- 1Department of Ecology and Evolutionary Biology, University of California, Los Angeles, Los Angeles, CA, United States
- 2The Rocky Mountain Biological Laboratory, Crested Butte, CO, United States
Many animal signals used for mate choice assessment are condition dependent, but less is known about the condition dependence of other biologically important signals. We asked whether yellow-bellied marmot (Marmota flaviventer) alarm calls varied as a function of parasite infection and/or neutrophil:lymphocyte ratios (a measure of immunological challenge). We found that marmots infected with Eimeria, an intestinal parasite, had noisier calls. This is potentially because of an immunostimulating effect of Eimeria infection which may draw energy from nonvital functions. The results suggest calls potentially contain information about parasite status which could be used by receivers to estimate a caller's condition. Future studies are required to determine whether infection influences caller reliability and how receivers respond to alarm calls from parasitized individuals.
Introduction
Many animal signals such as skin or feather color and vocalizations are condition dependent, which means that signal expression varies as a function of an individual's health or nutritional status (Appleby and Redpath, 1997; Von Schantz et al., 1999; Møller et al., 2000; Scheuber et al., 2003). Much of the literature on condition-dependent morphological traits is focused on mate choice. For instance, the large literature on carotenoids and guppy (Poecilia reticulata) coloration shows that bright skin color is sexually selected (Kodric-Brown, 1989) because it is an indication of health (Grether et al., 2004) and/or foraging ability (Grether et al., 2001). This is the case because carotenoids are a limiting factor in pigment production and are relatively scarce in the environment (Grether et al., 1999); animals with bright skin coloration have consumed more carotenoids than dull males and may therefore be better at foraging (Grether et al., 2001). This relationship between physical or physiological condition and signal structure extends to acoustic signals as well.
In many species, acoustic structure of vocal signals can vary based on state-dependent factors such as age (Simmons and Zuk, 1992), the amount of energy reserves within muscles (Bevier, 1997), body size and mass (Appleby and Redpath, 1997), hormone levels (Fusani et al., 1994; Marler and Ryan, 1996), and nutritional state (Noguera et al., 2010). In fact, the acoustic variation in these vocal signals are often used by conspecifics to select healthy mates (Fusani et al., 1994; Beani and Dessi-Fulgheri, 1995) which suggests vocal signals are an indicator of health and physiological condition. Furthermore, Appleby and Redpath (1997) also suggested that calls can be costly to emit and may therefore be an honest indicator of physiological condition.
The present study focuses specifically on the potential information content of acoustic signals as indicators of health status. Immune system activation, a common indicator of health or disease has been associated with increases in the inter-pulse interval of cricket (Gryllus bimaculatus) songs (Fedorka and Mousseau, 2006), and parasitic infections have been associated both with fewer terminal notes in white-crowned sparrow (Zonotrichia leucophrys oriantha) songs (Munoz et al., 2010) and with increases in note frequency and decreases in length of calls in tawny owls (Strix aluco) (Appleby and Redpath, 1997). Because crickets (Gryllus campestris) increase the energetic investment in their stridulatory signals with increased food availability (Scheuber et al., 2003), one explanation for these changes in the structure of acoustic signals is that they are sensitive to anything that influences energy allocation (such as disease or body condition). Similarly, sparrows infected with avian malaria, which effects the ability of their blood to bind to oxygen, had fewer terminal notes, again providing a concrete mechanism linking infection status to vocal output.
The acoustic structure of alarm calls and other vocalizations produced when animals are stressed may be influenced by both external factors (e.g., predator type, degree of risk, etc.), internal factors (physiology, health status), and individual factors (e.g., anatomical differences). For instance, in dogs (Canis lupus familiaris), individuals which were recently unhealthy (defined by recent treatment in a veterinary clinic) emitted barks in which the harmonic to noise ratios were significantly higher than in the calls of healthy dogs (Riede et al., 2001), and in pigs (Sus scrofa), increased pain and stress resulted in calls that were more clear and piercing (Puppe et al., 2005). More specifically, physiological stress–which may result from infections–can affect the Weiner entropy of alarm calls. For instance, as fecal glucocorticoid levels increased, yellow-bellied marmot (Marmota flaviventer) alarm calls became less noisy (as measured by Weiner entropy) (Blumstein and Chi, 2012). While the effects vary, all of these previous studies provide evidence for the association between physiological stress and/or condition and the structure of vocalizations produced by stressed animals. Such relationships are not entirely unexpected as infection and mounting an immunological response may reduce energy to allocate to vocalizations (Scheuber et al., 2003; Fedorka and Mousseau, 2006) and this may potentially influence the structure of alarm calls.
