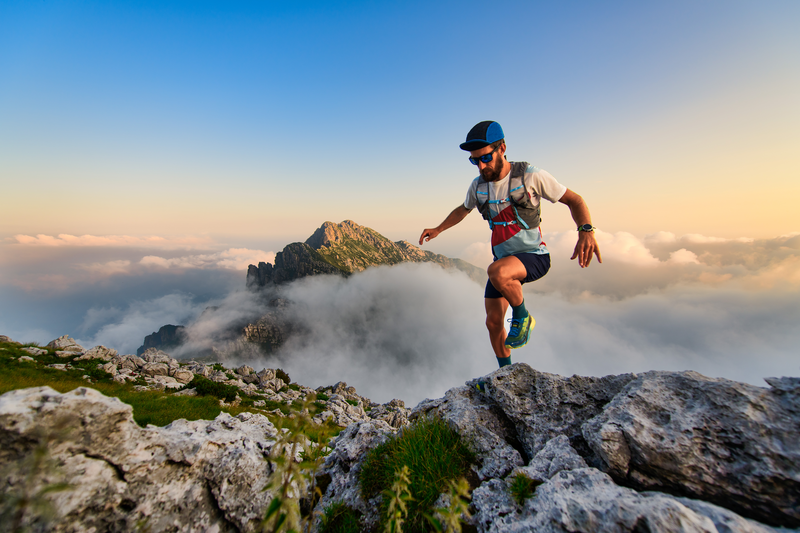
94% of researchers rate our articles as excellent or good
Learn more about the work of our research integrity team to safeguard the quality of each article we publish.
Find out more
ORIGINAL RESEARCH article
Front. Ecol. Evol. , 09 April 2019
Sec. Population, Community, and Ecosystem Dynamics
Volume 7 - 2019 | https://doi.org/10.3389/fevo.2019.00111
Resource competition is a major driver of dispersal: an emigrating individual leaves more resources to its kin. Existing models of sex-biased dispersal rarely consider intersexual competition for resources. Instead, male reproductive success is often solely assumed to depend on female availability, implying a tacit assumption that male presence never depletes resources, such as food, that are of interest to female kin. In reality, both male and female offspring typically consume resources on their natal site before departing to consume resources elsewhere, and sexually dimorphic body sizes imply that the resource needs can differ. The goal of our study is to investigate how intersexual competition for resources can affect the evolution of sex-specific dispersal, via competition between kin of the same sex or different sexes and the subsequent success elsewhere. Our individual-based simulation model allows not only the dispersal probability but also its timing to evolve. We consider dispersal timing because later dispersal yields a longer period of kin competition than dispersal that occurs soon after independence. We also highlight the role of sex-specific income/capital breeding strategies, which is understudied in both empirical and theoretical literature of sex-specific dispersal. We show that sex biases in dispersal probability and timing are sensitive to the presence of intersexual competition, sexual differences in capital vs. income breeding strategies, and sexual dimorphism in the quantity of resources consumed. Males may evolve to disperse earlier if they also consume more food, as a result of selection to reduce intersexual kin competition. Alternatively, males may evolve to disperse less as well as later than females, if male fitness depends more on resource accumulation (e.g., building a large body to succeed in mating competition under polygyny) whereas female fitness depends more on reliable income (e.g., in species that require extended periods of maternal foraging), even if both sexes are equally competitive in consuming resources. Although the more dispersive sex is often the earlier departing sex, we also find cases where the only clear dimorphism is found in dispersal timing. We thus encourage more studies on the timing aspect of sex-biased dispersal.
Dispersal alleviates competition for local resources: whatever it is that an individual needs to live, mate, and reproduce, it will not continue taking away these items from its local competitors once it has dispersed. This simple fact is the basis of numerous dispersal models (Hamilton and May, 1977; Gandon, 1999; Perrin and Mazalov, 2000; Bach et al., 2006; Brom et al., 2016), including models aimed at understanding sex-biased dispersal. Males and females typically differ in the kind of resources that limit their reproductive success, with a broad-brush characterization stating that female availability limits male fitness, while females may be limited by e.g., food, nest sites, or the ability to “convert resources into offspring” (Trivers, 1972; Emlen and Oring, 1977; Clutton-Brock and Harvey, 1978). Consequently, much of our theoretical understanding of sex-biased dispersal is based on understanding local mate competition for males and local resource competition for females (Perrin and Mazalov, 2000; Lawson Handley and Perrin, 2007; Li and Kokko, 2019).
Intuitively, it appears clear that an assumption “females need food while males only need females” represents an extreme case. In reality, a more usual situation is that both male and female offspring consume resources on their natal site before departing to consume resources elsewhere. Thus, even if female availability remains the major factor that limits male reproductive success, it does not follow that males always leave resources untouched that could have been of interest to females. Intersexual competition for food can be intense: in birds, for example, females (when they are subordinate in intersexual resource competition) can suffer from male presence, as they get displaced to use worse or more distant foraging (micro)habitats (Peters and Grubb, 1983; Pasinelli, 2000; Michler et al., 2011; for an opposite pattern in raptors with females as the dominant sex and female-biased sexual size dimorphism, see Temeles, 1986). Intersexual competition for food is also well studied in mammals, where males are often the larger sex: for example, male elephant seals in South Georgia population account for 59% of the annual energy expenditure and thereby become the major ecological competitors of the local females (Boyd et al., 1994).
Against this background, it is surprising that intersexual competition for resources is only rarely taken into consideration in mathematical models of the evolution of sex-specific dispersal. Two published studies have done this by contrasting two extreme scenarios (Leturque and Rousset, 2004; Henry et al., 2016): the local population is regulated by considering either (1) individuals of a given sex are affected only by the presence of same-sex conspecifics in the context of regulation, or (2) all local individuals contribute to density dependence (this introduces intersexual competition). In both studies, the general pattern was more male-biased dispersal if both sexes contribute to density regulation, but the details differ considerably: in Leturque and Rousset (2004), dispersal coevolves with primary sex ratio biases, while in Henry et al. (2016), the joint regulation scenario also added stochasticity to the system.
