- 1Department of Organismic and Evolutionary Biology, Harvard University, Cambridge, MA, United States
- 2Department of Mechanical and Aerospace Engineering, Princeton University, Princeton, NJ, United States
- 3School of Chemical Engineering, Oklahoma State University, Stillwater, OK, United States
- 4Interdisciplinary Toxicology Program, Oklahoma State University, Stillwater, OK, United States
Neonicotinoids are a globally prevalent class of pesticides that can negatively affect bees and the pollination services they provide. While there is evidence suggesting that colony size may play an important role in mitigating neonicotinoid exposure in bees, mechanisms underlying these effects are not well understood. Here, a recently developed agent-based computational model is used to investigate how the effects of sub-lethal neonicotinoid exposure on intranest behavior of bumblebees (Bombus impatiens) are modulated by colony size. Simulations from the model, parameterized using empirical data on bumblebee workers exposed to imidacloprid (a common neonicotinoid pesticide), suggest that colony size has significant effects on neonicotinoid-sensitivity within bumblebee nests. Specifically, differences are reduced between treated and untreated workers in larger colonies for several key aspects of behavior within nests. Our results suggest that changes in both number of workers and nest architecture may contribute to making larger colonies less sensitive to pesticide exposure.
Introduction
Agricultural use of neonicotinoid pesticides may negatively affect bees (Cresswell, 2011; Rundlöf et al., 2015; Woodcock et al., 2016, 2017) and the ecosystem services they provide (Stanley et al., 2015a) by altering worker behavior (Gill et al., 2012; Whitehorn et al., 2012; Kessler et al., 2015). Neonicotinoids disrupt learning and navigation of foraging bees (Palmer et al., 2013; Stanley et al., 2015b; Tan et al., 2015) and may impair colony growth (Laycock et al., 2012; Whitehorn et al., 2017) through reductions in resource intake (Gill et al., 2012; Feltham et al., 2014).
Workers within bee nests are likely exposed to neonicotinoids (Rundlöf et al., 2015; Mitchell et al., 2017), which can affect their locomotion and attraction to light (Tosi and Nieh, 2017), thermoregulation (Tosi et al., 2016; Crall et al., 2018b; Potts et al., 2018), and hygienic behaviors (Wu-Smart and Spivak, 2016; Tsvetkov et al., 2017). The neonicotinoid imidacloprid reduces activity and alters spatial dynamics and social interactions within bumblebee (Bombus impatiens) colonies (Crall et al., 2018b).
Mechanistic models of behavior within bee colonies can consider complex impacts of pesticides and other stressors under different scenarios (Bryden et al., 2013; Laycock and Cresswell, 2013; Sponsler and Johnson, 2016; Cresswell, 2017; Henry et al., 2017). Models have been developed for populations of bumblebees (Sponsler and Johnson, 2016; Thorbek et al., 2016; Betti et al., 2017; Henry et al., 2017; Becher et al., 2018) with and without exposure to neonicotinoids.
Colony size is a factor in sensitivity to environmental stressors as it plays a role in the resilience and conservation of social insects (Straub et al., 2015; Fisher et al., 2019). Colony size may affect susceptibility to neonicotinoid pesticides. One study (Rundlöf et al., 2015) found no significant effects of neonicotinoid exposure on honeybees (large colony sizes, >10,000), intermediate effects on bumblebees (moderate colony size, <500 workers), and very strong effects on solitary bees, under the same exposure conditions. Some negative effects of imidacloprid on honeybees were shown to be stronger in smaller colonies (Wu-Smart and Spivak, 2016).
The effects of colony size on worker behavior within nests in response to pesticide exposure are not well understood. Colony size may improve resilience to pesticides as a direct effect of larger population size by decreasing the chances of colony failure when a small number of workers are lost to disease or death or behavioral impairment (Straub et al., 2015). Alternatively, larger colonies could mitigate the effects of exposure on the behavior of individuals. Changes in interaction rate or nest architecture associated with larger colony size could modulate the effects of pesticide exposure on individuals and colony performance through the division of labor, behavioral development, or social interactions associated with large social insect colonies.
