- Department of Behavioural Ecology and Evolutionary Genetics, Max Planck Institute for Ornithology, Seewiesen, Germany
Theoretical models predict that parents feeding offspring should partially compensate for the reduced care of their partner. However, for incubating birds, the level of compensation may depend on how reduced care changes the risk of entire brood failure, for example due to clutch predation, and on individual variation in the timing of depletion of energy stores. Although biparental incubation dominates in non-passerines, short-term manipulations of care during incubation are scarce. Here, we describe the response of 25 semipalmated sandpipers (Calidris pusilla) to an unexpected ~12-h absence (experimental removal) of their partner in the middle of the 21-day incubation period. During the period when the removed partner would have taken over to start its regular ~12-h incubation bout, parents compensated partially for the absence of their partner's care (mean: 59%, 95%CI: 49–70%). However, individuals varied in their response from no to full compensation, independent of parental sex. In contrast to incubation in undisturbed nests or by uniparental species, nest attendance of compensating parents tended to be higher during the warmer part of the day. Whereas compensation was unrelated to before-experimental share of incubation, parents that left the nest from a further distance upon human approach (more aware of or more “responsive” to their environment) compensated more. The quality of incubation in the after-experimental period, i.e., after return of the partner, was lower than usual, but improved quickly over time. In seven nests where the removed parent never returned, the widowed partner attended the nest for 0–10 days (median: 4), which suggests that widowed semipalmated sandpipers can adjust their incubation behavior to that observed in uniparental incubators. To conclude, our results indicate that biparental incubators are willing to tolerate a missed or irregular incubation bout of their partner. We speculate that all individuals would compensate fully, but that some fail because they deplete their energy stores, while others may be less responsive to or initially unaware of the absence of their partner.
Introduction
Biparental care can be seen as a complex social behavior where females and males cooperate in the rearing of their offspring. Although both parents gain from parental care provided by either of the parents, each parent only pays the costs of its own care. Consequently, each parent would have higher overall reproductive success if the other parent provided a larger share of the care (Trivers, 1972; Lessells, 2012). How do parents achieve cooperation in the face of this conflict?
Established theoretical models predict that parents should partially compensate for a reduction in their partner's care when an increase in parental care increases breeding success, but with diminishing returns (Houston and Davies, 1985; McNamara et al., 1999, 2003; reviewed by Lessells, 2012). These models have been mainly developed for and experimentally tested in passerine birds feeding their nestlings. In such tests, partial compensation seems the average response, but the response of parents varied substantially between studies (Harrison et al., 2009). Indeed, partial compensation is unlikely when breeding attempts fail due to a small decrease in parental care (Jones et al., 2002), when parents lack information about the brood need (including each other's effort) or lack the capacity to compensate (Johnstone and Hinde, 2006). These circumstances might be typical for biparental incubation of eggs in birds. The first (Jones et al., 2002) especially applies to species nesting in environments where unattended eggs are at high risk of predation (e.g., in colonies; Dearborn, 2001; Jones et al., 2002). The latter two, i.e., insufficient information about the brood need and lacking the capacity to compensate (Johnstone and Hinde, 2006), likely occur in species where the off-duty parent leaves the nest for several hours (e.g., Grant, 1982; Blanken and Nol, 1998; Wiebe, 2008) or even days (e.g., Johnstone and Davis, 1990; Weimerskirch, 1995; Gauthier-Clerc et al., 2001) and stays at distances from the nest that preclude instantaneous communication with the incubating partner, i.e., parents can communicate only when exchanging their incubation duties.
Biparental incubation of eggs prevails in 50% of avian families (and in 80% of non-passerine ones; Deeming, 2002). Within and across species, parents of biparental nests have higher reproductive success than parents incubating alone (Chalfoun and Martin, 2007; Smith et al., 2012; Bulla et al., 2017). Yet, increased incubation demands lead to higher mass loss and reduced immune function of the incubating parent, as well as to long-term fitness costs, e.g., through reduced capacity to provision offspring, reduced success rate of second clutches or reduced fecundity in the subsequent year (Heaney and Monaghan, 1996; Hanssen et al., 2005; de Heij et al., 2006). Note that such fitness costs of incubation hold for both uniparental and biparental parents, as even biparental parents trade off nest attendance with foraging time, especially if a parent is forced to forage when food supply is relatively low (Monaghan and Nager, 1997).
To test the response of incubating parents to absent or reduced care of their partner and to test for the negotiation between incubating parents, experimental manipulation of the parent must be moderate, so that it will not cause this parent to completely abandon its breeding attempt. A temporary, reversible manipulation fulfills this criterion and mimics naturally occurring, short-term deficiencies in the partner's care (e.g., its absence from the nest for a few hours during its incubation bout or its delayed return to the nest to exchange duties; Chaurand and Weimerskirch, 1994; Bulla et al., 2014). Temporary manipulations show an individual's immediate response to a sudden reduction in investment by its mate, and can also be used to investigate how the pair shares (or renegotiates) incubation duties after the manipulation ends (i.e., whether the manipulation and the partner's response influence subsequent care). In such experiments, the focus needs to be on between-individual differences in compensation. Indeed, when some individuals compensate fully while others not at all, evaluations at the population level would suggest partial compensation, where in fact no individuals partially compensate.
Many experimental studies investigated how parents respond to reduced care of their partner during biparental incubation, but the two dominant experimental approaches have long-term and irreversible effects for the focal breeding attempt. The first approach is to completely remove one parent, creating a situation of no care, reflecting permanent nest desertion (Burley, 1980; Erckmann, 1981; Bowman and Bird, 1987; Brunton, 1988; Duckworth, 1992; Pinxten et al., 1995). The second approach is to handicap one parent, creating a situation of reduced care, for example by experimentally increasing plasma testosterone levels in males (De Ridder et al., 2000; Alonso-Alvarez, 2001; McDonald et al., 2001; Schwagmeyer et al., 2005) or by attaching weights to one of the parents (Wiebe, 2010). In contrast, reversible manipulations of female and male incubation effort (e.g., by short-term supplemental feeding or temporary removal of one parent) with evaluation of between-individual differences in response to this manipulation are scarce (Gibbon et al., 1984; Kosztolányi et al., 2003; Kosztolanyi et al., 2009).
Here, we experimentally investigated the response of semipalmated sandpiper (Calidris pusilla) parents to the temporary absence of a partner during incubation. Semipalmated sandpipers are small shorebirds (22–32 g) that breed in the Arctic. Earlier studies suggested that they are obligate biparental incubators, i.e., the participation of both parents is required for successful incubation (Hicklin and Gratto-Trevor, 2010). Although recent work suggested that some parents successfully incubated a clutch on their own (Bulla et al., 2017), these may be exceptions favored by environmental circumstances. Incubating semipalmated sandpipers rarely feed during their incubation bout, within which they attend their nest 95% of the time (Bulla et al., 2014, 2015b). The incubation bouts last on average 11.5 h for females and 10.7 h for males (Bulla et al., 2014) and the off-duty parent is usually out of hearing distance of the incubating partner (Bulla et al., 2015b).
In the middle of the incubation period, we removed a parent at the end of its regular incubation bout and released it 24 h later. In this way, the temporarily widowed bird became responsible not only for its own incubation bout (control period), but also for the following “incubation bout” of its partner (treated period). We investigated the change in nest attendance between control and treated period, assessed how variable this change was between individuals, and whether it was sex-dependent. We anticipated four possible scenarios for how the temporarily widowed parent would respond (no compensation, full compensation, and two scenarios of partial compensation; Figure 1). We then tested whether the following three factors might explain diversity in the compensation response. (a) Time of day or tundra temperature: because it is less energetically demanding to incubate in the middle of the day when it is warm (Norton, 1973; Vleck, 1981; Kersten and Piersma, 1986; Williams, 1996; Nord et al., 2010; see Figure S1 in “Electronic Supplement—Figures” from Bulla et al., 2014 for relationship between temperature and the time of day, and Figure S1 from Bulla, 2019 for the same relationship during experimental bouts), we predict a higher level of compensation during the day compared to the colder night hours. Alternatively, the level of compensation during the day may be lower than during the night, if the amount of incubation is determined by either the need for incubation, which decreases with higher ambient temperatures during the day, or by foraging efficiency, which is also higher during the warmer parts of the day. (b) Incubation share prior to removal: individuals that have invested less in parental care in the current breeding attempt compared to other individuals may be either more reluctant to compensate or they may have more resources left for compensation (Bowman and Bird, 1987; Duckworth, 1992). (c) Individual variation in escape distance from the nest upon approach of a human: incubating individuals face a trade-off between the risk of increased adult mortality and the risk of breeding failure. As individuals that stay on the nest for longer when approached by a human take more “personal risk” to maximize nest protection, we expect them to compensate more than individuals that leave early upon human approach and thus minimize “personal risk” at the cost of reduced nest protection. Indeed, unattended eggs are at higher risk of predation (e.g., Dearborn, 2001; Jones et al., 2002), overheating (e.g., in deserts; AlRashidi et al., 2011) or severe cooling (e.g., in the Arctic or Antarctica; Gauthier-Clerc et al., 2001; Bulla et al., 2014). Alternatively, escape distance may reflect an individual's awareness of and “responsiveness” to the environment—i.e., behavioral syndrome or coping style (Koolhaas et al., 1999; Coppens et al., 2010). Individuals that stay on the nest for longer when approached by a human may be less “responsive” and hence less likely to detect the absence of the partner or less prone to compensate.
