- Department of Biological Sciences, University of Rhode Island, Kingston, RI, United States
While prey responses to predators reduce the threat of consumption, the physiological costs of these responses can be considerable. This is especially true for organisms that lack effective anti-predator defenses and must rely on camouflage or mimicry for protection. The luna moth, Actias luna, is a large saturniid native to Eastern North America that is preyed on and parasitized by a wide variety of predators and parasitoids. We report the results of two separate experiments assessing the responses of Actias larvae to predatory wasps (Vespula maculifrons) that were rendered non-lethal but remained able to move freely, as well as in a control (wasp-free) treatment. We determined whether these responses were predator-specific by also testing the response of Actias larvae to a similarly-sized but harmless scavenging fly. In both experiments, (A) Actias larvae in the wasp treatment died at a higher rate than those in the control treatments; and (B) larval survival in the fly and control treatments did not differ. Despite similar Actias survival in the fly and control treatments, fly-treatment larvae that died appeared to respond similarly to flies as other larvae did to wasps. In both years, larvae that died in the fly and wasp treatments gained virtually no weight between the start of the experiment and their death, suggesting that they may have succumbed to starvation. Our results, replicated over 2 years, illustrate the high cost of anti-predator responses and are the first report of lethal risk effects in caterpillars.
Introduction
Predation risk can affect various aspects of prey behavior and physiology (Lima and Dill, 1990; Werner and Peacor, 2003; Adamo, 2012; Sheriff and Thaler, 2014). These changes are generally seen as adaptive since they reduce the likelihood of capture and/or consumption. Because they require prey to alter their investment in activities such as foraging, however, these changes can also negatively affect growth, physical condition, fecundity, and, in the most extreme cases, survival (Zanette et al., 2011; Siepielski et al., 2014; Duong and Mccauley, 2016). Exposure to predator cues decrease tadpole survival (Hettyey et al., 2015), for instance, and have a similar effect on dragonflies (McCauley et al., 2011) and grasshoppers (Schmitz et al., 1997).
Lepidopteran larvae are important terrestrial herbivores attacked by a wide variety of predators and parasitoids. Because they are slow-moving and lack a hard exoskeleton, they often rely on camouflage to avoid detection (Lichter-Marck et al., 2015). Foraging-related movement greatly increases caterpillar predation risk (Bernays, 1997), and camouflaged species may be especially likely to forego feeding in response to risk (Ruxton et al., 2004). Even individuals that resume feeding may suffer from the combined impact of reduced energy intake and the physiological costs of stress responses (Sheriff and Thaler, 2014).
The luna moth Actias luna (Lepidoptera: Saturniidae; Actias hereafter) is a silkmoth native to eastern North America (Wagner, 2005). The solitary larvae of this species require 3–6 weeks to mature, during which time camouflage provides their primary protection against predators and parasitoids (Tuskes et al., 1996). [Sourakov (2018); p. 488] characterized Actias defenses against predation as “…relying mostly on cryptic colouration and being motionless when not feeding,” although they possess spines that may reduce their vulnerability to vertebrate predators (Sourakov, 2018). Vespid wasps (Hymenoptera: Vespidae) are generalist predators that hunt caterpillars (Stamp and Bowers, 1988; Lichtenberg and Lichtenberg, 2003); we have repeatedly observed yellowjacket wasps (Vespula maculifrons; wasps hereafter) attacking and killing Actias larvae in the field (A. Baranowski, personal observation).
We conducted two separate experiments measuring the survivorship of Actias caterpillars in the presence of wasps rendered unable to either sting or bite. We determined whether these responses were predator-specific by also exposing caterpillars to (1) wasp-sized scavenging flies that had been similarly treated; and (2) a no-insect control. We hypothesized that survivorship would be lowest in the wasp treatment, and higher in both the fly and no-insect treatments.
