- 1Otago Palaeogenetics Laboratory, Department of Zoology, University of Otago, Dunedin, New Zealand
- 2Canterbury Museum, Christchurch, New Zealand
- 3Manaaki Whenua Landcare Research, Lincoln, New Zealand
- 4Department of Anatomy, University of Otago, Dunedin, New Zealand
New Zealand's unique biodiversity is the product of at least 55 million years of geographic isolation, supplemented by persistent transoceanic migration. Palaeontological and genetic evidence suggest most New Zealand avifauna has colonized from Australia. We synthesize evolutionary genetic studies to show a previously unrecognized clustering of divergence times in Australian and New Zealand bird species pairs, across the avian phylogeny at the beginning of the Pleistocene, around 2.5 million years ago. The timing coincides with major climatic and vegetation changes with the initiation of the Plio-Pleistocene glacial cycles. Recent anthropogenic impacts and environmental modifications are replicating in some important ways Pleistocene glacial landscapes, resulting in a new wave of avian “native invaders” into New Zealand.
Background
New Zealand has long been regarded as a model system for understanding Southern Hemisphere biogeography and evolution. It is one of the most isolated continental fragments (Zealandia; Mortimer et al., 2017) of the supercontinent Gondwana that was completely severed by ocean around 55 million years ago (Mya) (Roelants and Bossuyt, 2005). It has an excellent fossil record (Worthy and Holdaway, 2002; Worthy et al., 2017), and is home to extant taxa that are known from the old supercontinent (e.g., Giribet and Boyer, 2010; Easton et al., 2017; Wallis and Jorge, 2018).
While its geographic isolation and geological history appears to demand a vicariance explanation for the origin of its biodiversity, there is increasing evidence that New Zealand is rather a “fly paper of the Pacific” (McGlone, 2005) with a constant flow of immigrant taxa (Flemming, 1962; McDowall, 2008; Gibbs, 2017; Wallis and Jorge, 2018). Many New Zealand plants and animals, once considered ancient, have been shown to be more recent transoceanic immigrants arriving long after the separation of Zealandia from eastern Gondwana (e.g., Knapp et al., 2005; Mitchell et al., 2014). The oceans surrounding the New Zealand archipelago are a permeable barrier (e.g., McCulloch et al., 2017) and, while the focus has been on species mobility, establishment and persistence of immigrants is just as important (Boessenkool et al., 2009; Collins et al., 2014; Rawlence et al., 2015, 2017a; Grosser et al., 2016; Waters and Grosser, 2016; Waters et al., 2017).
Birds are often highly mobile and many are adept at forming breeding populations after crossing ocean barriers (e.g., white eyes Zosterops spp.; Cornetti et al., 2015). Most post-Gondwanan avian immigration to New Zealand is from the closest landmass, Australia (e.g., Scofield et al., 2017; Wallis and Jorge, 2018), which is favorably situated with regard to prevailing winds and ocean currents, although some taxa have their nearest relatives in Asia (e.g., Gibb et al., 2015) or Melanesia (e.g., Boon et al., 2001).
New Zealand has endured more profound geological, topographic, and climatic changes since its separation from eastern Gondwana than any other continental landmass, Antarctica aside (Mortimer et al., 2017). Shortly after the separation of Zealandia, thinning and cooling of its continental crust as rifting ceased resulted in a loss of buoyancy and submergence. No more than 7% of the original Zealandian landmass remained emergent (Mortimer et al., 2017). The portion that was to be the New Zealand archipelago sank; weathering and erosion wore down the original mountainous landmass, and rising sea levels completed a process that culminated in the near-complete marine inundation (up to 80% of New Zealand) during the Oligocene 35–25 Mya (Worthy et al., 2017). Zealandia is therefore best thought of not as a coherent terrestrial entity, but rather as a region of the ocean where diverse geological processes have created a scatter of archipelagos and ephemeral islands (Grandcolas, 2017). While a complete submergence of the New Zealand region has been suggested (Landis et al., 2008), recent geological, palaeontological (e.g., Conran et al., 2014; Scott et al., 2014; Worthy et al., 2017) and genetic research (e.g., Carr et al., 2015; Wallis and Jorge, 2018) strongly favors a small emergent archipelago at that time. Wallis and Jorge (2018) synthesized previous evolutionary genetic studies and found around 75% of 248 New Zealand lineages survived the marine transgression in situ, the majority non-volant terrestrial and freshwater taxa, and trees.
A highly diverse flora and fauna (e.g., Saint Bathans Fauna, Worthy et al., 2017) flourished under the subtropical climate of the early to middle Miocene. The first significant mountain ranges in the South Island arose in the middle Miocene (Nathan et al., 1986). Rapid uplift along the Alpine Fault system (which runs the length of the Southern Alps) began ca. 8 Mya. These rising ranges intercepted the prevailing westerly wind flow, creating rain-shadow climates in the east from the late Miocene onwards (Chamberlain et al., 1999). Climatic change, disruption of the previous low-lying stable landscape and an ever-changing archipelago configuration accelerated biotic turnover with the loss of earlier taxa and a continuing influx of new types (McGlone et al., 2016). An abrupt reorganization of the plate tectonic regime ca. 5 Mya was followed by rapid uplift of erosion-resistant greywacke that began ca. 4 Mya in the far south, and propagated northwards, with tall mountainous relief as late as 1.3 Mya in the northwest of the South Island (Batt and Braun, 1999).
Mountains of the northeastern South Island, the axial ranges of the North Island and the Volcanic Plateau arose later, and in the southern North Island, mountain ranges began to form only about 500, 000 years ago (McGlone et al., 2001). Global cooling from 2.7 Mya initiated the Pleistocene cycles of cold glacials and warm interglacials (Wallis et al., 2016; Craw et al., 2017). Local rain shadow aridification intensified in the southern and eastern regions of New Zealand (Craw et al., 2013). During the Pleistocene glacials the dense evergreen forests that had dominated the archipelago since separation from eastern Gondwana were fragmented into coastal fringes or inland patches on favorable sites from the middle of the North Island southwards, with continuous forest largely confined to northern New Zealand (Newnham et al., 2013). Over most of the archipelago during glacial maxima, shrubland-grassland, and bare ground mosaics prevailed (Newnham et al., 2013).
