- 1Unidad de Recursos Naturales, Centro de Investigación Científica de Yucatán A. C., Mérida, Mexico
- 2Laboratorio de Genética Ecológica y Evolución, Departamento de Ecología Evolutiva, Instituto de Ecología, Universidad Nacional Autónoma de Mexico, Mexico City, Mexico
A wealth of plant species used by humans for different purposes, but mainly as food, originated and domesticated in the Mesoamerican region. Papaya (Carica papaya) is the third most cultivated tropical crop worldwide, and it has been hypothesized that Mesoamerica is the most likely center of its origin and domestication. In support of it, many wild populations of papaya occur throughout Mesoamerica and hence represent the gene pool of genetic variability for further evolution and future crop management. Despite its importance, a dearth of information exists regarding the status of wild populations of papaya, as compared to the extent of knowledge, and interest, on domesticated varieties. We review the evidence on the extant wild populations of papaya, as well as its origin and distribution. Also, we synthetize what is known on the domestication history of the species, including the domestication syndrome that distinguishes wild and domesticated papayas. Moreover, we make an account of the use of genetic markers to assess genetic diversity of wild and domesticated papaya, and discuss the importance of papaya as the first species with a transgenic cultivar to be released for human consumption, and one that has its complete genome sequenced. Evidence from different disciplines strongly suggest that papaya originated and was domesticated in Mesoamerica, and that wild populations in the region possess, still, high genetic diversity compared to the domesticated papaya. Finally, we outline papaya as an excellent model species for genomic studies that will help gain insight into the domestication process and improvement of papaya and other tropical crops.
Carica Papaya
Papaya (Carica papaya L.) is a fast-growing, short-lived, tropical tree, cultivated for its fruit, papain, pectin, and antibacterial substances (Niklas and Marler, 2007). Nowadays papaya is grown widely in tropical and subtropical lowland regions around the world, and the trade amounted nearly $200,000 million dollars by 2009 (Evans and Ballen, 2012).
Carica papaya is a member of the Caricaceae family and is the most economically important species in the family (Carvalho and Renner, 2012). C. papaya is the only member of the genus after its rehabilitation from the Vasconcella group which was considered part of the genus Carica, until the year 2000 (Badillo, 2000). The Caricaceae family originated in Africa where two extant species occur. The dispersal to Central America from Africa occurred ca. 35 million years ago (MYA), possibly by floating vegetation carried by ocean currents (Carvalho and Renner, 2012). The Caricaceae members arrived to South America from Central America between 27 and 19 MYA, when the Central America land bridge already had begun to form, facilitating the range expansion from Mexico to South America (Carvalho and Renner, 2012). It is estimated that C. papaya diverged from its sister clade about 25 MYA, and belongs to a small clade restricted to Mexico, Guatemala and El Salvador that includes four species: three perennial herbs, Jarilla chocola, J. heterophylla, and J. nana that occur in seasonal tropical forests, and the treelet Horovitzia cnidoscoloides, endemic to Oaxaca in Mexico (Carvalho and Renner, 2014).
Papaya possess a morphological structure and development according to Corner's model of architecture (Hallé et al., 1978): a monopodial, single, orthotropic and nonbranching trunk constructed by one vegetative meristem, with axillary inflorescences, hence with indeterminate growth. Carica papaya produces huge palmate-shaped leaves. Female flowers are produced at the axils of the leaf petiole, and later the fruits will occupy that position along the stem. Male individuals produce inflorescences. Three sex types of papaya are known: female, male, and hermaphrodite trees. In wild populations, most individuals are diclinous (dioecy), whereas cultivated papayas are dioecious or hermaphrodites (Carvalho and Renner, 2012; Chávez-Pesqueira et al., 2014). Cultivars can inbred, resulting in stable characteristics across generations (Manshardt, 1992). For gynodioecious cultivated papaya, it has been reported that two-thirds of the plants correspond to hermaphrodites and one-third to female plants, though dioecious cultivars exist (VanBuren et al., 2015). Sex determination for the three types of papaya, female, male, and hermaphrodite plants, is genetically regulated by the pairing of sex chromosomes (Carvalho and Renner, 2012), through a sex-linked region that behaves like an XY sex chromosome. For male and hermaphrodite individuals, sex is controlled by slightly different Y chromosome regions: Yh in hermaphrodites and Y in males (VanBuren et al., 2015). Female plants produce flowers and fruits all year round in tropical regions; however, in subtropical areas, despite continuous flowering, fruit set is decreased during drier seasons (Gonsalves, 1998).
Papaya is the third most cultivated tropical crop world-wide, Brazil and India are the largest producers of papaya although Mexico is the main exporter (Evans and Ballen, 2012). Under cultivation papaya trees grow fast, producing mature fruits within 9–12 months after planting. Commercially, a density of 1,500–2,500 trees per hectare, can produce from 125,000 to 300,000 lbs per hectare, per year (Gonsalves, 1998). Among common fruits, papaya is ranked first on nutritional scores for the percentage of vitamin A, vitamin C, potassium, folate, niacin, thiamine, riboflavin, iron and calcium, and fiber (Huerta-Ocampo et al., 2012). Moreover, fruits, stems, leaves and roots of papaya are used in a wide range of medical applications and papain production (Ming et al., 2008). Commercial production of papain is directed for protein digestion, mainly as a red meat tenderizer, for the brewing of beer, and the skin treatment of warts and scars (Ming et al., 2012). Because of its rapid growth, continuous harvest and multiple uses, papaya is widely common in home gardens of tropical regions (Manshardt, 1992).
Wild, undomesticated, populations of papaya still grow in many regions of Mesoamerica, but it is common in naturally disturbed tropical forests (Chávez-Pesqueira and Núñez-Farfán, 2016). On occasion, wild papaya is also found in home gardens of ethnic groups in southern Mexico (Paz and Vázquez-Yanes, 1998) where it is used to make jams and dried crystallized fruit candy (Chávez-Pesqueira and Núñez-Farfán, pers. obs). In the rain forests of southern Mexico, wild papaya trees behave like a typical fast-growing, short-lived, nomadic tree (Van Steenis, 1958); they establish rapidly and reach maturity and reproduction only in recent (1–5 years, Paz and Vázquez-Yanes, 1998) and moderately large canopy gaps in mature rain forest, as well as in early secondary forests or “acahuals,” and man-made clearings. Because of this, wild papaya is rare in the rain forest since it depends on the creation of new canopy gaps to persist and disperse (Chávez-Pesqueira and Núñez-Farfán, 2016). In mature forests, papaya plants mainly die due to shading by other trees that “close” the canopy gaps by lateral growth. Because of its nomadic nature, wild papaya represents a key element in the regeneration dynamic of tropical and sub-tropical forests along its natural distribution (Chávez-Pesqueira et al., 2014).
