- 1Faculty of Environmental Sciences and Natural Resource Management, Norwegian University of Life Sciences, Ås, Norway
- 2Norwegian Institute for Nature Research, Trondheim, Norway
- 3Department of Forestry and Wildlife Management, Faculty of Applied Ecology and Agricultural Sciences, Inland Norway University of Applied Sciences, Koppang, Norway
- 4Department of Surgery, University of Minnesota, Minneapolis, MN, United States
- 5Department of Wildlife, Fish and Environmental Studies, Swedish University of Agricultural Sciences, Umeå, Sweden
- 6Faculty of Chemistry, Biotechnology and Food Science, Norwegian University of Life Sciences, Ås, Norway
Innovations in biologging have offered new possibilities to better understand animals in their natural environment. Biologgers can be used by researchers to measure the impact of human disturbances on wildlife and guide conservation decisions. In this study, the behavioral and physiological responses of brown bears (Ursus arctos) to hunts using dogs (Canis lupus familiaris) and human encounters were assessed to better understand the impact of human outdoor activities on brown bears. In Scandinavia, brown bear hunting and the use of dogs during hunts is increasing in popularity. Nonetheless, not every hunt leads to a killed bear. This means that for each bear that is shot, multiple bears may be chased but not killed. In addition, bears can also be disturbed when encountering non-hunting humans. Heart rates, body temperatures, GPS coordinates and dual-axis activity data were collected from 52 simulated hunts (a simulated hunt using dogs with the bear allowed to flee at the end) and 70 human encounters (humans intentionally approaching the bear) that were carried out on 28 free-ranging female brown bears in two study areas in Sweden. The results showed that: (1) simulated hunts had a greater impact and induced a greater energy cost than human encounters; (2) the amount of time bears rested the day after the simulated hunts increased linearly with the duration of the simulated hunts, implying a lasting behavioral impact relative to the intensity of the disturbance. Although not tested in this study, brown bears that are repeatedly disturbed by dog hunts and human encounters may be unable to compensate the disturbances' energy cost, and their fitness may, therefore, be altered. If it is the case, this effect should be accounted for by managers.
Introduction
Innovations in biologging have increasingly been used in the past few decades to better understand animals in their natural environment (Wilmers et al., 2015). The use of biologgers can also allow researchers to measure human disturbances and guide conservation decisions (Wilson et al., 2015). Hunting practices raise ethical questions and have spurred research on the physiology and behavior of pursued animals (for example: foxes (Vulpes vulpes) (Kreeger et al., 1989), pumas (Puma concolor) (Bryce et al., 2017) or red deer (Cervus elaphus) (Jarnemo and Wikenros, 2014). Several variables may be used to measure how human outdoor activities disturb wild animals and to determine if the disturbances may incur extra energy costs. Longer traveled distances (Rode et al., 2007), higher heart rates (Kreeger et al., 1989; Ditmer et al., 2015) or higher body temperature (Evans et al., 2016) have, for example, been considered as indicators of disturbances that lead to extra energy costs. In addition, animals may run to flee, with the higher speeds demanding more energy (Pagano et al., 2018). They may also need to recover and rest after a disturbance, preventing their compensation of the lost energy.
Brown bears (Ursus actos) have been a game species in Sweden since 1943 (Swenson et al., 2017) with a hunting season starting on August 21st and ending when the quotas set by the county board are filled, or at the latest on October 15th. Family groups (adult females with dependent cubs) are protected but there are no age or sex specifications applied to the hunting quotas (Bischof et al., 2008). In Sweden, legal hunting by humans represents the primary cause of mortality for adult brown bears (Bischof et al., 2017), with the most common hunting method being based on the use of trained hunting dogs (Canis lupus familiaris) (Bischof et al., 2008). Dogs are released to pursue the bears with the hunters following and attempting to shoot the bear (hereafter dog hunts). Bear hunting is increasing in popularity with the number of hunters specialized in bear hunting rising (Swenson et al., 2017). There are no statistics known for Sweden, but when hunting black bears (Ursus americanus) in a similar way in Virginia (USA), the recorded success is generally low [20%, Vaughan and Inman (2002)]. This means that for each bear that is shot, multiple bears may be chased but not killed.
Hunting is not the only human outdoor activity that affects bears in Sweden. Bears flee when encountered by humans (Moen et al., 2012) and change their movement patterns for at least 2 days following the event (Ordiz et al., 2013b). In areas with higher road densities, bears adopt movement patterns that are more nocturnal and less diurnal, to avoid human activity (Ordiz et al., 2014). Bears avoid denning near roads (Elfström et al., 2008) and select more concealed denning sites near humans (Sahlén et al., 2011). Brown bears have a general anti-predator behavior toward humans. A behavior that is not only affected by hunting activities, but also by the year-round presence of humans. It is therefore important to understand the impacts of both dog hunts and human encounters on brown bears.
To better understand how human outdoor activities influence brown bears in Scandinavia, simulated hunts using dogs with the bear allowed to flee at the end (hereafter simulated hunts) and human encounters (humans intentionally approaching the bear) were conducted on GPS-collared female brown bears. For each experiment, five variables were measured: (1) the distance traveled by the bears, (2) their speed, (3) their heart rate, (4) their body temperature, and (5) their resting behavior. This was done within three different time periods: (1) during a control period (3 days prior to an experiment), (2) during the day of the experiment and (3) during the 2 days following the experiment. The following hypotheses were tested by comparing the five variables at the different time periods: [H1] Dog hunts and human encounters are a source of physiological and behavioral disturbance for brown bears. [H2] The physiological and behavioral impacts of dog hunts on brown bears are greater than the impact of human encounters. [H3] Dog hunts that last longer in time have greater physiological and behavioral impacts on brown bears. [H4] Dog hunts and human encounters have lasting physiological and behavioral effects on brown bears.
