- 1Department of Research and Conservation, Memphis Zoo, Memphis, TN, United States
- 2Department of Wildlife Ecology and Conservation, University of Florida, Gainesville, FL, United States
An animal's physiology should be closely adapted to its environment to optimize organismal function, so physiological traits may be mismatched when animals are introduced to new locations. For introduced amphibians, mismatched traits involving hydric balance or performance across hydric states could have profound impacts on their abundance and distribution, and thus their invasion success. We examined susceptibility to dehydration in invasive Cuban treefrogs (Osteopilus septentrionalis) and two sympatric native treefrogs (Hyla spp.) in Florida, USA, by measuring rates of evaporative water loss, rates of water uptake, and sensitivity of locomotor performance to dehydration. Of the three species, Cuban treefrogs were the most susceptible to dehydration by experiencing the highest rate of evaporative water loss and lowest rate of water uptake. Green treefrogs (Hyla cinerea) were the most resistant to dehydration by losing water the slowest and gaining water the fastest, and squirrel treefrogs (Hyla squirella) were intermediate in rates of water loss and uptake. Dehydrating frogs by 20% of their fully hydrated body masses affected the locomotor performance of all species by decreasing maximum jump lengths, decreasing speed, and increasing successive jump lengths. The maximum jump lengths of Cuban treefrogs and green treefrogs were more sensitive to dehydration than those of squirrel treefrogs. Despite this sensitivity, Cuban treefrogs still outperformed both native species in maximum jump length and speed, even when controlling for body size. Consequently, the performance of dehydrated Cuban treefrogs was similar to that of fully hydrated native treefrogs. Our results suggest that Cuban treefrogs are more susceptible to dehydration and its effects than several native competitors; however, they may be able to at least partly compensate for these effects with their large body size, superior locomotor performance, and strong affinity for refugia. Our findings highlight different strategies used by frogs to maintain water balance and suggest that Cuban treefrog densities may be higher in areas with greater moisture availability and habitat complexity, including urban and densely forested areas. Native treefrogs may experience stronger competition and predation from Cuban treefrogs in these areas, which supports anecdotal reports of native frog declines.
Introduction
As amphibians made the evolutionary transition from aquatic to terrestrial habitats, many species developed morphological, physiological, and behavioral adaptations that reduced the risk of desiccation, including changes in rates of evaporative water loss and water uptake, increased tolerance to dehydration, and water-conserving behaviors (Toledo and Jared, 1993; Jørgensen, 1997). Although many amphibians have little resistance to water loss and must live in or near water and use behavioral strategies to avoid desiccating conditions, such as nocturnal activity and fossoriality (Wells, 2007), some species have specialized adaptations to reduce and tolerate water loss. For example, some species can tolerate losing up to 50% of their body mass to water loss (Thorson, 1955), and others can substantially decrease evaporative water loss by secreting lipids from specialized glands and spreading them over their bodies (Blaylock et al., 1976; Lillywhite et al., 1997; Barbeau and Lillywhite, 2005), or by forming cocoons from sloughed skin (McClanahan et al., 1976; Withers, 1998). The wide variation in tolerance to dehydration among species is broadly related to their ecological habits, with arboreal species exhibiting high resistance to evaporative water loss, terrestrial species showing moderate resistance, and semi-aquatic species showing low resistance (Thorson, 1955; Wygoda, 1984; Young et al., 2005). Similarly, high rates of water uptake have been reported in species inhabiting arid areas (Bentley et al., 1958; Van Berkum et al., 1982; Tingley et al., 2012).
In addition to maintaining water balance, another challenge that amphibians face in terrestrial environments is maintaining ecological performance while dehydrated. The locomotor performance of amphibians is often sensitive to dehydration, with increasing levels of dehydration causing decreased jump lengths, speed, and endurance (Moore and Gatten, 1989; Rogowitz et al., 1999; Titon et al., 2010; Tingley et al., 2012). Because locomotor performance affects the ability to capture prey and escape from predators, decreased performance due to dehydration can compromise predator escape abilities and foraging efficiency (Preest and Pough, 2003), and consequently, can reduce fitness (Wassersug and Sperry, 1977; Walton, 1988; Miles, 2004; Husak, 2006). The hydric sensitivity of locomotor performance differs among species and is often related to local environmental conditions. Amphibians inhabiting dry areas, which presumably face a greater risk of desiccation than amphibians in wet areas, can often maintain a high level of locomotor performance while dehydrated. For example, dehydrated cane toads (Rhinella marina) from an arid area had greater endurance and longer jump lengths than did dehydrated conspecifics from a mesic area (Tingley et al., 2012), and the savanna-dwelling cururu toad (R. schneideri) moved longer distances during timed trials when dehydrated than did two congeners found in rainforest (Titon et al., 2010).
