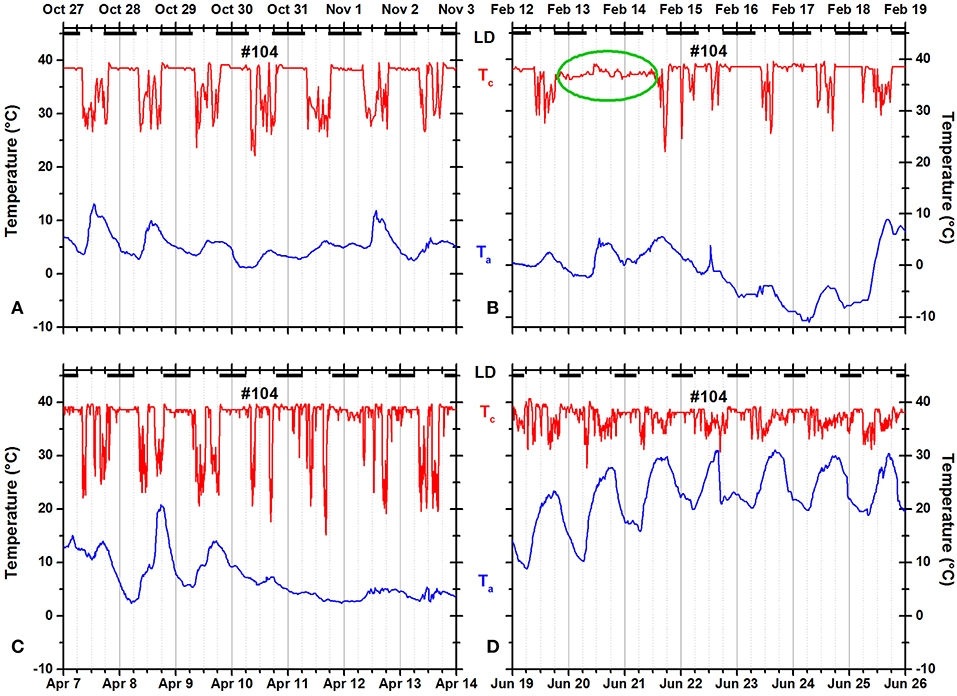
Figure 9. Records of ambient temperature (Ta, blue) and neck skin temperature (Tc, red) for fox squirrel #104 in (A) fall, (B) winter, (C) spring, and (D) summer. The dark horizontal bars at the top of each panel indicate the duration of the dark phase of the natural light–dark cycle. The green oval in (B) marks a day when the squirrel did not leave its insulated nest.
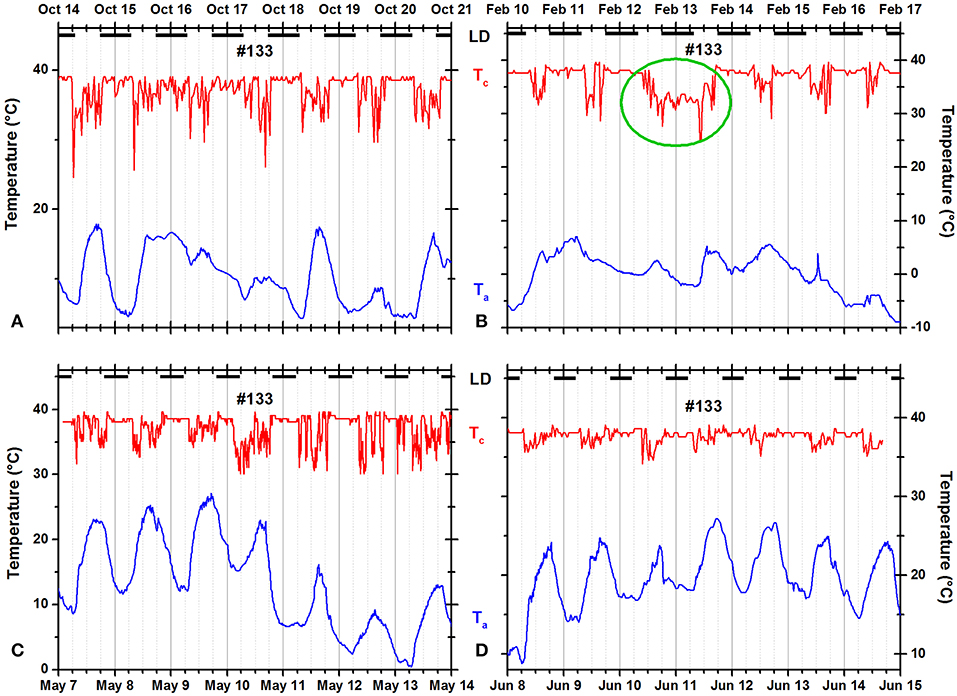
Figure 10. Records of ambient temperature (Ta, blue) and neck skin temperature (Tc, red) for fox squirrel #133 in (A) fall, (B) winter, (C) spring, and (D) summer. The dark horizontal bars at the top of each panel indicate the duration of the dark phase of the natural light–dark cycle. The green oval in (B) marks a day when the squirrel did not visit its insulated nest for more than 30 h.