Our goal is to elucidate the mechanisms underlying the effects of neonicotinoid exposure on worker behavior within bumblebee nests and how these effects are modulated by colony size. We use our spatially-explicit agent-based model called BeeNestABM (Crall et al., 2018b; Ford Versypt et al., 2018) to test the hypothesis that the effects of exposure on worker behavior within bumblebee nests are reduced in larger colonies. Bumblebee colonies range from a single individual during colony founding by a solitary queen up to several hundred workers (Michener and Laberge, 1954). Inside colonies, interactions with nestmates and nest architecture can have important effects on worker behavior. Interactions with nestmates directly affect activity and task switching in colonies (Renner and Nieh, 2008; Crall et al., 2018b) and could modulate behavioral impacts of pesticides. The physical structure of the nest (e.g., food pots and developing brood) can modulate worker behavior by carrying information on larval development and hunger (Heinrich, 1974; Dornhaus and Chittka, 2005; den Boer and Duchateau, 2006). Because neonicotinoids directly reduce activity, which indirectly alters spatial and social dynamics (Crall et al., 2018b), increased interactions with nestmates or nest structures in larger colonies could mitigate the impacts of neonicotinoid exposure.
Model Description
BeeNestABM was parameterized using empirical data (Crall et al., 2018b) and simulates bumblebee activity and interactions within a nest. The code and documentation are available (Ford Versypt et al., 2018). Here, we briefly describethe model.
BeeNestABM simulates movements of bees within a nest on short time scales allowing for interactions with nestmates and nest structures. The state variables at each time step and for each bee are the x- and y-coordinates, heading angle, speed, and activity state. The simulation is initialized with a user-specified number of bees. Bees are initialized with random positions inside a nest arena (0.2 × 0.2 m for all simulations here). The model is iterated in steps of 0.5 s. Nest structures are placed in 1 cm apart in a square grid covering a centered, user-specified fraction of the arena.
The model consists of three processes that occur at each time step. First, distances between bees and the nest structures (Figure 1A) are calculated. Next, the activity state for each bee (Figures 1C–G) is updated. Finally, the coordinates for each active bee are updated using the movement rules (Figure 1B).
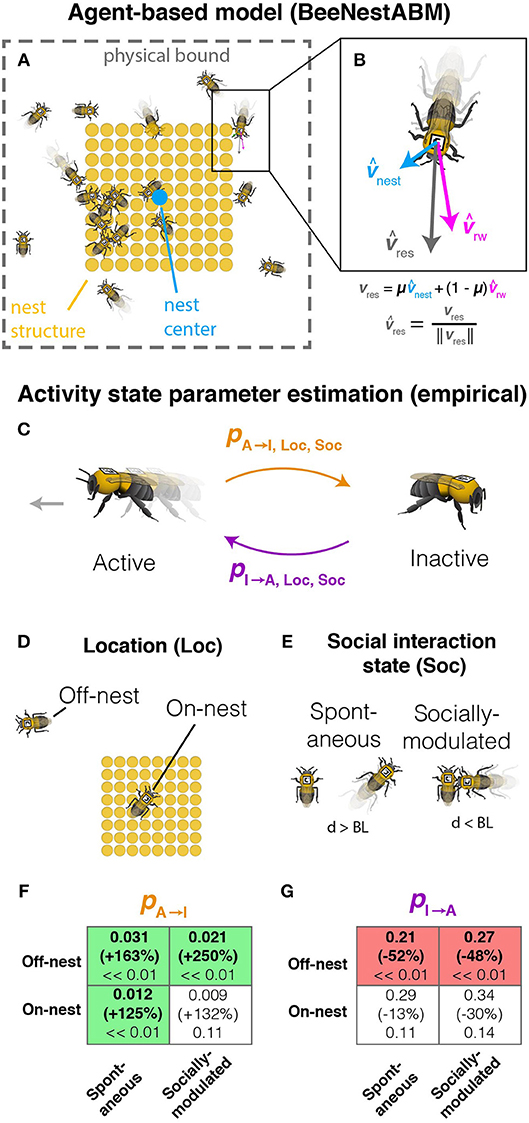
Figure 1. Parameter estimation and model description. (A) Schematic diagram of model simulation, including individual workers, nest structure (filled tan circles), nest center (blue circle), and physical boundaries. (B) Model for simulated worker movement showing the random walk (vrw), nest attraction (vnest), and resultant (vres) movement vectors. (C) Activity switching probabilities (active to inactive, pA→I, and inactive to active, pI→A) are measured separately depending on whether bees are (D) located on or off the nest structure or (E) in physical contact with a nestmate. (F,G) Changes in probability of switching from active to inactive (pA→I, F) and switching from inactive to active state (pI→A, G) as a result of imidacloprid exposure. Probability changes are shown separately for combinations of location (Loc) and social interaction state (Soc). For each box, upper values show parameter estimates for untreated bees, middle values (in parentheses) show the percent change in the estimated parameters in bees fed 1.0 ng imidacloprid (compared to controls), and lower values are p-values. Bold values indicate significant effects, with box color indicating the direction of change (red for decreasing, green for increasing). Figure adapted from Figure S4 and data from Figure S5 in Crall et al. (2018b).
