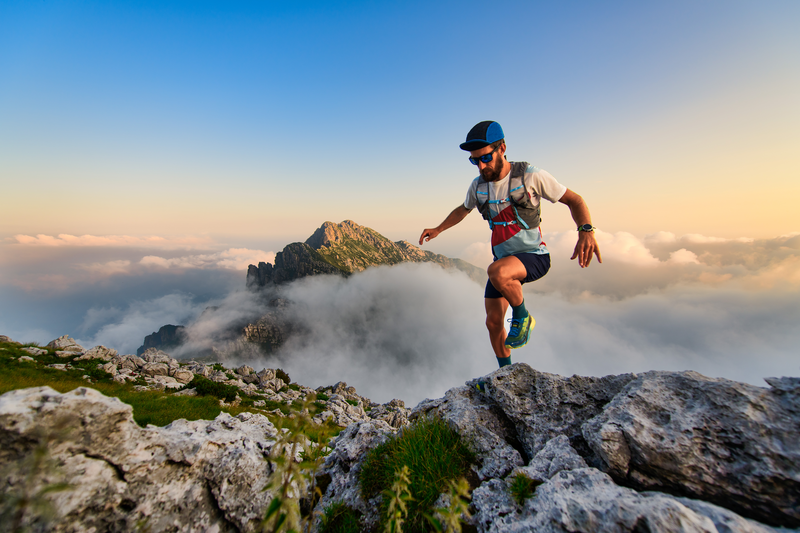
94% of researchers rate our articles as excellent or good
Learn more about the work of our research integrity team to safeguard the quality of each article we publish.
Find out more
ORIGINAL RESEARCH article
Front. Ecol. Evol. , 25 January 2019
Sec. Conservation and Restoration Ecology
Volume 6 - 2018 | https://doi.org/10.3389/fevo.2018.00242
This article is part of the Research Topic How Prides of Lion Researchers are Evolving to be Interdisciplinary View all 12 articles
Across Africa, lions (Panthera leo) are heavily persecuted in anthropogenic landscapes. Trans-disciplinary research and virtual boundaries (geofences) programmed into GPS-tracking transmitters offer new opportunities to improve coexistence. During a 24-month pilot study (2016–2018), we alerted communities about approaching lions, issuing 1,017 alerts to four villages and 19 cattle posts. Alerts reflected geofence breaches of nine lions (2,941 monitoring days) moving between Botswana's Okavango Delta and adjacent agro-pastoral communities. Daily alert system costs per lion were US$18.54, or $5,460.24 per GPS deployment (n = 13). Alert-responsive livestock owners mainly responded by night-kraaling of cattle (68.9%), significantly reducing their losses (by $124.61 annually), whereas losses of control group and non-responsive livestock owners remained high ($317.93 annually). Community satisfaction with alerts (91.8%) was higher than for compensation of losses (24.3%). Study lions spent 26.3% of time monitored in geofenced community areas, but accounted for 31.0% of conflict. Manual alert distribution proved challenging, static geofences did not appropriately reflect human safety or the environment's strong seasonality that influenced cattle predation risk, and tracking units with on-board alert functions often failed or under-recorded geofence breaches by 27.9%. These insufficiencies prompted the design of a versatile and autonomous lion alert platform with automated, dynamic geofencing. We co-designed this prototype platform with community input, thereby incorporating user feedback. We outline a flexible approach that recognizes conflict complexity and user community heterogeneity. Here, we describe the evolution of an innovative Information and Communication Technologies-based (ICT) alert system that enables instant data processing and community participation through interactive interfaces on different devices. We highlight the importance of a trans-disciplinary co-design and development process focussing on community engagement while synthesizing expertise from ethnography, ecology, and socio-informatics. We discuss the bio-geographic, social, and technological variables that influence alert system efficacy and outline opportunities for wider application in promoting coexistence and conservation.
Globally, large predators struggle with the consequences of human population growth and development. An iconic example is the African lion (Panthera leo). Lion numbers and distribution have decreased precipitously over the past century with many regional populations continuing to decline, putting them at risk of local extinction (Riggio et al., 2013; Henschel et al., 2014; Bauer et al., 2015). The situation is exacerbated along protected area boundaries where human retaliation for livestock losses and indiscriminate persecution can inflict heavy losses that reverberate throughout protected areas (e.g., Loveridge et al., 2016). Edge effects have far-reaching demographic consequences for lions (Woodroffe and Frank, 2005) and conflict with people remains the single biggest threat to their persistence. Particularly worrying is the continent-wide use of poison to control lions (Ogada, 2014; Supplementary Data 1) as this indiscriminate method also drives the declines of other endangered biota (Ogada et al., 2016).
Despite decades of applied conflict management research (Trinkel and Angelici, 2016; van Eeden et al., 2018), sustainable coexistence of rural communities with lions has yet to be achieved in many countries (Bauer et al., 2015). The successful mitigation of conflict primarily depends on changes in people's behaviors and risk management (Reddy et al., 2017), requiring trans-disciplinary research and conservation approaches that appropriately reflect the human dimensions of coexistence (Bennett et al., 2017; Pooley et al., 2017). This, inevitably, entails the direct involvement of rural communities in the design and testing of coexistence strategies. Because they bear the risks and costs of coexistence, Africa's communities are the key stakeholder of lion conservation outside protected areas. Mirroring a global omission in biodiversity conservation (Sterling et al., 2017), Africa's communities rarely have direct access to lion monitoring information and are often marginalized during conservation development processes.
Recent advances in Global Positioning System (GPS) tracking technology have revolutionized our understanding of wildlife movements and ecology (Kays et al., 2015). Beyond the mere tracking of fauna, innovative integrations of geofences (i.e., virtual boundaries that can trigger alerts when transgressed), automated data processing, and modern communication networks offer opportunities for use of wildlife tracking technology in various conservation contexts (Wall et al., 2014). For example, geofence applications can be designed to reduce human-induced wildlife mortalities (e.g., Sheppard et al., 2015). Dynamic geofencing can improve human safety by integrating near real-time processing of situational awareness, i.e., the continuous evaluation of relative risk (Zimbelman et al., 2017). In the case of lions and people (and their livestock), conflict typically manifests along well-defined land use and land tenure boundaries such as protected area edges. Simplistically, we can express conflict (the risk of undesirable interactions) as the probability of direct contact between these actors at any point in time. In other words, what is the real-time proximity of lions to people and livestock? Using proximity-based risk rules that are linked to geofences may, therefore, provide an opportunity to reduce the likelihood of conflict between people, their livestock, and lions. Informing people about the presence of lions in anthropogenic landscapes (by geofence triggered early warning) might enable them to exercise the changes in behavior upon which successful coexistence relies (Reddy et al., 2017).
The Okavango Delta lion population in northern Botswana represents one of the last strongholds for the long-term survival of the species (Riggio et al., 2013; Bauer et al., 2015). The Delta's eastern panhandle region constitutes a critical lion conservation area with on-going conflict (Weise et al., 2018), widespread persecution (Supplementary Figure 1) and important dispersal linkages with other lion areas in the Kavango Zambezi Transfrontier Conservation Area (KAZA-TFCA). In this anthropogenic landscape with multiple edges, we tested the efficacy of alerting rural communities about approaching lions to improve human and livestock safety. Here, we reflect critically on experiences from the system's 2-year pilot stage (Figure 1) with daily online data checks, static risk geofences, subjective evaluation of geofence breaches and manual alert distribution. We evaluate pilot study results in terms of conflict, technology performance, community satisfaction and feedback, financial costs, and the ecological implications for human and livestock safety. We provide details of the bio-geographic, social, and technological variables that influence alert efficacy. Following identification of the core challenges of effective early warning, we employed an adaptive, trans-disciplinary research design (Figure 1) to develop a versatile, autonomous, Information and Communication Technologies-based (ICT) lion alert system, capable of delivering near real-time alerts through interactive community interfaces. The evolution of this prototype platform was founded on a co-development strategy with maximum community participation and feedback. Our results highlight the importance of multi-disciplinary research that synthesizes ethnography, ecology, and socio-informatics. Finally, we outline opportunities for the platform's wider application in wildlife conservation.
Figure 1. Timeline of key research activities and lion alert system elements during the pilot study. Numbers in row “Lion GPS tracking” represent monthly sample sizes. Numbers in row “Geofences” indicate which virtual boundaries (see Figure 2) triggered alerts.
Between 13 May 2016 and 12 May 2018 (the pilot study), we studied lions, conflict and cattle (Bos taurus) (Figure 1) across communities living at the boundary of the NG/11 and NG/12 multi-use areas (settlement, agriculture, livestock, and wildlife tourism) located along the northern edge of Botswana's Okavango Delta (Figure 2). The area supports high levels of biodiversity (Ramberg et al., 2006), forms part of UNESCO's 1000th World Heritage Site, Ramsar Site No. 879, and provides critical linkage habitat with protected areas in the KAZA-TFCA. The study area comprised five main villages, 44 remote cattle posts (i.e., small, often seasonal, homesteads with a cattle night enclosure), and intermittent settlements (Figure 2) with ~5,000 resident inhabitants from three ethnic groups, namely the Hambukushu, the Bayeyi, and the Basarwa (Mendelsohn and el Obeid, 2004). The main subsistence activities entail household-specific combinations of agro-pastoralism with small business; most families subsist on < $US 500 (hereafter $) monthly income. Increasing conflict with lions and elephants (Loxodonta africana) significantly impacts agro-pastoral households (Songhurst, 2017; Weise et al., 2018). Livestock are mainly a socio-cultural commodity; most cattle roam freely across unrestricted communal pastures shared with wildlife, and their management is haphazard, with minimal day-time herding (< 10%) and irregular night-time confinement (~40%) in predator-proof enclosures (see Weise et al., 2018 for additional detail). Through herd counts, we estimated a total standing herd of 16,500 cattle in 2017. The Department of Wildlife and National Parks (DWNP) compensates livestock owners for lion-related stock losses at average national cattle market values (Department of Wildlife National Parks, 2013). Owners report livestock losses to the nearest DWNP or police office and compensation is granted following case-specific evaluation of the supporting evidence (for additional procedural detail see Songhurst, 2017).