Nocturnal ONT occurred occasionally in many fox squirrels. Seven of 14 squirrels spent at least one entire night outside their insulated nests—mostly during warm nights but exceptionally also during winter (for example, Figure 10B). The pooled data showed little evidence for seasonality of nocturnal ONT. Only fall (0.556 ± 1.306 h) and winter (0.171 ± 0.761 h) were significantly different from each other (Tukey HSD test, p < 0.00001). In contrary to the pooled data for all animals, two of the only three individual animals recorded over all seasons showed significant seasonality in the duration of nocturnal ONT. Squirrel 104 spent the least amount outside of its nest in winter (0.072 ± 0.296 h) and the most amount in summer (0.526 ± 1.002 h). A Tukey HSD test reached significance (p < 0.05) for the differences between fall and summer and winter and summer. Squirrel 133 spent also the least amount of nocturnal ONT in winter (0.225 ± 1.105 h) and the most in fall (1.612 ± 2.017 h). Fall vs. winter and fall vs. spring as well as winter vs. summer were significantly different (Tukey HSD, p < 0.05). Contrary to the frequent occurrence of ONT during night, the example of continuous in-nest time for more than 24 h shown in Figure 9B was the only occurrence of this type in our dataset.
Comparison Between the Intra- and Inter-individual Data Variability
Indices of intra- and inter-individual variabilities were calculated by analysis of consecutive data segments of individual animals and simultaneous (or randomized) segments of different individuals. Inter-individual and intra-individual variabilities were generally comparable to each other regarding time of first exit from the nest, number of exits per day, and time spent outside the nest per day in all four seasons (Table 5). For the four seasons combined, inter-individual variability exceeded intra-individual variability for total time outside the nest, and this difference was caused by a difference in the spring (Table 5).
Discussion
General Results
Individual squirrels differed significantly in the number of exits (and by this in the number of activity periods), diurnal nest time, total nest time, and nocturnal ONT. All recorded variables also showed significant seasonal variation. To a much lesser degree, age and recording interval contributed to the variance in the data, while the sex of squirrels only showed a significant effect in the interaction of sex and season (Figure 4). We also detected substantial day-to-day variations in the dependent variables of individual squirrels (Figure 5) that were partially correlated to weather events (Table 2).
Tc was barely and insignificantly negatively correlated with Ta in winter, and only slightly higher but significantly inversely correlated in summer. The inverse nature of these correlations shows that Tc was not merely following fluctuations of Ta in any season. Compared to significant correlations between Ta and other environmental factors such as snow fall (Table 2), the Tc-Ta correlations were smaller and, in the majority, insignificant. We are therefore confident that Tc is a reliable proxy to infer nest entries and exits.
Number of Nest Exits
Individual squirrels showed slightly more unimodal than bimodal days and only rarely exited more frequently than twice per day (Figure 3). The number of daily exits showed a distinct seasonality with significantly more exits in summer than in winter. In addition, the number of exits was significantly correlated with the hours of daylight and weather parameters, especially the daily maximum temperature (Table 2). These seasonal trends agree with the results of most studies on fox squirrels (Hicks, 1949; Adams, 1984; Koprowski and Corse, 2005) and other tree squirrels (Thompson, 1977; Wauters et al., 1992), which reported bimodal patterns in summers, and unimodal patterns in winter. However, in the current study, we found gradual and erratic rather than categorical and continuous changes in the seasonal distribution of activity patterns in individual squirrels with the occurrence of bimodal days in winter (Figure 2) and unimodal days in summer (Figure 10), substantial day-to-day fluctuations (Figure 5A), and more nest exits occurring in fall/winter than summer in some squirrels suggest that the seasonal variability in the activity patterns is driven not only by changes in the photoperiod and temperature (Halle and Stenseth, 2000) but also by (stochastic) environmental factors such as unfavorable weather (Table 2 and Williams et al., 2014; Studd et al., 2016), predation (Eriksen et al., 2011; Connolly and Orrock, 2017), social interactions (Schmidt et al., 2008; Agostini et al., 2012), and/or intrinsic individual characteristics such as personality (Boon et al., 2008; Careau et al., 2008) and chronotype (Refinetti et al., 2016; Alós et al., 2017). As in the case shown in Figure 2, on the same day, December 20, 2012, two of four animals (#111 and #147) showed a bimodal pattern, while the other two animals showed a unimodal pattern. It is unlikely that weather conditions influenced the activity patterns of these animals in different ways, but we cannot exclude other possible extrinsic factor such as predation or social interactions, or intrinsic differences between the animals. There are only two studies known to us comparing individual activity patterns in tree squirrels over the seasons of the year (Studd et al., 2016; Anders et al., 2017). Anders et al. (2017) found unimodal, bimodal, and even trimodal patterns in the Eurasian red squirrel (Sciurus vulgaris) in Japan during the months of July to October. We also found trimodal and even polymodal patterns in some seasons of the current study. However, these patterns often occurred during the warmer months when the gradient between Tc and Ta was reaching levels below 10°C and the detection of entries and exits was not always satisfactory (Figures 9, 10). Nevertheless, as Anders et al. (2017) used a data logger capable of recording collar temperature and light intensity simultaneously, their detection of trimodal patterns supports that some of the trimodal or polymodal patterns we detected might not be artifacts. Studd et al. (2016) used an almost identical design to the current study to investigate the activity patterns of lactating female North American red squirrels (Tamiasciurus hudsonicus) in southwestern Yukon, Canada, between February and June. The authors found an overall pattern of transition from unimodal daily activity centered at the end of the day on cold days to bimodal daily activity at intermediate temperatures and unimodal daily activity centered at the beginning of the day on warm days. Studd et al. (2016) confirmed the influence of weather conditions previously reported by Williams et al. (2014) also in lactating females from the same population of red squirrels using light sensors. Both studies found trimodal patterns in lactating females. We also found trimodal patterns in the current study (e.g., several days in Figure 10C). The animal shown (#133) is a female and the month of May falls within the predominant lactation period for fox squirrels (Koprowski, 1994); thus, it is possible that this squirrel was lactating and needed to forage more food to cover the higher energy demand (Havera, 1979) while having to return in regular intervals to nurse her offspring leading to a trimodal pattern (Williams et al., 2014; Studd et al., 2016).
