- 1AgResearch Limited, Christchurch, New Zealand
- 2Bio-Protection Research Centre, School of Biological Sciences, University of Canterbury, Christchurch, New Zealand
- 3Bio-Protection Research Centre, Lincoln University, Lincoln, New Zealand
Sustainable and integrated pest management often involves insect parasitoids. However, the effectiveness of parasitoids biocontrol has often failed, frequently for obscure reasons. A parasitoid's success is partly due to its behavioral response to pest density, i.e. its consumer functional response. For many years in New Zealand, a braconid parasitoid, Microctonus hyperodae successfully suppressed a severe ryegrass weevil pest, Listronotus bonariensis. However, there is now evidence that this has severely declined, but that the extent of decline can depend on the pasture species. Here, we tested whether the current functional responses of M. hyperodae to L. bonarensis in two of the most common New Zealand pasture grasses (Lolium multiflorum and L. perenne) reflect observed differences in field parasitism and whether this functional response has changed over time. Our analysis involved data from 1993 and 2018. We found a type I functional response in L. multiflorum in both years, but the slope of the relationship declined over time. There was no evidence for any type of functional response in L. perenne. This lack of response in L. perenne coincided with consistently found lower parasitism rates on this host plant than in L. multiflorum; both in the field and laboratory. Here, we found that apparently declining searching efficiency was correlated with the decline in parasitism. This observation supports the hypothesis that parasitism decline could be the result of evolution of resistance based on enhanced evasive behavior by L. bonariensis.
Introduction
Adequate global nutritious and healthy food production in the face of growing human population will require sustainable pest management methods (Bruinsma, 2003; Ramankutty et al., 2018). Insect parasitoids are often a significant component of integrated pest management in agriculture. Also parasitoids are important subjects of behavioral and population studies because they are remarkably common in nature, are frequently easy to raise and to handle and, mostly, key species for the control of many insect pests (Godfray et al., 1994; Fernández-Arhex and Corley, 2003; Begg et al., 2017). However, in general, biological control based on parasitoids has more often failed than succeeded, with difficulties in identifying the reasons (Hawkins and Cornell, 1999; Fernández-Arhex and Corley, 2003).
Among the attributes thought to be related to the success of parasitoid biocontrol agents is the behavior of individual parasitoids in response to host density (Huffaker et al., 1971; Barlow et al., 1993; Berryman, 1999). Such behavior is called ‘consumer functional response’ and is defined as the relationship between the number of hosts attacked by a predator as a function of prey density (Solomon, 1949; Holling, 1959, 1966). Functional response studies have been used by evolutionary biologists and ecologists to clarify co-evolutionary relationships, and to infer basic mechanisms underlying the interactions of predator-prey or parasitoid-host behavior (Houck and Strauss, 1985). Holling (1959) described three types of functional responses. The type I response describes a linear relationship between the number of hosts parasitised and host density, where the slope is the parasitoid's searching efficiency. The type II response is an asymptotic parasitism curve that constantly decelerates as host numbers increase, due to the time it takes the parasitoid to handle the host. The asymptote reflects the maximum attack rate. The type III response is a sigmoid curve: as host density rises, the response initially accelerates due to the parasitoid becoming increasingly efficient at finding hosts (attack rate increases or handling time decreases) then levels off under the influence of handling time or satiation (Berryman, 1999; Hassell, 2000).
Holling (1959) suggested that the type II response may be typical of invertebrate predators (including parasitoids) because they are limited by a fixed time to search for hosts. However, later work suggested that parasitoids may well-display type III response (Van Lenteren and Bakker, 1975; Hassell et al., 1977) because, at least theoretically, predators that exhibit a type III response points to their ability to regulate their hosts (Bernal et al., 1994). Related to this, the ability of parasitoids to locate hosts at low densities has become one criterion for the selection of candidates for classical biocontrol introductions (Barlow et al., 1993).