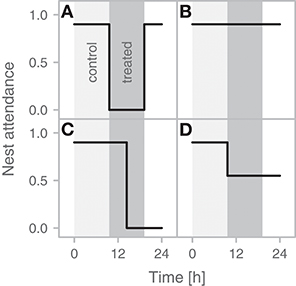
Figure 1. Possible compensation strategies for temporal absence of a partner. Zero on the x-axis represents the time when a parent is experimentally removed. The light gray area represents the typical incubation bout of the focal parent, i.e., the control period. The dark gray area represents the removed parent's expected incubation bout, i.e., the treated period. The black line indicates the change in nest attendance over time. (A) No compensation—an individual leaves the nest at the end of its incubation bout and returns when its next bout is expected to start—reflects a decision by the bird not to change its investment in response to the absence of its partner, or a lack of knowledge about the partner's absence (the bird may simply leave to forage at the end of its bout, as it typically does, without noticing the partner's absence). (B) Full compensation—an individual continuously incubating for the entire expected bout of its partner—arises if the incubating bird waits for the partner to return before ending its own bout, assuming that it has not yet reached its energetic limits. (C) Partial compensation—an individual continues incubating, but gives up and leaves at a certain point in time (e.g., when its energy stores are depleted) so that it goes from full to no compensation within its partner's “bout.” (D) Partial compensation—an individual continues incubating, but with lower nest attendance as it leaves the nest for short feeding bouts, that is, it starts behaving like a species that incubates uniparentally (Bulla et al., 2017).
To further investigate how the experiment influenced subsequent parental care (i.e., whether parents tolerate or retaliate the absence of their partner), we explored the incubation pattern after the removed parent returned. Specifically, we investigated how nest attendance, length of incubation bouts and probability and length of exchange gaps differed before and after the experiment.
Thus, the aim of our study was to address the following three questions. (1) How long does an individual persist in its incubation effort, while getting no feedback or interaction from its mate, before it abandons the breeding attempt? (2) When focal birds continue incubation in the absence of their partner, how does the incubation rhythm change over time and what are possible correlates of those patterns? (3) How do parents respond in terms of incubation patterns to the return of the removed parent?
Material and Methods
Study Site
We conducted the experiment in a population of semipalmated sandpipers near Utqiaġvik (Barrow), Alaska (71.32°N, 156.65°W), between 1 June and 4 July 2013. The study area and species are already described in detail elsewhere (Ashkenazie and Safriel, 1979a; Bulla et al., 2014). The area has continuous daylight throughout the breeding season, but environmental conditions such as ambient temperature show consistent and substantial diurnal fluctuations. Ambient temperatures are generally low, below 5°C, but surface tundra temperatures can reach up to 28°C (Supplementary Figure S1 in Bulla et al., 2014).
Recording Incubation and Escape Distance
The general procedure for monitoring incubation is described in detail elsewhere (Bulla et al., 2014, 2015a). In short, nests were found by systematically searching the tundra and by observing the behavior of birds flushed from the nest during laying or incubation. If a nest was found during laying, we estimated the start of incubation by assuming that the female laid one egg per day and started incubation when the clutch was complete (usually four, rarely three eggs). If nests were found with a full clutch, we estimated the start of incubation based on the median height and angle at which the eggs floated in water (as described in Liebezeit et al., 2007).
We captured adults and attached a plastic flag to the tarsus, which contained a glass passive tag (9.0 × 2.1 mm, 0.087 g, http://www.biomark.com/). The presence of parents on the nest was registered every 5 s by a custom made radio frequency identification device (RFID) with a thin antenna loop around the nest cup connected to a reader. Incubation was further determined by comparing nest temperature, measured with a high resolution temperature-probe, and surface tundra temperature, measured by an MSR145 data logger (MSR Electronics GmbH) placed next to the nest. In addition, out of 29 experimental nests 12 nests were video recorded for some days and 15 nests were protected against avian predators using enclosures made of mesh wire (Figure S2a in Bulla, 2019); neither the video cameras nor the enclosures influenced the incubation behavior (our unpublished data).
Unless parents were banded in previous years or caught with a mistnet prior to breeding, experienced scientists or well-trained field assistants caught incubating parents with a spring (bow net) trap triggered from a distance (~10–20 m) by a fishing line. This method allows for precise timing of catching and each individual thus spent only a few seconds in the trap and was banded, measured and sampled within ~10 min. The sex of individuals was known from previous years, or estimated from body measurements and later confirmed by molecular analyses using DNA extracted from a ca. 50 μl blood sample taken from the brachial vein at first capture (Bulla et al., 2014, 2015a).
Whenever we visited a nest, we observed when the incubating individual left and either estimated our distance to the nest or marked our position with a GPS to calculate the exact distance (see Bulla et al., 2016b). Before the initiation of the experiment, we visited nests (including finding the nest) on average 6 times (median; range: 3–11). The focal parent has thus seen us by the nest (after the clutch was complete and the RFID system was placed on the nest) on average twice before the start of the experiment (median; range: 1–7). In this way we obtained on average two observations of escape distance per individual (median; range: 0–4). For 11 individuals we only had a single estimate. For one individual we had no escape distance estimate, so we imputed this value (following the procedure outlined in Nakagawa and Freckleton, 2011) as the median of 1,000 imputations generated by the “Amelia” function in the “Amelia” R package (Honaker et al., 2011) with the range of likely escape distances (0–80 m) as a prior (note that excluding this individual did not change the results of the analyses). Escape distance was moderately repeatable (unpublished data from the same population; R = 0.49, 95%CI: 0.37–0.58, N = 275 observations of 123 individuals) and in the pre-experimental period changed little over time (Figure S3 in Bulla, 2019). Thus, here we used the median escape distance per individual.
Experimental Procedure
At 29 nests we temporarily removed one parent (henceforth, the “removed parent”) around the 11th day of the 19–21 day incubation period, shortly before we expected its partner (henceforth, the “focal parent”) to return to incubate (Figure 2, red line). The median period between capture of the “removed” parent and return of the “focal” parent was 1.7 h (range: 9.5 min−5.3 h). Such exchange gaps, although rare, also occur naturally (range: 0–6.7 h; 4% of exchanges with gaps > 10 min; Bulla et al., 2014), that is, parents occasionally return to an un-incubated nest. We assessed the expected return of the partner (i.e., planned the capture of the “removed parent”) by downloading the RFID data and visualizing the incubation pattern from the previous days. We alternated between removing the male and the female at a nest with a spring trap (for details see “Recording incubation” section above). Except in one case, we did not see the focal parent near the nest during the capture of its partner. The 29 removed individuals were at least 1-year old with body mass at capture between 22 g and 29.7 g (median 25 g).
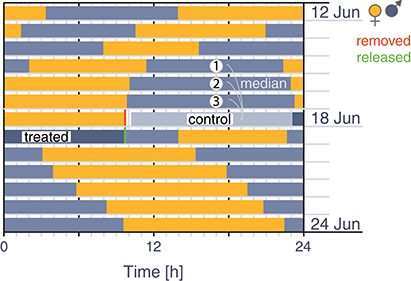
Figure 2. Schematic illustration of the experimental procedure. Rectangles indicate incubation bouts of a pair (female: yellow, male: blue-gray). We removed an incubating parent (here, the female) in the middle of the incubation period (indicated by the red vertical line), shortly before we expected its partner (the focal parent; here the male) to return. After 24 h we released the removed parent in the vicinity of its nest (the release time is indicated by the green vertical line). Thus, the focal parent incubated its “natural” incubation bout (“control” indicated by gray rectangle), defined as the period starting with the arrival of the focal parent on the nest and lasting for the length of the median incubation bout (estimated from the three previous incubation bouts of the focal parent). The remaining 12–13 h (i.e., the time between the end of the control period and the release of the removed parent) define the “treated period” of the focal parent (indicated by dark blue rectangle).
After 24 h we released the removed parent in the vicinity of its nest (Figure 2, green line). In this way, the focal parent incubated its “natural” incubation bout (henceforth, the “control period”; Figure 2, gray rectangle), which at this stage of incubation typically lasts about 10–11 h (Bulla et al., 2014). The remaining 12–13 h during which the partner was removed were then considered the “treated period” of the focal parent (Figure 2, dark blue rectangle).