Human arrival around 750 years ago (Wilmshurst et al., 2008, 2011), during a period of relative climatic stability (Wanner et al., 2008; Allen, 2012; Waters et al., 2017), resulted in the extinction of nearly 50% of the unique avifauna (Tennyson and Martinson, 2007) and widespread destruction of forests (McWethy et al., 2014). Fire induced open shrub and grassland habitats structurally similar to those of the Pleistocene glacials (McGlone, 2009).
Much of the non-endemic indigenous biodiversity of New Zealand is of Australian origin (for example, over 200 plant species are shared between Tasmania and New Zealand; Jordan, 2001) and typical of open habitats, which suggests the Plio-Pleistocene glacials created new opportunities for Australian biota (McGlone et al., 2001; McGlone, 2006; Wood et al., 2017). Here, through a synthesis of molecular dating studies utilizing modern and ancient DNA, we can demonstrate the effect of Plio-Pleistocene environmental changes on the New Zealand avifauna, but also narrow down the timing for the start of the avian influx.
Plio-Pleistocene Climatic Changes and Their Impact on the New Zealand Avifauna
To establish a breeding population in New Zealand, an immigrant Australian bird species requires an ecological niche similar to that of its home range, be that niche broad or restricted (e.g., Grosser et al., 2016), but with time and isolation is likely to develop new, distinctive niches. The Australasian black-winged stilt (Himantopus himantopus leucocephalus), for example, is the Indo-Australian representative of a widespread species, while its New Zealand sister taxa the black stilt (Himantopus novaezelandiae) has specialized in braided river ecosystems.
While a large number of Australian bird species are regular vagrants to New Zealand (Gill et al., 2010; Miskelly et al., 2015), establishment rates are very low. However, the chances of a significant subset of these vagrants establishing over geological time is high. All else being equal, establishment over the Neogene, that is the last 23 Mya (Wallis and Jorge, 2018), should have resulted in an even spread of divergence times between Australian and New Zealand sister species. During most of the Neogene southern Australia and New Zealand were at similar latitudes and shared a similar moist, warm climate and dense forest cover (Heenan and McGlone, 2013). This niche compatibility would have favored immigration. However, New Zealand lacked mammalian predators and the avian fauna had to contend with aerial predation alone. The extant native avifauna expends less energy on anti-predator strategies and therefore tends to be more resource-efficient than exotic birds (Worthy and Holdaway, 2002). For instance, low clutch sizes are typical of deep-endemic New Zealand bird species (Worthy and Holdaway, 2002). We argue that this factor reduced the probability of Australian birds establishing. Of the 15 New Zealand–Australasian avian sister species pairs examined by Wallis and Jorge (2018), seven had mean divergence dates prior to the Plio-Pleistocene transition, ranging in age from ~33 to 7 Mya. Most of these species pairs were forest specialists, with a small proportion of wetland and marine species (Marchant and Higgins, 1990). Examples include New Zealand's tui (Prosthemadera novaeseelandiae) and bellbird (Anthornis melanura), and Australia's white-fronted chat (Epithianura albifrons), with divergence times of approximately 15 Mya; and New Zealand and Australian Petroica robins—divergence time 3.5 to 5 Mya (Gibb et al., 2015; Kearns et al., 2018).
While Neogene divergences are relatively few and spread over a long period of time (Wallis and Jorge, 2018), a number of divergence times between well-accepted Australian and New Zealand avian sister species (see also Rawlence and Waters, 2018) converge around the Late Pliocene and Early Pleistocene (Figure 1; Table 1). The majority of Australian birds in these species pairs (see Table 1) are open habitat specialists (Marchant and Higgins, 1990). The pairs are spread across the avian phylogeny and include raptors (spotted harrier Circus assimilis and the extinct Eyles' harrier C. teauteensis [diverged 2.4 Mya]; little eagle Hieraaetus morphnoides and the extinct Haast's eagle Hieraaetus moorei [diverged 2.2 Mya]; Knapp et al., 2019); rails (Southwest Pacific swamp-hen Porphyrio melanotus and the Moho P. mantelli [diverged 1.47 Mya]; Southwest Pacific swamp-hen and South Island takahe P. hochstetteri [diverged 2.4 Mya]; both Garcia and Trewick, 2015), and ravens (the Australian forest raven Corvus tasmanicus and the extinct New Zealand raven C. antipodum [diverged 1.7 Mya]; Scofield et al., 2017). Waders (Australasian black-winged stilt H. himantopus leucocephalus and the black stilt H. novaezelandiae; Chambers and MacAvoy, 1997; Wallis, 1999) are perhaps an outlier to this general pattern, with an estimated mean divergence time of only about 1 Mya. However, the Australasian black-winged/black stilt split is an estimate based off percentage sequence divergence (i.e., 5% partial D-loop/control region equating to a divergence time of ca. 1 Mya; Wallis, 1999)—all other divergence dates are based off partial or whole mitogenome phylogenetic analyses incorporating fossil calibration points.