For many years the impact of the severe ryegrass pest, Argentine stem weevil (ASW) Listronotus bonariensis (Kuschel) (Coleoptera: Curculionidae), on New Zealand's improved grassland ecosystems was successfully suppressed by the South American braconid parasitoid control agent Microctonus hyperodae Loan (Hymenoptera: Braconidae) (Barker and Addison, 2006). However, this is no longer the case; that M. hyperodae is losing its efficacy has been highlighted by recent systematic field and laboratory studies designed to compare historical and contemporary parasitism rates (Goldson et al., 2014a,b, 2015; Goldson and Tomasetto, 2016; Tomasetto et al., 2017c, 2018). These studies also found significant differences in L. bonariensis parasitism rates in two common grasses in New Zealand's improved pastures, tetraploid Lolium multiflorum Lam. and diploid L. perenne L. The reduced parasitism rate was particularly apparent in L. perenne (Tomasetto et al., 2017a,b). The possible mechanisms of this decline over time and the causes of the differences in parasitism rates between grass types, have yet to be fully determined.
In this study, we tested (1) whether the current functional response of M. hyperodae to the density of its host pest, L. bonarensis, differs between two of the most common pasture grass species where parasitism rates have been shown to differ significantly in New Zealand agroecosystems, and (2) whether the functional response of M. hyperodae to L. bonarensis density has changed over the last 25 years, potentially being related to the changes in parasitism rates.
Materials and Methods
Experimental Design
Weevil adults were collected from a Lincoln farm (−43.6281, 172.4361) using a modified leaf blowing machine (Goldson et al., 2000) between 3rd and 4th January 2018. These were then purged of egg and larval parasitoids by storing them for 41 to 42 days at ambient laboratory temperatures (23 ± 2°C) and 16:8 L:D photoperiod (similar settings to those of Goldson and Tomasetto (2016). The M. hyperodae pupae, which emerged from these weevils, were then reared to obtain adult parasitoids for this study while the unparasitised portion of the L. bonariensis population was used for the experiment.
Ten 2 × 2 m field cages were set up each over a 2 × 4 m tarpaulin. Inner mesh barriers, attached with strips of Velcro, were set up to divide each cage into four arenas, resulting in 40 total arenas. A spray foam sealant and duct tape were used to seal the cage edges (Figure S1A). All experimental work was established on 14th February 2018 using 450 × 340 mm × 640 mm translucent plastic bins. Twenty 550 mm × 305 mm × 45 mm propagation trays of L. multiflorum and 20 trays with L. perenne were assigned in each bin (Figure S1B). We used tetraploid L. multiflorum (cv. Hogan) and tetraploid L. perenne (cv. Bealy) without endophytes, which can confer upon the grasses resistance to weevils that could affect the interaction with parasitoids. The description of these cultivars amongst New Zealand's pastureland species can be found in Stewart et al. (2014).
We used an experimental regression design for this study (Quinn and Keough, 2002), where we manipulated the density of weevils in each arena for the two different grass species. In each arena, we placed one tray with either L. multiflorum or L. perenne, and these were stocked with two parasitoids together with one of ten weevil densities (nweevils = 25, 50, 75, 100, 125, 150, 175, 200, 225, 250). These combinations of weevil densities and pasture species were randomly allocated to the arenas. After 10 days, the weevils were removed from the cages by flotation in a concentrated ammonium sulfate solution (Proffitt et al., 1993), and frozen at −20°C prior to being dissected to assess percent parasitism rates (i.e. number of parasitized weevils per total number of weevils dissected).
To determine whether functional responses have changed with time, we used historical data from a similar experiment on L. multiflorum (with low levels of fungal alkaloids such as peramine) carried out between October 1991 and February 1993 by Barlow et al. (1993). The latter study was also conducted on flat land, used same soil type, the same time of the year and a virtual monoculture of L. multiflorum. However, in their publication their analysis was restricted to the October 1991 data. But, in the interests of seasonal congruency we used Barlow et al. (1993) unpublished February 1993 data to compare with February 2018 parasitism data. Further, we took into account the host density range in Barlow et al. (1993) experiment when designing the experiment described here, to make possible comparisons between the years (they used 25, 50, 125, and 200 weevils/m2). In Barlow et al. (1993) however, only a fraction of weevils were found at the end of the experiment. We used the number of recovered weevils from both experiments to compare the results.