In-nest and Out-of-nest Times
From fall through summer, fox squirrels spent increasingly longer periods inside of their nests during daytime while total time spent in the nest during the full 24-h cycle of day and night was highest in winter (Figure 6). Nocturnal out-of-nest activity was highest in fall and lowest in winter.
There were also significant differences between individual squirrels in nest time during daylight hours, total nest time and nocturnal ONT. While longer in-nest times during the long daylight hours of summer can simply be a consequence of longer days, long in-nest times in winter can be caused by the long nights and colder temperatures of this season. The surprising and seemingly contradictory difference between in-nest time during daylight and during the entire 24-h activity cycle is based on significantly shorter in-nest time during the daylight hours in fall as compared to any other month (Figure 6A). We speculate that this difference makes sense in the light of the fox squirrel's natural history, with fall being the food caching season and with the approaching reproductive season in winter (Koprowski 1994). Nocturnal ONT, which was always short (on average below 37 min in the pooled data) but was more than four times longer in fall than in winter can also not be simply related to the changes in photoperiod. Here we suspect again a joint influence of the still mild night temperatures in fall, the end of the food-caching season, and the approaching major mating season causing significantly longer nocturnal ONT in fall compared to winter. These assumptions are supported by our findings that both photoperiod and certain weather conditions especially daily maximum temperatures were significantly correlated to total ONT (Table 2).
In the study by Studd et al. (2016) that we cited above in the discussion of the number of nest exits, temperature also highly affected the proportion of time spent out of the nest during morning hours (from 10% at −30°C to approximately 100% at temperatures of 0°C). As temperatures increased from −20°C to 15°C, time spent out during afternoon declined from 75% to 10%, and time spent out during the evening declined from 100% to almost 0%. Anders et al. (2017) found that the daily activity time of red squirrels generally increased with increasing temperature and decreased with increased precipitation and average wind speed across both years. We can confirm this trend for temperature but not for precipitation and wind speed (Table 2). Studd et al. (2016) confirmed the influence of weather conditions previously reported by Williams et al. (2014) also in lactating females from the same population of red squirrels using light sensors.
Total nest time allows a rough estimate of the proportion of rest per 24 h. In the pooled data, squirrels spend about 66–68% of 24 h in their nests in spring, summer, and fall, and 77% in winter. As visual focal animal observations (Altmann, 1974) of six squirrels during various hours of the day when the animals were outside of their nests indicated that the animals were sitting or lying quietly, apparently resting, during 45–67% of the time observed (Wassmer and Refinetti, 2016), the total rest time might be considerably longer, probably in the realm of 80–85%. Studd et al. (2016) described nest attendance patterns of lactating red squirrels (Tamiasciurus hudsonicus) as highly variable as the daily proportion of time spent out of the nest during civil daylight hours ranged from 5% to 85% with an average of 45%. Diurnal in-nest time of fox squirrels (Sciurus niger) in this study was very similar ranging from 0 to 96% with an average of 43%.
Timing of First Exits
In all seasons, first exits of individual squirrels were significantly concentrated around a narrow window of time in the early to late morning (Figure 7) and were in many cases significantly different from each other (Table 3). However, none of the individual squirrels recorded during multiple seasons was clearly and consistently an early or late riser. In summer, first exits of individual squirrels were more spread out, whereas in winter, individual squirrels exited their nest much closer to each other, which is expected as winter days are substantially shorter than summer days. Interestingly this mimics the distribution of activity onsets in the nocturnal Ord's kangaroo rat (Dipodomys ordii) (White and Geluso, 2007), which also shows a wider range of individual activity onsets in summer and a much narrower range in winter. Enright (1966) showed that in house finches kept under controlled conditions in a laboratory, cooler temperature at a constant photoperiod and shorter photoperiods at the same ambient temperature caused delayed activity onsets. Our results indicate that both daylength and temperature had simultaneously the same effects on fox squirrels in the wild. As first exits constitute activity onsets, these results are complementary to the (seasonal) changes of the unimodal–polymodal gradient and the total in-nest/out-of-nest time (proportion of activity/rest) and are therefore aspects of the overall activity pattern of fox squirrels.
