- State Key Laboratory of Ecological Pest Control for Fujian and Taiwan Crops, College of Plant Protection, Fujian Agriculture and Forestry University, Fuzhou, China
Large-scale patterns of species richness is an important issue in biogeography and ecology. The Qinghai-Tibetan Plateau (QTP) is a biodiversity hotspot in the world, which has an important status in the zoogeographical realms. Here, we analyzed the diversity patterns of Hemipteran insects in the QTP, and tested whether the patterns can be jointly explained by modern environmental as well as historical factors. A comprehensive geographic distribution dataset consisting of 1,166 Hemipteran species, which belong to 510 genera and 53 families, was compiled and used in our analyses. Patterns of richness were mapped into a grid-based map with a spatial resolution of 0.5° × 0.5°. An unbalanced diversity pattern of the Hemipteran insects in the QTP was presented, with more species in the eastern and southern parts of the plateau, while few species in the northern and main surface of the plateau. The northwestern Sichuan, the southern Gansu, the southeastern Tibet, the northwestern Yunnan and the eastern Qinghai were identified as diversity hotspots of species richness. Further analyses based on General linear models and Random Forest indicated that the diversity patterns of Hemipteran insects were influenced by both contemporary environmental factors and historical factors (e.g., habitat heterogeneity, climate stability, energy availability). Specifically, the species richness patterns of all Hemipteran insects in the QTP have been mainly affected by elevation range, temperature annual range, min temperature of coldest month, mean temperature of coldest quarter and the temperature change since the Last Glacial Maximum. In contrast, the water-related variables have relatively small effects on species richness. In addition, although habitat heterogeneity was indicated the most important factor for different suborders of Hemiptera, the climate stability was another dominate factor for Heteroptera and Auchenorrhyncha, while Sternorrhyncha was more affected by historical climate change.
Introduction
Large-scale patterns of species richness and underlying mechanisms are central issues in biogeography and ecology (Qian and Ricklefs, 2000; Ricklefs, 2004; Kreft and Jetz, 2007; Jetz et al., 2012). Investigating these issues are not only helpful to understand the evolution of species, but also crucial to the biodiversity conservation (Pennisi, 2005). For instance, Chi et al. (2017) proposed to establish new nature reserves for 30 counties by exploring the geographical distribution patterns of threatened medicinal plants in China. Zhang et al. (2015) suggested to establish nature reserves specifically designed for orchids in southwestern China and Hainan Island by studying the distribution pattern of 1,449 orchid species in China. Many studies on the patterns of global species diversity have been carried out over the past decades (Myers et al., 2000). Most studies have shown that the spatial patterns of species richness are highly relevant to contemporary environmental and historical factors such as ambient energy, water availability, climate stability, habitat heterogeneity and historical climate change (Currie and Paquin, 1987; Currie, 1991; Fine and Ree, 2006; Jansson and Davies, 2008). Although their relative importance remains controversial, it is generally approved that species diversity patterns are jointly influenced by these factors (Hawkins and Porter, 2003; Svenning and Skov, 2005). Furthermore, there are various hypotheses have been proposed to explain the mechanisms of species diversity patterns (Palmer, 1994; Willig et al., 2003; Wang et al., 2009). For instance, the ambient energy hypothesis regards that the geographical pattern of species richness is mainly caused by the direct control of energy on the physiological activities of species (Turner et al., 1988). The water-energy dynamic hypothesis states that species diversity patterns are affected by both water and energy (Hawkins et al., 2003a). The habitat heterogeneity hypothesis predicts that diverse topography and habitats can increase species diversity (Jiménez et al., 2009). The freezing tolerance hypothesis holds that in cold regions, many species cannot survive because they cannot tolerate the cold winter. As winter temperature decreases, species diversity gradually decreases (Hawkins, 2001; Hawkins et al., 2003a).
The Qinghai-Tibetan Plateau (QTP) is the largest and highest plateau in the world with the average elevation above 4,000 m (Zhang et al., 2002). The intensive uplift and formation of the QTP is considered as the most important geological event in the Miocene-Pliocene era (Zhou et al., 2006), which not only has changed the natural environment of the plateau itself, but also has strongly impacted on the surrounding environment as well as the global climate (Chen et al., 1999; Shi et al., 1999; Li et al., 2001). Considering the significance of the QTP region in global change studies, it has become a research hotspot in recent decades (Zhang et al., 2002). In addition, the QTP region plays an important role in the field of biogeography (Huang et al., 2006), which spans three biodiversity hotspots in the world, including the mountains of south-west China, the Himalaya, and the Indo-Burma, respectively (listed by Conservation International: www.biodiversityhotspots.org/xp/Hotspots). The QTP region has been widely regarded as the center of species diversity for many groups (e.g., Huang, 1981; Zhang, 1999; Tang et al., 2006). Previously limited studies on the diversity patterns of different taxonomic groups in the QTP have shown that most species are located in the margins of the plateau, such as most of the birds congregate in the south-eastern margin of the plateau (Zhang et al., 2016), the species richness of vascular plants is higher in the northeastern and southeastern areas than in the hinterland of the QTP (Yan et al., 2013), and the diversity centers of aphids are also scattered on the edges of the plateau (Huang et al., 2006). It was reported that energy and water availability regulated the richness patterns of vascular plants in the QTP (Yan et al., 2013). The overall species richness patterns of birds in the QTP are mainly explained by topographical heterogeneity and temperature amplitude (Zhang et al., 2016), and also impacted by geological history of the QTP (Lei et al., 2014). According to the geological history and prominent topographic structures of the QTP, historical factors are expected to have impact on the biodiversity patterns in the QTP. Considering there are still few studies on the mechanism of diversity patterns in the QTP, more taxonomic groups are needed to test the impact of contemporary environmental and historical factors on the diversity patterns in this region.
Hemiptera (Arthropoda: Insecta) is one of the most important and diverse groups in insects (Kristensen, 1991; Schuh and Slater, 1995), which is consist of four suborders, Heteroptera, Sternorrhyncha, Auchenorrhyncha, and Coleorrhyncha (Li et al., 2017). It is estimated that the recorded number of Hemipteran species is about one hundred thousand (Zhang, 2011). Most of the Hemipteran insects are phytophagous and widely distributed all over the world. Many of them have a wide range of host plants and are important pests in agriculture and forestry, such as aphids, scale insects, leafhoppers and planthoppers (Forero, 2008; Guo and Yuan, 2016). In the QTP region, in spite of many taxonomic studies of these groups have been published, there have been few studies on the Hemipteran insects that focus on the spatial patterns except for the aphids (Huang et al., 2006, 2008). Based on our previous studies (Huang et al., 2006, 2008) on the diversity patterns of aphids, we assume that there should be a general distribution pattern for more phytophagous Hemipteran insects in the QTP. Besides, the patterns of species richness should be influenced by different factors. It is a good opportunity to test whether the diversity patterns of herbivorous insects are affected by both modern ecological factors and historical factors by using the data of the Hemipteran insects.
In this study, we aim to (1) summarize the taxonomic diversity of Hemipteran insects in the QTP; (2) analyze the richness patterns and identify diversity hotspots of Hemipteran insects; (3) test the relative effect of the modern environmental factors and historical factors on the pattern of species richness.