Parasitism Rates Comparison and Functional Response Analysis
We used an ANOVA on a generalized linear model to test whether parasitism rates were different between the two species of pasture in the 2018 experiment, and a separate ANOVA on a generalized linear model to test the differences between the L. multiflorum experiment of 1993 and 2018. In both models we used “quasi-binomial” error distribution to account for overdispersion of the data.
By dissecting the weevils recovered from the arenas at the end of the experiment we tested for a Type I parasitism response using a generalized linear model with a Gaussian error distribution. The same model was also applied to the historical data (February 1993). To test for Type II and III responses, we fitted Holling (1959) models to the data using the tools in the FRAIR R package (Pritchard et al., 2017) in R version 3.4.1 (R Development Core Team, 2018). These models were optimized using maximum likelihood estimation combined with a robust approach to fitting nonlinear models. A binomial likelihood function was used allowing for optimisation on the basis of arbitrary probability distributions (including Z-statistics and p-values; Bolker et al., 2013).
The slope of the Type I functional response represents the parasitoid's searching efficiency (Holling 1959), therefore we tested whether parasitism rates were related to parasitoid searching efficiency by using a generalized linear model (with Gaussian error distribution) of the parasitism rate as a function of the slope of the Type I response.
Results
We found that parasitism rates were significantly higher in L. multiflorum than in L. perenne under the 2018 experimental conditions (p = 0.01, F = 7.8, df = 18; Figure 1A). Also, overall parasitism rates were significantly higher in the 1993 experiment than in 2018 (p < 0.005, F = 31.5, df = 28; Figure 1A).
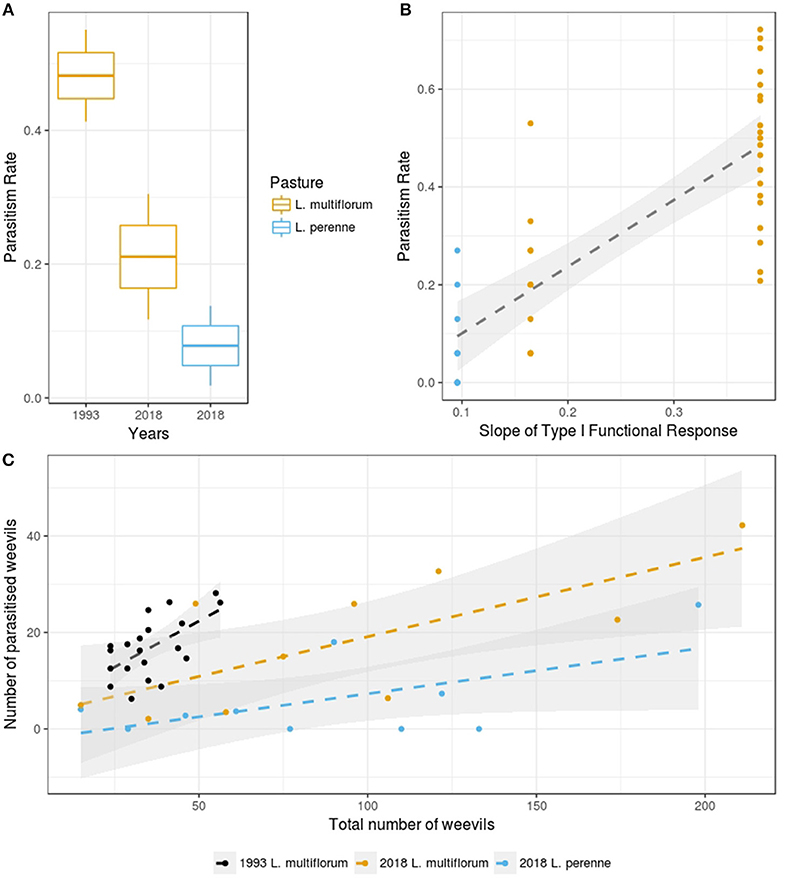
Figure 1. (A) Parasitism rates for the different years and the different pastures of the current experiment. The boxes show the mean and standard error of the data, and the whiskers show the standard deviation of the data (yellow colors indicate Lolium multiflorum and blue colors indicate L. perenne). (B) Relationship between the slope of the Type I functional response and parasitism rates for the combined historical and current data (i.e., 1993 and 2018 data). The 95% confidence intervals are based on bootstrapped model fitting (yellow dots indicate Lolium multiflorum and blue dots indicate L. perenne). (C) Relationship between recovered weevil density and number of weevils parasitized (black dots indicate 1993 data in L. multiflorum, yellow dots indicate current data in L. multiflorum and blue dots indicate current data in L. perenne). The 95% confidence intervals are based on bootstrapped model fitting.