Activity State Updates
Activity-switching probabilities depend on location on or off the nest structures (Figure 1D), proximity to nestmates (Figure 1E), and treatment group (Figures 1C–G). Workers are assigned probabilities calculated previously (Crall et al., 2018b Supplement) from the means of empirical data of automatically tracked (Crall et al., 2015) bumblebees. These probabilities represent the probabilities of switching between active (moving) and inactive (stationary) between time steps, calculated for different combinations of social interaction state and location (Figure 1). Probabilities for treated bees are set equivalent to controls for parameters that did not differ significantly between control and treated workers (Figures 1F,G). Treated bees have activity-switching probabilities that make bees less likely to initiate movement either spontaneously or after physical contact with a nestmate and more likely to become immobilized, with stronger effects when treated bees are located off the nest structures (Figures 1F,G).
In the dataset (Crall et al., 2018b), workers were exposed orally to an acute dose of either 0 ng (control) or 1.0 ng imidacloprid at 87 ppb (treated) in a sucrose solution and allowed to behave freely in a nest arena. 1.0 ng was approximately equal to the cumulative imidacloprid consumed per worker in a single day of chronic feeding on nectar with environmentally realistic imidacloprid concentrations (6 ppb). While that study found qualitatively similar effects on behavior in workers exposed to imidacloprid either acutely or chronically, the behavioral effects during chronic exposure varied with time of day. To limit model complexity, we did not incorporate circadian effects but focused on acute exposure data collected at the same time of day.
Movement Rules
Previously inactive bees are set at a speed sampled from the empirical speed distribution. Bees previously moving are updated to the sampled speed if it is within specified limits of the current speed; otherwise, there is a 10% probability of switching to the sampled value from the current speed. The heading angle for each bee is the weighted angular mean of a random walk angle fluctuating within 90° of the current angle and the angle toward the environmental stimuli determined by the net displacement between the bee and nest structures (Figure 1B). The environmental stimuli represent attractions to the nest structures with weights estimated using empirical data (μ = 0.067 and 0.052 for control and treated bees, respectively). After location updates, movement is truncated for each bee that would move outside the perimeter.
Results and Discussion
We used BeeNestABM to examine the effects of colony size and exposure treatment intensities (the proportion of workers within the colony exposed to imidacloprid) on worker behavior. We ran simulations where two aspects of colony size (numbers of nest structures and of workers) were varied simultaneously from 4 to 441, spanning the ecologically relevant colony sizes for bumblebees, to maintain a 1:1 worker:nest structure ratio across a range of treatment intensities (Figure 2). To examine the relative importance of nest architecture and number of workers, we ran simulations varying these parameters independently (Figures S1, S2). For each combination of colony size and exposure intensity, we estimated mean values across all simulations and individuals for four behavioral metrics (Figures 2A–D and Figures S1, S2).