Materials and Methods
Study Area
In Sweden, brown bears are distributed into three main populations (Norman, 2016). This study was carried out from 2014 to 2016 in two different areas covering the southernmost and northernmost bear subpopulations (61.50°N; 15.06°E & 66.76°N; 21.02°E). In both areas, the landscape is hilly and mostly covered by managed productive forest, mainly composed of Scots pine (Pinus sylvestris), Norway spruce (Picea abies) and birch (Betula spp.).
Data Collection
Human encounters and simulated hunts were conducted on 28 free-ranging female brown bears. The bears were equipped with a VHF transmitter implant (M1255B, Advanced Telemetry Systems, Isanti, USA); a GPS-Plus collar with GSM modems or Iridium modems with an included VHF transmitter and a dual-axis motion sensor (Vectronic Aerospace GmBh, Berlin, Germany); cardiac biologgers (Reveal XT, Medtronic, Minneapolis, USA) implemented with modified software (BearWare, Medtronic, Minneapolis, USA); and temperature biologgers (DST Centi-T, Star-Oddi, Gardabaer, Iceland). The biologgers were surgically implanted in the bears with the cardiac biologgers subcutaneous to the left of the sternum and the temperature biologgers in the abdomen of the bear (Arnemo and Evans, 2017). The cardiac biologgers continuously recorded the bears' inter-beat intervals (R-R, in milli seconds), based on electrocardiogram (ECG) measurements. Every 2 min the mean R-R interval was converted into a heart rate in beats per minute (bpm). The body temperatures of the bears were measured every 4 min with an accuracy of ± 0.01°C. The activity of the bear was measured as the true acceleration in two orthogonal directions at a frequency of six to eight times per second. The average of the activity values over 5 min for each orthogonal direction was then recorded in the GPS collar with its associated date and time (Friebe et al., 2014).
GPS coordinates of the bears were recorded by default every 30 min or every hour. During the human encounters and the simulated hunts, a GPS location was recorded every minute for 3 or 4 h. During some of the simulated hunts, the dogs were equipped with Ultra High Frequency (UHF) transmitters (Vectronic Aerospace GmbH, Berlin, Germany) that emitted a signal every second, triggering the recording of GPS coordinates every 70 s in the bears' GPS collars at ≤500 m. The GPS collars on the brown bears scanned for UHF signals for 1.5 s every 8 s, and the recording ended automatically 60 min after the last detection of a dog collar. Humans (hereafter observers) were equipped with hand-held Garmin GPSMAP 60CSx or Astro 320 and the dogs were equipped with T 5 or DC40 Dog Devices, with all types of equipment being set to record a GPS coordinate every second (Garmin Ltd., Olathe, USA).
For more details on how the bears were captured and immobilized, please refer to Arnemo and Evans (2017). All captures were approved by the Swedish Ethical Committee on Animal Research (application numbers C7/12 and C18/15) and the Swedish Environmental Protection Agency (application numbers NV-00741-18 and NV-01758-14).
Experimental Design
Following the same methods presented by Moen et al. (2012), the human encounters were started between 8:30 and 14:30 local time (GMT+2). The observers walked toward the bear, intending to pass the bear at an approximate distance of 50 m, whilst talking to each other or to themselves if alone, simulating hikers. When the bear had been passed or when the bear ran away, they returned to the car and made sure not to encounter the bear a second time. The 70 human encounters were carried out in 2014 (1st June−7th August; n = 17), in 2015 (8th June−28th July; n = 24) and in 2016 (2nd June−20th August; n = 29). The minimum distance recorded between the observers and the bear was on average 55 ± 27 m (median 51 m, minimum 18 m, maximum 137 m; n = 70). In total, 25 female bears were used, with five subadults, 16 adults and four that were used when they were both subadults and adults.
Simulated hunts were started between 8:00 and 17:00. The observers with hunting dogs kept on a leash walked toward the bear until a dog showed interest in the scent of the bear, either in the wind or from its tracks, before the dogs were released and allowed to pursue the bear (Figure 1). The simulated hunt was stopped when the dogs came back to the observers or by the observers calling in or intercepting the path of the dogs. The simulated hunts were intended to last about 2 h but ended both earlier and later due to practicalities. During the same simulated hunt, one to six different dogs were used. However, only a maximum of two dogs were let loose simultaneously to hunt the bear. Bears were considered to be disturbed by both types of experiments if the minimum distance recorded between the bear and the observers or dogs at any time was < 200 m.