We found no evidence in the 2018 data of any functional response in the L. perenne treatment. There was also a significant positive relationship between the slopes of the Type I functional responses in the L. multiflorum in 1993 and 2018 experiments (p < 0.005, R2 = 0.62; Figure 1B). We found no evidence of type II and III functional responses in any of the years or pasture types. However, we found a type I responses in L. multiflorum in both the historical 1993 data (p-value = 0.005, t = 3.14, slope = 0.38) and in 2018 data (p = 0.015, t = 3.07, slope = 0.16; Figure 1C).
Discussion
This study measured ASW parasitism rates by M. hyperodae under different host-parasitoid ratios in two common New Zealand ryegrass pasture species under controlled experimental conditions. We found a Type I host-parasitoid functional response using data collected from L. multiflorum plants in both 1993 and current experiment. However, the slopes of these functional responses were different, with the historical one being significantly greater than the current one. This suggests a decline in searching efficiency in L. multiflorum since 1993, indicating that the efficacy of parasitoid biocontrol had declined in this grass species and corresponds to that which has been generally found elsewhere, especially in L. perenne (Goldson and Tomasetto, 2016).
Based on the data they collected in October 1991, (Barlow et al., 1993) did not find a parasitoid functional response in L. multiflorum. However, they noted that this was possibly due to the significant weevil mortality in the cages where the weevil populations had declined to such an extent that there was effectively no weevil density treatments (Goldson et al., 1993). ASW collected in October typically show high mortality due to overwintering stress and senescence (Goldson et al., 2011). A similar decline in ASW density did not occur in this study despite the rigors of collection, rearing and release into the cages. This is highly likely to have been because the February collected weevils were only recently emerged.
Under the controlled experimental conditions used in this study, the lack of functional response in the L. perenne plots suggests that parasitism rates were not affected by ASW density. Moreover, parasitism rates in L. perenne grass were far lower than those found in L. multiflorum. This conforms to earlier findings in the field and laboratory experiments which showed that host-plant species did indeed significantly affect parasitism rates (Goldson and Tomasetto, 2016; Tomasetto et al., 2017a,c). As a possible mechanism for this, it has been posited that the tendency for tetraploid L. multiflorum to have fewer and larger tillers may offer less opportunity to behavioral-based escape responses by the weevil (Tomasetto et al., 2017b). Such behaviourally-mediated resistance to M. hyperodae by ASW was originally suggested by Goldson et al. (2015) based on the observation that the weevils tended to move off the foliage toward the soil when parasitoids were introduced (Gerard, 2000). It is further hypothesized that this sort of response has become enhanced through consistent parasitoid selection pressure and is under investigation elsewhere. In point of fact, the escape-response hypothesis has been supported by this study which showed the significant decline in the slope of the Type I functional response in L. multiflorum (from 0.38 to 0.16) since the 1990s, indicating a reduction in searching efficiency (via increased evasion by ASW).