While any vocalization may be described by a variety of acoustic measurements, we focused here on changes in Weiner entropy of alarm calls. These calls are emitted when animals encounter predators; a fear-inducing situation. Prior work has shown that deterministic chaos or noise (which was used to simulate deterministic chaos), and other acoustic non-linearities (e.g., subharmonics, biphonation, rapid frequency shifts, and rapid amplitude shifts) are associated with fear in humans and animals (Blumstein and Recapet, 2009; Blumstein et al., 2010, 2012; Townsend and Manser, 2011; Slaughter et al., 2013; Blesdoe and Blumstein, 2014), and changes in Weiner entropy are associated with fear-driven stressors (Blumstein and Chi, 2012).
Given that our understanding of the relationship between physiological condition and the structure of alarm vocalizations is in its infancy, we asked whether call noisiness (which we measured by Weiner entropy) is associated with: (1) the presence of specific intestinal parasites; (2) total intestinal parasite diversity; and (3) marmots' neutrophil:lymphocyte ratio. Prior work has shown that call structure is influenced by intestinal parasite load in tawny owls (Redpath et al., 2000) and that specific parasites differentially effect vocal structure in white-crowned sparrows, as described above (Gilman et al., 2007). Thus, we expected that parasite infection would modify marmot alarm call structure but the precise way that it would was an open question. Additionally, because the energy required to mount an immune response (measured by N:L ratios) may cause energy to be reallocated toward improving health rather than defense or signal production (Scheuber et al., 2003; Fedorka and Mousseau, 2006), we expected that there would be a relationship between call structure and N:L ratio as well.
If the nonlinearity and fear hypothesis explained call noisiness, then we would expect that individual parasites, an increase in parasite diversity, and increased N:L ratios would be associated with increased noisiness. Interestingly, prior work showed that marmots with higher baseline glucocorticoid levels produced calls with reduced, rather than increased, Weiner entropy (Blumstein and Chi, 2012) suggesting that more arousable individuals may siphon energy away from call mechanics. Thus, the question of whether factors that influence perceived vulnerability or risk are associated with increased noisiness remains unresolved.
Materials and Methods
Subjects and Data Collection
We studied a wild population of yellow-bellied marmots in and around the Rocky Mountain Biological Laboratory during their active seasons from 2003 to 2017. We aimed to live-trap marmots every other week during the snow-free part of their active season (May–September) during which animals were marked (ear tags for permanent identification and fur dye that lasted until their next moult) and a number of physiological samples were collected.
If marmots produced alarm calls when trapped, we recorded these human-elicited alarm calls using an Audix OM-3xb microphone (frequency response: 40 Hz−20 kHz) located 20–40 cm from the trap. Prior work has shown that yellow-bellied marmot calls communicate risk, not predator type (Blumstein and Armitage, 1997). Thus, by standardizing the context of call production, we could focus on physiological correlates of call structure. The calls were recorded onto either a Sony PCM-M1 digital audio tape recorder or a Marantz PMD 660 direct to disk recorder. All samples were saved at 44.1 kHz and 16 bit resolution. Recordings with noticeable background noise or calls that were clipped were removed from subsequent analyses. Each call was edited into a 1 s file for subsequent analysis in Sound Analysis Pro (Ofer Tchernichovski, City College of New York). Calls are generally much shorter than 1 s; thus 1 s was chosen to standardize the measurements. This sound clip always contained the entire call. Sound Analysis Pro calculates Wiener entropy. Wiener entropy ranges from 0 (pure noise) to negative values that indicate increasing structure (or decreased entropy). Thus, noisier calls had values closer to 0.