An animal's physiology should be closely adapted to its environment to optimize organismal function, so physiological traits may be mismatched when animals are introduced to new locations. For introduced amphibians, mismatched traits involving hydric balance or ecological performance across hydric states could have profound impacts on their abundance and distribution. Environmental conditions that differ from those experienced in their native range could also facilitate adaptations in physiology or behavior, such as those that occurred in introduced cane toads when they invaded an arid region of Australia (Tingley et al., 2012). The Cuban treefrog (Osteopilus septentrionalis) is native to the Caribbean and has been introduced to Florida, USA, where it negatively impacts native amphibians by competing with them and preying upon them (Meshaka, 2001; Wyatt and Forys, 2004; Smith, 2005; Knight et al., 2009; Rice et al., 2011). Observational data suggest that Cuban treefrogs may be limited by water availability. For example, Cuban treefrogs are more strongly associated with more urban areas than natural areas (largely due to artificial water sources), and they are more abundant in wetlands and hardwood hammocks than in pine forests (Meshaka, 2001; Johnson, 2007). Furthermore, Cuban treefrogs that were displaced to old-field habitat rapidly moved to nearby hardwood hammock or bottomland forest, which also suggests that they prefer more mesic habitats (McGarrity and Johnson, 2010). The habitat of Cuban treefrogs in their native range has not been described in detail, but observations suggest that they are also associated with urban and forested areas in Cuba, the Cayman Islands, and the Bahamas (Meshaka, 2001).
We examined susceptibility to dehydration in Cuban treefrogs and two sympatric native treefrogs (Hyla spp.) in Florida by comparing rates of evaporative water loss, rates of water uptake, and sensitivity of locomotor performance to dehydration. The goal of our study was to investigate innate differences in these properties among species, rather than size-related differences, so we used frogs of similar body sizes (Table 1). Based on apparent preferences for moist microhabitats, as described above, we hypothesized that Cuban treefrogs would be more susceptible to dehydration than the native species by exhibiting higher rates of evaporative water loss, lower rates of water uptake, and locomotor performance that is more compromised by dehydration. Examining these physiological traits will enhance our knowledge of how the Cuban treefrog is affected by water availability, which may influence their habitat use and distribution, and thus their niche overlap with native species.
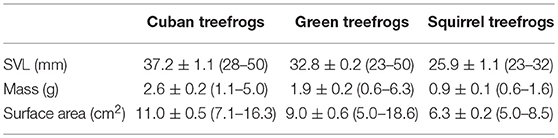
Table 1. Summary of morphometric measurements for frogs used in this study, including the mean ± SE (range) snout-vent length (SVL), body mass, and surface area (adjusted to account for the water-conserving posture; see methods for details) for each species.
Materials and Methods
Ethics Approval Statement
This study was carried out in accordance with the recommendations of the Institutional Animal Care and Use Committees at the Memphis Zoo and University of Florida. The protocol was approved by the Institutional Animal Care and Use Committees at the Memphis Zoo (17-110) and University of Florida (201709995).
Frog Collection and Husbandry
We collected 30 Cuban treefrogs (O. septentrionalis), 30 green treefrogs (Hyla cinerea) and 30 squirrel treefrogs (Hyla squirella) from the University of Florida and four private properties in Gainesville, Florida. Frogs were captured by hand or collected from PVC pipe refugia over a three-day period (19–21 October 2017), and the experiments took place within the following 2 weeks. Immediately upon capture, we recorded each frog's snout-vent length (SVL) and body mass in the field (Table 1). Frogs were housed individually in ventilated plastic containers (12 cm in diameter, 14 cm in height) with moist paper towels and fed crickets twice per week. At the end of the study, the frogs were maintained in the laboratory for future experiments.
Rates of Evaporative Water Loss and Water Uptake
We measured rates of evaporative water loss and water uptake for all frogs. We first determined the masses of fully hydrated frogs by weighing them after they had been submerged in 3 cm of bottled drinking water for 30 min and their bladders had been emptied by gently pressing their abdomens. Frogs were then dehydrated until 20% of their fully hydrated body masses had been lost by placing them in wind tunnels in front of an electric fan (Titon et al., 2010; Tingley et al., 2012). Wind tunnels were constructed from rectangular plastic containers (11 × 7 × 5 cm) with screened ends. While in the wind tunnels, frogs were weighed every 3 min until they lost 20% of their body mass. We observed the posture of each frog every 3 min during the trial and calculated the proportion of time spent in the water-conserving posture (legs folded under the body and ventral surface pressed to the substrate). All measurements and observations were performed carefully to avoid disturbing the frogs. The goal of our study was to compare physiological differences in water loss rates among three species, rather than differences that were related to body size or behavior. Larger frogs lose water slower than smaller frogs due to a smaller surface-area-to-volume ratio, and frogs in the water-conserving posture lose water slower than frogs in active postures because less surface area is exposed to the air. Therefore, to accurately compare differences in resistance to water loss among species, we controlled for the exposed surface area of each frog due to its body size and posture. We calculated rates of evaporative water loss by dividing the slopes of regressions of body masses vs. time by each frog's surface area. Surface area (cm2) was calculated using the following equation based on body mass (McClanahan and Baldwin, 1969): surface area = 9.90 (body mass)0.56. We adjusted surface area values by assuming that the water-conserving posture exposes two-thirds of a frog's total surface area (Withers et al., 1982). We tested for effects of species on rates of evaporative water loss (expressed as mg/cm2/min) using a linear model. We controlled for the time spent in the water-conserving posture by including the residuals from a regression of the proportion of time spent in the water-conserving posture vs. water loss rate as a fixed effect.