The number of nest exits was significantly inversely correlated with the timing of the first daily exits in the pooled data for all seasons. This means that the early riser chronotype showed significantly more nest exits than the late riser chronotype (Table 4). The larger number of exits might reflect a behavioral trait of early risers or simply the availability of a longer daily activity time. Under controlled laboratory conditions, early-rising antelope ground squirrels were found to have significantly longer daily activity times (Refinetti et al., 2016), and this association may occur also in the fox squirrel, which would contribute to the higher number of nest exits.
Continuous Nest Occupancy for More Than 24 h and Nocturnal Out-of-nest Time
Nocturnal ONT occurred occasionally in many fox squirrels—mostly during warm nights but exceptionally also during winter (for example, Figure 10B). While most nocturnal ONT occurred only for brief periods, some events took the entire night. In the pooled data and in two out of three animals recorded in all seasons, nocturnal ONT was shortest in winter (0.1–0.2 h) and longest in fall or summer (0.5–1.6 h). Except for a recent publication (Anders et al., 2017) using continuously logged temperature data as well, nocturnal ONT was not known to occur in tree squirrels so far. This clearly demonstrates a key advantage of data loggers to discover previously hidden details of animal behavior and natural history. We can only speculate why fox squirrels spent time outside of their nests during some nights. Temperatures outside of the nests might be more comfortable in summer than the insulated nests or invite for short trips for urination and defecation. In colder nights, such as the documented example in Figure 10B, it might be that the squirrel's nest was destroyed or not available.
While nocturnal ONT was detected repeatedly, continued nest occupancy for more than 24 h was only found once in the entire data set (Figure 9B). Squirrel 104 is a female, but the middle of February would be relatively late for parturition to happen. However, the date falls well into the lactation period while weather conditions were not specifically unfavorable (no precipitation or snow, relatively warm with highs around 3.5°C and lows at −6°C).
Nocturnal ONT (or more general, activity during the typically inactive time of the activity cycle) and days with unusual patterns are two more dimensions that should be considered to describe the bandwidth of activity patterns more inclusively.
Comparison Between the Intra- and Inter-individual Data Variability
The inter-individual variabilities of total ONT and the number of exits were either higher than the intra-individual variabilities or comparable to them (Table 5). These results underline the importance of studying the activity patterns of individual tree squirrels to complement and contrast pooled data for entire groups and populations but also hint at the astonishing day-to-day variabilities within the same squirrels (Figure 5). Very few researchers have compared intra- and inter-individual variabilities of circadian rhythms. We are aware of only four studies that conducted such comparison for the rhythms of body temperature (Refinetti and Piccione, 2005; Romeijn and Van Someren, 2011), melatonin secretion (Barassin et al., 1999), and cortisol secretion (Selmaoui and Touitou, 2003). In one of these studies (Refinetti and Piccione, 2005), four different species were used: the laboratory rat, the 13-lined ground squirrel, the domestic dog, and the horse. Intra- and inter-individual variabilities were computed for the mean level, range of oscillation, acrophase, and robustness of the rhythm. The variabilities differed in different parameters of the rhythm and in different species but, whenever there was a difference between inter-individual variability and intra-individual variability, the latter was always smaller than the former. That is, the day-to-day variability of an individual's rhythm never exceeded the variability between the rhythms of different individuals, which is the same as we found in this study on fox squirrels.
Conclusions
The results of our study illustrate the importance and advantages of collecting activity-related data from individual animals. Such data allow the estimation of how much individual fox squirrels differ from each other and how much they can fluctuate from day to day. We found that the magnitude of inter-individual variability of a number of behavioral variables was similar to the magnitude of intra-individual (day-to-day) variability. Although on most days the squirrels spent the night in their nests and exited the nest one or more times during the day, there was considerable variation (10–20% of the mean) from one squirrel to another and from day to day for the same animal.
We were working with a relatively small sample size and, due to the storage limitations of iButtons, with brief bouts of data. The idiosyncratic behaviors of individual fox squirrels that we documented might therefore not be entirely representative of a larger population of fox squirrels. However, some of the patterns and behaviors described here were not known before and illustrate the value of using data loggers to discover hidden details of the lives of even common species living in close proximity to humans in a suburban to urban setting.
There are possible methodological shortcomings in the use of collar temperature as a proxy of activity. In late spring/summer when higher ambient temperatures occur, the overnight patterns of Tc of several animals indicated that they did not spend the night in insulated nests (e.g., Figures 9D, 10C). On such days it is much more difficult—and sometimes impossible—to detect and distinguish in-nest time from ONT using our method. We therefore plan to supplement and extend the current study by using combined temperature and light sensors (Anders et al., 2017). We also plan to look at the patterns recorded for female fox squirrels during their expected gestation and lactation periods and compare these results to Studd et al. (2016) and Williams et al. (2014).