Methods
2016 Experiment
In July 2016, a newly-emerged captive female Actias luna was mated to a wild male at East Farm (Kingston RI), an agricultural research facility managed by the University of Rhode Island. Eggs were incubated in a 473 ml polypropylene deli cup (Pactiv brand, Lake Forest, IL) and began hatching 10 days later. Hatchlings were offered hickory (Carya glabra) foliage, with waste removed and new foliage added as needed. Larvae were reared communally within the cup until the 2nd instar. To prevent overcrowding, larvae were then transferred to a 6 l polypropylene bin (Sterilite brand, Townsend, MA) until they averaged 1.5 cm in length, when they were transferred to a 12 l bin. Twenty-six days after hatching, 54 larvae were individually weighed (mean 0.673 ± 0.045 [SE] g) and transferred into individual 6 l bins containing hickory foliage kept hydrated using water-filled floral tubes. Once all larvae had been transferred, each bin was randomly assigned to one of three 18-bin treatment groups: a predator that had been rendered non-lethal, a similarly-treated harmless detritivore, and no-insect control.
Bins in the non-lethal predator (“wasp”) treatment each contained a single adult V. maculifrons collected from either flowers or overripe fruit; prior to the experiment, we had repeatedly observed wasps attacking and dismembering free-living Actias larvae. Captured wasps were first anesthetized by brief chilling in a freezer; when the adults were motionless, we applied one drop of UV-bonded plastic (Bondic brand, Aurora, ON) to both the mandible and stinger. Once each drop was applied, we immediately hardened it via exposure to a UV light. This procedure rendered each wasp “non-lethal,” alive and mobile but unable to either sting or bite potential prey; their non-lethal nature was confirmed via our repeated handling of test specimens with no stings or bites. We explored whether our addition of the glue affected wasp behavior via a pilot experiment in which we visually assessed the behavior of non-lethal and lethal wasps added singly to plastic bins. When resting, the non-lethal wasps spent more time grooming their mandibles than the lethal wasps; there were no other noticeable differences in time of flight or exploratory behavior. The wasp in each bin was checked daily and replaced with a new wasp when it died.
Bins in the harmless detritivore (“fly”) treatment each contained a single adult scavenging fly (families Caliphoridae and Sarcophagidae), of similar size as the wasps, collected from trash or reared from eggs. As with the wasps, we added UV-bonded plastic to the mouthparts and terminal abdominal segment of each fly. Each fly was handled the same way we handled the wasps. Flies were checked daily and replaced with a new one as needed.
Bins in the no-insect (“control”) treatment each received a single section of bamboo toothpick of wasp length, with one dot of UV-bonded plastic dots added to the end. Each bamboo toothpick was replaced every 2 days to simulate the level of disturbance received by the other two treatments.
After the experiment started, each bin was checked daily; food was replaced, waste removed, and treatments renewed as necessary. Larvae were weighed weekly and at either pupation or death; time (days) to either event was recorded for each larva. Each treatment was replicated 18 times for a total of 54 larvae.
2017 Experiment
To ensure that our results were robust, we repeated the experiment in 2017. The two experiments were identical except for the following differences. We collected eggs from three pairings (= broods) of different captive Actias females with wild males; eggs and larvae from the three different broods were held in separate containers. Brood one larvae hatched on July 13–18, brood two larvae hatched on August 3, and brood three larvae hatched on August 8. Larvae were reared on Juglans nigra throughout the experiment because of greater vegetation availability, and cut foliage was kept hydrated using microcentrifuge tubes filled with agar water (3 g/L agar:water). This latter procedure kept foliage fresh while preventing floral tube leakage. Larvae reaching their 3rd instar were transferred in groups of 25 to individual 6 l bins to prevent overcrowding. Data collection for experiment #2 was the same as for experiment #1, with the exception that the brood identity of each larva was recorded. A total of 86 larvae were used in the experiment, with each treatment replicated 28–29 times. Of these larvae, 21 larvae were from brood one (n = 7/treatment), 40 from brood two (n = 13–14), and 25 from brood three (n = 7–9).
Statistical Analysis
For experiment #1, we analyzed treatment-level differences in larval outcome [died, pupated] by fitting a GLM with a binomial distribution and logit link (maximum likelihood estimation method). We used GLM with a normal distribution and identity link to assess differences in percentage weight gain at, and time to, death or pupation, analyzed separately for each outcome. All p-values were obtained using likelihood-ratio χ2 tests.