Here we are interested in considering features of real-life dispersal that appear lacking in the current body of theory concerning sex-biased dispersal and male-female resource use asymmetries. First, males do not only “potentially eat something” – in many species they are the larger sex, which comes with a greater potential to deplete local resources (Clutton-Brock and Harvey, 1978; Boyd et al., 1994; Rankin and Kokko, 2007; Kawatsu, 2018). Second, the timing of dispersal is an understudied factor in dispersal evolution; for rare exceptions in an insect mating context, see Hirota (2005, 2007). The relative timing of density regulation is known to alter predictions of dispersal rates (Sasaki and de Jong, 1999; Ravigné et al., 2004; Débarre and Gandon, 2011; Massol and Débarre, 2015), but we are not aware of a model that would expand on the logic that the timing of dispersal, not merely the occurrence (disperse vs. philopatry) of it, can evolve via kin selection. If juveniles (e.g., siblings) compete for the same resources as long as they reside together, then an earlier departure will save more of the resources to kin. This could, however, impose costs on the dispersers themselves. If successful dispersal requires good condition (Bowler and Benton, 2005; Naef-Daenzer and Grüebler, 2008; del Mar Delgado et al., 2010; Edelman, 2011, 2014; Mayer et al., 2017), then the safer option might be to wait and not disperse too early (though the opposite is possible too, e.g., if poor condition means the local site is poor and it is better to escape as quickly as possible, see Bowler and Benton, 2005; Mestre and Bonte, 2012).
If we are to understand sex-specific dispersal decisions that are impacted by male and female resource use over the entire time window during which dispersal is possible, it is clearly necessary to specify the extent to which early vs. late accumulation of resources contributes to breeding success in the end. In other words, it becomes necessary to link the effects of dispersal with the concepts of income and capital breeding (Stephens et al., 2009). An income breeder's reproductive success is determined by very recent resource acquisition; a capital breeder is the opposite. Although we are not aware of a systematic quantitative study of sex differences in capital vs. income aspects of sex-specific life histories, it is plausible to assume these to be variable, given the vast differences in sex roles across the animal kingdom (Janicke et al., 2016). As a whole, an interesting trade-off arises: an individual of a given sex might aid the success of kin by dispersing, but the effect on relatives of either sex can depend on timing decisions interacting with how much early resource use determines reproductive success in later life, as well as on the relative amount of resource use by the dispersing individual (possibly higher for males). By staying longer in the natal habitat, an individual can improve its condition and thus cope better with the energetic costs and hazards such as predation, but at the cost of competing with its kin. While the evolution of condition-dependent dispersal probability has been modeled in the context of asexual reproduction (Bonte and de la Peña, 2009; Kisdi et al., 2012), we believe ours to be the first study investing the timing aspect of dispersal in the context of intersexual resource competition.
The complexity of interactions between intersexual resource competition, sexual dimorphism in resource use and sex-specific capital/income breeding strategies goes beyond what can be captured by a simple analytical model. Therefore, we build an individual-based simulation model to analyze the complex evolutionary dynamics, and in particular, we allow the probability and timing of dispersal to coevolve.
We model a species inhabiting an environment that consists of S sites. Each site offers the same resource availability, enough to produce n offspring in each generation. Generations are discrete. Individuals are diploid, with balanced sex ratio at birth. Each individual has 4 unlinked loci, of which ϕf and ϕm determine the dispersal probability, and τf and τm determine the dispersal timing. Loci ϕf and τf are expressed only in females, and loci ϕm and τm are expressed only in males. In both sexes, the timing locus is not expressed if the individual does not disperse based on its ϕ value. Timing is relevant because individuals stop using resources that could be used by the same-sex and opposite-sex kin in the natal site as soon as they disperse. To avoid trivial results where all dispersal happens as early as possible, our model includes a counteracting force, so that it is “selfishly” better to delay dispersal: dispersal is risky and it is easier to survive this stage in the life cycle if condition has already been accumulating for a while via feeding in the natal site.
We consider a variety of scenarios for sex differences. First, we consider either the presence or absence of intersexual competition for food. While the complete lack of such competition is probably rare (implemented by assuming that males and females eat from completely “different pots,” i.e., consume different types of food), it is conceptually useful as it removes any effect that male dispersal has on female condition (or vice versa), thus allowing us to examine this effect when comparing to the case where sexes do compete for the same food (intersexual competition is present). Second, males and females may differ in whether condition obeys a long memory of past accumulation (capital breeding) or mainly reflects very recent feeding history (income breeding); we model this as a continuum rather than a dichotomous choice [in line with both empirical and theoretical literature (e.g., Pélisson et al., 2013; Stephens et al., 2014; Davis et al., 2016; Williams et al., 2017; Varpe and Ejsmond, 2018)]. Third, we assume that males and females may differ in their food requirements (e.g., because of different body size); we model this by assuming that males may need more (if β > 1) or less (if β < 1) food to reach an equivalent condition increase to that of females, and that the greater (or smaller) need is matched with greater (or smaller) actual intake (i.e., sex differences in intake ability matches sex differences in need).
