- 1DGIMI, INRA, University of Montpellier, Montpellier, France
- 2CBGP, INRA, IRD, CIRAD, Montpellier SupAgro, Montferrier-sur-Lez, France
- 3DIASCOPE, INRA, Mauguio, France
- 4AGAP, University of Montpellier, CIRAD, INRA, Montpellier SupAgro, Montpellier, France
- 5UCO, University of Cordoba, Cordoba, Spain
Hyposoter didymator (Hymenoptera, Ichneumonidae) is a generalist solitary endoparasitoid of noctuid larvae. In the present work, we tested whether populations of H. didymator were divided in several genetically distinct taxa as described for many other generalist parasitoid species, and whether differences in H. didymator parasitism rates were explained by the insect host species and/or by the plant on which these hosts were feeding on. The genetic analysis of natural populations collected in different regions in France and Spain on seven different insect hosts and seven different host plants (775 individuals) showed that H. didymator populations belong to a unique single taxon. However, H. didymator seems to be somewhat specialized. Indeed, in the fields it more often parasitized Helicoverpa armigera compared to the other host species collected in the present work. Also, H. didymator parasitism rates in field conditions and semi-field experimental studies were dependent on the host plants on which H. armigera larvae are feeding. Still, H. didymator can occur occasionally on non-preferred noctuid species. One hypothesis explaining the ability of H. didymator to switch hosts in natura could be related to fluctuating densities of the preferred host over the year; this strategy would allow the parasitoid to avoid seasonal population collapses.
Introduction
Endoparasitoids are insect wasps. Free-living when they are adults, they complete their larval development within the body of another insect eventually killing it. Because of their particular lifestyle, they have intricate physiological interactions with their hosts that allow them to optimally exploit host resources (Harvey, 2005). Therefore, many endoparasitoids tend to have narrow host ranges and parasitize one or a few phylogenetically related species, which often share similar biological or ecological characteristics (Godfray, 1994; van Veen et al., 2008). However, there is a continuum in amplitude of host ranges within endoparasitoids, some being highly specialized (e.g., Hyposoter horticola; Lei and Hanski, 1998) and others reported as generalists (e.g., Cotesia marginiventris; Tamò et al., 2006). Generalists can exploit a broad range of hosts, and because of their ability to shift to another host when one host population is at low density, they should have a higher overall fitness compared to specialists in case of fluctuating host resources. On the other side, specialized parasitoids are thought to be more efficient compared to generalists in exploiting a particular host because of the trade-off between host range and host-use efficiency (reviewed in Gagic et al., 2016). According to Loxdale et al. (2011), “generalism is unlikely to be maintained in nature through speciation,” and for many generalist endoparasitoid species, they are in fact a complex of several specialized taxa reproductively isolated and genetically differentiated.
Host range can vary depending on two main parasitoid features: its behavioral responses to hosts (and host environment) allowing successful host detection, and its ability to develop or not in the host. Development will depend on host suitability (e.g., allowing or inhibiting parasitoid development and survival) and quality (e.g., provision of nutritional resources allowing or not the parasitoid to reach optimal body size and development time) which may vary depending on the species, development stage, etc. (Antolin et al., 2006). It also depends on the ability for the parasitoid to regulate certain aspects of host biology. Hence, many endoparasitoids use a large range of strategies to modulate host physiology for their own benefit (i.e., in a way that increases offspring fitness). Better known strategies are injection of immune depressing venom toxins by the ovipositing female (Poirié et al., 2014) or production in female reproductive tract of mutualist virus particles then delivered into the host (Pichon et al., 2015; Strand and Burke, 2015).
We focused here on the Palearctic species Hyposoter didymator (Ichneumonidae; Campopleginae) whose host range includes several closely related moth species within the Noctuidae family. H. didymator is an important biological control agent of greenhouse pests in the whole Mediterranean region. It has been reported in France, Spain, Italy, but also in the Near East, central Asia, and north Africa (http://www.catalogueoflife.org/col/details/database/id/68). In Europe, its published natural hosts are Chrysodeixis chalcites and Lacanobia oleracea (Reudler-Talsma et al., 2007), although H. didymator attacks other species, including Helicoverpa armigera (Carl, 1978; Bar et al., 1979; Torres-Vila et al., 2000), Mythimna loreyi (Sertkaya and Bayram, 2005) or Spodoptera littoralis, a species native to Africa but present in Spain (Hatem et al., 2016). H. didymator can also successfully reproduce in novel hosts in the laboratory and can be maintained successfully on the Nearctic species Spodoptera frugiperda, a non-natural host for this parasitoid (Dorémus et al., 2014). Actually, host suitability and quality for H. didymator appear to be more dependent on the phylogenetic relatedness of the hosts than on a previous encounter between H. didymator and the host species (Harvey et al., 2012). In H. didymator, success of parasitism relies on a mutualist endogenous polydnavirus named Hyposoter didymator Ichnovirus (HdIV). The virus replicates in the female's ovaries and viral particles are injected along with the egg into the host larva during oviposition. Infection by HdIV of host tissues leads to modulation of the host physiology and development to render it suitable for the wasp development (Dorémus et al., 2014).
The present work arises from the observation that H. didymator is widely distributed and currently known as a generalist endoparasitoid of several noctuid larvae feeding on different plants. Our goal was to know whether or not there is a host-associated differentiation in H. didymator. For this purpose, we performed a set of experiments and analyses to test: (i) whether or not populations of H. didymator are divided into several genetically distinct taxa, and (ii) whether H. didymator parasitism rates were linked to host species and/or to the host plants on which these hosts were feeding.
Materials and Methods
Population Genetic Structure
Collections of H. didymator Populations
Collections of H. didymator were undertaken over a period of 5 years (2011–2016) in France and Spain. Depending of the year, the site and the flights of the insect host species, the sampling was done from late April to November (most commonly from June to October). Sampling was performed on several host plants in more than 30 sites in France and 3 sites in Southern Spain. In those sites and host plants, we collected larvae of several noctuid species, mainly H. armigera, Autographa gamma, Spodoptera exigua, and S. littoralis. The characteristics of each sample (geographical location, the insect host from which it emerged and the host plant on which the insect host was collected from) is detailed in Supplemental Table 1.
Because H. didymator is a solitary parasitoid that develops in young larval stages, only early stages (L1 to L4) of noctuid moth larvae were collected. Upon collection, these noctuid larvae were brought to the quarantine laboratory and maintained on their host plants or on artificial diet until parasitoid cocoon formation and adult emergence. The number of adult parasitoids that emerged was recorded for each sampling site.
When adult parasitoids from species other than H. didymator emerged from the noctuid hosts, their genera or species have been roughly identified using COI barcoding (Hebert et al., 2003) when collected for the first time. Briefly, genomic DNA was extracted from adults using Wizard® Genomic DNA Purification Kit (Promega) according to manufacturer instructions. Genomic DNA (gDNA) was used as a template for PCR amplification using COI specific primers (LCO1490: 5′- GGT CAA CAA ATC ATA AAG ATA TTG G−3′ and HC02198: 5′-TAA ACT TCA GGG TGA CCA AAA AAT CA−3′, as described in Folmer et al. (1994). The 50 μl PCR reaction typically contained 2 μl of gDNA (200 ng), 0.25 μl of Taq DNA polymerase (Promega), 10 μl of 5X Taq Buffer (Promega), 2 μl of each primer (10 μM), 4 μl of MgCl2 (50 mM), 1 μl of dNTP (1 mM) and ultrapure water, with the following thermal cycle: initial denaturing for 5 min at 95°C; pairing for 30 cycles (30 s at 95°C, 45 s at 58°C, and 1 min at 72°C); final extension 5 min at 72°C. PCR amplification products were sent for sequencing by Eurofins, and annotated subsequent to a Blastn similarity search against NCBI nr database. The sequences are available in Data Sheet 1.