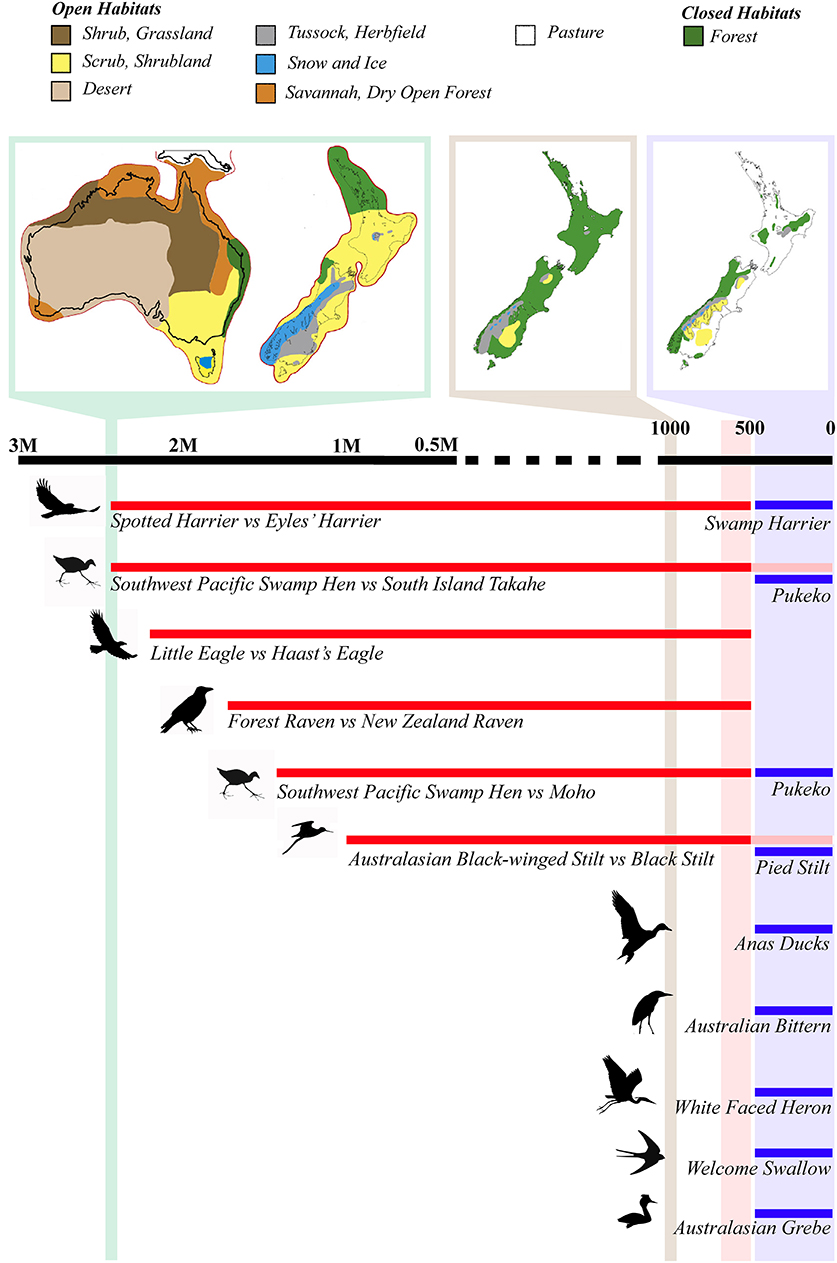
Figure 1. Large scale synchronous biological turnover in avifauna during the Plio-Pleistocene transition and Late Holocene of New Zealand. Schematic of the Australian avian lineages that colonized New Zealand ca. 2.5 Mya with the increase in open habitat in New Zealand, and after the arrival of humans due to widespread Holocene forest clearance and species extinction. The Last Glacial Maximum vegetation of Australia and New Zealand (green bar), as a proxy for the onset of Pleistocene glaciations 2.7 Mya; pre-human Holocene forest cover (ca. 1000 years ago; tan bar); and post-human vegetation (ca. 500 years ago; blue bar) are shown above the timeline to illustrate the amount of available open vs. closed habitat (modified from Goldberg et al., 2015). Divergence time estimates are mean values based on published molecular divergence dates. The red bar represents the megafaunal extinction period in New Zealand, from the arrival of humans ca. 1280 AD to the consequent extinctions ca. 1450 AD, while the blue bar represents the post-extinction era, when numerous Australian avian species colonized New Zealand.
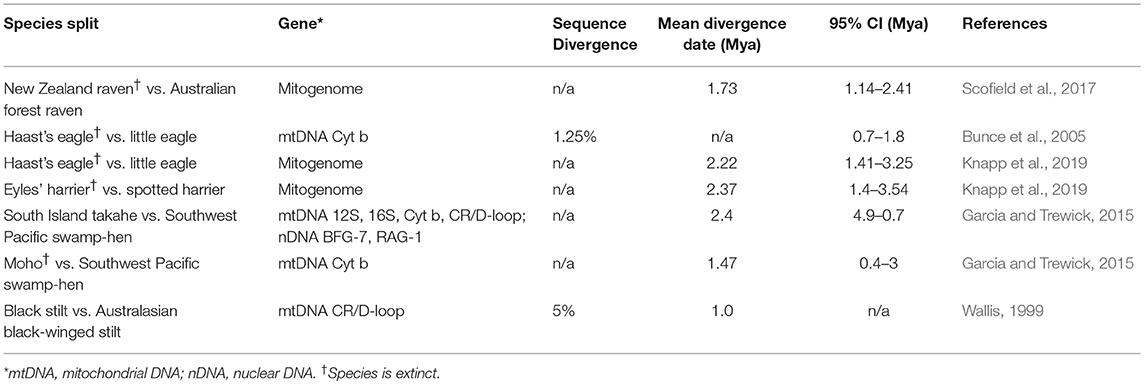
Table 1. Divergence time estimates for splits between Australian and New Zealand avian species pairs.
While the number of Australian-New Zealand sister species for which we have divergence times is limited, the emerging pattern of early Pleistocene divergences appears to represent a watershed moment in avian evolution in New Zealand. This being so, which factors have driven the increased establishment success for the Australian avifauna?
The aridification of Australia and the expansion of open habitats intensified from around 10 Mya onwards (MacPhail, 1997; McLeish et al., 2007) while New Zealand remained wet and densely forested (Heenan and McGlone, 2013). The Australian avifauna gained a large number of open-country birds while the New Zealand avifauna remained largely forest-dwellers. The onset of the Pleistocene glacial cycles led to the periodic reduction and fragmentation of forest in New Zealand and the development of open shrubland-grassland habitats, particularly in the rain-shadow region to the east. With the contemporaneous rapid rise of the Southern Alps, increased eastern aridity and formation of fast-flowing braided rivers provided permanent, although limited open country niches during otherwise forested interglacials (Rawlence et al., 2012; Newnham et al., 2013).