As part of the routine live-trapping between 2003 and 2008, we also collected fecal samples from marmots that defecated in the trap or while we were processing them for a subsequent study of intestinal parasites. The sample was stored in formalin immediately. Within 6 months, fecal samples were analyzed by performing fecal floats using Ova FloatTM Zn 118 (zinc sulfate heptahydrate; Butler Animal Health Supply, Dublin, OH, USA). Up to one sample was used for each individual in a given month. The wet slides were then scored for presence of three fecal-orally transmitted (MacNeal, 1904) intestinal parasites: Ascaris (a nematode), Eimeria (a coccidian), and Entamoeba (a protozoan) (Lopez et al., 2013). Intestinal parasites have been associated with decreased food intake and anorexia (Jones et al., 2006; Laurenson et al., 2011) and in marmots, Ascaris has been associated with less time spent foraging (Chmura et al., 2016).
Finally, also as part of routine live-trapping, we collected up to a 2 ml blood sample from the femoral vein of the marmots, placed it in a heparin-filled tube, and made a thin film blood smear within 2 hours of collection (Chmura et al., 2016). Slides were stained using the Hema 3 Stat Pack (Thermo Fisher Scientific, Inc., Waltham, MA, USA) (Wey and Blumstein, 2012). We used a standard procedure to calculate the neutrophil:lymphocyte (N:L) ratio where we counted neutrophils, lymphocytes, basophils, and monocytes up to 100 cells or for 30 min (whichever came first). Trypanosoma (which may be transmitted by fleas; MacNeal, 1904) presence was a binary measure—either present or not—and was noted during the white blood cell counting. Trypanosoma has been associated with more time spent foraging and less time spent vigilant in marmots (Chmura et al., 2016).
There are a number of quantifiable traits associated with health status. We used neutrophil:lymphocyte (N:L) ratios as an indicator of changes in health. Prior work has found that increases in N:L ratios are associated with continuously high levels of glucocorticoids indicating chronic stress (Swan and Hickman, 2014). Changes in N:L ratios have also been associated with injury and infection in koalas (Phascolarctos cinereus, Bolliger and Backhouse, 1960) and neutrophils play a critical role in mounting an immunological defense against systemic infections in mice (Conlan, 1997). Higher N:L ratios can therefore indicate the immunological challenge of certain infections or in this case, can indicate activation of an immune response and allocation of energy away from antipredator defenses.
Only alarm calls with either an associated blood sample or fecal parasite data (defined as collected within 10 days of the call) were used in the study. The sample sizes were therefore different for the alarm call to N:L ratio and alarm call to Trypanosoma presence as opposed to the analysis of the alarm calls to intestinal parasites. The sample size for the N:L ratio and Trypanosoma presence consisted of 836 calls from 107 individuals whereas the sample size for the fecal parasite presence consisted of 536 calls from 62 individuals. In total (both blood and fecal analyses) 146 individuals were represented (70 males and 76 females) for which we had 3-5 calls from each individual recorded at a given recording session.
Statistical Analysis
We fitted a series of linear mixed effects models using lme4 (Bates and Maechler, 2018) and lmerTest (Kuznetsova et al., 2018) in R (R Development Core Team, 2009) to explain variation in Wiener entropy. Fixed effects included age, sex, and one of the measures of condition—neutrophil:lymphocyte ratio, the presence of Trypanosoma, the presence of Ascaris, Eimeria, Entamoeba, or the total number of species of fecal parasites (i.e., the sum of Ascaris, Eimeria, and Entamoeba). Marmot identity was fitted as a random effect. We examined residuals to confirm the models were appropriate for the data structure.
Ethics
Marmots were studied under annual permits issued by the Colorado Division of Wildlife (TR-917). All procedures were approved under research protocol ARC 2001-191-01 by the University of California Los Angeles Animal Care Committee on May 13, 2002, and renewed annually.