Finally the results presented here are consistent with those in earlier studies where parasitism rates was found to be higher in tetraploid L multiflorum than diploid L. perenne (Goldson et al., 2015; Goldson and Tomasetto, 2016). Should selection pressure be the underlying mechanism for this decline, we should expect its expression to be most apparent in L. perenne, this species being the most common pasture grass in New Zealand.
Author Contributions
FT and PC conceived the idea. SB, FT, and PC collected the data. PC analyzed the data with advice from FT. All authors contributed to the writing of the manuscript.
Funding
The work was funded by the AgResearch Curiosity Fund granted to FT.
Conflict of Interest Statement
FT, SB, and SG were employed by AgResearch Ltd.
The remaining authors declare that the research was conducted in the absence of any commercial or financial relationships that could be construed as a potential conflict of interest.
Acknowledgments
The authors gratefully acknowledge Jason Tylianakis (University of Canterbury) for kindly provided field cages and for his expert technical and editorial advice. Mark McNeill (AgResearch) for providing the historical data. Philippa Gerard and John Kean (AgResearch) for editorial advice. Janet Montgomery (Agriseeds) for kindly provided seeds. Shaun Monk (Grasslanz) for kindly provided the collection sites. John Proffitt assisted energetically with the cage assembling. Malvika Bana contributed to the experiment establishment.
Supplementary Material
The Supplementary Material for this article can be found online at: https://www.frontiersin.org/articles/10.3389/fevo.2018.00215/full#supplementary-material
References
Barker, G. M., and Addison, P. J. (2006). Early impact of endoparasitoid Microctonus hyperodae (Hymenoptera: Braconidae) after its establishment in Listronotus bonariensis (Coleoptera: Curculionidae) populations of northern New Zealand pastures. J. Econ. Entomol. 99, 273–287. doi: 10.1093/jee/99.2.273
Barlow, N. D., Goldson, S. L., McNeill, M. R., and Proffitt, J. R. (1993). “Measurement of the attack behaviour of the parasitoid microctonus hyperodae as a classical biological control agent of argentine stem weevil listrontous bonariensis,” in Proceedings of the 6th Australasian Conference on Grassland Invertebrate Ecology. (ed.) R. A. Prestidge (Hamilton Ruakura Agricultural Centre).
Begg, G. S., Cook, S. M., Dye, R., Ferrante, M., Franck, P., Lavigne, C., et al. (2017). A functional overview of conservation biological control. Crop Prot. 97, 145–158. doi: 10.1016/j.cropro.2016.11.008
Bernal, J. S., Bellows, T. S., and Gonzalez, D. (1994). Functional response of Diaeretiella rapae (McIntosh)(Hym., Aphidiidae) to Diuraphis noxia (Mordwilko)(Hom., Aphididae) hosts. J. Appl. Entomol. 118, 300–309. doi: 10.1111/j.1439-0418.1994.tb00804.x
Berryman, A. A. (1999). “The theoretical foundations of biological control,“ in Theoretical Approaches to Biological Control,” eds B. A. Hawkins and H. V. Cornell (Cambridge: Cambridge University Press), 3–21.
Bolker, B. M., Gardner, B., Maunder, M., Berg, C. W., Brooks, M., Comita, L., et al. (2013). Strategies for fitting nonlinear ecological models in R, AD Model Builder, and BUGS. Methods Ecol. Evol. 4, 501–512. doi: 10.1111/2041-210X.12044
Fernández-Arhex, V., and Corley, J. C. (2003). The functional response of parasitoids and its implications for biological control. Biocontrol Sci. Technol. 13, 403–413. doi: 10.1080/0958315031000104523
Gerard, P. J. (2000). “Ryegrass endophyte infection affects Argentine stem weevil adult behaviour and susceptibility to parasitism,” in Proceedings of the New Zealand Plant Protection Conference, ed S. M. Zydenbos (Christchurch: New Zealand Plant Protection Society).