We model the following life cycle. Competition for breeding positions happens within each habitat patch. Each patch allows only one female to breed, and she is assumed to mate monandrously with one male in the same patch. The probability that an individual outcompetes same-sex competitors (and thus obtains a breeding position) is assumed to be proportional to the focal individual's condition relative to that of its competitors. Next, n offspring are formed with their sex determined randomly and with Mendelian inheritance of all loci, but with a small mutation rate μ which is identical across all loci. If a mutation happens at a dispersal probability locus (ϕf or ϕm), the allele mutates to take the value of a random real number drawn from a uniform distribution between 0 and 1. A mutation that happens at a dispersal timing locus (τf or τm) causes the mutant allele to take a random integer value from 1 to the last possible time point for dispersal, denoted T.
Generations are discrete, thus all individuals of the parental generation die after breeding (regardless of their own breeding success). Newborn individuals spend a total of T time units accumulating condition by feeding. Each unit of feeding can be performed either in the natal or in a post-dispersal breeding patch, and the consumption of local food resources occurs in competition with other individuals in the same patch. The accumulation of condition α of an individual of sex i (i takes m or f) during a time unit from t − 1 to t is αi(t) = bi α i(t − 1) + R/(nf + βnm) if males and females compete for the same food, otherwise (in the absence of intersexual competition for food), αf(t) = bf α f(t − 1) + Rf/nf for females, and αm(t) = bm α m(t − 1) + Rm/βnm for males. Here bf and bm adjusts the gradient between income/capital breeding, which can differ between males and females (if bi = 0, condition only reflects the most recent food intake). R is the total amount of available food per site and per unit time (R = Rm + Rf in case males and females consume different resources); nf and nm are the current number of females and males at the focal site. The parameter β reflects sex differences in food requirements, but note that in the case of both sexes competing for the same food, male and female condition accumulates locally at the same rate, as the larger food requirements of males (β > 1) are matched by their larger intake rate. Male resource acquisition in this case depletes resources in a manner that is felt by females and males alike, thus β appears in the equations for both sexes. On the other hand, in the absence of intersexual competition for food, β > 1 does not harm female condition accumulation. Now males compete purely among themselves, and their greater resource use (combined with greater need) translates into a slower condition accumulation than what is achieved by the female subpopulation.
Whether an individual is going to disperse is determined solely by an individual's dispersal probability, quantified as the mean value of the two alleles at ϕf or ϕm for females and males, respectively. Dispersers then also express their timing alleles, with the length of the pre-dispersal period equal to the mean allelic value at τf or τm depending on the sex. For example, an individual whose dispersal phenotype combines a dispersal probability 0.5 (e.g., allelic values 0.4 and 0.6) with timing 5 (e.g., allelic values 3 and 7) will, at the beginning of time step 5, disperse with probability 0.5 (and remain philopatric with the complementary probability 0.5). No other time step leads to dispersal for this individual. If dispersal occurred, it either leads to death (see below) or moves the individual to a random site (for computational simplicity we do not exclude returning to the natal site, as this only occurs with a small probability 1/S, and S is set to 1,000 in the simulations). Survived dispersers feed in the post-dispersal breeding habitat afterwards (including time step 5 in the example above).
Dispersal mortality rate of an individual of sex i with condition α is modeled as . This function has the desirable properties that an individual with zero condition dies with certainty during dispersal, and the mortality rate decreases with increasing individual condition. The coefficient makes situations comparable across scenarios by correcting for the fact that absolute values for condition become larger if condition accumulates under capital breeding scenarios with a high bi. This scaling ensures that continual eating of 1 unit of food per time unit leads asymptotically to a dispersal mortality of m0.
We initialize the population to consist of individuals with all dispersal probability alleles set to 0.5 and all timing alleles set to 10 (halfway of T = 20, the length of the annual cycle in our computed examples). The Octave/Matlab script for the simulation is provided in the Online Repository.
The presence of intersexual competition for food can make sex differences in dispersal probability disappear (Figures 1a,b). When males need more food than females (above the black dashed lines in each of the panels in Figure 1), males might need to feed for longer to reach the same condition. The conditional nature of this statement arises because it is also possible that males eat correspondingly more per time unit, canceling the time disadvantage caused by their higher need. We assume that males can fulfill their higher requirements by eating some food that, in their absence, would have been eaten by females; this is possible in our intersexual competition scenario but impossible if resource competition occurs solely within one sex. In the latter case, males will lag behind females in condition accumulation, and as poor condition increases dispersal mortality, dispersal ends up being costlier for males than for females [or vice versa, depending on the sign of ln(β)]. This explains why the equilibrium dispersal probability evolves to be female-biased when the food requirements of males are higher than that of females, and vice-versa (Figure 1a). Whenever males disperse less, they also disperse later than do females (Figure 1c, green areas), which ameliorates but does not completely remove the difference in costs paid (Figure 1e, when ln(β) > 0 females still have lower dispersal mortality than males).
Figure 1. Female-male difference between (a,b) equilibrium dispersal probability, (c,d) dispersal timing, and (e,f) dispersal associated mortality, in the absence (left panels) or presence (right panels) of intersexual competition for food. In each panel, the x-axis represents the income/capital breeding parameter (bm = bf), and the y-axis represents the relative food consumption of males to females [ln(β)]. Simulation parameteres: S = 1, 000, n = 8, μ = 0.01, m0 = 0.1,T = 20, R = 8, Rf = Rm = 4, β ranges from 0.5 to 2 with steps equal to 0.1, bm = bf range from 0.1 to 0.9, also with steps equal to 0.1. Each simulation is run for 22,000 generations and the value in each pixel is calculated as the mean of the last 20,000 generations.
When the model includes intersexual competition for food, the situation changes. The timing pattern flips completely: now if males need more food, they disperse earlier (Figure 1d). Note that condition accumulation is now assumed to occur at the same rate between sexes (males being able to cover their needs and leaving less food for females). The earlier timing of male dispersal leads to a subtly higher dispersal mortality for males than females (Figure 1f), with no sex bias in dispersal probability (Figure 1b).