Results
After controlling for significant age effects (p < 0.001) and non-significant sex effects (p = 0.514 for N:L ratio and 0.523 for Trypanosoma), neither the neutrophil to lymphocyte ratio (p = 0.925) nor the presence of Trypanosoma (p = 0.568) had a significant effect on Wiener entropy (Tables 1A,B). We did however find a significant effect of Eimeria on marmot alarm calls (p < 0.001) such that animals infected with Eimeria produced noisier calls with higher Wiener entropy values (Table 1C). Similarly, animals with more intestinal parasites in general produced calls with higher Wiener entropy (p = 0.008) (Table 1D). After adjusting for age and sex, we found no significant effects of Ascaris (p = 0.708) or Entamoeba (p = 0.231) on the noisiness of marmot alarm calls (Table 1C).
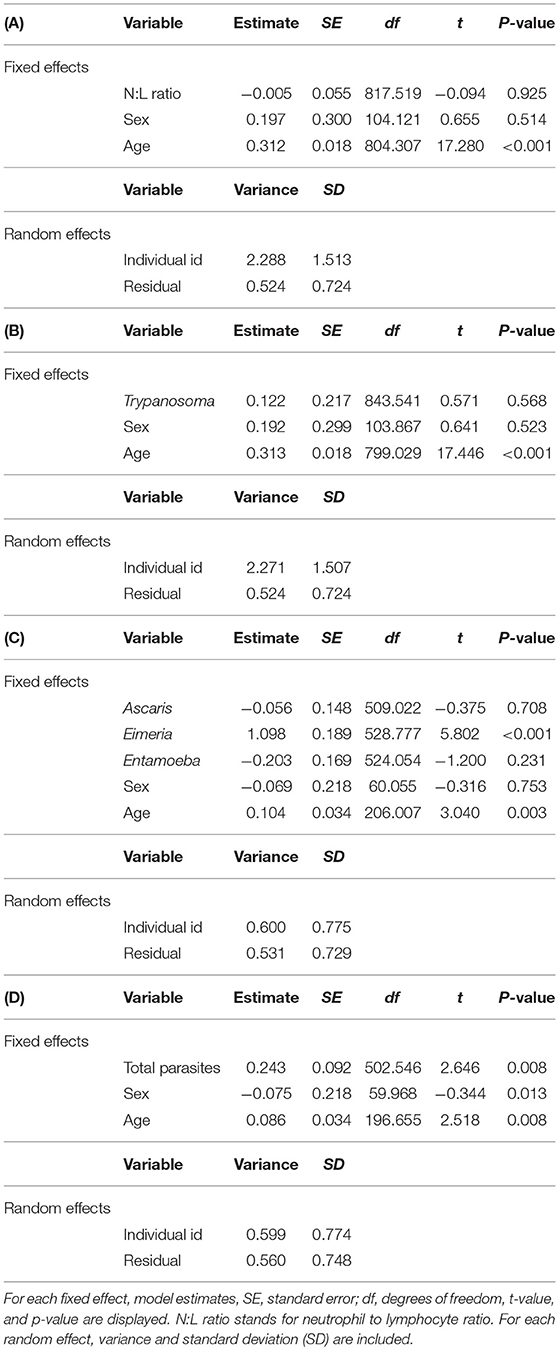
Table 1. Results from linear mixed effects models explaining variation in alarm call entropy as a function of: (A) N:L ratio, (B) the presence or absence of Trypanosoma sp., (C) the presence or absence of each individual intestinal parasite, and (D) intestinal parasite diversity.
Discussion
Despite a growing literature that shows a relationship between vocal structure and disease or health status (Appleby and Redpath, 1997; Fedorka and Mousseau, 2006; Munoz et al., 2010; Noguera et al., 2010), the Weiner entropy of yellow-bellied marmot alarm calls was not significantly associated with the presence of Ascaris, Entamoeba, or Trypanosoma, or by an individual's neutrophil:lymphocyte ratio. Alarm calls were, however, significantly noisier for marmots infected with Eimeria and for marmots with higher fecal parasite diversity.