Figure 2. Design of the lion alert system during the pilot study (May 2016–May 2018) showing the placement of virtual geofences across the study area in the northern Okavango Delta, Botswana. Geofences 1 and 2 were programmed into lion GPS tracking units deployed until end of 2017, Geofence 3 into units deployed from January 2018 onward.
The Okavango Delta experiences annually variable seasonal flooding (Murray-Hudson, 2009), the extent of which strongly influences the socio-ecology of lions (Kotze et al., 2018). We considered three climatic seasons: (1) Wet season (January–April) with rising flood levels, >80% of annual rainfall, and surface water availability in seasonal pans in NG/11; (2) Early dry season (May–August) with a progression from peak Delta flooding to low flood levels in NG/12, no rains, cold winter temperatures, and the drying up of seasonal pans in NG/11; and (3) Late dry season (September–December) with dry seasonal pans, consistently high mid-day temperatures (>35°C), minimal rainfall, and surface water restricted to the last permanent channels in NG/12. Detailed bio-geographic and socio-cultural descriptions are available from Mendelsohn and el Obeid (2004), Pröpper et al. (2015), and Sianga and Fynn (2017). The regional mix of dry savannah woodlands with wetland habitats provides critical functional heterogeneity of seasonal habitats for wild and domestic herbivores (Fynn et al., 2015; Weise et al., 2019).
We tracked nine adult study lions (four females, five males) from different social groups with combined VHF radio-GPS Iridium satellite transponders that enabled near real-time transmission of positional data and that were equipped with on-board geofencing functions. Tagging of specific lions focused on individuals with known or suspected conflict histories and was conducted in the immediate vicinity of communal grazing pastures. All GPS units were programmed to transmit geofence breach and exit SMS messages to the researchers. Transponders weighed < 1.5% of adult body weight and were equipped with automated drop-off mechanisms. Until December 2016, we tracked four lions with Telonics TGW-4570-3 units (Telonics Inc., Mesa, AZ, USA) that recorded and relayed five daily locations, however switching into 2-hourly sampling mode when breaching Geofences 1 or 2 (Figure 2). From December 2016 onwards, we followed lions using Vectronic Vertex Plus v2.1 units (Vectronic Aerospace GmbH, Berlin, Germany) that transmitted GPS locations every 2 hours (h) (Figure 1). The six units deployed in December 2016 were programmed to report Geofence 1 breaches. Following the revision of geofences (see Geofence Placement), the three units deployed in January 2018 were programmed to report Geofence 3 breaches (Figures 1, 2). At any one time, we tracked between three and six social groups simultaneously. In addition, we recorded lion group compositions and identification details (using whisker spot differentiation) during direct field monitoring and from high-resolution photographs that were sourced from wildlife tourism enterprises operating in the study area.
Because spatial outliers often represented lions entering community areas, we calculated seasonal home ranges as 100% Minimum Convex Polygons (MCP) (Mohr, 1947). We computed range metrics with QGIS 2.18 (QGIS Development Team, 2016) using the AniMove 1.4.2 (Boccacci et al., 2014) extension. We calculated home range centroids and percentage overlap with community areas using QGIS geoprocessing tools. For each lion, we also calculated the duration (minutes) between consecutive GPS locations. For any duration < 250 min (nominally 4 h), we calculated the distance between locations using the Haversine formula (Sinnott, 1984), which compensates for the curvature of the earth's surface, and calculated the mean speed for that interval by dividing distance by duration. Speeds were converted to km/h for analysis purposes. Each speed calculation was assigned to a time of day using the midpoint of the associated time interval.
Between January and December 2017, we deployed SPOT TraceTM GPS tracking units on 42 domestic cattle (forty one females, one male) from 29 herds (see Weise et al., 2019 for additional herd and tracker details). Monitored cattle represented herds from four main villages and 18 cattle posts. Herds were sampled using a stratified-random approach that acknowledged each sampling location's proportional contribution to the study area's entire standing herd in 2017. At each location, specific herds were randomly selected from all local herds. Monitoring focused on lead animals and had no influence on the herd's management. We programmed trackers to record and relay GPS positions at hourly intervals, or, if trackers had been stationary for >1 h, at first detection of movement via an in-built motion sensor.
Prior to the pilot study's start in May 2016, we manually created static alert boundaries Geofences 1 and 2 (Figure 2) in Google Earth. Geofence 1 (grazing) reflected the known extent of livestock grazing areas (2015–2016), whilst Geofence 2 (village) represented the known subsistence activity area, i.e., the area where humans might encounter lions on foot. Geofence 1 had the primary objective of improving livestock safety by allowing owners to collect cattle from grazing lands as lion approached, whilst Geofence 2 aimed at improving human safety.
Based on a cumulative MCP that contained all human settlements and cattle posts, 95% of cattle GPS data recorded in 2017, and 90% of lion-related livestock depredation locations (pilot study cases), we created Geofence 3 as a static alert polygon in December 2017 (Figure 2). We discarded the outermost 5% of cattle positions and 10% of predation incidents as these reflected outliers of unguarded herds moving beyond community areas into tourism concessions. Geofence 3 served as an updated alert boundary and was programmed into lion GPS units deployed in January 2018 (Figure 1) with the objective of improving both human and livestock safety.
During the alert pilot study (13 May 2016 – 12 May 2018), we received geofence breach alerts from lion GPS units via SMS notification. Regardless of the time of day, we immediately relayed breach information to the headmen of all villages and cattle posts within 8.0 km linear distance of Geofence 1 (grazing land) breach locations, and within 5.0 km linear distance of Geofence 2 (village) breach locations. We informed all communities within 8.0 km linear distance of Geofence 3 breach locations, assuming that this window would provide a safety buffer of at least 2 h, sufficiently long to take precautions.
In agreement with traditional customs, we initially alerted village and cattle post headmen who had previously agreed to forward alerts to livestock owners in their communities. Each recipient then distributed messages further, resulting in a snowball distribution system. In the course of the pilot study, we expanded alert distribution to other community members who requested to receive alerts directly, e.g., conflict-affected livestock owners (see Conflict Investigations and Lion Conflict Involvement). Participation was voluntary and there was no discrimination by ethnicity, gender, age, location, or profession. We did not distribute accurate lion GPS latitude/longitude information but informed recipients about the identity of approaching lions and an approximated distance and direction. Geographically distinct villages and cattle posts were alerted separately.
Following alert malfunctions by the collars and the deployment of new transponders in December 2016, we also checked lion GPS locations online (via the manufacturer's data portal) at least twice per day (usually around sunrise and sunset) and alerted communities about geofence breaches in case collars did not detect breaches or failed to transmit breach SMSs (Figure 1). On randomly selected dates, we recorded the work effort for manual data checks and alert distribution. Additionally, we recorded all financial costs pertaining to the implementation of this pilot alert system, including any expenses for GPS units and data fees, veterinary fees, deployment expeditions, and staff cost for alerting effort.
To evaluate the validity of initial geofences (Geofences 1 and 2; Figure 2), we calculated seasonal cattle-lion encounter risk levels as well as a human safety buffer calibrated by lion movement speed.
We mapped all investigated lion-related livestock depredation incidents and seasonal cattle-lion encounter risk levels into a 1.0 km × 1.0 km grid of the study area. For each lion GPS location recorded in 2017 (n = 13,503), we determined the closest GPS time match for simultaneously tracked cattle (n = 69,793 locations), considering a maximum time difference of 6 h. We then calculated the separation distance between these locations using the Haversine formula (Sinnott, 1984). Based on distances, we assigned a preliminary risk level to all 1.0 km2 grid cells that contained lion locations:
0 - No risk (separation >5.0 km);
1 - Lowest risk (separation < 5.0 km and >1.0 km); and
2 - Intermediate risk (separation < 1.0 km).
For all data points, we then determined the frequency of any cell being assigned as intermediate risk, and for multiple counts, refined the risk level by adding further levels as:
3 - High risk (lion-cattle separation distance < 1.0 km occurred 2 or 3 times); and
4 - Vertically high risk (lion-cattle separation distance < 1.0 km occurred >3 times).
Any cells containing investigated lion kills were assigned level 4, very high risk.
To calculate the human risk area, we created a 5.5 km radius circular buffer around each human settlement. The value for this radius is based on the maximum hourly distance traveled by any lion in this study. We then mapped buffers into a 1.0 km2 grid of the study area, and marked each cell whose centroid overlapped within any of the buffer zones. The ensemble of marked cells comprises the human risk area.
Livestock owners voluntarily reported depredation incidents by carnivores for further investigation. During investigations, we recorded attack location (latitude/longitude), date, time, livestock characteristics and value, evidence of responsible carnivore species and their numbers, details of protective measures and herd management, and the owner's opinions about conflict lion management, compensation of losses, and the lion alert system. To allow for a guided process with maximum flexibility, we employed semi-structured interviews (Brockington and Sullivan, 2003) that were administered through open and closed questions. All respondents participated voluntarily and anonymously. We also recorded all livestock loss claims to large carnivores reported to the local DWNP office. These data have no accurate GPS reference and are collated at the village level.
To determine the involvement of study lions in investigated depredation incidents, we cross-referenced each incident location against all lion GPS data 24 h before and after the event. We used a proximity-association rule to infer responsibility. We considered study lions as responsible for an incident if: (1) they were directly observed at the site; (2) they were located within 250 m of the attack site within 6 h of the estimated attack time; or, in case positional data were sparse, (3) they were located within 500 m of the attack site within 12 h of the estimated attack time.
For a grounded understanding of human-lion interactions, researchers need to grasp the complexity of social circumstances that influence community life, interactions with predators and conflict (Dickman, 2010; Pooley et al., 2017). During this study, we maintained a permanent research presence in the northern Okavango Delta. We conducted several semi-structured interview surveys (Figure 1) that yielded important insights into the various dimensions of conflict and social practices of the alert system's stakeholders.