Genotyping
From the sampling performed between 2011 and 2016, we obtained a total of 775 H. didymator adults (451 females) originated from 8 regions, 7 insect hosts and on 7 host plants (Table 1 and Supplemental Table 1). DNAs were extracted using the DNeasy Blood & Tissue Kit (Qiagen). Each individual was genotyped at the 14 polymorphic microsatellite loci developed by Audiot et al. (2014). They included 10 loci named HD located within the H. didymator genome and 4 loci named Hdiv located within the H. didymator ichnovirus genome. Hdiv6 was within the segment Hd23 (GenBank KJ586309) and overlaps with the HdIV gene Rep1_Hd23, Hdiv7 was within the segment Hd7 (GenBank KJ586326) and was located close to the gene U1_Hd7, Hdiv8 was located at the extremity of the proviral segment Hd4 (GenBank KJ586330) and Hdiv9 was within the segment Hd11 (GenBank KJ586322) and was located between two viral ankyrin genes (Vank3_Hd11 and Vank4_Hd11). The forward primer of each locus was 5′end-labeled with a fluorescent dye: FAM (Eurogentec), VIC, NED or PET (Applied Biosystems) as described in Audiot et al. (2014). The 14 loci were amplified in two multiplex (M1 and M2) PCRs and analyzed in two runs. The PCRs were performed on a Mastercycler ep gradient S (Eppendorf) using the Qiagen Multiplex PCR Kit. They were conducted in 10 μL reaction volume containing the QIAGEN Multiplex PCR Master Mix (1x) (including Taq, dNTPs and a final concentration of 3 mM of MgCl2), 0.2 μM of each primer, and 1 μL of genomic DNA. PCRs started with an initial activation step at 95°C for 15 min, followed by 32 cycles with denaturation at 94°C for 30 s, annealing at 58°C for 1.5 min, extension at 72°C for 1 min and final extension of 60°C for 30 min. The PCR products were diluted 100-fold, then 2 μL of each diluted PCR product were mixed with 18 μL of mix for sequencing (8 μL of GeneScan 500 LIZ Size Standard plus 2 mL of Hi-Di Formamide (Applied Biosystems) prepared for one plate). The samples were separated and detected on an ABI 3130 automated sequencer and analyzed using GeneMapper v.3.7 (Applied Biosystems, USA).
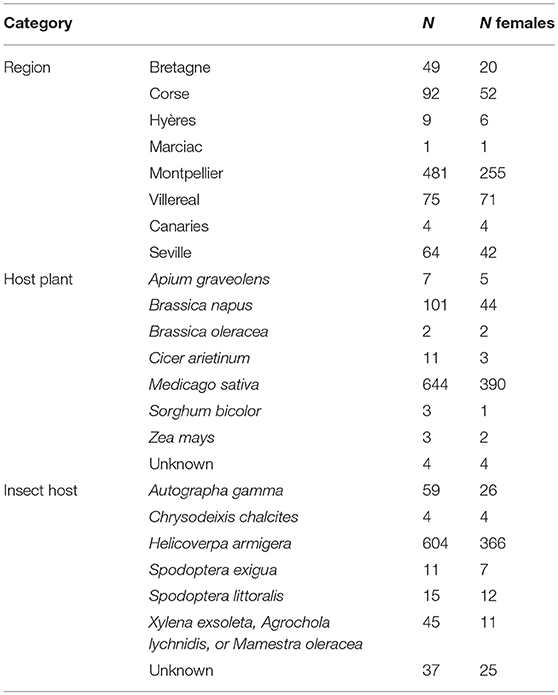
Table 1. Number of total (N) and females (N females) of Hyposoter didymator samples that were genotyped by microsatellite sequencing.
Host Plant Preferences
Experimental Set-Up
This non-choice experiment was conducted with BioQuip Greenhouse Cages (cat#1466E) installed outdoors (within a containment dispositive since H. armigera is classified as a quarantine species in France; see description in Supplemental Figure 1) to prevent contamination and escapes. The dispositive included eight 2-m-high cages covering a surface area of 9 m2 (3 × 3 m) built with iron frames covered with an insect-proof netting (150 μm). The experiment took place at the DIASCOPE INRA research station (Mauguio, France).
Each cage contained three patches—each patch containing several plants—of either alfalfa (Medicago sativa), corn (Zea mays) or chickpeas (Cicer arietinum), probes allowing temperature and hygrometry monitoring and a drip irrigation system. For each host plant species, we performed five replicates over the summer season.
Laboratory Insect Strains
The H. armigera strain originated from adults captured in 2014 in Montpellier region using pheromone and light traps. Adults collected in these traps were brought to the laboratory, placed in cages containing alfalfa (Medicago sativa) plants, and allowed for mating and oviposition. Eggs were collected and, after hatching, larvae were kept on alfalfa plants until being used in the experiment.
The H. didymator DGIMI's laboratory colony originated from wasps issued from H. armigera larvae collected on alfalfa fields in Montpellier region, and maintained on S. frugiperda for more than 50 generations. The Spodoptera frugiperda DGIMI's laboratory strain (corn variant) originated from insects collected in La Réunion, then reared in the DGIMI's insectarium for more than 10 years on Poitout artificial diet (Poitout et al., 1972) at 25°C.
Experiment
On each cage, during the first day of the experiment (day 1), twenty H. armigera larvae (early third instars) were deposited on each patch (leading to a total of 60 larvae per cage). Within each cage, 6 mated 3-days old H. didymator females and 2 H. didymator males were released 6 h after depositing the H. armigera larvae. Six days after, all plants were carefully leafed out to count the number of live, dead or missing host larvae. At the end of the experiment, parasitoids were searched for, and the number of live or dead wasps was recorded. Host larvae which were alive were then brought to the laboratory to calculate the parasitism rate (estimated as the number of formed H. didymator cocoons).
Data Analyses
Population Genetic Structure
Genetic clustering
Bayesian clustering analyses were conducted using the software STRUCTURE (Pritchard et al., 2000), version 2.3.2 (Hubisz et al., 2009). This version of STRUCTURE offers the possibility to use samples characteristics (i.e., geographical location, insect host and host plant on which the adult of H. didymator was originated from) as a prior and to give more prior weight on clustering outcomes that are correlated with these samples characteristics. Analyses were performed using (1) all microsatellite loci (i.e., using HD and Hdiv loci), (2) all 10 HD loci (i.e., excluding the 4 Hdiv loci) and (3) all 4 Hdiv loci (i.e., excluding the 10 HD loci).
We varied the number of putative genetic clusters (K) between 1 and 4 and performed 20 independent runs for each value of K. Each Markov chain was run for 200,000 steps, after a 50,000-step burn-in period, using the admixture model for correlations of allele frequencies across clusters, with the default value for parameter α and the default prior for Fst. As K is increased the most divergent groups are expected to separate into distinct clusters first (Pritchard et al., 2000; Fontaine et al., 2007). To determine the optimal K value, we compared the plots of Ln P(D) and ΔK (Evanno et al., 2005) using STRUCTURE HARVESTER v0.6.94 (Earl and vonHoldt, 2012). In brief, ΔK is a measure of the second order rate of change in the likelihood of K and its maximum indicates the breakpoint in the slope of the distribution of lnP(D) for the different K values tested.
Genetic differentiation
Across all individuals, the overall genetic diversity at each microsatellite locus was calculated using GENEPOP 4.0 (Rousset, 2008). For each sample of H. didymator containing at least 20 females (n = 7 samples), we tested for deviation from Hardy Weinberg equilibrium (HWE) for each locus and over all loci using Fisher's exact test as implemented in GENEPOP 4.0. Because males H. didymator are haploids, these deviations from HWE were tested using females only.
For each locus, across all loci, across HD loci and across Hdiv loci, we estimated the genetic differentiation—using the Fst values (Weir and Cockerham, 1984)—overall samples and between each pair of samples containing at least 20 individuals (n = 12 samples).
We also performed a hierarchical analysis of molecular variance (AMOVA) (Excoffier et al., 1992), based on allele frequency information, using the hierfstat package (Goudet, 2005) and the version 3.4.4 of R (R Core Team, 2015). This analysis allowed us to assess the relative effect of the geographical location, host plants and insect hosts and on H. didymator population structure with respect to total genetic variation. The AMOVA was performed using all loci, using the HD loci and using the Hdiv loci.