Godfray, H. C. J., Hassell, M. P., and Holt, R. D. (1994). The population dynamic consequences of phenological asynchrony between parasitoids and their hosts. J. Anim. Ecol. 63, 1–10. doi: 10.2307/5577
Goldson, S. L., Barron, M. C., Kean, J. M., and van Koten, C. (2011). Argentine stem weevil (Listronotus bonariensis, Coleoptera: Curculionidae) population dynamics in Canterbury, New Zealand dryland pasture. Bull. Entomol. Res. 101, 295–303. doi: 10.1017/S0007485310000507
Goldson, S. L., McNeill, M. R., and Proffitt, J. R. (1993). ”Unexplained mortality amongst parasitized Listronotus bonariensis in the presence of the parasitoid Microctonus hyperodae under caging conditions,” in Proceedings of the 6th Australasian Conference on Grassland Invertebrate Ecology (Hamilton: Ruakura Agricultural Centre).
Goldson, S. L., Proffitt, J. R., Fletcher, L. R., and Baird, D. B. (2000). Multitrophic interaction between the ryegrass Lolium perenne, its endophyte Neotyphodium lolii, the weevil pest Listronotus bonariensis, and its parasitoid Microctonus hyperodae. N. Z. J. Agr. Res. 43, 227–233. doi: 10.1080/00288233.2000.9513423
Goldson, S. L., and Tomasetto, F. (2016). Apparent acquired resistance by a weevil to its parasitoid is influenced by host plant. Front. Plant Sci. 7:1259. doi: 10.3389/fpls.2016.01259
Goldson, S. L., Tomasetto, F., and Popay, A. J. (2014a). Biological control against invasive species in simplified ecosystems: its triumphs and emerging threats. Curr. Opin. Insect Sci. 5, 50–56. doi: 10.1016/j.cois.2014.09.003
Goldson, S. L., Tomasetto, F., and Popay, A. J. (2015). Effect of Epichloë endophyte strains in Lolium spp. cultivars on Argentine stem weevil parasitism by Microctonus hyperodae. N. Z. Plant Prot. 68, 204–211. Available online at: https://www.nzpps.org/journal/68/nzpp_682040.pdf
Goldson, S. L., Wratten, S. D., Ferguson, C. M., Gerard, P. J., Barratt, B. I. P., Hardwick, S., et al. (2014b). If and when successful classical biological control fails. Biol. Control 72, 76–79. doi: 10.1016/j.biocontrol.2014.02.012
Hassell, M., Lawton, J., and Beddington, J. (1977). Sigmoid functional responses by invertebrate predators and parasitoids. J. Anim. Ecol. 46, 249–262. doi: 10.2307/3959
Hassell, M. P. (2000). The Spatial and Temporal Dynamics of Host-Parasitoid Interactions. London: Oxford University Press.
Hawkins, B. A., and Cornell, H. V. (1999). Theoretical Approaches to Biological Control. Cambridge: Cambridge University Press.
Holling, C. S. (1959). Some characteristics of simple types of predation and parasitism. Can. Entomol. 91, 385–398. doi: 10.4039/Ent91385-7
Holling, C. S. (1966). The functional response of invertebrate predators to prey density. Mem. Ent. Soc. Can. 98, 5–86. doi: 10.4039/entm9848fv
Houck, M. A., and Strauss, R. E. (1985). The comparative study of functional responses: experimental design and statistical interpretation. Can. Entomol. 117, 617–629. doi: 10.4039/Ent117617-5
Huffaker, C., Messenger, P., and DeBach, P. (1971). “The natural enemy component in natural control and the theory of biological control,” in Handbook of Biological Control, ed C. Huffaker (New York, NY: Academic Press), 16–67.