In addition to interviews with conflict-affected livestock owners (n = 78; see section Conflict Investigations and Lion Conflict Involvement), we recorded conflict lion management suggestions from a randomly sampled control group of livestock owners (n = 53). Again, we asked their opinions about conflict lion management, the government's compensation scheme, and the lion alert system. For 12 months preceding the pilot study, and during alert distribution, we recorded livestock depredation by lions in terms of number of livestock lost, percentage stock loss, and financial value. Furthermore, we interviewed all senior village headmen (n = 12) using open-ended questions. Regardless of current wildlife legislation and conflict mitigation activities tested by the researchers, we asked headmen to state their three main aspirations about how conflict lions should be managed. For all interviews, we grouped common answers and ranked them in order of priority, assigning weight scores of 3, 2, and 1 in declining order.
To determine community perceptions of the pilot alert system across the wider community, we also conducted a qualitative study using a participatory action research approach (Kemmis and McTaggart, 2005) (Figure 1). Social scientists interviewed a randomly sampled group of local inhabitants, trying to understand how local residents perceive and deal with lions, given the presence of a pilot system designed to improve the terms of coexistence. Semi-structured interviews (n = 36) were conducted in those villages with highest conflict. For triangulation of responses, we included a diverse group of people that comprised different ages, both genders, varying levels of education, and from different villages and cattle posts (Supplementary Data 2). A local assistant translated questions into one of the three main local languages (Setswana, Hambukushu, Bayeyi). Interviews focused on the daily life of participants, the use of digital technologies and their integration into routines, knowledge, and attitudes toward lions, the lion alert pilot system, and the participant's social life in the community. Researchers documented visits via field notes, video, and photos, transcribing insights on the day of interviews. All interview materials were analyzed using qualitative content analysis (QCA) (Schreier, 2014). Analyses focused on key aspects relating to the conflict mitigation potential of the pilot lion alert system. Analyses were corroborated by external researchers who had not been involved in interviews. We took care to use findings from different researchers and interview series to ensure a broad perspective and to offset any observer bias.
Following the pilot study, we involved communities in the design of an autonomous ICT-based lion alert platform (Figure 1), intended to distribute alerts flexibly and automatically, and to a larger group of recipients. Similar to social media, the transformed alert system has a user interface, thus requiring direct community feedback during the development phase for effective implementation. The study communities are diverse in terms of their ethnicity, languages, socio-economic status, cultural traditions, literacy (Mendelsohn and el Obeid, 2004; Hanemann, 2005; Songhurst, 2017), and exposure to modern communications technology (Ertl, 2018). Consequently, a variety of technological, cultural and individual barriers could inhibit the efficacy of an ICT-based lion alert distribution platform (Irani et al., 2010; Mutula et al., 2010; Vitos et al., 2017; Ertl, 2018).
We conducted co-design workshops in Gunotsoga, Eretsha, and Beetsha, the villages that had experienced highest conflict and received most alerts (Figure 3). To facilitate maximum community consultation, workshops followed a participatory design approach (Schuler and Namioka, 1993). We developed prototype designs for different telecommunication devices and purposes using the Axure (2017) tool. Printed and digital illustrations were used to visualize the existing system (Supplementary Figure 2) as well as proposed outputs under an autonomous platform (Supplementary Figures 3, 4). Paper versions were utilized to engage target groups with limited experience in technology use (Gubbiotti et al., 1997; Vitos et al., 2017). Workshop participants (n = 35) comprised traditional village leadership, Village Development Committee chairpersons, representatives of local farmers' associations, livestock owners, and herdsmen, with an age range from 21 to 80 years. These represented a large degree of diversity in terms of occupation, location, age, literacy, herd sizes (range: 1–220), husbandry practices, conflict with lions, and experience with the pilot alert system (Supplementary Data 3). Workshops lasted between 2.5 and 4 h and participants were divided into two working groups per village.
Figure 3. Proportional distribution of cattle, livestock losses to lions, lion alert messages received and investigated (invest.) conflict locations across the study area in northern Botswana. Except for cattle holdings that were determined in 2017, data represent the lion alert pilot study from 13 May 2016 until 12 May 2018.
In each village, co-designing involved a two-tiered process with a pre-defined set of workshop implementation guidelines. Initial workshops focused on assessing and discussing experiences, benefits, and challenges from the lion alert pilot study while also reflecting on system elements and processes (Supplementary Figure 2). These workshops included focus group interviews (Byrne and Sahay, 2007; Pruneau et al., 2018), mapping and creative ideation and discussion sessions (Gubbiotti et al., 1997; Pruneau et al., 2018), in which participants expressed their aspirations and expectations toward alert delivery by an autonomous platform. Following several days of reflection, follow-up workshops focused on iterating the existing system and co-designing the functionality of the autonomous platform, capturing specific user requirements. Participants tested our preliminary, printed or digital designs (Supplementary Figures 3, 4), which we modified according to their feedback.
During this step-wise consultation process, we discussed all elements pertaining to the data acquisition phase, data processing, system components, and future alert output and distribution. We determined literacy levels, the types of communication technology used, and their integration into daily routines. Further, we determined user-specific preferences regarding alert frequencies, alert contents, message formats (i.e., text, image, sound, or voice message) in relation to telecommunication devices, and languages. Combining users' needs and new functions, we designed a feedback portal that allows for independent user registration and reporting of lion encounters in community areas, livestock movements and depredation events.
All means are presented ± one standard error.
Prior to alerting (May 2015–April 2016), depredation by lions affected 63.7% of livestock owners, with a mean annual loss of 4.1 ± 0.7% of stock owned (range: 0–60.0%; n = 102). This is equivalent to a mean of 1.8 ± 0.2 livestock per owner (range: 0–13) and a mean compensation value of $319.12 ± $408.67 per owner (range: $0–$2,351.00).
During the pilot study (13 May 2016–12 May 2018), community members directly encountered lions on at least 57 occasions (i.e., those reported to the researchers), including one near-fatal incident in which a male lion attacked a group of livestock owners (seriously injuring one person) who had attempted to kill the animal. In addition, livestock owners reported 290 incidents of livestock depredation by carnivores to the DWNP, with an annual compensation value of $41,316 (Table 1). Lions were responsible for >87% of reported predation incidents and total livestock lost (Table 1). Due to different compensation valuation rules (Department of Wildlife National Parks, 2013), lion-related losses amounted to >97% of the total compensation value (Table 1). Lions predominantly predated on cattle (96.9% of livestock killed) (Table 1). The associated impact was not evenly distributed across the study area; Gunotsoga village incurred disproportionately high losses in relation to cattle numbers (Figure 3). Depredation was highest during low-flood months (September–February), comprising 60.8% of incidents (n = 255) and 61.5% of stock losses (n = 291) (Supplementary Data 4). At this time, lions have unrestricted access to communal grazing pastures and cattle roam further into core lion habitat by following receding flood waters in NG/12, thereby increasing depredation risk significantly (Weise et al., 2019).
Table 1. Reported livestock losses to carnivores with associated compensation value ($US), 13 May 2016–12 May 2018.
Corresponding with the Delta's flooding regime, lion home range sizes and percentage overlap with geofenced community areas were highly variable, exhibiting strong seasonality (Figure 4; Supplementary Figure 5). Males had significantly larger seasonal home ranges than females (U = 20; Z = −2.76; p = 0.006), whereas females, on average, spent nearly twice as much time in geofenced community areas (Table 2). This was influenced by females PleoF003 and PleoF005 raising cubs in community areas. Seasonal home ranges were largest during the late dry season (Figure 4) when lion movements are no longer restricted by seasonal flooding. Home range overlap with community areas was highest during the wet season (Figure 4) when wild lion prey like plains zebra (Equus burchelli) and Cape buffalo (Syncerus caffer) follow early rains and migrate out of the Delta into NG/11 dryland grazing areas causing a northward shift in lion home ranges (panels c and f in Supplementary Figure 5). Seasonal home range overlap with geofenced community areas significantly decreased (rho = −0.756; p < 0.001, n = 25) with increasing home range centroid distance to the nearest settlement (Supplementary Figure 6).
Figure 4. Comparison of 100% Minimum Convex Polygon lion home range size (A) and percentage home range overlap with geofenced community areas (B) by season and genders. Blue asterisks show mean values. Sample sizes are shown in parentheses. The early dry season lasted from May to August, the late dry season from September to December, and the wet season from January to April. 100% MCPs were only calculated for individuals with sufficient GPS data (>60 days).
Study lions were predominantly nocturnal, exhibiting peaks in movements between 16.00 and 05.00 local time (Figure 5). Correspondingly, 81.4% of lion attacks on livestock with known time of day (n = 97) occurred during night hours, significantly more than during the day (χ2 = 38.36; df = 1; p < 0.001). While males and females exhibited similar activity rhythms (Figure 5), males, on average, moved 2.63 ± 0.26 times faster during peak activity hours, and with a maximum speed of 5.47 km/h.
Figure 5. Mean movement speed of lions by hour of day. Speed was inferred by measuring displacement between successive GPS fixes, adjusted to hourly intervals.
Study lions spent 26.3% of all time monitored within geofenced areas (Table 2), with a mean of 21.9 ± 8.0% per individual (range: 0–60.9%; n = 9). Geofence transgressions were highly variable in terms of frequency and duration (Table 2). Four of the nine study lions only sporadically breached geofences (cumulative n = 12) (Supplementary Figure 5), whereas five lions accounted for 92.8% of all breaches (Table 2), and with a bias toward females (χ2 = 2.91; df = 1; p = 0.088), two of which raised seven cubs to >12 months age in community areas. During cub-rearing, individual transgressions by females lasted as long as 89.6 days and 161.1 days, respectively, whereas the median duration of transgressions across all individuals was 24 h (Table 2). Due to cub-rearing, female transgressions, on average, lasted significantly longer than those of males (T = 1.86; p = 0.032).
We investigated 116 (45.5%) of the 255 lion-related livestock predation incidents reported for compensation (Table 1) and cross-referenced depredation sites against GPS paths. Spatial association analyses showed that the nine study lions were responsible for 36 incidents (31.0%), whilst un-tagged lions accounted for the majority of investigated losses (69.0%). This result demonstrates the effect of partial sampling (i.e., low population representation by tagged study lions) on alert utility. From a total of 277 lion observations (88 by researchers, 189 from tourism sources with photo evidence), we estimated that the nine study lions represented 12.3% of all resident individuals (>24 months age) and 41.2% of known female prides and male coalitions in the study area.
