- 1Institute of Zoology, University of Regensburg, Regensburg, Germany
- 2Department of Biological Sciences, Northern Illinois University, DeKalb, IL, United States
- 3Institute of Organic Chemistry, Technische Universität Braunschweig, Braunschweig, Germany
- 4Faculty of Chemistry and Pharmacy, University of Regensburg, Regensburg, Germany
- 5Institute of Biophysics and Physical Biochemistry, University of Regensburg, Regensburg, Germany
Sex pheromones play a crucial role in the mate finding process of insects. The information has to be encoded in a species specific manner to avoid fitness costs due to courtship by or even mismating with closely related species. Hence, sex pheromones normally diversify when new species evolve. Pheromones of closely related species typically vary in their chemical composition, but the compounds they use are often biosynthetically related. The biosynthetic pathways of insect pheromones are variations of conserved pathways that lead to primary and secondary metabolites. A switch from one pathway to another, particularly in species that use the same type of pheromone glands, is the exception. Here we use chemical analyses and behavioral bioassays to examine the male sex pheromone of the parasitoid wasp Urolepis rufipes. The genus Urolepis is most closely related to Nasonia and Trichomalopsis, forming the so-called “Nasonia group.” All Nasonia species and T. sarcophagae, the only Trichomalopsis species studied so far, produce fatty acid-derived hydroxylactones in their rectal vesicle. The pheromones are deposited on the ground and other substrates and attract virgin females. We show that males of U. rufipes produce a sex pheromone in the same gland and use it in the same manner as the other species of the Nasonia group. Structure elucidation and stable isotope labeling experiments revealed, however, that the U. rufipes pheromone is 2,6-dimethyl-7-octene-1,6-diol, a monoterpenoid derived from the mevalonate pathway. This suggests a biosynthetic switch between the fatty acid and isoprenoid metabolism in the Nasonia group.
Introduction
The mate finding process of animals typically includes sexual communication via visual, auditory, or olfactory signals (Smith and Harper, 2003). Most insects produce sex pheromones to attract potential mates from a distance and to exchange information at close range about their species, sex, or individual quality (Johansson and Jones, 2007; Wyatt, 2014). Sex pheromones have to encode information in a species-specific manner to prevent costly sexual interactions with unsuitable mates or even mismating with closely related species. As a consequence, sex pheromones typically diversify when new species evolve (Smadja and Butlin, 2009). Insect pheromones are mostly blends of several compounds which are synthesized in specialized glands or tissues and often occur in species-specific ratios (Schulz and Francke, 2010). The biosynthetic pathways by which insects produce sex pheromones are often variations of the conserved pathways leading to common primary and secondary metabolites, such as fatty acids, amino acids, aromatics, polyketides or isoprenoids (Tillman et al., 1999; Morgan, 2004; Jurenka et al., 2017).
Novel pheromone phenotypes can evolve either quantitatively by shifts of the relative proportions of the same components or qualitatively by the gain or loss of single components. This process can occur gradually by subtle changes over evolutionary time or by saltational shifts generating phenotypes that are greatly or completely different from the antecedent ones (Symonds and Wertheim, 2005; Symonds and Elgar, 2008; Symonds et al., 2009). New pheromone components can derive from simple chemical modifications of existing ones, for instance by enzymatic oxidation, reduction, acetylation, decarboxylation or epimerization (Symonds and Elgar, 2008; Ruther et al., 2016). Even when pheromones of closely related species differ considerably in their chemical composition, the compounds they use are often biosynthetically related (Tillman et al., 1999; Symonds and Elgar, 2008; Wyatt, 2014). Much less common is a switch from one pathway to another, particularly in species that use the same type of pheromone glands. For example, in moths virtually all known female sex pheromones are fatty acid derivatives which are either synthesized de novo from acetyl-CoA units (Type I pheromones) or produced by modification of dietary polyunsaturated fatty acids (Type II) (Ando et al., 2004). In bark beetles, the vast majority of aggregation pheromones are isoprenoids synthesized de novo via the mevalonate pathway (Blomquist et al., 2012) and many compounds are shared by numerous bark beetle species from different genera (El-Sayed, 2018).
Parasitoid wasps are excellent models to study pheromone communication in insects (Niehuis et al., 2013; Ruther, 2013; Weiss et al., 2013). In particular, the genus Nasonia (Ashmead, 1904), which includes the genetic model organism N. vitripennis (Nv), has allowed significant advances in our understanding of the behavioral, biochemical, genetic, and evolutionary aspects of pheromone communication. Apart from Nv, the genus consists of the species N. longicornis (Nl), N. giraulti (Ng), and N. oneida (No), all of which parasitize the pupae of numerous fly species. Nv is cosmopolitan and sympatric with Ng and No in eastern North America and with Nl in western North America (Darling and Werren, 1990; Raychoudhury et al., 2010; Werren et al., 2010).
Nasonia males deposit volatile sex pheromones on substrates, such as host pupae from which females are about to emerge or the ground in the vicinity of these hosts (Mair and Ruther, 2018). A male does this by dragging the tip of his abdomen, leaving behind short, white marks. Nv males show increased marking behavior after copulation and after mere contact with a female during courtship (Barrass, 1969; Van Den Assem et al., 1980; Steiner and Ruther, 2009). Marked areas are highly attractive to virgin females (Ruther et al., 2007), and males are arrested by their own marks (Van Den Assem et al., 1980; Ruther et al., 2008). Studies on Nv have shown that the female's response is plastic, with her becoming unresponsive to the pheromone after mating (Ruther et al., 2007, 2014). This switch in responsiveness occurs quickly and is independent of sperm transfer. Instead, the switch correlates with the female's receptivity signal (lowering of the antennae and simultaneous opening of the genital orifice), which she exhibits during courtship by a mounted male (Ruther et al., 2010; Ruther and Hammerl, 2014; Lenschow et al., 2018).
The pheromones of all Nasonia species contain (4R,5S)-5-hydroxy-4-decanolide (RS) as a major component, whereas only Nv males produce significant amounts of its epimer, (4R,5R)-5-hydroxy-4-decanolide (RR). RR is produced enzymatically from RS by short chain dehydrogenases/reductases (Niehuis et al., 2013; Ruther et al., 2016). Nv males emerge without the pheromone in the gland, but titers increase within the first 2 days after emergence (Ruther et al., 2007). Traces of 4-methylquinazoline (MQ) also occur in the pheromone glands of all Nasonia species, synergizing the female response to the hydroxylactones and mediating site fidelity of males at marked areas (Ruther et al., 2008, 2011). Stable isotope labeling experiments with Nv have revealed that both RS and RR are derived from fatty acid metabolism, with oleic and linoleic acid functioning as precursors (Abdel-Latief et al., 2008; Blaul and Ruther, 2011; Blaul et al., 2014).
The genus Nasonia is closely related to the genera Trichomalopsis (Crawford, 1913) and Urolepis (Walker, 1846), and all three genera have been proposed to form a monophyletic taxon, the so-called “Nasonia group” (Burks, 2009). As for the 57 Trichomalopsis species described so far (Noyes, 2017), only T. sarcophagae has been studied with respect to its pheromone communication (Niehuis et al., 2013). The male sex pheromone components of T. sarcophagae match those of Nl, Ng and No, i.e., consist of RS and MQ. In contrast, sex pheromones of the three described Urolepis species, U. maritima, U. singularis, and U. rufipes (Ur) (Gibson, 2000; Noyes, 2017), have not yet been identified, although recent studies suggest that Ur males produce a sex pheromone (Cooper and King, 2015). Like the Nasonia species, Ur is a pupal parasitoid of several Diptera such as house flies and stable flies (Stenseng et al., 2003) and has been found together with Nv in North American dairies (Smith and Rutz, 1991; Gibson and Floate, 2004) as well as in European pig and cattle farms (Skovgard and Jespersen, 2000). Ur males exhibit substrate-borne marking similar to what has been described for Nasonia males (Cooper and King, 2015). Ur females spend more time near these markings, and males show site fidelity at their own marks and defend them aggressively against other males. Furthermore, Ur males increase marking activity in the presence of females and when fed honey (Cooper and King, 2015). Virgin females prefer areas where multiple males have marked over areas where a single male has marked (Wittman et al., 2016). Taken together, these results suggest that Ur males use a substrate-borne sex pheromone in a similar manner as described for Nasonia males.