Cooling and mountain building meant that by the early Pleistocene New Zealand has, for the first time, extensive expanses of open habitats functionally comparable to those prevalent in Australia since 10 Mya. The arriving pre-adapted open habitat specialists from Australia therefore encountered niches familiar to them, but alien to the largely forest-adapted New Zealand avifauna. While some indigenous forest specialists may have evolved to occupy these new niches, we suggest they were largely filled by immigration of Australian specialists—a much more rapid process. Once isolated in New Zealand in the absence of terrestrial mammalian predators, these colonizers evolved into distinctive species different from both their Australian ancestors and the older endemic New Zealand avifauna (Rawlence and Waters, 2018).
Some other Australian-New Zealand avifaunal species pairs are likely to have divergence times ca. 2.5 Mya. The largest group of candidate species pairs are waterfowl and rails including the Australian coot (Fulica atra australis) and the extinct New Zealand coots (F. prisca and chathamensis; Trewick, 1997); Australian black swan (Cygnus atratus) and the extinct Pouwa (C. sumnerensis; Rawlence et al., 2017a); Cape Barren goose (Cereopsis novaehollandiae) and the extinct North/South Island goose (Cnemiornis gracilis and calcitrans); Australian shelduck (Tadorna tadornoides) and paradise shelduck (Tadorna variegata); and several other Anseriform species pairs (e.g., Tennyson and Martinson, 2007). Some of these species pairs are controversial (see Dussex et al., 2018 cf. Rawlence and Waters, 2018), but future research focusing on lineage divergence within a rigorous statistical framework will resolve these issues.
History Repeats: Avifaunal COLONIZATION in the Post-Extinction Era
The arrival of Polynesians in the late thirteenth century AD (Wilmshurst et al., 2008, 2011), and later Europeans and their commensal animals, dramatically reshaped New Zealand ecosystems (McGlone et al., 1994; Worthy and Holdaway, 2002; Tennyson and Martinson, 2007). Nearly 50% of New Zealand's endemic avifauna (including taxa such as the New Zealand raven, Eyles' harrier, Haast's eagle, Moho, New Zealand coot and the Pouwa) were extinct by ca. 1450–1500 AD within a few hundred years of Polynesian settlement (Tennyson and Martinson, 2007; Boessenkool et al., 2009; Rawlence et al., 2017a,b). Avifaunal extinctions and habitat modification occurred during a period of climatic stability (Wanner et al., 2008; Allen, 2012; Waters et al., 2017) and we can be sure extinction resulted from hunting, habitat destruction and predation by introduced predators (e.g., Polynesian dog/kuri, Canis familaris; Polynesian rat/kiore Rattus exulans; Tennyson and Martinson, 2007; Seersholm et al., 2018). The disappearance of native birds dramatically increased niche availability (e.g., Collins et al., 2014; Grosser et al., 2016; Waters and Grosser, 2016; Waters et al., 2017). Mammalian predators would have also increased the competitive status of avian colonists which, unlike endemics, have predator avoidance behaviors and larger, more frequent clutches.
Widespread burning led to the loss of at least 30% of Holocene forests (covering 85–90% of New Zealand) and their replacement by fast growing open shrubland-fern-grassland mosaics subject to repeated fire (McGlone, 1989). The loss was more complete in the drier, eastern, “rain shadow” areas, and fire-prone tussock grassland and shrubland spread across the foothills and plains (McGlone, 1989, 2001; Perry et al., 2012). Such habitat was restricted in the Holocene and the interglacials but was widespread during the glacials (Newnham et al., 2013). While by no means identical to the grasslands, shrublands, and wetlands of the glacial periods, functionally this newly transformed habitat resembled them closely (McGlone, 2006; Newnham et al., 2013). Loss of forest decreased catchment level evapotranspiration leading to higher water yield and thus the proliferation of open, fire-swept sedge and reed swamps where previously wooded wetlands had prevailed (McGlone, 2009). Fire had been restricted and infrequent before human arrival and few New Zealand plants were adapted to it (McGlone, 2009; McGlone et al., 2014; Perry et al., 2014). Conspecific Australian plants (most already present) or endemic species closely related to Australian plants were among the few adapted to fire and spread rapidly, including bracken fern (Pteridium esculentum), raupo (Typha orientalis), tussock grasses, manuka (Leptospermum scoparium), kanuka (Kunzea ericoides), and cabbage tree (Cordyline australis). Forest clearance by European settlers from the early nineteenth century onwards converted large areas to pasture or shrubland (McWethy et al., 2014).
Human transformation of the New Zealand landscape, and increased niche availability, increased the number of Australian avifauna establishing (Waters and Grosser, 2016) to levels not seen since the early Pleistocene. As many as 16 species of Australian avifauna self-introduced (Holdaway et al., 2001; Waters and Grosser, 2016), often filling the niches vacated by extinct or decimated native species. Examples of such extinction-recolonisation patterns include rails (Southwest Pacific swamp-hen/pukeko Porphyrio melanotus replacing Moho/South Island takahe P. hochstetteri/mantelli), raptors (swamp harrier Circus approximans replaced Eyles' harrier C. teauteensis), and waders (pied stilt Himantopus leucocephalus replacing black stilt H. himantopus) (Worthy and Holdaway, 2002; Waters and Grosser, 2016). None of these occur in either natural or early archaeological sites (1280-1450 AD) that date to before the decline of their New Zealand congeners (Worthy and Holdaway, 2002; Gill et al., 2010). A similar pattern may have occurred between the subantarctic islands and the New Zealand mainland. Many current coastal and marine species were sparsely represented as occasional subantarctic vagrants in coastal archaeological middens but only established breeding populations after the demise of their mainland congeners due to Polynesian exploitation (e.g., Boessenkool et al., 2009; Rawlence et al., 2015, 2017a; Waters et al., 2017).
McGlone (2006) noted that the more transformed New Zealand lowland habitats were the more likely the dominant taxa are generalist indigenous species of Australian and southwest Pacific origin. These include the same “species” that first colonized during the early Pleistocene (e.g., Rawlence et al., 2017a). For example, 1–2.5 Mya, the Australian ancestors of the Southwest Pacific swamp-hen and Australasian black-winged stilt evolved into South Island takahe, Moho, and black stilt. Since human settlement, Southwest Pacific swamp-hen and pied stilt have reappeared. For some self-introductions there was a lag period of up to several hundred years before population expansion (e.g., spoonbills cf. black-fronted dotterels; Gill et al., 2010). Not all have been successful: numerous species regularly occur as vagrants but have not been able to form breeding populations (e.g., cattle egret, fairy martin; Gill et al., 2010).