Materials and Methods
Study Area and Distribution Dataset of the Hemipteran Insects
The QTP lies between 26°00′12″and 39°46′50″N, and 73°18′52″and 104°46′59″E, spans 2,945 km from east to west, passing through 1,532 km in the north-south direction, and occupies ~2.6 million km2, which account for 26.8% of the total land area of China (Zhang et al., 2002). It is surrounded by high mountains with Kunlun mountain in the north, Himalayas mountains in the south, Karakoram mountain in the west and the steep Hengduan mountains in the east. We followed this definition of study area, same as Huang et al. (2006) did.
Species categories and distribution records of Hemipteran insects were compiled primarily from the below resources: (1) Fauna Sinica published before this study (including aphids, plant bugs, scale insects, treehopper, flower bug, plant hopper and damsel bug); (2) the catalog of insect type specimens of China which indexed all insect type specimens from 1950 to 2010 (Cui et al., 2007, 2009; Bai et al., 2014); (3) the Global Biodiversity Information Facility (GBIF, http://www.gbif.org/); (4) literature survey for new Hemipteran species during 2000–2017 based on the Zoological Records; (5) supplementary raw data from published literature (e.g., Huang et al., 2006; Liu et al., 2009; Yuan et al., 2014; Wei et al., 2016); (6) the academic dissertations on Hemiptera groups searched by using the China Knowledge Resource Integrated Database; (7) specimen collection records from the Insect Systematics and Diversity lab at Fujian Agriculture and Forestry University.
The dataset mainly included the latest checklist of Hemipteran insects in the QTP and distribution information (distribution sites, longitudes and latitudes). The taxonomic information were further validated by using Catalog of Life as well as taxonomic websites for specific groups. For the distribution records which provided longitudes and latitudes in original data sources, the georeferenced data were kept directly. For those only provided the names of distribution sites, the geographic coordinates were determined by using the Google map. The final dataset includes a total of 3,011 records, which represents a comprehensive distribution dataset for Hemipteran insects in the QTP. The original data deposited in the DataOpen repository: http://www.dataopen.info/home/datafile/index/id/184.
Mapping the Distributions
Based on the dataset, the distributions of the Hemipteran insects were imported into the outline map of the QTP using the software ArcGIS 10.2 (ESRI, Inc., Redlands, California, USA). To determine an appropriate scale to present our data, we primitively tested three grid scales, 1°, 0.5°, 0.25°, to investigate the richness patterns, respectively. Based on these analyses (see Supplementary Figure 1), to present decent maps of richness patterns, the 0.5° was chosen to be used in final analyses. The distribution map of all species was overlaid onto a grid-based map with a resolution of 0.5° × 0.5° in order to identify the diversity centers of species richness. The richness patterns of three taxonomic categories (family, genus and species) were displayed on the grid cells according to statistical calculations. In total, 1,083 distribution grid cells were used in our analyses, including the incomplete grid cells located on the border of the QTP. All distribution maps were compiled with Behrman equal-area projection.
Environmental Data
In order to explore the environmental determinants driving the diversity pattern of the Hemipteran insects in the QTP, we selected environmental factors that represent contemporary and historical climates for analysis. The selection of factors was mainly based on the routine indicated in previous studies as well as the potential importance of factors to phytophagous insects.
The contemporary climate data were obtained from the WorldClim website (http://www.worldclim.org) with the resolution of 30 arc-second (Fick and Hijmans, 2017). Mean annual temperature (MAT), Mean temperature of warmest quarter (MTWAQ), Mean temperature of coldest quarter (MTCQ), Min temperature of coldest month (MTCP) and Max temperature of warmest month (MTWP) were used as surrogates of the energy availability; Mean annual precipitation (MAP), Precipitation of warmest quarter (PWAQ), and Precipitation of coldest quarter (PCQ) were used as surrogates of the water availability; Temperature annual range (TAR, defined as the difference between MTWP and MTCP) and Precipitation seasonality (PS, coefficient of variation) were used as surrogates of the climate stability.
The Last Glacial Maximum (LGM) climate data were also obtained from the WorldClim website (http://www.worldclim.org), including the Mean annual temperature (MAT) and Mean annual precipitation (MAP) during LGM which were reconstructed by MIROC-ESM model (Watanabe et al., 2011). Followed a previous study (Wang et al., 2017), we calculated the absolute values of the anomaly in the Mean annual temperature (MAT) and Mean annual precipitation (MAP) between the LGM and the present (Tano and Pano) to represent the historical climate change since the LGM (i.e., |MAT LGM – MAT present| and |MAP LGM – MAP present|). We also used normalized difference vegetation index (NDVI) (1-km2 resolution; data comes from the Environment and Ecology Scientific Data Center of western China, National Natural Science Foundation, China http://westdc.westgis.ac.cn) and elevation range (ELE) to represent the habitat heterogeneity. ELE was defined as the difference between the maximum elevation and the minimum elevation inside each grid cell by with a digital elevation model derived from the Global 30 Arc-Second Elevation Dataset (GTOPO30) at a resolution of 1-km (https://eros.usgs.gov/elevation-products).
Environmental data of all grids with a spatial resolution of 0.5° × 0.5° were extracted by calculating the average of each grid cell. Data extraction were performed using the software of ArcGIS 10.2 (ESRI, Inc., Redlands, California, USA) and R x64 3.4.1(http://www.r-project.org/).
Data Analysis
Richness was defined as the total number of species or genus or family in each grid cell. General linear models (GLMs) was applied to test the relationships between species richness of Hemipteran insects and environmental variables because the frequency distribution of species richness conformed to the Poisson distribution, which was consistent with previous studies (Wang et al., 2012; Liu et al., 2017). It should be noted that the quasipoisson parameter was set to make up for the overdispersion in our data (Crawley, 2007). So the GLMs with quasipoisson errors was used to assess the explanatory power of the environmental variables by implementation of the glm function in R x64 3.4.1(http://www.r-project.org/). Following Liu et al. (2017), we used the adjusted R2adj (%) to measure the explanatory power of each variable, which was calculated as: R2adj (%) = 100 × (1 – (residuals deviance/model DF)/(species richness deviance/residuals DF)). The spatial autocorrelation could inflate type I error, so modified t-test was used to test the significance levels of each regression coefficient, which in order to eliminate the effects of spatial autocorrelation in the significance test (Dutilleul et al., 1993). The species richness was log10-converted before analysis.
In order to compare with the results of GLMs analysis, the Random Forest, which was another way to explore the relationships between species richness and environmental factors, was employed to evaluate the relative importance of all variables. What needs to be pointed out is that Random Forest is not only insensitive to the spatial autocorrelation, but also can deal with the non-linear relationship between species richness and variables (Breiman, 2001; Marmion et al., 2009). Based on the function of randomForest in R x64 3.4.1, we obtained the value of the relative importance of each environmental factors, which is expressed in terms of node purity.