For experiment #2, we assessed the individual effects of treatment, brood identity, and their interaction on larval outcome by fitting a GLMM with a binomial error distribution and logit link function. Because the Hessian matrix suggested quasi-complete separation, we reran the model using bias-adjusted estimates (Firth adjusted maximum likelihood). Brood identity was used as a random effect in the model, and p-values were obtained by performing likelihood-ratio χ2 tests. A linear mixed effects modeling approach was also used to analyze weight at and time to pupation (for surviving larvae), or weight at and time to death. These variables were analyzed separately for each outcome; treatment was coded as a fixed effect and brood identity as a random effect. Chi-square and p-values were obtained as above.
All analyses were conducted using JMP 9.0.0 (SAS Institute, Cary NC).
Results
2016 Experiment
More wasp-cue larvae died than in the other two treatments. Only 17% of wasp-exposed larvae pupated, vs. 50% of larvae in the fly-cue and control treatments [Figure 1A; = 6.04, p = 0.049]. Of the larvae that pupated, mass at pupation and time to pupation did not differ among the three treatments (both p > 0.20; Table 1).
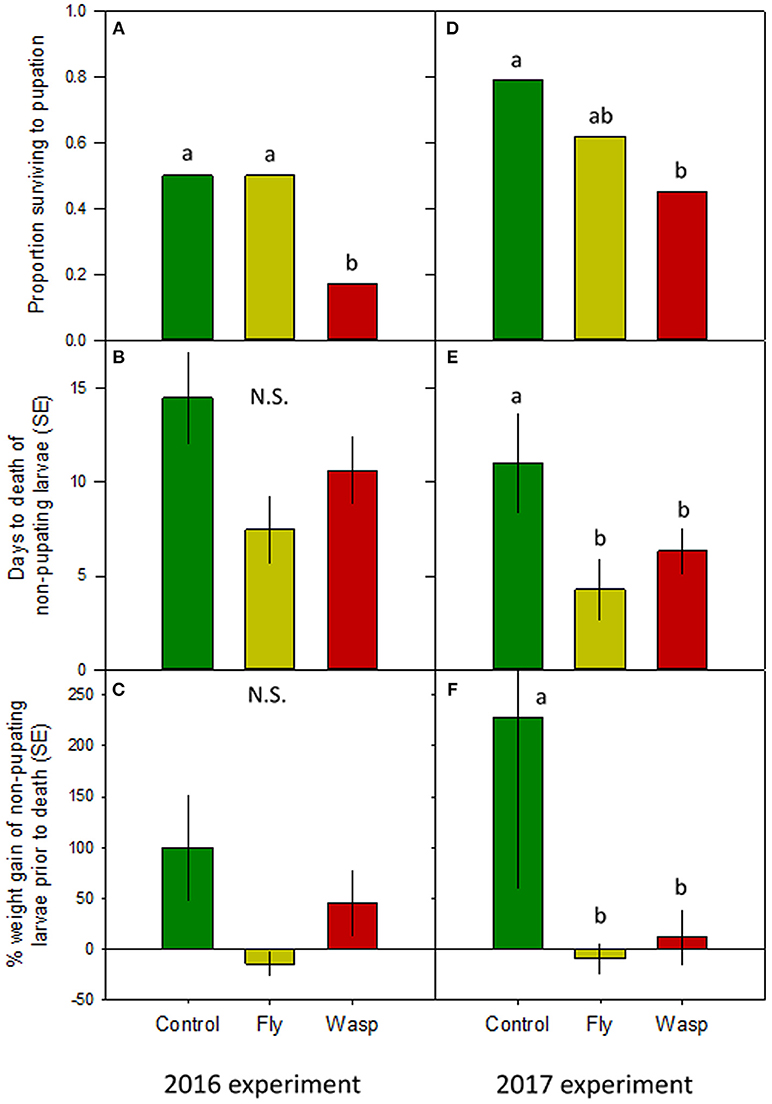
Figure 1. Impact of varying risk cues on Actia luna larval development. Left-hand panels: 2016 experiment (n = 18/treatment); right-hand panels: 2017 experiment (n = 28–29/treatment). (A,D): Proportion of A. luna larvae surviving to pupation. (B,E): Mean ± SE days from the start of the experiment to death of non-pupating larvae. (C,F): Percentage ± SE weight gain from hatching to death of non-pupating larvae. Green bars: no risk cues; yellow bars: risk cues from harmless scavenging fly; red bars: risk cues from Vespula sp. predatory wasp. Lower-case letters indicate treatments similar at α = 0.05 (post-hoc Tukey HSD); N.S. = no significant differences among treatments.