Some recent Australian colonists may have been competition-limited generalists in their native Australia whereas the endemic New Zealand birds they replaced were habitat specialists (Worthy and Holdaway, 2002). The endemic Eyles' harrier was a specialist forest harrier, whereas the ancestral swamp harrier in Australia is only found within ca. 15 km of swamps. However, after its recent arrival in New Zealand the swamp harrier has adapted to closed forest, open farmland and the sub-Antarctic islands (Worthy and Holdaway, 2002; Knapp et al., 2019).
If open land habitat facilitates the establishment of Australian birds in New Zealand, why do divergences cluster in the early-mid Pleistocene and in the last few centuries, rather than spiking during each of the Pleistocene glaciations? A constant stream of Australian avifauna must have arrived as vagrants during Pleistocene glaciations when suitable open habitat was maximized and yet, as far as can be told, few if any established. A possible contributing factor to this pattern may be that we have not yet identified all the early colonizers as the pre-late Pleistocene record is rather sparse. We know some did not survive until human arrival. For example, the large, flightless Fleming's rail (Pleistorallus flemingi) is only found, to date, in strata approximately 1 Mya (Worthy, 1997). Nevertheless, we suggest that this pattern largely resulted from ecological “founder takes all” and “density dependent blocking” processes (Waters et al., 2013) once available niches were filled in the early Pleistocene. Polynesian settlement and then European colonization emptied many of these niches via extinctions.
A different pattern of colonization has occurred in species associated with habitats that have always been shared between New Zealand and Australia, for example coastal/marine and some swamp species (cf. forest specialists; McGlone, 2006; Gill et al., 2010; Gibb et al., 2015; Waters and Grosser, 2016; Wallis and Jorge, 2018). Endemism is lower and less deep in these groups, and there has been more regular contact. For example, during 2013–2014 AD interval, nine vagrant taxa of open habitat/wetlands were recorded in New Zealand vs. 26 vagrant seabird taxa (Miskelly et al., 2015).
Conclusion
While Australia has always been a major source for the New Zealand avifauna, the lack of mammalian predators in New Zealand kept Neogene avian immigration to a trickle through competition from the endemic resource-efficient forest specialists. The onset of the Pleistocene glacial cycles at 2.7 Mya and associated mountain building, reduced the forest cover and created new, open habitats similar in many respects to those widespread in Australia over millions of years. As a result, there was an influx of open habitat specialists from Australia representing a watershed moment in the evolution of New Zealand's avifauna. Human settlement from the thirteenth century on has, as with the Pleistocene glaciations, created a vast amount of open habitat and has further tilted the balance in favor of immigration through the introduction of mammalian predators. Elimination of 50% of the previous terrestrial avifauna and failure of many of the surviving species to adapt to mammalian predation has resulted in a fresh wave of self-introduced avian invaders.
Author Contributions
NR, RPS, MM, and MK devised the study, analyzed the data, and wrote the manuscript.
Funding
NR and MK are funded by a Royal Society of New Zealand Marsden Grant (16-UOO-096) and a Rutherford Discovery Fellowship (14-UOO-007), respectively.
Conflict of Interest Statement
The authors declare that the research was conducted in the absence of any commercial or financial relationships that could be construed as a potential conflict of interest.
Acknowledgments
We thank Jon Waters and Graham Wallis for helpful discussion about this manuscript, and Lachie Scarsbrook for help with figure construction. We thank both reviewers whose comments greatly improved this manuscript.
References
Allen, M. S. (2012). Molluscan foraging efficiency and patterns of mobility amongst foraging agriculturalists: a case study from northern New Zealand. J. Arch. Sci. 39, 295–307. doi: 10.1016/j.jas.2011.09.013
Batt, G. E., and Braun, J. (1999). The tectonic evolution of the Southern Alps, New Zealand: insights from fully thermally coupled dynamical modelling. Geophys. J. Int. 136, 403–420. doi: 10.1046/j.1365-246X.1999.00730.x
Boessenkool, S., Austin, J. J., Worthy, T. H., Scofield, P., Cooper, A., Seddon, P. J., et al. (2009). Relict of colonizer? Extinction and range expansion of penguins in southern New Zealand. Proc. B 276, 815–821. doi: 10.1098/rspb.2008.1246
Boon, W. M., Kearvell, J. C., Daugherty, C. H., and Chambers, G. M. (2001). Molecular Systematics and Conservation of Kakariki (Cyanoramphus spp.). Wellington: Department of Conservation, 176.
Bunce, M., Szulkin, M., Lerner, H. R., Barnes, I., Shapiro, B., Cooper, A., et al. (2005). Ancient DNA provides new insights into the evolutionary history of New Zealand's extinct giant eagle. PLoS Biol. 3:e9. doi: 10.1371/journal.pbio.0030009
Carr, L. M., McLenachan, P. A., Waddell, P. J., Gemmell, N. J., and Penny, D. (2015). Analyses of the mitochondrial genome of Leiopelma hochstetteri argues against the full drowning of New Zealand. J. Biogeogr. 42, 1066–1076. doi: 10.1111/jbi.12482
Chamberlain, C. P., Poage, M. A., Craw, D., and Reynolds, R. C. (1999). Topographic development of the Southern Alps recorded by the isotopic composition of authigenic clay minerals, South Island, New Zealand. Chem. Geol. 155, 279–294. doi: 10.1016/S0009-2541(98)00165-X
Chambers, G. K., and MacAvoy, E. S. (1997). Molecular Genetic Analysis of Hybridization. Wellington: Department of Conservation, 105.