Parasitism Rates
Parasitism rates were analyzed with logistic regression models, i.e., generalized linear models (GLMs) and generalized linear mixed models (GLMMs) with binomial error distribution and logit link function. In field collection survey, we constructed a GLM to test the dependence of parasitism rates achieved by H. didymator on the fixed factors host species, host plant species, locality and year of sampling. In the cage experiment, we used a GLMM to test if parasitism rates achieved by H. didymator on the host H. armigera were significantly affected by the fixed factor host plant (chickpeas, alfalfa and corn) using the cage ID as random factor. When overdispersion was detected in the models, we corrected for this by fitting quasi-binomial distributions in order to have a multiplicative overdispersion factor. Model fit was assessed with residual plots. Significance of each fixed factors in GLMs and GLMMs was determined using likelihood ratio tests (LRTs) comparing the full model with and without the factor in question, starting with higher-order interactions (Crawley, 2007). Post-hoc comparisons were carried out using the glht function found in the multcomp package of the R statistical software (Bretz et al., 2010).
A quantitative food web with the data recorded from the field survey of noctuid species and their larval parasitoids was constructed using the bipartite package (Dormann et al., 2016) of the R statistical software. In the food web graphic, the bottom bars represent the insect hosts and the top bars represent parasitoid species. The width of the bars is a proportional representation of the host and parasitoid species abundances.
Results
Population Genetic Structure
Microsatellite markers were used to test whether populations of H. didymator attacking noctuid larvae correspond to a single species or are subdivided into several genetically differentiated taxa.
Most loci were at HWE in all tested populations (see Supplemental Table 2) suggesting a random mating between individuals and a low frequency of null alleles at these loci. Analyses of genetic disequilibrium between the 14 loci indicate that, except HD59 and HD90, they segregate independently from each other (see Supplemental Table 3)—hence, the information given by each locus on the population genetic structure is, with one exception, independent from each other.
The genetic differentiation between the 12 populations with at least 20 individuals (i.e., with enough power to test the occurrence of a genetic differentiation between them), although significantly different from zero, was very low. The Fst value overall populations and overall loci equaled 0.0068 and the pairwise Fst values ranged between -0.0113 and 0.0247 (see Supplemental Table 4). The most differentiated population was the population from Corsica (which emerged from H. armigera collected on alfalfa). The Fst values between this population and the 11 other populations varied between 0.0073 and 0.0247. The Fst values of all the other pairwise comparison, except one, was < 0.001.
The overall genetic differentiation at the 4 Hdiv loci was higher than at the 10 HD loci (Fst = 0.0176 vs. 0.0031). The pairwise comparisons, showed that the higher differentiation of the Corsican population from the other populations was due to a higher level of differentiation at the Hdiv than at the HD loci. Indeed, overall Hdiv loci, Fst values between this population and the 11 other populations varied between 0.0376 and 0.0754, whereas the pairwise Fst values overall HD loci were all < 0.0075.
Accordingly, the results of Bayesian population structure analyses did not reveal any significant genetic differentiation, be when considering all loci, HD loci or Hdiv loci. The estimated logarithm of likelihood for data analyzed with Structure was highest for K = 1 (Figure 1). Indeed, the LnP(D) plot only shows a strong drop off in model fit after K = 2. For K > 1, none of the 775 individuals could be assigned to a given cluster as each individual had a similar probability of belonging to each cluster. The ΔK also suggested only one discernible grouping was present within the data.
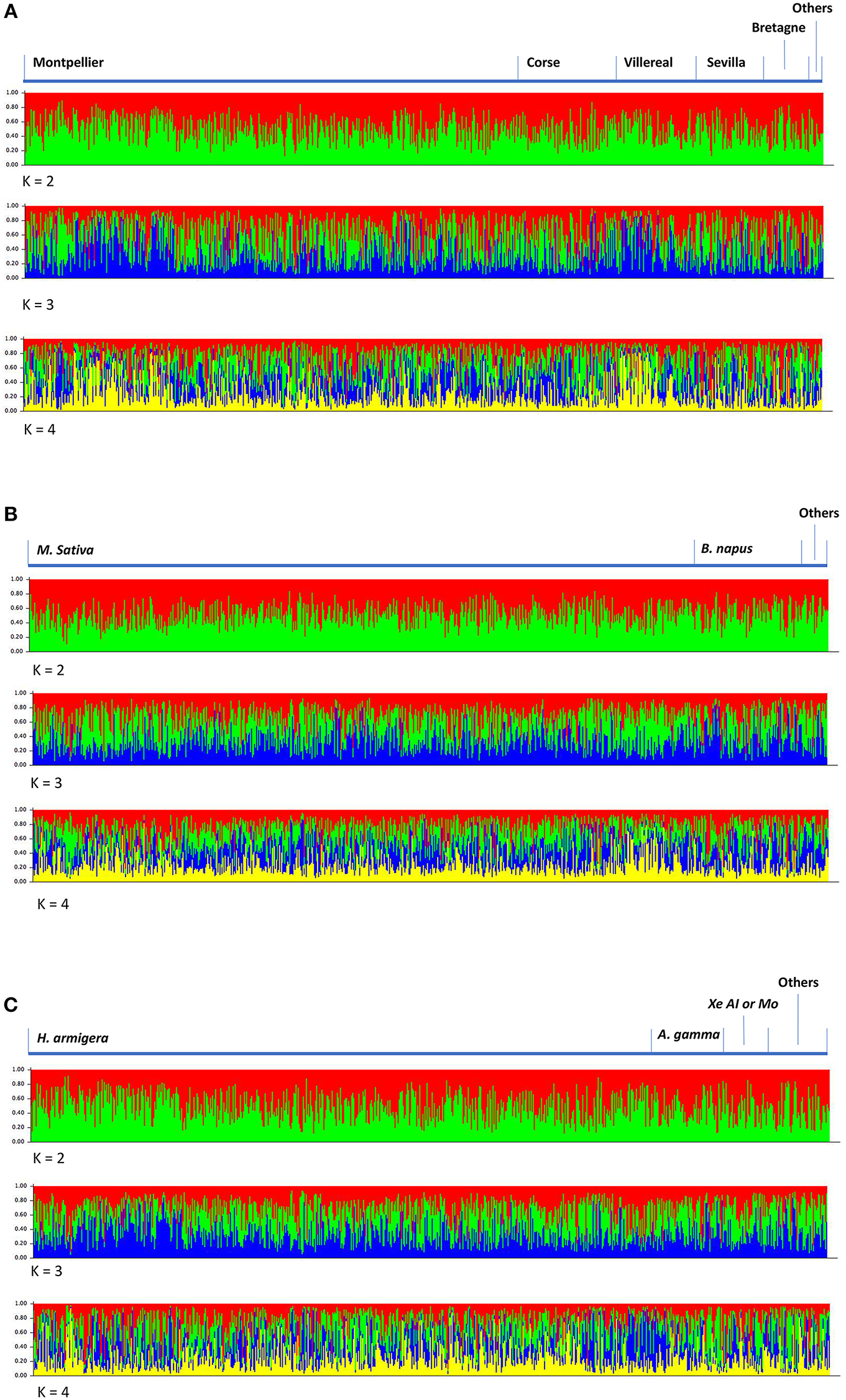
Figure 1. H. didymator population structure from Bayesian STRUCTURE analyses using 14 microsatellite loci for different values of k. Analyses were carried on depending on wasp geographic origin (A), host plant (B) or host species (C).
Finally, the AMOVA analyses showed that the variation between samples collected on different locations, different host plants and different insect host explained, respectively only 3.3, 0.8, and 0.2% of the total variance while the variation among individuals within samples and within individuals explained 9.8 and 85.9% of the total variation, respectively (Table 2). The analyses restricted to HD or Hdiv gave rather similar results (Table 2).
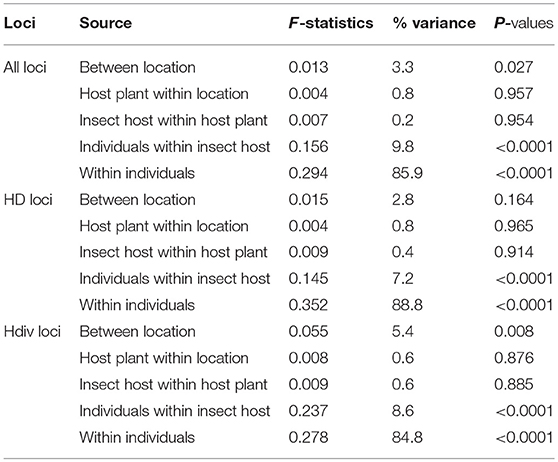
Table 2. AMOVA analysis results. HD loci are located within Hyposoter didymator genome excluding viral sequences; Hdiv loci are within integrated viral sequences.