All study lions that transgressed geofence boundaries were involved in livestock depredation, but males were significantly more conflict prone when controlling for monitoring time (χ2 = 4.98; df = 1; p = 0.026). The two females that reared cubs in community areas were involved in 58.3% (n = 21) of all depredation incidents involving study lions. The four individuals with home range centroids nearer settlements (< 10.0 km distance) and highest home range overlap with community areas (>33.3%) were significantly more involved in conflict (χ2 = 25.31; df = 1; p < 0.001), accounting for 86.1% (n = 31) of incidents attributable to study lions.
We alerted the community about approaching lions on 188 days, or 25.8% of study days, representing 366 lion alert days when considering multiple animals on the same day. In total, we sent 1,017 alert SMS to 66 recipients in four villages and 19 cattle posts (Table 2; Supplementary Data 5). Alert messages represented 166 geofence breaches (males: 72; females: 94) resulting in 304 original alerts and 694 reminder messages when lions spent prolonged periods in community areas, as well as nine incidents of un-tagged lions detected near cattle posts (19 alerts) (Table 2). Five lions accounted for 97.1% of alerts issued (Table 2), with females disproportionately represented (χ2 = 45.88; df = 1; p < 0.001). Reflecting the variable movements of individual study lions (see Lion Movements and Geofence Transgressions) and the contribution of un-collared individuals to depredation (69.0%), the distribution and frequency of alerts (Figure 3; Supplementary Data 6) differed significantly from that expected by livestock losses (χ2 = infinite value; df = 4; p < 0.001).
The mean daily researcher effort for retrieving and checking location data of 3–6 active collars and associated alert distribution via SMS messages on 116 randomly selected days was 40.8 ± 1.1 min (range: 14–71 min). The number of active units weakly correlated with daily effort (rho = 0.142).
QCA of 36 semi-structured interviews revealed that people value their livestock for reasons other than monetary income. Livestock are predominantly a cultural commodity, provide food security (especially in times of drought when crops fail), and are sold context-dependently, e.g., when owners pay school fees or funeral costs. Respondents regarded livestock husbandry as a full-time commitment, yet very few employ herders (Weise et al., 2018). Based on the narratives of daily life routines, husbandry entails night-time kraaling, release of herds in the morning hours, and searches for stray animals.
Interviews with 12 community headmen and 78 depredation-affected livestock owners yielded 161 conflict lion management suggestions that reflected eight common themes. The majority of responses entailed removing lions from community land by lethal control, translocation or effective fencing, comprising 50.9% of the total priority weight (Table 3). This mirrors results from QCA such that the majority perception of lions was negative (75.0%, n = 27). Together with improvements of general livestock protection and the state-funded compensation scheme, expansion of the lion alert system featured among secondary lion management approaches, ranking fourth in terms of overall priority weight (Table 3). The alert system's priority weight was higher for conflict-affected livestock owners (10.3%) than community headmen (5.4%). In terms of conflict management characteristics, most community members expected a form of physical separation from free-ranging lions (54.6% priority weight), corresponding with QCA results in that community members felt that “coexistence with lions was not possible.” Lion management suggestions were significantly biased toward symptomatic, reactive mitigation strategies (61.0% priority weight), followed by proactive, protective strategies (30.8% priority weight) and no-interference management approaches (8.0% priority weight) (Table 3) (χ2 = 121.76; df = 2; p < 0.001).
Livestock owner satisfaction was higher for alerts than the state-funded damage compensation scheme. Of all 78 livestock owners interviewed during depredation investigations, 65 commented about their perceptions of the alert system and compensation scheme. Of these, 56.9% had previously received compensation for losses, whilst 75.4% had previously received lion alerts (Supplementary Data 7). Only 24.3% of compensation recipients (n = 9) were satisfied with the compensation scheme. QCA showed that compensation did not improve people's situation. Instead, respondents felt “left alone” as the DWNP does not have the resources to attend to their lion conflict reports. The key reasons for dissatisfaction included “insufficient compensation amounts” (85.7%, n = 24) and/or “delayed compensation payments” (35.7%, n = 10).
Conversely, 91.8% of lion alert recipients found messages beneficial (n = 45), whilst 6.1% (n = 3) stated that they “no longer wished to receive them.” QCA corroborated that, despite prevailing uncertainties about appropriate responses to approaching lions, the pilot alert system was perceived as beneficial and respondents requested its “continuation and expansion.” Of the 65 conflict-affected livestock owners, 90.8% (n = 59) wished to receive lion alerts via SMS in the future (Supplementary Data 7).
The 45 livestock owners who perceived alerts as beneficial stated different benefits and actions. The most common response was livestock kraaling for increased night-time protection (68.9%), followed by changes in cattle grazing directions and areas (15.6%), setting of deterrence fires at homesteads (4.4%) and active herding of cattle (2.2%). Other stated benefits included a feeling of increased personal security due to awareness about lion presence (13.3%) and an improved understanding of lion movements and ecology (17.8%). However, another 32 alert recipients stated that they did not take actions because they “did not know what to do” (59.4%) and/or “feared dangerous encounters with elephant, buffalo or lions during night hours” (81.3%). QCA confirmed that community members “rarely encounter lions,” contributing to an uncertainty of how to respond when direct interactions occur.
During the pilot study, mean annual livestock losses of those owners who acted upon alerts (n = 49) significantly decreased in terms of number of stock lost (U = 876.5; Z = −2.29; p = 0.021), compensation value (T = −2.38; p = 0.021) and percentage of stock lost (T = −3.07, p = 0.003) (Figure 6). Their mean annual losses were significantly less than those incurred by a randomly sampled control group (n = 53) in terms of number of stock lost (U = 945.5; Z = 2.36; p = 0.018), compensation value (T = −2.34; p = 0.010) and percentage stock loss (T = 1.91; p = 0.029) (Figure 6), despite 23 control group livestock owners (43.4%) also regularly receiving lion alerts but choosing not to act. By comparison, prior to receiving alerts (2015–2016), mean annual losses did not differ significantly between alert-sensitive livestock owners and control group owners (number of livestock lost: U = 1209; Z = 0.596; p = 0.548, compensation value: T = 0.14; p = 0.440, percentage stock lost: T = 1.424; p = 0.078) (Figure 6). There were no significant changes in the mean annual number of stock lost (U = 1308; Z = −0.60; p = 0.541), mean compensation value (T = 0.48; p = 0.628) or mean percentage stock lost (T = 0.88; p = 0.379) when comparing pre-alert and pilot study losses incurred by control group livestock owners (Figure 6). Alert-sensitive livestock owners reduced losses even though reported lion depredation increased by nearly 300% during the pilot study: year 1 = 74 livestock, year 2 = 214 livestock. In financial terms, livestock owners who acted upon alerts reduced their mean annual loss to lions by $124.61 (59.6%), equivalent of a percentage stock loss reduction of 3.4% (Figure 6). During the pilot study, the mean annual loss incurred by alert-sensitive livestock owners was $134.40 less (57.7%) than that by control group livestock owners (Figure 6).
Figure 6. Comparison of annual lion-related livestock losses (A), percentage stock loss (B), and associated compensation value ($US) (C) for responsive alert recipients before (A) and during alerts (B) and a control group of non-responsive recipients before alerts (C) and during alerts (D). Blue asterisks show mean values. Sample sizes are shown in parentheses. Livestock losses were recorded from May 2015 until April 2016 for the pre-alert phase and from November 2016 until October 2017 for the alert phase.
Considering all associated expenses, the total cost of GPS-collaring nine adult lions for inclusion in the alert system was $70,983.04 (n = 13 deployments), with a mean deployment cost of $5,460.24 ± $493.89 for units equipped with on-board geofence breach technology (range: $3,821.10–$10,764.40). GPS tracking technology and associated data fees contributed 46.8% to the total cost, whilst veterinary expenses accounted for 44.5% of the total, the remainder accruing from local staff, vehicle, and expedition expenses. The 13 geofence-enabled units were active for a total of 3,829 tracking days [including days for first units prior to commencement of the pilot study (n = 888)], giving an approximate daily alert system cost of $18.54 per lion. Daily cost would decrease to $4.99 if all units had operated until the end of their expected lifetimes.
In light of an annual livestock damage of approximately $40,407 caused by lions (Table 1), and assuming that early warning would effectively prevent depredation, the observed daily alerting cost merits the inclusion of only six lions (8.2% of the estimated population), whereas 22 individuals (30.4% of the population) could be included if GPS units functioned reliably. Early warning financially breaks even if the inclusion of a specific lion, on average, can prevent the depredation of 12 livestock per year, emphasizing that GPS tracking efforts need to be focused on habitual livestock raiders.
The performance and reliability of GPS tracking units was highly variable in terms of complete daily tracking data received (range: 2.3–92.6%; Supplementary Data 8) and the proportion of scheduled GPS locations received (range: 51.1–95.9%; Supplementary Data 9). We replaced four active collars in December 2016. At the end of the pilot study, four units still transmitted data daily, whereas three units (23.1% of deployments) malfunctioned between 22 and 71 days after deployment, and two units were deactivated following lion deaths.
During the pilot study, GPS units (n = 13 deployments) delivered complete data sets on only 28.8% of all lion tracking days (n = 2,941 with a 24 h cycle), with a mean of 27.9 ± 7.7% per deployment (Supplementary Data 8). Increasing the GPS sampling frequency from five daily locations (Telonics units, n = 875 tracking days) to 12 (Vectronic units, n = 2,066 tracking days) decreased the daily success rate of receiving complete data from 55.9 to 17.3%, respectively. There was no significant difference in the mean percentage of complete GPS tracking days between collars fitted on male (n = 7 deployments) and female (n = 6 deployments) lions (U = 10; Z = −1.50; p = 0.133). Despite the low number of complete tracking days, the 13 transmitters delivered 83.3% of the expected 29,609 lion GPS locations (Table 4; Supplementary Data 9). The mean percentage of scheduled GPS fixes received per lion was 81.2 ± 1.6% (range: 74.8–87.3%, n = 9). A randomized sample of 286 GPS data gaps (both collar types combined) showed that GPS fix and transmission failures resulted in a mean data gap duration of 6.9 ± 0.3 h (range: 0–124 h). The modal duration of GPS data gaps was 4 h, with maximum durations of 36 h for Telonics units (five daily locations) and 124 h for Vectronic units (12 daily locations).