Our model does not have spatiotemporal variation in resource availability, thus the sole reason for an individual to disperse early is to switch resource use to a site other than where philopatric kin are gathering resources (while the reason to disperse late is that condition has accumulated to make it safer). In the absence of intersexual competition for resources, a male's dispersal can be interpreted as alleviating local mate competition as well as local resource competition (among male kin). But in the presence of intersexual competition, a departing male can also leave more resources to his sisters, who then increase their chances of reproduction – by either outcompeting potential immigrant females if they stay philopatric, or surviving dispersal better if they later depart themselves, followed by improved chances of acquiring breeding positions.
Whether intersexual competition is present or not, the sex bias in dispersal timing is largest when bf and bm are large (capital rather than income breeding) and when sexes differ markedly in resource use [large absolute value of ln(β)].
Above, we discussed cases where males and females differ in food consumption but are identical with respect to the income vs. capital breeding continuum (Figure 1). We now proceed to show that sex differences in the relative importance of resources acquired in the past can play an important role in determining the direction and magnitude of sex-biased dispersal. We first illustrate this with a case where males and females are not dimorphic with respect to intake rate (β = 1) (Figure 2a). Under this scenario, the sex that is more of an income breeder (i.e., has the smaller bi) disperses more (Figures 2a.1, a.2) as well as earlier (Figures 2a.3, a.4). Since the roles of males and females are identical except for the income/capital breeding aspect, the results in each panel are all symmetric about the diagonal, regardless of the presence or absence of intersexual competition for resources. The results along the dotted lines along the diagonal in Figure 2a correspond to the horizontal dotted lines in the matching panels of Figure 1.
Figure 2. Female-male difference in dispersal probability when (a) males and females have the same need of food consumption (β = 1), and when (b) males need twice as much food as females (β = 2). In each panel, the x and y axes represent the income/capital breeding parameters of females (bf) and males (bm), respectively. The parameters range from 0.1 to 0.9 with equal steps of 0.1; larger values mean more emphasis on capital (long-term resource acquisition). Panels in each row use the same color scale. The other simulation parameters and simulation conditions are the same as in Figure 1.
Dispersal probability and timing evolve to be more strongly sexually dimorphic in the presence of intersexual resource competition than in its absence (colors in Figures 2a.2, 2a.4 are deeper than in Figures 2a.1, 2a.3). Under intersexual competition, the sex that is more capital breeding than the other evolves to be more reluctant to disperse (both less often and later in timing) and suffers less from dispersal mortality; consequently, the sex difference in dispersal mortality remains smaller (colors in Figure 2a.6 is lighter than in Figure 2a.5).
Once we reintroduce a sex difference in food requirements (exemplified with males having the larger intake, β = 2, in Figure 2b), the presence or absence of intersexual competition for food can affect not only the magnitude but also the direction of sex-bias in dispersal probability and timing, and the results are no longer symmetric about the diagonal (except for dispersal probability in Figure 2b.2). In the absence of intersexual competition (left column in Figures 2a,b), males that require more food than females do not actually eat more than females, as they solely compete with other males for food. This slows their condition accumulation and creates male reluctance to disperse. The parameter region where females disperse more and earlier increases in size, so that in a large region under the diagonal line, males disperse less and later than females (Figures 2b.1, 2b.3) even where the capital/income breeding difference on its own would predict the opposite. Note that male reluctance to disperse ameliorates but does not fully compensate their higher dispersal mortality (females still survive better through the dispersal phase, Figure 2b.5), in agreement with the corresponding results in the left column of Figure 1.
When intersexual resource competition is present, the situation changes because male feeding can now harm female condition accumulation. The higher food intake of males now expands the parameter region where they disperse earlier than females (compare Figures 2b.4 to 2a.4), while causing little change to sex-specific dispersal probabilities (compare Figures 2b.2 to 2a.2). This is in agreement with the lack of impact of relative food intake of males on dispersal probabilities in Figure 1b. When the presence of male siblings causes significant harm to their sisters (through competition for food), kin selection can drive males to depart earlier even when they are more capital breeding than their sisters (purple region above the diagonal, Figure 2b.4). Similar to the results in Figure 1f, the earlier timing of male dispersal when intersexual competition is present causes dispersal mortality of males to be slightly higher than females (Figure 2b.6).
Our results show that intersexual resource competition can complicate predictions of sex-biased dispersal, and that timing of dispersal can be used to detect kin-selected patterns that would remain invisible if one only quantified the overall probability of dispersal. For example, even if overall dispersal probabilities do not differ between the sexes, males may evolve to depart earlier (or later) than females. This can happen as a result of both sexes feeding on the same resources, with males requiring more food (note that we assume they also meet their larger requirements, which is plausible if larger body size boosts behavioral dominance while simultaneously increasing energetic demands). This asymmetry implies that dispersal improves indirect fitness more if the departing individual is a male (whose local harm to kin is greater) than if it is a female.
The above is only one possible pattern, as sexes can also differ with respect to the capital vs. income breeding strategy. When females are closer to being capital breeders while male success is better described as income breeding, females evolve to disperse less as well as later than males; the reverse condition leads to the reverse outcome. This applies in the absence of other sex differences; if such differences exist, the net effect depends on an interaction between the location of the sexes on the capital/income axis and the sex-specific resource requirements.