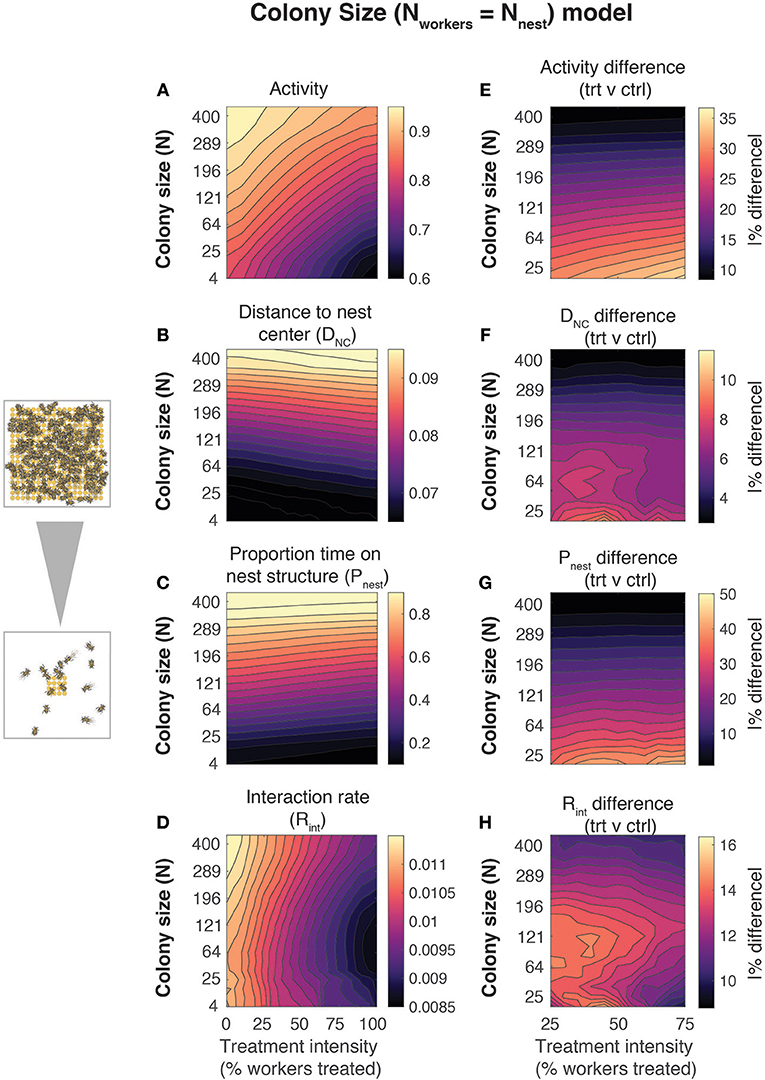
Figure 2. Effects of colony size on emergent behavior in bumblebee nests. Mean behavioral metrics (left column) and relative differences between treated and control workers (right column) as a function of colony size and treatment intensity (% of workers treated). Data are shown for four metrics: (A,E) activity, (B,F) distance to the nest center (DNC), (C,G) proportion of time spent on the nest structure (Pnest), and (D,H) interaction rate (Rint). Values are the mean across all workers and replicate simulations at a given parameter set. The number of simulation iterations at each parameter set was varied with colony size (# of iterations = 10000/N, where N = # of workers). Phase spaces smoothed using local averaging over adjacent values (smoothing window: ±10% for treatment intensity, (sqrt(N-2))2:(sqrt(N+2))2 for Nnest/Nworkers). For all simulations, the number of nest structure elements (Nnest) and the number of workers (Nworkers) were varied simultaneously to maintain a 1:1 nest structure: worker ratio. Differences between control and treated workers (right column) were calculated as a percentage of mean absolute value, abs([trt – ctrl]/mean(trt, ctrl)])*100, where trt denotes the treated workers and ctrl denotes the control group.
Exposure intensity and colony size significantly affected worker behavior within nests. Larger colonies had higher activity levels (Figure 2A), mean distance to the nest center (Figure 2B), and proportions of time spent on the nest structure (Figure 2C), with interaction rates (Figure 2D) decreasing at intermediate colony sizes. Higher treatment intensity was associated with substantial declines in activity and interaction rate (Figures 2A,D), a weak increase in DNC (Figure 2B), and a weak decrease in Pnest (Figure 2C). Simulations isolating the effects of variation in nest size and number of workers separately suggest that the effects of colony size are driven primarily by changes in the size and number of nest structures, especially for DNC and (trivially) Pnest (Figure S1).
We found evidence that the relative difference between control and treated workers varied significantly with colony size (Figures 2E–H). Relative differences between control and treated workers in activity (Figure 2E), DNC (Figure 2F), and Pnest (Figure 2G) were reduced in larger colonies, while relative differences in interaction rate were greatest at intermediate colony sizes (Figure 2H). The reduced differences between control and treated workers in larger colonies are likely driven primarily by the weaker effects of imidacloprid on activity parameters when located on the nest structures (Figures 1F,G and Figure S2). We found qualitatively similar effects for absolute differences between control and treated workers for most metrics (Figure S3), with the exception of proportion of time spent on the nest structure, which varied most with colony size (Figure 2 and Figure S3).