In order to investigate the more detailed relationships between the species richness and environmental factors for different groups in Hemiptera, the data set were divided according to suborders and the same analyses were implemented. Only the families with more than 50 distribution records were included during the analyses. As a group restricted to some areas in the southern Hemisphere, the suborder of Coleorrhyncha was not recorded in our data set. Therefore, the results for the other three suborders, Heteroptera, Sternorrhyncha, and Auchenorrhyncha, were shown in the manuscript.
Results
Species Diversity of Hemipteran Insects in the QTP
A total of 1,166 Hemipteran species were recorded in the checklist of species, which belong to 510 genera, 53 families. The detailed taxonomic information for each family were presented in Supplementary Table 1. Among all Hemipteran groups, the Cicadellidae represented by 274 species was the most abundant family, accounting for 23.5% of the total species, followed by the Aphididae (222 species) and Miridae (179 species) representing 19.04 and 15.35% of all species, respectively. The two families with the highest genus diversity are Cicadellidae (116 genera, 22.75% of all genera) and Aphididae (106 genera, 20.78% of all genera). However, some families such as Aphelocheiridae, Asterolecaniidae, Berytidae, Caliscelidae, Cydnidae, Fulgoridae, Heterogastridae, Issidae only had 1 genus and 1 species in the dataset.
Distribution Patterns of Species Richness
The general distribution pattern of all Hemipteran insects in the QTP was an unbalanced pattern with more species distributed in the eastern and southern parts of the plateau and few species in the northern edge and the main surface of the plateau (Figure 1). The distribution patterns of three taxonomic categories (family, genus and species) were almost the same (Figure 1). Higher diversity of species were concentrated on five areas as follows: (1) the northwestern Sichuan; (2) the southern Gansu; (3) the southeastern Tibet; (4) the northwestern Yunnan, and (5) the eastern Qinghai, which were identified as species diversity centers (Figure 1).
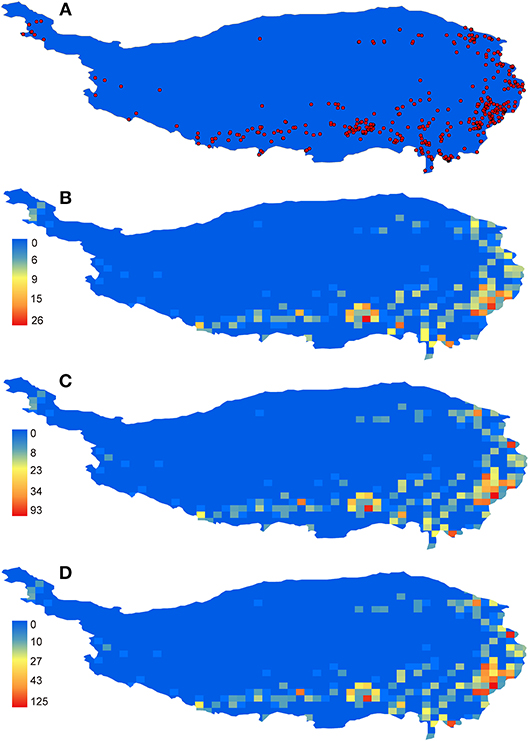
Figure 1. The distribution and richness patterns of Hemipteran insects in the QTP. (A) all records, (B) family richness, (C) genus richness, and (D) species richness.
Relationships Between Species Richness and Environmental Factors
GLMs indicated that the pattern of species richness for all Hemipteran insects was influenced by a variety of factors (Table 1). Some variables belonging to the historical climate change (such as Tano), habitat heterogeneity (such as ELE and NDVI), climate stability (such as TAR), and energy availability (such as MTCP, MTCQ, and MAT) had stronger explanatory power than the water-related variables (Table 1).Specifically, Tano was the strongest predictor accounting for 23.90% (P = 0.0236) of the explanatory power, followed by ELE with the adjusted R2adj was 21.45% (P = 5e-04), TAR (R2adj = 21.41, P = 0.0022), NDVI (R2adj = 18.06%, P = 0.1172), MTCP (R2adj = 17.74, P = 0.0045), MTCQ (R2adj = 16.72%, P = 0.0064), and MAT (R2adj = 14.98%, P = 0.0159). The R2adj of all the variables associated with water were all below 10% (i.e., MAP, PWAQ, PCQ, PS, and Pano). In general terms, climate stability variables (whether historical or contemporary climate), habitat heterogeneity variables, and temperature-related variables primarily determined the patterns of Hemipteran insects in the QTP.
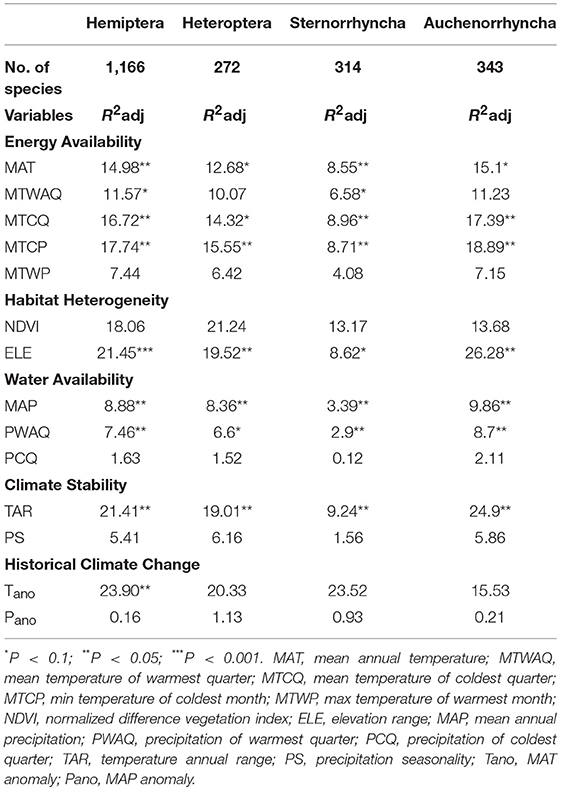
Table 1. Coefficient of determination R2adj (%) of the General linear models for all selected variables. Modified t-test was used to test the significance.
The results of Random Forest for all Hemipteran insects were basically consistent with that of GLMs (Figure 2A). The most important environmental factors are almost identical, but there are some differences in the order of relative importance. Variables representing habitat heterogeneity were indicated with highest importance. The Random Forest also indicated more explanatory power of some indicators related to water availability, such as MAP and PWAQ, than the GLMs.