After dehydrated frogs were used in locomotor performance trials (as described below), they were immediately rehydrated. To measure rates of water uptake, we placed frogs in plastic containers with 3 cm of bottled drinking water. Every 3 min, frogs were removed from the containers, blotted gently with paper towel to remove excess water, and weighed. These techniques have been used to measure water uptake rates in other studies (Titon et al., 2010; Tingley et al., 2012). Frog body masses were recorded every 3 min until they reached their fully hydrated masses or for a maximum of 30 min. We calculated rates of water uptake by dividing the slopes of regressions of body masses vs. time by each frog's fully hydrated body mass. We used a linear model to test for effects of species on rates of water uptake (expressed as mg/g/min).
We investigated whether rates of evaporative water loss were related to rates of water uptake by using a linear model that controlled for time spent in the water-conserving posture by including the residuals from a regression of the proportion of time spent in the water-conserving posture vs. water loss as a fixed effect. We also tested for potential effects of species and body size (SVL) on water-conserving behavior (proportion of time in the water-conserving posture) during dehydration trials using a linear model. Finally, we examined the natural hydration levels of frogs (calculated by dividing body masses recorded in the field by their fully hydrated body masses determined in the laboratory). We used a linear model to evaluate effects of species and body size (SVL) on natural hydration levels.
Locomotor Performance
We measured the locomotor performance of each frog when it was fully hydrated and dehydrated until 20% of its body mass was lost, as described above. Each frog was tested at each hydric state in a random order, with trials for the same frog occurring at least 48 h apart. Frogs were allowed to jump five times on fabric taped to the floor of a long hallway at 25°C. Most frogs jumped readily, but those that were more reluctant to jump were gently prodded on the urostyle to encourage them to jump. We recorded the time it took each frog to complete five jumps, and we marked the location of each jump using dot stickers. After five jumps were completed, the length of each jump was measured to the nearest 0.5 cm. We used these data to examine the maximum distance jumped, the time to complete five jumps (when controlling for the total distance covered) as a measure of speed, and the change in jumping performance across the five jumps (slope of jump length vs. jump number) as a measure of endurance.
We used two separate linear mixed-effects models to examine whether the maximum jump lengths and the change in jump lengths over time were affected by hydric state, species, SVL, and the interaction between hydric state and species. We used a third linear mixed model to examine whether speed was affected by hydric state, species, SVL, and the interaction between hydric state and species, when controlling for the total distance covered by the five jumps as a fixed effect. For all models, we included individual frog identity as a random effect to account for the fact that each frog was tested at each hydric state and thus the jumping performance data across hydric states were not independent.
All statistical analyses were conducted in R statistical software (version 3.4.3). Linear models were performed in the stats package, linear mixed-effects models were performed in the nlme package, and all probability values were calculated using the car package. We used the visreg package to construct partial residual plots to examine the relationships between variables.
Results
Rates of Evaporative Water Loss and Water Uptake
Rates of evaporative water loss (F = 4.7908, DF = 2, P = 0.0107; Figure 1A) and water uptake (F = 14.1540, DF = 2, P < 0.0001; Figure 1B) differed among species and showed a similar pattern of water balance. Of the three species, the introduced Cuban treefrog was the most susceptible to dehydration by experiencing the highest rate of evaporative water loss and lowest rate of water uptake. The native green treefrog was the most resistant to dehydration by losing water the slowest and gaining water the fastest, and the native squirrel treefrog was intermediate in rates of water loss and gain. Within individual frogs, rates of water loss were negatively related to rates of water uptake (F = 7.3556, DF = 1, P = 0.0081; Figure 1C). Water-conserving behaviors differed among species (F = 4.4239, DF = 2, P = 0.0148, Figure 1D), but not body sizes (F = 0.1715, DF = 1, P = 0.6790). The amount of time spent in the water-conserving posture was lowest in Cuban treefrogs, highest in green treefrogs, and intermediate in squirrel treefrogs. The average natural hydration level of frogs upon immediate collection from the field was 96% (range: 76–100%) and did not differ among species (F = 0.1896, DF = 2, P = 0.8276) or body sizes (F = 1.8616, DF = 1, P = 0.1760).