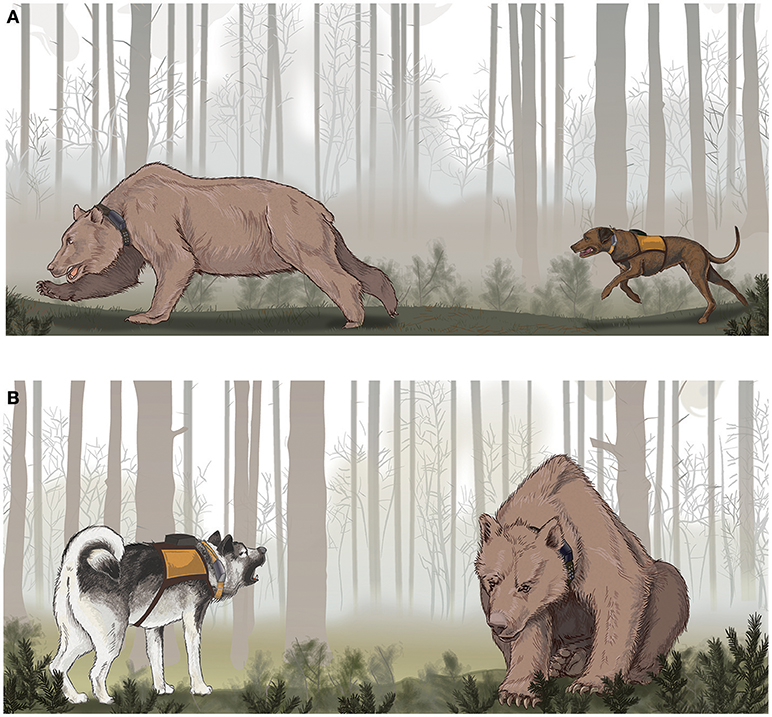
Figure 1. While being hunted, a brown bear can run in order to escape hunting dog(s) and sometimes end up lying on the ground with the dog(s) barking and running around it (baying). When the dog(s) is/are baying the bear, the hunter is typically sneaking in and shooting the bear. (A) Plott hound pursuing a bear during a simulated hunt. The dog is equipped with a collar (DC40 Dog Device) used to collect GPS coordinates, as well as a dog harness equipped with a UHF transmitter that triggers the recording of coordinates every 70 s in the GPS-Plus collar fitted on the bear. (B) Elkhound baying a bear during a simulated hunt. The same equipment as in (A) is illustrated. Scientific Illustration by Juliana D. Spahr, SciVisuals.com (reproduced with permission).
The 52 simulated hunts were carried out in 2014 (9th August−15th August; n = 4), in 2015 (11th June−2nd October; n = 19) and in 2016 (18th June−7th October; n = 29). The minimum distance recorded between the observers and / or the dogs and the bear was on average 20 ± 34 m (median 5 m, minimum 0 m, maximum 168 m; n = 52). The duration of a simulated hunt was defined as the time between when the observers and dogs started heading from the car toward the bear until when they were back at the car. Simulated hunts lasted on average 229 ± 104 min (median 198 min, minimum 67 min, maximum 508 min; n = 52). In total, 17 female bears were used, with two subadults, 14 adults and one that was used when it was both a subadult and an adult.
Some bears were used in multiple human encounters and / or simulated hunts during the same year. No research activities that could have affected the bears were carried out for at least 5 days before and 2 days after the experiments.
Data Processing and Statistical Analyses
All the data analyses and data processing were carried out using the statistical programming language and environment, R 3.4.2 (R Core Team, 2017). All data (GPS, activity, heart rate and body temperature) was stored in the WRAM database (Wireless Remote Animal Monitoring, Dettki et al., 2014).
Response Variables
The distance traveled by the bear was measured as the variable TRAVEL, which is the sum of all hourly displacements for each day. In this way, days with GPS coordinates taken with a different frequency were directly comparable. The distances (shortest distance between two points on the WGS84 ellipsoid) between the hourly positions were measured using the distGeo function from the geosphere package, version 1.5-7 (Hijmans, 2017). The maximum speed of the bear was measured as the variable MAXSPEED, which is the highest speed recorded of the day based on all hourly displacements within the 24 h. The heart rate of the bear was measured as the variable HEARTRATE30, which is the highest value measured by a rolling mean ran over all the measured heart rates of the day, with a window of 30 min and a constant forward shift of 2 min. When processing the heart rate data, a constant mismatch between the heart rate and the corresponding time was observed for some bears. The mismatch was corrected through using the correlation between the activity of the bear and its heart rate. Heart rate data was not used in the analysis if the activity data was not available or if the method led to a suggested time shift that was not consistent throughout the data. The body temperature of the bear was measured as the variable TbAREA, which is the area under the curve of the measured body temperature values and above the median body temperature of the bear over a 24-h period. The median body temperature was based on data from 1st June to 30th September for each bear-year. Days when the bears were involved in research activities (human encounters, simulated hunts or captures) were not included in this data set. The area was calculated using a trapezoidal approximation (all points are connected by a direct line forming multiple trapezoids) using the AUC function from the DescTools package, version 0.99.23 (Signorell, 2017). The resting behavior of the bear was measured as the variable REST, which is the amount of time the bear was resting in minutes during a day. The two values for each orthogonal, measured by the dual-axis motion sensor, were summed resulting in a variable ranging from 0 to 510. A value lower than 23 was considered resting behavior (Gervasi et al., 2006).
Explanatory Variables
Human encounters and simulated hunts were considered as two different treatments in a binary variable, hereafter named TYPE. The variable PERIOD consisted of four unique levels. The 1st level represents the control period defined as the mean values recorded during the 3 days prior to the human encounter day or simulated hunt day. The 2nd level represents the day of the experiment. The 3rd and 4th levels represent the following and second day after the experiment, respectively. The age of the bears was considered in the binary variable AGE with subadult bears <4 years old. The duration of the simulated hunts was also used as an explanatory variable.
Model Construction
Linear mixed effect models (LME) were fitted using TRAVEL, MAXSPEED, HEARTRATE30, TbAREA and REST as response variables, and TYPE, PERIOD, AGE and the interactions as explanatory variables. The experiments' ID nested in the bears' ID were added as random factors. The normality of the residuals was improved by a square root transformation of the response in the TRAVEL, MAXSPEED, HEARTRATE30 and the TbAREA models. The models were created using the lmer function from the lme4 package, version 1.1-14 (Bates et al., 2014) and fitted using the restricted maximum likelihood (REML) method, as the models were composed of small sample sizes. The final model was obtained by following the backward selection method. The significance of the variables and the interaction were computed using the Anova function from the car package, version 2.1-6 (Fox and Weisberg, 2011), with the type-III method. A pairwise analysis of the estimated marginal means (EMMs) was then performed to interpret the final models using the emmeans package, version 1.1. (Lenth, 2018). This method was used as the models had an unbalanced number of human encounters and simulated hunts (Table 1). The EMMs were based on a 0.95 confidence level with the Tukey correction method. Some of the 70 human encounters and 52 simulated hunts did not have data for all the response variables. For this reason, n varied between the different LME models (Table 1).