In the present study we report the identification of the male sex pheromone in Ur and compare several aspects of its pheromone communication with the results previously reported for Nasonia species. In particular, we investigate the production site, the influence of age and mating frequency on male pheromone titers, as well as the influence of previous mating on the females' pheromone response. Furthermore, we perform stable isotope labeling experiments to identify the biosynthetic pathway by which the pheromone is synthesized. Our results demonstrate striking similarities between Ur and the closely related Nasonia species with respect to production site, release behavior and plasticity of the female pheromone response. However, the sex pheromone of Ur males is an isoprenoid, suggesting a biosynthetic switch between fatty acid and isoprenoid metabolism in the Nasonia group.
Materials and Methods
Insects
The Ur strain used in this study was kindly provided by K. Floate and originated from cattle feedlots in southern Alberta, Canada. Ur can be reared conveniently in the lab on freeze-killed host pupae (Floate, 2002). We used freeze-killed pupae of the green bottle fly Lucilia caesar, except the initial comparative analysis and the bioassays with male extract and fractionated pheromone that used Ur that had been reared on the house fly, Musca domestica. Batches of approximately 100 host pupae were transferred to Petri dishes and thawed for 30 min at 24°C in an incubator. Subsequently, about 20 female Urolepis wasps per Petri dish were exposed to the hosts in an incubator at a 16:8 h light dark cycle, 25°C and 60% RH. After 13 days, parasitized fly pupae were kept singly in tubes to ensure virginity of emerging wasps. Mated females were produced by placing a virgin female and a virgin male together and observing them until mating was complete. Except where noted, females used were 3-day-old and allowed to acclimate to the laboratory conditions for at least 30 min before trials. To obtain enough males for the pheromone isolation, all-male broods were produced by using virgin females for parasitization. Because wasps are haplodiploid, virgin females produce only sons.
Pheromone Extraction and Quantification From Wasps
For an initial comparative analysis, 2-day-old virgin males and females (n = 5) were killed by freezing, transferred to a 1.5 ml glass vial equipped with a 200 μl micro insert, and extracted individually for 30 min with 30 μl of dichloromethane. After removal of the wasps, the extracts were analyzed by coupled gas chromatography-mass spectrometry (GC/MS). These analyses revealed that male extracts contained a compound later identified as 2,6-dimethyl-7-octene-1,6-diol (DMOD, 6 see section Results).
To investigate whether the rectal vesicle is the site of DMOD biosynthesis, 2-day-old unmated males (n = 8) were dissected with fine forceps (Dumont 5A) under a stereo microscope. Freeze-killed males were transferred to a drop of distilled water in a clean glass dish. Males were dissected by pulling the abdominal tip and spreading the internal genitalia and the intestinal tract in the water. Genitalia and gut were carefully separated using a fine needle and the yellowish-white spherical rectal vesicle was transferred to the glass vial for extraction.
Because the rectal vesicle is the production site of DMOD (see section Results), we extracted only dissected abdomens for quantitative analyses. To study the age-dependency of the DMOD titers in Ur males, we extracted the abdomens of males of different age (<1 h, 1 day, 2 days, n = 10 per age) in 30 μl dichloromethane containing 10 ng μl−1 methyl undecanoate (Sigma-Aldrich, Deisenhofen, Germany) as an internal standard. For DMOD quantification, peak areas of DMOD were related to the peak area of the internal standard.
To investigate the influence of male mating frequency on their DMOD titers, we extracted the abdomens of 2-day-old males that were virgin or had mated 1, 3 or 5 times prior to pheromone extraction. Ur males exhibit increased marking activity after mating (Cooper and King, 2015), and comparable tests with N. vitripennis revealed decreasing pheromone titers in multiply mated males (Steiner and Ruther, 2009). Each Ur male was exposed in a glass Petri dish (5 cm diameter) to a virgin female until mating was observed. After each mating, the female was removed and the male was kept alone for 10 min in the same glass dish to enable pheromone deposition. A clean glass Petri dish was used for each mating and the respective post-mating period. Each control male was transferred successively to 5 clean glass dishes without exposing him to females. DMOD extraction was done as described above for the age dependency of DMOD titers.
To test bioactivity of natural pheromone extracts and fractions thereof, we extracted batches of 30–50 1- to 3-day-old males for 30 min with dichloromethane (25 μl/wasp). These raw extracts were concentrated under a gentle stream of nitrogen to a concentration of 0.5 male equivalents per μl and used for the bioassay. Additionally, we isolated DMOD from the natural extracts either by size exclusion high performance liquid chromatography (SE-HPLC) (Sperling et al., 2015) or by solid phase extraction (SPE) on silica gel. For SE-HPLC, raw extracts of 30–50 males (see section Pheromone Extraction and Quantification From Wasps) were concentrated to 20 μl and separated on a 300 × 7.5 mm PLgel SE-HPLC column (particle size 5 μm, pore size 100 Å) (Agilent Technologies, Waldbronn, Germany) with DCM as mobile phase as described previously (Sperling et al., 2015). The DMOD fraction eluted between 7.40 and 8.00 min, and purity was controlled by GC/MS (Supplementary Figure S1). A total of 45 μg pheromone was purified. Dichloromethane was evaporated under a gentle stream of nitrogen, and the residue was re-dissolved in deuterated dichloromethane and used for nuclear magnetic resonance (NMR) spectroscopy experiments (see section Nuclear Magnetic Resonance (NMR) Experiments). Subsequently, the same pheromone fraction was diluted with dichloromethane to a DMOD concentration of 100 ng μl−1 and used for the bioassay (see section Bioassays). For SPE, male-derived raw extracts were applied to conditioned 100 mg silica gel cartridges and rinsed twice with 500 μl dichloromethane each. Subsequently, DMOD was eluted twice with 500 μl methanol each. The methanol was carefully evaporated under a gentle stream of nitrogen and the residue was dissolved in dichloromethane, resulting in a final concentration of 0.5 male equivalents per μl. This DMOD fraction was used for the bioassay.
Quantification of Deposited Pheromone
DMOD deposited by individual males was quantified by GC/MS using thermal desorption (TD) sampling as described by Blaul and Ruther (2011). For this purpose, empty 89 × 5 mm inner diameter TD glass tubes were filled at one end with 50 mg of Tenax TA (Supelco, Bellefonte, PA). The adsorbent layer of 25 mm was fixed using fine mesh metal screens (Supelco). One microliter of an internal standard solution containing 100 ng μl−1 methyl undecanoate (Sigma-Aldrich, Deisenhofen, Germany) in methanol was applied to the adsorbent, and the tube was purged for 5 min with a nitrogen flow of 100 ml min−1 to remove the solvent. Subsequently, 1-day-old males were released into the empty side of the adsorption tubes and kept there for 24 h. Males were either virgin (n = 12) or newly mated (n = 10). TD tubes were purged every 6 h for 30 min with charcoal filtered air at a flow rate of 60 ml min−1 (adsorbent upwind) to trap the volatilized DMOD proportion. By this means, losses of DMOD during sampling were kept to a minimum and both the pheromone adsorbed to the substrate and the volatilized proportion could be detected when the tube was thermally desorbed. TD sampling was done using an automated Shimadzu TD20 thermal desorption unit coupled to the QP2010 Plus GC/MS system (Shimadzu GmbH, Duisburg, Germany) as described previously (Blaul and Ruther, 2011).
Stable Isotope Labeling Experiment
To investigate whether mevalonic acid is a precursor for the biosynthesis of DMOD in Ur males, we performed labeling experiments using racemic 3-fold deuterated mevalonic acid lactone (MAL-d3, 4-hydroxy-4-(methyl-d3)tetrahydro-2H-pyran-2-one) (Santa Cruz Biotechnology, Heidelberg, Germany). MAL-d3 is hydrolyzed under physiological conditions to mevalonic acid (Figure 6B) which can be incorporated into products of isoprenoid metabolism (Dewick, 2002). We used 3-day-old parasitoid males on the assumption that they were widely depleted of intrinsically synthesized mevalonic acid and thus would have a higher tendency to use the externally applied precursor. Prior to application, males were cold-sedated on an ice bath, and 0.2 μl of a solution containing 10 μg μl−1 MAL-d3 dissolved in acetone was applied to the abdominal tip (n = 8) as described previously (Blaul et al., 2014). The pure solvent was applied to control wasps (n = 8). Applications were done between 2 and 3 pm, and males were freeze-killed the next morning and extracted for GC/MS analysis as described above.