Table 4. Proportions of expected and received GPS fixes from geofence-enabled lion tracking collars.
Early GPS units (May–December 2016) were programmed to report both geofence breaches and exit events. These transponders detected 44 geofence breaches, including 42 Geofence 1 transgressions and two Geofence 2 transgressions. However, in 22.7% of cases (n = 10), breach messages were only received after lions had already left the geofence again, in 15.9% of cases (n = 7) the units failed to transmit exit messages, and in 11.4% of cases (n = 5) the units failed to transmit breach messages but reported exits. Compared with lion movement paths plotted from GPS locations, on-board geofence functions under-detected true Geofence 1 breaches (n = 51) by 17.6%, whilst missing 80.0% of true Geofence 2 breaches (n = 10) across 6 months of operation. In combination with delayed breach messages, the community received alerts about 74.5% of true Geofence 1 breaches (n = 38) and 30.0% of true Geofence 2 breaches (n = 3), the latter including one reminder. These irregularities caused us to return to daily manual data checks and alert distribution. Moreover, of the nine units deployed subsequently (December 2016 and January 2018), four (44.4%) did not detect any geofence breaches (although they occurred), whilst three (33.3%) failed altogether within 11 weeks of deployment.
Based on a GPS distance matrix of lion and cattle GPS locations, and the locations of investigated lion conflict, Figure 7 shows the seasonal distribution of lion risk to cattle. Very high risk was widespread during the early wet season when lions still had unobstructed access to communal grazing lands before annual floods arrive, and two females raised cubs in community lands. Arbitrarily defined Geofence 1 (i.e., the original grazing lands boundary) contained 88.2 and 91.3% of high and very high risk cells during the wet and early dry seasons, respectively. However, as the risk interface progressively changed throughout the year, moving in relation to receding flood waters, Geofence 1 only contained 59.5% of high and very high risk cells during the late dry season, revealing the need for dynamic geofencing that appropriately reflects seasonally changing environmental conditions and associated predation risk.
Figure 7. Seasonal geo-assessment of lion risk to cattle, showing (a) the wet, (b) the early dry, and (c) the late dry seasons of 2017. Risk levels are mapped into a 1.0 km × 1.0 km grid across the study area. Each grid cell is hot-cold color-coded to indicate relative risk level. Yellow (1) and orange (2 or 3) indicate the number of instances in which lions approached to within 1.0 km of tracked cattle. Red cells are those in which either kills occurred, or there were >3 records of lions within 1.0 km of tracked cattle.
Similarly, Figure 8 demonstrates that the arbitrarily drawn village safety boundary (Geofence 2) only partially protected human settlements. Geofence 2 contained only 75.4% of the estimated 1,278 km2 risk area and excluded one cattle post while another was partially excluded. Additionally, 24 cattle posts and two villages appeared too close to Geofence 2 to permit sufficient time for adequate precaution. Based on a 1 h human safety buffer calibrated to the maximum hourly speed recorded for any lion (5.47 km/h), safety alerts should be triggered further from village areas and cattle posts. Human settlements change over time, requiring dynamic geofencing to reflect these changes (e.g., adjusted Geofence 3, Figure 2).
Figure 8. Comparison of Geofence 2 placement with geo-assessment of lion risk to people. Risk was calibrated to the maximum hourly speed moved by any lion in this study (5.47 km/h) and mapped into a 1.0 km × 1.0 km grid across the study area. Red cells indicate risky areas within 1 h distance from permanent settlements.
The communities are technologically marginalized, with sporadic access to electricity and the Internet. Despite these infrastructural challenges, mobile phones are ubiquitous in this rural part of Botswana (as elsewhere in Africa) and are, therefore, an ideal medium for receiving alerts. All workshop participants (n = 35) owned mobile phones but predominantly used these for basic functions such as calling relatives. The prevalence of feature and smart phones varied by village (Figure 9), with an overall smart phone representation of 23.5%. Participant literacy was low (62.9%), digital literacy even lower (14.3%), compromising the delivery of lion alerts via text or image messages only. Community members speak five different languages, with Setswana being the only one common to everyone. For future alerting, desired message formats differed geographically and a combination of image and voice messages was most popular (Figure 9). Respondents remarked that feature phones prohibit the use of imagery to convey all necessary alert information (lion distance and direction), necessitating combinations with text and voice formats (Supplementary Figure 3). Participants also suggested the incorporation of specific sounds and ringtones (e.g., lion roar) to distinguish alerts from other messages.
Figure 9. Village-specific use of feature and smart phones and expected lion alert message formats as determined during co-design workshops.
Workshop participants advocated for the development of an information feedback loop in the form of a community portal with application interfaces that enable users to report their own lion observations and depredation incidents, but also to retrieve lion alert information independently. Respondents commented that such a portal would improve reporting speed and ease while relinquishing the reliance on private mobile devices, which may not be charged or have insufficient credit for reporting. Co-design workshops also revealed the need for additional digital literacy training and community mapping of the local environment to enable accurate reporting of feedback via digital maps (Mapedza et al., 2003; Vitos et al., 2017). The preferred method of ensuring personal data security and privacy was password protection, which participants knew from social media. Meanwhile, 30 min was the preferred frequency of lion information updates on the portal. The variety of user-specific requirements and contexts demonstrates the necessity for a flexible, customized lion alert platform that provides a range of interactive features.
In combination with the logistical and technological challenges encountered during the pilot study, community co-design workshops prompted the development of an autonomous, automated lion alert platform (Figure 10). This development provides maximum versatility in terms of data acquisition and integration, geofence creation, alert distribution, and community participation. The system's key features and capabilities are outlined in Box 1 and Supplementary Figure 4.
Figure 10. Visualization of the autonomous, automated lion alert system with key features and functionalities.
Box 1. Key features and capabilities of the autonomous lion alert system.
1. Real-time integration of diverse environmental data and community feedback (e.g., lion encounters, cattle movements, depredation sites);
2. Autonomous, objective geofence computation based on available input data and a priori threshold setting (e.g., critical distances and areas);
3. Flexible implementation of village-specific geofences;
4. Dynamic geofencing, including static and mobile virtual boundaries, and possible attractors and deterrents for depredation;
5. Automated, instant alert delivery via multiple media formats to a diversity of communication technology devices;
6. Integration and configuration of risk evaluation parameters;
7. Interactive community portal with data report and entry interfaces;
8. Independent user registration;
9. User-specific selection of preferred alert message types (text, image, sound, or combinations thereof) and languages based on literacy levels and communications technology; and
10. Options for alert delivery via social media platforms (e.g., WhatsApp or Facebook groups) or supporting devices such as sirens and light installations.
The new system is based on the computation of implicit surfaces for defining dynamic geofences. It automatically retrieves, and integrates, positional data from any actors equipped with GPS transponders as well as supporting environmental information from online databases (e.g., seasonal flooding levels). Virtual boundaries can be adjusted either manually or by machine-learning algorithms that process GPS and other relevant data in near real-time, predicting risk areas while automatically adjusting geofences. Conflict is defined by empirically determined risk thresholds (e.g., see Seasonal Cattle Depredation Risk and Human Safety). Based on our results, the literature, and community feedback, we identified 26 key variables (Supplementary Data 10) that influence conflict likelihood and, therefore, require empirical consideration in computation of flexible geofences. We developed the prototype in Java with Java Database Connectivity for a Structured Query Language. The system is currently implemented on a Windows 2016 server with PostgreSQL. Depending on the type of tracking technology utilized, it can be compartmentalized into GPS storage with data processing on any other platform. Autonomous data integration and processing removes reliance on predefined, static geofences and on-board alert functionality. The system is currently hosted at the Department of Economic Disciplines, Faculty III of Siegen University, Germany.
The platform enables independent, password-secured and anonymous user registration via cell phone number (Supplementary Figure 4A). Subscribers can then select their preferred alert format for different phone types (e.g., Supplementary Figure 3). We designed alert applications for smart and feature phones, ensuring that feature phones provide the same functionalities. Registered users receive alerts instantly. Instead of data processing time, alert frequency is determined by the sampling regime of GPS transponders and the availability of corroborating data.
To enable maximum community participation and ownership as well as system sustainability, we co-designed a suite of alert portal interfaces (Supplementary Figure 4). These will be accessible via tablet computers installed at each village's administrate office, with guidance provided by specially trained community members. Interfaces include an overview of current and past alerts, the reporting of lion encounters or tracks, the reporting of depredation incidents, information on lions, display of geofence(s), and personal settings (Supplementary Figure 4). All features are delivered in Setswana language. Following others (Medhi et al., 2006; Vitos et al., 2017), we utilized visual elements to support interface use by illiterate subscribers. Map interfaces are currently based on distance zones that resemble active geofences, an approximation that was understood by workshop participants. In future, digital maps will provide an intuitive avenue for reporting of relevant information according to geo-physical landmarks such as trees, lagoons, and islands that all community members can relate to. For feature phones, we programmed USSD-based (locally used to recharge phone credit) and voice-prompted reporting interfaces that have shown success in other participatory design projects (Weld et al., 2018). For illiterate users, automated voice prompts will be used to replace text prompts. Similar speech-based interfaces have successfully been tested with illiterate target groups (Raza et al., 2018). The autonomous platform allows for the instant integration of community feedback into alert calculations (Figure 10), increasing the representation of un-tagged lions in alerting and community ownership of the process.