Hence, all samples of H. didymator collected on different insect hosts and/or on the different host plants appears to belong a single genetic cluster.
Parasitism Rates in Natural Conditions
Parasitism rates by H. didymator varied greatly in the different sampling sites (GLM, χ2 = 590.57, df = 5, p < 0.001) and years (χ2 = 32.81, df = 4, p < 0.001), ranging from 0 to almost 60% (Supplemental Table 1), and were significantly affected by both the host species (χ2 = 520.39, df = 4, p < 0.001) and the host plant (χ2 = 465.77, df = 9, p < 0.001). The parasitism rate was indeed higher on H. armigera (~10.4%) than on all the other host species collected during this survey (Figure 2A). The parasitism rates on A. gamma and S. exigua were very low (~1.5%) but significantly higher than on S. littoralis (0.2%).
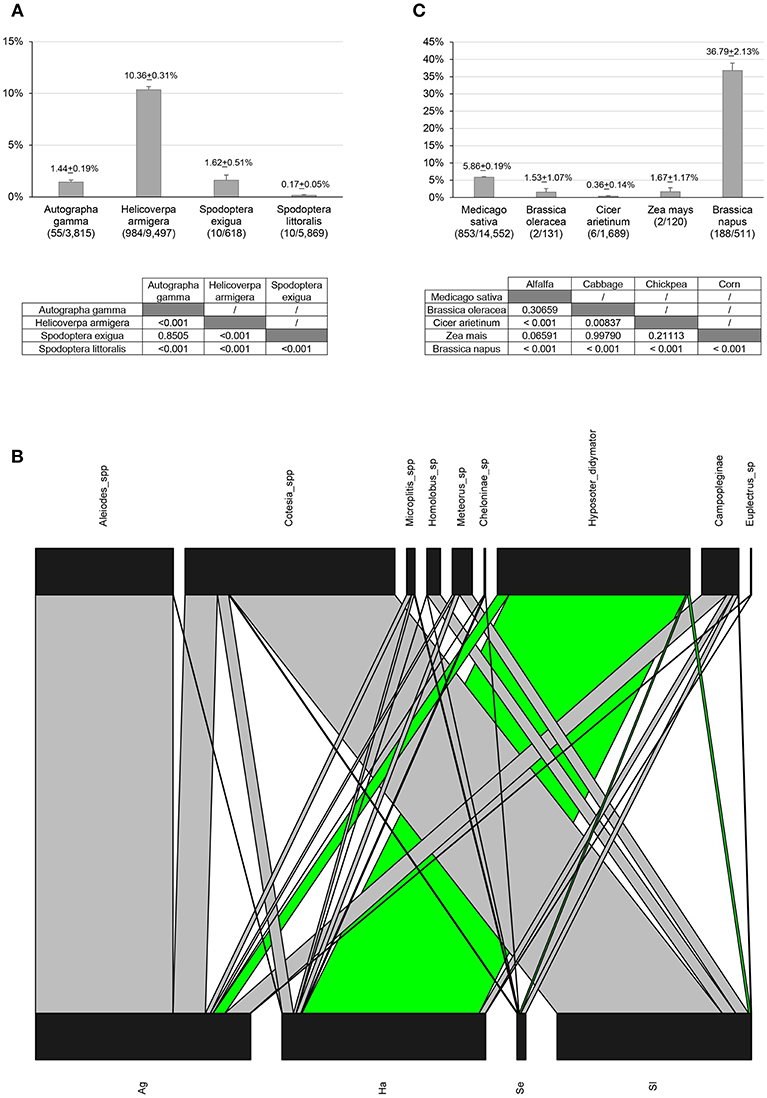
Figure 2. Natural parasitism survey. (A) Parasitism rates (%±SE) by H. didymator for 4 noctuid species. Statistical analyses are indicated below the graph (parasitism rates pairwise comparisons). (B) Quantitative food-web network: larval parasitoid communities interacting with H. didymator. The bottom bars represent the host species (Ag= Autographa gamma, Ha= Helicoverpa armigera, Se= Spodoptera exigua, Sl= Spodoptera littoralis) whereas the top bars represent parasitoid species. The width of the bars is a proportional representation of species abundances. The host-parasitoid interactions involving H. didymator are depicted in green. (C) Parasitism rates (%±SE) by H. didymator for 5 different host plants. Statistical analyses are indicated below the graph (parasitism rates pairwise comparisons). For A and C: the number of parasitized caterpillars relative to the number of caterpillars collected in the fields is indicated in parentheses at the bottom of the graph axis; refer to the Supplemental Table 1 for details on the collected samples.
Hyposoter didymator was also the parasitoid species that emerged the most frequently from H. armigera parasitized larvae collected in the fields, even though a whole cohort of other parasitoid species (including tachinids) emerged from this species (Figure 2B; Supplemental Table 5). Conversely, H. didymator appeared to be only occasional on A. gamma, which was mainly parasitized by braconids from the Aleiodes genus. Similarly, S. littoralis was mainly parasitized by braconids from the Cotesia, Meteorus and Homolobus genera and only in a few cases by H. didymator (Supplemental Table 5).
Regarding the host plant, H. didymator seemed to preferentially parasitize noctuids feeding on rapeseed than those feeding on other crops, with parasitism rate reaching 37% (Supplemental Table 6). Hosts were slightly more often parasitized on alfalfa (~6%) than on corn and cabbage (1–2%). Finally, noctuid larvae captured on chickpeas displayed the lowest parasitism rates (>0.5%). Regarding the host plant (Figure 2C), parasitism rates were higher when caterpillars were feeding on rapeseed (36.8%) than on alfalfa (5.9%) or cabbage and corn (1.5 and 1.7~2%, respectively).
Hence, although all samples of H. didymator apparently belong to a single species, this parasitoid probably does not parasitize noctuid larvae at random: indeed, parasitism rates observed in natural conditions strongly suggest host plant and insect host preferences.
Host Plant Preference
Results obtained on plants placed within cages indicate a significant effect of the host plant on parasitism rates (GLMM, χ2 = 67.98, df = 2, p < 0.001). Parasitism rates of H. armigera larvae (Figure 3; Supplemental Table 7) were significantly higher on alfalfa (~82%) than on corn (Z = 4.91, p < 0.001) and chickpeas (Z = 7.87, p < 0.001). H. armigera larvae feeding on corn plants were also significantly more parasitized (~53%) than on chickpeas (~7%) (Z = 5.11, p < 0.001). The observed differences in parasitism rates among host plants are in agreement with the H. didymator host plant preference observed in natura.
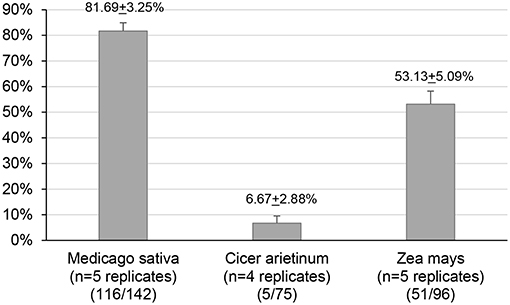
Figure 3. Host plant preference. Helicoverpa armigera larvae total parasitism rates (%±SE) by Hyposoter didymator depending on the host plant on which H. armigera larvae were feeding. The number of parasitized caterpillars relative to the number of caterpillars recovered in the experiment (all replicates merged) is indicated in parentheses at the bottom of the graph axis.