When only considering the simulated hunts data, the variables TRAVEL, MAXSPEED, HEARTRATE30, TbAREA, and REST were used as response variables in LME models, with the duration of the simulated hunts, the variable AGE and the interaction as explanatory variables. In these models, the variables TRAVEL, MAXSPEED and TbAREA were square root transformed to improve the normality of the residuals. The models were created following the same method as the method presented for the previous LME models but were created using the lme function from the nlme package, version 3.1-131 (Pinheiro et al., 2017). The model included the ID of the bears as a random factor, as some simulated hunts were carried out on the same bears (Table 1).
In the models HEARTRATE30 and REST with PERIOD, TYPE, AGE and the interactions as explanatory variables, the variable AGE and the corresponding interactions were not kept in the models after using the backward selection method. However, the interaction between PERIOD and TYPE was significant (all P < 0.0001). Both the PERIOD and TYPE variables were thus used in the pairwise analysis of the EMMs. In the models TRAVEL, MAXSPEED, and TbAREA, the variables AGE, PERIOD and TYPE were kept in the models after using the backward selection method. All three explanatory variables were therefore used in the pairwise analysis of the EMMs.
Results
The distances traveled by both adult and subadult bears were longer during the day of the simulated hunts than during the corresponding control periods (post hoc test: estimated difference: both adult and subadult: 755 ± 15 m, P < 0.0001, both 10% longer, range measured difference: Adult: −3 to 22 km, Subadult: −1 to 12 km) and longer than during the day of the human encounters (post hoc test: estimated difference both adult and subadult: = 527 ± 22 m, P < 0.0001, Adult: 3 %, Subadult: 4% longer). Adult and subadult bears did not travel longer distances on the day of the human encounters than during the corresponding control periods (both P = 0.13).
Both adult and subadult bears ran faster during the day of the simulated hunts than during the corresponding control periods (post hoc test: estimated difference: Adult: 679 ± 61 m h−1, P < 0.0001, 31% faster, range measured difference: −280 to 6360 m h−1, Subadult: 455 ± 94 m h−1, P = 0.0002, 17% faster, range measured difference:−680 to 3580 m h−1) and ran faster than during the day of the human encounters (post hoc test: estimated difference: both: 450 ± 62 m h−1, P < 0.0001, Adult 10 % faster, Subadult: 16 % faster). Adult bears also ran faster the day of the human encounters than during the corresponding control periods (post hoc test: estimated difference: Adult: 216 ± 53 m h−1, P = 0.005, 3% faster, range measured difference: −1470 −8850 m h−1). Subadults, however, did not run faster on the day of the human encounters than during the corresponding control periods (P > 0.90).
The maximum heart rates were higher during the day of the simulated hunts than during the corresponding control periods (post hoc test: estimated difference = 7 ± 0.06 bpm, P < 0.0001, 7% higher, range measured difference: 4–106 bpm) and higher than during the day of the human encounters (post hoc test: estimated difference = 3 ± 0.12 bpm, P < 0.0001, 3% higher). Bears did not have a different maximum heart rate the day of the human encounters than during the corresponding control periods (P = 0.18, maximum measured difference: −50–106 bpm).
The body temperature areas of the adult bears were greater during the day of the simulated hunts than during the corresponding control periods (post hoc test: estimated difference = 1553 ± 59 TbAREA, P < 0.0001, 12% greater) but not for subadult bears (P = 0.15). The body temperature areas of the bears were not greater during the day of the simulated hunts than during the day of the human encounters (Adult: P = 0.64, Subadult: P = 0.57). The body temperature areas of both adult and subadult bears were not different on the day of the human encounters compared to during the corresponding control periods (both P > 0.90).
The day after the simulated hunts, bears rested more than during the corresponding control periods (post hoc test: estimated difference = 68 ± 19 min, P = 0.008, 1% more, range measured difference: −4 h 15 min−5 h 15 min) but not more than during the day after the human encounters (P = 0.62). The day after the human encounters, bears did not rest more than during the corresponding control periods (P > 0.90). The amount of time bears rested the day after the simulated hunts increased linearly with the duration of the simulated hunts (Figure 2). Bears did not rest less during the day of the human encounters or the simulated hunts than during the corresponding control periods (both P > 0.90). Bears did not rest less during the day of the simulated hunts than the day of the human encounters (P = 0.18).
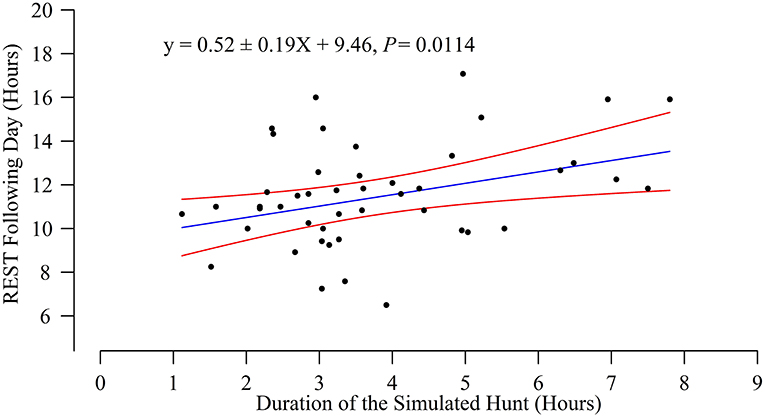
Figure 2. The day after the simulated hunts, female bears rested more if the simulated hunts were longer (P = 0.0114). The 46 simulated hunts are represented by the black dots with the regression line, as well as the lower and upper 95% confidence interval. The model REST ~ Duration of the simulated hunt*AGE was fitted with the Bear ID as a random factor. The variable AGE was not kept after using the backward selection method (Interaction: P = 0.34, AGE: P = 0.29).