Collins, C. J., Rawlence, N. J., Prost, S., Anderson, C. N., Knapp, M., and Scofield, R. P., et al. (2014). Extinction and recolonization of coastal megafauna following human arrival in New Zealand. Proc. B. 281:20140097. doi: 10.1098/rspb.2014.0097
Conran, J. G., Mildenhall, D. C., Lee, D. E., Lindqvist, J. K., Shepherd, C., and Beu, A. G., et al. (2014). Subtropical rainforest vegetation from Cosy Dell, Southland: plant fossil evidence for Late Oligocene terrestrial ecosystems. N. Z. J. Geol. Geophys. 57, 236–252. doi: 10.1080/00288306.2014.888357
Cornetti, L., Valente, L. M., Dunning, L. T., Quan, X., Black, R. A., Hébert, O., et al. (2015). The genome of the “great speciator” provides insights into bird diversification. Gen. Biol. Evol. 7, 2680–2691. doi: 10.1093/gbe/evv168
Craw, D., Druzbicka, J., Rugaut, C., and Waters, J. (2013). Geological controls on palaeo-environmental change in a tectonic rain shadow, southern New Zealand. Palaeogeogr. Palaeoclimatol. Palaeoecol. 370, 103–116. doi: 10.1016/j.palaeo.2012.11.024
Craw, D., Upton, P., Waters, J., and Wallis, G. (2017). Biological memory of the first Pleistocene glaciation in New Zealand. Geology 45, 595–598. doi: 10.1130/G39115.1
Dussex, N., Taylor, H. R., Irestedt, M., and Robertson, B. C. (2018). When genetic and phenotypic data do not agree: the conservation implications of ignoring inconvenient taxonomic evidence. N. Z. J. Ecol. 42, 284–290. doi: 10.20417/nzjecol.42.13
Easton, L. J., Rawlence, N. J., Worthy, T. H., Tennyson, A. J. D., Scofield, R. P., Easton, C. J., et al. (2017). Testing species limits of New Zealand's Leiopelmatid frogs through morphometric analyses. Zool. J. Linn. Soc. 183, 431–444. doi: 10.1093/zoolinnean/zlx080
Garcia, R. J. C., and Trewick, S. A. (2015). Dispersal and speciation in purple swamphens (Rallidae: Porphyrio). Auk 132, 140–155. doi: 10.1642/AUK-14-114.1
Gibb, G. C., England, R., Hartig, G., McLenachan, P. A., Smith, B. L., McCormish, B. J., et al. (2015). New Zealand passerines help clarify the diversification of major songbird lineages during the Oligocene. Gen. Biol. Evol. 7, 2983–2995. doi: 10.1093/gbe/evv196
Gill, B. J., Bell, B. D., Chambers, G. K., Medway, D. G., Palma, R. L., Scofield, R. P., et al. (2010). Checklist of the Birds in New Zealand, Norfolk and Macquarie Islands, and The Ross Dependency Antarctica, 4th Edn. Wellington: Te Papa Press in association with the Ornithological Society of New Zealand.
Giribet, G., and Boyer, S. L. (2010). ‘Moa's Ark’ or ‘Goodbye Gondwana’: is the origin of New Zealand's terrestrial invertebrate fauna ancient, recent, or both? Invert. Syst. 24, 1–8. doi: 10.1071/IS10009
Goldberg, J., Morgan-Richards, M., and Trewick, S. A. (2015). Intercontinental island hopping: colonization and speciation of the grasshopper genus Phaulacridium (Orthoptera: Acrididae) in Australasia. Zool. Anz. 255, 71–79. doi: 10.1016/j.jcz.2015.02.005
Grandcolas, P. (2017). Ten false ideas about New Caledonia biogeography. Cladistics 33, 481–487. doi: 10.1111/cla.12176
Grosser, S., Rawlence, N. J., Anderson, C. N. K., Smith, I. W. G., Scofield, R. P., and Waters, J. M. (2016). Invader or resident? Ancient-DNA reveals rapid species turnover in New Zealand. Proc. B. 283:20152879. doi: 10.1098/rspb.2015.2879
Heenan, P. B., and McGlone, M. S. (2013). Evolution of New Zealand alpine and open-habitat plant species during the late Cenozoic. N. Z. J. Ecol. 37, 105–113.
Holdaway, R. N., Worthy, T. H., and Tennyson, A. J. D. (2001). A working list of breeding bird species of the New Zealand region at first human contact. N. Z. J. Zool. 28, 119–187. doi: 10.1080/03014223.2001.9518262
Jordan, G. J. (2001). An investigation of long-distance dispersal based on species native to both Tasmania and New Zealand. Aust. J. Bot. 49, 333–340. doi: 10.1071/BT00024
Kearns, A. M., Joseph, L., Thierry, A., Malloy, J. F., Cortes-Rodriguez, M. N., and Omland, K. E. (2018). Diversification of Petroica robins across the Australo-Pacific region: first insights into the phylogenetic affinities of New Guinea's highland robin species. Emu. doi: 10.1080/01584197.2018.1498744. [Epub ahead of print].
Knapp, M., Stöckler, K., Havell, D., Delsuc, F., Sebastiani, F., and Lockhart, P. J. (2005). Relaxed molecular clock provides evidence for long-distance dispersal of Nothofagus (Southern Beech). PLoS Biol. 3:e14. doi: 10.1371/journal.pbio.0030014
Knapp, M., Thomas, J. E., Haile, J., Prost, S., Ho, S. Y. W., Dussex, N., et al. (2019). Mitogenomic evidence of close relationships between New Zealand's extinct giant raptors and small-sized Australian sister-taxa. Mol. Phylogenet. Evol. 134, 122–128. doi: 10.1016/j.ympev.2019.01.026
Landis, C. A., Campbell, H. J., Begg, J. G., Mildenhall, D. C., Paterson, A. M., and Trewick, S. A. (2008). The Waipounamu erosion surface: questioning the antiquity of the New Zealand land surface and terrestrial fauna and flora. Geol. Mag. 145, 173–197. doi: 10.1017/S0016756807004268
MacPhail, M. (1997). Late Neogene climates in Australia: fossil pollen-and spore-based estimates in retrospect and prospect. Austral. J. Bot. 45, 425–464. doi: 10.1071/BT96052
Marchant, S., and Higgins, P. J. (1990). Handbook of Australian, New Zealand and Antarctic birds, Vol. 1 Ratites to Ducks. Melbourne, VIC: Oxford University Press.