GC/MS Analysis
Aliquots (1 μl) of extracts and fractions were analyzed on a Shimadzu QP2010 Plus GC/MS system equipped with a non-polar BPX5 or a polar BP-20 capillary column (both columns 30 m x 0.25 mm inner diameter, 0.25 μm film thickness) (SGE Analytical Science Europe, Milton Keynes, UK). Samples were injected at 280°C (BP-20: 240°C) in splitless mode using an AOC 20i auto sampler. Helium was used as the carrier gas at a linear velocity of 40 cm s−1. The initial oven temperature of 80°C was increased at 5°C min−1 to 280°C (BP-20: 240°C) and held for 30 min. Enantioselective GC/MS was performed on chiral ß-Dex 225 column (= 25% 2,3-di-O-acetyl-6-O-tert-butyldimethylsilyl-ß-cyclodextrin in polydimethylsiloxane, 30 m, 0.25 mm inner diameter, 0.25 μm film thickness) (Supelco, Bellefonte, PA). Injector temperature for enantioselective GC was 220°C; the linear velocity of the carrier gas was 30 cm s−1. The temperature program started at 100°C and was increased by 0.25°C min−1 to the final temperature of 220°C. For all analyses, the mass spectrometer was operated in the electron ionization (EI) mode at 70 eV, and the mass range was m/z 35–600. Given that the EI mass spectrum did not show a reasonable molecular ion (see section Results), we analyzed a pheromone extract also by GC (parameter as given above for the non-polar column) coupled to atmospheric pressure chemical ionization mass spectrometry (APCI-MS) using an Agilent high resolution Q-TOF 6540 UHD mass spectrometer (conditions: mass range m/z 60–600; scan rate: 5 spectra s−1; drying gas: 6 L min−1 at 300°C; corona discharge current: 1 μA).
Nuclear Magnetic Resonance (NMR) Experiments
NMR spectra were recorded with Bruker Avance 600 and 800 NMR spectrometers operating at a 1H frequency of 600.03 and 800.2 MHz, respectively. Measurements were performed in a 5 mm TXI (Avance 600) or TCI (Avance 800) cryoprobe. Approximately 45 μg of the sample was dissolved in deuterated dichloromethane (see section Pheromone Extraction and Quantification From Wasps). For the assignment of the chemical shifts standard 1D-NOESY, 2D-COSY, 2D-TOCSY (80 ms mixing time), 2D-ROESY (250 ms mixing time), 2D-[1H,13C] HSQC [1.725 ms mixing time (1JC−H = 145 Hz)], and 2D-[1H,13C] HMBC [50 ms mixing time (10 Hz coupling)], and 1D 13C spectra (including DEPT45 and DEPT135) were recorded at 278 K and carbon frequencies of 150.88 and 201.21 MHz, respectively. The chemical shifts were indirectly referenced to TMS using the residual 1H signal at 5.32 ppm and 13C signal at 53.84 ppm of methylene chloride (Fulmer et al., 2010).
Chemical Synthesis
Synthesis of racemic 2,6-dimethyl-7-octene-1,6-diol started with a catalytic Riley oxidation of racemic linalyl acetate 1 in 50 % yield (Umbreit and Sharpless, 1977). Subsequent Parikh-Doering oxidation furnished aldehyde 3 in 93 % yield (Tojo and Fernandez, 2006; Figure 1). Reduction of unsaturated aldehyde 3 was accomplished by a biomimetic approach. Hantzsch ester 7 was used as an NADH analog in a 1,4-conjugate addition, catalyzed by the trifluoroacetic acid salt of tert-butyl valinate 8 (Martin and List, 2006). The catalyst was prepared by anion exchange of the corresponding commercially available hydrochloride salt, whereas Hantzsch ester 7 was prepared as reported previously (Eey and Lear, 2010). Sodium borohydride reduction gave alcohol 5 in 20% yield. Finally, acetate cleavage by saponification with K2CO3 in methanol/water furnished the target compound 2,6-dimethyl-7-octene-1,6-diol (6) in 63% yield. Full details of the procedures are given in the Electronic Supplementary Material.
Bioassays
Clean arenas were used for each trial (Figure 2). Females were each presented with two filter paper disks simultaneously. Depending on the experiment (see Table 1), one filter paper disk received 2 μl of a male extract (representing one male equivalent), 300 ng of purified natural DMOD (representing approximately the mean pheromone titer of a 1-day-old male), or 300 and 1,000 ng of synthetic racemic DMOD. The other disk was treated with the respective amount of pure dichloromethane (solvent control). The pheromone and solvent sides were switched between trials to minimize any side effects.
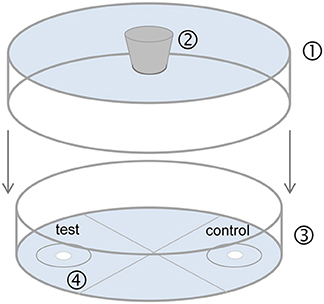
Figure 2. Static two-choice olfactometer used for bioassays. (1) Bottom of a plastic Petri dish (9 cm diameter, 0.8 cm) with a hole (1 cm diameter) in the center for female entrance; (2) rubber stopper; (3) glass Petri dish (9 cm diameter, 1.5 cm height) divided into four quarters of equal size; (4) odor fields with filter paper (1 cm diameter, each disk 5 cm apart, attached with double sided tape) for application of pheromone extract (test) and pure solvent (control). A 2 cm diameter circle was drawn around each filter disk. After application of the test substances, evaporation of solvent and an equilibration time of 5 min, the stopper was removed and the arena was flipped so that the opening was over a female thus allowing the female to enter the olfactometer.
Prior to testing, the female had been tapped out of the glass vial in which she had emerged, onto the clean desk. Depending on the experiment, we tested virgin or mated females and we recorded either the circle around the filter disks entered first by the female (first choice), or we recorded for 5 min the time females spent in the circles around each filter disks (residence time). The residence time was recorded using the scientific software The Observer XT (Noldus, Wageningen, The Netherlands).
In experiments 1 and 2, we tested whether crude extracts and purified DMOD are arresting (residence time, experiment 1) and attractive (first choice, experiment 2) toward virgin females and whether mating triggers a switch in responsiveness as has been shown for females of Nv (Ruther et al., 2007, 2010). In experiment 3 we asked whether the pheromone response of females is switched off after receptivity signaling, like in Nv (Ruther et al., 2010), or whether copulation and sperm transfer are essential for the switch. Copulation was prevented by allowing the male to court the female, but then brushing the male off the female with a fine paint brush just as the male began to back up for copulation. In experiment 4 we tested the response of virgin females to synthetic racemic DMOD.
Statistical Analysis
Pheromone titers of males of different age and mating history were compared by a Kruskal-Wallis H-test followed by pairwise Mann-Whitney U-tests with sequential Bonferroni correction. Deposited DMOD amounts of virgin and mated males were compared by a Mann-Whitney-U-test. Residence time in the test and control fields of the olfactometer was analyzed by a Wilcoxon matched pairs test. First choice between the test and control fields was analyzed by a two-sided binominal test.
Results
The comparative GC/MS analysis of whole body extracts from male and female Ur revealed the presence of a male-specific compound with the linear retention indices 1,350 and 2,155 on non-polar BPX-5 and polar BP-20 stationary phases, respectively (Figure 3). The EI mass spectrum of the compound (Figure 4A) showed a base peak at m/z 71 and the highest mass at m/z 157. A mass difference of only 12 mass units to the next lighter fragment (m/z 145) indicated, however, that m/z 157 does not represent the molecular ion of the compound. Therefore, we analyzed the extract by GC/APCI-MS. This analysis revealed an exact mass of 190.1801 for the quasi molecular ion ([MNH], Figure 4B) matching the empirical formula C10H24O2N. Two subsequent losses of H2O from the ion [MH+] furthermore suggested the presence of two hydroxyl groups.
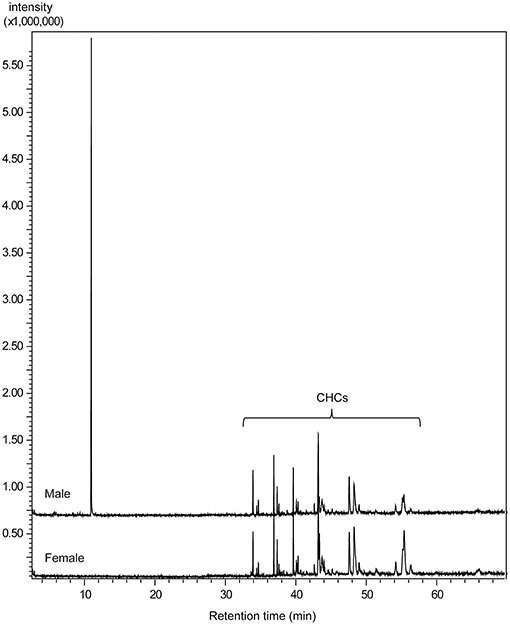
Figure 3. Total ion current chromatograms obtained by GC/MS analysis of dichloromethane extracts from male and female U. rufipes on a non-polar BPX5 capillary column (CHCs = cuticular hydrocarbons).