Ethics Statement
All work was conducted with approval of the Institutional Animal Care and Use Committee of Siena Heights University and followed appropriate guidelines as outlined by Sikes et al. (2012).
Author Contributions
TW conceived, designed, and performed the data collection. TW and RR analyzed the data and wrote/edited the paper. RR designed the custom software used in the data analysis.
Funding
This research was funded by the Biology Department of Siena Heights University and a Siena Heights University Faculty Development Grant.
Conflict of Interest Statement
The authors declare that the research was conducted in the absence of any commercial or financial relationships that could be construed as a potential conflict of interest.
Acknowledgments
We would like to thank the Siena Heights University Biology Department and Faculty Development Grant for supporting this research, Cindy Canale, Institute of Evolutionary Biology and Environmental Studies, University of Zurich, for the initial design of the collar, Siena Heights students Ryan Gumbleton and Crystal Wilcoxen for assistance in animal trapping and the preparation of collars, and John L. Koprowski, University of Arizona, Robert McCleery, University of Florida, and Murray M. Humphries, McGill University, for advice. We would also like to thank the Boise State University Psychology Department for supporting this research.
Supplementary Material
The Supplementary Material for this article can be found online at: https://www.frontiersin.org/articles/10.3389/fevo.2019.00179/full#supplementary-material
References
Adams, C. E. (1984). Diversity in fox squirrel spatial relationships and activity rhythms. Tex. J. Sci. 36, 197–203.
Agostini, I., Holzmann, I., and Di Bitetti, M. S. (2012). Influence of seasonality, group size, and presence of a congener on activity patterns of howler monkeys. J. Mammal. 93, 645–657. doi: 10.1644/11-MAMM-A-070.1
Alós, J., Martorell-Barcel,ó, M., and Campos-Candela, A. (2017). Repeatability of circadian behavioural variation revealed in free-ranging marine fish. R. Soc. Open Sci. 4:160791. doi: 10.1098/rsos.160791
Altmann, J. (1974). Observational study of behavior: sampling methods. Behaviour 49, 227–267. doi: 10.1163/156853974x00534
Anders, J. L., Uchida, K., Watanabe, M., Tanio, I., Shimamoto, T., Hamada, M., et al. (2017). Usefulness and limitation of a tiny light-temperature logger to monitor daily activity levels of arboreal squirrels in temperate areas. Mamm. Res. 62, 397–404. doi: 10.1007/s13364-017-0326-0
Aschoff, J. (1966). Circadian activity pattern with 2 peaks. Ecology 47, 657–662. doi: 10.2307/1933949
Barassin, S., Saboureau, M., Kalsbeek, A., Bothorel, B., Vivien-Roels, B., Malan, A., et al, (1999). Interindividual differences in the pattern of melatonin secretion of the Wistar rat. J. Pineal Res. 27, 193–201.
Bennett, A. F. (1987). “Interindividual variability: an underutilized resource,” in New directions in ecological physiology, eds M. E. Feder, A. F. Bennett, W. W. Burggren, and R. B. Huey (New York, NY: Cambridge University Press), 147–69.
Boon, A. K., Réale, D., and Boutin, S. (2008). Personality, habitat use, and their consequences for survival in North American red squirrels Tamiasciurus hudsonicus. Oikos 117, 1321–1328. doi: 10.1111/j.0030-1299.2008.16567.x
Bordignon, M., and Monteiro-Filho, E. L. A. (2000). Behaviour and daily activity of the squirrel Sciurus ingrami in a secondary araucaria forest in southern Brazil. Can. J. Zool. 78, 1732–1739. doi: 10.1139/cjz-78-10-1732
Careau, V., Thomas, D., Humphries, M. M., and Reale, D. (2008). Energy metabolism and animal personality. Oikos 117, 641–653. doi: 10.1111/j.0030-1299.2008.16513.x
Ciuti, S., Muhly, T. B., Paton, D. G., McDevitt, A. D., Musiani, M., and Boyce, M. S. (2012). Human selection of elk behavioural traits in a landscape of fear. Proc. R. Soc. Lond. B Biol. Sci. 279:4407. doi: 10.1098/rspb.2012.1483
Connolly, B. M., and Orrock, J. L. (2017). Habitat-specific capture timing of deer mice (Peromyscus maniculatus) suggests that predators structure temporal activity of prey. Ethology 124, 105–112. doi: 10.1111/eth.12708
Cooper, E. B., Taylor, R. W., Kelley, A. D., Martinig, A. R., Boutin, S., Humphries, M. M., et al. (2017). Personality is correlated with natal dispersal in North American red squirrels (Tamiasciurus hudsonicus). Behaviour 154, 939–961. doi: 10.1163/1568539X-00003450
Dall, S. R. X., and Boyd, I. L. (2002). Provisioning under the risk of starvation. Evol. Ecol. Res. 4, 883–896.