These results could reflect how each parasite stimulates or suppresses the immune system. Yun et al. (2000) noted that many Eimeria oocysts are required to generate an immune response, and we note that it was not difficult to detect Eimeria—marmots shed many oocysts. By contrast, many parasites have immunosuppressive effects and this includes Ascaris (Faquim-Mauro and Macedo, 1998), Entamoeba (Soboslay et al., 2006; Lejeune et al., 2009), and Trypanosoma (Hirokawa et al., 1981; Albright et al., 1990). The changes we saw in the alarm calls may be explained by Eimeria stimulating an immune response such that individuals divert energy away from vocalizations and toward immune response and therefore may not have sufficient energy to enable them to produce “proper” calls. By contrast, marmots infected with an immunosuppressive parasite may not divert energy and hence could maintain the ability to emit proper calls. If this is the case, call acoustics is indeed an indicator of the caller's condition. Alternatively, infected individuals are more vulnerable and hence produce higher-risk calls (see below). Whether this potential information is used by receivers remains an open question.
Both conspecifics and predators can use information contained in calls and it is profitable to view a potential communication system from both perspectives. From the conspecific's perspective, a sick caller may be less reliable if they are more vulnerable than normal and this enhanced vulnerability is communicated acoustically. Rodents are indeed capable of associating individuals with reliability (Hare and Atkins, 2001; Blumstein et al., 2004). However, prior work has shown that physiological stress is associated with calls with less Weiner entropy—scared marmots articulate their calls (Blumstein and Chi, 2012). If the valence of entropy is such that more scared animals produce less noisy calls, then these results suggest that Eimeria influences how marmots perceive risk; infected marmots perceive less risk, not more risk. Some parasites with complex multi-host lifecycles are known to influence risk assessment (e.g., Toxoplasma gondii, Berdoy et al., 2000), but by doing so those parasites increase the likelihood that they reach their next host. This is not the case with Eimeria which reproduces inside marmots. Future studies should look for associations between Eimeria infection and other antipredator traits (e.g., maximum running speed). Regardless of the mechanism, this information about risk perception may translate to a less reliable caller resulting in perceivers acting less vigilant or habituating to the calls from sick individuals (Hare and Atkins, 2001).
From, a predator's perspective a sick caller might be more attractive in that it may be less able to defend itself. Prior work has found that less popular or more docile marmots are more likely to call (Fuong et al., 2015) and that altering these calls may be due to an increased vulnerability from being in a smaller group or otherwise more socially isolated (Fuong et al., 2015). Sick marmots may also be less able to defend themselves and therefore more vulnerable. If marmots do indeed alter their call structure when they are more vulnerable, this could explain the difference in entropy of marmots infected with Eimeria. By signaling their vulnerability, sick marmots could conceivably solicit help from others—something that we have not obviously noticed in the field, but nevertheless requires more detailed study to properly reject.
While the potential consequences of the results are speculative, these results combined with prior results from a variety of species, suggest that internal state, including parasite status, may generally influence the structure of a variety of vocalizations, including alarm vocalizations. From an applied perspective, such information could be used to non-invasively monitor an individual's health status. Future work determining whether and how marmots respond to the calls from healthy and sick individuals would shed more light on whether, and how, this potential information is used. Additionally, studying the underlying affects of immune system response on vocalizations may help determine which vocalizations suggest disease or other health information in wild populations.
Data Availability Statement
The raw data supporting the conclusions of this manuscript will be made available by the authors, without undue reservation, to any qualified researcher.
Author Contributions
DB conceived idea, collected field data, and guided analyses. KN measured alarm calls and helped analyze data. Both authors wrote the manuscript.
Funding
National Geographic Society, UCLA (Faculty Senate and the Division of Life Sciences), a Rocky Mountain Biological Laboratory research fellowship, NSF IDBR-0754247, and DEB-1119660 and 1557130 all to DB, and DBI-0242960, 0731346, and 1226713 to the RMBL.
Conflict of Interest Statement
The authors declare that the research was conducted in the absence of any commercial or financial relationships that could be construed as a potential conflict of interest.
Acknowledgments
We thank the many marmoteers who collected data over the years and two reviewers for very constructive comments.
References
Albright, J. W., Holmes, K. L., and Albright, J. F. (1990). Fluctuations in subsets of splenocytes and isotypes of Ig in young adult and aged mice resulting from Trypanosoma musculi infections. J. Immunol. 144, 3970–3979.
Appleby, B. M., and Redpath, S. M. (1997). Indicators of male quality in the hoots of tawny owls (Strix aluco). J. Raptor Res. 31, 65–70.