Two main differences in flower morphology and fruit size distinguish wild and domesticated papayas; wild papayas bear either male or female flowers (i.e., dioecious; Chávez-Pesqueira et al., 2014), instead of hermaphrodite flowers, like most cultivated varieties. Wild papayas produce very small fruits (no more than 8 cm in diameter; Chávez-Pesqueira and Núñez-Farfán, pers. obs.), with numerous seeds and a thin mesocarp, almost lacking pulp (Carvalho and Renner, 2012; Figure 1). Carica papaya is mainly pollinated by sphingid moths (Vega-Frutis and Guevara, 2009) and skippers. Seed dispersal is likely carried by birds, bats and small mammals that consume the fruits (Niklas and Marler, 2007; Chávez-Pesqueira and Núñez-Farfán, 2016).
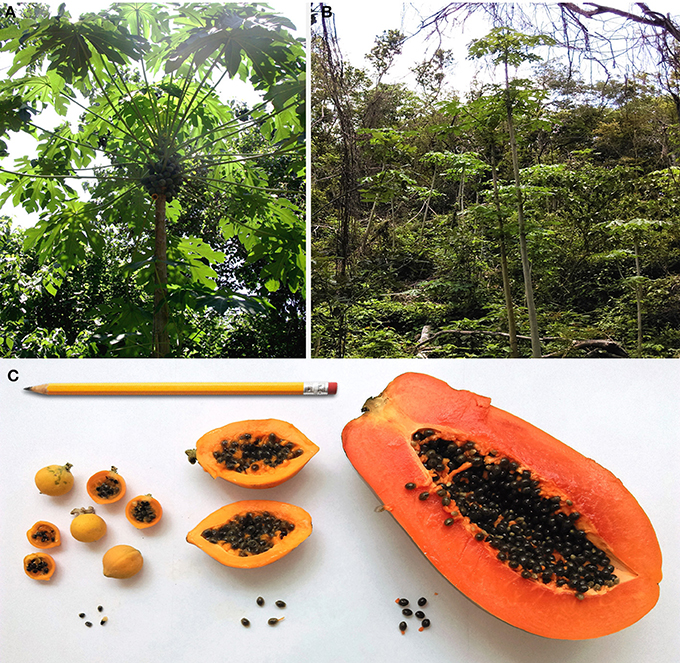
Figure 1. (A) Wild female papaya tree with fruits in Yucatan, Mexico. (B) Wild population of Carica papaya in Yucatan, Mexico. (C) Wild fruits, intermediate form fruits (possible hybrid between wild and domesticated plants), and domesticated papaya fruit (Maradol variety).
In southern Mexico, isolated individuals of papaya, and some in home gardens, show intermediate fruit phenotypes between wild and cultivated plants (Paz and Vázquez-Yanes, 1998). These phenotypes suggest introgression due to occasional mating between population types (Chávez-Pesqueira et al., 2014). Since wild C. papaya represents the genetic reservoir of the species (Chávez-Pesqueira and Núñez-Farfán, 2016), exhaustive efforts should be made to know and preserve this important species in its wild form. Moreover, because papaya is one of the most economically important tropical crops in the world, and wild populations still occur naturally, the species represents an ideal system to study, in depth, the process of domestication. Here, we present a review of the existent knowledge on the origin, distribution and domestication of papaya, and discuss the usage of genetic and genomic methods to study the domestication of this valuable species.
Origin and Distribution
Different authors suggest a Mesoamerican origin of C. papaya, comprising southern Mexico to Central America (Vavilov, 1926; Storey, 1976), although no direct archaeological evidence regarding the center of origin of papaya has been reported as yet (Fuentes and Santamaría, 2014). One reason for the lack of archaeological data is the difficulty to identify papaya from phytoliths, and their pollen grains have been hardly found (Carvalho and Renner, 2012). Together, the presence of undomesticated populations in Mexico and Central America and its cultivation in the region predating the Spanish conquest of Mexico, support the Mesoamerican hypothesis (Carvalho and Renner, 2014; Fuentes and Santamaría, 2014).
Phylogenetic evidence of the Caricaceae family, also supports a Mesoamerican origin of papaya. Carvalho and Renner (2012) obtained a molecular phylogeny of the Caricaceae family using chloroplast and nuclear data (4,711 bp) of the 34 species in the family. This is the only phylogeny using members from all the Caricaceae family. Their resulting phylogeny points that Carica papaya is more closely related to the genus Horovitzia, endemic to Mexico, and Jarilla, endemic to Mexico and Guatemala, than to the South American genus Vasconcellea, as previously thought (Carvalho and Renner, 2014). Moreover, Carica, Jarilla and Horovitzia show an unilocular ovary, whereas the remaining South American Caricaceae possess 5-locular ovaries (Carvalho and Renner, 2012). This morphological synapomorphy supports the family phylogeny.
Further evidence is offered by the origin of the Yh chromosome in cultivated hermaphrodite individuals of papaya (VanBuren et al., 2015). By sequencing the entire male-specific region of the Y chromosome and comparing it with the previous sequences of the hermaphrodite-specific region of the Yh chromosome, (Wang et al., 2012) ,VanBuren et al. (2015) found that the Yh chromosome possess lower nucleotide diversity as compared to the Y. This reduced variability is consistent with a genetic bottleneck scenario possibly brought about by domestication, and suggests that dioecy is ancestral in C. papaya. Given the ubiquity of dioecy in wild populations of papaya in Mesoamerica, an origin for the species in this region is a more parsimonious hypothesis. Yet, genomic studies can aid to determine the origin of some innovations related with domestication.
High values of genetic variation of wild populations, and wild relatives, are expected to be found in the centers of origin of crop species (Gepts and Papa, 2003). Additionally, domestication is expected to reduce genetic diversity and provoke selective sweeps in genes associated to characters target of domestication (Purugganan and Fuller, 2009). A recent phylogeographic analysis of 19 wild populations of papaya in Mexico using nuclear and chloroplast markers, revealed high values of genetic diversity (Chávez-Pesqueira and Núñez-Farfán, 2016). The higher genetic diversity was found in locations of southern Mexico, suggesting this region as a genetic reservoir for the species (see below). Summing up, evidence strongly suggests that C. papaya originated in Mesoamerica, likely in southern Mexico.
Regarding the natural distribution of papaya, this has been suggested to range from the northern tropical limit of Mexico to Costa Rica in Central America (Aradhya et al., 1999; Carvalho and Renner, 2012). However, the precise assessment of its natural distribution is still lacking. One reason for this is the scarcity of studies in wild populations of papaya, and the paucity of herbarium specimens indicating whether a specimen belongs to a cultivated or wild individual. Fuentes and Santamaría (2014) performed searches in herbaria around the world to explore the distribution of papaya. Although they did not distinguish between wild and cultivated plants, their results show that most specimens belong to Mexico and to a lesser extent to Central America Unfortunately, the present rates of deforestation and habitat fragmentation within the proposed distribution range of wild papaya is high enough as to endanger the persistence of plant species (Barlow et al., 2016). This, coupled with the lack of information on the state of many wild varieties of important crop species, warn us about the relevance of studying and conserving wild populations and wild relatives of papaya and other crop species.