There was no difference between the control periods of the human encounters and the control periods of the simulated hunts (adults or subadults) for the distance traveled, the maximum speed, the maximum heart rate, the body temperature area or how long the bear rested (Table 2, all P > 0.13). There was no difference between the first and second days following the human encounters or the simulated hunts, compared to the corresponding control periods, for the distance traveled, the maximum speed, the maximum heart rate or the body temperature areas of the bears (all P > 0.09). The duration of the simulated hunts had no effect on the distance traveled, maximum speed, maximum heart rate, body temperature area or how long the bear rested the day of the simulated hunt (all P > 0.15).
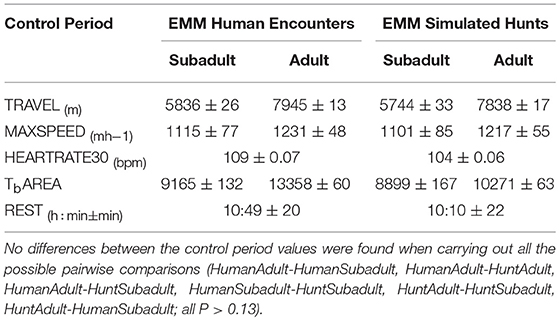
Table 2. Estimated marginal means (EMM) for the control periods of the human encounters and the control periods of the simulated hunts.
Discussion
During human encounters, adult bears ran faster than during the corresponding control periods, but did not have higher heart rates, higher body temperatures or travel longer distances. There were no significant differences between the day of the human encounters and the corresponding control periods in any of these variables for subadults. These results provide support for the hypothesis that human encounters are a behavioral disturbance for brown bears [H1] but do not support the hypothesis that human encounters are a physiological disturbance for brown bears.
Contrary to human encounters, simulated hunts led to a clear disturbance with longer traveled distances, higher speeds and higher heart rates than during the corresponding control periods or the human encounters. Adult female bears also had greater body temperatures compared with the control periods. These results support the hypothesis that dog hunts represent both a behavioral and physiological disturbance [H1]. The results also support the hypothesis that dog hunts have a greater impact on brown bears than human encounters [H2].
During simulated hunts, bears were pursued by dogs and may have been forced to flee in a more dramatic way than when moving away from encountered humans, which is most likely the reason why simulated hunts had a greater impact. Simulated hunts were also characterized by smaller minimum distances as dogs were able to come closer to the bears than observers were during the human encounters. Because running faster can be associated with higher energy costs (Pagano et al., 2018), human encounters and dog hunts can be considered a direct energy cost for adult female brown bears, with dog hunts having the highest impact. Simulated hunts also led to longer traveled distances, higher heart rates and, for adult females, greater body temperature areas, giving further support to the idea that dog hunts represent an important energy cost. The fact that bears use more energy while running than other quadrupedal mammals (Pagano et al., 2018) suggests that human encounters and dog hunts may represent relatively large energy costs in bears.
If an adult female bear is frequently disturbed by human encounters and dog hunts, its body condition may be affected by the energy cost of these disturbances. Females do not always reach their energetic needs, e.g., poor berry seasons affect the reproductive success of lightweight female bears in Sweden (Hertel et al., 2018). Adult females give birth during the denning period and depend on their fat reserves for the gestation and lactation of their cubs (Robbins et al., 2012; Lopez-Alfaro et al., 2013). Even if observed mating, captive adult female brown bears having a body fat content lower than 20% do not give birth (Robbins et al., 2012). Lopez-Alfaro et al. (2013), using an energy consumption model, estimated that with a body fat content below 19%, a female would not be able to reproduce during a hibernation period that lasts over 120 days. In Sweden, adult females spend on average 181 days in winter dens (Friebe et al., 2001). Energy costs due to human encounters and dog hunts may thus affect the body condition of adult female bears and ultimately affect their fitness by altering the reproductive success.
Higgins (1997) compared the body condition of 13 adult female black bears from non-hunted populations with 20 adult female black bears from a hunted population and found an indication that black bears from the hunted population may be lighter and in worse physical condition (P = 0.09). However, Massopust and Anderson (1984) carried out eight dog hunts on five black bears with a maximum of two dog hunts per individual and observed no injuries or abnormal weights on the bears when captured at their den. It is possible that more than two dog hunts are needed to affect the weight of the bears. Tourism (bear viewing), experimentally simulated for one summer in an undisturbed bear area in Alaska, did not lead to losses in weights, nor any changes in body condition (Rode et al., 2007). However, the studied bear population could feed on salmon (Oncorhynchus kisutch and Oncorhynchus nerk), a more nutrient-rich alimentation than the berries eaten by the Scandinavian brown bears (Welch et al., 1997), giving the bears a better opportunity to maintain their body condition.