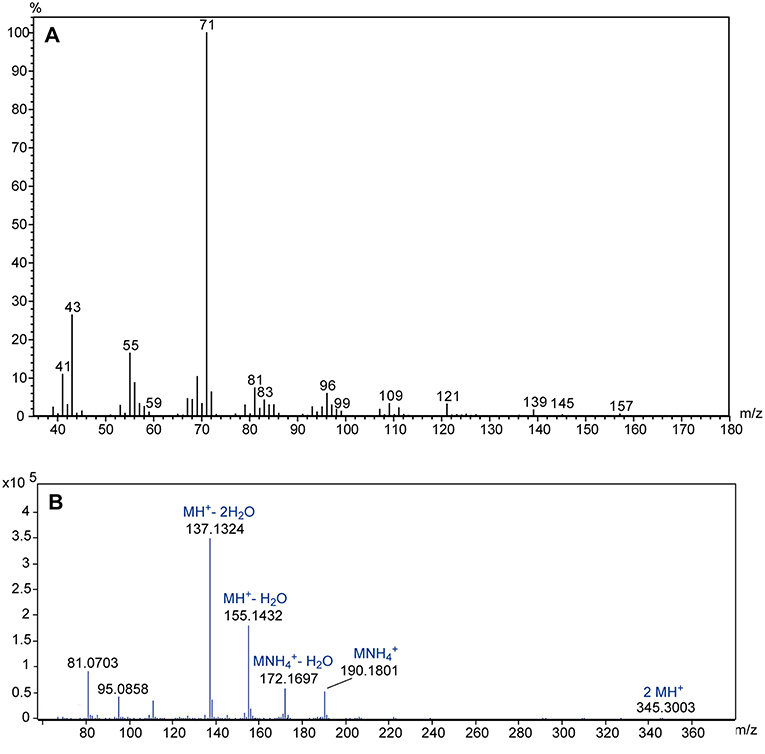
Figure 4. (A) EI mass spectrum of the male-specific compound extracted from U. rufipes; (B) high resolution APCI mass spectrum; suggested empirical formulas are given above the accurate masses of selected diagnostic ions.
[1H, 13C] HSQC with a mixing time of 1.725 ms (1JC−H = 145 Hz) allowed the detection of all carbon resonances except the tertiary carbon in position 6 as well as the resonances of the protons directly coupled to the respective carbon atoms. In the [1H, 13C] HMBC optimized for long range couplings all expected couplings to neighboring carbons as well as the carbon in position 6 could be observed (Supplementary Figure S2). 1H-TOCSY-spectroscopy was used to confirm the assignments leading to the chemical structure of DMOD. The chemical shifts are listed in Table 2. Within the limits of error they are identical to spectra obtained from synthetic DMOD.
With the suggested structure in hand, synthetic material was prepared according to Figure 1. GC/MS analysis of the synthetic product 6 revealed that all four stereoisomers eluted as a single peak on both the non-polar and the polar stationary phase. Mass spectra, 13C- and 1H-NMR-spectra as well as linear retention indices matched the natural product. Enantioselective GC/MS analysis of the racemate on the chiral β-DEX 225 column separated all four DMOD isomers and revealed that only the stereoisomer eluting first is produced by the males (Supplementary Figure S3). The absolute configuration of this compound, however, remains to be established by enantioselective synthesis of the four DMOD stereoisomers.
GC/MS analysis of dissected tissues revealed that Ur males produce DMOD in the rectal vesicle (Supplementary Figure S1).
Male pheromone titers were almost zero in newly emerged males (mean ± SEM: 4.6 ± 2.5 ng) but increased within the first 2 days after emergence (day one: 333 ± 57 ng; day two: 510 ± 81 ng). Although the variability of pheromone titers increased in mated males compared to virgin males, the mating history had no significant effect on the pheromone titers of Ur males and the presence of a female did not influence the deposited pheromone amounts (Figures 5A–C).
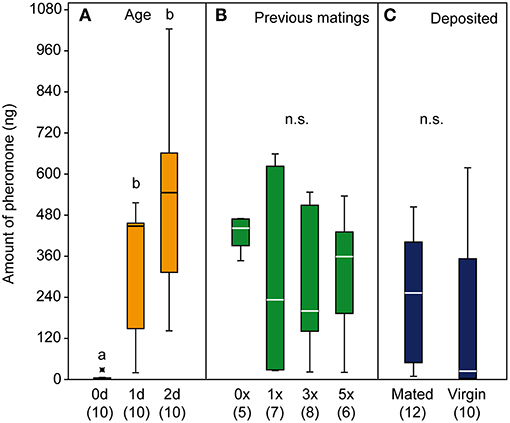
Figure 5. Quantification of the pheromone 2,6-dimethyl-7-octene-1,6-diol (DMOD) from male U. rufipes wasps (A,B) and from male deposits (C). Pheromone titres of 0, 1, and 2-d old males (A) and 2-d old males with 0, 1, 3, and 5 previous matings (B). Amounts of pheromone deposited within 24 h by single 1-d old virgin or newly mated males (C). Box-and-whisker plots show median (horizontal line), 25–75% quartiles (box), maximum/minimum range (whiskers) and outliers (°>1.5 and *>3 x above box height). Different lowercase letters indicate significant differences at p < 0.001; data analysis by Kruskal-Wallis test followed by multiple pairwise Mann-Whitney U-tests with sequential Bonferroni correction.
The mass spectrum of DMOD from MAL-d3 treated males showed an additional ion at m/z 74 indicative of a mass shift of the base peak m/z 71 by three mass units. This shift can be explained by the deuterated methyl group at C6 of the DMOD molecule (Figure 6C). The incorporation rate determined using the ion pair m/z 71/74 was 1.7 ± 0.2 % (mean ± SEM). A second ion indicating the incorporation of the precursor was m/z 46 resulting from a mass shift of the ion m/z 43 by three mass units. Deuterium labeled compounds are characterized by decreased retention times in comparison to the respective fully hydrogenated derivatives (Matucha et al., 1991). Hence, the presence of the deuterium label in the ions m/z 74 and 46 was supported by a retention time decreased by approximately 3 s in comparison to the unlabeled ions m/z 71 and 43, respectively (Figures 6D,E). Due to the relatively low incorporation rates, no diagnostic ions were detected in the mass spectrum of labeled DMOD that would have indicated the incorporation of two deuterated isoprene units.