Managing undesirable human-wildlife interactions requires adaptive strategies. In northern Botswana, communities seek safety from lions, primarily via physical separation and lion control. However, the continued lethal removal of damage-causing lions threatens population viability (Loveridge et al., 2016; Trinkel et al., 2017); fencing is expensive (Packer et al., 2013), ecologically problematic (Trinkel and Angelici, 2016), and can shift problems elsewhere (Osipova et al., 2018); while translocations are resource-intensive and have historically shown low success rates (Stander, 1990). By default, separation undermines coexistence. Consequently, separation also compromises the sustainable conservation of large free-ranging lion populations (Creel et al., 2013) that depend on connectivity between key ecosystems in human-inhabited conservation landscapes such as the KAZA-TFCA (Cushman et al., 2016). Early warning by geofence-triggered alerts provides an alternative mechanism that facilitates non-lethal and non-permanent separation at flexible spatio-temporal scales. Our study demonstrates that alerting rural livestock owners about approaching lions promotes improvements in livestock protection that result in significantly reduced losses, thereby facilitating the behavioral changes that coexistence requires (Reddy et al., 2017). Despite the immediate benefits of improving human and livestock safety, the success of early warning depends on effective system implementation by overcoming a variety of technological, human, and environmental challenges (Box 2).
Box 2. Opportunities and challenges of lion alerts identified during the pilot study.Opportunities:
1) Increasing human safety by preventing dangerous encounters, e.g., during subsistence activities;
2) Encouraging livestock protection and husbandry practices (e.g., herding and kraaling);
3) Reducing livestock depredation, thus increasing food and economic security;
4) Improving tolerance of lions in human-dominated landscapes;
5) Increasing awareness and knowledge about local lions and their ecology; and
6) Advancing digital literacy and intra-community communication in partner communities.
Challenges:
Anthropogenic
1) Manual alert distribution depends on researcher/manager effort (and availability) in terms of checking GPS units daily and issuing alerts;
2) Risk of misuse of alert information to persecute lions;
3) Geofence breach messages dispatched by collars may be missed during night hours when breach probability is highest;
4) Alert distribution via community SMS snowball system is subject to intra-community relationships—not all farmers may receive messages from others;
5) System's effectiveness depends on human response to alerts;
6) Risk of increasing resentment toward and fear of lions via continuous reminders of their presence, i.e., artificial reinforcement of perceived threats;
7) Non-probabilistic, subjective interpretation of breach messages, and lion movement trajectory by researcher/manager is prone to human error, introducing the risk of informing the wrong communities;
8) Risk of desensitizing people to the relative threat posed by lions via frequent reminders of their presence without direct dangerous interactions;
9) Subjective selection of focal lions by researchers may bias population representation—conflict lions may exhibit strong avoidance of humans, thus being under-represented in the monitored sample;
10) System components may be difficult to understand for rural communities with little previous exposure to modern communications technology;
Technology
11) Efficient alert distribution via cell-phone network depends on reliable network coverage in remote areas, but also power supply to charge phones, retrieve GPS data, and distribute alerts—in January 2017, the system collapsed during the rainy season when public power supply and network coverage was insufficient during a high conflict period;
12) Limited reliability and accuracy of GPS-tracking technology causes delays in distributing alerts, influencing alert frequency, timing, and relevance;
13) On-board geofence functions are static and may not operate reliably or timely;
14) Lifespan of GPS-tracking technology limits system effectiveness and feasibility;
Ecology
15) Impact of tagging only one individual per group: lion mortality, changing group compositions and variable cohesiveness, immigration, and emigration affect population representation and system effectiveness;
Information
16) System effectiveness is scale-dependent—appropriate population representation (i.e., tagging all adult lions/groups simultaneously) across large areas is difficult to achieve in terms of financial feasibility and logistics; and
17) Objective, probabilistic establishment of relevant geofences requires a wealth of empirical bio-geographic data that may not always be available, or difficult to obtain (Supplementary Data 10).
The efficacy of lion alerts directly hinges upon user responses. To enable responses, the system needs to deliver messages to a variety of communication devices in a timely and understandable fashion (Medhi et al., 2006; Sherwani et al., 2009). Considering the user community's heterogeneity in terms of literacy, attitudes toward modernization, use of communication technology, and language and message style preferences, this poses challenges (Box 2). Careful pre-studies of the socio-cultural contexts, co-design of the system's functionalities, and alert distribution via modern socio-informatics are imperative to engage users satisfactorily and sustainably. For example, a user-friendly device for community data collection, the CyberTracker, provides adaptable, yet easily understandable, interfaces that are designed to enable geo-referencing of environmental information by rural inhabitants with different educational backgrounds (Ansell and Koenig, 2011). Co-design workshops revealed the need for information feedback loops that enable active participation in risk management, thus increasing community ownership. Instead of eroding traditional environmental skills and practices, an ICT-based alert platform provides an interactive avenue for their propagation and inclusion in environmental decision making (also see Ansell and Koenig, 2011). Other, maybe less apparent, benefits of a participatory strategy include the community's perception of “being heard,” improved digital literacy, and access to WiFi through the installation of community alert portals in remote villages.
Human-lion conflicts are complex (Dickman, 2010) and early warning efficacy strongly depends on our ability to quantify risk and its many drivers (Supplementary Data 10). In the Okavango Delta, lions and their wild and domestic prey live in a seasonally changing ecosystem (Murray-Hudson, 2009; Fynn et al., 2015). Changes in conflict likelihood correspond with seasonality as it influences lion and cattle movements, associated predation risk, and lion socio-ecology (Kotze et al., 2018; Weise et al., 2019). Translating these ecological changes, and their high levels of spatio-temporal variation, into risk probabilities in near real-time is a central prerequisite for alert efficacy, one that requires dynamic geofencing. It also requires intensive monitoring of different ecological variables (Supplementary Data 10) and can benefit from machine learning to infer behavioral patterns from animal GPS data (Valletta et al., 2017). Early warning will be most effective in situations where boundaries are clearly definable. Geofence design and breach detection must account for the speed of actors, likelihood of attack, and GPS fix schedules. With an ability to travel up to 5.47 km in 1 h, lions could breach geofences and enter villages without detection, even with short GPS fix intervals that considerably reduce transponder battery lifetime. Static geofences programmed into GPS transponders cannot sufficiently reflect the variability of ecosystems and conflict complexity. Here, we present an autonomous platform for the instant computation of dynamic geofences and flexible delivery to a variety of users.
Human-lion conflicts and their effective mitigation are scale-dependent (Montgomery et al., 2018a). The conservation benefit of individual- or group-based conflict mitigation methods decreases as economic cost increases in relation to population size (Shivik, 2004) and conflict area. Therefore, expensive interventions such as alerting are most feasible in conflict hotspots with highest conservation significance (e.g., by maintaining effective connectivity between populations), and where the majority of actors can be tracked cost-effectively. At the currently high cost of intensive GPS monitoring (Thomas et al., 2011), the on-going implementation of an early warning system requires significant resources, especially in high conflict zones bordering protected areas where lion mortality is high (Trinkel et al., 2017) requiring frequent tagging of new actors. We demonstrate the importance of appropriate population representation as our study lions accounted for 31% of the regional conflict. Partial collaring of the lion population resulted in focal alerting, not always benefiting the communities with highest losses. Alert systems will be most meaningful where the movements of actors and the effects of local attractors (e.g., cattle post locations or seasonally shifting prey density) can be combined into risk proxies that are computed as changing virtual boundaries. Tracking technology failures prevented the effective monitoring of lions and the detection of geofence breaches (Box 2), thus decreasing alert efficacy. Autonomous implementation of geofencing and alert functions through a stand-alone platform not only provides maximum system flexibility but also increases alert reliability. With continuous advances in modern animal tracking systems in terms of unit size and weight, cost, and independence from on-board battery supply (Tomkiewicz et al., 2010; Thomas et al., 2011), the feasibility of GPS-tagging actors increases, thus also improving the scalability of early warning and its overall cost-efficiency by reducing labor effort.
We can use lions and livestock depredation as conflict proxies for a universal challenge, the increasing interface between people, livestock and wildlife. Globally, 262 wild terrestrial vertebrates, including 53 threatened species, interact detrimentally with people (Torres et al., 2018). Our lion alert platform is neither species- nor context-specific, lending itself to various conservation applications (also see Wall et al., 2014). Amongst others, possible scenarios include elephants approaching human settlements or crop fields (human and food security), a rhino leaving the safety of a reserve core management area (biodiversity security), or a buffalo herd approaching cattle (disease transmission risk). In each of these cases, the risk of an undesirable interaction is defined by critical distance thresholds, expressible as a likelihood of interaction and risk. As more and more wildlife are tracked via GPS (Kays et al., 2015), and advances in both animal-borne and non-invasive technologies provide cheaper and more reliable sensor options, the feasibility of implementing alert systems in human-dominated wildlife habitats increases. Our autonomous platform can be tailored to any risk scenario, given that the relevant vector data are available and conflict risk can be expressed in an algorithmic manner. In the case of alerting communities about lions, the new system permits the evolution from an experienced-based pilot phase into an experimental phase. Reducing human errors associated with manual alerting, for example by subjective interpretation of lion movement trajectories toward specific cattle posts or villages, is paramount.
An automated, autonomous platform provides a robust and objective mechanism with maximum versatility for creating dynamic, ecologically relevant virtual conflict boundaries and maximum flexibility in issuing customized alerts in a timely and culturally appropriate fashion. Successful conflict mitigation, via methods such as alerting, is highly context-specific and usually cannot be inferred from disciplinary knowledge alone. It requires adaptive co-design that encompasses local knowledge, a detailed understanding of the affected parties, and relevant research findings (Pooley et al., 2017; Montgomery et al., 2018b). Here, we chose a trans- and multi-disciplinary development approach involving local stakeholders as well as researchers from different disciplines to progress “from a science for society to a science with society” (Scholz and Stauffacher, 2009). The inherent complexity of human-carnivore conflict, both ecologically (Supplementary Data 10) and socio-culturally (Dickman, 2010), demands a direct and constructive collaboration between science and society. This interaction needs to identify and address the different dimensions of conflict and, therefore, can only be realized with a multi-disciplinary research and development strategy (Pooley et al., 2017; Montgomery et al., 2018b). Omission to do so will inevitably result in unsustainable or ineffective conflict mitigation efforts, particularly if the safety or livelihood of rural communities is at stake. System automation does not provide a panacea, however, as it cannot resolve all the challenges of early warning (Box 2). For instance, the utility of any early warning system, autonomous or otherwise, strongly depends on the rigorous identification and continuous tracking of its key actors. We demonstrate that this can be costly and difficult to achieve. Therefore, care should be taken to avoid reliance on early warning as a stand-alone mitigation method. The prototype alert platform (Figure 10), alongside other conflict reduction measures such as vigilant full-time herding (Weise et al., 2019), will be implemented in 2019, including further refinement of system processes and monitoring of its efficacy.