Human encounters and dog hunts do not seem to have a profound lasting impact on bears, because there was no difference in distances traveled, maximum speeds, maximum heart rates, and body temperature areas between the control periods and the 2 days after the experiments. This may seem surprising as brown bears change their movement patterns for at least 2 days after human encounters (Ordiz et al., 2013b). Our study was based on hourly displacements and may underestimate the real movement pattern of the bears and thus the behavioral disturbance. The effects found in our study may also have been underestimated, because the bears could have been disturbed during the control periods, making the differences smaller between the experimental days and the control periods. All the human encounters in the project were conducted in the summer and many during the berry picking season, making it likely that the bears also encountered other humans during the control periods. Simulated hunts were not carried out during the bear hunting season, but a few simulated hunts were conducted during the moose hunting season. Dogs used for hunting moose (Alces alces) may also chase bears (Bischof et al., 2008).
Bears rested more the day after a simulated hunt compared with the control period and if the simulated hunt was longer. These results support the hypothesis that longer dog hunts may have a greater impact [H3], and that dog hunts may have a lasting behavioral effect on brown bears [H4]. The longer rest after simulated hunts suggests that bears may be fatigued by dog hunts. Longer dog hunts on deer (Cervus elaphus) increased the disturbance impact and were associated with a higher concentration in enzymes related to muscle breakdown (Bateson and Bradshaw, 1997). Deer that are shot after a long hunt present physiological signs of extreme exhaustion (Bateson and Bradshaw, 1997). Bears may suffer from similar physiological impacts, and may have to increase their rest to recover from it. Bateson and Bradshaw (1997) concluded that red deer were not well-adapted to fleeing dogs. Bears may be even less adapted to dog hunts than red deer as they are energetically less efficient when running compared to other quadrupedal mammals (Pagano et al., 2018). After prolonged exercise, extra energy consumption can be measured during the recovery time (Børsheim and Bahr, 2003). This may also represent an extra post-disturbance energy cost.
Brown bear hunting in Sweden has known impacts beyond the initial offtake of direct mortality, such as altering life history traits (Bischof et al., 2017; Frank et al., 2017) or inducing an increase in sexually-selected infanticide through an increased male turnover (Swenson et al., 1997). Furthermore, adult bears that would naturally face low mortality rates (Bischof and Zedrosser, 2009; Bischof et al., 2009, 2017) adopt antipredator behaviors in response to human hunting pressure (Ordiz et al., 2013a). For example, when the hunting season starts, bears decrease their foraging activity during the time of the day with the highest risk of being shot, forcing them to forage less efficiently and in areas with poorer berry quality (Hertel et al., 2016). This antipredator behavior, combined with the direct energy costs of human encounters and dog hunts may lead to a lower fitness if it prevents adult females from reaching the required body condition threshold for successful breeding.
Conclusion
Dog hunts represent a greater physiological and behavioral source of disturbance for female brown bears than human encounters. Adult female bears were behaviorally disturbed by human encounters but did not travel longer distances or have different heart rates and body temperatures. Simulated hunts had lasting behavioral effects on bears by inducing longer resting periods the day following the actual experiment. Longer resting periods found after longer simulated hunts suggested that the impact of dog hunts increases with their duration. By representing an energy cost, human encounters and dog hunts could lower the fitness of adult female bears if experienced frequently. Further research is needed to assess if bears that are repeatedly disturbed by dog hunts and human encounters are unable to compensate the disturbances' energy cost and if this affects their fitness. Nonetheless, if it is the case, this effect should be accounted for by managers. Human encounters have a lower impact than dog hunts on the bears but are not restricted in time like dog hunts. Thus, they may have a lower impact per se but may still have an important impact due to their higher frequency. Distance traveled, speeds, hearts rates, body temperature and resting behavior are universal variables that can be used on other animals to assess the impact of human activities. Looking at behavioral and physiological variables within the same study helps to have a better understanding of the disturbance.
Ethics Statement
All captures were approved by the Swedish Ethical Committee on Animal Research (application numbers C7/12 and C18/15) and the Swedish Environmental Protection Agency (application numbers NV-00741-18 and NV-01758-14).
Author Contributions
LG, JA, and O-GS conceived the ideas and designed the methodology. AE, JA, and O-GS instrumented the bears and recovered devices in the field with input from TL. Pre-database, data-management and submission to WRAM (wireless remote animal monitoring, SLU) was conducted by BF. All authors contributed to the data analyses. LG led the writing of the manuscript. All authors reviewed the drafts and gave final approval for publication.
Funding
This study was funded by the Norwegian Environment Agency and conducted within the Scandinavian Brown Bear Research Project (SBBRP). The SBBRP has primarily been funded by the Swedish Environmental Protection Agency, the Austrian Science Fund, and the Swedish Association for Hunting and Wildlife Management. This is scientific paper 274 from the SBBRP. None of the funders had any role in the conduct of the research and/or preparation of the article.
Conflict of Interest Statement
TL is an employee of Medtronic, Inc. and all cardiac biologgers were donated by Medtronic.
The remaining authors declare that the research was conducted in the absence of any commercial or financial relationships that could be construed as a potential conflict of interest.
Acknowledgments
Veterinarians Martine Angel, Nuria Fandos Esteruelas, and AnneRandi Græsli contributed to device deployment and retrieval. Andrea Friebe, David Ahlqvist, Rasmus Boström and Sven Brunberg deserve a special mention for their help in the field as well as all the students and volunteers without whom the project would not have been possible.
References
Arnemo, J. M., and Evans, A. (2017). Biomedical Protocols for Free-Ranging Brown Bears, Wolves, Wolverines and Lynx. Inland Norway University of Applied Sciences, Campus Evenstad.