Discussion
Hyposoter didymator is currently described in the literature as a generalist parasitoid able to infest the larvae of several noctuid species in nature and to develop indifferently in a range of noctuid hosts in laboratory conditions. In one hand, our results were in line with this characteristic. In addition, we show that, at least in western Europe (France and Spain) and on the 7 insect hosts collected in this work, H. didymator populations are not subdivided in several genetically differentiated taxa but rather belong to a unique single taxon. This feature is somewhat different from other examples of insect species feeding on multiple hosts. Indeed, many insect species which were thought to be generalists turned out to actually be a complex of several genetically differentiated taxa more or less specialized on a specific host or set of hosts. This was true in many phytophagous insect genera like the moths Ostrinia (Malausa et al., 2007; Bourguet et al., 2014) and Plutella (Perry et al., 2018), the flies Ragholetis (Feder et al., 1999; Xie et al., 2008) or the aphid Acyrthosiphon pisum (Peccoud and Simon, 2010). This feature has also been documented for several species of parasitoid tachninid flies (Smith et al., 2007). In the case of Eupelmus vesicularis, a chalcid wasp long considered as one of the most polyphagous species, with more than 200 hosts from 4 insect orders, it was rather a problem of identification due to morphological similarities. Indeed, the species previously named E. vesicularis turned out to be actually a group of at least five species (Fusu, 2017). Cryptic species have been also identified among braconid species parasitizing lepidopteran [e.g., Cotesia melitaearum and C. acuminata (Kankare et al., 2005) and Cotesia flavipes complex (Muirhead et al., 2012)] or aphids [e.g., Aphelinus varipes species complex (Heraty et al., 2007) and Aphidius matricariae and A. urticae (Derocles et al., 2016)]. In the case of parasitoids, specialization is usually related to the host species use and there is often a lack of genetic structure related to host plant species (reviewed in Saskya van Nouhuys, 2016). As molecular studies progresses, it turns out that only a few parasitoid species appear to be true generalists and most parasitoid species have actually a narrower host range than originally assumed. However, some parasitoid species are described as generalists and may be able to switch regularly between different host species. For instance, the same genetic population of the egg parasitoid Ooencyrtus pityocampae can alternate seasonally between two hosts belonging to two different orders, the moth Thaumetopoea wilkinsoni and the bug Stenozygum coloratum (Samra et al., 2015).
Our results indicated that the overall genetic differentiation at the 4 Hdiv polydnavirus loci was higher than at the 10 HD nuclear loci. Polydnavirus proteins are directly subjected to host physiological resistance mechanisms, so polydnaviral genes were hypothesized to be potential good markers of parasitoid specialization (Whitfield et al., 2018). The role of polydnaviruses in parasitoid local adaptation has previously been reported for the braconid Cotesia sesamiae. Although C. sesamiae was initially considered as a generalist species developing on several lepidopteran hosts, two taxa of C. sesamiae were identified in Kenya that vary in their capacity to overcome the resistance of the lepidopteran host, a feature that was linked to allelic variations in viral genes (Branca et al., 2011; Kaiser et al., 2017; Gauthier et al., 2018). Although not differentiated in several genetically differentiated taxa, H. didymator probably does not parasitize all noctuid species indifferently and part of this specialization might be due, like C. sesamiae, to polydnaviruses, explaining a higher level of genetic differentiation at Hdiv loci.
Based on the results of the parasitism rates recorded in natural populations, we can hypothesize that H. didymator actually do have preferences for some insect hosts notably for H. armigera. Although not done here, due to problems in maintaining a H. armigera strain in the laboratory, further studies on H. didymator life history traits on this host species compared to others will be necessary.
According to other studies, H. didymator displays some preferences to and has a better development—i.e., a higher fitness—in some noctuid species than others. For instance, the percent of adult emergence was higher in H. armigera compared to S. littoralis even using H. didymator adults emerged from parasitized S. littoralis (Hatem et al., 2016). In the same line, Reudler-Talsma et al. (2007) found a better larval development and a bigger adult size when H. didymator parasitized C. chalcites compared to S. exigua. Differences in life history traits and therefore in fitness on different hosts is a classical feature in parasitoid species. Such differences have been reported in several braconid species: Psytallia cosyrae (Mohamed et al., 2003), Cotesia glomerata (Harvey, 2000), Bracon hebetor (Ghimire and Phillips, 2010), or Aphidius ervi (Velasco-Hernández et al., 2017). Similarly, the ichneumonid Venturia canescens that emerged from Ephestia kuehniella were larger compared to those that emerged from Plodia interpunctella (Jones et al., 2015). Parasitoids develop inside a single host which provides their exclusive nutritional and physiological environment. Therefore, parasitoid fitness is closely linked to the innate host quality, which depends on the host species, size, developmental stage and on the plant on which it feeds (Ode, 2006).
Overall, our findings are in agreement with previous results suggesting that H. armigera is indeed a preferential host of H. didymator. For instance, in Spain, although some other parasitoid wasp species were detected, it was the parasitic complex of H. didymator—Cotesia kazak that parasitized more than 95% of H. armigera larvae in tomato crops (Torres-Vila et al., 2000). Similar results were obtained in Greece and Bulgaria (Carl, 1978). In Israel, H. didymator is similarly the most abundant parasitoid of H. armigera populations infesting cotton (Bar et al., 1979). H. didymator distribution covers Europe, and is not reported in Africa, except for Tunisia (https://cran.r-project.org/web/packages/bipartite/bipartite.pdf). The cotton bollworm H. armigera is an indigenous species for Africa and present in a large part of Europe (https://gd.eppo.int), where a single species is described (Colvin et al., 1994) although the population structure is still poorly resolved for this pest (Behere et al., 2007). Our and others' data indicate that H. armigera main parasitoid in southern Europe is H. didymator whereas in Africa, the bollworm is parasitized by other species (Van den Berg et al., 1988). Supplementary studies will be necessary to evaluate the evolutionary origin of the association between H. didymator and H. armigera in their common distribution area, and to decipher if H. didymator occurred in Europe before H. armigera and then switched to this new host after this moth established in European regions.
Finally, parasitism by H. didymator is also influenced by the host plant on which their hosts are feeding. In our survey in natural populations, noctuid larvae that fed on rapeseed and alfalfa were more frequently parasitized by H. didymator than larvae collected on other host plants (corn, chickpeas and some vegetables). Accordingly, in our controlled experiments performed in outdoor cages, parasitism rates of H. armigera larvae by H. didymator were significantly influenced by the host plant on which H. armigera were deposited, with higher parasitism rates on alfalfa than on corn or chickpeas. Our results are in agreement with previous studies performed on six host plants (sorghum, sunflower, cotton, soybean, chickpea, and pigeon pea) showing that H. didymator parasitism was the lowest on chickpea and the highest on soybean (Murray et al., 1995). Influence of host plant on parasitism is also a classical feature in host-parasitoid interaction. For instance, using a similar cage experiment, there was no parasitism of H. armigera larvae by the braconid Microplitis demolitor on chickpeas, whereas 60–75% of parasitism was recorded on maize, cotton or soybean (Murray and Rynne, 1994). The host plant complex had also a strong influence on the level of parasitism of Plutella xylostella by Diadegma mollipla (Hym., Ichneumonidae) with a higher proportion larvae parasitized on peas (2.6%) than on cabbage (0.9%) (Rossbach et al., 2006). In the case of H. didymator, the influence of the host plant can be explained by the fact that host larvae are more accessible in alfalfa where they consume the leaves, than in corn, a plant where larvae tend to act as a borer and hide within the stem or the cobs. Alternatively, the results could be explained by differences in odors emitted by the plant species in response to larvae feeding damage. It is known that several parasitoid species use herbivore-induced plant volatiles (HIPVs) to find their hosts (Vinson, 1998; Mumm and Dicke, 2010). In the future, it will be necessary to compare behavior of H. didymator to assess if the wasp is more attracted to the alfalfa plant compared to corn or chickpeas and whether wasp attraction can be explained by differences in the blend of HIPVs among plant species. Low parasitism rates on chickpeas have been previously reported (Murray et al., 1995) and could be related to the production of acidic exudates by the leaves which may be detrimental (repulsive) to the parasitoid. Overall, our results nonetheless suggest the existence of some plant-mediated specialization of H. didymator at the behavioral host location level.
In conclusion, H. didymator does parasitize larvae of several noctuid species feeding on numerous host plants. This polyphagy did not trigger any genetic differentiation at least here in the Western European populations of this wasp. Unlike many species which are in fact a complex of several subdivided populations specialized on different set of hosts, H. didymator sampled in natural populations in France and Spain belong to a unique taxon. This does not mean that this parasitoid is truly generalist, which parasitizes with the same level of efficacy all noctuid species. H. didymator rather appears to be specialized in some species and to occasionally attack other noctuid species on which it is probably maladapted. This might be explained by the ecology of H. didymator. The agricultural areas where H. didymator was collected are variable patchwork landscapes with many fields of different crops adjacent to each other and to natural areas, resulting in discrete habitat types. Hence, there may be large variability in host density and availability in H. didymator ecological habitat. In southern French regions, there are two main H. armigera flights and larvae of this pest are present from May until the end of October. However, in the spring H. armigera populations are low, and in alfalfa, they coexist with other a number of other noctuid species (e.g., A. gamma). This ability to shift to host species others than H. armigera—even if they may be less suitable—may has been selected for as an adaptation to seasonal population collapses.