How strong is the empirical evidence supporting our model? At present, efforts to evaluate this are hampered by the fact that broad patterns of sex-biased dispersal tend to be evaluated with respect to dispersal probability and/or dispersal distance (Clarke et al., 1997; Mabry et al., 2013; Trochet et al., 2016; Li and Kokko, 2019). We have shown that timing can also be kin-selected, but the comparative studies available to evaluate this factor are rather focused on delayed dispersal in cooperative breeding, where philopatry also offers individuals the opportunity to become helpers (Zhang et al., 2017). These additional kin-selected opportunities are interesting in their own right (Johnstone and Cant, 2008), but our model shows that interesting phenomena can arise even if the only form of helping and harming is the choice between dispersal and continuing to deplete local resources, with no other layers of social complexity. The role of kin competition in driving dispersal evolution as a whole can be difficult to detect empirically, because the observation that individuals successfully avoid competing with kin (through dispersal) can lead to an erroneous conclusion that the dismantled kin associations made kin selection irrelevant, despite dispersal having evolved precisely to achieve this dismantling [“ghost of competition past” (Connell, 1980), see Li and Kokko (2019) for discussing it in a dispersal context].
Additionally, there is a special challenge evaluating our predictions with respect to income vs. capital breeding this spectrum of breeding strategies tends to be considered for females, both in terms of theory (Houston et al., 2006; Stephens et al., 2014; Varpe and Ejsmond, 2018) and data (Meijer and Drent, 1999; Boyd, 2000; Davis et al., 2016). Attempts to compare the location of males and females along the capital-income axis are rare (see Stephens et al., 2009 for a discussion), despite much interest in the broader question of sex-specific life histories (reviews: Bonduriansky et al., 2008; Immonen et al., 2018). We would like to encourage both empirical and theoretical studies to continue exploring this topic, especially as our treatment necessarily has not been able to consider all possible interactions between current resource intake and the current vs. future condition of self and others. For example, one possibility we did not include is that individuals of one sex might need more food and simultaneously be disproportionally able, or unable, to meet the requirements. Also, our decision to keep the spatial structure simple (equivalent to Hamilton and May's classic 1977 study) means that we have not considered e.g., whether dispersal of income and capital breeders might differ if fitness of the former is more sensitive to fluctuating resources, or whether female demographic dominance (the fact that patch-level reproductive output responds more to the number of female than male breeders, see Harts et al., 2014) might alter the predictions if more than one breeder of each sex is permitted per site.
With these general observations in place, we would like to comment on a few case studies that provide food for thought. Many lekking birds follow the general avian rule of males being the philopatric sex (e.g., black grouse: Höglund et al., 1999; Caizergues and Ellison, 2002; sage grouse: Dunn and Braun, 1985; greater prairie-chicken: Kemink and Kesler, 2013), others show no sex bias in dispersal (e.g., capercaillie: Mäki-petäys et al., 2007). As an intriguing exception, great bustards (Otis tarda) show male-biased dispersal where juvenile males—the sex that grows faster and continues to grow for a longer period (Johnsgard, 1991)—disperse earlier and travel farther away than female offspring (Alonso et al., 1998). This species has the largest sexual size dimorphism among all extant birds, with mature males averaging 2.48 times the weight of females (Alonso et al., 2009). Mothers supplement chicks with extra food for about 200 (or up to 300 in some cases) days, with rates of feeding gradually declining (Alonso et al., 1998). Sexual size dimorphism emerges during this time, and males (that also receive more food from their mother than their female siblings, see Alonso et al., 1998) become independent more than a month earlier than females. This concurs with our predictions in the first part of the results, forming an interesting contrast to discussions of male philopatry in those species in which males are suspected to gain kin selected benefits of remaining together as adults to attract females (Höglund et al., 1999; Krakauer, 2005). In short, it is possible that the same force—kin selection—can pull toward male philopatry via the benefits of cooperative alliances but also, via resource use, push males to disperse early and far, if dimorphism in resource use is strong.
As we stated above, studies about timing of dispersal are much rarer than those documenting the propensity and/or distance of dispersal, especially with respect to condition accumulation. A rare example of a detailed study of condition is on banner-tailed kangaroo rats (Dipodomys spectabilis), which shows just how complicated the question can become. The rodent lacks either strong sex-biased dispersal (Waser et al., 2006; Edelman, 2011) or sexual size dimorphism (142.3 and 142.0 mm or body length for adult males and females, respectively, Best, 1988). Timing is somewhat sexually dimorphic, however: males and females have been found to disperse at similar ages when population density was low, but at high density, females delayed dispersal more than males did (Edelman, 2014). Since the reproductive success of males often depends more strongly on body size than that of females due to stronger sexual selection and male-male competition, it has been argued that mothers may benefit from allowing sons to remain at home until they reach a good-enough condition, an idea related to our “capital breeding in males” interpretation; this interpretation is supported by experimental evidence that food supplementation made sons disperse earlier while there was no effect on daughters' dispersal timing (Edelman, 2011). That sons respond to good feeding conditions at home (Edelman, 2011), while daughters instead respond to high density (Edelman, 2014), may reflect sex-specific difficulties of securing a breeding position (a mound) elsewhere, as dispersing females have been observed to move longer distances (Waser et al., 2006). It should be noted, however, that vacant positions can be rare in both sexes, and the largest effects in Edelmans' models were factors related to resource and kin competition that impacted both sexes, and sex only had a relatively subtle effect in his studies, leading us to place this species near the diagonal of Figure 2a.
The examples we discussed above represent particularly well documented yet isolated cases. Clearly, it would be interesting to have more data systematically collected on the timing of dispersal, in addition to probabilities or distances. Obviously, the probability and timing of dispersal can be influenced by multiple factors beyond what we have incorporated in the current model, including mate searching, inbreeding and outbreeding, population densities affecting success at the natal site and elsewhere, and spatiotemporal environmental variation (Morbey and Ydenberg, 2001; Matthysen, 2012; Starrfelt and Kokko, 2012; for an interesting case study with multiple influences see Arnold, 1990). We envisage these factors to lead to interesting extensions for the current model. Additionally, for species where comprehensive empirical data on the life history traits and sex-specific dispersal patterns are available, it might be interesting to build and study models of more species-specific details. For example, our model assumptions used discrete generations to reduce the number of additional assumptions made: with iteroparity one would have to specify what happens to individuals who failed to find a vacant spot (die, or form a floater pool), and whether territory takeovers are possible or vacancies only occur when breeders die. Although alternative life histories to the one we modeled are unlikely to destroy our general conclusion that consumption of resources can impact dispersal via intersexual competition, further work could usefully tie such considerations with general ideas of sex-biased dispersal (Li and Kokko, 2019). In general, we believe that both the complexity of kin effects across the two sexes, and timing issues that are more broadly applicable than the extreme case of delayed dispersal of cooperative breeders, warrant more theoretical as well as empirical attention.