Alerting communities about lions devolves important ecological knowledge and sensitive information to Africa's key lion conservation stakeholders, the people that live with lions. It encourages active risk management, thereby moving beyond the symptomatic treatment of damage. Recipient communities, however, are not homogenous. In addition, technological limitations, the complexity of human-carnivore conflicts, and the variability of the natural environments in which these occur, complicate effective early warning, which requires a combination of expertise that synthesizes ethnography, ecology, livestock management, conservation psychology, and socio-informatics. Our development of a versatile, autonomous lion alert platform emphasizes the critical importance of a trans-disciplinary approach to mitigating human-lion conflicts.
We conducted research under permit numbers EWT 8/36/4 XXVII (61) and EWT 8/36/4 XXXVIII (63) granted by the Ministry of Environment, Wildlife and Tourism in Botswana. We interviewed human subjects and monitored livestock and lions with ethics approval from the University of Pretoria (no.: EC170525-120, EC170525-120a) and the Institutional Animal Care and Use Committee of the University of Massachusetts (Protocol no.: 2014-0083).
FW, AS, KS, HH, KA, MWH, MH, VW, and MT conceived the ideas and designed the methodology. FW, HH, KA, MT, and KS collected the data. FW, KS, HH, KA, and MH analyzed the data. FW, HH, AS, KS, MWH, and MS wrote the manuscript. All authors contributed critically to the drafts and gave final approval for publication.
The authors declare that the research was conducted in the absence of any commercial or financial relationships that could be construed as a potential conflict of interest.
We thank the Ministry of Environment, Wildlife, and Tourism in Botswana for granting permission to conduct this study. We thank G. Bigl and C. Winterbach for constructive ideas and criticisms, and E. Verreynne for veterinary support. We thank R. Grinko, V. Wenzelmann, C. Dimbindo, and E. LeFlore for assistance with field work and co-design workshops, and the Ecoexist Project for assistance with livestock tracking. Comments by two reviewers improved the quality of the paper. We thank G. S. Flaxman, S. Eckhardt, and J. Walls for logistical support. We thank community members and leadership. This work was supported by the National Geographic Big Cats Initiative (grant numbers: B5-15, B10-16, B6-17), WWF's INNO fund (grant no.: 17-03), and Stichting SPOTS, NL, and its supporters. FW was supported by a post-doctoral fellowship at the University of Pretoria. MS was supported by a National Research Foundation Incentive grant. MWH was supported by the Australia-Africa Universities Network.
The Supplementary Material for this article can be found online at: https://www.frontiersin.org/articles/10.3389/fevo.2018.00242/full#supplementary-material
Ansell, S., and Koenig, J. (2011). CyberTracker: an integral management tool used by rangers in the Djelk indigenous protected area, central Arnhem land, Australia: feature. Ecol. Manag. Restor. 12, 13–25. doi: 10.1111/j.1442-8903.2011.00575.x
Bauer, H., Chapron, G., Nowell, K., Henschel, P., Funston, P., Hunter, L. T. B., et al. (2015). Lion (Panthera leo) populations are declining rapidly across Africa, except in intensively managed areas. Proc. Natl. Acad. Sci. U.S.A. 112, 14894–14899. doi: 10.1073/pnas.1500664112
Bennett, J. W., Roth, R., Klain, S. C., Chan, K., Christie, P., Clark, D. A., et al. (2017). Conservation social science: understanding and integrating human dimensions to improve conservation. Biol. Conserv. 205, 93–108. doi: 10.1016/j.biocon.2016.10.006
Boccacci, F., González, V., and Bruy, A. (2014). AniMove Version 1.4.2. Available online at: https://github.com/geomatico/sextante_animove
Brockington, D., and Sullivan, S. (2003). “Qualitative methods,”. in Development Fieldwork: A Practical Guide, eds R. Scheyvens and D. Storey (London: Sage Publications), 57–74.
Byrne, E., and Sahay, S. (2007). Participatory design for social development: a South African case study on community-based health information systems. Inf. Technol. Dev. 13, 71–94. doi: 10.1002/itdj.20052
Creel, S., Becker, M. S., Durant, S. M., M'Soka, J., Matandiko, W., Dickman, A. J., et al. (2013). Conserving large populations of lions - the argument for fences has holes. Ecol. Lett. 16:1413. doi: 10.1111/ele.12145
Cushman, S. A., Elliot, N. B., Macdonald, D. W., and Loveridge, A. J. (2016). A multi-scale assessment of population connectivity in African lions (Panthera leo) in response to landscape change. Landsc. Ecol. 31, 1337–1353. doi: 10.1007/s10980-015-0292-3
Department of Wildlife and National Parks (2013). Compensation Guidelines for Damages Caused by Elephants and Lion. Department of Wildlife and National Parks, Ministry of Environment, Wildlife and Tourism.
Dickman, A. J. (2010). Complexities of conflict: the importance of considering social factors for effectively resolving human-wildlife conflict: Anim. Conserv. 13, 458–466. doi: 10.1111/j.1469-1795.2010.00368.x
Ertl, T. (2018). Digitale Informations- und Kommunikationstechnologie zur Lösung Eines Mensch-Tier-Konfliktes im Okavango-Delta. M.Sc. dissertation, University of Siegen, Germany.
Fynn, R. W. S., Murray-Hudson, M., Dhliwayo, M., and Scholte, P. (2015). African wetlands and their seasonal use by wild and domestic herbivores. Wetlands Ecol. Manag. 23, 559–581. doi: 10.1007/s11273-015-9430-6
Gubbiotti, G., Malagò, P., Fin, S., Tacchi, S., Giovannini, L., Bisero, D., et al. (1997). “First workshop: designing reading technologies with indigenous learners in Namibia,” in Proceedings of ACM Woodstock Conference (El Paso, TX: ACM Press).
Hanemann, U. (2005). Literacy in Botswana. Hamburg: UNESCO Institute for Education. Available online at: http://unesdoc.unesco.org/images/0014/001460/146005e.pdf
Henschel, P., Coad, L., Burton, C., Chataigner, B., Dunn, A., MacDonald, D., et al. (2014). The lion in West Africa is critically endangered. PLoS ONE 9:e83500. doi: 10.1371/journal.pone.0083500
Irani, L., Vertesi, J., Dourish, P., Philip, K., and Grinter, R. E. (2010). “Postcolonial computing: a lens on design and development,” in Proceedings of the 2010 CHI Conference on Human Factors in Computing Systems-CHI'10 (Atlanta, GA: ACM Press), 1311–1320.
Kays, R., Crofoot, M. C., Jetz, W., and Wikelski, M. (2015). Terrestrial animal tracking as an eye on life and planet. Science 348:aaa2478. doi: 10.1126/science.aaa2478
Kemmis, S., and McTaggart, R. (2005). “Participatory action research: communicative action and the public sphere,” in The Sage Handbook of Qualitative Research, eds D. K. Norman and L. S. Yvonna (Thousand Oaks, CA: Sage Publications), 559–603.
Kotze, R., Keith, M., Winterbach, C. W., Winterbach, H. E. K., and Marshal, J. P. (2018). The influence of social and environmental factors on organization of African lion (Panthera leo) prides in the Okavango Delta. J. Mamm. 99, 845–858. doi: 10.1093/jmammal/gyy076
Loveridge, A. J., Valeix, M., Chapron, G., Davidson, Z., Mtare, G., and Macdonald, D. W. (2016). Conservation of large predator populations: demographic and spatial responses of African lions to the intensity of trophy hunting. Biol. Conserv. 204, 247–254. doi: 10.1016/j.biocon.2016.10.024
Mapedza, E., Wright, J., and Fawcett, R. (2003). An investigation of land cover change in Mafungautsi Forest, Zimbabwe, using GIS and participatory mapping. Appl. Geogr. 23, 1–21. doi: 10.1016/S0143-6228(02)00070-X
Medhi, I., Sagar, A., and Toyama, K. (2006). Text-free user interfaces for illiterate and semi-literate users. in 2006 International Conference on Information and Communication Technologies and Development (Berkeley, CA: IEEE), 72–82.
Mohr, C. O. (1947). Table of equivalent populations of North American small mammals. Am. Midl. Nat. 37, 223–249. doi: 10.2307/2421652
Montgomery, R. A., Elliott, K. C., Hayward, M. W., Gray, S. M., Millspaugh, J. J., Riley, S. J., et al. (2018b). Examining evident interdisciplinarity among prides of lion researchers. Front. Ecol. Evol. 6:49. doi: 10.3389/fevo.2018.00049
Montgomery, R. A., Hoffmann, C. F., Tans, E. D., and Kissui, B. (2018a). Discordant scales and the potential pitfalls for human-carnivore conflict mitigation. Biol. Conserv. 224, 170–177. doi: 10.1016/j.biocon.2018.05.018
Murray-Hudson, M. (2009). Floodplain Vegetation Responses to Flood Regime in the Seasonal Okavango Delta, Botswana. University of Florida.
Mutula, S., Grand, B., Zulu, S., and Sebina, P. (2010). Towards an Information Society in Botswana: ICT4D Country Report. in Research paper presented at Thetha Sangonet ICT4D Forum.