Author Contributions
A-NV and DB managed the project and analyzed the data. MF managed the insect collections with the help of EV-O, VJ, LM, and AP. PA performed the microsat analyses along with AP and VJ. The host plant preference experiment was done by MF, MV, SM, and MD. Statistical analyses were conducted by AC. The manuscript was written by A-NV and DB with the help of AC, LM, and AP.
Funding
This work has been provided by ANR (ABC-PaPoGen, ref. ANR-12-ADAP-0001) and by the Département Santé des Plantes et Environnement from INRA. Microsatellite data were produced through molecular genetic analysis technical facilities of the Labex Centre Méditerranéen de l'Environnement et de la Biodiversité.
Conflict of Interest Statement
The authors declare that the research was conducted in the absence of any commercial or financial relationships that could be construed as a potential conflict of interest.
Acknowledgments
The authors thanks Romain Reinosa (BTS student), who participated to the outdoor cage experiment, as well as Clotilde Gibard and Gaétan Clabots from the DGIMI insectarium for the insects they have furnished. We thank the quarantine insect platform (PIQ), member of the Vectopole Sud network, for providing the infrastructure needed for pest insect experimentations. Many people helped us to collect noctuids. Special thanks to José Manuel Durán, Laboratorio de Producción y Sanidad Vegetal, Sevilla for his help. We are grateful to the Chambres d'Agriculture de Corse, Bretagne and Var who had informed us on the presence of noctuids in natura and helped in finding the plots. Thanks to Ernesto Fuentes and Primitivo Caballero, Universidad Pública de Navarra who send us cocoons from Canarias. And many thanks to all the producers in France and Spain who allowed us to collect in their fields. Funding of the work has been provided by ANR (ABC-PaPoGen, ref. ANR-12-ADAP-0001) and by the Département Santé des Plantes et Environnement from INRA. Microsatellite data were produced through molecular genetic analysis technical facilities of the Labex Center Méditerranéen de l'Environnement et de la Biodiversité.
Supplementary Material
The Supplementary Material for this article can be found online at: https://www.frontiersin.org/articles/10.3389/fevo.2019.00020/full#supplementary-material
Supplemental Table 1. List of samples collected in different localities in France and Spain. For each sampling are indicated the number of noctuid larvae collected, the number of noctuid larvae that survived once brought to the laboratory, the number of obtained parasitized larvae, and the parasitism rate (estimated as the number of parasitized larvae per number of larvae that survived). Are also indicated the number of H. didymator (Hd) that have been used for genotyping using microsatellite markers (total and number of females). Some of the parasitoid genotyped emerged from cocoons found in the field, or were caught in the fields as adults (indicated by the mention “cocoons/adults found). Slash (/) indicates the samplings for which the exact number of noctuid larvae is not known. na, non-applicable.
Supplemental Table 2. Results showing that all HD and Hdiv microsatellite loci were at Hardy-Weinberg Equilibrium (HWE). Analyses were performed using individuals emerged from H. armigera larvae collected on different locations and different host plants.
Supplemental Table 3. Genetic disequilibrium analyses between the 14 HD and Hdiv microsatellite loci.
Supplemental Table 4. Pairwise FST values for all, HD and Hdiv loci, considering different locations, host plants and noctuid host species. Xe, Al or Mo: Xylena exsoleta, Agrochola lychnidis, or Mamestra oleracea.
Supplemental Table 5. Diversity of parasitoids emerged from the different collected noctuid larvae, Autographa gamma (Ag), Helicoverpa armigera (Ha), Spodoptera exigua (Se) and S. littoralis (Sl), on different host plants.
Supplemental Table 6. Parasitism rates in natural populations, depending on the noctuid host species or on their host plants. Compared to the main text, all noctuid host species collected and all the plants prospected are indicated.
Supplemental Table 7. Plant preference experiments. For each set of experiments for each host plant, are indicated the number of Hyposoter didymator (Hd) still alive at the end of the experiment, the number of recovered Helicoverpa armigera larvae (Ha), the number of parasitized H. armigera larvae and the parasitism percent.
Supplemental Figure 1. Experimental set-up for host plant preference tests.
Data Sheet 1. Barcoding of parasitoids emerged from field-collected noctuid larvae. Above: table with the results for Blastn similarity searches against NCBI nr database. Below: list of COI sequences.
References
Antolin, M. F., Bjorksten, T. A., and Vaughn, T. T. (2006). Host-related fitness trade-offs in a presumed generalist parasitoid, Diaeretiella rapae (Hymenoptera: Aphidiidae). Ecol. Entomol. 31, 242–254. doi: 10.1111/j.1365-2311.2006.00769.x
Audiot, P., Jouan, V., Frayssinet, M., Volkoff, A. N., and Bourguet, D. (2014). Isolation and characterization of microsatellites in the endoparasitic ichneumonid wasp carrying a polydnavirus Hyposoter didymator. Conserv. Genet. Resour. 6, 21–23. doi: 10.1007/s12686-013-0036-0
Bar, D., Gerling, D., and Rossler, Y. (1979). Bionomics of the principal natural enemies attacking Heliothis armigera in cotton fields in Israel. Environ. Entomol. 8, 468–474. doi: 10.1093/ee/8.3.468
Behere, G. T., Tay, W. T., Russell, D. A., Heckel, D. G., Appleton, B. R., Kranthi, K. R., et al. (2007). Mitochondrial DNA analysis of field populations of Helicoverpa armigera (Lepidoptera: Noctuidae) and of its relationship to H. zea. BMC Evol. Biol. 7:117. doi: 10.1186/1471-2148-7-117
Bourguet, D., Ponsard, S., Streiff, R., Meusnier, S., Audiot, P., Li, J., et al. (2014). ‘Becoming a species by becoming a pest' or how two maize pests of the genus Ostrinia possibly evolved through parallel ecological speciation events. Mol. Ecol. 23, 325–342. doi: 10.1111/mec.12608
Branca, A., LE Ru, B. P., Vavre, F., Silvain, J. F., and Dupas, S. (2011). Intraspecific specialization of the generalist parasitoid Cotesia sesamiae revealed by polyDNAvirus polymorphism and associated with different Wolbachia infection. Mol. Ecol. 20, 959–971. doi: 10.1111/j.1365-294X.2010.04977.x
Bretz, F., Hothorn, T., and Westfall, P. H. (2010). Multiple Comparisons Using R. Boca Raton, FL: Chapman and Hall/CRC.
Carl, K. P. (1978). Heliothis Armigera: Parasite Survey and Introduction of Apanteles Kazak to New Zealand. Commonwealth Institute of Biological Control, European Station, Delemont.
Colvin, J., Cooter, R. J., and Patel, S. (1994). Laboratory mating behaviour and compatibility of Helicoverpa armigera (Lepidoptera: Noctuidae) originating from different geographical regions. J. Econ. Entomol. 87, 1502–1506. doi: 10.1093/jee/87.6.1502
Crawley, M. J. (2007). The R Book. Chichester: John Wiley and Sons, Wiley Ltd. doi: 10.1002/9780470515075
Derocles, S. A. P., Plantegenest, M., Rasplus, J. Y., Marie, A., Evans, D. M., Lunt, D. H., et al. (2016). Are generalist Aphidiinae (Hym. Braconidae) mostly cryptic species complexes? Syst. Entomol. 41, 379–391. doi: 10.1111/syen.12160
Dorémus, T., Cousserans, F., Gyapay, G., Jouan, V., Milano, P., Wajnberg, E., et al. (2014). Extensive transcription analysis of the Hyposoter didymator Ichnovirus genome in permissive and non-permissive Lepidoptera host species. PLoS ONE 9:e104072. doi: 10.1371/journal.pone.0104072
Dormann, C. F., Fründ, J., Blüthgen, N., and Gruber, B. (2016). Package ‘Bipartite': Visualizing Bipartite Networks and Calculating Some Ecological Indices. Available online at: http://www.catalogueoflife.org/col/details/database/id/68
Earl, D. A., and vonHoldt, B. M. (2012). STRUCTURE HARVESTER: a website and program for visualizing STRUCTURE output and implementing the Evanno method. Conserv. Genet. Resour. 4, 359–361. doi: 10.1007/s12686-011-9548-7
Evanno, G., Regnaut, S., and Goudet, J. (2005). Detecting the number of clusters of individuals using the software STRUCTURE: a simulation study. Mol. Ecol. 14, 2611–2620. doi: 10.1111/j.1365-294X.2005.02553.x
Excoffier, L., Smouse, P. E., and Quattro, J. M. (1992). Analysis of molecular variance inferred from metric distances among DNA haplotypes: application to human mitochondrial DNA restriction data. Genetics 131, 479–491.