Domestication
The limited occurrence of wild populations of C. papaya and its four closest relative species in Mexico and Central America, are in line with the domestication of papaya in the Mesoamerican region (Carvalho and Renner, 2012). Mesoamerica is considered as one of the World's centers of plant domestication (Harlan, 1971; Pohl et al., 1996). The Maya was the most important culture present in that region before the conquest of Mexico by Spain in the sixteenth century, and probably one of the first to cultivate and trade the fruits of C. papaya (Colunga-GarcíaMarín and Zizumbo-Villarreal, 2004). Moreover, it has been suggested that the enzime papain was used by the Mesoamerican people to tenderize meat by wrapping it in papaya leaves and that this knowledge was then taken to Europe after the Spanish colonization (Larqué-Saavedra, 2016). By the time of the conquest of Mexico, it is believed that papaya was cultivated by native people all the way from southern Mexico to the Isthmus of Panama, where it was locally known as olocoton (Storey et al., 1986).
In the sixteenth century the Spaniards were probably the first responsible for the spread of papaya beyond Mesoamerica (Carvalho and Renner, 2014). It was introduced into the Hispaniola island (nowadays Haiti and Santo Domingo) in 1,521. There, it acquired the Carib Indian name ababai, that would then be changed to papaia, papia, papeya, and finally papaya. From this island, it spread to other West Indian islands, such as Jamaica, where it was known as pawpaw; to Cuba where it was named fruta bomba; to Venezuela known as lechosa; and to Brazil and Argentina where it is called mamao and mamón, respectively. In 1526 papaya was taken outside of America to Indonesia, and then spread commercially throughout the East Indies and tropical Asia (Storey et al., 1986). After that, it rapidly spread into other Asian countries and finally to Africa, brought by European colonial powers, such as Portugal, Denmark, Great Britain and France (Manshardt, 2014).
Moreover, because the papaya seeds have a moderate period of longevity, it is likely that this trait aided to its rapid spread throughout the tropics, where it has existed practically since man has recorded modern history (Schroeder, 1958). Nowadays, there are many varieties of papaya cultivated in most tropical and subtropical regions of the world, differing in traits such as fruit size, color, flavour, and tree size (Moore, 2014).
Domesticated plants commonly display a suite of traits, defined as the “domestication syndrome,” that distinguish them from their wild populations or relatives. In general, they differ in traits related to yield, food usage, and cultivation (Martínez-Ainsworth and Tenaillon, 2016). For papaya, domesticated plants have become morphologically and physiologically different in relation to their wild counterparts (Paz and Vázquez-Yanes, 1998). The principal characters of papaya that have been studied as target of selection under domestication are: tree size, fruit size, sex types, and morphology, and germinability of seeds. Whereas tree size has been selected to become smaller to facilitate fruit harvesting (Niklas and Marler, 2007), selection has been focused on enlarging the fruit size and increasing the ovary wall (pulp) for human consumption (Coppens d'Eeckenbrugge et al., 2005; Manshardt, 2014). As a result, several varieties of papaya are cultivated around the world.
Regarding sex types, in dioecious fruit crops, mutations inducing hermaphroditism have been associated with domestication; this is the case of species like strawberry, grape and papaya (Janick, 2006). In the Caricaceae family, dioecy is the ancestral stateand it has been suggested that hermaphrodite individuals in papaya, resulted from a natural mutation in male plants and were likely selected by humans for its favorable fruit phenotype (Ueno et al., 2015). Moreover, the region of the chromosome that produces hermaphrodite papaya plants, which occurs only in cultivated varieties, arose only ~4,000 years ago (higher posterior density of 95%: 1,400–6,700 years ago; VanBuren et al., 2015). Although crop plant domestication in Mesoamerica occurred around 6,200 years ago, the estimation coincides with the rise of the Maya civilization. Given that hermaphrodite individuals are rarely found in wild populations of Mesoamerica, this strongly supports that papaya was domesticated by the Mayans or other Mesoamerican cultures thousands of years ago. It is believed that the rare allele for hermaphroditism in wild populations was probably selected by early domesticators favoring mutants with red flesh color over the typical yellow/orange color of wild papayas (Manshardt, 2014).
Finally, seeds from wild and domesticated papayas differ in size, germination rate, dormancy, and light sensitivity. Paz and Vázquez-Yanes (1998) found that seeds of wild papayas are smaller than those of cultivated plants in southern Mexico, and that they can remain dormant if buried for long periods of time, reflecting their strong light requirement for germination. In cultivated papaya, seeds germinate with a higher probability, lessening the importance of specific environmental conditions to germinate (Paz and Vázquez-Yanes, 1998). This domestication syndrome has been reported for other crops (Doebley et al., 2006; Gepts, 2010).
Genetics and Genomics
Understanding crop domestication is crucial to fulfill the demand for improving yield and quality of crops (Tang et al., 2010). Knowledge of the genetic diversity within crop species is essential to understand their origin, domestication and evolutionary relationships, and to efficiently develop strategies for the conservation of their genetic resources, and effective crop improvement (Moore, 2014). Furthermore, crops represent excellent systems for the study of rapid evolution. For the case of papaya, the species now represents an important model in genetic and genomic studies; papaya is one of the first plant species to have its genome sequenced (Ming et al., 2008) and the first transgenic cultivar released for human consumption (Manshardt, 2014). In this section, we briefly review some advances of genetic and genomic methods in papaya; from what is known about the state of wild populations, to recent advances in domesticated varieties. It must be noted, however, that recent specialized reviews about genomics in papaya have been published elsewhere (Ming et al., 2012; Ming and Moore, 2014; Tripathi et al., 2014).
A recurrent consequence of domestication is the reduction of genetic diversity due to the 2-fold effect of genetic drift and selection that operate during the domestication process (Doebley et al., 2006; Gepts, 2014). Assessing the functional variation of wild genetic resources to expand the usable genetic diversity is of utmost importance for breeding and improvement programs (Martínez-Ainsworth and Tenaillon, 2016). However, little information is available about the genetic diversity of wild varieties of important crop species, with relevance for conservation and management (Chávez-Pesqueira and Núñez-Farfán, 2016).