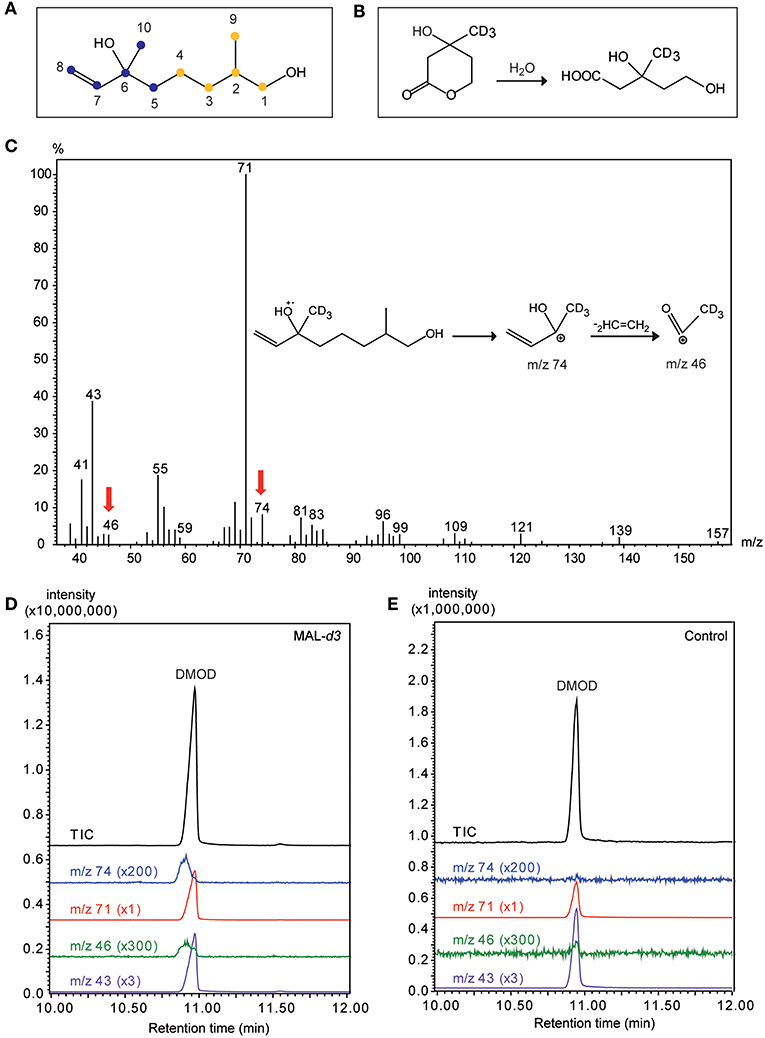
Figure 6. Mevalonic acid lactone is a precursor of the male sex pheromone in U. rufipes. (A) Structure of 2,6-dimethyl-7-octene-1,6-diol (DMOD). The two isoprene units indicating the isoprenoid origin are indicated by differently colored dots. Numbers refer to the NMR-data given in Table 2. (B) Hydrolysis of racemic MAL-d3 to threefold deuterated (±)-mevalonic acid-d3. (C) EI mass spectrum of DMOD extracted from a male U. rufipes after treatment with MAL-d3. Red arrows indicate diagnostic ions resulting from the incorporation of MAL-d3. Insert shows putative structures of the diagnostic ions m/z 74 and m/z 46. (D,E) Total ion chromatograms (TIC) and diagnostic ion chromatograms (43/46 and m/z 71/74, magnification factors given in parentheses) of pheromone extracts from males treated with an acetone solution of MAL-d3 or the pure solvent (Control).
In our behavioral bioassays, virgin females were attracted to and arrested by filter paper disks treated with both crude abdomen extracts and natural DMOD purified by solid phase extraction on a polar adsorbent or size exclusion chromatography, respectively (Figures 7A,B, 8). Likewise, virgin females were attracted by the racemic mixture of all four DMOD stereoisomers (Figures 7C,D) demonstrating that DMOD is indeed used by Ur males as a sex pheromone to attract virgin females. Mated females, however, were no longer attracted to or arrested by the male sex pheromone (Figure 7E). More detailed observations revealed that this switch in responsiveness of mated Ur females is independent of sperm transfer. Females that had signaled receptivity during courtship but were prevented from copulating with the male became nonetheless unresponsive to the male sex pheromone (Supplementary Figure S4).
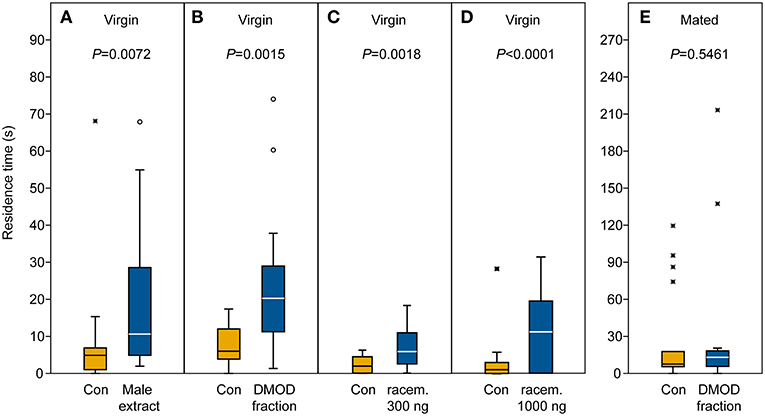
Figure 7. Arrestment of females to the male-derived sex pheromone. Given are the residence times of (A–D) virgin and (E) mated U. rufipes females in the two odor fields of a static two-choice olfactometer when given the choice between a solvent control (Con) and (A) a male-derived pheromone extract and (B,E) natural 2,6-dimethyl-7-octene-1,6-diol (DMOD) purified by SE-HPLC, or a synthetic DMOD racemate (C,D). Box-and-whisker plots show median (horizontal line), 25–75% quartiles (box), maximum/minimum range (whiskers) and outliers [°>1.5 × above box height; *>3 × above box height; data analysis by Wilcoxon matched pairs tests (n = 20)].
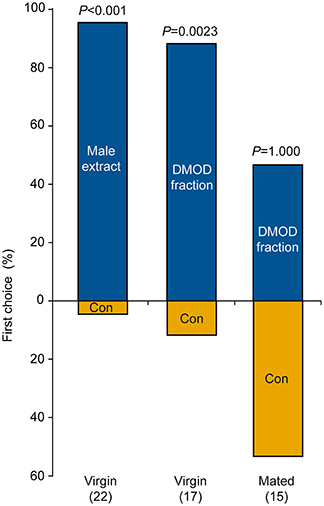
Figure 8. Attraction of females to a male-derived pheromone extract or a natural 2,6-dimethyl-7-octene-1,6-diol (DMOD) fraction purified by solid phase extraction on silica gel. Given are the first choices of virgin or mated U. rufipes females for the two odor fields of a static two-choice olfactometer. Females were given the choice between a solvent control (Con) and one male equivalent of pheromone extract or DMOD fraction; data analysis by a two-sided binominal test (number of replicates in parentheses).
Discussion
The present study demonstrates that Ur males use DMOD as a volatile sex pheromone to attract virgin females, functionally similar to the pheromone used by males of the Nasonia species and T. sarcophagae (Ruther et al., 2007; Niehuis et al., 2013). Thus, the use of male sex pheromones has been shown in all three genera of the “Nasonia group.” Our data and previous studies reveal numerous parallels between the Nasonia and Ur pheromone communication in that (a) the male sex pheromone is produced in the rectal vesicle (Abdel-Latief et al., 2008) and released through the anal orifice by abdomen dipping (Steiner and Ruther, 2009; Cooper and King, 2015); (b) males emerge without the pheromone but titers increase within the first 2 days after emergence (Ruther et al., 2007); (c) pheromone markings are attractive only for virgin females; mated females do not respond to the male pheromone (Ruther et al., 2007); (d) unlike in tephritid fruit flies (Jang, 1995, 2002), this virgin to mated switch in responsiveness is independent of sperm transfer but correlates with receptivity signaling during courtship (Ruther et al., 2010). In Nv, the switch in responsiveness is mediated by oral fatty acid ethyl esters which are released by the male to the female antennae during courtship (Ruther and Hammerl, 2014). Dopamine release in the female brain is the neuromodulator underlying the switch (Lenschow et al., 2018). Further studies are necessary to elucidate whether the same mechanism is involved in Ur's virgin to mated female switch in responsiveness and in previously reported post-mating modifications of mating behavior (King and Miller, 2018).
Despite the many parallels outlined above, Ur diverges in a decisive feature from the Nasonia and Trichomalopsis species studied so far, and this is the biosynthetic pathway by which the male sex pheromone is synthesized. The incorporation of deuterated mevalonic acid lactone into DMOD by Ur males clearly indicates that they synthesize it via the mevalonate pathway. Hence, a switch between two basic biosynthetic pathways (fatty acid and isoprenoid metabolism) has occurred during pheromone evolution in the Nasonia group, while parameters such as production site and release mode remained unchanged.
Which of the two pathways is ancestral and which is derived will remain inconclusive until the phylogenetic relationships among the genera of the Nasonia group are better understood. Phylogenetic analyses of the Nasonia group based on 28S rDNA, cytochrome b and cytochrome oxidase II sequences suggest that the species rich genus Trichomalopsis is rendered paraphyletic by the genera Nasonia and Urolepis, and that T. sarcophagae is the sister group of Nasonia (Burks, 2009). Close relationship between T. sarcophagae and Nasonia is consistent with the qualitative pheromone composition of T. sarcophagae males being the same as that of the Nasonia species, except for Nv having evolved RR as an additional pheromone component. The biosynthetic switch found in the present study might question that Urolepis is a member of the Nasonia group. However, that facts that Ur produces a male-derived sex pheromone and uses it in a similar way like the Nasonia species support the close relationship given that preliminary chemical analyses of other species from the tribus Pteromalini such as Pteromalus puparum, Muscidifurax raptorellus, M. uniraptor, and Dibrachis cavus revealed that these species do not use male-derived sex pheromones (J.R., unpublished data). Further phylogenetic and pheromone analyses of the hitherto unstudied Trichomalopsis and Urolepis species are needed to improve our understanding of the exact species relationships and the pheromone evolution in the Nasonia group and to conclude whether the biosynthetic switch occurred gradually or saltationally (Symonds and Elgar, 2008).