McCulloch, G. A., Wallis, G. P., and Waters, J. M. (2017). Does wing size shape insect biogeography? Evidence from a diverse regional stonefly assemblage. Glob. Ecol. Biogeogr. 26, 93–101. doi: 10.1111/geb.12529
McDowall, R. M. (2008). Process and pattern in the biogeography of New Zealand – a global microcosm. J. Biogeogr. 35, 197–212. doi: 10.1111/j.1365-2699.2007.01830.x
McGlone, M. S. (1989). The Polynesian settlement of New Zealand in relation to environmental and biotic changes. N. Z. J. Ecol. 12, 115–129.
McGlone, M. S. (2001). The origin of the indigenous grasslands of southeastern South Island in relation to pre-human woody ecosystems. N. Z. J. Ecol. 25, 1–15.
McGlone, M. S. (2005). Goodbye Gondwana. J. Biogeogr. 32, 739–740. doi: 10.1111/j.1365-2699.2005.01278.x
McGlone, M. S. (2006). “Becoming New Zealanders: immigration and the formation of the biota,” in Biological Invasions in New Zealand, eds R. B. Allen and W. G. Lee (Berlin: Springer), 17–32.
McGlone, M. S. (2009). Postglacial history of New Zealand wetlands and implications for their conservation. N. Z. J. Ecol. 33, 1–23.
McGlone, M. S., Anderson, A. J., and Holdaway, R. N. (1994). “An ecological approach to the Polynesian settlement of New Zealand,” in The Origins of the First New Zealanders, ed D.G. Sutton (Auckland: Auckland University Press), 136–163.
McGlone, M. S., Buitenwerf, R., and Richardson, S. J. (2016). The formation of the oceanic temperate forests of New Zealand. N. Z. J. Bot. 54, 128–155. doi: 10.1080/0028825X.2016.1158196
McGlone, M. S., Duncan, R. P., and Heenan, P. B. (2001). Endemism, species selection and the origin and distribution of the vascular plant flora of New Zealand. J. Biogeogr. 28, 199–216. doi: 10.1046/j.1365-2699.2001.00525.x
McGlone, M. S., Perry, G. L. W., Houliston, G. J., and Connor, H. E. (2014). Fire, grazing and the evolution of New Zealand grasses. N. Z. J. Ecol. 38, 1–11
McLeish, M. J., Chapman, T. W., and Schwarz, M. P. (2007). Host-driven diversification of gall-inducing Acacia thrips and the aridification of Australia. BMC Biol. 5:3. doi: 10.1186/1741-7007-5-3
McWethy, D. B., Wilmshurst, J. M., Whitlock, C., Wood, J. R., and McGlone, M. S. (2014). A high-resolution chronology of rapid forest transitions following Polynesian arrival in New Zealand. PLoS ONE 9:e111328. doi: 10.1371/journal.pone.0111328
Miskelly, C. M., Crossland, A. C., Sagar, P. M., Saville, I., and Bell, E. A. (2015). Vagrant and extra-limital bird records accepted by the Birds New Zealand Records Appraisal Committee 2013-2014. Notornis 62, 85–95.
Mitchell, K. J., Llamas, B., Soubrier, J., Rawlence, N. J., Worthy, T. H., Wood, J., et al. (2014). Ancient DNA reveals elephant birds and kiwi are sister taxa and clarifies ratite bird evolution. Science 344, 898–900. doi: 10.1126/science.1251981
Mortimer, N., Campbell, H. J., Tulloch, A. J., King, P. R., Stagpoole, V. M., Wood, R. A., et al. (2017). Zealandia: earth's hidden continent. GSA Today 27, 27–35. doi: 10.1130/GSATG321A.1
Nathan, S., Anderson, H. J., Cook, R. A., Herzer, R. H., Hoskins, R. H., Raine, J. I., et al. (1986). Cretaceous and Cenozoic Sedimentary Basins of the West Coast Region, South Island, New Zealand. Wellington: Department of Scientific and Industrial Research.
Newnham, R., McGlone, M. S., Moar, N. T., Wilmshurst, J. M., and Vandergoes, M. (2013). The vegetation cover of New Zealand at the Last Glacial Maximum. Quat. Sci. Rev. 74, 202–214. doi: 10.1016/j.quascirev.2012.08.022
Perry, G. L. W., Wilmshurst, J. M., and McGlone, M. S. (2012). Reconstructing spatial vulnerability to forest loss by fire in pre-historic New Zealand. Glob. Ecol. Biogeog. 21, 1029–1041. doi: 10.1111/j.1466-8238.2011.00745.x
Perry, G. L. W., Wilmshurst, J. M., and McGlone, M. S. (2014). Ecology and the long-term history of fire in New Zealand. N. Z. J. Ecol. 38, 157–176.