Conclusions
Our results support the hypothesis that larger colonies buffer the effects of neonicotinoid exposure on behavior within bumblebee nests: differences between control and treated workers in several key emergent behavioral parameters were reduced in larger colonies (Figures 2A–D). The dominant effects of nest structure size (Figures S1, S2) suggest that nest architecture, rather than altered rates of interaction with nestmates, is the primary driver of reduced effects of imidacloprid exposure in large colonies, although higher worker numbers did have qualitatively similar, but quantitatively weaker, effects for several metrics (Figure S2). The strong effects of nest architecture found here result directly from the empirical observation that neonicotinoids have particularly strong effects on activity when workers are located on the nest structure (Crall et al., 2018b). The specific mechanisms driving this patterns are not clear but suggest that some aspects of the nest structure (potentially including chemical signals derived from the brood, den Boer and Duchateau, 2006 or from nestmates, Richardson and Gorochowski, 2015 laid on the nest structure) play a direct role in regulating worker behavior. Being on the nest structure stimulates movement (Figure 1), potentially mitigating the negative effects of neonicotinoids on activity.
The mitigating effects of colony size likely translate into significant impacts on colony performance. Location on the nest structure is a strong proxy for direct brood care and maintenance behavior within the nest (Crall et al., 2018a). Our results lead to the testable predictions that (a) similar levels of exposure will lead to stronger effects on nest behavior in smaller colonies and that (b) this would lead to stronger reductions in per-worker nursing rates and brood growth in smaller colonies. For bumblebees, increased sensitivity at small colony sizes is especially relevant because exposure during colony initiation by a solitary queen or during early developmental stages can substantially impair colony performance (Baron et al., 2017; Leza et al., 2018).
Our model improves understanding of how colony size and pesticide exposure affect behavior within bumblebee nests. However, the scope of the model is limited to a simplified set of behaviors. In the future, the model could be expanded to more complex behaviors of bumblebees (Cameron, 1989; Crall et al., 2018a). Combining this model with colony dynamic processes at larger spatial and longer time scales, including foraging, growth, and landscape dynamics (Becher et al., 2018) could help assess how the impacts of neonicotinoids on behavior translate to long-term colony performance.
Author Contributions
JC, BD, and AF contributed equally to this work. JC, BD, and AF designed the study and wrote the computational code. JC shared relevant experimental data from a previous study and conducted the in silico experiments. JC, BdB, BD, and AF analyzed results of the in silico experiments and wrote the manuscript.
Conflict of Interest Statement
The authors declare that the research was conducted in the absence of any commercial or financial relationships that could be construed as a potential conflict of interest.
Acknowledgments
The authors acknowledge support from the Statistical and Applied Mathematical Sciences Institute (SAMSI) and the organizers of the workshop titled Data-Driven Modeling of Collective Behavior and Emergent Phenomena in Biology that enabled the collaboration. JC was supported in part by funding from the Rockefeller Foundation and the Winslow Foundation. BdB was supported by the NSF (IOS-1557913), the Alfred P. Sloan Foundation, The Klingenstein-Simons Fellowship, and the Smith Family Foundation.
Supplementary Material
The Supplementary Material for this article can be found online at: https://www.frontiersin.org/articles/10.3389/fevo.2019.00051/full#supplementary-material
Figure S1. Independent effects of nest structure size and worker number on emergent behavior in bumblebee nests. Mean behavioral metrics when the number of nest structural elements (Nnest, left column) and number of workers (Nworkers, right column) are independently varied. Data are shown for four metrics: (A,E) activity, (B,F) distance to the nest center (DNC), (C,G) proportion of time spent on the nest structure (Pnest), and (D,H) interaction rate (Rint). Values are the mean across all workers and replicate simulations at a given parameter set. The number of simulation iterations at each parameter set was varied with colony size (# of iterations = 10000/N, where N = # of workers). Phase spaces smoothed using local averaging over adjacent values (smoothing window: ±10% for treatment intensity, (sqrt(N-2))2: (sqrt(N+2))2 for Nnest/Nworkers). For each metric, identical color scales are used for the left and right columns.
Figure S2. Independent effects of nest structure size and worker number on relative effect size of imidacloprid. Relative difference between treated and untreated workers when the number of nest structures (Nnest, left column) and number of workers (Nworkers, right column) are independently varied. Data are shown for four metrics: (A,E) activity, (B,F) distance to the nest center (DNC), (C,G) proportion of time spent on the nest structure (Pnest), and (D,H) interaction rate (Rint). Differences calculated as in Figure 2. The number of simulation iterations at each parameter set was varied with colony size (# of iterations = 10000/N, where N = # of workers). Phase spaces smoothed using local averaging over adjacent values (smoothing window: ±10% for treatment intensity, (sqrt(N-2))2: (sqrt(N+2))2 for Nnest/Nworkers). For each metric, identical color scales are used for the left and right columns.