A second feature by which the pheromone communication in Ur differs from Nasonia concerns the quantitative dynamics of pheromone release. Nv males show increased pheromone marking after contact with a female and after copulation (Barrass, 1969; Van Den Assem et al., 1980; Steiner and Ruther, 2009). Consequently, multiply mated males suffer both sperm and pheromone depletion, and pheromone markings of those males are less attractive than those of virgins (Ruther et al., 2009). Like Nv, Ur males make more marks when held with a female and after copulation (Cooper and King, 2015). Unlike Nv, mated and multiply mated Ur males did not have lower pheromone titers than virgins. Mated Ur males also did not deposit a greater amount of pheromone through marking than virgin males did (Figures 5B,C). Thus, mated Ur males make more marks without depositing a greater amount of pheromone. One possibility is that males make shorter marks after mating, despite making more marks. The length of marks varies in Ur, but whether it differs between virgin and mated males has not been examined.
That Nv males, but not Ur males, deposit more pheromone after interaction with a female may result from their differing life histories, which may select for the deposition of lower pheromone amounts per site in Ur. Nv is a gregarious species with typically 15–30 wasps emerging from the same host (Whiting, 1967), and sib-mating is common (Shuker et al., 2004). Females emerge in rapid succession; thus, the presence of one virgin female reliably predicts the very nearby occurrence of others, which will be attracted to the male sex pheromone. Nv males are territorial and defend the exit holes of hosts aggressively against their competitors (Mair and Ruther, 2018) and Nv males do not fly (Weston et al., 1999). In contrast, Ur males have full wings and fly, and Ur generally produces only one wasp per host. However, Ur can be described as quasigregarious (Cooper et al., 2013) where the distribution of their hosts is highly clumped, as can also be the case for Nv hosts (Werren, 1983; King, 1990). A group of hosts may even consist of thousands of hosts in contact with each other. However, hosts also sometimes occur farther apart. As a result, the presence of one virgin female is probably a less reliable predictor of the presence of other nearby virgin females for Ur than for Nv. In addition, Ur males may benefit by conserving pheromone to later mark sites that they fly to in search of additional females.
DMOD is a minor compound in several plant-derived materials, such as rose petals (Knapp and Winterhalter, 2000), birch leaves (Schulz et al., 1997), and wine (Oliveira et al., 2004). Enzymatic conversion of (±)-linalool by the cytochrome P450 CYP76C1 from Arabidopsis thaliana also results in the formation of DMOD (Boachon et al., 2015). In insects, DMOD has only been reported once as a minor compound, in the male “hairpencils” of the butterfly Danaus genutia (Francke et al., 1989), but whether DMOD is involved in the pheromone communication of this species remains unknown. Hence the present study is the first report of DMOD as an insect pheromone. Insect pheromones are typically blends of several compounds enhancing each other additively or synergistically. In Ur, however, there is no evidence for minor compounds being involved in the pheromone function. Our enantioselective GC/MS analysis revealed that only one of the four possible stereoisomers occurs in Ur males. The fact that the racemic DMOD mixture attracted females at both tested doses suggests that the non-natural stereoisomers do not inhibit the females' response to the natural product. As a next step, enantioselective organic syntheses are needed to clarify the absolute configuration of the natural product.
Interspecific sexual interactions are not uncommon in sympatric parasitoid wasp species that parasitize fly pupae. Males of all Nasonia species exhibit some level of courtship to chemical cues present on the cuticle of both conspecific and congeneric females (Buellesbach et al., 2013, 2018; Mair et al., 2017). Males of Nv and T. sarcophagae even respond reciprocally to the cuticular lipids of heterogeneric females (Buellesbach et al., 2018). Ur is partially sympatric with Nv and T. sarcophagae. It remains to be investigated whether there is similar overlap of courtship-related communication with these species and whether the male-derived abdominal sex attractant DMOD has evolved as a prezygotic isolation mechanism to avoid the costs of interspecific mating.
Ethics Statement
Observational experiments with live insects are excluded from legislation in Germany. The studied species is not endangered. Insects used for pheromone identification were freeze-killed prior to extraction. Insects were kept under near natural conditions on freeze-killed hosts.
Author Contributions
JR initiated the study, coordinated the structure elucidation, performed the stable isotope labeling, and enantioselective GC/MS analyses, analyzed data, and wrote the first draft of the manuscript. TW and BK developed and performed the behavioral bioassays. FF and SF performed behavioral bioassays and the quantitative pheromone analyses. WK and HK performed the NMR spectroscopy. JK performed the GC/APCI-MS analysis. CG and SS synthesized the synthetic racemate of 2,6-dimethyl-7-octene-1,6-diol. All authors read and revised the manuscript.
Funding
This research was funded by the Deutsche Forschungsgemeinschaft (DFG) Grant Ru717-10/2 to JR and by the Verband der Chemischen Industrie (VCI) to HK.
Conflict of Interest Statement
The authors declare that the research was conducted in the absence of any commercial or financial relationships that could be construed as a potential conflict of interest.
Acknowledgments
We thank K. Floate for providing a starter culture of U. rufipes and Melanie Schlossberger for rearing the insects and Derdar Dilek for technical assistance during synthesis.
Supplementary Material
The Supplementary Material for this article can be found online at: https://www.frontiersin.org/articles/10.3389/fevo.2019.00026/full#supplementary-material
References
Abdel-Latief, M., Garbe, L. A., Koch, M., and Ruther, J. (2008). An epoxide hydrolase involved in the biosynthesis of an insect sex attractant and its use to localize the production site. Proc. Natl. Acad. Sci. U.S.A. 105, 8914–8919. doi: 10.1073/pnas.0801559105
Ando, T., Inomata, S., and Yamamoto, M. (2004). Lepidopteran sex pheromones. Chem. Pherom. Other Semiochem. I 239, 51–96. doi: 10.1007/b95449
Ashmead, W. H. (1904). Classification of the chalcid flies of the superfamily Chalcidoidea, with descriptions of new species in the Carnegie Museum, collected in South America by Herbert H. Smith. Mem. Carnegie Mus. 1, 225–555. doi: 10.5962/bhl.title.10341
Barrass, R. (1969). Preening and abdomen dipping by the male Mormoniella vitripennis (Walker) (Hymenoptera, Pteromalidae) after courtship. Behaviour 35, 304–312. doi: 10.1163/156853969X00279
Blaul, B., and Ruther, J. (2011). How parasitoid females produce sexy sons: a causal link between oviposition preference, dietary lipids and mate choice in Nasonia. Proc. R. Soc. B Biol. Sci. 278, 3286–3293. doi: 10.1098/rspb.2011.0001
Blaul, B., Steinbauer, R., Merkl, P., Merkl, R., Tschochner, H., and Ruther, J. (2014). Oleic acid is a precursor of linoleic acid and the male sex pheromone in Nasonia vitripennis. Insect Biochem. Mol. Biol. 51, 33–40. doi: 10.1016/j.ibmb.2014.05.007
Blomquist, G. J., Jurenka, R., Schal, C., and Tittiger, C. (2012). “Pheromone production: biochemistry and molecular biology,” in Insect Endocrinology, ed. L.I. Gilbert (San Diego, CA: Academic Press), 523–567.
Boachon, B., Junker, R. R., Miesch, L., Bassard, J. E., Hofer, R., Caillieaudeaux, R., et al. (2015). CYP76C1 (cytochrome P450)-mediated linalool metabolism and the formation of volatile and soluble linalool oxides in Arabidopsis flowers: a strategy for defense against floral antagonists. Plant Cell 27, 2972–2990. doi: 10.1105/tpc.15.00399
Buellesbach, J., Gadau, J., Beukeboom, L. W., Echinger, F., Raychoudhury, R., Werren, J. H., et al. (2013). Cuticular hydrocarbon divergence in the jewel wasp Nasonia: evolutionary shifts in chemical communication channels? J. Evol. Biol. 26, 2467–2478. doi: 10.1111/jeb.12242
Buellesbach, J., Vetter, S. G., and Schmitt, T. (2018). Differences in the reliance on cuticular hydrocarbons as sexual signaling and species discrimination cues in parasitoid wasps. Front. Zool. 15:22. doi: 10.1186/s12983-018-0263-z
Burks, R. A. (2009). Phylogenetics of Pteromalidae and Eulophidae (Hymenoptera: Chalcidoidea) With a Study of Cranial Bridges in Chalcidoidea. Ph.D. Doctor of Philosophy, University of Califormia Riverside.