Bates, D., Mächler, M., Bolker, B., and Walker, S. (2014). Fitting linear mixed-effects models using lme4. J. Stat Soft. 67, 1–48. doi: 10.18637/jss.v067.i01
Bateson, P., and Bradshaw, E. L. (1997). “Physiological effects of hunting red deer (Cervus elaphus),” in Proceedings of the Royal Society of London B: Biological Sciences. (Great Britain: The Royal Society), 1707–1714.
Bischof, R., Bonenfant, C., Rivrud, I. M., Zedrosser, A., Friebe, A., Coulson, T., et al. (2017). Regulated hunting re-shapes the life history of brown bears. Nat. Ecol. Evol. 2, 116–123. doi: 10.1038/s41559-017-0400-7
Bischof, R., Fujita, R., Zedrosser, A., Söderberg, A., and Swenson, J. E. (2008). Hunting patterns, ban on baiting, and harvest demographics of brown bears in Sweden. J. Wildl. Manag. 72, 79–88. doi: 10.2193/2007-149
Bischof, R., Swenson, J. E., Yoccoz, N. G., Mysterud, A., and Gimenez, O. (2009). The magnitude and selectivity of natural and multiple anthropogenic mortality causes in hunted brown bears. J. Anim. Ecol. 78, 656–665. doi: 10.1111/j.1365-2656.2009.01524.x
Bischof, R., and Zedrosser, A. (2009). The educated prey: consequences for exploitation and control. Behav. Ecol. 20, 1228–1235. doi: 10.1093/beheco/arp124
Børsheim, E., and Bahr, R. (2003). Effect of exercise intensity, duration and mode on post-exercise oxygen consumption. Sports Med. 33, 1037–1060. doi: 10.2165/00007256-200333140-00002
Bryce, C. M., Wilmers, C. C., and Williams, T. M. (2017). Energetics and evasion dynamics of large predators and prey: pumas vs. hounds. PeerJ. 5:e3701. doi: 10.7717/peerj.3701
Dettki, H., Brode, M., Giles, T., and Hallgren, J. (2014). “Wireless remote animal monitoring (WRAM)-A new international database e-infrastructure for management and sharing of telemetry sensor data from fish and wildlife,” in 7th International Congress on Environmental Modelling and Software. (San Diego, CA), 122–128.
Ditmer, M. A., Vincent, J. B., Werden, L. K., Tanner, J. C., Laske, T. G., Iaizzo, P. A., et al. (2015). Bears show a physiological but limited behavioral response to unmanned aerial vehicles. Curr. Biol. 25, 2278–2283. doi: 10.1016/j.cub.2015.07.024
Elfström, M., Swenson, J. E., and Ball, J. P. (2008). Selection of denning habitats by Scandinavian brown bears Ursus arctos. Wildl. Biol. 14, 176–187. doi: 10.2981/0909-6396(2008)14<176:SODHBS>2.0.CO;2
Evans, A. L., Singh, N. J., Fuchs, B., Blanc, S., Friebe, A., Laske, T. G., et al. (2016). Physiological reactions to capture in hibernating brown bears. Conserv. Physiol. 4, 1–11. doi: 10.1093/conphys/cow061
Frank, S. C., Ordiz, A., Gosselin, J., Hertel, A., Kindberg, J., Leclerc, M., et al. (2017). Indirect effects of bear hunting: a review from Scandinavia. Ursus 28, 150–164. doi: 10.2192/URSU-D-16-00028.1
Friebe, A., Evans, A. L., Arnemo, J. M., Blanc, S., Brunberg, S., Fleissner, G., et al. (2014). Factors affecting date of implantation, parturition, and den entry estimated from activity and body temperature in free-ranging brown bears. PLoS ONE 9:e101410. doi: 10.1371/journal.pone.0101410
Friebe, A., Swenson, J. E., and Sandegren, F. (2001). Denning chronology of female brown bears in central Sweden. Ursus 12, 37–45.
Gervasi, V., Brunberg, S., and Swenson, J. E. (2006). An individual-based method to measure animal activity levels: a test on brown bears. Wildl. Soc. Bull. 34, 1314–1319. doi: 10.2193/0091-7648(2006)34<1314:AIMTMA>2.0.CO;2
Hertel, A. G., Bischof, R., Langval, O., Mysterud, A., Kindberg, J., Swenson, J. E., et al. (2018). Berry production drives bottom–up effects on body mass and reproductive success in an omnivore. Oikos 127, 197–207. doi: 10.1111/oik.04515
Hertel, A. G., Zedrosser, A., Mysterud, A., Støen, O.-G., Steyaert, S. M., and Swenson, J. E. (2016). Temporal effects of hunting on foraging behavior of an apex predator: do bears forego foraging when risk is high? Oecologia 182, 1019–1029. doi: 10.1007/s00442-016-3729-8
Higgins, K. (1997). Hunting Dynamics, Condition Estimates, and Movements of Black Bears Hunted With Hounds in Virginia. Master thesis, Virginia Polytechnic Institute and State University.
Hijmans, R. J. (2017). Geosphere: Spherical Trigonometry. R package Version 1.5−7. Available online at: https://CRAN.R-project.org/package=geosphere
Jarnemo, A., and Wikenros, C. (2014). Movement pattern of red deer during drive hunts in Sweden. Eur. J. Wildl. Res. 60, 77–84. doi: 10.1007/s10344-013-0753-4
Kreeger, T. J., Monson, D., Kuechle, V. B., Seal, U. S., and Tester, J. R. (1989). Monitoring heart rate and body temperature in red foxes (Vulpes vulpes). Can. J. Zool. 67, 2455–2458.