Feder, J. L., Williams, S. M., Berlocher, S. H., McPheron, B. A., and Bush, G. L. (1999). The population genetics of the apple maggot fly, Rhagoletis pomonella, and the snowberry maggot, R. zephyria. Implications for models of sympatric speciation. Entomol. Exp. Appl. 90, 9–24. doi: 10.1046/j.1570-7458.1999.00419.x
Folmer, O., Black, M., Hoeh, W., Lutz, R., and Vrijenhoek, R. (1994). DNA primers for amplification of mitochondrial cytochrome c oxidase subunit I from diverse metazoan invertebrates. Mol. Mar. Biol. Biotechnol. 3, 294–299.
Fontaine, M. C., Baird, S. J., Piry, S., Ray, N., Tolley, K. A., Duke, S., et al. (2007). Rise of oceanographic barriers in continuous populations of a cetacean: the genetic structure of harbour porpoises in Old World waters. BMC Biol. 25, 5–30. doi: 10.1186/1741-7007-5-30
Fusu, L. (2017). An integrative taxonomic study of European Eupelmus (Macroneura) (Hymenoptera: Chalcidoidea: Eupelmidae), with a molecular and cytogenetic analysis of Eupelmus (Macroneura) vesicularis: several species hiding under one name for 240 years. Zool. J. Linn. Soc. 181, 519–603. doi: 10.1093/zoolinnean/zlw021
Gagic, V., Petrović-Obradovi,ć, O., Fründ, J., Kavallieratos, N. G., Athanassiou, C. G., Starý, P., et al. (2016). The effects of aphid traits on parasitoid host use and specialist advantage. PLoS ONE 11:e0157674. doi: 10.1371/journal.pone.0157674
Gauthier, J., Gayral, P., Le Ru, B. P., Jancek, S., Dupas, S., Kaiser, L., et al. (2018). Genetic footprints of adaptive divergence in the bracovirus of Cotesia sesamiae identified by targeted re-sequencing. Mol. Ecol. 27, 2109–2123. doi: 10.1111/mec.14574
Ghimire, M. N., and Phillips, T. W. (2010). Suitability of different lepidopteran host species for development of Bracon hebetor (Hymenoptera: Braconidae). Environ. Entomol. 39, 449–458. doi: 10.1603/EN09213
Godfray, H. C. J. (1994). Parasitoids Behavioral and Evolutionary Ecology Editions. Princeton, NJ: Princeton University Press.
Goudet, J. (2005). HIERFSTAT, a package for r to compute and test hierarchical F-statistics. Mol. Ecol. 5, 184–186. doi: 10.1111/j.1471-8286.2004.00828.x
Harvey, J. A. (2000). Dynamic effects of parasitism by an endoparasitoid wasp on the development of two host species: implications for host quality and parasitoid fitness. Ecol. Entomol. 25, 267–278. doi: 10.1046/j.1365-2311.2000.00265.x
Harvey, J. A. (2005). Factors affecting the evolution of development strategies in parasitoid wasps: the importance of functional constraints and incorporating complexity. Entomol. Exp. Appl. 117, 1–13. doi: 10.1111/j.1570-7458.2005.00348.x
Harvey, J. A., Ximénez de Embún, M. G., Bukovinszky, T., and Gols, R. (2012). The roles of ecological fitting, phylogeny and physiological equivalence in understanding realized and fundamental host ranges in endoparasitoid wasps. J. Evol. Biol. 25, 2139–2148. doi: 10.1111/j.1420-9101.2012.02596.x
Hatem, A. E., Shawer, D., and Vargas Osuna, E. (2016). Parasitism and Optimization of Hyposoter didymator (Hymenoptera: Ichneumonidae) Rearing on Spodoptera littoralis and Helicoverpa armigera (Lepidoptera: Noctuidae). J. Econ. Entomol. 109, 1058–1063. doi: 10.1093/jee/tow028
Hebert, P. D., Cywinska, A., Ball, S. L., and DeWaard, J. R. (2003). Biological identifications through DNA barcodes. Proc. R. Soc. 270, 313–321. doi: 10.1098/rspb.2002.2218
Heraty, J. M., Woolley, J. B., Hopper, K. R., Hawks, D. L., Kim, J. W., and Buffington, M. (2007). Molecular phylogenetics and reproductive incompatibility in a complex of cryptic species of aphid parasitoids. Mol. Phylogenet. Evol. 45, 480–493. doi: 10.1016/j.ympev.2007.06.021
Hubisz, M. J., Falush, D., Stephens, M., and Pritchard, J. K. (2009). Inferring weak population structure with the assistance of sample group information. Mol. Ecol. Resour. 9, 1322–1332. doi: 10.1111/j.1755-0998.2009.02591.x
Jones, T. S., Bilton, A. R., Mak, L., and Sait, S. M. (2015). Host switching in a generalist parasitoid: contrasting transient and transgenerational costs associated with novel and original host species. Ecol. Evol. 5, 459–465. doi: 10.1002/ece3.1333
Kaiser, L., Dupas, S., Branca, A., Herniou, E. A., Clarke, C. W., Capdevielle-Dulac, C., et al. (2017). The Cotesia sesamiae story: Insight into host-range evolution in a Hymenoptera parasitoid and implication for its use in biological control programs. Genetica 145, 455–468. doi: 10.1007/s10709-017-9989-3
Kankare, M., Stefanescu, C., van Nouhuys, S., and Shaw, M. R. (2005). Host specialization by Cotesia wasps (Hymenoptera: Braconidae) parasitising species-rich Melitaeini (Lepidoptera: Nymphalidae) communities in north-eastern Spain. Biol. J. Lin. Soc. 86, 45–65. doi: 10.1111/j.1095-8312.2005.00523.x
Lei, G. C., and Hanski, I. (1998). Spatial dynamics of two competing specialist parasitoids in a host metapopulation. J. Anim. Ecol. 67, 422–433. doi: 10.1046/j.1365-2656.1998.00204.x
Loxdale, H. D., Lushai, G., and Harvey, J. A. (2011). The evolutionary improbability of ‘generalism' in nature, with special reference to insects. Biol. J. Linn. Soc. 103, 1–18. doi: 10.1111/j.1095-8312.2011.01627.x
Malausa, T., Dalecky, A., Ponsard, S., Audiot, P., Streiff, R., Chaval, Y., et al. (2007). Genetic structure and gene flow in French populations of two Ostrinia taxa: host races or sibling species? Mol. Ecol. 16, 4210–4222. doi: 10.1111/j.1365-294X.2007.03457.x
Mohamed, S. A., Overholt, W. A., Wharton, R. A., Lux, S. A., and Eltoum, E. M. (2003). Host specificity of Psyttalia cosyrae (Hymenoptera: Braconidae) and the effect of different host species on parasitoid fitness. Biol. Control 28, 155–163. doi: 10.1016/S1049-9644(03)00099-9
Muirhead, K. A., Murphy, N. P., Sallam, N., Donnellan, S. C., and Austin, A. D. (2012). Phylogenetics and genetic diversity of the Cotesia flavipes complex of parasitoid wasps (Hymenoptera: Braconidae), biological control agents of lepidopteran stemborers. Mol. Phylogenet. Evol. 63, 904–914. doi: 10.1016/j.ympev.2012.03.003
Mumm, R., and Dicke, M. (2010). Variation in natural plant products and the attraction of bodyguards involved in indirect plant defense. Can. J. Zool. 88, 628–667. doi: 10.1139/Z10-032
Murray, D. A. H., and Rynne, K. P. (1994). Effect of host plant on parasitism of Helicoverpa armigera (Lep : Noctuidae) by Microplitis demolitor (Hym. : Braconidae). Entomophaga 39, 251–255. doi: 10.1007/BF02373029
Murray, D. A. H., Rynne, K. P., Winterton, S. L., Bean, J. A., and Lloyd, R. J. (1995). Effect of host plant on parasitism of Helicoverpa armigera (Hubner) (Lepidoptera: Noctuidae) by Hyposoter didymator thunberg (Hymenoptera : Ichneumonidae) and Cotesia kazak (Telenga) (Hymenoptera: Braconidae). J. Aust. Ent. Soc. 34, 71–73. doi: 10.1111/j.1440-6055.1995.tb01284.x
Ode, P. J. (2006). Plant chemistry and natural enemy fitness: effects on herbivore and natural enemy interactions. Annu. Rev. Entomol. 51, 163–185. doi: 10.1146/annurev.ento.51.110104.151110
Peccoud, J., and Simon, J. C. (2010). The pea aphid complex as a model of ecological speciation. Ecol. Entomol. 35, 119–130. doi: 10.1111/j.1365-2311.2009.01147.x
Perry, K. D., Baker, G. J., Powis, K. J., Kent, J. K., Ward, C. M., and Baxter, S. W. (2018). Cryptic Plutella species show deep divergence despite the capacity to hybridize. BMC Evol. Biol. 201818:77. doi: 10.1186/s12862-018-1183-4
Pichon, A., Bezier, A., Urbach, S., Aury, J. M., Jouan, V., Ravallec, M., et al. (2015). Recurrent DNA virus domestication leading to different parasite virulence strategies. Sci. Adv. 1:e1501150. doi: 10.1126/sciadv.1501150
Poirié, M., Colinet, D., and Gatti, J. L. (2014). Insights into function and evolution of parasitoid wasp venoms. Curr. Opin. Insect Sci. 6, 52–60. doi: 10.1016/j.cois.2014.10.004
Poitout, S., Bues, R., and et Le Rumeur, C. (1972). Elevage Sur Milieu Artificiel Simple de deux Noctuelles Parasites du coton Earias insulana et Spodoptera littoralis. Ent. Exp. and Appl. 15, 341–350. doi: 10.1111/j.1570-7458.1972.tb00219.x
Pritchard, J. K., Stephens, M., and Donnelly, P. (2000). Inference of population structure using multilocus genotype data. Genetics 155, 945–959.