Early on, the use of genetics in tropical fruit crops was confined to the development of isozyme and dominant PCR-based markers and their use for germplasm diversity analysis and clonal fingerprinting; however, information from dominant markers is of limited use in genomic applications (Litz and Padilla, 2012). Since then, codominant markers, known as simple sequence repeat (SSR) markers or microsatellites, have been developed for several tropical fruit crops and used for parentage analysis, clonal fingerprinting, genetic diversity analysis, and development of genetic linkage maps. Nowadays, the increased capability of DNA sequencing by next generation methods, is leading to increased interest in tropical tree fruit crops and to new opportunities to increase the rate of genetic gain in breeding programs (Litz and Padilla, 2012). Recent approaches in massive sequencing tools, such as de novo genome sequencing, whole genome resequencing, reduction of genome complexity using restriction enzymes, transcriptome analysis, and epigenetic studies are becoming of great importance in domestication studies (Guerra-García and Piñero, 2017).
Genetic knowledge of papaya has been accelerated with the advances in molecular markers, linkage and physical maps, comparative genomics studies, and the sequencing of its genome (Tripathi et al., 2014). For genetic diversity studies, some molecular markers have been developed for papaya, mainly SSRs (Ocampo et al., 2006; Eustice et al., 2008; De Oliveira et al., 2010a; Fang et al., 2016). However, most studies have focused on cultivated papaya, with a dearth of studies addressing the genetic diversity and structure of wild populations.
Our recent surveys about the genetic diversity and structure of wild papaya in its natural distribution in northern Mesoamerica (Mexico) (Chávez-Pesqueira et al., 2014; Chávez-Pesqueira and Núñez-Farfán, 2016), have revealed high levels of genetic diversity for the species in the wild. Using both nuclear SSRs and chloroplast DNA markers in 355 individuals of 19 natural populations, we found a mean observed heterozygosity of 0.681 (range of 0.409–0.783) and a mean haplotidic diversity of 0.701 (range of 0.307–0.934) (Chávez-Pesqueira and Núñez-Farfán, 2016). The area with the higher genetic diversity for both markers occur in southeast Mexico, near the region of the Tehuantepec Isthmus. With the chloroplast DNA, we found a lack of phylogeographic structure but a recent structure with the microsatellite data (RST = 0.149). At the local scale, we also found a negative effect of habitat fragmentation for wild populations of papaya in a fragmented rainforest in Los Tuxtlas rainforest in southern Mexico (Chávez-Pesqueira et al., 2014). Populations that inhabit forest fragments showed a reduced genetic diversity, higher population differentiation, and less migrants. Together, these studies suggest that wild papaya has maintained genetic connectivity among populations throughout time; however, populations are becoming recently structured probably due to human disturbances of its natural habitat, like habitat fragmentation, rendering important conservation concerns for the species in its wild form. Efforts to conserve the natural genetic resources of the species should be addressed as well to assure the conservation of the domesticated varieties through genetic improvement.
Genetic diversity of cultivated and feral papayas has shown to be, in general, lower than wild populations: (1) Using AFLPs markers, a genetic similarity of 0.880 has been reported for 63 cultivated papaya accessions (Kim et al., 2002); (2) an expected heterozygosity (He) ranging from 0.54 to 0.69 in cultivars from the Caribbean region (Ocampo Pérez et al., 2005); (3) a mean He of 0.59 using 51 polymorphic loci from 30 accessions and 18 landraces from different countries, mainly Brazil (De Oliveira et al., 2010b); (4) He ranges of 0.51–0.64 in feral papayas of Costa Rica (Brown et al., 2012); and (5) a mean He of 0.011 in Maradol cultivars in the Pacific coast of Mexico (Chávez-Pesqueira et al., unpublished data). Years of selective breeding would explain the low genetic diversity in the domesticated papayas brought about the domestication bottleneck.
During domestication, gene flow between wild and domesticated conspecific plants plays an important role in addition to other evolutionary forces such as genetic drift and selection. In many cases, crop plants and their wild progenitors belong to the same biological species (Gepts, 2014; Rendón-Anaya et al., 2017), as in papaya, or cohabit with close wild relatives. There is morphological evidence of gene flow between wild and domesticated forms in papaya in southern Mexico (Paz and Vázquez-Yanes, 1998; Chávez-Pesqueira and Núñez-Farfán, 2016; Figure 1C). However, there are no studies evaluating the ecological and evolutionary consequences of this phenomenon. Considering the introduction of transgenic papayas amid the natural distribution of wild populations of C. papaya (see below), would represent a potential ecological risk that demands consideration.
Papaya is known as the world's first transgenic cultivar to have been released for commercial production (in Hawaii, USA), in 1998 by public institutions in the USA (Tecson Mendoza et al., 2008; Manshardt, 2014). This transgenic variety was developed to control the papaya ringspot virus (PRSV). The PRSV acts destroying the photosynthetic capacity of the leaves, leading first to a decrease in fruit quality and yield, loss of vegetative vigor, and eventual death of plants (Lius et al., 1997). After a spread of the virus in 1994 that nearly killed all cultivars in Puna Island in Hawaii, efforts to develop a resistant variety to the PRSV began. Two varieties successfully controlled the virus infection and saved the papaya production in Hawaii: Rainbow and SunUp. According to Gonsalves (2014), transgenic papaya acreage in the year 2012 was about 85% in Hawaii. However, some concern raised regarding the potential effects of gene flow between transgenic and nontransgenic cultivars. Experiments were carried out to assess this; from 2004 through 2009, Gonsalves et al. (2012) studied transgene flow of “Rainbow” (transgenic) to “Kapoho” (nontransgenic) cultivars in Hawaii. Their results showed that observed transgene pollen dispersal to hermaphrodite “Kapoho” from neighboring plants of the “Rainbow” variety was very low, but from “Rainbow” to female “Kapoho” individuals, it was higher (Gonsalves, 2014), confirming the existence of gene flow in some amount among cultivars. Although cultivars of transgenic papaya are confined to Hawaii, little is known about the ecological, evolutionary and commercial consequences of possible transgene flow in the center of origin of papaya where natural populations occur, and where attempts to release transgenic cultivars have prevailed (Silva-Rosales et al., 2010). Nowadays, transgenic papaya from Hawaii is consumed in USA, Canada, and Japan, but no studies have been made to assess the possible movement of seeds to papaya's origin center. Because transgenic papaya saved Hawaii's papaya industry, it has been recognized as a model species of translational biotechnology and the best-characterized commercial transgenic crop. Given that PRSV is widespread in papaya cultivars around the world, papaya SunUp could serve as a transgenic germplasm source to breed suitable virus-resistant cultivars (Ming et al., 2008). However, despite these achievements, many countries have rejected transgenic crops; in 2010, only transgenic papaya and plum had been approved for human consumption (Litz and Padilla, 2012).