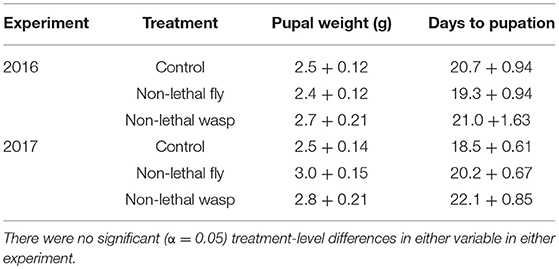
Table 1. Weight at pupation (g) ± SE and days to pupation ± SE for larvae surviving to pupation in the 2016 (top) and 2017 (bottom) experiments.
Although the treatments did not affect pupated larvae, there was a marginal between-treatment difference in the time to death of larvae dying prior to pupation (Figure 1B). Control larvae that died prior to pupation lived seven and 4 days longer than larvae in the fly- and wasp-cue treatments, respectively [X2(2df) = 5.15, p = 0.076]. Larvae that died prior to pupation also gained similar amounts of weight prior to their death [Figure 1C; X2(2df) = 4.30, p = 0.116].
2017 Experiment
Although more larvae in all three treatments survived to pupation (39 and 61% survival in 2016 and 2017, respectively), only the wasp-cue treatment differed from the control [Figure 1D; = 13.30, p = 0.001]. As in 2016, there were no treatment-level differences in time to, or weight gain at, pupation (both p > 0.3; Table 1).
There were substantial treatment-level differences between larvae that died before pupation. Larval longevity was greatest in the control treatment and lower in the fly- and wasp-cue treatments [Figure 1E; X2(2df) = 6.18, p = 0.045]; a similar pattern was seen in percentage weight gain prior to death [Figure 1F; X2(2df) = 6.36, p = 0.042].
The three broods differed overall and in their treatment response (Supplementary Information). Overall survival was highest for brood three [88%; “brood”: X2(2df) = 15.3, p < 0.001], while brood one responded most strongly to the treatments [“treatment*brood”: X2(4df) = 12.9, p = 0.012]. Brood three also took longer to pupate [X2(2df) = 14.8, p < 0.001] and gained more weight prior to pupation [X2(2df) = 9.2, p = 0.010]; this likely reflects the greater number of brood three larvae surviving to pupation.
While we did not take any data on predator or prey behavior, the wasps and flies appeared to behave similarly in both experiments. For the first several hours following their individual addition to a Actias-containing plastic bin, both types of insects spent most of their time flying between perches where they sat while attempting to groom their mouthparts. Wasps appeared more agitated than flies during the grooming period and would often buzz their wings while grooming; this behavior was never observed with flies. After this first period, both wasps and flies were predominantly found walking on the walls of the plastic bin with occasional short (5–8 cm) flights between walls. Neither type of insect appeared interested in the Actias larva and were only rarely observed in physical contact with it.
Discussion
Our two experiments, conducted in different years with different populations, found that predation risk decreases Actias survival by ~55% (66 and 43% in 2016 and 2017, respectively) relative to control treatments, while exposure to a similarly-sized and -treated detritivorous fly did not. This appears to be the first direct evidence that risk alone can increase prey mortality in lepidopterans.