Specifically, we defined the control period as the period starting with the arrival of the focal bird on the nest (after removal of its partner) and lasting for the length of the median incubation bout. We estimated the median incubation bout from the three previous (before-experimental) incubation bouts of the focal bird. We then defined the treated period as the time between the end of the control period and the release of the removed parent (Figure 2).
Nest attendance, defined as the proportion of time a bird was sitting on the nest, was derived from temperature data (Bulla, 2014; Bulla et al., 2014), except in one nest where temperature measurements failed. In this case, attendance was derived from RFID readings because temperature-based and RFID-based attendance highly correlate: r Pearson = 0.79, 95%CI: 0.76–0.82, N = 1,584 incubation bouts from 2011 (Bulla et al., 2013, 2014). Excluding this nest did not change the results. Note that temperature-based incubation is more accurate than RFID-based incubation as the RFID system sometimes fails to register an incubating parent (see Supporting Actograms in Bulla et al., 2016a).
Four nests were excluded from analyses of compensation because (a) a focal parent deserted the nest prior to treatment (one nest), (b) depredation (two nests), and (c) the wrong bird (the partner who just started its incubation bout) was removed (one nest). Thus, 25 experimental nests with 12 females and 13 males as focal parents were used in the analyses.
Captive Conditions
The removed parent was kept in a cardboard box (21 × 30 × 25 cm) in a shed which was sheltered from rain and wind (Figure S2b in Bulla, 2019). The size of the box was based on experience with keeping and transporting shorebirds and on a removal study using other shorebird species (Parra et al., 2014). The bottom of the box was lined with tundra (fresh for every bird) and contained fresh water and a feeding tray (Figure S2c in Bulla, 2019). The first eight removed birds were not fed for 12 h, and provided with food for the remaining 12 h; the remaining birds had access to food throughout (see the detailed Ethical statement in the Supporting information in Bulla, 2019).
Initially, the food consisted of 100 mealworms (~7.5 g) per 12 h. The energetic content that birds can metabolize from mealworms is ~24.2 kJ/g (Bell, 1990). Thus, ~7.5 g of worms provided ~181 kJ, which is 3–20 times more energy than the estimated daily energetic requirement of a semipalmated sandpiper during the incubation period (19–59 kJ/day; Ashkenazie and Safriel, 1979b; see also their Table 4 and their Figure 3 with higher estimates for other breeding stages such as egg laying), or nearly 50% more than the estimated energy expenditure during resting (123 kJ/day using Norton's (1973) equation for resting metabolic rate, assuming a 27 g bird, a median temperature of 6.2°C and assuming that an oxygen consumption of 1 L is the equivalent of 20.1 kJ). Note that the resting estimate (123 kJ) is from measurements in a closed system respirometer (Norton, 1973), while the other one (19–59 kJ) is based on energy uptake estimated by (a) using available data on diet composition, the energy content of prey items and their digestibility in combination with (b) observed feeding rates and estimated prey capture rates (Ashkenazie and Safriel, 1979b). Nevertheless, it turned out that this was not enough (the first two captive birds ate nearly all mealworms) and hence we adjusted the food amount and supplemented mealworms with cat food (for six birds, four of which ended up not eating it) or increased the amount of mealworms to 125–200 per 12 h (for all remaining birds). Except for one individual all birds had leftover mealworms in their tray at the end of the captive period (median = 11 mealworms, range: 0–106, N = 27 released parents, not 29 because 2 females died—see Ethical statement in the Supporting information in Bulla, 2019).
We checked and weighed each individual after capture, after 12 h and at the end of the 24-h captive period. The median mass difference between start and end of the captive period was −1.3 g (range: −3.1 to 0.3 g, N = 27 released parents). Note that here and elsewhere we use absolute mass difference because absolute and relative mass difference are strongly correlated (r Pearson = 0.99, 95%CI: 0.93–1.1, N = 27 released parents).
Statistical Analyses
Compensation for Absence of the Partner
We assessed whether and how the focal parent compensated for the absence of its partner by comparing nest attendance between the control and the treated period (Figure 2). In other words, compensation was defined as the ratio of total nest attendance time during the treated and the control period, expressed as percentage. Because parents rarely spend all of the time of an incubation bout attending the nest (Bulla et al., 2014), compensation values >100% are possible and indicate higher nest attendance during the treated than during the control period (“over-compensation”).
To test for the difference in nest attendance between control and treated period we used linear mixed-effect models with nest attendance as the dependent variable and period (control or treated) as a categorical predictor. To account for the paired (within-individual) design of the experiment, we included bird ID as a random intercept.
Nest attendance may differ depending on the length of the control or treated period (referred to as “period length”), but controlling for this period length did not improve the model fit; the model with period length was half as likely as the simple model (Table S1 in Bulla, 2019). Hence here, and in the subsequent analyses, we made inferences from the simpler model without period length.
Next we tested whether the amount of compensation was sex-specific by comparing a model with period (control or treated) in interaction with sex, with the initial model without sex (Table S1 in Bulla, 2019).
Explaining the Diversity in Compensation
To explore potential drivers of the diversity in compensation, we used linear models to test whether nest attendance during the treated period depended on (a) the time of day (defined as mid-point of the treated period, transformed to radians and represented by a sine and cosine), (b) median tundra temperature during the experimental bout (measured near the nest), (c) escape distance from the nest upon approach of a human, estimated as median escape distance of an individual prior to the experiment (see Bulla et al., 2016b), and (d) the proportion of time the focal bird was incubating before we removed its partner (estimated as median share of daily incubation, without exchange gaps, during 3 days prior to treatment).
We also assessed the relative importance of these four variables by comparing the four univariate models and a full model including tundra temperature, escape distance, and share of incubation (Table S2 in Bulla, 2019). We used “tundra temperature” instead of “time of day” in the full model, because both variables are correlated (rPearson = −0.56, 95%CI: −0.92 to −0.21, N = 25 nests; time is represented by sine of radians) and the univariate model with “tundra temperature” had a lower AICc-value than the model with “time of day.” We used nest attendance during the treated period (instead of compensation), because attendance correlated strongly with compensation (rPearson = 1, 95%CI: 0.96–1.02, N = 25 nests), and because the results are then directly comparable to those from the analysis of nest attendance during the control period and under natural conditions (Table S3 in Bulla, 2019). Note that time of day and tundra temperature were confounded by sex (Figure S4 in Bulla, 2019), because the timing of incubation differs between males and females (Bulla et al., 2014).
After-Experimental Effects
We explored how incubation changed after the removed parent returned to the nest (after-experimental period) by comparing—for each parent—nest attendance and the length of the last three before-experimental incubation bouts with nest attendance and the length of the first three after-experimental bouts. For these bouts we also compared the presence and length of exchange gaps. To this end, we constructed mixed-effect models with nest attendance (proportion), bout length (in hours), presence of exchange gap (binomial response; 0 = no gap, 1 = gap present), and length of exchange gap (in minutes) as separate response variables, and period (before or after the experiment) in interaction with day in the incubation period (day) as predictors. Day was mean-centered within each nest, so that negative values represent the before- and positive values the after-experimental period. To control for non-independence of data we entered bird ID as a random intercept and day as a random slope. We assessed the importance of the interaction and of the type of parent (focal vs. removed) by comparing models with and without the interaction, and with and without parent type (Tables S4 and S5 in Bulla, 2019). In addition, we explored (a) whether the after-experimental nest attendance and bout length of the removed parent were related to its absolute mass change while in captivity and (b) whether the after-experimental nest attendance and bout length of the focal parent were related to its level of compensation (proportion) during the treated period. We also investigated whether these relationships were sex specific. Bird ID was entered as a random intercept, and mass change or compensation as random slopes (Table S6 and S7 in Bulla, 2019).
General Procedures
R version 3.3.0 (R-Core-Team, 2016) was used for all statistical analyses and the “lme4” R package (Bates et al., 2015) for fitting the mixed-effect models. The models were fitted with maximum likelihood. We used the “sim” function from the “arm” R package and non-informative prior-distribution (Gelman and Hill, 2007; Gelman and Su, 2015) to create a sample of 2,000 simulated values for each model parameter (i.e., posterior distribution). We report effect sizes and model predictions by the medians, and the uncertainty of the estimates and predictions by the Bayesian 95% credible intervals (CI) represented by 2.5 and 97.5 percentiles from the posterior distribution of the 2,000 simulated or predicted values. We estimated the variance components with the “lmer” or “glmer” function from the “lme4” R package (Bates et al., 2015).
By necessity, the dependent variables varied more for the treated or the after-experimental period than for the control or before-experimental period. We controlled for this heteroscedasticity by scaling the dependent variable within period. However, because these models generated similar results as the simpler models and because the simpler models are on the original scale and hence easier to interpret, we report only the outcomes of the simpler models.