With the recent advances of DNA next generation sequencing a great interest has been advocated to crops. Papaya is the fifth flowering plant (after Arabidopsis, rice, poplar, and grape) to have its genome sequenced (Kanchana-Udomkan et al., 2014). A draft genome (approximately 3x coverage) was obtained from a transgenic female cultivated papaya from the SunUp variety from Hawaii, using a whole-genome shotgun approach (Ming et al., 2008). The assembled contigs summed up 73% of the genome (271 Mb) with 370 Mb of spanning scaffolds (Wei and Wing, 2008). Further efforts were made to complete the draft genome of papaya; Yu et al. (2009) constructed a BAC (Bacterial Artificial Chromosome) based physical map to support the sequence assembly with an estimated genome coverage of 95.8%. The papaya genome has shown to be three times the size of the Arabidopsis genome, containing fewer genes, with a minimal angiosperm gene set of 13,311. Amplifications in gene number with roles in the evolution of tree-like habit, deposition and remobilization of starch reserves, attraction of seed dispersal agents, and adaptation to tropical day lengths have been identified (Ming et al., 2008).; Moreover, the small genome and diploid nature (2n = 18) of the species, render papaya as an excellent model for genomics (Ming and Moore, 2014; Tripathi et al., 2014).
Recent genetic and genomic methods have revealed interesting facts about domestication in papaya. For instance, Wu et al. (2017) found evidence of a recent emergence of the y allele and its selection in red fruited cultivation. Analysing the levels and patterns of genetic diversity at the CYC-b locus (y allele) and six loci in a 100-kb region flanking this locus, they found evidence of a strong selective sweep, as genetic diversity showed a reduction at the recessive y allele in comparison with the dominant Y allele present in the yellow-fleshed papayas (wild and cultivated). By means of a haplotype network, the authors suggest that the y allele likely originated in the wild and was introduced into the domesticated varieties during the domestication of papaya. However, they found shared haplotype structure among some wild, feral, and cultivated haplotypes near the y allele, suggesting a successive escape of the y allele from red cultivars back into wild populations, probably through feral intermediates in Costa Rica (Wu et al., 2017). In other study, Porter et al. (2009), using a genome-wide analysis, revealed that papaya has relatively few nucleotide-bindings sites (NBS) encoding genes, but structurally diverse showing a novel subgroup. This group of genes are the most common in plant disease resistance proteins. The authors argue that the absence of recent genome duplication and relatively low gene number in papaya, may explain the apparent scarcity NBS gene number, and that this could function as pathogen surveillance, making papaya suitable for functional studies and a better understanding of plant resistance at the genetic level (Porter et al., 2009).
Furthermore, papaya has been recognized as an excellent model for studies about sex determination in plants (Ming et al., 2012; Aryal and Ming, 2014), sex chromosome evolution (Weingartner and Moore, 2012), origin and evolution of dioecy in the Caricaceae family, and the identification of candidate genes and genome-wide DNA markers for papaya improvement (Ming et al., 2012). These studies have helped to expand the knowledge for tropical fruit tree genomics. Moreover, phylogenetically, papaya belongs to the Brassicales, and can serve as an excellent out-group to study genome evolution in the Brassicaceae family, given that it shares a common ancestor with Arabidopsis, about 72 MYA (Ming et al., 2012). Undoubtedly, the sequencing of the papaya genome has opened avenues to investigate the function of many genes for which little is known. The sequenced genome will facilitate knowledge about the genomic regions associated to diverse aspects of plant defense, plant growth, development of leaves, roots, and fruits, flowering, as well as fruit ripening, and circadian clock (Tripathi et al., 2014).
Regarding its commercial use, a variety of markers have been developed to distinguish between the different sexes in juvenile plants (Somsri et al., 1997; Deputy et al., 2002; Lemos et al., 2002; Urasaki et al., 2002; for a complete review of molecular markers in papaya see Kanchana-Udomkan et al., 2014). Most markers achieved to distinguish between male/hermaphroditic and female individuals. This aided in eliminating females, given that hermaphrodites are preferred for their bigger and elongated fruits, rather than the rounded female fruits that require greater container space for shipping (Tripathi et al., 2014). However, in commercial production, males are useless and with these markers, males couldn't be distinguished. Recently, Liao et al. (2017), used a large male-specific retrotransposon insertion of 8396 bp to develop two papaya male-specific markers. Given that sex determination is only possible around 6 months after germination, these markers largely facilitate the elimination of undesired plants. Finally, sex markers are also useful in ecological and evolutionary studies that require the estimation of effective population sizes which can be obtained with the sex ratio of populations (Chávez-Pesqueira et al., 2014).
In spite of these advances, many questions remain unsolved for papaya, mainly for the wild counterpart. For example, the lack of knowledge about the consequences of gene flow from domesticated and/or transgenic populations. Moreover, few studies have evaluated genetic regions of wild individuals (standing variation) for crop improvement purposes (but see Vázquez Calderón et al., 2014).
The use of genomics in tropical crop breeding will greatly assist in solving many difficult challenges in the face of global warming and climate change (Guerra-García and Piñero, 2017). Compared to annual crops and temperate trees, genetic improvement of tropical fruit trees has been limited (Litz and Padilla, 2012). The use of genomics for the development of new tropical cultivars adapted to higher temperatures and increased droughts will become necessary to alleviate food security in the upcoming years (Abberton et al., 2016). The organization of genetic diversity in wild relatives represent important genetic resources that can facilitate the development of climate-tolerant cultivars (Gepts, 2014).
Finally, the continuous advances of genomic tools in crop species will also serve to gain insights into the ecological and evolutionary knowledge of domestication in tropical crops. For instance, the functional genomics aspects of domestication will help assess how many and which genes show differences in expression between wild and domesticated types (Sarah et al., 2017), which factors affect gene expression, and a better understanding of biotic interactions and how these have affected crop evolution, among many others (Gepts, 2014).
Plant domestication represents one of the most relevant events in human history. Today, papaya is one of the most important economically crop species worldwide. Understanding the ecology, evolutionary history and domestication process of such species is necessary to maintain food security in the future and counteract upcoming threats of overpopulation and climate change.
Final Remarks
The understanding of the origin and domestication of crop species has become a topic of great interest and has advanced importantly through combining approaches from diverse disciplines (Martínez-Ainsworth and Tenaillon, 2016). Papaya represents the third most produced crop in the tropics worldwide and an important source of commercial uses for humans. It was most probably originated and domesticated in Mesoamerica where wild populations still occur and distribute in Mexico and Central America. In nature, wild papayas play an important role in the regeneration of their natural habitat and possess high levels of genetic variation, representing the genetic wealth and evolutionary potential of the species. Finally, papaya is an excellent model for genomic studies as it is one of the first plant species to have its complete genome sequenced. However, there is still much more knowledge needed from this important species, mainly about its wild populations and the evolutionary process of domestication.