Pritchard, D. W., Paterson, R. A., Bovy, H. C., and Barrios-O'Neill, D. (2017). Frair: an R package for fitting and comparing consumer functional responses. Methods Ecol. Evol. 8, 1528–1534. doi: 10.1111/2041-210X.12784
Proffitt, J. R., Ferguson, C. M., McNeill, M. R., Goldson, S. L., Macnab, H., and Barratt, B. I. P. (1993). “A comparison of sampling methods for adult Listronotus bonariensis (Kuschel),” in Proceedings of the 6th Australasian Conference on Grassland Invertebrate Ecology, ed. R.A. Prestidge (Hamilton: Ruakura Agricultural Centre), 67–72.
Quinn, G. P., and Keough, M. J. (2002). Experimental Design and Data Analysis for Biologists. Cambridge University Press.
R Development Core Team (2018). R: A language and Environment for Statistical Computing. Vienna:R Foundation for Statistical Computing (Accessed 10th April 2018).
Ramankutty, N., Mehrabi, Z., Waha, K., Jarvis, L., Kremen, C., Herrero, M., et al. (2018). Trends in global agricultural land use: implications for environmental health and food security. Annu. Rev. Plant. Biol. 69, 789–815. doi: 10.1146/annurev-arplant-042817-040256
Solomon, M. E. (1949). The natural control of animal populations. J. Anim. Ecol. 18, 1–35. doi: 10.2307/1578
Stewart, A. V., Kerr, G., Lissaman, W., and Rowarth, J. (2014). Pasture and Forage Plants for New Zealand. Dunedin:New Zealand Grassland Association.
Tomasetto, F., Cianciullo, S., Reale, M., Attorre, F., Olaniyan, O., and Goldson, S. L. (2018). Breakdown in classical biological control of Argentine stem weevil: a matter of time. BioControl 63, 521–531. doi: 10.1007/s10526-018-9878-4
Tomasetto, F., Goldson, S. L., and Olaniyan, O. (2017a). “Italian ryegrass does it better: effect of Lolium multiflorum on Argentine stem weevil parasitism by Microctonus hyperodae,” in Invertebrate Ecology of Australasian Grasslands. Proceedings of the Ninth ACGIE. ed S. N. Johnson (Hawkesbury, NSW: Western Sydney University).
Tomasetto, F., Olaniyan, O., and Goldson, S. (2017b). Ploidy in Lolium spp. cultivars affects Argentine stem weevil parasitism by Microctonus hyperodae. N. Z. Plant Prot. 70, 326–326. doi: 10.30843/nzpp.2017.70.98
Tomasetto, F., Tylianakis, J. M., Reale, M., Wratten, S. D., and Goldson, S. L. (2017c). Intensified agriculture favors evolved resistance to biological control. Proc. Natl. Acad. Sci. U.S.A. 114, 3885–3890. doi: 10.1073/pnas.1618416114
Keywords: attack rates, host density, invertebrate species, predator-prey interaction, natural enemy, weevil
Citation: Tomasetto F, Casanovas P, Brandt SN and Goldson SL (2018) Biological Control Success of a Pasture Pest: Has Its Parasitoid Lost Its Functional Mojo? Front. Ecol. Evol. 6:215. doi: 10.3389/fevo.2018.00215
Received: 12 July 2018; Accepted: 28 November 2018;
Published: 14 December 2018.
Edited by:
René F. H. Sforza, European Biological Control Laboratory (USDA-ARS), FranceReviewed by:
Andrea Sciarretta, University of Molise, ItalyAdam J. Vanbergen, INRA UMR1347 Agroécologie, France
Copyright © 2018 Tomasetto, Casanovas, Brandt and Goldson. This is an open-access article distributed under the terms of the Creative Commons Attribution License (CC BY). The use, distribution or reproduction in other forums is permitted, provided the original author(s) and the copyright owner(s) are credited and that the original publication in this journal is cited, in accordance with accepted academic practice. No use, distribution or reproduction is permitted which does not comply with these terms.
*Correspondence: Federico Tomasetto, ZmVkZXJpY290b21hc2V0dG9AZ21haWwuY29t