Bates, D., and Maechler, M. (2018). lme4: Linear Mixed-Effects Models Using 'Eigen' and S4 Classes. R package version 1.1–17.
Beani, L., and Dessi-Fulgheri, F. (1995). Mate choice in the grey partridge Perdix perdix: role of physical and behavioural male traits. Anim. Behav. 49, 347–356. doi: 10.1006/anbe.1995.0047
Berdoy, M., Webster, J. P., and MacDonald, D. W. (2000). Fatal attraction in rats infected with Toxoplasma gondii. Proc. Biol. Sci. 267, 1591–1594. doi: 10.1098/rspb.2000.1182
Bevier, C. (1997). Utilization of energy substrates during calling activity in tropical frogs. Behav. Ecol. Sociobiol. 41, 343–352. doi: 10.1007/s002650050394
Blesdoe, E. K., and Blumstein, D. T. (2014). What is the sound of fear? Behavioral responses of white-crowned sparrows Zonotrichia leucophrys to synthesized nonlinear acoustic phenomena. Curr. Zool. 60, 534–541. doi: 10.1093/czoolo/60.4.534
Blumstein, D. T., and Armitage, K. B. (1997). Alarm calling in yellow-bellied marmots: I. The meaning of situationally-variable alarm calls. Anim. Behav. 53, 143–171. doi: 10.1006/anbe.1996.0285
Blumstein, D. T., Bryant, G. A., and Kaye, P. (2012). The sound of arousal in music is context-dependent. Biol. Lett. 8, 744–747. doi: 10.1098/rsbl.2012.0374
Blumstein, D. T., and Chi, Y. Y. (2012). Scared and less noisy: glucocorticoids are associated with alarm call entropy. Biol. Lett. 8, 189–192. doi: 10.1098/rsbl.2011.0832
Blumstein, D. T., Davitian, R., and Kay, P. D. (2010). Do film soundtracks contain nonlinear analogues to influence emotion? Biol. Lett. 6, 751–754. doi: 10.1098/rsbl.2010.0333
Blumstein, D. T., and Recapet, C. (2009). The sound of arousal: the addition of novel non-linearities increases responsiveness in marmot alarm calls. Ethology 115, 1074–1081. doi: 10.1111/j.1439-0310.2009.01691.x
Blumstein, D. T., Verneyre, L., and Daniel, J. C. (2004). Reliability and the adaptive utility of discriminating among alarm callers. Proc. R. Soc. B 271, 1851–1857. doi: 10.1098/rspb.2004.2808
Bolliger, A., and Backhouse, T. (1960). The blood of the koala (Phascolarctos cinereus). Aust. J. Zool. 8, 363–370. doi: 10.1071/ZO9600363
Chmura, H. E., Wey, T. W., and Blumstein, D. T. (2016). Assessing the sensitivity of foraging and vigilance to internal state and environmental variables in yellow-bellied marmots (Marmota flaviventris). Behav. Ecol. Sociobiol. 70, 1901–1910. doi: 10.1007/s00265-016-2195-y
Conlan, J. W. (1997). Critical roles of neutrophils in host defense against experimental systemic infections of mice by Listeria monocytogenes, Salmonella typhimurium, and Yersinia enterocolitica. Infect. Immun. 65, 630–635.