Author Contributions
MC-P and JN-F conceived the review and the outline, and searched for literature. MC-P wrote the manuscript. JN-F reviewed drafts of the manuscript and contributed to writing the final version. Both authors were involved in the final editing and review of the paper.
Funding
Funding was provided by Comisión Nacional para el Conocimiento y Uso de la Biodiversidad (CONABIO) and Dirección General del Sector Primario y Recursos Naturales Renovables of Secretaría de Medio Ambiente y Recursos Naturales (SEMARNAT), project WQ003 “Análisis para la determinación de los centros de origen y diversidad genética de Carica papaya (Caricaceae).”
Conflict of Interest Statement
The authors declare that the research was conducted in the absence of any commercial or financial relationships that could be construed as a potential conflict of interest.
The handling Editor declared a shared affiliation, though no other collaboration, with one of the authors JN and states that the process nevertheless met the standards of a fair and objective review.
Acknowledgments
We thank Rosalinda Tapia-López for her help in logistics and lab work in our studies with papaya.
References
Abberton, M., Batley, J., Bentley, A., Bryant, J., Cai, H., Cockram, J., et al. (2016). Global agricultural intensification during climate change: a role for genomics. Plant Biotechnol. J. 14, 1095–1098. doi: 10.1111/pbi.12467
Aradhya, M. K., Manshardt, R. M., Zee, F., and Morden, C. W. (1999). A phylogenetic analysis of the genus Carica L.(Caricaceae) based on restriction fragment length variation in a cpDNA intergenic spacer region. Genet. Resour. Crop Evol. 46, 579–586. doi: 10.1023/A:1008786531609
Aryal, R., and Ming, R. (2014). Sex determination in flowering plants: papaya as a model system. Plant Sci. 217, 56–62. doi: 10.1016/j.plantsci.2013.10.018
Badillo, V. M. (2000). Carica L. vs. Vasconcella St. Hil. (Caricaceae) con la rehabilitación de este último. Ernstia 10, 74–79.
Barlow, J., Lennox, G. D., Ferreira, J., Berenguer, E., Lees, A. C., Mac Nally, R., et al. (2016). Anthropogenic disturbance in tropical forests can double biodiversity loss from deforestation. Nature 535, 144–147. doi: 10.1038/nature18326
Brown, J. E., Bauman, J. M., Lawrie, J. F., Rocha, O. J., and Moore, R. C. (2012). The structure of morphological and genetic diversity in natural populations of Carica papaya (Caricaceae) in Costa Rica. Biotropica 44, 179–188. doi: 10.1111/j.1744-7429.2011.00779.x
Carvalho, F. A., and Renner, S. S. (2012). A dated phylogeny of the papaya family (Caricaceae) reveals the crop's closest relatives and the family's biogeographic history. Mol. Phylogenet. Evol. 65, 46–53. doi: 10.1016/j.ympev.2012.05.019
Carvalho, F. A., and Renner, S. S. (2014). “The phylogeny of the Caricaceae,” in Genetics and Genomics of Papaya, eds R. Ming and P. H. Moore (New York, NY: Springer), 81–94.
Chávez-Pesqueira, M., and Núñez-Farfán, J. (2016). Genetic diversity and structure of wild populations of Carica papaya in Northern Mesoamerica inferred by nuclear microsatellites and chloroplast markers. Ann. Bot. 118, 1293–1306. doi: 10.1093/aob/mcw183
Chávez-Pesqueira, M., Suárez-Montes, P., Castillo, G., and Núñez-Farfán, J. (2014). Habitat fragmentation threatens wild populations of Carica papaya (Caricaceae) in a lowland rainforest. Am. J. Bot. 101, 1092–1101. doi: 10.3732/ajb.1400051
Colunga-GarcíaMarín, P., and Zizumbo-Villarreal, D. (2004). Domestication of plants in Maya lowlands. Econ. Bot. 58, 101–110. doi: 10.1663/0013-0001(2004)58[S101:DOPIML]2.0.CO;2
Coppens d'Eeckenbrugge, G., Restrepo, M. T., Jiménez, D., and Mora, E. (2005). “Morphological and isozyme characterization of common papaya in Costa Rica,” in I International Symposium on Papaya, Vol. 740 (Genting Highlands), 109–120.
De Oliveira, E. J., dos Santos Silva, A., de Carvalho, F. M., Dos Santos, L. F., Costa, J. L., de Oliveira Amorim, V. B., et al. (2010a). Polymorphic microsatellite marker set for Carica papaya L. and its use in molecular-assisted selection. Euphytica 173, 279–287. doi: 10.1007/s10681-010-0150-y
De Oliveira, E. J., Amorim, V. B. O., Matos, E. L. S., Costa, J. L., da Silva Castellen, M., Pádua, J. G., et al. (2010b). Polymorphism of microsatellite markers in papaya (Carica papaya L.). Plant Mol. Biol. Rep. 28, 519–530. doi: 10.1007/s11105-010-0180-6
Deputy, J. C., Ming, R., Ma, H., Liu, Z., Fitch, M. M., Wang, M., et al. (2002). Molecular markers for sex determination in papaya (Carica papaya L.). Theor. Appl. Genet. 106, 107–111. doi: 10.1007/s00122-002-0995-0
Doebley, J. F., Gaut, B. S., and Smith, B. D. (2006). The molecular genetics of crop domestication. Cell 129, 1309–1321. doi: 10.1016/j.cell.2006.12.006
Eustice, M., Yu, Q., Lai, C. W., Hou, S., Thimmapuram, J., Liu, L., et al. (2008). Development and application of microsatellite markers for genomic analysis of papaya. Tree Genet. Genomes 4, 333–341. doi: 10.1007/s11295-007-0112-2
Evans, E. A., and Ballen, F. H. (2012). An overview of Global Papaya Production, Trade, and Consumption. Gainesville, FL: University of Florida. Publication FE913.
Fang, J., Wood, A., Chen, R., and Ming, R. (2016). Molecular basis of off-type microsatellite markers in papaya. Euphytica 209, 323–339. doi: 10.1007/s10681-015-1630-x
Fuentes, G., and Santamaría, J. M. (2014). “Papaya (Carica papaya L.): origin, domestication, and production,” in Genetics and Genomics of Papaya, eds R. Ming and P. H. Moore (New York, NY: Springer), 3–16.