All authors listed have made a substantial, direct and intellectual contribution to the work, and approved it for publication.
This work was supported by the Swiss National Science Foundation project funding (Div. I-III) Nr. 163374 granted to HK and the career funding Ambizione Nr. 180145 granted to X-YL.
The authors declare that the research was conducted in the absence of any commercial or financial relationships that could be construed as a potential conflict of interest.
X-YL would like to thank Toomas Tammaru for constructive discussions on empirical studies about the income/capital breeding continuum. Both authors are grateful to the two reviewers for their helpful comments and suggestions.
Alonso, J., Martín, E., Alonso, J., and Morales, M. B. (1998). Proximate and ultimate causes of natal dispersal in the great bustard Otis tarda. Behav. Ecol. 9, 243–252.
Alonso, J. C., Magaña, M., Alonso, J. A., Palacín, C., Martín, C. A., and Martín, B. (2009). The most extreme sexual size dimorphism among birds: allometry, selection, and early juvenile development in the great bustard (Otis tarda). Auk 126, 657–665. doi: 10.1525/auk.2009.08233
Arnold, W. (1990). The evolution of marmot sociality: I. why disperse late? Behav. Ecol. Sociobiol. 27, 229–237.
Bach, L. A., Thomsen, R., Pertoldi, C., and Loeschcke, V. (2006). Kin competition and the evolution of dispersal in an individual-based model. Ecol. Model. 192, 658–666. doi: 10.1016/j.ecolmodel.2005.07.026
Bonduriansky, R., Maklakov, A., Zajitschek, F., and Brooks, R. (2008). Sexual selection, sexual conflict and the evolution of ageing and life span. Funct. Ecol. 22, 443–453. doi: 10.1111/j.1365-2435.2008.01417.x
Bonte, D., and de la Peña, E. (2009). Evolution of body condition-dependent dispersal in metapopulations. J. Evol. Biol. 22, 1242–1251. doi: 10.1111/j.1420-9101.2009.01737.x
Bowler, D. E., and Benton, T. G. (2005). Causes and consequences of animal dispersal strategies: relating individual behaviour to spatial dynamics. Biol. Rev. 80, 205–225. doi: 10.1017/S1464793104006645
Boyd, I. L. (2000). State-dependent fertility in pinnipeds: contrasting capital and income breeders. Funct. Ecol. 4, 623–630. doi: 10.1046/j.1365-2435.2000.t01-1-00463.x
Boyd, I. L., Arnbom, T. A., and Fedak, M. A. (1994). “Biomass and energy consumption of the south georgia population of southern elephant seals,” in Elephant Seals: Population Ecology, Behavior, and Physiology, eds. B. J. Le Boeuf and R. M. Laws (Berkeley, CA: University of California Press), 98–120.
Brom, T., Massot, M., Legendre, S., and Laloi, D. (2016). Kin competition drives the evolution of sex-biased dispersal under monandry and polyandry, not under monogamy. Anim. Behav. 113, 157–166. doi: 10.1016/j.anbehav.2016.01.003
Caizergues, A., and Ellison, L. N. (2002). Natal dispersal and its consequences in black grouse Tetrao tetrix. Ibis 144, 478–487. doi: 10.1046/j.1474-919X.2002.00040.x
Clarke, A. L., Sæther, B.-E., and Røskaft, E. (1997). Sex biases in avian dispersal: a reappraisal. Oikos 79, 429–438.
Clutton-Brock, T., and Harvey, P. H. (1978). Mammals, resources and reproductive strategies. Nature 273, 191–195.
Connell, J. H. (1980). Diversity and the coevolution of competitors, or the ghost of competition past. Oikos 35, 131–138.
Davis, R. B., Javoiš, J., Kaasik, A., Õunap, E., and Tammaru, T. (2016). An ordination of life histories using morphological proxies: Capital vs. income breeding in insects. Ecology 97, 2112–2124. doi: 10.1002/ecy.1435
Débarre, F., and Gandon, S. (2011). Evolution in heterogeneous environments: between soft and hard selection. Am. Natural. 177, E84–E97. doi: 10.1086/658178
del Mar Delgado, M., Penteriani, V., Revilla, E., and Nams, V. O. (2010). The effect of phenotypic traits and external cues on natal dispersal movements. J. Anim. Ecol. 79, 620–632. doi: 10.1111/j.1365-2656.2009.01655.x
Dunn, P. O., and Braun, C. E. (1985). Natal dispersal and lek fidelity of sage grouse. Auk 102, 621–627.
Edelman, A. J. (2011). Sex-specific effects of size and condition on timing of natal dispersal in kangaroo rats. Behav. Ecol. 22, 776–783. doi: 10.1093/beheco/arr050
Edelman, A. J. (2014). Multiple socioecological factors influence timing of natal dispersal in kangaroo rats. Behav. Ecol. Sociobiol. 68, 1123–1131. doi: 10.1007/s00265-014-1723-x
Emlen, S. T., and Oring, L. W. (1977). Ecology, sexual selection, and the evolution of mating systems. Science 197, 215–223.