Faquim-Mauro, E. L., and Macedo, M. S. (1998). The immunosuppressive activity of Ascaris suum is due to high molecular weight components. Clin. Exp. Immunol. 114, 245–251. doi: 10.1046/j.1365-2249.1998.00723.x
Fedorka, K. M., and Mousseau, T. A. (2006). Immune system activation affects male sexual signal and reproductive potential in crickets. Behav. Ecol. 18, 231–235. doi: 10.1093/beheco/arl067
Fuong, H., Maldonado-Chaparro, A., and Blumstein, D. T. (2015). Are social attributes associated with alarm calling propensity? Behav. Ecol. 26, 587–592. doi: 10.1093/beheco/aru235
Fusani, L., Beani, L., and Dessi-Fulgheri, F. (1994). Testosterone affects the acoustic structure of male call in the grey partridge Perdix perdix. Behaviour 128, 301–310. doi: 10.1163/156853994X00307
Gilman, S., Blumstein, D. T., and Foufopoulos, J. (2007). The effect of Hemosporidian infections on white-crowned sparrow singing behavior. Ethology 113, 437–445. doi: 10.1111/j.1439-0310.2006.01341.x
Grether, G. F., Hudon, J., and Endler, J. A. (2001). Carotenoid scarcity, synthetic pteridine pigments and the evolution of sexual coloration in guppies (Poecilia reticulata). Proc. R. Soc. Lond. B 268:1473. doi: 10.1098/rspb.2001.1624
Grether, G. F., Hudon, J., and Millie, D. F. (1999). Carotenoid limitation of sexual coloration along an environmental gradient in guppies. Proc. R. Soc. Lond. B 266:1426. doi: 10.1098/rspb.1999.0781
Grether, G. F., Kasahara, S., Kolluru, G. R., and Cooper, E. L. (2004). Sex-specific effects of carotenoid intake on the immunological response to allografts in guppies (Poecilia reticulata). Proc. R. Soc. Lond. B 271, 45–49. doi: 10.1098/rspb.2003.2526
Hare, J. F., and Atkins, B. A. (2001). The squirrel that cried wolf: reliability detection by juvenile Richardson's ground squirrels (Spermophilus richardsonii). Behav. Ecol. Sociobiol. 51, 108–112. doi: 10.1007/s002650100414
Hirokawa, K., Eishi, Y., Albright, J. W., and Albright, J. F. (1981). Histopathological and immunocytochemical studies of Trypanosoma musculi infection of mice. Infect. Immun. 34, 1008–1017.
Jones, O. R., Anderson, R. M., and Pilkington, J. G. (2006). Parasite-induced anorexia in a free-ranging mammalian herbivore: an experimental test using Soay sheep. Can. J. Zool. 84, 685–692. doi: 10.1139/z06-045
Kodric-Brown, A. (1989). Dietary carotenoids and male mating success in the guppy: an environmental component to female choice. Behav. Ecol. Sociobiol. 25, 393–401. doi: 10.1007/BF00300185
Kuznetsova, A., Brockhoff, P. B., and Christensen, R. H. B. (2018). lmerTest: Tests in Linear Mixed Effects Models. R package version 3.0–1.
Laurenson, Y., Bishop, S. C., and Kyriazakis, I. (2011). In silico exploration of the mechanisms that underlie parasite-induced anorexia in sheep. Br. J. Nutr. 106, 1023–1039. doi: 10.1017/S0007114511001371
Lejeune, M., Rybicka, J. M., and Chadee, K. (2009). Recent discoveries in the pathogenesis and immune response toward Entamoeba histolytica. Future Microbiol. 4, 105–116. doi: 10.2217/17460913.4.1.105
Lopez, J., Wey, T. W., and Blumstein, D. T. (2013). Patterns of parasite prevalence and individual infection in yellow-bellied marmots. J. Zool. 291, 296–303. doi: 10.1111/jzo.12076
MacNeal, W. J. (1904). The life-history of Trypanosoma lewisi and Trypanosoma brucei. J. Infect. Dis. 1, 517–543. doi: 10.1093/infdis/1.4.517
Marler, C. A., and Ryan, M. J. (1996). Energetic constraints and steroid hormone correlates of male calling behaviour in the tungara frog. J. Zool. 240, 397–409. doi: 10.1111/j.1469-7998.1996.tb05294.x
Møller, A. P., Biard, C., Blount, J. D., Houston, D. C., Ninni, P., Saino, N., et al. (2000). Carotenoid-dependent signals: indicators of foraging efficiency, immunocompetence or detoxification ability? Avian Poultry Biol. Rev. 11, 137–159.
Munoz, N. E., Blumstein, D. T., and Foufopoulos, J. (2010). Immune system activation affects song and territorial defense. Behav. Ecol. 21, 788–793. doi: 10.1093/beheco/arq054
Noguera, J. C., Morales, J., Perez, C., and Velando, A. (2010). On the oxidative cost of begging: antioxidants enhance vocalizations in gull chicks. Behav. Ecol. 21, 479–484. doi: 10.1093/beheco/arq005
Puppe, B., Schön, P. C., Tuchscherer, A., and Manteuffel, G. (2005). Castration-induced vocalisation in domestic piglets, Sus scrofa: complex and specific alterations of vocal quality. Appl. Anim. Behav. Sci. 95, 67–78. doi: 10.1016/j.applanim.2005.05.001
R Development Core Team (2009). R: A Language and Environment for Statistical Computer. Vienna: R Foundation for Statistical Computing.