DeCoursey, P. J., Walker, J. K., and Smith, S. A. (2000). A circadian pacemaker in free-living chipmunks: essential for survival? J. Comp. Physiol. A Neuroethol. Sens. Neural. Behav. Physiol. 186, 169–180. doi: 10.1007/s003590050017
Dingemanse, N. J., and Wolf, M. (2013). Between-individual differences in behavioural plasticity within populations: causes and consequences. Anim. Behav. 85, 1031–1039. doi: 10.1016/j.anbehav.2012.12.032
Ditgen, R. S., Shepherd, J. D., and Humphrey, S. R. (2007). Big cypress fox squirrel (Sciurus niger avicennia) diet, activity and habitat use on a golf course in southwest Florida. Am. Midl. Nat. 158, 403–414. doi: 10.1674/0003-0031(2007)158[403:Bcfssn]2.0.Co;2
Enright, J. T. (1966). Influences of seasonal factors on the activity onset of the house finch. Ecology 47, 662–666. doi: 10.2307/1933950
Eriksen, A., Wabakken, P., Zimmermann, B., Andreassen, H. P., Arnemo, J. M., Gundersen, H., et al. (2011). Activity patterns of predator and prey: a simultaneous study of GPS-collared wolves and moose. Anim. Behav. 81, 423–431. doi: 10.1016/j.anbehav.2010.11.011
Fisher, N. I. (1993). Statistical Analysis of Circular Data. New York, NY: Cambridge University Press.
Gosling, S. D. (2001). From mice to men: what can we learn about personality from animal research? Psychol. Bull. 127, 45–86. doi: 10.1037/0033-2909.127.1.45
Halle, S., and Stenseth, N. C. (2000). Activity Patterns in Small Mammals: An Ecological Approach. New York, NY: Springer.
Havera, S. P. (1979). Energy and nutrient cost of lactation in fox squirrels. J. Wildl. Manage 43, 958–965. doi: 10.2307/3808281
Helm, B., Visser, M. E., Schwartz, W., Kronfeld-Schor, N., Gerkema, M., Piersma, T., et al. (2017). Two sides of a coin: ecological and chronobiological perspectives of timing in the wild. Philos. Trans. R. Soc. Lond. B Biol. Sci. 372:246. doi: 10.1098/rstb.2016.0246
Hertel, A. G., Swenson, J. E., and Bischof, R. (2017). A case for considering individual variation in diel activity patterns. Behav. Ecol. 28, 1524–1531. doi: 10.1093/beheco/arx122
Hicks, E. A. (1949). Ecological factors affecting the activity of the western fox squirrel, Sciurus niger rufiventer (Geoffroy). Ecol. Monogr. 19, 287–302. doi: 10.2307/1943272
Higginson, A. D., McNamara, J. M., and Houston, A. I. (2012). The starvation–predation trade-off predicts trends in body size, muscularity, and adiposity between and within taxa. Am. Nat. 179, 338–350. doi: 10.1086/664457
Horne, J. A., and Ostberg, O. (1976). A self-assessment questionnaire to determine morningness–eveningness in human circadian rhythms. Int. J. Chronobiol. 4, 97–110.
Huggins, J. G., and Gee, K. L. (1995). Efficiency and selectivity of cage trap sets for gray and fox squirrels. Wildl. Soc. Bull. 23, 204–207. doi: 10.2307/3782791
Jodice, P. G. R., and Humphrey, S. R. (1992). Activity and diet of an urban-population of big cypress fox squirrels. J. Wildl. Manage 56, 685–692. doi: 10.2307/3809461
Johnson, C. H., Elliott, J. A., and Foster, R. (2003). Entrainment of circadian programs. Chronobiol. Int. 20, 741–774. doi: 10.1081/CBI-120024211
Kanda, L. L., Fuller, T. K., and Friedland, K. D. (2005). Temperature sensor evaluation of opossum winter activity. Wildl. Soc. Bull. 33, 1425–1431. doi: 10.2193/0091-7648(2005)33[1425:tseoow]2.0.co;2
Koprowski, J. L., and Corse, M. C. (2005). Time budgets, activity periods, and behavior of Mexican fox squirrels. J. Mammal. 86, 947–952. doi: 10.1644/1545-1542(2005)86[947:Tbapab]2.0.Co;2
Labyak, S. E., Lee, T. M., and Goel, N. (1997). Rhythm chronotypes in a diurnal rodent, Octodon degus. Am. J. Physiol. Regul. Integr. Comp. Physiol. 273 (3 Pt 2), R1058–R1066. doi: 10.1152/ajpregu.1997.273.3.R1058
Lamb, T. D. (1995). Photoreceptor spectral sensitivities: common shape in the long-wavelength region. Vis. Res. 35, 3083–3091. doi: 10.1016/0042-6989(95)00114-F
Larivée, M. L., Boutin, S., Speakman, J. R., McAdam, A. G., and Humphries, M. M. (2010). Associations between over-winter survival and resting metabolic rate in juvenile North American red squirrels. Funct. Ecol. 24, 597–607. doi: 10.1111/j.1365-2435.2009.01680.x
Lazerte, S. E., and Kramer, D. L. (2011). Using thermosensitive radiotelemetry to document rest and activity in a semifossorial rodent. Wildl. Soc. Bull. 35, 481–488. doi: 10.1002/wsb.74
Lone, K., Loe, L. E., Meisingset, E. L., Stamnes, I., and Mysterud, A. (2015). An adaptive behavioural response to hunting: surviving male red deer shift habitat at the onset of the hunting season. Anim. Behav. 102, 127–138. doi: 10.1016/j.anbehav.2015.01.012
Masuda, K., and Zhdanova, I. V. (2010). Intrinsic activity rhythms in Macaca mulatta: their entrainment to light and melatonin. J. Biol. Rhythms 25, 361–371. doi: 10.1177/0748730410379382
McNamara, J. M., Higginson, A. D., and Verhulst, S. (2016). The influence of the starvation–predation trade-off on the relationship between ambient temperature and body size among endotherms. J. Biogeogr. 43, 809–819. doi: 10.1111/jbi.12695
Moore, D. S., Notz, W., and Fligner, M. A. (2013). The Basic Practice of Statistics. New York, NY: W.H. Freeman.