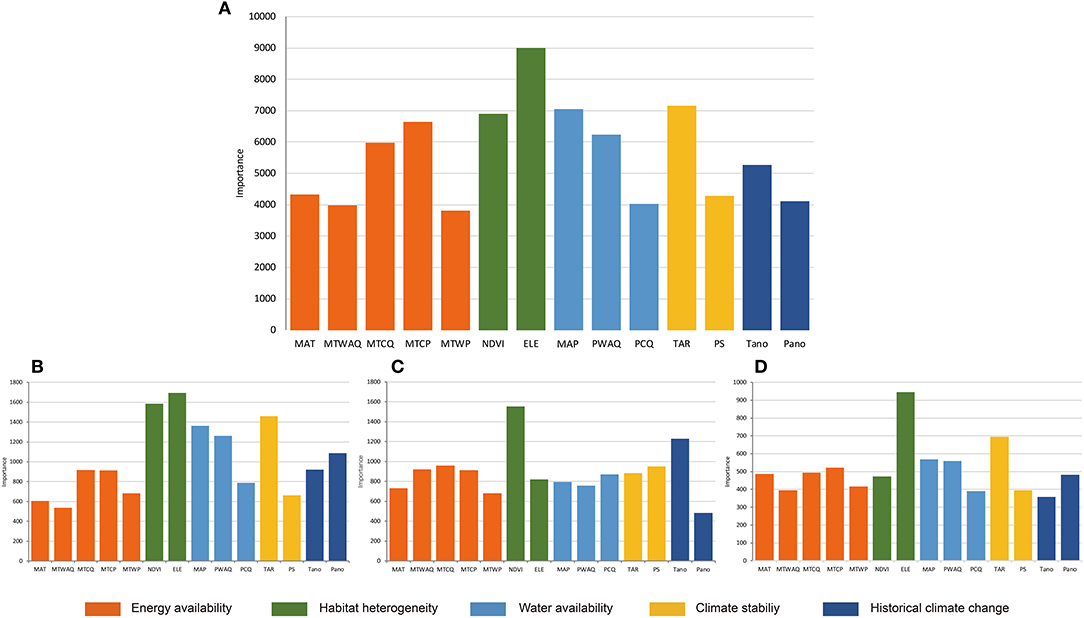
Figure 2. Results of Random Forest for the importance of all variables for (A) Hemiptera; (B) Heteroptera; (C) Sternorrhyncha; (D) Auchenorrhyncha. Importance values (y-axis) are represented by the increase in node purity. MAT, mean annual temperature; MTWAQ, mean temperature of warmest quarter; MTCQ, mean temperature of coldest quarter; MTCP, min temperature of coldest month; MTWP, max temperature of warmest month; NDVI, normalized difference vegetation index; ELE, elevation range; MAP, mean annual precipitation; PWAQ, precipitation of warmest quarter; PCQ, precipitation of coldest quarter; TAR, temperature annual range; PS, precipitation seasonality; Tano, MAT anomaly; Pano, MAP anomaly.
Based on the results of the two analyses, the richness patterns of Hemipteran insects in the QTP were affected by different factors, but their relative importance varied. Overall, habitat heterogeneity and climate stability were the most important determinants.
By combining the results of GLMs and Random Forest for different suborders, it was shown that generally the habitat heterogeneity was the most important factor for all suborders (Table 1, Figures 2B–D). However, the relative importance of different factors varied for different suborders. Besides habitat heterogeneity, the GLMs analysis indicated climate stability is another important factor for the three suborders, while the Random Forest analysis indicated that climate stability is more important for the Heteroptera and Auchenorrhyncha, but historical climate change is more important for Sternorrhyncha.
Discussion
Diversity Patterns and Distribution Centers of Hemipteran Insects in the QTP
The species richness of Hemipteran insects was higher in the edges of the QTP, particularly in the eastern and southern marginal areas of the plateau. Similar patterns of species richness has also been revealed by previous studies for such as aphids (Huang et al., 2006, 2008), birds (Zhang et al., 2016) as well as vascular plants (Yan et al., 2013). Although fewer distributions on the plateau central surface may indicate relative lack of collection effort, it is more likely that this reflects a reasonable distribution pattern if considering the geological history of this region and the cold weather and vegetation types caused by high altitude (Li et al., 2001; Yan et al., 2013). Considering most Hemipteran insects are phytophagous, the low diversity of vegetation types (Yan et al., 2013) limits the diversity of Hemipteran insects on the plateau surface. Besides, the quaternary glacial movement accompanied by the uplift of the QTP (Yang et al., 2008) may cause extinction of Hemipteran species on the plateau surface and migration from higher to lower altitudes. On the contrary, the complex topography, various types of vegetation and diverse habitats in marginal areas of the QTP (An et al., 2001; Densmore et al., 2007) can continuously provide appropriate habitats for Hemipteran insects. Our study, together with previous studies, may indicate the spatially unbalanced distribution pattern is not limited to Hemipteran insects and general for more taxonomic groups.
In this study, five diversity centers for Hemipteran insects were identified in the QTP. The northwestern Sichuan province and northwestern Yunnan province are located in the Hengduan mountains region which is commonly now known as not only one of the 35 biodiversity hot spots in the world (Boufford, 2014) but also a transitional zone between the QTP and the Yungui Plateau (Xu et al., 2010). The characteristic of this region is the extreme terrain undulation (Wu et al., 2013; Boufford, 2014) and deep gorges flanked by towering mountains in the north and south direction, which results in various types of climate and vegetation (Anderson et al., 2005) for a variety of habitats for Hemipteran insects. The southeastern Tibet region, which is located on the southern boundary of the QTP. The most prominent feature of this region is the strong differentiation of climate and vegetation types along altitudes (Zhang and Zhong, 1988), which provide the potential for higher diversity of Hemipteran insects. The southern Gansu province and eastern Qinghai province lie in the transitional region from the plateau hinterland to the surrounding hilly region. The species diversity may be affected by the complicated environmental factors in these areas on account of the marginal effects generated by the communication between adjacent ecosystems (Huang et al., 2008).
Effects of Environmental Factors on the Patterns of Species Richness
The pattern of species richness is the result of a combination of different determinants including contemporary environmental and historical factors (Svenning and Skov, 2005). The results of GLMs and Random Forest for all Hemipteran insects were generally consistent in this study, which demonstrate that the richness patterns of Hemipteran insects can be explained by different factors (e.g., habitat heterogeneity, climate stability, environmental energy, water availability) with varied relative importance.
Both analyses indicated that the variables of habitat heterogeneity (especially ELE) are critical in explaining the overall species richness patterns of Hemipteran insects. Based on the distribution patterns of environmental variables in the QTP (Figure 3), the biodiversity centers mentioned above share common features of more types of vegetation and higher elevation difference even >6,000 m (Figures 3A,B). According to the habitat heterogeneity hypothesis, species diversity may be affected by habitat heterogeneity through the increase of suitable niches as well as species differentiation (Bhattarai et al., 2004; Stein et al., 2014, 2015). The strong correlation between habitat heterogeneity and species richness was also reported for vascular plants in the QTP (Mao et al., 2013). This also validates our results when considering the close relationship between Hemipteran insects and their host plants (e.g., Li et al., 2017). A previous study on avian species richness in the Hengduan mountains also found that the topographic heterogeneity had a marked impact on the species patterns of birds (Wu et al., 2013), suggesting habitat heterogeneity has a significant effect on the richness of many groups in the QTP.