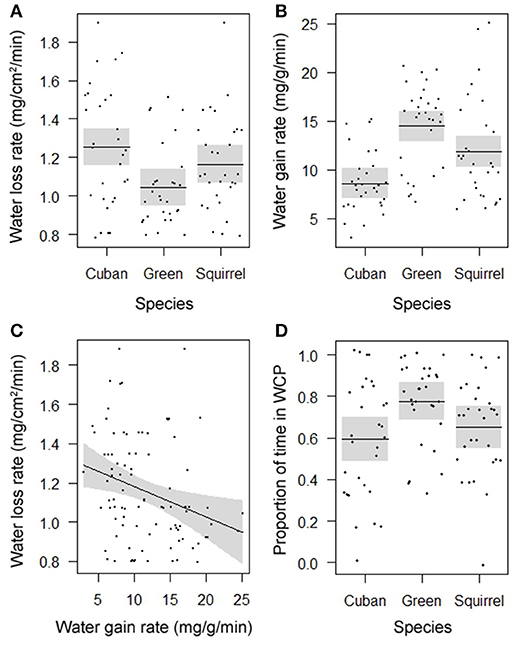
Figure 1. Partial residual plots with lines depicting means (in A,B,D) or regressions (in C) and 95% confidence bands (shaded), controlling for all factors in the statistical models that are not shown in the figures, showing the significantly different rates of (A) evaporative water loss and (B) water uptake for three species, (C) the significant correlation between these rates for individual frogs, and (D) significant differences in the proportion of time spent in the water-conserving posture (WCP) among three species.
Locomotor Performance
The maximum distances jumped by frogs (when controlling for SVL) were affected by hydric state, species, and the interaction between hydric state and species (Table 2, Figure 2A). Fully hydrated frogs jumped farther than dehydrated frogs in all species, but the magnitude of the effects of dehydration on maximum jump lengths differed by species (Figure 2A). Cuban treefrogs and green treefrogs were similarly affected by dehydration, with maximum jump lengths decreasing by an average of 22% (18 cm) and 23% (14 cm), respectively (Figure 2A). Both of these species were more sensitive to dehydration than squirrel treefrogs, which experienced a 12% decrease (7 cm), on average (Figure 2A). Overall, Cuban treefrogs had longer maximum jump lengths in comparison to the native species, even when controlling for body size. When Cuban treefrogs were dehydrated, their maximum jump lengths were similar to those of native frogs when they were fully hydrated (Figure 2A).
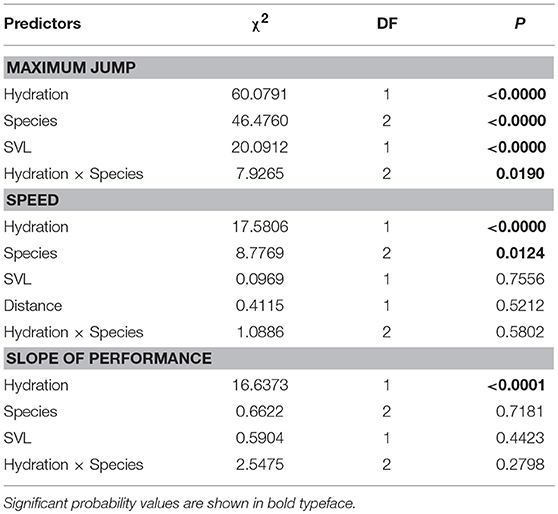
Table 2. Statistical results from three separate linear models examining the effects of different variables on the locomotor performance of frogs, including maximum jump length, speed, and change in jumping performance across five jumps (slope of jump length vs. jump number).
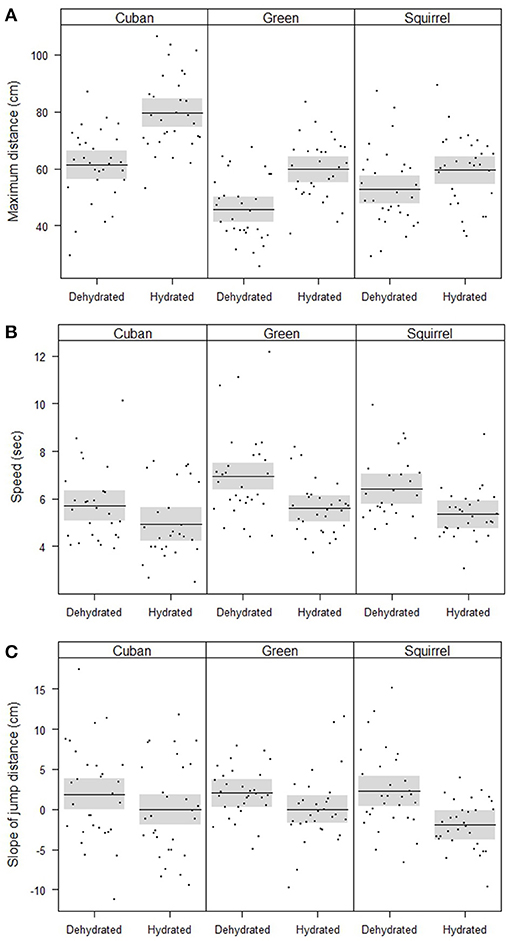
Figure 2. Partial residual plots with means (lines) and 95% confidence bands (shaded), controlling for all factors in the statistical models that are not shown in the figures, showing the locomotor performance of frogs, including (A) the significant interaction between species and hydric state on the maximum jump distance, (B) significant effects of species and hydric state on speed, and (C) the significant effect of hydric state on the change in jump distance across five jumps (slope of jump distance vs. jump number).