In all model comparisons we assessed the model fit by Akaike's Information Criterion corrected for sample size (AICc; Anderson, 2008) generated by the “AICc” function from the “AICcmodavg” R package (Mazerolle, 2016).
Results
Compensation for Absence of Parental Care
Typically, parents partially compensated for the absence of their partner's care (Figure 3A). The nest attendance (proportion of total time on the nest) in the treated period was on average 0.38 (95%CI: 0.27–0.49) lower than in the control period (Figure 3B; Table S1 in Bulla, 2019). This translates to a 59% (95%CI: 49–70%) compensation for the absence of the partner. The level of compensation was similar for females and males (Figure 3B, Table S1 in Bulla, 2019; the model containing sex in interaction with treatment was less likely than the model without this interaction).
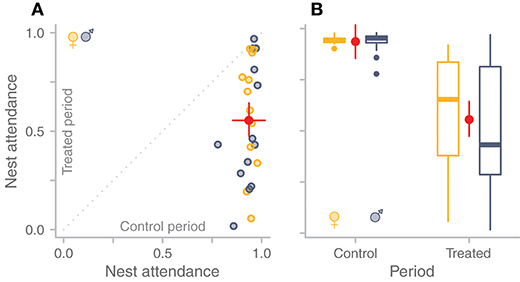
Figure 3. Compensation for the temporary absence of the partner. (A,B) The control period reflects the regular incubation bout of the focal parent, while the treated period is the period during which the focal parent's partner should have incubated, but was held in captivity. Yellow indicates female, blue-gray male. Red dots with bars indicate model predictions with 95%CI (Table S1 in Bulla, 2019). (A) Compensation by each individual (N = 25). The gray dashed line indicates full compensation. Each dot represents nest attendance of one individual; points below the line represent various degrees of partial compensation or no compensation (zero). (B) Nest attendance in the control and treated period for females (N = 12) and males (N = 13) separately. Box plots depict median (horizontal line inside the box), the 25th and 75th percentiles (box), the 25th and 75th percentiles ±1.5 times the interquartile range or the minimum/maximum value, whichever is smaller (bars), and the outliers (dots).
The compensation response of individual parents ranged from no to full compensation (2–101%, median = 57%; Figure 3A). Birds achieved similar levels of partial compensation using various “strategies” (Figure 4). Some individuals gradually decreased their nest attendance over the experimental period; some compensated fully for part of the experimental period, but then either reduced their nest attendance, left the nest completely unattended, or left the nest unattended but came back later. Remarkably, the individuals with nearly no compensation during the treated period simply returned to the nest at the expected time for their next incubation bout, that is, they continued their pre-experimental incubation routine (Figure 4, top row). In contrast, the parents that fully compensated left the nest unattended after continuously incubating for more than 24 h (Figure 4, panels in the two bottom rows).
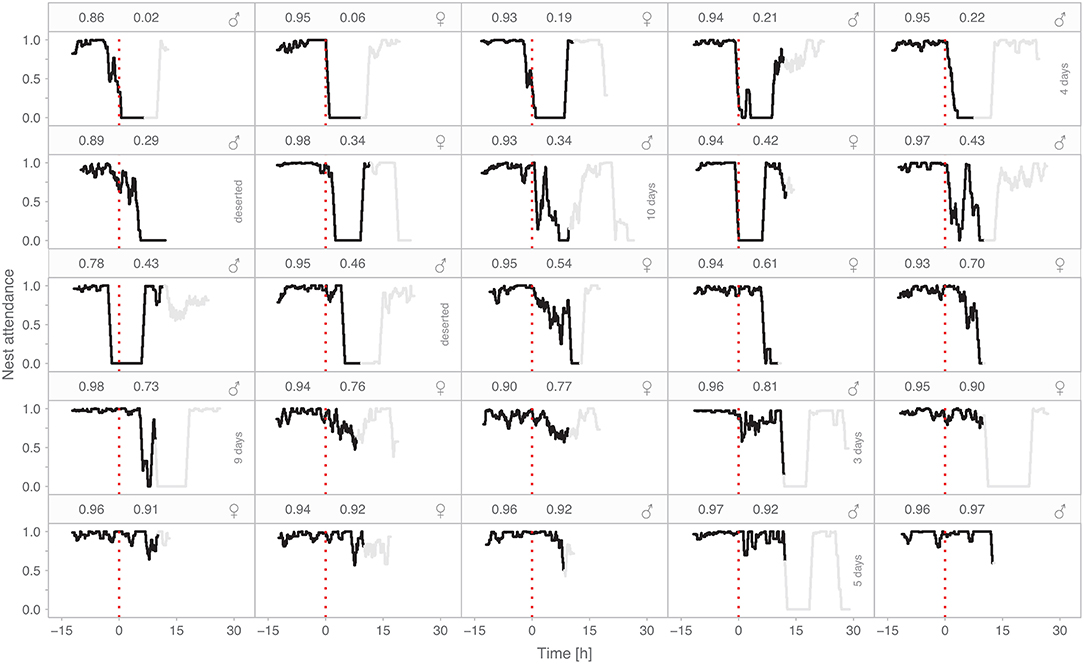
Figure 4. Diverse compensation responses by individual parents in the absence of their partner. Each panel represents one of 25 focal individuals. Panels are ordered according to nest attendance within the treated period such that the individual with the lowest nest attendance is in the top-left panel. Black lines show hourly nest attendance (proportion of time the parent is on the nest, depicted as a running hourly mean) during the experimental period (i.e., from the return of the focal parent until the release of the removed parent). The red dotted lines (time zero) indicate the end of the control period (i.e., the regular incubation bout of the focal parent; negative values) and the start of the treated period (compensation period, positive values). Gray lines indicate the hourly nest attendance of the focal bird from the moment the removed parent was released until it returned to the nest. In seven nests the removed parent never returned, so we show a maximum of 30 h after the start of the treated period and note whether the incubating parent deserted within this period, or for how many days the individual continued incubating uniparentally.
After releasing 23 parents from captivity, five parents (all females) never returned to their nest. An additional two removed females never returned, but we excluded their nests from the main analyses, because one was partially depredated during the incubation bout prior to removal, while in the other the focal bird (male) had already deserted the nest before the treated period started.
Widowed males continued incubating for another 0–10 days (median = 4 days, N = 7, 5 females that never returned and two that died). The males then deserted the nest (N = 6, in one of these nests only three eggs remained, so one egg might have hatched) or the nest was depredated (N = 1).
Explaining the Diversity in Compensation
During undisturbed situations (the before-experimental period or non-experimental nests), nest attendance slightly decreased during the warmer part of the day (blue-gray and green in Figure 5A). However, parents that compensated for the absence of their partner during the warmer part of the day tended to have higher nest attendance (yellow in Figure 5A). Indeed, nest attendance of compensating parents increased with increasing median tundra temperature during the compensation period (Figure 5B). Compensation seemed unrelated to the focal parent's share of incubation (proportion) during the before-treatment period (Figure 5C), but there was a tendency for parents with long escape distance (i.e., parents that may have been more sensitive to disturbance) to compensate more (Figure 5D). The models with temperature had the greatest support of the four models (see Table S2 in Bulla, 2019).
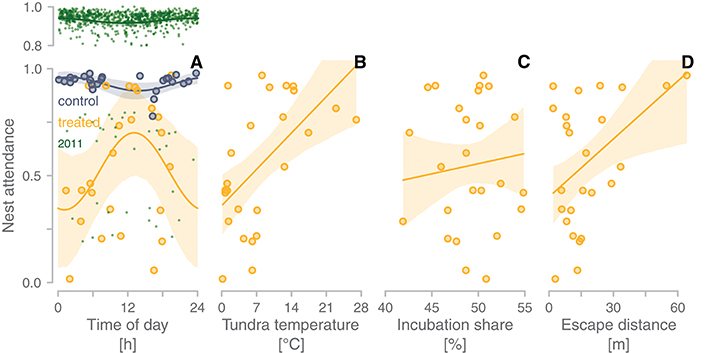
Figure 5. Correlates of compensation for the absence of the partner. (A–D) Relationships between nest attendance (proportion of the total time the bird is on the nest) during the time the removed partner would have incubated (treated period) and the mid-time of this treated period (A), the median tundra temperature at the nest during the treated period (B), the focal parent's share of incubation during the before-experimental period (C), and its median escape distance from the nest prior to the experiment (D). Yellow dots represent individual observations (N = 25); yellow lines with yellow-shaded areas indicate model predictions with 95%CI for the treated period (Table S2 in Bulla, 2019) from a univariate model (A) and from a model containing temperature, incubation share and escape distance (B–D; the effects of other predictors were kept constant). (A) To emphasize how the relationship of nest attendance with time of day differs between the treated period and the natural, undisturbed situation, we added the observations and predictions from the control period (i.e., the regular incubation bout of the focal parent; blue-gray) and from non-experimental nests from the 2011 breeding season (green, to avoid cluttering data between 0.8-1.0 are shown in a separate, upper panel; Table S3 in Bulla, 2019; the 2011 data come from Bulla et al., 2013, 2014). (D) Note that escape distance estimates were unrelated to the number of times we visited the nest (i.e., how often the focal parent had seen us prior to the experiment; linear model estimate = −0.8 m, 95%CI: −5 to 4 m) and that the number of visits also did not explain variation in the level of compensation (1%, 95%CI: −7 to 9%). Also, the time when we trapped the focal parent was unrelated to compensation (Figure S5 in Bulla, 2019).