Murray, I. W., and Smith, F. A. (2012). Estimating the influence of the thermal environment on activity patterns of the desert woodrat (Neotoma lepida) using temperature chronologies. Can. J. Zool. 90, 1171–1180. doi: 10.1139/z2012-084
Nelson, W., Tong, Y. L., Lee, J. K., and Halberg, F. (1979). Methods for cosinor-rhythmometry. Chronobiologia 6, 305–323.
Pfeffer, M., Wicht, H., von Gall, C., and Korf, H.-W. (2015). Owls and larks in mice. Front. Neurol. 6:101. doi: 10.3389/fneur.2015.00101
Quinn, J. L., Cole, E. F., Bates, J., Payne, R. W., and Cresswell, W. (2012). Personality predicts individual responsiveness to the risks of starvation and predation. Proc. R. Soc. B 279, 1919. doi: 10.1098/rspb.2011.2227
Randler, C., Schredl, M., and Göritz, A. S. (2017). Chronotype, sleep behavior, and the big five personality factors. SAGE Open 7:2158244017728321. doi: 10.1177/2158244017728321
Refinetti, R., and Piccione, G. (2005). Intra- and inter-individual variability in the circadian rhythm of body temperature of rats, squirrels, dogs, and horses. J. Therm. Biol. 30, 139–146. doi: 10.1016/j.jtherbio.2004.09.003
Refinetti, R., Wassmer, T., Basu, P., Cherukalady, R., Pandey, V. K., Singaravel, M., et al. (2016). Variability of behavioral chronotypes of 16 mammalian species under controlled conditions. Physiol. Behav. 161, 53–59. doi: 10.1016/j.physbeh.2016.04.019
Reighard, S. L., Bucholz, A. D., Haahr, J. A., and Jenks, J. A. (2004). Fox squirrel weight and age structure in mast and non-mast forests. Proc. Soc. Dak. Acad. Sci. 83, 47–54.
Roche, D. G., Careau, V., and Binning, S. A. (2016). Demystifying animal ‘personality’ (or not): why individual variation matters to experimental biologists. J. Exp. Biol. 219, 3832. doi: 10.1242/jeb.146712
Romeijn, N., and Van Someren, E. J. W. (2011). Correlated fluctuations of daytime skin temperature and vigilance. J. Biol. Rhythms 26, 68–77. doi: 10.1177/0748730410391894
Schmidt, K., Nakanishi, N., Izawa, M., Okamura, M., Watanabe, S., Tanaka, S., et al. (2008). The reproductive tactics and activity patterns of solitary carnivores: the Iriomote cat. J. Ethol. 27:165. doi: 10.1007/s10164-008-0101-4
Schroeder, M. A., and Robb, L. A. (2005). “Criteria for gender and age,” in Techniques for Wildlife Investigations and Management. Ed C. E. Braun (Bethesda, MD: The Wildlife Society), 303–38.
Selmaoui, B., and Touitou, Y. (2003). Reproducibility of the circadian rhythms of serum cortisol and melatonin in healthy subjects: a study of three different 24-h cycles over six weeks. Life Sci. 73, 3339–3349. doi: 10.1016/j.lfs.2003.05.007
Siegel, S., and Castellan, N. J. (1988). Nonparametric Statistics for the Behavioral Sciences. New York, NY: McGraw-Hill.