Figure S3. Absolute differences between treated and control workers across treatment intensity and colony size. Simulations are the same as in Figure 2. Relative differences for (A) activity, (B) distance to the nest center (DNC), (C) proportion of time spent on the nest structure (Pnest), and (D) interaction rate (Rint) are shown as the absolute difference (rather than % difference in the right column of Figure S2).
References
Baron, G. L., Jansen, V. A. A., Brown, M. J. F., and Raine, N. E. (2017). Pesticide reduces bumblebee colony initiation and increases probability of population extinction. Nat. Ecol. Evol. 1, 1308–1316. doi: 10.1038/s41559-017-0260-1
Becher, M. A., Twiston-Davies, G., Penny, T. D., Goulson, D., Rotheray, E. L., and Osborne, J. L. (2018). Bumble-BEEHAVE: a systems model for exploring multifactorial causes of bumblebee decline at individual, colony, population and community level. J. Appl. Ecol. 55, 2790–2801. doi: 10.1111/1365-2664.13165
Betti, M., LeClair, J., Wahl, L., and Zamir, M. (2017). Bee++: an object-oriented, agent-based simulator for honey bee colonies. Insects 8:31. doi: 10.3390/insects8010031
Bryden, J., Gill, R. J., Mitton, R. A. A., Raine, N. E., and Jansen, V. A. A. (2013). Chronic sublethal stress causes bee colony failure. Ecol. Lett. 16, 1463–1469. doi: 10.1111/ele.12188
Cameron, S. A. (1989). Temporal patterns of division of labor among workers in the primitively eusocial bumble bee, Bombus griseocollis (Hymenoptera: Apidae). Ethology 80, 137–151. doi: 10.1111/j.1439-0310.1989.tb00735.x
Crall, J. D., Gravish, N., Mountcastle, A. M., and Combes, S. A. (2015). BEEtag: a low-cost, image-based tracking system for the study of animal behavior and locomotion. PLoS ONE 10:e0136487. doi: 10.1371/journal.pone.0136487
Crall, J. D., Gravish, N., Mountcastle, A. M., Kocher, S. D., Oppenheimer, R. L., Pierce, N. E., et al. (2018a). Spatial fidelity of workers predicts collective response to disturbance in a social insect. Nat. Commun. 9:1201. doi: 10.1038/s41467-018-03561-w
Crall, J. D., Switzer, C. M., Oppenheimer, R. L., Ford Versypt, A. N., Dey, B., Brown, A., et al. (2018b). Neonicotinoid exposure disrupts bumblebee nest behavior, social networks, and thermoregulation. Science 362, 683–686. doi: 10.1126/science.aat1598
Cresswell, J. E. (2011). A meta-analysis of experiments testing the effects of a neonicotinoid insecticide (imidacloprid) on honey bees. Ecotoxicology 20, 149–157. doi: 10.1007/s10646-010-0566-0
Cresswell, J. E. (2017). A demographic approach to evaluating the impact of stressors on bumble bee colonies. Ecol. Ent. 42, 221–229. doi: 10.1111/een.12376
den Boer, S. P. A., and Duchateau, M. J. H. M. (2006). A larval hunger signal in the bumblebee Bombus terrestris. Insect. Soc. 53, 369–373. doi: 10.1007/s00040-006-0883-8
Dornhaus, A., and Chittka, L. (2005). Bumble bees (Bombus terrestris) store both food and information in honeypots. Behav. Ecol. 16, 661–666. doi: 10.1093/beheco/ari040
Feltham, H., Park, K., and Goulson, D. (2014). Field realistic doses of pesticide imidacloprid reduce bumblebee pollen foraging efficiency. Ecotoxicology 23, 317–323. doi: 10.1007/s10646-014-1189-7
Fisher, K., West, M., Lomeli, A. M., Woodard, S. H., and Purcell, J. (2019). Are societies resilient? challenges faced by social insects in a changing world. Insect. Soc. 66, 5–13. doi: 10.1007/s00040-018-0663-2
Ford Versypt, A. N., Crall, J. D., and Dey, B. (2018). BeeNestABM: an open-source agent-based model of spatiotemporal distribution of bumblebees in nests. J Open Source Software 3:718. doi: 10.21105/joss.00718
Gill, R. J., Ramos-Rodriguez, O., and Raine, N. E. (2012). Combined pesticide exposure severely affects individual- and colony-level traits in bees. Nature 491, 105–108. doi: 10.1038/nature11585
Heinrich, B. (1974). Pheromone induced brooding behavior in Bombus vosnesenskii and B. edwardsii (Hymenoptera: Bombidae). J. Kans. Entomol. Soc. 47, 396–404.