After-Experimental Effects
In the 18 nests where the removed parent returned to incubate, parents differed markedly in how long it took them to return after we released them from captivity: median (range) = 7.36 h (0.26–16.85 h). The overall quality of incubation during the after-experimental period was lower than during the before-experimental period (Figure 6; Tables S4 and S5 in Bulla, 2019): nest attendance was lower, incubation bouts were shorter and exchange gaps, although they did not occur more frequently, were longer (Figure 6A). Despite the different treatments, these effects were similar for focal and removed parent, and they did not differ between males and females (Figure 6B, Tables S4 and S5 in Bulla, 2019). However, parents seemed to recover from the effect of the treatment, because nest attendance tended to increase, bouts became longer, and gaps shorter with days after the experimental period (Figure 6C). During the after-experimental period, nest attendance tended to be lower and incubation bouts shorter in males (but not in females) that lost more mass while in captivity (Figure 7A, Table S6 in Bulla, 2019). Although the level of compensation seemed unrelated to nest attendance in after-experimental bouts, females (but not males) that compensated more tended to have shorter bouts (Figure 7B, Table S7 in Bulla, 2019).
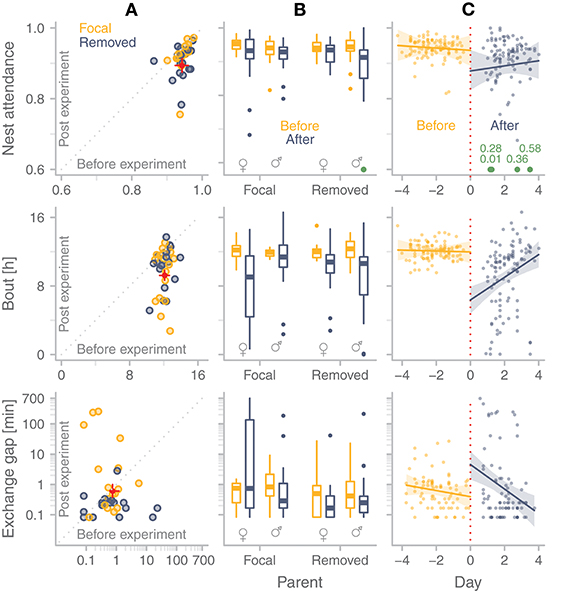
Figure 6. Differences in quality of incubation before and after the experimental period. (A) Median nest attendance, median bout length, and median non-zero exchange gap duration for each individual in the period before and after the experiment. Dots represent medians for focal parents (yellow) and for removed parents (blue-gray; Nattendance and Nbout = 36 individuals with before and after-treatment data, Ngap = 33 individuals with before and after-treatment gaps, 3 individuals had no gaps either in before, in after-treatment or in both). Red dots with bars indicate model predictions with 95%CI (Tables S4, S5, “simple model” in Bulla, 2019). (B) Comparison of nest attendance, bout length, and exchange-gap length between the period before (yellow) and after (blue-gray) the experiment for the focal and the removed parent and for each sex separately (Nattendance and Nbout = 214 bouts, Ngap = 164 exchange gaps). Box plots depict median (horizontal line inside the box), the 25th and 75th percentiles (box), the 25th and 75th percentiles ±1.5 times the interquartile range or the minimum/maximum value, whichever is smaller (bars), and the outliers (dots). For nest attendance, the green dot represents four outliers (described in C). (C) Temporal changes in nest attendance, bout or gap length of focal and removed individuals (combined) in the period before (yellow, negative values) and after the experiment (blue-gray, positive values). Dots represent individual observations and lines with shaded areas indicate model predictions with 95%CI (Tables S4, S5, “day model” in Bulla, 2019). See (B) for sample sizes. The red dotted line indicates the day when one of the parents was removed. In the nest attendance graph, four values are outside the range of the y-axis; these are indicated in green with their actual nest attendance value. In case of nest attendance, the model including day was slightly less supported than the simple model. In case of bout length and exchange gap duration, the model including day was much more supported than the simple model. For exchange-gap duration, the model that also contained parent type (focal or removed) was even more likely (Tables S4, S5 in Bulla, 2019).
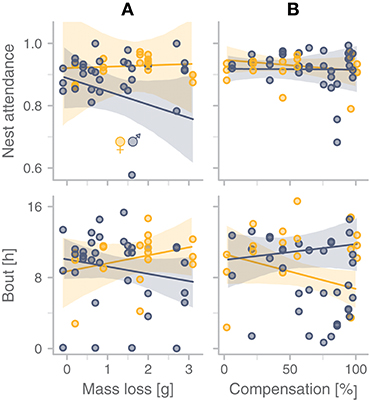
Figure 7. Predictors of nest attendance and bout length during the after-experimental period. (A) Relationship between mass loss of the removed parent while in captivity and its nest attendance and bout length during the post-experimental period. (B) Relationship between the amount of compensation by the focal parent during the time the removed partner would have incubated (treated period) and the focal parent's nest attendance and bout length during the after-experimental period. (A,B) Yellow indicates females, blue-gray males; dots represent individual observations and lines with shaded areas indicate model predictions with 95%CI. In all four cases the model with sex fitted the data better (Tables S6, S7 in Bulla, 2019).
Discussion
Diverse Compensation
Our results indicate that semipalmated sandpipers on average partially compensated for the temporal absence of care from their partner, which seems in line with the general prediction of partial compensation from established parental care models (Houston and Davies, 1985; McNamara et al., 1999, 2003). However, parents varied greatly in how they responded (Figure 4): some parents did not compensate at all, some compensated partially, and some fully. We discuss three possible explanations for this diversity, which contradicts the full or no compensation prediction of the incubation model (Jones et al., 2002).
First, the variation in compensation may reflect variation in how parents value their nest. Semipalmated sandpipers have one nesting attempt per breeding season and early nests may have higher chances to succeed (Hicklin and Gratto-Trevor, 2010). Also, if the nest fails, parents from early nests have a higher probability of re-nesting than parents from nests initiated later (Hicklin and Gratto-Trevor, 2010). Thus, the level of compensation may reflect nest initiation date. However, nest attendance (level of compensation) was unrelated to nest initiation date (−0.3% per day; 95%CI: from −3 to 3% per day, N = 25; Figure S5c in Bulla, 2019).
Second, in semipalmated sandpipers, and in many other biparentally incubating shorebirds, the contribution of both parents is thought to be essential for successful incubation (Poole, 2005). Thus, according to theory, parents faced with a temporary absence of their partner should either fully compensate or desert the nest (Jones et al., 2002). However, a reduction in incubation effort may not always lead to complete loss of the breeding attempt. For example, an individual may reduce the length of its incubation bouts, and the partner may either incubate longer or the eggs may be left uncovered for longer periods than previously. Even when a parent completely deserts, a single parent might successfully incubate a clutch, e.g., during warmer periods, near the end of the incubation period, or when a parent has larger energy reserves (Bulla et al., 2017). This means that there might be some room for one parent to exploit the investment of the other parent. Indeed, one permanently widowed parent incubated uniparentally for 10 days (see Actograms in Bulla, 2017) and some non-experimental nests hatched after 14 days of uniparental incubation (Bulla et al., 2017). Thus, the varying circumstances among nests (e.g., date, parental condition) could translate into various compensation levels.
Third and alternatively, semipalmated sandpiper parents may always attempt to compensate fully, but sometimes fail to do so, (a) because their energy stores get depleted, (b) because they are less willing to risk their own survival, or (c) because they are less aware of or responsive to the absence of their partner at the nest. We explore these possibilities.