Sih, A., Bell, A. M., Johnson, J. C., and Ziemba, R. E. (2004). Behavioral syndromes: an integrative overview. Q. Rev. Biol. 79, 241–277. doi: 10.1086/422893
Sikes, R. S., Paul, E., and Beaupre, S. J. (2012). Standards for wildlife research: taxon-specific guidelines versus US Public Health Service Policy. Bioscience 62, 830–834. doi: 10.1525/bio.2012.62.9.9
Sokolove, P. G., and Bushell, W. N. (1978). Chi square periodogram—its utility for analysis of circadian-rhythms. J. Theor. Biol. 72, 131–160. doi: 10.1016/0022-5193(78)90022-X
Speakman, J. R. (2008). The physiological costs of reproduction in small mammals. Philos. Trans. R. Soc. Lond. B Biol. Sci. 363, 375–398. doi: 10.1098/rstb.2007.2145
Stamps, J. A. (2007). Growth-mortality tradeoffs and ‘personality traits’ in animals. Ecol. Lett. 10, 355–363. doi: 10.1111/j.1461-0248.2007.01034.x
Studd, E. K., Boutin, S., McAdam, A. G., and Humphries, M. M. (2016). Nest attendance of lactating red squirrels (Tamiasciurus hudsonicus): influences of biological and environmental correlates. J. Mammal. 97, 806–814. doi: 10.1093/jmammal/gyw010
Tester, J. R. (1978). “Analysis of circadian rhythms of free-ranging mammals,” in Fourth International Symposium on Biotelemetry, eds H. J. Klewe and H. P. Kimmich (Braunschweig: International Symposium on Biotelemetry), 167–70.
Thompson, D. C. (1977). Diurnal and seasonal activity of the grey squirrel (Sciurus carolinensis). Can. J. Zool. 55, 1185–1189. doi: 10.1139/z77-153
Vink, J. M., Groot, A. S., Kerkhof, G. A., and Boomsma, D. I. (2001). Genetic analysis of morningness and eveningness. Chronobiol. Int. 18, 809–822. doi: 10.1081/CBI-100107516
Wassmer, T. (2017). Detailed illustrated description of iButton collars for small mammals. Figshare Digital Repository. doi: 10.6084/m9.figshare.5149834.v1
Wassmer, T., and Refinetti, R. (2016). Daily activity and nest occupation patterns of fox squirrels (Sciurus niger) throughout the year. PLoS ONE 11:e0151249. doi: 10.1371/journal.pone.0151249
Wauters, L., Swinnen, C., and Dhondt, A. A. (1992). Activity budget and foraging behavior of red squirrels (Sciurus vulgaris) in coniferous and deciduous habitats. J. Zool. 227, 71–86.
Wauters, L. A., and Dhondt, A. A. (1987). Activity budget and foraging behaviour of the red squirrel (Sciurus vulgaris Linnaeus, 1758) in a coniferous habitat. Z. Säuget. Mamm. Biol. 52, 341–353.
White, J. A., and Geluso, K. (2007). Seasonal differences in onset of surface activity of Ord's kangaroo rat (Dipodomys ordii). J. Mammal. 88, 234–240. doi: 10.1644/05-Mamm-a-312r3.1
Williams, C. T., Wilsterman, K., Kelley, A. D., Breton, A. R., Stark, H., Humphries, M. M., et al. (2014). Light loggers reveal weather-driven changes in the daily activity patterns of arboreal and semifossorial rodents. J. Mammal. 95, 1230–1239. doi: 10.1644/14-MAMM-A-062
Williams, T. D. (2008). Individual variation in endocrine systems: moving beyond the ‘tyranny of the Golden Mean’. Philos. Trans. R. Soc. Lond. B Biol. Sci. 363:3. doi: 10.1098/rstb.2007.0003
Wolf, M., and Weissing, F. J. (2012). Animal personalities: consequences for ecology and evolution. Trends Ecol. Evol. 27, 452–461. doi: 10.1016/j.tree.2012.05.001
Zhang, J., Hull, V., Ouyang, Z., He, L., Connor, T., Yang, H., et al. (2017). Modeling activity patterns of wildlife using time-series analysis. Ecol. Evol. 7, 2575–2584. doi: 10.1002/ece3.2873
Zhao, Z. J., Song, D. G., Su, Z. C., Wei, W. B., Liu, X. B., and Speakman, J. R. (2013). Limits to sustained energy intake. XVIII. Energy intake and reproductive output during lactation in Swiss mice raising small litters. J. Exp. Biol. 216, 2349–2358. doi: 10.1242/Jeb.078436
Keywords: activity patterns, seasonality, environmental conditions, personality, chronotype, sciuridae, circadian rhythm, foraging behavior
Citation: Wassmer T and Refinetti R (2019) Individual Daily and Seasonal Activity Patterns in Fox Squirrels (Sciurus niger) Quantified by Temperature-Sensitive Data Loggers. Front. Ecol. Evol. 7:179. doi: 10.3389/fevo.2019.00179
Received: 26 November 2018; Accepted: 06 May 2019;
Published: 29 May 2019.
Edited by:
Deseada Parejo, University of Extremadura, SpainReviewed by:
Emily K. Studd, McGill University, CanadaInger Suzanne Prange, Appalachian Wildlife Research Institute, United States
Copyright © 2019 Wassmer and Refinetti. This is an open-access article distributed under the terms of the Creative Commons Attribution License (CC BY). The use, distribution or reproduction in other forums is permitted, provided the original author(s) and the copyright owner(s) are credited and that the original publication in this journal is cited, in accordance with accepted academic practice. No use, distribution or reproduction is permitted which does not comply with these terms.
*Correspondence: Thomas Wassmer, twassmer@sienaheights.edu