Henry, M., Becher, M. A., Osborne, J. L., Kennedy, P. J., Aupinel, P., Bretagnolle, V., et al. (2017). Predictive systems models can help elucidate bee declines driven by multiple combined stressors. Apidologie 48, 328–339. doi: 10.1007/s13592-016-0476-0
Kessler, S. C., Tiedeken, E. J., Simcock, K. L., Derveau, S., Mitchell, J., Softley, S., et al. (2015). Bees prefer foods containing neonicotinoid pesticides. Nature 521, 74–76. doi: 10.1038/nature14414
Laycock, I., and Cresswell, J. E. (2013). Repression and recuperation of brood production in Bombus terrestris bumble bees exposed to a pulse of the neonicotinoid pesticide imidacloprid. PLoS ONE 8:e79872. doi: 10.1371/journal.pone.0079872
Laycock, I., Lenthall, K. M., Barratt, A. T., and Cresswell, J. E. (2012). Effects of imidacloprid, a neonicotinoid pesticide, on reproduction in worker bumble bees (Bombus terrestris). Ecotoxicology 21, 1937–1945. doi: 10.1007/s10646-012-0927-y
Leza, M., Watrous, K. M., Bratu, J., and Woodard, S. H. (2018). Effects of neonicotinoid insecticide exposure and monofloral diet on nest-founding bumblebee queens. Proc. Royal Soc. B Biol. Sci. 285:20180761. doi: 10.1098/rspb.2018.0761
Michener, C. D., and Laberge, W. E. (1954). A large bombus nest from Mexico. Psyche 61, 63–67. doi: 10.1155/1954/41470
Mitchell, E. A. D., Mulhauser, B., Mulot, M., Mutabazi, A., Glauser, G., and Aebi, A. (2017). A worldwide survey of neonicotinoids in honey. Science 358, 109–111. doi: 10.1126/science.aan3684
Palmer, M. J., Moffat, C., Saranzewa, N., Harvey, J., Wright, G. A., and Connolly, C. N. (2013). Cholinergic pesticides cause mushroom body neuronal inactivation in honeybees. Nat. Commun. 4:1634. doi: 10.1038/ncomms2648
Potts, R., Clarke, R. M., Oldfield, S. E., Wood, L. K., Hempel de Ibarra, N., and Cresswell, J. E. (2018). The effect of dietary neonicotinoid pesticides on non-flight thermogenesis in worker bumble bees (Bombus terrestris). J. Insect Phys. 104, 33–39. doi: 10.1016/j.jinsphys.2017.11.006
Renner, M. A., and Nieh, J. C. (2008). Bumble bee olfactory information flow and contact-based foraging activation. Insect. Soc. 55, 417–424. doi: 10.1007/s00040-008-1021-6
Richardson, T. O., and Gorochowski, T. E. (2015). Beyond contact-based transmission networks: the role of spatial coincidence. J. R. Soc. Interface 12:20150705. doi: 10.1098/rsif.2015.0705
Rundlöf, M., Andersson, G. K. S., Bommarco, R., Fries, I., Hederström, V., Herbertsson, L., et al. (2015). Seed coating with a neonicotinoid insecticide negatively affects wild bees. Nature 521, 77–80. doi: 10.1038/nature14420
Sponsler, D. B., and Johnson, R. M. (2016). Mechanistic modeling of pesticide exposure: the missing keystone of honey bee toxicology. Environ. Toxicol. Chem. 36, 871–881. doi: 10.1002/etc.3661
Stanley, D. A., Garratt, M. P. D., Wickens, J. B., Wickens, V. J., Potts, S. G., and Raine, N. E. (2015a). Neonicotinoid pesticide exposure impairs crop pollination services provided by bumblebees. Nature 528, 548–550. doi: 10.1038/nature16167
Stanley, D. A., Smith, K. E., and Raine, N. E. (2015b). Bumblebee learning and memory is impaired by chronic exposure to a neonicotinoid pesticide. Sci. Rep. 5:16508. doi: 10.1038/srep16508
Straub, L., Williams, G. R., Pettis, J., Fries, I., and Neumann, P. (2015). Superorganism resilience: eusociality and susceptibility of ecosystem service providing insects to stressors. Curr. Opin. Ins. Sci. 12, 109–112. doi: 10.1016/j.cois.2015.10.010