Gepts, P. (2010). Crop domestication as a long-term selection experiment. Plant Breed. Rev. 24, 1–44. doi: 10.1002/9780470650288.ch1
Gepts, P. (2014). The contribution of genetic and genomic approaches to plant domestication studies. Curr. Opin. Plant Biol. 18, 51–59. doi: 10.1016/j.pbi.2014.02.001
Gepts, P., and Papa, R. (2003). Possible effects of (trans) gene flow from crops on the genetic diversity from landraces and wild relatives. Environ. Biosafety Res. 2, 89–103. doi: 10.1051/ebr:2003009
Gonsalves, D. (1998). Control of papaya ringspot virus in papaya: a case study. Ann. Rev. Phytopahol. 36, 415–437. doi: 10.1146/annurev.phyto.36.1.415
Gonsalves, D. (2014). “Hawaii's transgenic papaya story 1978–2012: a personal account,” in Genetics and Genomics of Papaya, eds R. Ming and P. H. Moore (New York, NY: Springer), 115–142.
Gonsalves, D., Gonsalves, C., Carr, J., Tripathi, S., Matsumoto, T., Suzuki, J., et al. (2012). Assaying for pollen drift from transgenic ‘Rainbow’ to nontransgenic ‘Kapoho’ papaya under commercial and experimental field conditions in Hawaii. Trop. Plant Biol. 5, 153–160. doi: 10.1007/s12042-011-9090-5
Guerra-García, A., and Piñero, D. (2017). Current approaches and methods in plant domestication studies. Bot. Sci. 95, 345–362. doi: 10.17129/botsci.1209
Hallé, F., Oldeman, R. A. A., and Tomlinson, P. B. (1978). Tropical Trees and Forests. An Architectural Analysis. Berlin: Springer-Verlag.
Harlan, J. R. (1971). Agricultural origins: centers and noncenters. Science 174, 468–474. doi: 10.1126/science.174.4008.468
Huerta-Ocampo, J. Á., Osuna-Castro, J. A., Lino-López, G. J., Barrera-Pacheco, A., Mendoza-Hernández, G., De León-Rodríguez, A., et al. (2012). Proteomic analysis of differentially accumulated proteins during ripening and in response to 1-MCP in papaya fruit. J. Proteomics 77, 2160–2169. doi: 10.1016/j.jprot.2012.01.015
Janick, J. (2006). “Genetic alteration associated with fruit domestication,” in II International Symposium on Loquat, Vol. 750 (Palermo), 27–36.
Kanchana-Udomkan, C., Ford, R., and Drew, R. (2014). “Molecular markers in papayas,” in Genetics and Genomics of Papaya, eds R. Ming and P. H. Moore (New York, NY: Springer), 355–375.
Kim, M. S., Moore, P. H., Zee, F., Fitch, M. M., Steiger, D. L., Manshardt, R. M., et al. (2002). Genetic diversity of Carica papaya as revealed by AFLP markers. Genome 45, 503–512. doi: 10.1139/g02-012
Larqué-Saavedra, A. (2016). Prehispanic biotechnology in Mesoamerica. Rev. Fitotec. Mex. 39, 107–115.
Lemos, E. G. M., Silva, C. L. S. P., and Zaidan, H. A. (2002). Identification of sex in Carica papaya L. using RAPD markers. Euphytica 127, 179–184. doi: 10.1023/A:1020269727772
Liao, Z., Yu, Q., and Ming, R. (2017). Development of male-specific markers and identification of sex reversal mutants in papaya. Euphytica 213:53. doi: 10.1007/s10681-016-1806-z
Litz, R. E., and Padilla, G. (2012). “Genetic transformation of fruit trees,” in Genomics of Tree Crops, eds R. J. Schnell and P. M. Priyadarshan (New York, NY: Springer), 117–153.
Lius, S., Manshardt, R. M., Fitch, M. M., Slightom, J. L., Sanford, J. C., and Gonsalves, D. (1997). Pathogen-derived resistance provides papaya with effective protection against papaya ringspot virus. Mol. Breed. 3, 161–168. doi: 10.1023/A:1009614508659
Manshardt, R. (1992). “Papaya,” in Biotechnology of perennial Fruit Crops, eds F. A. Hammerschlag and R. E. Litz (Oxford: Cambridge University Press), 489–511.
Manshardt, R. (2014). “History and future of the solo papaya,” in Genetics and Genomics of Papaya, eds R. Ming and P. H. Moore (New York, NY: Springer), 95–113.
Martínez-Ainsworth, N. E., and Tenaillon, M. I. (2016). Superheroes and masterminds of plant domestication. C. R. Biol. 339, 268–273. doi: 10.1016/j.crvi.2016.05.005
Ming, R., Hou, S., Feng, Y., Yu, Q., Dionne-Laporte, A., Saw, J. H., et al. (2008). The draft genome of the transgenic tropical fruit tree papaya (Carica papaya Linnaeus). Nature, 452, 991–996. doi: 10.1038/nature06856
Ming, R., Yu, Q., and Moore, P. H. (2012). “Papaya genome and genomics,” in Genomics of Tree Crops, eds R. J. Schnell and P. M. Priyadarshan (New York, NY: Springer), 241–259.
Moore, P. H. (2014). “Phenotypic and genetic diversity of papaya,” in Genetics and Genomics of Papaya (New York, NY: Springer), 35–45.
Niklas, K. J., and Marler, T. E. (2007). Carica papaya (Caricaceae): a case study into the effects of domestication on plant vegetative growth and reproduction. Am. J. Bot. 94, 999–1002. doi: 10.3732/ajb.94.6.999
Ocampo, J., d'Eeckenbrugge, G. C., Bruyère, S., de Bellaire, L. D. L., and Ollitrault, P. (2006). Organization of morphological and genetic diversity of Caribbean and Venezuelan papaya germplasm. Fruits 61, 25–37. doi: 10.1051/fruits:2006003
Ocampo Pérez, J., Coppens d'Eeckenbrugge, G., Risterucci, A. M., Dambier, D., and Ollitrault, P. (2005). “Papaya genetic diversity assessed with microsatellite markers in germplasm from the Caribbean region,” in I International Symposium on Papaya, Vol. 740 (Genting Highlands), 93–101.
Paz, L., and Vázquez-Yanes, C. (1998). Comparative seed ecophysiology of wild and cultivated Carica papaya trees from a tropical rain forest region in Mexico. Tree Physiol. 18, 277–280. doi: 10.1093/treephys/18.4.277
Pohl, M. D., Pope, K. O., Jones, J. G., Jacob, J. S., Piperno, D. R., deFrance, S. D., et al. (1996). Early agriculture in the Maya lowlands. Lat. Am. Antiq. 7, 355–372. doi: 10.2307/972264
Porter, B. W., Paidi, M., Ming, R., Alam, M., Nishijims, W. T., and Zhu, Y. J. (2009). Genome-wide analysis of Carica papaya reveals a small NBS resistance gene family. Mol. Genet. Genomics 281, 609–626. doi: 10.1007/s00438-009-0434-x
Purugganan, M. D., and Fuller, D. Q. (2009). The nature of selection during plant domestication. Nature 457, 843–848. doi: 10.1038/nature07895
Rendón-Anaya, M., Montero-Vargas, J. M., Saburido-Álvarez, S., Vlasova, A., Capella-Gutierrez, S., Ordaz-Ortiz, J. J., et al. (2017). Genomic history of the origin and domestication of common bean unveils its closest sister species. Genome Biol. 18:60. doi: 10.1186/s13059-017-1190-6
Sarah, G., Homa, F., Pointet, S., Contreras, S., Sabot, F., Nabholz, B., et al. (2017). A large set of 26 new reference transcriptomes dedicated to comparative population genomics in crops and wild relatives. Mol. Ecol. Res. 17, 565–580. doi: 10.1111/1755-0998.12587
Schroeder, C. A. (1958). The origin, spread and improvement of the avocado, sapodilla and papaya. Indian J. Hort 5, 116–128.