Jumping speed was affected by hydric state and species (when controlling for the distance covered and SVL) but there was no evidence for an interaction between these two factors (Table 2, Figure 2B). Dehydrated frogs took 16% (1 sec) longer, on average, to complete five jumps than fully hydrated frogs (Figure 2B). Of the three species, Cuban treefrogs had the fastest speed, green treefrogs had the slowest speed, and squirrel treefrogs had an intermediate speed (Figure 2B). The change in jump lengths across the five jumps (slope of jump length vs. jump number) also differed by hydric state, but not by species or by the interaction between these factors, when controlling for SVL (Table 2, Figure 2C). In all species, the slope for dehydrated frogs was positive, whereas the slope for fully hydrated frogs was zero or negative (Figure 2C); this indicates that dehydrated frogs tended to increase their jump lengths over successive jumps, whereas successive jump lengths of hydrated frogs tended to remain the same or decrease.
Discussion
The ability of amphibians to maintain hydric balance and locomotor performance across hydric states influences their habitat use, geographic distribution, and evolution (Jørgensen, 1997; Wells, 2007). For introduced amphibians, such as the Cuban treefrog in Florida, these physiological traits may affect their invasion success and impacts on native species resulting from competition and predation. To fully understand hydric balance in amphibians, rates of evaporative water loss and water uptake should both be considered, and our study is the first to do this for introduced Cuban treefrogs and two native competitors in Florida. We found that rates of water loss and water uptake differed among species and were negatively correlated (Figure 1). Of the three species, the invasive Cuban treefrog was the most susceptible to dehydration by experiencing the highest rate of evaporative water loss and lowest rate of water uptake. The green treefrog was the most resistant to dehydration by losing water the slowest and gaining water the fastest, and the squirrel treefrog was intermediate in rates of water loss and gain. Our water loss findings match those of a study that showed that green treefrogs had the lowest rate of water loss of five arboreal species, including Cuban treefrogs (Wygoda, 1984). However, results from these two studies contradict another study that found that green treefrogs had the highest rate of water loss of six arboreal species, including squirrel treefrogs (Barbeau and Lillywhite, 2005). Native treefrogs in Florida secrete waterproofing lipids from specialized glands and use wiping movements to spread them over their bodies, which reduces evaporative water loss (Barbeau and Lillywhite, 2005). It is not known whether Cuban treefrogs produce lipids or use wiping behaviors, but they have co-ossification of the cranial skin that is thought to reduce evaporative water loss from the head (Seibert et al., 1974). By occupying small refugia and using their casqued heads to seal the entrances (de Andrade and Abe, 1997; Navas et al., 2002), Cuban treefrogs can reduce water loss through a combination of their physiology and behavior. When frogs were placed in front of a fan during dehydration trials, the amount of time spent in the water-conserving posture was lowest in Cuban treefrogs, highest in green treefrogs, and intermediate in squirrel treefrogs. This suggests that species with a higher physiological tolerance to dehydration may rely more on their physiology to withstand dry conditions, whereas species that are less tolerant of dehydration may be more likely to avoid dry conditions by seeking out more favorable microhabitats.
Because Cuban treefrogs had the highest rate of evaporative water loss and lowest rate of water uptake of the species we examined, they may be less tolerant of dry conditions than other frogs of similar sizes. Species that inhabit arid habitats typically lose water more slowly and absorb water more rapidly than species associated with more mesic habitats (Warburg, 1965; Walker and Whitford, 1970; de Andrade and Abe, 1997; Navas et al., 2004; Young et al., 2005). This might explain why Cuban treefrogs are more abundant in wetlands and hardwood hammocks than in pine forests in Florida, and why they are strongly associated with urban areas in their introduced and native ranges (Meshaka, 2001; Johnson, 2007). Urban areas provide greater water availability than many natural areas due to artificial sources of water, such as ornamental ponds and lawn/garden irrigation. When Cuban treefrogs were displaced to old-field habitat in another study, they rapidly moved to nearby hardwood hammock or bottomland forest, which also suggests that they prefer forested areas over more open areas (McGarrity and Johnson, 2010). In addition to providing more moisture, habitats that Cuban treefrogs prefer also provide more potential refugia than non-preferred habitats. Cuban treefrogs' high affinity for refugia (Meshaka, 2001) enables them to invade urban areas and forested areas with high structural complexity and abundant refugia, such as tree hollows. Among the native frogs we examined, we found that squirrel treefrogs lost water more quickly and absorbed water more slowly than green treefrogs. Squirrel treefrogs have been observed using refugia that were more enclosed than refugia used by green treefrogs (Goin, 1958), and squirrel treefrogs also used PVC pipe refugia with a smaller diameter more often than green treefrogs (Zacharow et al., 2003), which may reflect their higher susceptibility to dehydration.