The impact of predator cues may reflect the heavy reliance of Actias larvae on camouflage for predator defense. While their spines and strong grip on twigs and branches may deter vertebrate predators (Sourakov, 2018), Vespula sp. wasps dismember larger caterpillars in situ (Lichtenberg and Lichtenberg, 2003). Feeding by caterpillars greatly increases their vulnerability to wasp predation (Bernays, 1997), and Actias that perceive risk “freeze” in place (A. Baranowski, personal observation). Confining larvae and wasps together (the drawbacks of which are discussed below) decreases or stops feeding, as indicated by the minimal weight gain of larvae dying in the wasp treatment (Figure 1F). Exposure to foraging honeybees similarly reduces feeding, and thus plant damage, by Spodoptera exigua caterpillars (Tautz and Rostás, 2008). The fact that honeybees pose no threat to S. exigua suggests that hymenopteran buzzing, especially in combination with volatile and visual cues, may be a general risk cue for caterpillars (Tautz and Markl, 1978).
The risk-induced increase in Actias mortality is consistent with findings from aquatic predator-prey systems in which predator cues reduced the survivorship of both tadpoles (Hettyey et al., 2015) and larval dragonflies (McCauley et al., 2011). In a terrestrial system, Stamp and Bowers (1991) used data on weight gain of buckmoth (Hemileuca lucina) caterpillars in the presence and absence of wasps to infer the risk-induced increase in caterpillar mortality. They estimated that exposure to wasps reduced survival by 20.3% via reductions in food intake that slowed growth and increased the larval period. Our study builds on theirs by providing the first directly-measured evidence that risk increases mortality in a terrestrial predator-prey system. More generally, the strong responses of multiple lepidopteran species to risk cues (Tautz and Markl, 1978; Stamp, 1997; Johnson et al., 2007) suggests that similar results may occur in a range of systems and play an important but relatively unappreciated role in plant-herbivore-natural enemy interactions in natural and managed ecosystems.
Low mortality in the fly treatment (Figures 1A,D) can be interpreted as suggesting that Actias differentiate between predators and other similarly-sized but harmless flying insects. This interpretation agrees with work in both aquatic and terrestrial systems (e.g., Bass and Gerlai, 2008; Zanette et al., 2011) showing that prey can distinguish between cues from similarly-sized dangerous and harmless species. It is not, however, consistent with our data on larvae that died prior to pupation. If Actias perceived flies as less risky, the time to death (Figures 1B,E) and weight gain prior to death (Figures 1C,F) of fly-exposed larvae should be either similar to, or slightly less than, the control treatment. Instead, both metrics were identical to those seen in the wasp treatment: fewer larvae died in the fly treatment, but those that did appeared to respond as strongly to flies as their counterparts did to wasps. This may suggest that individual Actias have different “risk thresholds” that determine their reaction to cues (i.e., the shy-bold continuum; Sih et al., 2012). Larvae with high risk thresholds would err on the side of boldness and continue to forage even when a predator might be present. Conversely, larvae with lower thresholds would cease feeding even when exposed to low-risk cues such as the buzzing of a fly. Such risk thresholds would also explain the similar size and larval period of successfully-pupating larvae; individuals that did not perceive their environment as risky should have similar times to and size at pupation. If true, then our work may point more toward Actias larvae distinguishing between different risk levels rather than discriminating between predatory wasps and similarly-sized but harmless flies.
The brood-level differences we observed suggest the potential for ecologically-relevant intraspecific variation in risk responses (Bolnick et al., 2011). Since the broods emerged at different times, we cannot rule out the possibility that our results are explained by phenology rather than genetic differences. Our increasing awareness of the impact of maternal stress on offspring phenotypes (Sheriff et al., 2018), however, argues strongly for additional research into this topic.
The fact that risk alone is sufficient to reduce caterpillar survival suggests several important areas of future research. Perhaps the most important is the nature of the predator cue; while other researchers have found “buzzing” important in caterpillar risk assessment (Tautz and Markl, 1978; Tautz and Rostás, 2008), chemical and visual cues may well complement and enhance auditory inputs. In retrospect, it would have been useful to include a “no-contact” treatment in which the non-lethal wasps were prevented from having the opportunity to physically touch the caterpillar; this would have allowed us to determine whether direct contact between the predator and its prey contributed to the observed effect. It is also important to consider how continuously confining caterpillars and cues together in an enclosed space might have affected our results. Although many studies have used continual risk exposure to understand its effects (reviewed in Ferrari et al., 2009), the variability of risk in natural settings should affect caterpillar responses. Finally, our work highlights the need for future studies linking risk-mediated changes in foraging time (Johnson et al., 2007) and assimilation efficiency (Thaler et al., 2012) to individual survival.