(a) Parents of biparental species with continuous incubation (i.e., with close to 100% nest attendance) do not feed while incubating (Chaurand and Weimerskirch, 1994; Weimerskirch, 1995; Dearborn, 2001; Bulla et al., 2014, 2015b); thus, a single parent is unable to incubate continuously (with high nest attendance) for many days, because it has to eat. This implies that full compensation is only possible as long as the energetic reserves last. We find some support for this explanation. As we demonstrate, even the parents that compensated fully left the nest unattended after some time (Figure 4), that is, they did not (could not) continue incubating for three “typical” incubation bouts. Thus, the fully compensating parents differed from the not- or partially-compensating parents by “deserting” the nest considerably later. Further evidence comes from the observation that parents that were treated during the warmer part of a day (when incubation is presumably less energetically demanding) tended to compensate more (i.e., had higher nest attendance) than parents treated during the colder part of a day. This contrasts with the typical nest attendance patterns when both parents are present (Figure 5A), as well as with nest attendance patterns of uniparentally incubating species (Cartar and Montgomerie, 1985; Løfaldli, 1985; Reneerkens et al., 2011). In both these cases, nest attendance drops during the warmer part of a day, probably because the eggs cool down slower and because food availability and hence foraging efficiency is higher. Thus, our results are consistent with the idea that the compensating parents might have tried but could not compensate fully when it was cold. Although parents likely decide to stay or leave the nest based on their energy stores and nutritional status, they may also respond to other cues, e.g., related to the past behavior or other qualities of their partner. Specifically, they can respond to whether the partner was present on the nest or not. They can also communicate (“negotiate”) about future investment during the exchange on the nest.
(b) The level of compensation may result from how long a parent waited for its partner to return—i.e., variation in resolving the trade-off between the risk of increased adult mortality and the risk of breeding failure. Contrary to the expectation, we found that individuals with a shorter escape distance—those maximizing egg protection while risking own mortality—tended to compensate less during the experimental period (Figure 5D).
(c) An alternative idea is that the level of compensation depends solely on the perceived absence of the partner's nest attendance. Unlike chick feeding, where parents can feed simultaneously, incubation is a mutually exclusive behavior, because only a single parent can incubate at a time. Hence, a parent cannot increase its share of incubation without communicating with its partner. However, the off-nest parent is often far away from the nest, clearly out of hearing range of its partner (Bulla et al., 2015b). Also, observations show that the incubating parent sometimes leaves the nest before its partner returns to incubate (Ashkenazie and Safriel, 1979b; Bulla et al., 2014, 2015b, 2017); thus, the incubating parent may “assume” that its mate will return and continue incubation. As a result, variation in compensation may be related to variation in two behaviors. First, variation in compensation may reflect how often or how soon the partner checked its nest. Our experimental design controlled for this possibility, but we know that off-duty parents rarely come to the vicinity of the nest, unless they attempt an exchange (Bulla et al., 2015b). Second, variation in compensation may reflect how long it took a parent to realize its partner is absent—“responsiveness.” Indeed, some permanently widowed parents continued their typical incubation schedule for several days, leaving the nest unattended during their partner's supposed bout, before changing to a uniparental incubation pattern with constant nest attendance at “night” (cold part of the day) and lower nest attendance during the “day” when temperatures are higher (Bulla et al., 2017). This suggests that it took some time before the parent realized that its partner had deserted or at least before it responded to the desertion (see Actograms in Bulla, 2017). Accordingly, we found that parents with long escape distance compensated more than those with short escape distance (Figure 5D), suggesting that parents that leave the nest early upon human approach may be more responsive to what is happening in their environment and realize earlier that their partner is absent.
Energetic constraints and responsiveness may well act together. Thus, those parents that are responsive to the absence of their partner, and have the resources to wait for their partner's delayed return, may do so, whereas parents that are less responsive or do not have the resources for full compensation, may compensate partially or not at all. Such an explanation is in line with predictions of parental care models: parents should vary in their compensation response based on the likelihood of brood failure in the absence of care, the parent's current condition and their knowledge about (or—as we suggest—their responsiveness to) their partner's condition or the need of the brood (Jones et al., 2002; Johnstone and Hinde, 2006). In this case, the need of the brood can be translated to the risk of temperature-related embryo death (or developmental problems affecting future fitness) or the risk of clutch predation.
Nest Desertion
After release from captivity, 5 out of 23 released parents never returned to incubate. In all cases, the non-returning parent was the female of the pair, which is similar to what has been shown in northern flickers, Colaptes auratus (Wiebe, 2010). Females might be more sensitive to stress, because they already laid the eggs (typically, a four-egg clutch is laid in 5 days and has a similar total mass as an average female; Hicklin and Gratto-Trevor, 2010). In semipalmated sandpipers, females also tend to desert the brood before or after hatching (Hicklin and Gratto-Trevor, 2010; Bulla et al., 2017). However, we found no marked differences between females and males in the level of compensation during the partner's absence (Figure 3), or in post-experimental quality of incubation—be it for the focal or the returned individuals (Figure 6).
After-Experimental Effects
After we released the removed parent the quality of incubation was lower than before the experiment, but it improved quickly with time (Figure 6); already 3 days after the experiment, parents seemed to incubate as usual. These after-experimental effects were generally similar for the focal and the removed parent (Figure 6B), suggesting that the stress caused by the absence of the partner (including the compensation) might have been similar to the stress of captivity. An alternative explanation for the lower incubation quality in the after-experimental period is that parents needed to “renegotiate” how much they invest, or realign their incubation schedules. In either case, the rapid return to “normal” quality of incubation suggests that parents compensated fully. In other words, the focal bird resumed its typical incubation bouts after the partner returned and did not “retaliate” against its removed partner by reducing the length of its subsequent bouts.
Mass loss of the removed parent during captivity and the amount of compensation of the focal parent during the experiment were poor predictors of the after-treatment incubation behavior (Figure 7). This suggests that the after-experimental effects are not related to energetic constraints, confirming earlier work (Bulla et al., 2015a, 2016b) and that the parents are tolerant of short-term irregularities or reductions in their mate's parental care. In accordance with previous work (Wiebe, 2010), biparental incubators seem willing to “forgive” and fully compensate a missed or irregular incubation bout of their partner. This seems an adaptive strategy to facilitate survival of the clutch.
Conclusions and Suggestions for Further Work
Our finding that biparentally incubating shorebirds on average partially compensate for the temporal absence of their partner corroborates the predictions of established models (Houston and Davies, 1985; McNamara et al., 1999, 2003) and results of a meta-analysis (Harrison et al., 2009). However, individual responses were highly diverse, from no to full compensation, possibly depending on environmental factors such as ambient temperature (Figure 5B) and food availability or on the “responsiveness” of parents to the absence of their partner (Figure 5D). Whether the diversity of compensation responses during incubation represents noise around the mean or biologically relevant diversity—possibly shaped by energetic constraints and parental “responsiveness”—awaits future empirical investigation. We speculate that all individuals attempted full compensation, but that some failed because their energy stores became depleted, or because they were less “responsive” to the absence of their partner.
If (full) compensation is energetically constrained, then supplemental feeding or heating the eggs of the focal parent (see Bulla et al., 2015a) should lead to full compensation in all individuals, or at least to reduced individual variation in the level of compensation. Note, however, that under regular biparental incubation, saving energy for the incubating parent by experimentally heating the eggs or insulating the nest did not change the length of incubation bouts (Bulla et al., 2015a). On the other hand, if parental responsiveness drives the level of compensation, and if this is an individual-specific trait, then the level of compensation should be repeatable.
Our study also reveals that regardless of the immediate response to the absence of the partner, the focal individuals did not “retaliate” after the removed parent returned and continued incubating as usual.
Ethics Statement
All field procedures were performed in accordance with the relevant guidelines and regulations, and approved by the U.S. Department of the Interior, U.S. Fish and Wildlife Service, and State of Alaska Department of Fish and Game (permit no. 23520, MB210494-2, 13-122). The access to Iñupiat lands in Barrow vicinity was granted by 2013 Arctic Science & Wildlife Research Land Use Permits from UIC Science. For further details see detailed Ethical statement in Supporting information (Bulla, 2019).
Author Contributions
MB and BK conceived the study. MB with help of AR collected the data. MB and AR managed the database. MB analyzed the data with help from MV and input from BK. MB and BK wrote the manuscript. All authors contributed to the final paper. MB with BK revised the paper.
Funding
This work was supported by the Max Planck Society (to BK). MB finished this work while supported by an EU Horizon 2020 Marie Curie individual fellowship (4231.1 SocialJetLag) at NIOZ Royal Netherlands Institute for Sea Research, and by the Czech University of Life Sciences (CIGA: 2018421).
Conflict of Interest Statement
The authors declare that the research was conducted in the absence of any commercial or financial relationships that could be construed as a potential conflict of interest.
Acknowledgments
We thank F. Heim, L. Verlinden, and M. Schneider for help in the field, M. Hanson and E. Burnet from UMIAQ and R. Lanctot for assistance with logistics, A. Girg for the genetic sexing, F. Korner-Nievergelt, and Y. Araya for advice on the statistical analyses, and D. Starr-Glass, B. Bulla, P. B. D'Amelio, E. Schlicht, Paul A. Smith, K. Wiebe, and Á. Pogány for constructive comments on the manuscript. MB thanks Bare and Maje for patience and support. MB did this work as a PhD student in the International Max Planck Research School for Organismal Biology. The pre-print of this work is available from bioRxiv https://doi.org/10.1101/117036.