Cooper, J. L., Burgess, E. R., and King, B. H. (2013). Courtship behavior and detection of female receptivity in the parasitoid wasp Urolepis rufipes. J. Insect Behav. 26, 745–761. doi: 10.1007/s10905-013-9390-8
Cooper, J. L., and King, B. H. (2015). Substrate-borne marking in the parasitoid wasp Urolepis rufipes (Hymenoptera: Pteromalidae). Environ. Entomol. 44, 680–688. doi: 10.1093/ee/nvv017
Darling, D. C., and Werren, J. H. (1990). Biosystematics of Nasonia (Hymenoptera, Pteromalidae) - two new species reared from birds nests in North-America. Ann. Entomol. Soc. Am. 83, 352–370. doi: 10.1093/aesa/83.3.352
Dewick, P. M. (2002). The biosynthesis of C-5-C-25 terpenoid compounds. Nat. Prod. Rep. 19, 181–222. doi: 10.1039/b002685i
Eey, S. T. C., and Lear, M. J. (2010). A bismuth(III)-catalyzed Friedel–Crafts cyclization and stereocontrolled organocatalytic approach to (–)-platensimycin. Org. Lett. 12, 5510–5513. doi: 10.1021/ol102390t
El-Sayed, A. M. (2018). The Pherobase: Database of Pheromones and Semiochemicals. Available online at: http://www.pherobase.com (Accessed September 6).
Floate, K. D. (2002). Production of filth fly parasitoids (Hymenoptera: Pteromalidae) on fresh and on freeze-killed and stored house fly pupae. Biocontrol Sci. Technol. 12, 595–603. doi: 10.1080/0958315021000016252
Francke, W., Bartels, J., Baader, E., Krohn, S., Tengo, J., Schulz, S., et al. (1989). Terpenoids from bark beetles, solitary bees and danaine butterflies. Pure Appl. Chem. 61, 539–542. doi: 10.1351/pac198961030539
Fulmer, G. R., Miller, A. J. M., Sherden, N. H., Gottlieb, H. E., Nudelman, A., Stoltz, B. M., et al. (2010). NMR chemical shifts of trace impurities: common laboratory solvents, organics, and gases in deuterated solvents relevant to the organometallic chemist. Organometallics 29, 2176–2179. doi: 10.1021/om100106e
Gibson, G. P. (2000). Differentiation of the species of Urolepis (Hymenoptera: Chalcidoidea: Pteromalidae), potential biocontrol agents of filth flies (Diptera: Muscidae). Can. Entomol. 132, 391–410. doi: 10.4039/Ent132391-4
Gibson, G. P., and Floate, K. D. (2004). Filth fly parasitoids on dairy farms in Ontario and Quebec, Canada. Can. Entomol. 136, 407–417. doi: 10.4039/n03-006
Jang, E. B. (1995). Effects of mating and accessory gland injections on olfactory-mediated behavior in the female Mediterranean fruit fly, Ceratitis capitata. J. Insect Physiol. 41, 705–710. doi: 10.1016/0022-1910(95)00015-M
Jang, E. B. (2002). Physiology of mating behavior in mediterranean fruit fly (Diptera: Tephritidae): Chemoreception and male accessory gland fluids in female post-mating behavior. Fla. Entomol. 85, 89–93. doi: 10.1653/0015-4040(2002)085[0089:POMBIM]2.0.CO;2
Johansson, B. G., and Jones, T. M. (2007). The role of chemical communication in mate choice. Biol. Rev. 82, 265–289. doi: 10.1111/j.1469-185X.2007.00009.x
Jurenka, R., Blomquist, G. J., Schal, C., and Tittiger, C. (2017). Biochemistry and molecular biology of pheromone production. Refer. Module Life Sci. doi: 10.1016/B978-0-12-809633-8.04037-1 [Epub ahead of print].
King, B. H. (1990). Interspecific differences in host (Diptera, Muscidae) size and species usage among parasitoid wasps (Hymenoptera, Pteromalidae) in a poultry house. Environ. Entomol. 19, 1519–1522. doi: 10.1093/ee/19.5.1519
King, B. H., and Miller, K. A. (2018). Mating status effects on sexual response of males and females in the parasitoid wasp Urolepis rufipes. J. Insect Behav. 31, 144–157. doi: 10.1007/s10905-018-9667-z
Knapp, H., and Winterhalter, P. (2000). Mass spectra and retention indices of monoterpene diols from rose petals. J. Essent. Oil Res. 12, 392–399. doi: 10.1080/10412905.2000.9699544
Lenschow, M., Cordel, M., Pokorny, T., Mair, M. M., Hofferberth, J., and Ruther, J. (2018). The post-mating switch in the pheromone response of Nasonia females is mediated by dopamine and can be reversed by appetitive learning. Front. Behav. Neurosci. 12:14. doi: 10.3389/fnbeh.2018.00014
Mair, M. M., Kmezic, V., Huber, S., Pannebakker, B. A., and Ruther, J. (2017). The chemical basis of mate recognition in two parasitoid wasp species of the genus Nasonia. Entomol. Exp. Appl. 164, 1–15. doi: 10.1111/eea.12589
Mair, M. M., and Ruther, J. (2018). Territoriality and behavioural strategies at the natal host patch differ in two microsympatric Nasonia species. Anim. Behav. 143, 113–129. doi: 10.1016/j.anbehav.2018.07.015
Martin, N. J. A., and List, B. (2006). Highly enantioselective transfer hydrogenation of α,β-unsaturated ketones. J. Am. Chem. Soc. 128, 13368–13369. doi: 10.1021/ja065708d
Matucha, M., Jockisch, W., Verner, P., and Anders, G. (1991). Isotope effect in gas-liquid chromatography of labeled compounds. J. Chromatogr. 588, 251–258. doi: 10.1016/0021-9673(91)85030-j
Niehuis, O., Büllesbach, J., Gibson, J. D., Pothmann, D., Hanner, C., Navdeep, M., et al. (2013). Behavioural and genetic analyses of Nasonia shed light on the evolution of sex pheromones. Nature 494, 345–348. doi: 10.1038/nature11838
Noyes, J. S. (2017). Universal Chalcidoidea Database. World Wide Web Electronic Publication. Available online at: http://www.nhm.ac.uk/chalcidoids (Accessed September 6, 2018).
Oliveira, J. M., Araujo, I. M., Pereira, S. M., Maia, J. S., Amaral, A. J., and Maia, M. O. (2004). Characterization and differentiation of five “vinhos verdes” grape varieties on the basis of monoterpenic compounds. Anal. Chim. Acta 513, 269–275. doi: 10.1016/j.aca.2003.10.020
Raychoudhury, R., Desjardins, C. A., Buellesbach, J., Loehlin, D. W., Grillenberger, B. K., Beukeboom, L., et al. (2010). Behavioral and genetic characteristics of a new species of Nasonia. Heredity 104, 278–288. doi: 10.1038/hdy.2009.147
Ruther, J. (2013). “Novel insights into pheromone-mediated communication in parasitic hymenopterans,” in Recent Advances in Chemical Ecology of Insect Parasitoids, eds. E. Wajnberg and S. Colazza. (Chichester: Wiley-Blackwell), 112–143.