Gandon, S. (1999). Kin competition, the cost of inbreeding and the evolution of dispersal. J. Theoret. Biol. 200, 345–364.
Harts, A. M., Schwanz, L. E., and Kokko, H. (2014). Demography can favour female-advantageous alleles. Proc. R. Soc. Lond. B Biol. Sci. 281:20140005. doi: 10.1098/rspb.2014.0005
Henry, R. C., Coulon, A., and Travis, J. M. (2016). The evolution of male-biased dispersal under the joint selective forces of inbreeding load and demographic and environmental stochasticity. Am. Natural. 188, 423–33. doi: 10.1086/688170
Hirota, T. (2005). The effect of female polyandry and sperm precedence on the evolution of sexual difference in dispersal timing. J. Evol. Biol. 18, 1395–1402. doi: 10.1111/j.1420-9101.2005.00973.x
Hirota, T. (2007). The evolution of sexual difference in dispersal strategy sensitive to population density. Appl. Entomol. Zool. 42, 173–179. doi: 10.1303/aez.2007.173
Höglund, J., Alatalo, R. V., Lundberg, A., RintamÎki, P. T., and Lindell, J. (1999). Microsatellite markers reveal the potential for kin selection on black grouse leks. Proc. R. Soc. B Biol. Sci. 266, 813–816.
Houston, A. I., Stephens, P. A., Boyd, I. L., Harding, K. C., and McNamara, J. M. (2006). Capital or income breeding? a theoretical model of female reproductive strategies. Behav. Ecol. 18, 241–250. doi: 10.1093/beheco/arl080
Immonen, E., Hämäläinen, A., Schuett, W., and Tarka, M. (2018). Evolution of sex-specific pace-of-life syndromes: genetic architecture and physiological mechanisms. Behav. Ecol. Sociobiol. 72:60. doi: 10.1007/s00265-018-2462-1
Janicke, T., Häderer, I. K., Lajeunesse, M. J., and Anthes, N. (2016). Darwinian sex roles confirmed across the animal kingdom. Sci. Adv. 2:e1500983. doi: 10.1126/sciadv.1500983
Johnsgard, P. A. (1991). Bustards, Hemipodes, and Sandgrouse: Birds of Dry Places. Oxford: Oxford University Press.
Johnstone, R. A., and Cant, M. A. (2008). Sex differences in dispersal and the evolution of helping and harming. Am. Natural. 172, 318–330. doi: 10.1086/589899
Kawatsu, K. (2018). Ecological effects of sex differ with trophic positions in a simple food web. Ecol. Evol. 8, 1239–1246. doi: 10.1002/ece3.3740
Kemink, K., and Kesler, D. (2013). Using movement ecology to inform translocation efforts: a case study with an endangered lekking bird species. Anim. Conserv. 16, 449–457. doi: 10.1111/acv.12015
Kisdi, E., Utz, M., and Gyllenberg, M. (2012). “Evolution of condition-dependent dispersal,” in Dispersal Ecology and Evolution, eds. J. Clobert, M. Baguette, T. Benton, and J. Bullock (Oxford: Oxford University Press), 139–151.
Krakauer, A. H. (2005). Kin selection and cooperative courtship in wild turkeys. Nature 434, 69–72. doi: 10.1038/nature03325
Lawson Handley, L. J., and Perrin, N. (2007). Advances in our understanding of mammalian sex-biased dispersal. Mol. Ecol. 16, 1559–1578. doi: 10.1111/j.1365-294X.2006.03152.x
Leturque, H., and Rousset, F. (2004). Intersexual competition as an explanation for sex-ratio and dispersal biases in polygynous species. Evolution 58, 2398–2408. doi: 10.1111/j.0014-3820.2004.tb00870.x
Li, X.-Y., and Kokko, H. (2019). Sex-biased dispersal: a review of the theory. Biol. Rev. 94, 721-736. doi: 10.1111/brv.12475
Mabry, K. E., Shelley, E. L., Davis, K. E., Blumstein, D. T., and Van Vuren, D. H. (2013). Social mating system and sex-biased dispersal in mammals and birds: a phylogenetic analysis. PLoS ONE 8:e57980. doi: 10.1371/journal.pone.0057980
Mäki-petäys, H., Corander, J., Aalto, J., Liukkonen, T., Helle, P., and Orell, M. (2007). No genetic evidence of sex-biased dispersal in a lekking bird, the capercaillie (Tetrao urogallus). J. Evol. Biol. 20, 865–873. doi: 10.1111/j.1420-9101.2007.01314.x
Massol, F., and Débarre, F. (2015). Evolution of dispersal in spatially and temporally variable environments: the importance of life cycles. Evolution 69, 1925–1937. doi: 10.1111/evo.12699
Matthysen, E. (2012). “Multicausality of dispersal: a review,” in Dispersal Ecology and Evolution, eds. J. Clobert, M. Baguette, T. G. Benton, and J. M. Bullock (Oxford: Oxford University Press)
Mayer, M., Zedrosser, A., and Rosell, F. (2017). When to leave: the timing of natal dispersal in a large, monogamous rodent, the eurasian beaver. Anim. Behav. 123, 375–382. doi: 10.1016/j.anbehav.2016.11.020
Meijer, T., and Drent, R. (1999). Re-examination of the capital and income dichotomy in breeding birds. Ibis 141, 399–414.