Tan, K., Chen, W., Dong, S., Liu, X., Wang, Y., and Nieh, J. C. (2015). A neonicotinoid impairs olfactory learning in Asian honey bees (Apis cerana) exposed as larvae or as adults. Sci. Rep. 5:10989. doi: 10.1038/srep10989
Thorbek, P., Campbell, P. J., and Thompson, H. M. (2016). Colony impact of pesticide-induced sublethal effects on honeybee workers: a simulation study using BEEHAVE. Environ. Toxicol. Chem. 36, 831–840. doi: 10.1002/etc.3581
Tosi, S., Démares, F. J., Nicolson, S. W., Medrzycki, P., Pirk, C. W. W., and Human, H. (2016). Effects of a neonicotinoid pesticide on thermoregulation of African honey bees (Apis mellifera scutellata). J. Insect Phys. 93–94, 56–63. doi: 10.1016/j.jinsphys.2016.08.010
Tosi, S., and Nieh, J. C. (2017). A common neonicotinoid pesticide, thiamethoxam, alters honey bee activity, motor functions, and movement to light. Sci. Rep. 7:15132. doi: 10.1038/s41598-017-15308-6
Tsvetkov, N., Samson-Robert, O., Sood, K., Patel, H. S., Malena, D. A., Gajiwala, P. H., et al. (2017). Chronic exposure to neonicotinoids reduces honey bee health near corn crops. Science 356, 1395–1397. doi: 10.1126/science.aam7470
Whitehorn, P. R., O'Connor, S., Wackers, F. L., and Goulson, D. (2012). Neonicotinoid pesticide reduces bumble bee colony growth and queen production. Science 336, 351–352. doi: 10.1126/science.1215025
Whitehorn, P. R., Wallace, C., and Vallejo-Marin, M. (2017). Neonicotinoid pesticide limits improvement in buzz pollination by bumblebees. Sci. Rep. 7:15562. doi: 10.1038/s41598-017-14660-x
Woodcock, B. A., Bullock, J. M., Shore, R. F., Heard, M. S., Pereira, M. G., Redhead, J., et al. (2017). Country-specific effects of neonicotinoid pesticides on honey bees and wild bees. Science 356, 1393–1395. doi: 10.1126/science.aaa1190
Woodcock, B. A., Isaac, N. J. B., Bullock, J. M., Roy, D. B., Garthwaite, D. G., Crowe, A., et al. (2016). Impacts of neonicotinoid use on long-term population changes in wild bees in England. Nat. Commun. 7:12459. doi: 10.1038/ncomms12459
Keywords: pollinator, neonicotinoids, bees, collective behavior, social insects, pesticides, agent-based modeling
Citation: Crall JD, de Bivort BL, Dey B and Ford Versypt AN (2019) Social Buffering of Pesticides in Bumblebees: Agent-Based Modeling of the Effects of Colony Size and Neonicotinoid Exposure on Behavior Within Nests. Front. Ecol. Evol. 7:51. doi: 10.3389/fevo.2019.00051
Received: 16 April 2018; Accepted: 12 February 2019;
Published: 04 March 2019.
Edited by:
Simon Garnier, New Jersey Institute of Technology, United StatesReviewed by:
Nigel E. Raine, University of Guelph, CanadaDino McMahon, Freie Universität Berlin, Germany
Copyright © 2019 Crall, de Bivort, Dey and Ford Versypt. This is an open-access article distributed under the terms of the Creative Commons Attribution License (CC BY). The use, distribution or reproduction in other forums is permitted, provided the original author(s) and the copyright owner(s) are credited and that the original publication in this journal is cited, in accordance with accepted academic practice. No use, distribution or reproduction is permitted which does not comply with these terms.
*Correspondence: Ashlee N. Ford Versypt, ashleefv@okstate.edu
†These authors have contributed equally to this work