Author Contributions
EP and AB designed the experiment, AB conducted the experiment, and EP and AB jointly analyzed the data and wrote the manuscript.
Conflict of Interest Statement
The authors declare that the research was conducted in the absence of any commercial or financial relationships that could be construed as a potential conflict of interest.
Acknowledgments
This paper benefitted greatly from the comments of J. Blundell, C. Conroy, J. Orrock, and two reviewers.
Supplementary Material
The Supplementary Material for this article can be found online at: https://www.frontiersin.org/articles/10.3389/fevo.2018.00220/full#supplementary-material
References
Adamo, S. A. (2012). The effects of the stress response on immune function in invertebrates: an evolutionary perspective on an ancient connection. Horm. Behav. 62, 324–330. doi: 10.1016/j.yhbeh.2012.02.012
Bass, S. L. S., and Gerlai, R. (2008). Zebrafish (Danio rerio) responds differentially to stimulus fish: The effects of sympatric and allopatric predators and harmless fish. Behav. Brain Res. 186, 107–117. doi: 10.1016/j.bbr.2007.07.037
Bernays, E. A. (1997). Feeding by lepidopteran larvae is dangerous. Ecol. Entomol. 22, 121–123. doi: 10.1046/j.1365-2311.1997.00042.x
Bolnick, D. I., Amarasekare, P., Araújo, M. S., Bürger, R., Levine, J. M., Novak, M., et al. (2011). Why intraspecific trait variation matters in community ecology. Trends Ecol. Evol. 26, 183–192. doi: 10.1016/j.tree.2011.01.009
Duong, T. M., and Mccauley, S. J. (2016). Predation risk increases immune response in a larval dragonfly (Leucorrhinia intacta). Ecology 97, 1605–1610. doi: 10.1890/15-1964.1
Ferrari, M. C. O., Sih, A., and Chivers, D. P. (2009). The paradox of risk allocation: a review and prospectus. Anim. Behav. 78, 579–585. doi: 10.1016/j.anbehav.2009.05.034
Hettyey, A., Tóth, Z., Thonhauser, K. E., Frommen, J. G., Penn, D. J., and Van Buskirk, J. (2015). The relative importance of prey-borne and predator-borne chemical cues for inducible antipredator responses in tadpoles. Oecologia 179, 699–710. doi: 10.1007/s00442-015-3382-7
Johnson, M.-L., Armitage, S., Scholz, B. C. G., Merritt, D. J., Cribb, B. W., and Zalucki, M. P. (2007). Predator presence moves Helicoverpa armigera larvae to distraction. J. Insect Behav. 20, 1–18. doi: 10.1007/s10905-006-9048-x
Lichtenberg, J. S., and Lichtenberg, D. A. (2003). Predation of caterpillars on understory saplings in an Ozark forest. Southeastern Nat. 2, 423–432. doi: 10.1656/1528-7092(2003)002[0423:POCOUS]2.0.CO
Lichter-Marck, I. H., Wylde, M., Aaron, E., Oliver, J. C., and Singer, M. S. (2015). The struggle for safety: effectiveness of caterpillar defenses against bird predation. Oikos 124, 525–533. doi: 10.1111/oik.01515
Lima, S., and Dill, L. (1990). Behavioral decisions made under the risk of predation: A review and prospectus. Can. J. Zool. 68, 619–640. doi: 10.1139/z90-092
McCauley, S. J., Rowe, L., and Fortin, M.-J. (2011). The deadly effects of “nonlethal” predators. Ecology 92, 2043–2048. doi: 10.1890/11-0455.1
Ruxton, G., Sherratt, T., and Speed, M. (2004). Avoiding Attack: The Evolutionary Ecology of Crypsis, Warning Signals and Mimicry. Oxford: Oxford University Press. doi: 10.1093/acprof:oso/9780198528609.001.0001
Schmitz, O., Beckerman, A., and O'brien, K. (1997). Behaviorally-mediated trophic cascades: effects of predation risk on food web interactions. Ecology 78, 1388–1399. doi: 10.1890/0012-9658(1997)078[1388:BMTCEO]2.0.CO;2