Dehydration affected the locomotor performance of all species by decreasing the maximum jump length, decreasing speed, and increasing (rather than decreasing) jump lengths over time, which is unlikely to indicate an increase in endurance over time and may instead represent a reluctance to move as the trial began(Figure 2). These changes in the movements of dehydrated frogs can compromise foraging efficiency and predator escape abilities, and consequently, can reduce fitness (Wassersug and Sperry, 1977; Walton, 1988; Miles, 2004; Husak, 2006). Frogs that jump farther and faster should be more likely to escape from common predators, such as snakes and wading birds. Higher-performing frogs should also be more successful at capturing invertebrate prey (Preest and Pough, 2003). Of the three species we examined, the jumping abilities of Cuban treefrogs and green treefrogs were the most sensitive to dehydration, with maximum jump lengths of dehydrated frogs decreasing by nearly twice as much as those of dehydrated squirrel treefrogs (Figure 2). The poorer locomotor abilities of Cuban treefrogs when dehydrated, relative to some native frogs, could contribute to their limited abundance in more open habitats (Meshaka, 2001), in combination with other physiological and behavioral factors.
Overall, we found that Cuban treefrogs were more susceptible to dehydration than similar-sized native treefrogs by losing water more rapidly and absorbing water more slowly. Furthermore, the locomotor performance of Cuban treefrogs was more compromised by dehydration than one of two native species. However, many Cuban treefrogs may be able to compensate for these effects in nature due to their large body sizes and superior jumping abilities. We used frogs of similar sizes in our study to investigate innate differences in water balance and dehydration sensitivity among species, rather than size-related differences. However, adult Cuban treefrogs can grow to be much larger than native treefrogs in Florida (50–100+ mm SVL, depending on sex and locality; Meshaka, 2001; McGarrity and Johnson, 2009), so even though they lost water more rapidly than the native species of the same size, larger frogs should lose water more slowly than smaller frogs due to a smaller surface-area-to-volume ratio. Larger frogs also jumped farther than smaller frogs, but even when controlling for body size, we found that Cuban treefrogs had stronger jumping abilities than the native frogs. Although dehydration had a stronger effect on the jumping performance of Cuban treefrogs than squirrel treefrogs, the maximum jump lengths and speed of dehydrated Cuban treefrogs were similar to those of both native frogs when they were fully hydrated (Figure 2). Therefore, the large body sizes and tremendous jumping performance of Cuban treefrogs may allow them to at least partly compensate for the effects of dehydration. However, any decreases in the jumping performance of dehydrated Cuban treefrogs should reduce their competitive advantage and place them on a more level playing field with native species.
In conclusion, we found that Cuban treefrogs were more susceptible to dehydration than two native competitors and their locomotor abilities were more sensitive to dehydration than one of two native species. The relatively poorer water balance of Cuban treefrogs could, in part, explain their lower abundance in more open habitats (Meshaka, 2001) and could affect their survival and activity during periods of drought. Native frogs may experience stronger competition from Cuban treefrogs in more mesic areas, such as urban areas and densely forested areas, which supports anecdotal reports that native frogs have declined in these areas (Meshaka, 2001; Johnson, 2007; Rice et al., 2011). Eliminating or reducing artificial sources of water (e.g., ornamental ponds and lawn/garden irrigation) and potential refugia (e.g., open pipes, pots, and other debris) may help reduce the abundance of Cuban treefrogs in urban areas, which could potentially reduce competition with native treefrogs. Although the geographic distribution of Cuban treefrogs in Florida appears to be limited primarily by low temperatures at the northern edge of their range (Rödder and Weinsheimer, 2009), water availability may also affect their distribution and abundance on a local scale. Selective pressure in dry habitats or during droughts could facilitate adaptations to improve water balance and maintain locomotor performance over a larger range of hydric states, as occurred in invasive cane toads when they invaded an arid region of Australia (Tingley et al., 2012). Our study demonstrates how a physiological mechanism may reduce the spread of a non-native species and its impacts on native species. Studies such as ours illustrate the role that physiology may play in reducing the threat of invasive species and, thus, in conserving natural resources.
Data Availability
The raw data supporting the conclusions of this manuscript will be made available by the authors upon request.
Author Contributions
ER and SJ designed the study. All authors collected the animals. ER and CR-B conducted the experiments. ER analyzed the data, prepared the figures, and wrote the first draft of the manuscript. All authors edited the manuscript.
Funding
This research was funded by the Memphis Zoo and University of Florida.
Conflict of Interest Statement
The authors declare that the research was conducted in the absence of any commercial or financial relationships that could be construed as a potential conflict of interest.
The handling Editor declared a past collaboration with one of the authors, ER.
Acknowledgments
We thank Sasha Benitez and Mari Ehlers for assistance with the experiments and frog husbandry, and Andrea Lucky, Rick Owen, and Perran Ross for allowing us to collect frogs from their properties. We are grateful to Brett Scheffers for lending us a balance. This study was conducted under permit LSSC-17-00076 issued by the Florida Fish and Wildlife Conservation Commission.