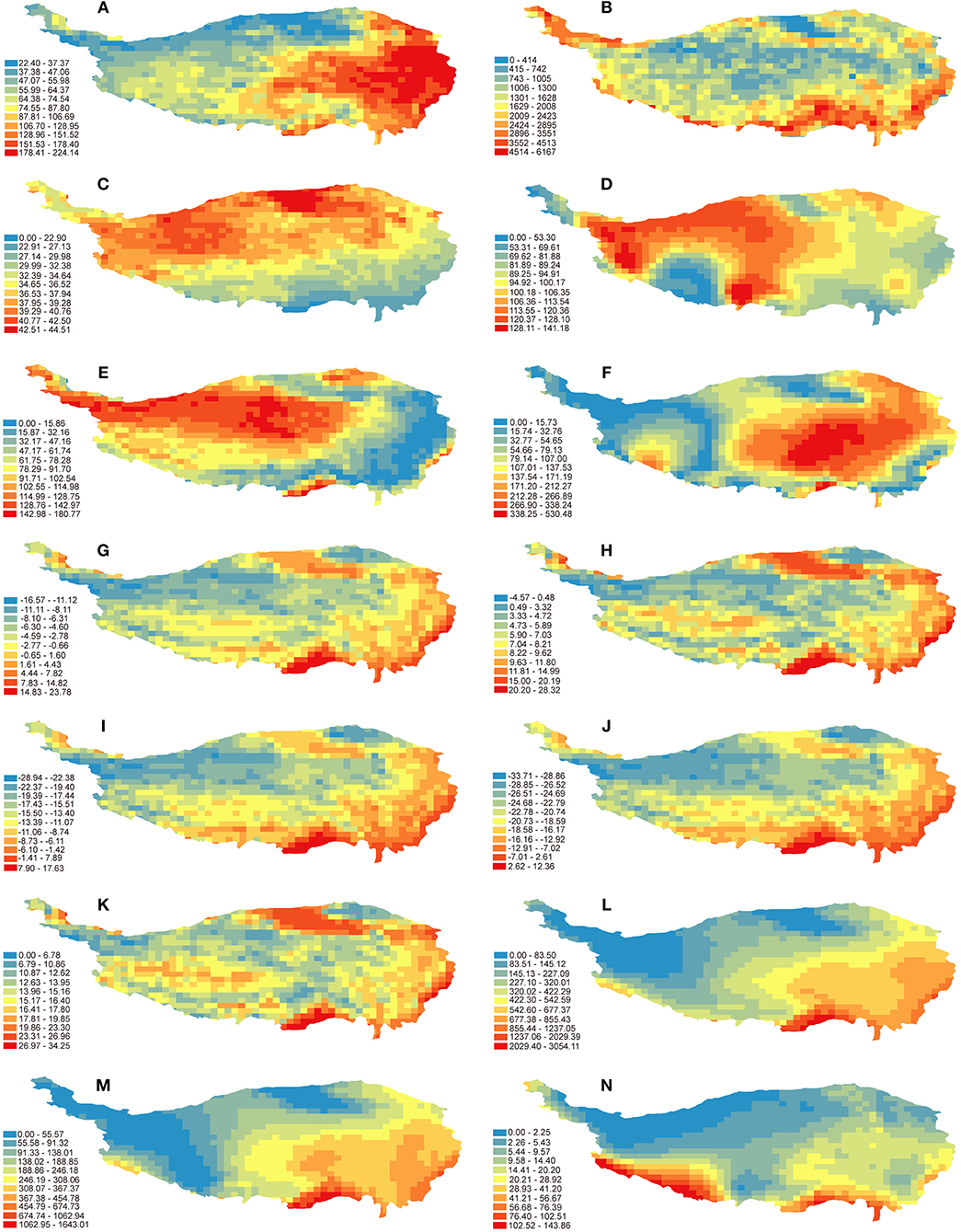
Figure 3. Distribution patterns of environmental variables in the QTP. (A) NDVI, normalized difference vegetation index; (B) ELE, elevation range; (C) TAR, temperature annual range; (D) PS, precipitation seasonality; (E) Tano, MAT anomaly; (F) Pano, MAP anomaly; (G) MAT, mean annual temperature; (H) MTWAQ, mean temperature of warmest quarter; (I) MTCQ, mean temperature of coldest quarter; (J) MTCP, min temperature of coldest month; (K) MTWP, max temperature of warmest month; (L) MAP, mean annual precipitation; (M) PWAQ, precipitation of warmest quarter; (N) PCQ, precipitation of coldest quarter.
Climatic stability is important driving force for geographical distribution of organisms. Our results showed that TAR (representing climate stability) had significant influence on the species richness of Hemipteran insects in the QTP. This is consistent with the climate stability hypothesis which states that stable climate will increase species richness in one region (Stevens, 1989). The five biodiversity centers identified in this study were located in areas with low annual temperature difference (Figure 3C), which is more suitable for species survival in these areas. The temperature variation from LGM till now (Tano; Table 1) was also one of the main influences, indicating that Hemipteran insects were sensitive to the long-term climate stability. Most of the plateau was covered by glaciers during the LGM (Shi et al., 1997), as well as the historical climate changes were much stronger on the plateau surface than the frontier area (Figure 3E), may result in less diversity of Hemipteran species in the northern high altitudes and main surface of the plateau. Besides, the marginal areas less affected by the quaternary glaciers have been supposed as refugia for animals and plants (Huang et al., 2005; Fan et al., 2008; Duan et al., 2011; Lei et al., 2014). The stable habitats in these areas should also help conserve more diversity of Hemipteran insects. It has been shown that quaternary climate changes or stability can explain diversity patterns among taxonomic groups in different regions (Araújo et al., 2008; Svenning et al., 2008; Sandel et al., 2011). Our results together with previous findings indicate that historical climate stability is an important factor affecting the pattern of species richness.
Previous studies have shown that ambient energy has significant effect on species diversity of different groups and sometimes the most important factor in predicting the geographical variation of species richness (Turner et al., 1987; Currie, 1991; Hawkins et al., 2003b; Wang et al., 2011). Based on our results, some temperature-related variables (MTCP, MTCQ, MAT) are also important for explaining the diversity patterns of Hemipteran insects in the QTP. This is consistent with the findings of birds in the QTP (Zhang et al., 2016) and woody plants in China (Wang et al., 2011). The eastern and southern marginal areas of the QTP are with higher mean annual temperature than that the hinterland of the plateau (Figure 3G), while the temperature on the plateau main surface is lower than the marginal areas during the coldest period (Figures 3I,J), together indicating that Hemipteran insects may prefer warmer habitats. The congruence between the distribution of temperature-related variables and the unbalanced diversity pattern of Hemipteran insects in the QTP echoes previous proposals that freezing tolerance ability of species limit their distributions (Hawkins, 2001; Hawkins et al., 2003b; Wiens and Donoghue, 2004).
Although the explanatory power of water availability obtained by GLMs analysis was relatively low compared with other factors, the Random Forest analysis showed that some of water-related variables (MAP and PWAQ) were almost as important as some variables of environmental energy (MTCQ and MTCP). This indicates that water and energy may jointly regulate the richness patterns of Hemipteran insects in the QTP, which is consistent with the water-energy dynamic hypothesis (O'Brien, 1998). Yan et al. (2013) also found that the richness patterns of woody plants in the QTP were jointly determined by water and energy. Besides, Wei et al. (2016) also showed that the distribution patterns of scale insects, one Hemipteran group, in China were jointly determined by temperature and precipitation. The sufficient energy and water in the eastern and southern edge areas of the QTP (Figures 3G,L) are conducive to photosynthesis and energy accumulation of plants (Gurevitch et al., 2002), thus these areas can maintain higher richness of plants as well as Hemipteran insects.