References
Alonso-Alvarez, C. (2001). Effects of testosterone implants on pair behaviour during incubation in the Yellow-legged Gull Larus cachinnans. J. Avian Biol. 32, 326–332. doi: 10.1111/j.0908-8857.2001.320406.x
AlRashidi, M., Kosztolányi, A., Shobrak, M., Küpper, C., and Székely, T. (2011). Parental cooperation in an extreme hot environment: natural behaviour and experimental evidence. Anim. Behav. 82, 235–243. doi: 10.1016/j.anbehav.2011.04.019
Anderson, D. R. (2008). Model Based Inference in the Life Sciences: A Primer on Evidence. New York, NY: Springer.
Ashkenazie, S., and Safriel, U. N. (1979a). Breeding cycle and behavior of the semipalmated sandpiper at barrow, Alaska. Auk 96, 56–67. doi: 10.2307/4085399
Ashkenazie, S., and Safriel, U. N. (1979b). Time-energy budget of the semipalmated sandpiper Calidris Pusilla at Barrow, Alaska. Ecology 60, 783–799. doi: 10.2307/1936615
Bates, D., Maechler, M., Bolker, B., and Walker, S. (2015). Fitting linear mixed-effects models using lme4. J. Stat. Softw. 67, 1–48. doi: 10.18637/jss.v067.i01
Bell, G. P. (1990). Birds and mammals on an insect diet: a primer on diet composition analysis in relation to ecological energetics. Stud. Avian Biol. 13, 416–422.
Blanken, M. S., and Nol, E. (1998). Factors affecting parental behavior in semipalmated plovers. Auk 115, 166–174.
Bowman, R., and Bird, D. M. (1987). Behavioral strategies of American kestrels during mate replacement. Behav. Ecol. Sociobiol. 20, 129–135. doi: 10.1007/bf00572635
Brunton, D. H. (1988). Sexual differences in reproductive effort: time-activity budgets of monogamous killdeer, Charadrius vociferus. Anim. Behav. 36, 705–717. doi: 10.1016/s0003-3472(88)80153-2
Bulla, M. (2014). R-SCRIPT and EXAMPLE DATA to extract incubation from temperature measurements. Figshare 18:50. doi: 10.6084/m9.figshare.1037545.v1
Bulla, M. (2017). Supporting information for ‘Flexible parental care: uniparental incubation in biparentally incubating shorebirds'. Open Science Framework. doi: 10.17605/OSF.IO/3RSNY
Bulla, M. (2019). Supporting information for 'Temporary mate removal during incubation leads to variable compensation in a biparental shorebird'. Open Science Framework. https://osf.io/mx82q/
Bulla, M., Cresswell, W., Rutten, A. L., Valcu, M., and Kempenaers, B. (2015a). Biparental incubation-scheduling: no experimental evidence for major energetic constraints. Behav. Ecol. 26, 30–37. doi: 10.1093/beheco/aru156
Bulla, M., Prüter, H., Vitnerová, H., Tijsen, W., Sládeček, M., Alves, J. A., et al. (2017). Flexible parental care: uniparental incubation in biparentally incubating shorebirds. Sci. Rep. 7:1. doi: 10.1038/s41598-017-13005-y
Bulla, M., Stich, E., Valcu, M., and Kempenaers, B. (2015b). Off-nest behaviour in a biparentally incubating shorebird varies with sex, time of day and weather. Ibis 157, 575–589. doi: 10.1111/ibi.12276
Bulla, M., Valcu, M., Dokter, A. M., Dondua, A. G., Kosztolányi, A., Rutten, A., et al. (2016a). Supporting Information for 'Unexpected diversity in socially synchronized rhythms of shorebirds'. Open Science Framework. doi: 10.17605/OSF.IO/WXUFM
Bulla, M., Valcu, M., Dokter, A. M., Dondua, A. G., Kosztolányi, A., Rutten, A., et al. (2016b). Unexpected diversity in socially synchronized rhythms of shorebirds. Nature. 540, 109–113. doi: 10.1038/nature20563
Bulla, M., Valcu, M., Rutten, A. L., and Kempenaers, B. (2013). Data from: biparental incubation patterns in a high-Arctic breeding shorebird: how do pairs divide their duties? Dryad Data Reposit. 25, 152–164. doi: 10.5061/dryad.nh8f0
Bulla, M., Valcu, M., Rutten, A. L., and Kempenaers, B. (2014). Biparental incubation patterns in a high-Arctic breeding shorebird: how do pairs divide their duties? Behav. Ecol. 25, 152–164. doi: 10.1093/beheco/art098
Burley, N. (1980). Clutch overlap and clutch size: alternative and complementary reproductive tactics. Am. Natur. 59, 223–246.
Cartar, R. V., and Montgomerie, R. D. (1985). The influence of weather on incubation scheduling of the white-rumped sandpiper (Calidris fuscicollis): a uniparental incubator in a cold environment. Behaviour 95, 261–289.
Chalfoun, A. D., and Martin, T. E. (2007). Latitudinal variation in avian incubation attentiveness and a test of the food limitation hypothesis. Anim. Behav. 73, 579–585. doi: 10.1016/j.anbehav.2006.09.010
Chaurand, T., and Weimerskirch, H. (1994). Incubation routine, body mass regulation and egg neglect in the Blue Petrel Halobaena caerulea. Ibis 136, 285–290. doi: 10.1111/j.1474-919X.1994.tb01097.x
Coppens, C. M., de Boer, S. F., and Koolhaas, J. M. (2010). Coping styles and behavioural flexibility: towards underlying mechanisms. Philos. Trans. R. Soc. Lond. B Biol. Sci. 365, 4021–4028. doi: 10.1098/rstb.2010.0217
de Heij, M. E., van den Hout, P. J., and Tinbergen, J. M. (2006). Fitness cost of incubation in great tits (Parus major) is related to clutch size. Proc. Biol. Sci. 273, 2353–2361. doi: 10.1098/rspb.2006.3584
De Ridder, E., Pinxten, R., and Eens, M. (2000). Experimental evidence of a testosterone-induced shift from paternal to mating behaviour in a facultatively polygynous songbird. Behav. Ecol. Sociobiol. 49, 24–30. doi: 10.1007/s002650000266
Dearborn, D. C. (2001). Body condition and retaliation in the parental effort decisions of incubating great frigatebirds (Fregata minor). Behav. Ecol. 12, 200–206. doi: 10.1093/beheco/12.2.200
Deeming, D. C. (2002). “Behaviour patterns during incubation,” in Avian Incubation, Behaviour, Environment and Evolution, ed D. C. Deeming (Oxford: Oxford University Press), 63–87.
Duckworth, J. W. (1992). Effects of mate removal on the behaviour and reproductive success of Reed Warblers Acrocephalus scirpaceus. Ibis 134, 164–170. doi: 10.1111/j.1474-919X.1992.tb08393.x
Erckmann, W. J. (1981). The Evolution of Sex-Role Reversal and Monogamy in Shorebirds. PhD Univ. Washington.
Gauthier-Clerc, M., Le Maho, Y., Gendner, J.-P., Durant, J., and Handrich, Y. (2001). State-dependent decisions in long-term fasting king penguins, Aptenodytes patagonicus, during courtship and incubation. Anim. Behav. 62, 661–669. doi: 10.1006/anbe.2001.1803
Gelman, A., and Hill, J. (2007). Data Analysis Using Regression and Multilevel/Hierarchical Models. Cambridge: Cambridge University Press.
Gelman, A., and Su, Y.-S. (2015). Arm: Data Analysis Using Regression and Multilevel/Hierarchical Models. Version 1.8-6. Available online at: http://CRAN.R-project.org/package=arm
Gibbon, J., Morrell, M., and Silver, R. (1984). Two kinds of timing in circadian incubation rhythm of ring doves. Am. J. Physiol. Regulat. Integr. Compar. Physiol. 247, R1083–R1087.
Grant, S. G. (1982). Avian Incubation: Egg Temperature, Nest Humidity, and Behavioral Thermoregulation in a Hot Environment. Washington, DC: The American Ornithologists' Union.
Hanssen, S. A., Hasselquist, D., Folstad, I., and Erikstad, K. E. (2005). Cost of reproduction in a long-lived bird: incubation effort reduces immune function and future reproduction. Proc. R. Soc. B Biol. Sci. 272, 1039–1046. doi: 10.1098/rspb.2005.3057
Harrison, F., Barta, Z., Cuthill, I., and Székely, T. (2009). How is sexual conflict over parental care resolved? A meta-analysis. J. Evol. Biol. 22, 1800–1812. doi: 10.1111/j.1420-9101.2009.01792.x
Heaney, V., and Monaghan, P. (1996). Optimal allocation of effort between reproductive phases: the trade-off between incubation costs and subsequent brood rearing capacity. Proc. Biol. Sci. 263, 1719–1724.