Redpath, S. M., Appleby, B. M., and Petty, S. J. (2000). Do male hoots betray parasite loads in Tawny Owls? J. Avian Biol. 31, 457–462. doi: 10.1034/j.1600-048X.2000.310404.x
Riede, T., Herzel, H., Hammerschmidt, K., Brunnberg, L., and Tembrock, G. (2001). The harmonic-to-noise ratio applied to dog barks. J. Acoust. Soc. Am. 110, 2191–2197. doi: 10.1121/1.1398052
Scheuber, H., Jacot, A., and Brinkhof, M. W. G. (2003). Condition dependence of a multicomponent sexual signal in the field cricket Gryllus campestris. Anim. Behav. 65, 721–727. doi: 10.1006/anbe.2003.2083
Simmons, L. W., and Zuk, M. (1992). Variability in call structure and pairing success of male field crickets, Gryllus bimaculatus: the effects of age, size and parasite load. Anim. Behav. 44, 1145–1152. doi: 10.1016/S0003-3472(05)80326-4
Slaughter, E. I., Berlin, E. R., Bower, J. T., and Blumstein, D. T. (2013). A test of the nonlinearity hypothesis in great-tailed grackles Quiscalus mexicanus. Ethology 119, 309–315. doi: 10.1111/eth.12066
Soboslay, P. T., Hamm, D. M., Pfafflin, F., Fendt, J., Banla, M., and Schulz-Key, H. (2006). Cytokine and chemokine responses in patients co-infected with Entamoeba histolytica/dispas, Necator americanus and Mansonella perstans and changes after anti-parasite treatment. Microbes Infect. 8, 238–247. doi: 10.1016/j.micinf.2005.06.019
Swan, M. P., and Hickman, D. L. (2014). Evaluation of the neutrophil-lymphocyte ratio as a measure of distress in rats. Lab Anim. 43, 276–282. doi: 10.1038/laban.529
Townsend, S. W., and Manser, M. B. (2011). The function of nonlinear phenomena in meerkat alarm calls. Biol. Lett. 7, 47–49. doi: 10.1098/rsbl.2010.0537
Von Schantz, T., Bensch, S., Grahn, M., Hasselquist, D., and Wittzell, H. (1999). Good genes, oxidative stress and condition-dependent sexual signals. Proc. R. Soc. Lond. B 266:1414. doi: 10.1098/rspb.1999.0597
Wey, T. W., and Blumstein, D. T. (2012). Social attributes and associated performance measures in marmots: bigger male bullies and weakly affiliating females have higher annual reproductive success. Behav. Ecol. Sociobiol. 66, 1075–1085. doi: 10.1007/s00265-012-1358-8
Keywords: Marmota flaviventer, alarm calls, communication, health, parasites
Citation: Nouri K and Blumstein DT (2019) Parasites Are Associated With Noisy Alarm Calls. Front. Ecol. Evol. 7:28. doi: 10.3389/fevo.2019.00028
Received: 01 October 2018; Accepted: 28 January 2019;
Published: 13 February 2019.
Edited by:
Mark A. Elgar, The University of Melbourne, AustraliaReviewed by:
Jennifer Kelley, University of Western Australia, AustraliaMichael Griesser, University of Zurich, Switzerland
Copyright © 2019 Nouri and Blumstein. This is an open-access article distributed under the terms of the Creative Commons Attribution License (CC BY). The use, distribution or reproduction in other forums is permitted, provided the original author(s) and the copyright owner(s) are credited and that the original publication in this journal is cited, in accordance with accepted academic practice. No use, distribution or reproduction is permitted which does not comply with these terms.
*Correspondence: Daniel T. Blumstein, bWFybW90c0B1Y2xhLmVkdQ==