For each suborder of Hemiptera, habitat heterogeneity is the common most important factor affecting their species richness, which is closely related to the phytophagous characteristics of Hemipteran insects (Li et al., 2017). The higher the heterogeneity of habitats, the richer their food resources are. Moreover, climate stability also has significant effects on the species richness of Heteroptera and Auchenorrhyncha, which indicates that these two suborders tend to live in regions with more stable contemporary climatic conditions. But for the Sternorrhyncha, their distribution patterns may have been more affected by climate changes during evolutionary history.
Conclusion
This study provides the first investigation of spatial patterns and determinants of the diversity of Hemipteran insects in the QTP. Basically there is an unbalanced pattern of species richness with the eastern and southern edges of the QTP hosting higher diversity of Hemipteran insects. Five diversity centers, namely the northwestern Sichuan, the southern Gansu, the southeastern Tibet, the northwestern Yunnan and the eastern Qinghai, were identified. Habitat heterogeneity and climatic stability are most important determinants for the diversity patterns of Hemipteran insects. However, the diversity patterns cannot be simply explained by only these two factors, instead jointly influenced by contemporary environmental factors and historical factors. We suggest that more studies based on datasets of other taxonomic groups are needed to further test whether such an unbalanced spatial pattern of species diversity in the QTP exist for more groups.
Author Contributions
XH and JL conceived and designed the study. JL, HL, YW, and LZ collected the data. JL and XH analyzed data and wrote the manuscript. XH contributed resources during the study.
Funding
This study was supported by National Key R&D Program of China (2016YFE0203100), National Natural Science Foundation of China (31772504), and Fujian Provincial Department of Science & Technology (2015J06005).
Conflict of Interest Statement
The authors declare that the research was conducted in the absence of any commercial or financial relationships that could be construed as a potential conflict of interest.
Acknowledgments
We thank Jian Zhang, Zhiheng Wang, Yunpeng Liu, and Jiufeng Wei for providing help in data collection and analysis.
Supplementary Material
The Supplementary Material for this article can be found online at: https://www.frontiersin.org/articles/10.3389/fevo.2019.00165/full#supplementary-material
References
An, Z., Kutzbach, J. E., Prell, W. L., and Porter, S. C. (2001). Evolution of Asian monsoons and phased uplift of the Himalaya-Tibetan Plateau since Late Miocene times. Nature 411, 62–66. doi: 10.1038/35075035
Anderson, D. M., Salick, J., Moseley, R. K., and Ou, X. (2005). Conserving the sacred medicine mountains: a vegetation analysis of Tibetan sacred sites in Northwest Yunnan. Biodivers. Conserv. 14, 3065–3091. doi: 10.1007/s10531-004-0316-9
Araújo, M. B., Nogués-Bravo, D., Diniz-Filho, J. A. F., Haywood, A. M., Valdes, P. J., and Rahbek, C. (2008). Quaternary climate changes explain diversity among reptiles and amphibians. Ecography 31, 8–15. doi: 10.1111/j.2007.0906-7590.05318.x
Bai, M., Cui, J. Z., Hu, J. Y., and Li, L. Z. (2014). Catalogue of the Insect Type Specimens Deposited in China, Vol. 3. Beijing: China Forestry Publishing House.
Bhattarai, K. R., Vetaas, O. R., and Grytnes, J. A. (2004). Fern species richness along a central Himalayan elevational gradient, Nepal. J. Biogeogr. 31, 389–400. doi: 10.1046/j.0305-0270.2003.01013.x
Chen, L. X., Liu, J. P., Zhou, X. J., and Wang, P. X. (1999). Impact of uplift of Qinghai-Xizang Plateau and change of land-ocean distribution on climate over Asia. Quat. Sci. 4, 314–329.
Chi, X., Zhang, Z., Xu, X., Zhang, X., Zhao, Z., Liu, Y., et al. (2017). Threatened medicinal plants in China: distributions and conservation priorities. Biol. Conserv. 210, 89–95. doi: 10.1016/j.biocon.2017.04.015
Cui, J. Z., Bai, M., Fan, R. J., and Wu, H. (2009). Catalogue of the Insect Type Specimens Deposited in China, Vol. 2. Beijing: China Forestry Publishing House.
Cui, J. Z., Bai, M., Wu, H., and Ji, L. Q. (2007). Catalogue of the Insect Type Specimens Deposited in China, Vol. 1. Beijing: China Forestry Publishing House.
Currie, D. J. (1991). Energy and large-scale patterns of animal-and plant-species richness. Am. Nat. 137, 27–49. doi: 10.1086/285144
Currie, D. J., and Paquin, V. (1987). Large-scale biogeographical patterns of species richness of trees. Nature 329, 326–327. doi: 10.1038/329326a0
Densmore, A. L., Ellis, M. A., Li, Y., Zhou, R., Hancock, G. S., and Richardson, N. (2007). Active tectonics of the Beichuan and Pengguan faults at the eastern margin of the Tibetan Plateau. Tectonics 26:TC4005. doi: 10.1029/2006TC001987
Duan, Y. Z., Gao, Q. B., Zhang, F. Q., Li, Y. H., Fu, P. C., and Chen, S. L. (2011). Phylogeographic analysis of the endemic species Sibiraea angustata reveals a marginal refugium in the Qinghai-Tibet Plateau. Nord. J. Bot. 29, 615–624. doi: 10.1111/j.1756-1051.2011.00999.x
Dutilleul, P., Clifford, P., Richardson, S., and Hemon, D. (1993). Modifying the t test for assessing the correlation between two spatial processes. Biometrics 49, 305–314. doi: 10.2307/2532625
Fan, Z. X., Bräuning, A., and Cao, K. F. (2008). Tree-ring based drought reconstruction in the central Hengduan Mountains region (China) since AD 1655. Int. J. Climatol. 28, 1879–1887. doi: 10.1002/joc.1689
Fick, S. E., and Hijmans, R. J. (2017). WorldClim 2: new 1-km spatial resolution climate surfaces for global land areas. Int. J. Climatol. 37, 4302–4315. doi: 10.1002/joc.5086
Fine, P. V., and Ree, R. H. (2006). Evidence for a time-integrated species-area effect on the latitudinal gradient in tree diversity. Am. Nat. 168, 796–804. doi: 10.1086/508635
Guo, Z. L., and Yuan, M. L. (2016). Research progress of mitochondrial genomes of Hemiptera insects. Sci. Sin. 46, 151–166. doi: 10.1360/N052015-00229
Gurevitch, J., Scheiner, S. M., and Fox, G. A. (2002). The Ecology of Plants. Sunderland: Sinauer Associates.