Sheriff, M. J., Dantzer, B., Love, O. P., and Orrock, J. L. (2018). Error management theory and the adaptive significance of transgenerational maternal-stress effects on offspring phenotype. Ecol. Evol. 2018, 1–10. doi: 10.1002/ece3.4074
Sheriff, M. J., and Thaler, J. S. (2014). Ecophysiological effects of predation risk; an integration across disciplines. Oecologia 176, 607–611. doi: 10.1007/s00442-014-3105-5
Siepielski, A. M., Wang, J., and Prince, G. (2014). Nonconsumptive predator-driven mortality causes natural selection on prey. Evolution 68, 696–704. doi: 10.1111/evo.12294
Sih, A., Cote, J., Evans, M., Fogarty, S., and Pruitt, J. (2012). Ecological implications of behavioural syndromes. Ecol. Lett. 15, 278–289. doi: 10.1111/j.1461-0248.2011.01731.x
Sourakov, A. (2018). Size, spines and crochets: defences of luna moth caterpillars against predation by brown anoles. J. Nat. Hist. 52, 483–490. doi: 10.1080/00222933.2018.1439540
Stamp, N. E. (1997). Behavior of harassed caterpillars and consequences for host plants. Oikos 79, 147–154. doi: 10.2307/3546099
Stamp, N. E., and Bowers, M. D. (1988). Direct and indirect effects of predatory wasps (Polistes sp.: Vespidae) on gregarious caterpillars (Hemileuca lucina: Saturnidae). Oecologia 75, 619–624. doi: 10.1007/BF00776428
Stamp, N. E., and Bowers, M. D. (1991). Indirect effect on survivorship of caterpillars due to presence of invertebrate predators. Oecologia 88, 325–330. doi: 10.1007/BF00317574
Tautz, J., and Markl, H. (1978). Caterpillars detect flying wasps by hairs sensitive to airborne vibration. Behav. Ecol. Sociobiol. 4, 101–110. doi: 10.1007/BF00302564
Tautz, J., and Rostás, M. (2008). Honeybee buzz attenuates plant damage by caterpillars. Curr. Biol. 18, R1125–R1126. doi: 10.1016/j.cub.2008.10.038
Thaler, J., Mcart, S., and Kaplan, I. (2012). Compensatory mechanisms for ameliorating the fundamental trade-off between predator avoidance and foraging. Proc. Natl. Acad. Sci. U.S.A. 109, 12075–12080. doi: 10.1073/pnas.1208070109
Tuskes, P. M., Tuttle, J. P., and Collins, M. M. (1996). The Wild Silk Moths of North America: A Natural History of the Saturniidae of the United States and Canada. Ithaca NY: Cornell University Press.
Wagner, D. L. (2005). Caterpillars of Eastern North America. Princeton NJ: Princeton University Press.
Werner, E., and Peacor, S. (2003). A review of trait-mediated indirect interactions in ecological communities. Ecology 84, 1083–1100. doi: 10.1890/0012-9658(2003)084[1083:AROTII]2.0.CO;2
Keywords: Actia luna, Vespula maculifrons, predation risk, predation, non-consumptive effect, anti-predator behavior
Citation: Baranowski AK and Preisser EL (2018) Predator Cues Increase Silkmoth Mortality. Front. Ecol. Evol. 6:220. doi: 10.3389/fevo.2018.00220
Received: 23 August 2018; Accepted: 04 December 2018;
Published: 18 December 2018.
Edited by:
Shannon J. McCauley, University of Toronto Mississauga, CanadaReviewed by:
Ben G. Van Allen, University of California, San Diego, United StatesPeter Schausberger, Universität Wien, Austria
Copyright © 2018 Baranowski and Preisser. This is an open-access article distributed under the terms of the Creative Commons Attribution License (CC BY). The use, distribution or reproduction in other forums is permitted, provided the original author(s) and the copyright owner(s) are credited and that the original publication in this journal is cited, in accordance with accepted academic practice. No use, distribution or reproduction is permitted which does not comply with these terms.
*Correspondence: Evan L. Preisser, preisser@uri.edu