References
Barbeau, T. R., and Lillywhite, H. B. (2005). Body wiping behaviors associated with cutaneous lipids in hylid tree frogs of Florida. J. Exp. Biol. 208, 2147–2156. doi: 10.1242/jeb.01623
Bentley, P. J., Lee, A. K., and Main, A. R. (1958). Comparison of dehydration and hydration of two genera of frogs (Heleioporus and Neobatrachus) that live in areas of varying aridity. J. Exp. Biol. 35, 677–684.
Blaylock, L. A., Ruibal, R., and Platt-Aloia, K. (1976). Skin structure and wiping behavior of phyllomedusine frogs. Copeia 1976, 283–295. doi: 10.2307/1443948
de Andrade, D. V., and Abe, A. S. (1997). Evaporative water loss and oxygen uptake in two casque-headed tree frogs, Aparasphenodon brunoi and Corythomantis greeningi (Anura, Hylidae). Comp. Biochem. Phys. A 118, 685–689.
Goin, O. B. (1958). A comparison of the nonbreeding habits of two treefrogs, Hyla squirella and Hyla cinerea. Q. J. Fla. Acad.Sci. 21, 49–60.
Husak, J. F. (2006). Does survival depend on how fast you can run or how fast you do run? Funct. Ecol. 20, 1080–1086. doi: 10.1111/j.1365-2435.2006.01195.x
Johnson, S. A. (2007). The Cuban Treefrog in Florida. Florida Cooperative Extension Service Publication WEC 218. Gainesville, FL: University of Florida Institute of Food and Agricultural Sciences.
Jørgensen, C. B. (1997). 200 years of amphibian water economy: from Robert Townson to the present. Biol. Rev. 72, 153–237.
Knight, C. M., Parris, M. J., and Gutzke, W. H. N. (2009). Influence of priority effects and pond location on invaded larval amphibian communities. Biol. Invasions 11, 1033–1044. doi: 10.1007/s10530-008-9314-2
Lillywhite, H. B., Mittal, A. K., Garg, T. K., and Agrawal, N. (1997). Wiping behavior and its ecophysiological significance in the Indian tree frog Polypedates maculatus. Copeia 1997, 88–100. doi: 10.2307/1447843
McClanahan, L. Jr., and Baldwin, R. (1969). Rate of water uptake through the integument of the desert toad, Bufo punctatus. Comp. Biochem. Phys. A 28, 381–389. doi: 10.1016/0010-406X(69)91351-6
McClanahan, L. L. Jr., Shoemaker, V. H., and Ruibal, R. (1976). Structure and function of the cocoon of a ceratophryd frog. Copeia 1976, 179–185. doi: 10.2307/1443788
McGarrity, M. E., and Johnson, S. A. (2009). Geographic trend in sexual size dimorphism and body size of Osteopilus septentrionalis (Cuban treefrog): implications for invasion of the southeastern United States. Biol. Invasions 11, 1411–1420. doi: 10.1007/s10530-008-9349-4
McGarrity, M. E., and Johnson, S. A. (2010). A radio telemetry study of invasive Cuban treefrogs. Fla. Sci. 73, 225–235.
Meshaka, W. E. (2001). The Cuban Treefrog in Florida: Life History of a Successful Colonizing Species. Gainesville, FL: University Press of Florida.
Miles, D. (2004). The race goes to the swift: fitness consequences of variation in sprint performance in juvenile lizards. Evol. Ecol. Res. 6, 63–75.
Moore, F. R., and Gatten, R. E. Jr. (1989). Locomotor performance of hydrated, dehydrated, and osmotically stressed anuran amphibians. Herpetologica 45, 101–110.
Navas, C. A., Antoniazzi, M. M., and Jared, C. (2004). A preliminary assessment of anuran physiological and morphological adaptation to the Caatinga, a Brazilian semi-arid environment. Int. Cong. Ser. 1275, 298–305. doi: 10.1016/j.ics.2004.08.061
Navas, C. A., Jared, C., and Antoniazzi, M. M. (2002). Water economy in the casque-headed tree-frog Corythomantis greeningi (Hylidae): role of behaviour, skin, and skull skin co-ossification. J. Zool. 257, 525–532. doi: 10.1017/S0952836902001103
Preest, M. R., and Pough, F. H. (2003). Effects of body temperature and hydric state on organismal performance of toads, Bufo americanus. Physiol. Biochem. Zool. 76, 229–239. doi: 10.1086/367948
Rice, K. G., Waddle, J. H., Miller, M. W., Crockett, M. E., Mazzotto, F. J., and Percival, H. F. (2011). Recovery of native treefrogs after removal of nonindigenous Cuban treefrogs (Osteopilus septentrionalis). Herpetologica 67, 105–117. doi: 10.1655/HERPETOLOGICA-D-10-00020.1
Rödder, D., and Weinsheimer, F. (2009). Will future anthropogenic climate change increase the potential distribution of the alien invasive Cuban treefrog (Anura: Hylidae)? J. Nat. Hist. 43, 1207–1217. doi: 10.1080/00222930902783752
Rogowitz, G. L., Cortés-Rivera, M., and Nieves-Puigdoller, O. (1999). Water loss, cutaneous resistance, and effects of dehydration on locomotion of Eleutherodactylus frogs. J. Comp. Physiol. B 169, 179–186.