Hawkins, B. A. (2001). Ecology's oldest pattern? Trends Ecol. Evol. 16, 470. doi: 10.1016/S0169-5347(01)02197-8
Hawkins, B. A., Field, R., Cornell, H. V., Currie, D. J., Guégan, J. F., Kaufman, D. M., et al. (2003a). Energy, water, and broad-scale geographic patterns of species richness. Ecology 84, 3105–3117. doi: 10.1890/03-8006
Hawkins, B. A., and Porter, E. E. (2003). Relative influences of current and historical factors on mammal and bird diversity patterns in deglaciated North America. Glob. Ecol. Biogeogr. 12, 475–481. doi: 10.1046/j.1466-822X.2003.00060.x
Hawkins, B. A., Porter, E. E., and Felizola Diniz-Filho, J. A. (2003b). Productivity and history as predictors of the latitudinal diversity gradient of terrestrial birds. Ecology 84, 1608–1623. doi: 10.1890/0012-9658(2003)084[1608:PAHAPO]2.0.CO;2
Huang, X. L., Lei, F. M., and Qiao, G. X. (2008). Areas of endemism and patterns of diversity for aphids of the Qinghai-Tibetan Plateau and the Himalayas. J. Biogeogr. 35, 230–240. doi: 10.1111/j.1365-2699.2007.01776.x
Huang, X. L., Qiao, G. X., and Lei, F. M. (2006). Diversity and distribution of aphids in the Qinghai-Tibetan Plateau-Himalayas. Ecol. Entomol. 31, 608–615. doi: 10.1111/j.1365-2311.2006.00819.x
Huang, X. L., Ren, S. S., and Qiao, G. X. (2005). Composition and characters of the aphid fauna in Hengduan Mountains region, China. Acta Zootaxonomica Sinica 30, 14–21.
Jansson, R., and Davies, T. J. (2008). Global variation in diversification rates of flowering plants: Energy vs. Clim. Change. Ecol. Lett. 11, 173–183. doi: 10.1111/j.1461-0248.2007.01138.x
Jetz, W., Thomas, G. H., Joy, J. B., Hartmann, K., and Mooers, A. O. (2012). The global diversity of birds in space and time. Nature 491, 444–498. doi: 10.1038/nature11631
Jiménez, I., Distler, T., and Jørgensen, P. M. (2009). Estimated plant richness pattern across northwest South America provides similar support for the species-energy and spatial heterogeneity hypotheses. Ecography 32, 433–448. doi: 10.1111/j.1600-0587.2008.05165.x
Kreft, H., and Jetz, W. (2007). Global patterns and determinants of vascular plant diversity. Proc. Natl. Acad. Sci. U.S.A. 104, 5925–5930. doi: 10.1073/pnas.0608361104
Lei, F. M., Qu, Y. H., and Song, G. (2014). Species diversification and phylogeographical patterns of birds in response to the uplift of the Qinghai-Tibet Plateau and Quaternary glaciations. Curr. Zool. 60, 149–161. doi: 10.1093/czoolo/60.2.149
Li, H., Leavengood Jr, J. M., Chapman, E. G., Burkhardt, D., Song, F., Jiang, P., et al. (2017). Mitochondrial phylogenomics of Hemiptera reveals adaptive innovations driving the diversification of true bugs. Proc. R. Soc. B Biol. Sci. 284, 1–10. doi: 10.1098/rspb.2017.1223
Li, J. J., Fang, X. M., Pan, B. T., Zhao, Z. J., and Song, Y. G. (2001). Late cenozoicintensive uplift of Qinghai-Xizang Plateau and its impacts on environments in surrounding area. Quat. Sci. 21, 381–391.
Liu, Y. P., Shen, Z. H., Wang, Q. G., Su, X. Y., Zhang, W. J., Shrestha, N., et al. (2017). Determinants of richness patterns differ between rare and common species: implications for Gesneriaceae conservation in China. Divers. Distrib. 23, 235–246. doi: 10.1111/ddi.12523
Liu, Z., Huang, X. L., Jiang, L. Y., and Qiao, G. X. (2009). The species diversity and geographical distribution of aphids in China (Hemiptera, Aphidoidea). Acta Zootaxonomica Sinica 34, 277–291.
Mao, L. F., Chen, S. B., Zhang, J. L., Hou, Y. H., Zhou, G. S., and Zhang, X. S. (2013). Vascular plant diversity on the roof of the world: spatial patterns and environmental determinants. J. Syst. Evol. 51, 371–381. doi: 10.1111/j.1759-6831.2012.00240.x
Marmion, M., Luoto, M., Heikkinen, R. K., and Thuiller, W. (2009). The performance of state-of-the-art modelling techniques depends on geographical distribution of species. Ecol. Model. 220, 3512–3520. doi: 10.1016/j.ecolmodel.2008.10.019
Myers, N., Mittermeier, R. A., Mittermeier, C. G., Da Fonseca, G. A. B., and Kent, J. (2000). Biodiversity hotspots for conservation priorities. Nature 403, 853–858. doi: 10.1038/35002501
O'Brien, E. (1998). Water-energy dynamics, climate, and prediction of woody plant species richness: an interim general model. J. Biogeogr. 25, 379–398. doi: 10.1046/j.1365-2699.1998.252166.x
Palmer, M. W. (1994). Variation in species richness: towards a unification of hypotheses. Folia Geobot. Phytotax. 29, 511–530. doi: 10.1007/BF02883148
Pennisi, E. (2005). What determines species diversity? Science 309:90. doi: 10.1126/science.309.5731.90
Qian, H., and Ricklefs, R. E. (2000). Large-scale processes and the Asian bias in species diversity of temperate plants. Nature 407, 180–182. doi: 10.1038/35025052
Ricklefs, R. E. (2004). A comprehensive framework for global patterns in biodiversity. Ecol. Lett. 7, 1–15. doi: 10.1046/j.1461-0248.2003.00554.x
Sandel, B., Arge, L., Dalsgaard, B., Davies, R. G., Gaston, K. J., Sutherland, W. J., et al. (2011). The influence of Late Quaternary climate-change velocity on species endemism. Science 334, 660–664. doi: 10.1126/science.1210173
Schuh, R. T., and Slater, J. A. (1995). True Bugs of the World (Hemiptera: Heteroptera): Classification and Natural History. Ithaca, NY: Cornell University press.
Shi, Y. F., Li, J. J., Li, B. Y., Yao, T. D., Wang, S. M., Li, S. J., et al. (1999). Uplift of the Qinghai-Xizang (Tibetan) Plateau and East Asia environmental change during late Cenozoic. Acta Geogr. Sin. 54, 20–28.
Shi, Y. F., Zheng, B. Y., and Yao, T. D. (1997). Glaciers and environments during the Last Glacial Maximum (LGM) on the Tibetan Plateau. J. Glaciol. Geocryol. 19, 97–113.