Rawlence, N. J., Kardamaki, A., Easton, L. J., Tennyson, A. J. D., Scofield, R. P., and Waters, J. M. (2017a). Ancient DNA and morphometric analysis reveal extinction and replacement of New Zealand's unique black swans. Proc. B 284:20170876. doi: 10.1098/rspb.2017.0876
Rawlence, N. J., Metcalf, J., Wood, J. R., Worthy, T. H., Austin, J. A., and Cooper, A. (2012). The effect of climate and environmental change on the megafaunal moa of New Zealand in the absence of humans. Quat. Sci. Rev. 50, 141–153. doi: 10.1016/j.quascirev.2012.07.004
Rawlence, N. J., Perry, G. L. W., Smith, I. W. G., Scofield, R. P., Tennyson, A. J. D., Matisoo-Smith, E. A., et al. (2015). Radiocarbon-dating and ancient DNA reveal rapid replacement of extinct prehistoric penguins. Quat. Sci. Rev. 112, 59–65. doi: 10.1016/j.quascirev.2015.01.011
Rawlence, N. J., Till, C. E., Easton, L. J., Spencer, H. G., Schuckard, R., Melville, D. S., et al. (2017b). Speciation, range contraction and extinction in the endemic New Zealand King Shag complex. Mol. Phylogenet. Evol. 115, 197–209. doi: 10.1016/j.ympev.2017.07.011
Rawlence, N. J., and Waters, J. M. (2018). The importance of recognising and conserving biological diversity: a reply to Dussex et al. (2018). N. Z. J. Ecol. 42, 291–293. doi: 10.20417/nzjecol.42.27
Roelants, K., and Bossuyt, F. (2005). Archaeobatrachian paraphyly and Pangaean diversification of crown-group frogs. Syst. Biol. 54, 111–126. doi: 10.1080/10635150590905894
Scofield, R. P., Mitchell, K. J., Wood, J. R., De Pietri, V. L., Jarvie, S., Llamas, B., et al. (2017). The origin and phylogenetic relationships of the New Zealand ravens. Mol. Phylogenet. Evol. 106, 136–143. doi: 10.1016/j.ympev.2016.09.022
Scott, J. M., Lee, D. E., Fordyce, R. E., and Palin, J. M. (2014). A possible Late Oligocene/Early Miocene rocky shoreline on Otago Schist. N. Z. J. Geol. Geophys. 57, 185–194. doi: 10.1080/00288306.2013.814575
Seersholm, F. V., Cole, T. L., Grealy, A., Rawlence, N. J., Greig, K. L., Knapp, M., et al. (2018). Subsistence practices, past biodiversity, and anthropogenic impacts revealed by New Zealand-wide ancient DNA survey. Proc. Natl. Acad. Sci. U.S.A. 115, 7771–7776. doi: 10.1073/pnas.1803573115
Trewick, S. A. (1997). Flightlessness and phylogeny amongst endemic rails (Aves: Rallidae) of the New Zealand region. Philos. Trans. R. Soc. B 352, 429–446. doi: 10.1098/rstb.1997.0031
Wallis, G. P. (1999). Genetic status of New Zealand black stilt (Himantopus novazelandiae) and impact of hybridization. Conserv. Advis. Sci. Notes 239, 1–22.
Wallis, G. P., and Jorge, F. (2018). Going under down under? Lineage ages argue for extensive survival of the Oligocene marine transgression on Zealandia. Mol. Ecol. 27, 4368–4396. doi: 10.1111/mec.14875
Wallis, G. P., Waters, J. M., Upton, P., and Craw, D. (2016). Transverse alpine speciation driven by glaciation. Trends Ecol. Evol. 31, 916–926. doi: 10.1016/j.tree.2016.08.009
Wanner, H., Beer, J., Butikofer, J., Crowley, T. J., Cubasch, U., Fluckiger, J., et al. (2008). Mid- to Late Holocene climate change: an overview. Quat. Sci. Rev. 27, 1791–1828. doi: 10.1016/j.quascirev.2008.06.013
Waters, J. M., Fraser, C. I., and Hewitt, G. M. (2013). Founder takes all: density-dependant processes structure biodiversity. Trends Ecol. Evol. 28, 78–85. doi: 10.1016/j.tree.2012.08.024
Waters, J. M., Fraser, C. I., Maxwell, J. J., and Rawlence, N. J. (2017). Did interaction between human pressure and Little Ice Age drive biological turnover in New Zealand? J. Biogeogr. 44, 1481–1490. doi: 10.1111/jbi.12991
Waters, J. M., and Grosser, S. (2016). Managing shifting species: ancient DNA reveals conservation conundrums in a dynamic world. Bioessays 38, 1177–1184. doi: 10.1002/bies.201600044
Wilmshurst, J. M., Anderson, A. J., Higham, T. F., and Worthy, T. H. (2008). Dating the late prehistoric dispersal of Polynesians to New Zealand using the commensal Pacific rat. Proc. Natl. Acad. Sci. U.S.A. 105, 7676–7680. doi: 10.1073/pnas.0801507105
Wilmshurst, J. M., Hunt, T. L., Lipo, C. P., and Anderson, A. J. (2011). High-precision radiocarbon dating shows recent and rapid initial human colonization of East Polynesia. Proc. Natl. Acad. Sci. U.S.A. 108, 1815–1820. doi: 10.1073/pnas.1015876108
Wood, J., Wilmshurst, J., Newnham, R., and McGlone, M. (2017). “Evolution and ecological change during the New Zealand Quaternary,” in Landscape and Quaternary Environmental Change in New Zealand, ed. J. Shulmeister (Amsterdam: Atlantis Press), 235–291.
Worthy, T. H. (1997). A mid-Pleistocene rail from New Zealand. Alcheringa 21, 71–78. doi: 10.1080/03115519708619186
Worthy, T. H., de Pietri, V. L., and Scofield, R. P. (2017). Recent advances in avian palaeobiology in New Zealand with implications for understanding New Zealand's geological, climatic and evolutionary histories. N. Z. J. Zool. 44, 177–211. doi: 10.1080/03014223.2017.1307235
Keywords: Australia, avifauna, birds, glacial, New Zealand, Pleistocene, Pliocene, speciation
Citation: Rawlence NJ, Scofield RP, McGlone MS and Knapp M (2019) History Repeats: Large Scale Synchronous Biological Turnover in Avifauna From the Plio-Pleistocene and Late Holocene of New Zealand. Front. Ecol. Evol. 7:158. doi: 10.3389/fevo.2019.00158
Received: 07 December 2018; Accepted: 23 April 2019;
Published: 14 May 2019.
Edited by:
Christopher Carcaillet, PSL Research University, Ecole Pratique des Hautes Etudes (EPHE), FranceReviewed by:
Bastien Llamas, University of Adelaide, AustraliaLeo Joseph, Ecosystem Sciences (CSIRO), Australia
Copyright © 2019 Rawlence, Scofield, McGlone and Knapp. This is an open-access article distributed under the terms of the Creative Commons Attribution License (CC BY). The use, distribution or reproduction in other forums is permitted, provided the original author(s) and the copyright owner(s) are credited and that the original publication in this journal is cited, in accordance with accepted academic practice. No use, distribution or reproduction is permitted which does not comply with these terms.
*Correspondence: Michael Knapp, bWljaGFlbC5rbmFwcEBvdGFnby5hYy5ueg==