Mestre, L., and Bonte, D. (2012). Food stress during juvenile and maternal development shapes natal and breeding dispersal in a spider. Behav. Ecol. 23, 759–764. doi: 10.1093/beheco/ars024
Michler, S. P., Nicolaus, M., Ubels, R., van der Velde, M., Komdeur, J., Both, C., et al. (2011). Sex-specific effects of the local social environment on juvenile post-fledging dispersal in great tits. Behav. Ecol. Sociobiol. 65, 1975–1986. doi: 10.1007/s00265-011-1207-1
Morbey, Y. E., and Ydenberg, R. C. (2001). Protandrous arrival timing to breeding areas: a review. Ecol. Lett. 4, 663–673. doi: 10.1046/j.1461-0248.2001.00265.x
Naef-Daenzer, B., and Grüebler, M. U. (2008). Post-fledging range use of great tit Parus major families in relation to chick body condition. Ardea 96, 181–190. doi: 10.5253/078.096.0204
Pasinelli, G. (2000). Sexual dimorphism and foraging niche partitioning in the middle spotted woodpecker Dendrocopos medius. Ibis 142, 635–644. doi: 10.1111/j.1474-919X.2000.tb04463.x
Pélisson, P.-F., Bel-Venner, M.-C., Giron, D., Menu, F., and Venner, S. (2013). From income to capital breeding: when diversified strategies sustain species coexistence. PLoS ONE 8:e76086. doi: 10.1371/journal.pone.0076086
Perrin, N., and Mazalov, V. (2000). Local competition, inbreeding, and the evolution of sex-biased dispersal. Am. Natural. 155, 116–127. doi: 10.1086/303296
Peters, W. D. and Grubb T. C. Jr. (1983). An experimental analysis of sex-specific foraging in the downy woodpecker, Picoides pubescens. Ecology 64, 1437–1443.
Rankin, D. J., and Kokko, H. (2007). Do males matter? the role of males in population dynamics. Oikos 116, 335–348. doi: 10.1111/j.0030-1299.2007.15451.x
Ravigné, V., Olivieri, I., and Dieckmann, U. (2004). Implications of habitat choice for protected polymorphisms. Evol. Ecol. Res. 6, 125–145. Available online at: http://www.evolutionary-ecology.com/abstracts/v06/1602.html
Sasaki, A., and de Jong, G. (1999). Density dependence and unpredictable selection in a heterogeneous environment: Compromise and polymorphism in the ESS reaction norm. Evolution 53, 1329–1342.
Starrfelt, J., and Kokko, H. (2012). “The theory of dispersal under multiple influences,” in Dispersal Ecology and Evolution, eds J. Clobert, M. Baguette, T. G. Benton, and J. M. Bullock (Oxford: Oxford University Press).
Stephens, P. A., Boyd, I. L., McNamara, J. M., and Houston, A. I. (2009). Capital breeding and income breeding: their meaning, measurement, and worth. Ecology 90, 2057–2067. doi: 10.1890/08-1369.1
Stephens, P. A., Houston, A. I., Harding, K. C., Boyd, I. L., and McNamara, J. M. (2014). Capital and income breeding: the role of food supply. Ecology 95, 882–896. doi: 10.1890/13-1434.1
Temeles, E. J. (1986). Reversed sexual size dimorphism: effect on resource defense and foraging behaviors of nonbreeding northern harriers. Auk 103, 70–78.
Trivers, R. (1972). “Parental investment and sexual selection,” in Sexual Selection and the Descent of Man, ed. B. Campbell (New Brunswick, NJ: Transaction Publishers), 136–188.
Trochet, A., Courtois, E. A., Stevens, V. M., Baguette, M., Chaine, A., Schmeller, D. S., et al. (2016). Evolution of sex-biased dispersal. Quart. Rev. Biol. 91, 297–320. doi: 10.1086/688097
Varpe, Ø., and Ejsmond, M. J. (2018). Trade-offs between storage and survival affect diapause timing in capital breeders. Evol. Ecol. 32, 623–641. doi: 10.1007/s10682-018-9961-4
Waser, P. M., Busch, J. D., McCormick, C. R., and DeWoody, J. A. (2006). Parentage analysis detects cryptic precapture dispersal in a philopatric rodent. Mol. Ecol. 15, 1929–1937. doi: 10.1111/j.1365-294X.2006.02893.x
Williams, C. T., Klaassen, M., Barnes, B. M., Buck, C. L., Arnold, W., Giroud, S., et al. (2017). Seasonal reproductive tactics: annual timing and the capital-to-income breeder continuum. Philos. Trans. R. Soc. B Biol. Sci. 372:20160250. doi: 10.1098/rstb.2016.0250
Keywords: dispersal probability, dispersal timing, income/capital breeding, intersexual competition, kin selection, local mate competition (LMC), local resource competition (LRC), sexual dimorphism
Citation: Li X-Y and Kokko H (2019) Intersexual Resource Competition and the Evolution of Sex-Biased Dispersal. Front. Ecol. Evol. 7:111. doi: 10.3389/fevo.2019.00111
Received: 08 October 2018; Accepted: 20 March 2019;
Published: 09 April 2019.
Edited by:
Anne Loison, Centre National de la Recherche Scientifique (CNRS), FranceReviewed by:
Jeremy Van Cleve, University of Kentucky, United StatesCopyright © 2019 Li and Kokko. This is an open-access article distributed under the terms of the Creative Commons Attribution License (CC BY). The use, distribution or reproduction in other forums is permitted, provided the original author(s) and the copyright owner(s) are credited and that the original publication in this journal is cited, in accordance with accepted academic practice. No use, distribution or reproduction is permitted which does not comply with these terms.
*Correspondence: Xiang-Yi Li, bGlAZXZvbGJpby5tcGcuZGU=
Disclaimer: All claims expressed in this article are solely those of the authors and do not necessarily represent those of their affiliated organizations, or those of the publisher, the editors and the reviewers. Any product that may be evaluated in this article or claim that may be made by its manufacturer is not guaranteed or endorsed by the publisher.
Research integrity at Frontiers
Learn more about the work of our research integrity team to safeguard the quality of each article we publish.