Stein, A., Beck, J., Meyer, C., Waldmann, E., Weigelt, P., and Kreft, H. (2015). Differential effects of environmental heterogeneity on global mammal species richness. Glob. Ecol. Biogeogr. 24, 1072–1083. doi: 10.1111/geb.12337
Stein, A., Gerstner, K., and Kreft, H. (2014). Environmental heterogeneity as a universal driver of species richness across taxa, biomes and spatial scales. Ecol. Lett. 17, 866–880. doi: 10.1111/ele.12277
Stevens, G. C. (1989). The latitudinal gradient in geographical range: how so many species coexist in the tropics. Am. Nat. 133, 240–256. doi: 10.1086/284913
Svenning, J. C., Normand, S., and Kageyama, M. (2008). Glacial refugia of temperate trees in Europe: Insights from species distribution modelling. J. Ecol. 96, 1117–1127. doi: 10.1111/j.1365-2745.2008.01422.x
Svenning, J. C., and Skov, F. (2005). The relative roles of environment and history as controls of tree species composition and richness in Europe. J. Biogeogr. 32, 1019–1033. doi: 10.1111/j.1365-2699.2005.01219.x
Tang, Z. Y., Wang, Z. H., Zheng, C. Y., and Fang, J. Y. (2006). Biodiversity in China's mountains. Front. Ecol. Environ. 4, 347–352. doi: 10.1890/1540-9295(2006)004[0347:BICM]2.0.CO;2
Turner, J. R. G., Gatehouse, C. M., and Corey, C. A. (1987). Does solar energy control organic diversity? Butterflies, moths and the British climate. Oikos 48, 195–205. doi: 10.2307/3565855
Turner, J. R. G., Lennon, J. J., and Lawrenson, J. A. (1988). British bird species distributions and the energy theory. Nature 335, 539–541. doi: 10.1038/335539a0
Wang, Q., Su, X. Y., Shrestha, N., Liu, Y. P., Wang, S. Y., Xu, X. T., et al. (2017). Historical factors shaped species diversity and composition of Salix in Eastern Asia. Sci. Rep. 7:42038. doi: 10.1038/srep42038
Wang, Z., Fang, J. Y., Tang, Z. Y., and Lin, X. (2011). Patterns, determinants and models of woody plant diversity in China. Proc. R. Soc. B Biol. Sci. 278, 2122–2132. doi: 10.1098/rspb.2010.1897
Wang, Z. H., Rahbek, C., and Fang, J. Y. (2012). Effects of geographical extent on the determinants of woody plant diversity. Ecography 35, 1160–1167. doi: 10.1111/j.1600-0587.2012.07786.x
Wang, Z. H., Tang, Z. Y., and Fang, J. Y. (2009). The species-energy hypothesis as a mechanism for species richness patterns. Biodivers. Sci. 17, 613–624. doi: 10.3724/SP.J.1003.2009.09161
Watanabe, S., Hajima, T., Sudo, K., Nagashima, T., Takemura, T., Okajima, H., et al. (2011). MIROC-ESM 2010: model description and basic results of CMIP5-20c3m experiments. Geosci. Model Dev. 4, 845–872. doi: 10.5194/gmd-4-845-2011
Wei, J. F., Niu, M. M., and Feng, J. N. (2016). Diversity and distribution patterns of scale insects in China. Ann. Entomol. Soc. Am. 109, 405–414. doi: 10.1093/aesa/sav159
Wiens, J. J., and Donoghue, M. J. (2004). Historical biogeography, ecology and species richness. Trends Ecol. Evol. 19, 639–644. doi: 10.1016/j.tree.2004.09.011
Willig, M. R., Kaufman, D. M., and Stevens, R. D. (2003). Latitudinal gradients of biodiversity: Pattern, process, scale, and synthesis. Ann. Rev. Ecol. Evol. Syst. 34, 273–309. doi: 10.1146/annurev.ecolsys.34.012103.144032
Wu, Y. J., Colwell, R. K., Rahbek, C., Zhang, C. L., Quan, Q., Wang, C. K., et al. (2013). Explaining the species richness of birds along a subtropical elevational gradient in the Hengduan Mountains. J. Biogeogr. 40, 2310–2323. doi: 10.1111/jbi.12177
Xu, L. B., Ou, X. J., Lai, Z. P., Zhou, S. Z., Wang, J., and Fu, Y. (2010). Timing and style of late Pleistocene glaciation in the Queer Shan, northern Hengduan Mountains in the eastern Tibetan Plateau. J. Quat. Sci. 25, 957–966. doi: 10.1002/jqs.1379
Yan, Y., Yang, X., and Tang, Z. Y. (2013). Patterns of species diversity and phylogenetic structure of vascular plants on the Qinghai-Tibetan Plateau. Ecol. Evol. 3, 4584–4595. doi: 10.1002/ece3.847
Yang, F. S., Li, Y. F., Ding, X., and Wang, X. Q. (2008). Extensive population expansion of Pedicularis longiflora (Orobanchaceae) on the Qinghai-Tibetan Plateau and its correlation with the quaternary climate change. Mol. Ecol. 17, 5135–5145. doi: 10.1111/j.1365-294X.2008.03976.x
Yuan, S., Huang, M., Wang, X. S., Ji, L. Q., and Zhang, Y. L. (2014). Centers of endemism and diversity patterns for typhlocybine leafhoppers (Hemiptera: Cicadellidae: Typhlocybinae) in China. Insect Sci. 21, 523–536. doi: 10.1111/1744-7917.12040
Zhang, C., Quan, Q., Wu, Y. J., Chen, Y. H., He, P., Qu, Y. H., et al. (2016). Topographic heterogeneity and temperature amplitude explain species richness patterns of birds in the Qinghai-Tibetan Plateau. Curr. Zool. 63, 131–137. doi: 10.1093/cz/zow024
Zhang, G. X., and Zhong, T. S. (1988). “Homoptera, Aphidoidea,” in Insects of Mt. Namjagbarwa region of Xizang, ed F. S. Huang (Beijing: Science Press), 167–171.
Zhang, Y. L., Li, B. Y., and Zheng, D. (2002). A discussion on the boundary and area of the Tibetan Plateau in China. Geogr. Res. 21, 1–8. doi: 10.11821/yj2002010001
Zhang, Z. (2011). Animal biodiversity: an outline of higher-level classification and survey of taxonomic richness. Zootaxa 3148, 1–237. doi: 10.11646/zootaxa.3148.1.1
Zhang, Z., Yan, Y., Tian, Y., Li, J., He, J. S., and Tang, Z. (2015). Distribution and conservation of orchid species richness in China. Biol. Conserv. 181, 64–72. doi: 10.1016/j.biocon.2014.10.026
Keywords: biodiversity, distribution, environmental variables, habitat heterogeneity, Hemiptera, Random Forest, species richness
Citation: Li J, Liu H, Wu Y, Zeng L and Huang X (2019) Spatial Patterns and Determinants of the Diversity of Hemipteran Insects in the Qinghai-Tibetan Plateau. Front. Ecol. Evol. 7:165. doi: 10.3389/fevo.2019.00165
Received: 24 January 2019; Accepted: 25 April 2019;
Published: 15 May 2019.
Edited by:
Hang Sun, Kunming Institute of Botany (CAS), ChinaReviewed by:
Saeed Mohammadi, University of Zabol, IranJian Zhang, East China Normal University, China
Copyright © 2019 Li, Liu, Wu, Zeng and Huang. This is an open-access article distributed under the terms of the Creative Commons Attribution License (CC BY). The use, distribution or reproduction in other forums is permitted, provided the original author(s) and the copyright owner(s) are credited and that the original publication in this journal is cited, in accordance with accepted academic practice. No use, distribution or reproduction is permitted which does not comply with these terms.
*Correspondence: Xiaolei Huang, aHVhbmd4bEBmYWZ1LmVkdS5jbg==