Lenth, R. (2018). Emmeans: Estimated Marginal Means, aka Least-Squares Means. R Package Version 1.1. Available online at: https://CRAN.R-project.org/package=emmeans
Lopez-Alfaro, C., Robbins, C. T., Zedrosser, A., and Nielsen, S. E. (2013). Energetics of hibernation and reproductive trade-offs in brown bears. Ecol. Model. 270, 1–10. doi: 10.1016/j.ecolmode1.2013.09.002
Massopust, J. L., and Anderson, R. K. (1984). “The response of black bears to being chased by hunting dogs,” in Proceeding Eastern Workshop Black Bear Management and Research, 59–65.
Moen, G. K., Stoen, O. G., Sahlen, V., and Swenson, J. E. (2012). Behaviour of solitary adult Scandinavian brown bears (Ursus arctos) when approached by humans on foot. PLoS ONE 7:e31699. doi: 10.1371/journal.pone.0031699
Norman, A. J. (2016). Genomic Studies of Contemporary Processes in Wild Populations. PhD thesis, Swedish University of Agricultural Sciences.
Ordiz, A., Bischof, R., and Swenson, J. E. (2013a). Saving large carnivores, but losing the apex predator? Biol. Conserv. 168, 128–133. doi: 10.1016/j.biocon.2013.09.024
Ordiz, A., Kindberg, J., Sæb,ø, S., Swenson, J. E., and Støen, O.-G. (2014). Brown bear circadian behavior reveals human environmental encroachment. Biol. Conserv. 173, 1–9. doi: 10.1016/j.biocon.2014.03.006
Ordiz, A., Støen, O. G., Sæb,ø, S., Sahlen, V., Pedersen, B. E., Kindberg, J., et al. (2013b). Lasting behavioural responses of brown bears to experimental encounters with humans. J. Appl. Ecol. 50, 306–314. doi: 10.1111/1365-2664.12047
Pagano, A. M., Carnahan, A. M., Robbins, C. T., Owen, M. A., Batson, T., Wagner, N., et al. (2018). Energetic costs of locomotion in bears: is plantigrade locomotion energetically economical? J. Exp. Biol. 221:jeb175372. doi: 10.1242/jeb.175372
Pinheiro, J., Bates, D., DebRoy, S., and Sarkar, D. R, Core Team, (2017). nlme: Linear and Nonlinear Mixed Effects Models. R Package Version, 3.1-131. Available online at: https://CRAN.R-project.org/package=nlme
R Core Team (2017). R: A Language and Environment for Statistical Computing. Vienna: R Foundation for Statistical Computing, Vienna, Austria. R Foundation for Statistical Computing.
Robbins, C. T., Ben-David, M., Fortin, J. K., and Nelson, O. L. (2012). Maternal condition determines birth date and growth of newborn bear cubs. J. Mammal. 93, 540–546. doi: 10.1644/11-mamm-a-155.1
Rode, K. D., Farley, S. D., Fortin, J., and Robbins, C. T. (2007). Nutritional consequences of experimentally introduced tourism in brown bears. J. Wildl. Manag. 71, 929–939. doi: 10.2193/2006-075
Sahlén, E., Støen, O.-G., and Swenson, J. E. (2011). Brown bear den site concealment in relation to human activity in Sweden. Ursus 22, 152–158. doi: 10.2192/URSUS-D-10-00007.1
Signorell, A. (2017). DescTools: Tools for Descriptive Statistics. R Package Version 0.99.23. Available online at: https://cran.r-project.org/package=DescTools
Swenson, J. E., Sandegren, F., Söderberg, A., Bjärvall, A., Franzén, R., and Wabakken, P. (1997). Infanticide caused by hunting of male bears. Nature 386, 450.
Swenson, J. E., Schneider, M., Zedrosser, A., Söderberg, A., Franzén, R., and Kindberg, J. (2017). Challenges of managing a European brown bear population; lessons from Sweden, 1943–2013. Wildl. Biol. 2017. doi: 10.2981/wlb.00251
Vaughan, M. R., and Inman, K. L. H. (2002). Hunter effort and success rates of hunting bears with hounds in Virginia. Ursus 13, 223–230.
Welch, C. A., Keay, J., Kendall, K. C., and Robbins, C. T. (1997). Constraints on frugivory by bears. Ecology 78, 1105–1119.
Wilmers, C. C., Nickel, B., Bryce, C. M., Smith, J. A., Wheat, R. E., and Yovovich, V. (2015). The golden age of bio-logging: how animal-borne sensors are advancing the frontiers of ecology. Ecology 96, 1741–1753.
Keywords: activity, body temperature, carnivore, heart rate, human disturbance, hunting dog
Citation: Le Grand L, Thorsen NH, Fuchs B, Evans AL, Laske TG, Arnemo JM, Sæbø S and Støen O-G (2019) Behavioral and Physiological Responses of Scandinavian Brown Bears (Ursus arctos) to Dog Hunts and Human Encounters. Front. Ecol. Evol. 7:134. doi: 10.3389/fevo.2019.00134
Received: 29 November 2018; Accepted: 03 April 2019;
Published: 24 April 2019.
Edited by:
Stan Boutin, University of Alberta, CanadaReviewed by:
Carl Soulsbury, University of Lincoln, United KingdomXimena J. Nelson, University of Canterbury, New Zealand
Copyright © 2019 Le Grand, Thorsen, Fuchs, Evans, Laske, Arnemo, Sæbø and Støen. This is an open-access article distributed under the terms of the Creative Commons Attribution License (CC BY). The use, distribution or reproduction in other forums is permitted, provided the original author(s) and the copyright owner(s) are credited and that the original publication in this journal is cited, in accordance with accepted academic practice. No use, distribution or reproduction is permitted which does not comply with these terms.
*Correspondence: Luc Le Grand, bHVjbGVncmFuZGNoQGdtYWlsLmNvbQ==