Ogada, D., Shaw, P., Beyers, R. L., Buij, R., Murn, C., Thiollay, J. M., et al. (2016). Another continental vulture crisis: Africa's vultures collapsing toward extinction. Conserv. Lett. 9, 89–97. doi: 10.1111/conl.12182
Ogada, D. L. (2014). The power of poison: pesticide poisoning of Africa's wildlife: poisoning Africa's wildlife with pesticides. Ann. N. Y. Acad. Sci. 1322, 1–20. doi: 10.1111/nyas.12405
Osipova, L., Okello, M. M., Njumbi, S. J., Ngene, S., Western, D., Hayward, M. W. H., et al. (2018). Fencing solves human-wildlife conflict locally but shifts problems elsewhere: a case study using functional connectivity modelling of the African elephant. J. Appl. Ecol. 55, 2673–2684. doi: 10.1111/1365-2664.13246
Packer, C., Loveridge, A., Canney, S., Caro, T., Garnett, S. T., Pfeifer, M., et al. (2013). Conserving large carnivores: dollars and fence. Ecol. Lett. 16, 635–641. doi: 10.1111/ele.12091
Pooley, S., Barua, M., Beinart, W., Dickman, A., Holmes, G., Lorimer, J., et al. (2017). An interdisciplinary review of current and future approaches to improving human-predator relations: improving human-predator relations. Conserv. Biol. 31, 513–523. doi: 10.1111/cobi.12859
Pröpper, M., Gröngröft, A., Finckh, M., Stirn, S., and De Cauwer, V. (2015). The Future Okavango: Findings, Scenarios and Recommendations for Action: Research Project Final Synthesis Report 2010-2015. University of Hamburg-Biocentre Klein Flottbek.
Pruneau, D., El Jai, B., Khattabi, A., Benbrahim, S., and Langis, J. (2018). “Using design thinking and facebook to accompany women in solving water problems in Morocco,” in Handbook of Sustainability Science and Research, ed W. Leal Filho (Cham: Springer International Publishing), 25–40.
QGIS Development Team (2016). QGIS Geographic Information System (Version 2.18.15 Las Palmas). Available online at: http://qgis.osgeo.org
Ramberg, L., Hancock, P., Lindholm, M., Meyer, T., Ringrose, S., Sliva, J., et al. (2006). Species diversity of the Okavango Delta, Botswana. Aqua. Sci. 68, 310–337. doi: 10.1007/s00027-006-0857-y
Raza, A. A., Saleem, B., Randhawa, S., Tariq, Z., Athar, A., Saif, U., et al. (2018). “Baang: a viral speech-based social platform for under-connected populations,” in Proceedings of the 2018 CHI Conference on Human Factors in Computing Systems - CHI'18 (Montreal, QC: ACM Press), 1–12.
Reddy, S. M. W., Montambault, J., Masuda, Y. J., Keenan, E., Butler, W., Fisher, J. R. B., et al. (2017). Advancing conservation by understanding and influencing human behavior: human behavior and nature. Conse. Lett. 10, 248–256. doi: 10.1111/conl.12252
Riggio, J., Jacobson, A., Dollar, L., Bauer, H., Becker, M., Dickman, A., et al. (2013). The size of savannah Africa: a lion's (Panthera leo) view. Biodivers. Conserv. 22, 17–35. doi: 10.1007/s10531-012-0381-4
Scholz, R. W., and Stauffacher, M. (2009). From a science for society to a science with society. Psychol. Rundschau 60, 242–280. doi: 10.1026/0033-3042.60.4.242
Schreier, M. (2014). “Qualitative content analysis,” in The Sage Handbook of Qualitative Data Analysis, ed Uwe Flick (Berlin: Free University Berlin), 170–183.
Schuler, D., and Namioka, A. (1993). Participatory Design: Principles and Practices. Boca Raton, FL; London; New York, NY: CRC Press.
Sheppard, J. K., McGann, A., Lanzone, M., and Swaisgood, R. R. (2015). An autonomous GPS geofence alert system to curtail avian fatalities at wind farms. Anim. Biotelesc. 3:43. doi: 10.1186/s40317-015-0087-y
Sherwani, J., Palijo, S., Mirza, S., Ahmed, T., Ali, N., and Rosenfeld, R. (2009). “Speech vs. touch-tone: telephony interfaces for information access by low literate users,” in 2009 International Conference on Information and Communication Technologies and Development (ICTD) (Doha: IEEE), 447–457.
Shivik, J. A. (2004). Non-lethal alternatives for predation management. Sheep Goat Res. J. 19, 64–71.
Sianga, K., and Fynn, R. (2017). The vegetation and wildlife habitats of the Savuti-Mababe-Linyanti ecosystem, northern Botswana. Koedoe 59:2. doi: 10.4102/koedoe.v59i2.1406
Songhurst, A. (2017). Measuring human-wildlife conflicts: comparing insights from different monitoring approaches: measuring human-wildlife conflict. Wildl. Soc. Bull. 41, 351–361. doi: 10.1002/wsb.773
Stander, P. (1990). A suggested management strategy for stock-raiding lions in Namibia. S. Afr. J. Wildl. Res. 20, 37–43.
Sterling, E. J., Betley, E., Sigouin, A., Gomez, A., Toomey, A., Cullman, G., et al. (2017). Assessing the evidence for stakeholder engagement in biodiversity conservation. Biol. Conserv. 209, 159–171. doi: 10.1016/j.biocon.2017.02.008
Thomas, B., Holland, J. D., and Minot, E. O. (2011). Wildlife tracking technology options and cost considerations. Wildl. Res. 38, 653–663. doi: 10.1071/WR10211
Tomkiewicz, S. M., Fuller, M. R., Kie, J. G., and Bates, K. K. (2010). Global positioning system and associated technologies in animal behaviour and ecological research. Philos. Trans. R. Soc. B 365, 2163–2176. doi: 10.1098/rstb.2010.0090
Torres, D. F., Oliveira, E. S., and Alves, R. R. N. (2018). Conflicts between humans and terrestrial vertebrates: a global review. Trop. Conserv. Sci. 11:194008291879408. doi: 10.1177/1940082918794084
Trinkel, M., and Angelici, F. M. (2016). “The decline in the lion population in africa and possible mitigation measures”. in Problematic Wildlife, ed F. M. Angelici (Cham, Springer), 45–68.
Trinkel, M., Fleischmann, P. H., and Slotow, R. (2017). Electrifying the fence or living with consequences? problem animal control threatens the long-term viability of a free-ranging lion population. J. Zool. 301, 41–50. doi: 10.1111/jzo.12387
Valletta, J. J., Torney, C., Kings, M., Thornton, A., and Madden, J. (2017). Applications of machine learning in animal behaviour studies. Anim. Behav. 124, 203–220. doi: 10.1016/j.anbehav.2016.12.005
van Eeden, L. M., Eklund, A., Miller, J. R. B., López-Bao, J. V., Chapron, G., Cejtin, M. R., et al. (2018). Carnivore conservation needs evidence-based livestock protection. PLoS Biol. 16:e2005577. doi: 10.1371/journal.pbio.2005577
Vitos, M., Altenbuchner, J., Stevens, M., Conquest, G., Lewis, J., and Haklay, M. (2017). “Supporting collaboration with non-literate forest communities in the congo-basin,” in Proceedings of the 2017 ACM Conference on Computer Supported Cooperative Work and Social Computing - CSCW'17 (Portland, OR: ACM Press), 1576–1590.
Wall, J., Wittemyer, G., Klinkenberg, B., and Douglas-Hamilton, I. (2014). Novel opportunities for wildlife conservation and research with real-time monitoring. Ecol. Appl. 24, 593–601. doi: 10.1890/13-1971.1
Weise, F. J., Fynn, R. W. S., Stein, A. B., Tomeletso, M., Somers, M. J., and Périquet, S. (2019). Can beef and beast coexist? Implications of seasonal cattle habitat selection for confict with lions in the northern Okavango Delta. Biol. Conserv.
Weise, F. J., Hayward, M. W., Casillas Aguirre, R., Tomeletso, M., Gadimang, P., Somers, M. J., et al. (2018). Size, shape and maintenance matter: a critical appraisal of a global carnivore conflict mitigation strategy – livestock protection kraals in northern Botswana. Biol. Conserv. 225, 88–97. doi: 10.1016/j.biocon.2018.06.023
Weld, G., Perrier, T., Aker, J., Blumenstock, J. E., Dillon, B., Kamanzi, A., et al. (2018). “eKichabi: information access through basic mobile phones in rural tanzania,” in Proceedings of the 2018 CHI Conference on Human Factors in Computing Systems - CHI'18 (Montreal, QC: ACM Press), 1–12.
Woodroffe, R., and Frank, L. G. (2005). Lethal control of African lions (Panthera leo): local and regional population impacts. Anim. Conserv. 8, 91–98. doi: 10.1017/S1367943004001829
Keywords: Panthera leo, conflict mitigation, geofencing, socio-informatics, alert system, early warning, coexistence, grounded design
Citation: Weise FJ, Hauptmeier H, Stratford KJ, Hayward MW, Aal K, Heuer M, Tomeletso M, Wulf V, Somers MJ and Stein AB (2019) Lions at the Gates: Trans-disciplinary Design of an Early Warning System to Improve Human-Lion Coexistence. Front. Ecol. Evol. 6:242. doi: 10.3389/fevo.2018.00242
Received: 19 October 2018; Accepted: 31 December 2018;
Published: 25 January 2019.
Edited by:
David Jack Coates, Department of Biodiversity, Conservation and Attractions (DBCA), AustraliaReviewed by:
Luke T. B. Hunter, Panthera Corporation, United StatesCopyright © 2019 Weise, Hauptmeier, Stratford, Hayward, Aal, Heuer, Tomeletso, Wulf, Somers and Stein. This is an open-access article distributed under the terms of the Creative Commons Attribution License (CC BY). The use, distribution or reproduction in other forums is permitted, provided the original author(s) and the copyright owner(s) are credited and that the original publication in this journal is cited, in accordance with accepted academic practice. No use, distribution or reproduction is permitted which does not comply with these terms.
*Correspondence: Florian J. Weise, Zmxvcmlhbi53ZWlzZUBnbWFpbC5jb20=
Disclaimer: All claims expressed in this article are solely those of the authors and do not necessarily represent those of their affiliated organizations, or those of the publisher, the editors and the reviewers. Any product that may be evaluated in this article or claim that may be made by its manufacturer is not guaranteed or endorsed by the publisher.
Research integrity at Frontiers
Learn more about the work of our research integrity team to safeguard the quality of each article we publish.