Hicklin, P., and Gratto-Trevor, C. L. (2010). “Semipalmated Sandpiper (Calidris pusilla),” in The Birds of North America Online, ed A. Poole (Ithaca: Cornell Lab of Ornithology).
Honaker, J., King, G., and Blackwell, M. (2011). Amelia II: a program for missing data. J. Stat. Softw. 45, 1–47. doi: 10.18637/jss.v045.i07
Houston, A. I., and Davies, N. B. (1985). “The evolution of cooperation and life history in the dunnock Prunella modularis,” in Behavioural Ecology: Ecological Consequences of Adaptive Behaviour, eds R. M. Sibly and R. H. Smith (Oxford: Blackwell Scientific Publications), 471–487.
Johnstone, R. A., and Hinde, C. A. (2006). Negotiation over offspring care—how should parents respond to each other's efforts? Behav. Ecol. 17, 818–827. doi: 10.1093/beheco/arl009
Johnstone, R. M., and Davis, L. S. (1990). Incubation routines and foraging-trip regulation in the gray-faced Petrel Pterodroma macroptera gouldi. Ibis 132, 14–20. doi: 10.1111/j.1474-919X.1990.tb01011.x
Jones, K. M., Ruxton, G. D., and Monaghan, P. (2002). Model parents: is full compensation for reduced partner nest attendance compatible with stable biparental care? Behav. Ecol. 13, 838–843. doi: 10.1093/beheco/13.6.838
Kersten, M., and Piersma, T. (1986). High levels of energy expenditure in shorebirds: metabolic adaptations to an energetically expensive way of life. Ardea 75, 175–188. doi: 10.5253/arde.v75.p175
Koolhaas, J., Korte, S., De Boer, S., Van Der Vegt, B., Van Reenen, C., Hopster, H., et al. (1999). Coping styles in animals: current status in behavior and stress-physiology. Neurosci. Biobehav. Rev. 23, 925–935. doi: 10.1016/S0149-7634(99)00026-3
Kosztolanyi, A., Cuthill, I. C., and Szekely, T. (2009). Negotiation between parents over care: reversible compensation during incubation. Behav. Ecol. 20, 446–452. doi: 10.1093/beheco/arn140
Kosztolányi, A., Székely, T., and Cuthill, I. C. (2003). Why do both parents incubate in the Kentish plover? Ethology 109, 645–658. doi: 10.1046/j.1439-0310.2003.00906.x
Lessells, C. M. (2012). “Sexual conflict,” in The Evolution of Parental Care, eds J. A. Royle, P. T. Smiseth, and M. Kölliker (Oxford: Oxford University Press), 150–170.
Liebezeit, J. R., Smith, P. A., Lanctot, R. B., Schekkerman, H., Tulp, I., Kendall, S. J., et al. (2007). Assessing the development of shorebird eggs using the flotation method: species-specific and generalized regression models. Condor 109, 32–47. doi: 10.1650/0010-5422(2007)109[32:atdose]2.0.co;2
Løfaldli, L. (1985). Incubation rhythm in the great snipe Gallinago media. Ecography 8, 107–112. doi: 10.1111/j.1600-0587.1985.tb01160.x
Mazerolle, M. J. (2016). AICcmodavg: Model Selection and Multimodel Inference Based on (Q)AIC(c). Version 2.0-4. Available online at: http://CRAN.R-project.org/package=AICcmodavg
McDonald, P. G., Buttemer, W. A., and Astheimer, L. B. (2001). The influence of testosterone on territorial defence and parental behavior in male free-living rufous whistlers, Pachycephala rufiventris. Hormones Behav. 39, 185–194. doi: 10.1006/hbeh.2001.1644
McNamara, J. M., Gasson, C. E., and Houston, A. I. (1999). Incorporating rules for responding into evolutionary games. Nature 401, 368–371.
McNamara, J. M., Houston, A. I., Barta, Z., and Osorno, J.-L. (2003). Should young ever be better off with one parent than with two? Behav. Ecol. 14, 301–310. doi: 10.1093/beheco/14.3.301
Monaghan, P., and Nager, R. G. (1997). Why don't birds lay more eggs? Trends Ecol. Evol. 12, 270–274.
Nakagawa, S., and Freckleton, R. P. (2011). Model averaging, missing data and multiple imputation: a case study for behavioural ecology. Behav. Ecol. Sociobiol. 65, 103–116. doi: 10.1007/s00265-010-1044-7
Nord, A., Sandell, M. I., and Nilsson, J.-Å. (2010). Female zebra finches compromise clutch temperature in energetically demanding incubation conditions. Funct. Ecol. 24, 1031–1036. doi: 10.1111/j.1365-2435.2010.01719.x
Norton, D. (1973). Ecological Energetics of Calidridine Sandpipers Breeding in Northern Alaska. Doctor of Philosophy, University of Alaska.
Parra, J. E., Beltrán, M., Zefania, S., Dos Remedios, N., and Székely, T. (2014). Experimental assessment of mating opportunities in three shorebird species. Anim. Behav. 90, 83–90. doi: 10.1016/j.anbehav.2013.12.030
Pinxten, R., Eens, M., and Verheyen, R. F. (1995). Response of male European starlings to experimental removal of their mate during different stages of the breeding cycle. Behaviour 132, 301–317.
Poole, A. (2005). “The birds of North America online,” ed A. Poole. (Ithaca, NY: Cornell Laboratory of Ornithology).
R-Core-Team (2016). R: A Language and Environment for Statistical Computing. R Foundation for Statistical Computing. R version 3.3.0. Available online at: http://www.R-project.org/
Reneerkens, J., Grond, K., Schekkerman, H., Tulp, I., and Piersma, T. (2011). Do uniparental Sanderlings Calidris alba increase egg heat input to compensate for low nest attentiveness? PLoS ONE 6:e16834. doi: 10.1371/journal.pone.0016834
Schwagmeyer, P. L., Schwabl, H. G., and Mock, D. W. (2005). Dynamics of biparental care in house sparrows: hormonal manipulations of paternal contributions. Anim. Behav. 69, 481–488. doi: 10.1016/j.anbehav.2004.04.017
Smith, P. A., Tulp, I., Schekkerman, H., Gilchrist, H. G., and Forbes, M. R. (2012). Shorebird incubation behaviour and its influence on the risk of nest predation. Anim. Behav. 84, 835–842. doi: 10.1016/j.anbehav.2012.07.004
Trivers, R. (1972). “Parental investment and sexual selection,” in Sexual Selection and the Descent of Man, ed B. Cambell (Chicago: Aldine Press), 136–179.
Weimerskirch, H. (1995). Regulation of foraging trips and incubation routine in male and female wandering albatrosses. Oecologia 102, 37–43. doi: 10.1007/bf00333308
Wiebe, K. L. (2008). Division of labour during incubation in a woodpecker Colaptes auratus with reversed sex roles and facultative polyandry. Ibis 150, 115–124. doi: 10.1111/j.1474-919X.2007.00754.x
Wiebe, K. L. (2010). Negotiation of parental care when the stakes are high: experimental handicapping of one partner during incubation leads to short-term generosity. J. Anim. Ecol. 79, 63–70. doi: 10.1111/j.1365-2656.2009.01614.x
Keywords: biparental incubation, compensation, cooperation, mate removal, nest attendance, nest desertion, parental effort, shorebirds
Citation: Bulla M, Valcu M, Rutten AL and Kempenaers B (2019) Temporary Mate Removal During Incubation Leads to Variable Compensation in a Biparental Shorebird. Front. Ecol. Evol. 7:93. doi: 10.3389/fevo.2019.00093
Received: 10 October 2018; Accepted: 11 March 2019;
Published: 12 April 2019.
Edited by:
James Luke Savage, University of Cambridge, United KingdomReviewed by:
Karen Wiebe, University of Saskatchewan, CanadaÁkos Pogány, Eötvös Loránd University, Hungary
Copyright © 2019 Bulla, Valcu, Rutten and Kempenaers. This is an open-access article distributed under the terms of the Creative Commons Attribution License (CC BY). The use, distribution or reproduction in other forums is permitted, provided the original author(s) and the copyright owner(s) are credited and that the original publication in this journal is cited, in accordance with accepted academic practice. No use, distribution or reproduction is permitted which does not comply with these terms.
*Correspondence: Martin Bulla, YnVsbGEubWFyQGdtYWlsLmNvbQ==
†Present Address: Martin Bulla, NIOZ Royal Netherlands Institute for Sea Research, Department of Coastal Systems, Texel, Netherlands; Faculty of Environmental Sciences, Czech University of Life Sciences Prague, Prague, Czechia