Ruther, J., Hagström, Å. K., Brandstetter, B., Hofferberth, J., Bruckmann, A., Semmelmann, F., et al. (2016). Epimerisation of chiral hydroxylactones by short-chain dehydrogenases/reductases accounts for sex pheromone evolution in Nasonia. Sci. Rep. 6:34697. doi: 10.1038/srep34697
Ruther, J., and Hammerl, T. (2014). An oral male courtship pheromone terminates the response of Nasonia vitripennis females to the male-produced sex attractant. J. Chem. Ecol. 40, 56–62. doi: 10.1007/s10886-013-0372-2
Ruther, J., Matschke, M., Garbe, L. A., and Steiner, S. (2009). Quantity matters: male sex pheromone signals mate quality in the parasitic wasp Nasonia vitripennis. Proc. R. Soc. B Biol. Sci. 276, 3303–3310. doi: 10.1098/rspb.2009.0738
Ruther, J., McCaw, J., Böcher, L., Pothmann, D., and Putz, I. (2014). Pheromone diversification and age-dependent behavioural plasticity decrease interspecific mating costs in Nasonia. PLoS ONE 9:e89214. doi: 10.1371/journal.pone.0089214
Ruther, J., Stahl, L. M., Steiner, S., Garbe, L. A., and Tolasch, T. (2007). A male sex pheromone in a parasitic wasp and control of the behavioral response by the female's mating status. J. Exp. Biol. 210, 2163–2169. doi: 10.1242/jeb.02789
Ruther, J., Steiner, S., and Garbe, L. A. (2008). 4-methylquinazoline is a minor component of the male sex pheromone in Nasonia vitripennis. J. Chem. Ecol. 34, 99–102. doi: 10.1007/s10886-007-9411-1
Ruther, J., Thal, K., Blaul, B., and Steiner, S. (2010). Behavioural switch in the sex pheromone response of Nasonia vitripennis females is linked to receptivity signalling. Anim. Behav. 80, 1035–1040. doi: 10.1016/j.anbehav.2010.09.008
Ruther, J., Thal, K., and Steiner, S. (2011). Pheromone communication in Nasonia vitripennis: abdominal sex attractant mediates site fidelity of releasing males. J. Chem. Ecol. 37, 161–165. doi: 10.1007/s10886-010-9898-8
Schulz, S., and Francke, W. (2010). “Pheromones of terrestrial invertebrates,” in Comprehensive Natural Products Chemistry, eds. L.N. Mander and H.W. Liu (Oxford: Elsevier), 153–223.
Schulz, S., Gross, J., and Hilker, M. (1997). Origin of the defensive secretion of the leaf beetle Chrysomela lapponica. Tetrahedron 53, 9203–9212. doi: 10.1016/s0040-4020(97)00618-2
Shuker, D. M., Reece, S. E., Whitehorn, P. R., and West, S. A. (2004). Sib-mating does not lead to facultative sex ratio adjustment in the parasitoid wasp, Nasonia vitripennis. Evol. Ecol. Res. 6, 473–480.
Skovgard, H., and Jespersen, J. B. (2000). Seasonal and spatial activity of hymenopterous pupal parasitoids (Pteromalidae and Ichneumonidae) of the house fly (Diptera: Muscidae) on Danish pig and cattle farms. Environ. Entomol. 29, 630–637. doi: 10.1603/0046-225x-29.3.630
Smadja, C., and Butlin, R. K. (2009). On the scent of speciation: the chemosensory system and its role in premating isolation. Heredity 102, 77–97. doi: 10.1038/hdy.2008.55
Smith, L., and Rutz, D. A. (1991). Seasonal and relative abundance of hymenopterous parasitoids attacking house fly pupae at dairy farms in central New York. Environ. Entomol. 20, 661–668. doi: 10.1093/ee/20.2.661
Sperling, S., Kühbandner, S., Engel, K. C., Steiger, S., Stökl, J., and Ruther, J. (2015). Size exclusion high performance liquid chromatography: re-discovery of a rapid and versatile method for clean-up and fractionation in chemical ecology. J. Chem. Ecol. 41, 574–583. doi: 10.1007/s10886-015-0584-8
Steiner, S., and Ruther, J. (2009). Mechanism and behavioral context of male sex pheromone release in Nasonia vitripennis. J. Chem. Ecol. 35, 416–421. doi: 10.1007/s10886-009-9624-6
Stenseng, L., Skovgard, H., and Holter, P. (2003). Life table studies of the pupal parasitoid Urolepis rufipes (Hymenoptera: Pteromalidae) on the house fly Musca domestica (Diptera: Muscidae) in Denmark. Environ. Entomol. 32, 717–725. doi: 10.1603/0046-225x-32.4.717
Symonds, M. R. E., and Elgar, M. A. (2008). The evolution of pheromone diversity. Trends Ecol. Evol. 23, 220–228. doi: 10.1016/j.tree.2007.11.009
Symonds, M. R. E., Moussalli, A., and Elgar, M. A. (2009). The evolution of sex pheromones in an ecologically diverse genus of flies. Biol. J. Linn. Soc. 97, 594–603. doi: 10.1111/j.1095-8312.2009.01245.x
Symonds, M. R. E., and Wertheim, B. (2005). The mode of evolution of aggregation pheromones in Drosophila species. J. Evol. Biol. 18, 1253–1263. doi: 10.1111/j.1420-9101.2005.00971.x
Tillman, J. A., Seybold, S. J., Jurenka, R. A., and Blomquist, G. J. (1999). Insect pheromones - an overview of biosynthesis and endocrine regulation. Insect Biochem. Mol. Biol. 29, 481–514. doi: 10.1016/s0965-1748(99)00016-8
Tojo, F., and Fernandez, M. I. (2006). Oxidation of Alcohols to Aldehydes and Ketones. New York, NY: Springer-Verlag.
Umbreit, M. A., and Sharpless, K. B. (1977). Allylic oxidation of olefins by catalytic and stoichiometric selenium dioxide with tert-butyl hydroperoxide. J. Am. Chem. Soc. 99, 5526–5528. doi: 10.1021/ja00458a072
Van Den Assem, J., Jachmann, F., and Simbolotti, P. (1980). Courtship behavior of Nasonia vitripennis (Hym., Pteromalidae): some qualitative, experimental evidence for the role of pheromones. Behaviour 75, 301–307. doi: 10.1163/156853980X00456
Walker, F. (1846). List of Specimens of the Hymenopterous Insects in the Collection of the British Museum, Part 1. Chalcidites. British Museum, London. doi: 10.5962/bhl.title.18394
Weiss, I., Roessler, T., Hofferberth, J., Brummer, M., Ruther, J., and Stökl, J. (2013). A nonspecific defensive compound evolves into a competition avoidance cue and a female sex pheromone. Nat. Commun. 4:2767. doi: 10.1038/ncomms3767
Werren, J. H. (1983). Sex-ratio evolution under local mate competition in a parasitic wasp. Evolution 37, 116–124. doi: 10.1111/j.1558-5646.1983.tb05520.x
Werren, J. H., Richards, S., Desjardins, C. A., Niehuis, O., Gadau, J., Colbourne, J. K., et al. (2010). Functional and evolutionary insights from the genomes of three parasitoid Nasonia species. Science 327, 343–348. doi: 10.1126/science.1178028
Weston, R. F., Qureshi, I., and Werren, J. H. (1999). Genetics of wing size differences between two Nasonia species. J. Evol. Biol. 12, 586–595. doi: 10.1046/j.1420-9101.1999.00048.x
Whiting, A. R. (1967). Biology of parasitic wasp Mormoniella vitripennis = Nasonia brevicornis (Walker). Quart. Rev. Biol. 42, 333–406. doi: 10.1086/405402
Wittman, T. N., Miller, K. A., and King, B. H. (2016). Finding prospective mates by the parasitoid wasp Urolepis rufipes (Hymenoptera: Pteromalidae). Environ. Entomol. 45, 1489–1495. doi: 10.1093/ee/nvw136
Keywords: 2,6-dimethyl-7-octene-1,6-diol, biosynthesis, mate finding, evolution, monoterpenoids, parasitoid, sex pheromone, Urolepis rufipes
Citation: Ruther J, Wittman T, Grimm C, Feichtner FS, Fleischmann S, Kiermaier J, King BH, Kremer W, Kalbitzer HR and Schulz S (2019) Male Sex Pheromone of the Parasitoid Wasp Urolepis rufipes Demonstrates Biosynthetic Switch Between Fatty Acid and Isoprenoid Metabolism Within the Nasonia Group. Front. Ecol. Evol. 7:26. doi: 10.3389/fevo.2019.00026
Received: 04 December 2018; Accepted: 23 January 2019;
Published: 12 February 2019.
Edited by:
Astrid T. Groot, University of Amsterdam, NetherlandsReviewed by:
Marjorie A. Lienard, Broad Institute, United StatesAngel Guerrero, Instituto de Química Avanzada de Cataluña (IQAC), Spain
Copyright © 2019 Ruther, Wittman, Grimm, Feichtner, Fleischmann, Kiermaier, King, Kremer, Kalbitzer and Schulz. This is an open-access article distributed under the terms of the Creative Commons Attribution License (CC BY). The use, distribution or reproduction in other forums is permitted, provided the original author(s) and the copyright owner(s) are credited and that the original publication in this journal is cited, in accordance with accepted academic practice. No use, distribution or reproduction is permitted which does not comply with these terms.
*Correspondence: Joachim Ruther, joachim.ruther@ur.de