Silva-Rosales, L., González-de-León, D., Guzmán-González, S., and Chauvet, M. (2010). Why there is no transgenic papaya in Mexico. Transgenic Plant J. 4, 45–51. Available online at: http://www.globalsciencebooks.info/Online/GSBOnline/images/2010/TPJ_4%28SI1%29/TPJ_4%28SI1%2945-51o.pdf
Somsri, S., Fletcher, R. J., Jobin, M., Drew, R., Lawson, W., and Graham, M. W. (1997). “Developing molecular markers for sex prediction in papaya (Carica papaya L.),” in International Symposium on Biotechnology of Tropical and Subtropical Species Part 2, Vol. 461 (Brisbane), 141–148.
Storey, W. B. (1976). “Papaya,” in Evolution of crop plants, ed N. W. Simmonds (London: Longman), 21–24.
Storey, W. B., Bergh, B., and Zentmyer, G. A. (1986). The origin, indigenous range and dissemination of the avocado. Calif Avocado Soc. Yearb. 70, 127–133.
Tang, H., Sezen, U., and Paterson, A. H. (2010). Domestication and plant genomes. Curr. Opin. Plant Biol. 13, 160–166. doi: 10.1016/j.pbi.2009.10.008
Tecson Mendoza, E. M., Laurena, A. C., and Botella, J. R. (2008). Recent advances in the development of transgenic papaya technology. Biotechnol. Ann. Rev. 14, 423–462. doi: 10.1016/S1387-2656(08)00019-7
Tripathi, S., Castro, L., Fermin, G., and Tennant, P. (2014). “Advances in papaya genomics,” in Omics Technologies and Crop Improvement, ed N. Benkeblia (Boca Raton, FL: CRC Press), 219–252.
Ueno, H., Urasaki, N., Natsume, S., Yoshida, K., Tarora, K., Shudo, A., et al. (2015). Genome sequence comparison reveals a candidate gene involved in male-hermaphrodite differentiation in papaya (Carica papaya) trees. Mol. Genet. Genomics 290, 661–670. doi: 10.1007/s00438-014-0955-9
Urasaki, N., Tokumoto, M., Tarora, K., Ban, Y., Kayano, T., Tanaka, H., et al. (2002). A male and hermaphrodite specific RAPD marker for papaya (Carica papaya L.). Theor. Appl. Genet. 104, 281–285. doi: 10.1007/s001220100693
VanBuren, R., Zeng, F., Chen, C., Zhang, J., Wai, C. M., Han, J., et al. (2015). Origin and domestication of papaya Yh chromosome. Genome Res. 25, 524–533. doi: 10.1101/gr.183905.114
Van Steenis, C. G. G. J. (1958). “Rejuvenation as a factor for judging the status of vegetation types: the biological nomad theory,” in Proceedings of the Symposium on Humid Tropics Vegetation, ed Kandy (Paris: UNESCO), 159–163.
Vavilov, N. I. (1926). The origin of the cultivation of ‘primary’ crops, in particular cultivated hemp. Stud. Origin cultivated Plants 16, 221–233.
Vázquez Calderón, M., Zavala León, M. J., Contreras Martín, F. A., Espadas y Gil, F., Navarrete Yabur, A., Sánchez Teyer, L. F., et al. (2014). New cultivars derived from crosses between commercial cultivar and a wild population of papaya rescued at its center of origin. J. Bot. 2014, 1–10. doi: 10.1155/2014/829354
Vega-Frutis, R., and Guevara, R. (2009). Different arbuscular mycorrhizal interactions in male and female plants of wild Carica papaya L. Plant Soil 322, 165. doi: 10.1007/s11104-009-9903-6
Wang, J., Na, J. K., Yu, Q., Gschwend, A. R., Han, J., Zeng, F., et al. (2012). Sequencing papaya X and Yh chromosomes reveals molecular basis of incipient sex chromosome evolution. Proc. Natl. Acad. Sci. U.S.A. 109, 13710–13715. doi: 10.1073/pnas.1207833109
Wei, F., and Wing, R. A. (2008). A fruitful outcome to the papaya genome project. Genome Biol. 9:227. doi: 10.1186/gb-2008-9-6-227
Weingartner, L. A., and Moore, R. C. (2012). Contrasting patterns of X/Y polymorphism distinguish Carica papaya from other sex chromosome systems. Mol. Biol. Evol. 29, 3909–3920. doi: 10.1093/molbev/mss196
Wu, M., Lewis, J., and Moore, R. C. (2017). A wild origin of the loss-of-function lycopene beta cyclase (CYC-b) allele in cultivated, red-fleshed papaya (Carica papaya). Am. J. Bot. 104, 1–11. doi: 10.3732/ajb.1600301
Keywords: Carica papaya, wild papaya, domestication, center of origin, distribution, genetic diversity, genomics, Mesoamerica
Citation: Chávez-Pesqueira M and Núñez-Farfán J (2017) Domestication and Genetics of Papaya: A Review. Front. Ecol. Evol. 5:155. doi: 10.3389/fevo.2017.00155
Received: 30 June 2017; Accepted: 20 November 2017;
Published: 01 December 2017.
Edited by:
Alejandro Casas, Instituto de Investigaciones en Ecosistemas y Sustentabilidad, Universidad Nacional Autónoma de Mexico, MexicoReviewed by:
Shabir Hussain Wani, Michigan State University, United StatesJoao Paulo Fabi, University of São Paulo, Brazil
Copyright © 2017 Chávez-Pesqueira and Núñez-Farfán. This is an open-access article distributed under the terms of the Creative Commons Attribution License (CC BY). The use, distribution or reproduction in other forums is permitted, provided the original author(s) or licensor are credited and that the original publication in this journal is cited, in accordance with accepted academic practice. No use, distribution or reproduction is permitted which does not comply with these terms.
*Correspondence: Mariana Chávez-Pesqueira, bWFyaWFuYS5jaGF2ZXpAY2ljeS5teA==