Seibert, E. A., Lillywhite, H. B., and Wassersug, R. J. (1974). Cranial coossification in frogs: relationship to rate of evaporative water loss. Physiol. Zool. 47, 261–265.
Smith, K. G. (2005). Effects of nonindigenous tadpoles on native tadpoles in Florida: evidence of competition. Biol. Conserv. 123, 433–441. doi: 10.1016/j.biocon.2005.01.005
Thorson, T. B. (1955). The relationship of water economy to terrestrialism in amphibians. Ecology 36, 100–116. doi: 10.2307/1931435
Tingley, R., Greenlees, M. J., and Shine, R. (2012). Hydric balance and locomotor performance of an anuran (Rhinella marina) invading the Australian arid zone. Oikos 121, 1959–1965. doi: 10.1111/j.1600-0706.2012.20422.x
Titon, B Jr., Navas, C. A., Jim, J., and Gomes, F. B. (2010). Water balance and locomotor performance in three species of neotropical toads that differ in geographical distribution. Comp. Biochem. Phys. A 156, 129–135. doi: 10.1016/j.cbpa.2010.01.009
Toledo, R. C., and Jared, C. (1993). Cutaneous adaptations to water balance in amphibians. Comp. Biochem. Phys. A 105, 593–608.
Van Berkum, F., Pough, F. H., Stewart, M. M., and Brussard, P. F. (1982). Altitudinal and interspecific differences in the rehydration abilities of Puerto Rican frogs (Eleutherodactylus). Physiol. Zool. 55, 130–136.
Walker, R. F., and Whitford, W. G. (1970). Soil water absorption capabilities in selected species of anurans. Herpetologica 26, 411–418.
Walton, M. (1988). Relationships among metabolic, locomotory, and field measures of organismal performance in the Fowler's toad (Bufo woodhousei fowleri). Physiol. Zool. 61, 107–118.
Warburg, M. R. (1965). Studies on the water economy of some Australian frogs. Aust. J. Zool. 13, 317–330.
Wassersug, R. J., and Sperry, D. G. (1977). The relationship of locomotion to differential predation on Pseudacris triseriata (Anura: Hylidae). Ecology 58, 830–839.
Wells, K. D. (2007). The Ecology and Behavior of Amphibians. Chicago, IL: University of Chicago Press.
Withers, P. C. (1998). Evaporative water loss and the role of cocoon formation in Australian frogs. Aust. J. Zool. 46, 405–418.
Withers, P. C., Hillman, S. S., Drewes, R. C., and Sokol, O. M. (1982). Water loss and nitrogen excretion in sharp-nosed reed frogs (Hyperolius nasutus: Anura, Hyperoliidae). J. Exp. Biol. 97, 335–343.
Wyatt, J. L., and Forys, E. A. (2004). Conservation implications of predation by Cuban treefrogs (Osteopilus septentrionalis) on native hylids in Florida. Southeast. Nat. 3, 695–700. doi: 10.1656/1528-7092(2004)003[0695:CIOPBC]2.0.CO;2
Wygoda, M. L. (1984). Low cutaneous evaporative water loss in arboreal frogs. Physiol. Zool. 57, 329–337.
Young, J. E., Christian, K. A., Donnellan, S., Tracy, C. R., and Parry, D. (2005). Comparative analysis of cutaneous evaporative water loss in frogs demonstrates correlation with ecological habits. Physiol. Biochem. Zool. 78, 847–856. doi: 10.1086/432152
Keywords: Cuban treefrog, dehydration, evaporative water loss, Hyla sp., introduced species, jumping performance, Osteopilus septentrionalis, water uptake
Citation: Roznik EA, Rodriguez-Barbosa CA and Johnson SA (2018) Hydric Balance and Locomotor Performance of Native and Invasive Frogs. Front. Ecol. Evol. 6:159. doi: 10.3389/fevo.2018.00159
Received: 24 June 2018; Accepted: 24 September 2018;
Published: 16 October 2018.
Edited by:
Caitlin R. Gabor, Texas State University System, United StatesReviewed by:
Ivan Gomez-Mestre, Consejo Superior de Investigaciones Científicas (CSIC), SpainInger Suzanne Prange, Ohio University, United States
James Watling, John Carroll University, United States
Copyright © 2018 Roznik, Rodriguez-Barbosa and Johnson. This is an open-access article distributed under the terms of the Creative Commons Attribution License (CC BY). The use, distribution or reproduction in other forums is permitted, provided the original author(s) and the copyright owner(s) are credited and that the original publication in this journal is cited, in accordance with accepted academic practice. No use, distribution or reproduction is permitted which does not comply with these terms.
*Correspondence: Elizabeth A. Roznik, YmV0c3kucm96bmlrQGdtYWlsLmNvbQ==