R Core Team (2015). R: A Language and Environment for Statistical Computing. R Foundation for statistical computing, Vienna.
Reudler-Talsma, J. H., Elzinga, J. A., Harvey, J. A., and Biere, A. (2007). Optimum and maximum host sizes at parasitism for the endoparasitoid Hyposoter didymator (Hymenoptera: Ichneumonidae) differ greatly between two host species. Environ. Entomol. 36, 1048–1053. doi: 10.1093/ee/36.5.1048
Rossbach, A., Löhr, B., and Vidal, S. (2006). Host shift to peas in the diamondback moth Plutella xylostella (Lepidoptera: Plutellidae) and response of its parasitoid Diadegma mollipla (Hymenoptera: Ichneumonidae). Bull. Entomol. Res. 96, 413–419. doi: 10.1079/BER2006445
Rousset, F. (2008). GENEPOP'007: a complete re-implementation of the GENEPOP software for windows and linux. Mol. Ecol. Resour. 8, 103–106. doi: 10.1111/j.1471-8286.2007.01931.x
Samra, S., Ghanim, M., Protasov, A., Branco, M., and Mendel, Z. (2015). Genetic diversity and host alternation of the egg parasitoid Ooencyrtus pityocampae between the pine processionary moth and the Caper Bug. PLoS ONE 10:e0122788. doi: 10.1371/journal.pone.0122788
Sertkaya, E., and Bayram, A. (2005). Parasitoid community of the loreyi leaf worm Mythimna (Acantholeucania) loreyi: Novel host-parasitoid associations and their efficiency in the eastern Mediterranean region of Turkey. Phytoparasitica 33, 441–449. doi: 10.1007/BF02981393
Smith, M. A., Wood, D. M., Janzen, D. H., Hallwachs, W., and Hebert, P. D. N. (2007). DNA barcodes affirm that 16 species of apparently generalist tropical parasitoid flies (Diptera, Tachinidae) are not all generalists. Proc. Natl. Acad. Sci. U.S.A. 104, 4967–4972. doi: 10.1073/pnas.0700050104
Strand, M. R., and Burke, G. R. (2015). Polydnaviruses: from discovery to current insights. Virology 479-480, 393–402. doi: 10.1016/j.virol.2015.01.018
Tamò, C., Ricard, I., Held, M., Davison, A. C., and Turlings, T. C. J. (2006). A comparison of naive and conditioned responses of three generalist endoparasitoids of lepidopteran larvae to host-induced plant odours. Anim. Biol. 56, 205–220. doi: 10.1163/157075606777304177
Torres-Vila, L. M., Rodríguez-Molina, M. C., Palo, E., Del Estal, P., and y Lacasa, A. (2000). El complejo parasitario larvario de Helicoverpa armigera Hübner sobre tomate en las Vegas del Guadiana (Extremadura). Bol. San. Veg. Plagas 26, 323–333.
Van den Berg, H., Waage, J. K., and Cock, M. J. W. (1988). Natural enemies of Helicoverpa armigera in Africa: A Review. Wallingford, CAB International.
van Nouhuys, S. (2016). Diversity, population structure, and individual behavior of parasitoids as seen using molecular markers. Curr. Opin. Insect Sci. 14, 94–99. doi: 10.1016/j.cois.2016.02.006
van Veen, F. J. F., Müller, C. B., Pell, J. K., and Godfray, H. C. J. (2008). Food web structure of three guilds of natural enemies: predators, parasitoids and pathogens of aphids. J. Anim. Ecol. 77, 191–200. doi: 10.1111/j.1365-2656.2007.01325.x
Velasco-Hernández, M. C., Desneux, N., Ramírez-Martínez, M. M., Cicero, L., and Ramirez-Romero, R. (2017). Host species suitability and instar preference of Aphidius ervi and Aphelinus abdominalis. Entomol. Gen. 36, 347–367. doi: 10.1127/entomologia/2017/0500
Vinson, S. B. (1998). The general host selection behavior of parasitoid Hymenoptera and a comparison of initial strategies utilized by larvaphagous and oophagous species. Biol. Control 11, 79–96. doi: 10.1006/bcon.1997.0601
Weir, B. S., and Cockerham, C. (1984). Estimating F-Statistics for the analysis of population structure. Evolution 38, 1358–1370.
Whitfield, J. B., Austin, A. D., and Fernandez-Triana, J. L. (2018). Systematics, biology, and evolution of microgastrine parasitoid wasps. Annu. Rev. Entomol. 63, 389–406. doi: 10.1146/annurev-ento-020117-043405
Keywords: Hyposoter didymator, Helicoverpa amigera, population genetic structure, host preferences, western European populations
Citation: Frayssinet M, Audiot P, Cusumano A, Pichon A, Malm LE, Jouan V, Vabre M, Malavieille S, Delalande M, Vargas-Osuna E, Bourguet D and Volkoff A-N (2019) Western European Populations of the Ichneumonid Wasp Hyposoter didymator Belong to a Single Taxon. Front. Ecol. Evol. 7:20. doi: 10.3389/fevo.2019.00020
Received: 09 November 2018; Accepted: 22 January 2019;
Published: 19 February 2019.
Edited by:
Casper Nyamukondiwa, Botswana International University of Science and Technology, BotswanaReviewed by:
Antonino Malacrinò, The Ohio State University, United StatesEric George Chapman, University of Kentucky, United States
Copyright © 2019 Frayssinet, Audiot, Cusumano, Pichon, Malm, Jouan, Vabre, Malavieille, Delalande, Vargas-Osuna, Bourguet and Volkoff. This is an open-access article distributed under the terms of the Creative Commons Attribution License (CC BY). The use, distribution or reproduction in other forums is permitted, provided the original author(s) and the copyright owner(s) are credited and that the original publication in this journal is cited, in accordance with accepted academic practice. No use, distribution or reproduction is permitted which does not comply with these terms.
*Correspondence: Denis Bourguet, denis.bourguet@inra.fr
Anne-Nathalie Volkoff, anne-nathalie.volkoff@inra.fr
†These authors share co-first authorship